- 1Department of Plastic Surgery, The First Hospital of China Medical University, Shenyang, China
- 2Department of Breast Surgery, Shengjing Hospital of China Medical University, Shenyang, China
In the past decade, mesenchymal stem cells (MSCs) tend to exhibit inherent tropism for refractory inflammatory diseases and engineered MSCs have appeared on the market as therapeutic agents. Recently, engineered MSCs target to cell surface molecules on immune cells has been a new strategy to improve MSC applications. In this review, we discuss the roles of multiple receptors (ICAM-1, Gal-9, PD-L1, TIGIT, CD200, and CXCR4) in the process of MSCs' immunosuppressive properties. Furthermore, we discuss the principles and strategies for developing receptor-regulated MSCs and their mechanisms of action and the challenges of using MSCs as immunosuppressive therapies.
Introduction
Stem cells therapy is considered as a new therapeutic approach for treating many diseases. Among them, mesenchymal stem cells (MSCs) are one of the primary stem cells, which have been widely used in clinical practice for more than 10 years, and have been proven to be safe and effective (1, 2). Many studies have shown that the immunomodulatory properties of MSCs were developed by suppressing a wide range of innate and adaptive immune cells, including lymphocytes, natural killer cells (NK cells), macrophages, and dendritic cells (DCs). However, the mechanism of MSCs in the modulation of immune cells is still under debate.
Evidence suggested that the immunosuppressive properties of MSCs were mediated by direct or indirect cell-to-cell communication to educate immune cells and ultimately regulate the disease-specific microenvironment (3, 4). Various surface molecules mediate direct cell-to-cell communication, such as chemokine receptors, co-inhibitory molecules, cytokine receptors, and adhesion molecules (3, 5–7). On the other hand, indirect cell-to-cell communication is achieved by extracellular vesicles secreted by MSCs, which included HGF, TSG-6, TGF-β, PGE2, IDO, IL-10, NO, HO-1, or HLA-G (4, 8–14). It is worth noting that some surface molecules also could be secreted to supernate (15, 16). The mechanism of MSCs-mediated immunomodulation remains controversial, which may be the result of multifactorial pathways. Although soluble paracrine factors are essential for MSCs-mediated immunity, more and more studies have shown that direct cell-to-cell communication has played a vital role in the immunoregulatory ability of MSCs (17). Both cell-to-cell contact dependent and independent pathways are essential to the immunosuppression of MSCs; however, it is challenging to explain the immunosuppression of MSCs mediated by direct cell-to-cell communication, paracrine manner seems to develop its immunosuppression effect all the time. Nevertheless, the direct cell-to-cell communication mediated by surface molecules may be helpful to attain the maximum immunosuppression by MSCs (3, 18, 19). Therefore, we focus on the immunomodulatory properties of MSCs mediated by cell surface molecules. Due to the state of cell surface molecules (membrane proteins and secreted proteins), we tend to call the immunosuppression of MSCs mediated by cellular surface molecules “direct cell-to-cell communication.” We introduce and discuss the latest advances in cellular surface molecules of MSCs and how they interact with immune cells and mediate immunosuppression.
Mesenchymal Stem Cells
MSCs are described as multipotent progenitor cells and were first identified in bone marrow in 1970 (20, 21). Subsequent reports disclosed that MSCs could differentiate into osteoblasts, adipocytes, and chondrocytes when exposed to appropriate conditions (5). Besides, MSCs could be isolated from a variety of mature tissues, including bone marrow, adipose tissues, umbilical cord blood, and placenta (3, 5, 22). Simultaneously, strong regeneration ability, easy extraction, low immunogenicity, and none tumorigenicity make MSCs an ideal source of stem cells for regenerative medicine, transplantation, and treatment of immune-related diseases (23–25). Those advantages interested researchers and grown clinical relevance, a need to establish a non-ambiguous and broadly accepted definition for these cells arose. Thus, in 2006 (21), the minimal criteria for defining MSCs established by the International Society for Cellar Therapy:
(1) Adhere to plastic with fibroblast-like morphology during culture;
(2) Ability to differentiate into osteoblasts, adipocytes, and chondrocytes in vitro;
(3) Expression of specific surface markers such as CD73, CD90, Sca-1, and CD105, and non-expression of CD14, CD11b, CD34, and CD45.
However, subsequent studies have found that MSCs from different tissues might express different specific surface markers. In our previous studies, adipose-derived stem cells (ADSCs) expressed CD10, CD13, and CD49d, and in most cases, bone marrow-derived MSCs or muscle-derived MSCs expressed more CD73, CD90, CD105, and CD44 (5, 26, 27). Thus, the concept of markers used to identify MSCs may be suitable for distinguishing MSCs from different tissue sources. In addition to these specific surface markers, MSCs also expressed other functional surface molecules, such as chemokine receptors, cytokine receptors, adhesion molecules, and co-inhibitory molecules. They performed different functions in different environments (28).
The Immunomodulatory Ability of Stem Cells
The immunomodulatory properties of MSCs distinguish them from other types of stem cells, including hematopoietic cells, embryonic cells, and induced pluripotent stem cells. Furthermore, many preclinical trials have also shown that the use of MSCs has been an effective way to treat refractory inflammatory diseases, such as acute graft-vs.-host disease (GVHD), autoimmune disease, and ulcer (29, 30). Currently, the detailed mechanism of the immunosuppressive properties of MSCs is still unclear. Increasing evidence indicated that MSCs could develop immunosuppressive properties by regulating the phenotypes and activities of innate and adaptive immune cells. In the microenvironment regulated by MSCs, T cells transformed into a tolerant Treg phenotype; macrophages transformed into an immunoregulatory M2-macrophages phenotype; NK cells transformed into non-free functional status, and MSCs could suppress the production of immunoglobulins and increase the production of regulatory B cells (Bregs) (31–34). Cell-to-cell contact dependent and independent ways to develop its' immunosuppression by blocking immune cell differentiation and cell cycle are described as the exact mechanisms (4, 5, 9, 17). When inflammatory mediators stimulated MSCs (e.g., IL-1β, TNF-α, etc.), PGE-2, TSG-6, TGF-β, and HO-1, etc. (4, 8–14) secreted by MSCs into microenvironment could act on immune cells and indirectly exert their immunoregulatory property. In addition to soluble factors, cellular surface molecules are also essential for the immunosuppression of MSCs. We found that direct cell-to-cell communication via Gal-9 and PD-L1 on the surface of ADSCs was more useful to trigger T cell apoptosis than indirect induction (5). Furthermore, cell-to-cell communication is also accompanied by the secretion of anti-inflammation cytokines by MSCs. It seems that direct cell-to-cell communication is a synergic effect that triggers the secretion of soluble factors, and synergistically promotes immune tolerance.
Clinical Application Status of MSCs
From 2010 to 2020, clinical trials registered in the Chinese Clinical Trial Registry (ChiCTR), EU Clinical Trials Registery (EU-CTR), and Americould ClinicalTrials.gov, using the term “mesenchymal stem cells” showed a linear growth trend each year (Figure 1).
Until March 30, 2020, MSCs have been used in a large number of clinical trials worldwide, and the safety of MSCs has been widely verified (29). To our surprise, there were several therapeutic drugs developed on the market based on MSCs (29, 30), but they showed poor results in clinical trials. In our opinion, the poor results are due to the lack of multiple sources and individual differences in MSCs, which in turn leads to repeatability and comparability. Besides, one of the crucial reasons is that the detailed mechanism of action of MSC treatment is still unclear. For example, there are contradictions among direct cell-to-cell communication, MSCs homing, and rapid clearance after intravenous transplantation of MSCs. Those contradictions could be improved by new technologies, such as the immobilization of cell technology (35). Therefore, a detailed study of the mechanism of action of MSCs in the body will be beneficial to the clinical application of MSCs.
In summary, the clinical application of MSCs is still in the exploratory stage. A large number of fundamental researches could reveal the specific mechanism of MSCs treatment under different disease conditions, and then effectively promote the clinical research process using MSCs. Furthermore, the standardization of manufacturing and treatment is also crucial for the clinical application of MSCs. We are optimistic that many stem cell products will meet the criteria for product registration and benefit humankind in the next 5 years.
Cell Surface Receptor-Mediated MSCs Immunosuppression
Although MSCs have been successfully used as seed cells for tissue repair, there is increasing evidence that MSCs have significant immunosuppressive properties against inflammatory diseases, but its molecular mechanism remains unclear. Extracellular vesicles and other soluble factors secreted from MSCs have been considered as the primary critical anti-inflammatory mediators (36–39), as the intensity of MSCs-EVs is close to the intensity of MSCs. However, some reports disclosed that direct cell-to-cell communication mediated by surface molecules also contributed to the immunosuppression of MSCs (4, 40, 41). Zhou et al. found that the direct cell-to-cell communication had more obvious immunosuppression effect on CD4+ and CD8+ T cells compared to indirect cell-to-cell communication, meanwhile, block some surface receptors would reduce the strength of ADSCs-immunosuppression effect, significantly (5, 10). The different perceptions of confused researchers, and we thought the different environments might cause different results. In the inflammatory environment, the surface receptor may be more critical than the paracrine effect of MSCs, because the inflammatory cytokine enhances the surface receptors expression and promote MSCs immigrate to the target site and exert immunosuppression effect in different stage. Those studies attracted our interests and promoted us to identify specific cell surface molecules expressed on ADSCs (42, 43). These surface molecules, including chemokine and chemotactic receptors, adhesion molecule cytokine and cytokine receptors, co-inhibitory molecules, and other surface markers, have been proven to be related to the immunosuppression properties of tumor cells. However, it is still unknown whether those surface molecules mediate the immunosuppression properties of MSCs. Furthermore, the roles of different surface molecules of MSCs in the immunoregulation of immune cells are still unclear.
In the following, we summarized recent studies describing the interactions between MSCs and different cell populations of the immune system to establish a tolerant microenvironment by direct cell-to-cell communication.
Adhesion Molecules
Adhesion molecules mediate the first step in cell-to-cell communication between MSCs and target cells expressed on MSCs (ICAM-1, VCAM-1, and P-selecting), which interact with corresponding receptors presented on target cells (6, 44, 45). As mention above, ICAM-1 is the highest expressed adhesion molecules on MSCs, so we focus on ICAM-1 to explain the role of adhesion molecules in the immunosuppression of MSCs.
ICAM-1
ICAM-1, also known as CD54, is a transmembrane protein that belongs to the immunoglobulins superfamily. ICAM-1 is mainly expressed in endothelium, leukocytes, fibroblast, and tumor cells (46, 47). Inflammatory cytokines such as IFN-γ, TNF-α, and IL-1β (42, 46, 47) could promote the interaction of ICAM-1 with its ligands LFA-1 or Mac-1, and then mediate the migration of leukocyte to local injury tissues.
Recent studies have shown that ICAM-1 has also been expressed on MSCs and functionally affected MSCs characteristics such as immunosuppression capacity (3, 6, 41, 48). ICAM-1 may play a decisive role in the regulation of immunosuppressive properties. It could act as an adhesion molecule to directly educate immune cells in vivo and in vitro (6, 41). It is well-known that MSCs could induce polarization of macrophages toward the M2-Mϕ phenotype through ICAM-1/LFA-1 (3, 48). Besides, MSCs were capable of reprogramming microglia into an “M2-like” phenotype (15). In addition to macrophage, ICAM-1 also mediated T cells' proliferation and functional impairment (15, 49). MSCs with higher ICAM-1 expression could inhibit DCs maturation and T cells immune response and even show promising effects in reducing transplantation rejection (45). These studies indicated that direct cell-to-cell communication through ICAM-1 was essential for MSCs to immunomodulate and controlled various immune cells. Moreover, ICAM-1 not only functions through direct cell-to-cell interaction but also promotes the paracrine effect of MSCs, which in turn works with these secreted cytokines synergistically promotes immune tolerance (6, 41, 50). However, different from stimulating protein phosphorylation in the downstream pathway of immune cells, ICAM-1 is considered to play a purely adhesive role in the immunosuppressive effect of MSCs (51). Once the immune cells attached to the inflammatory cytokine-stimulated MSCs, where a high concentration of immunosuppressive effector molecules could act on the immune cells and lead the immune cells to undergo apoptosis, cell cycle arrest, or phenotype-switch. So the Blockade of ICAM-1 could significantly reverse MSC-mediated immunosuppression in vitro and in vivo, and knockdown INOS or IDO, the immunosuppression effect would eliminate (52, 53), suggesting that adhesion molecules indeed cooperate with the effector molecules.
In conclusion, MSCs with higher ICAM-1 expression may have better immunosuppression ability, which may provide new potential therapeutic applications for some inflammatory diseases, such as asthma, IBD, and diabetic foot. Besides, MSCs with higher ICAM-1 expression could effectively migrate to injury sites and educate more immune cells. The further elucidation of the role of adhesion molecules in immune response will improve our understanding of immunoregulation and help to develop better adhesion molecule targeting therapies.
Co-inhibitory Molecules
Synergistic co-inhibitory molecules play an essential role in the activation and proliferation of immune cells. At present, PD-1, TIGIT, and Gal-9 are key immune molecules to mediate the immune escape process of tumor cells. In recent years, more and more studies have shown that co-inhibitory molecules on MSCs have also been related to the immunoregulatory ability of MSCs. Therefore, we focus on the Gal-9, TIGIT, and PD-1 families and discuss their immunosuppression properties.
Galectins
Galectins are known as a family of the β-galactoside-binding animal lectins expressed on the surface of various cells. Lectin expressed on the cell surface could form lattices and interact with other cells by binding to the corresponding receptor (54). Currently, 11 of the 15 galectins have been identified in human tissues (55). In general, galectins mediate many biological processes, including cell growth regulation, apoptosis, pre-mRNA splicing, intercellular and cell-matrix adhesion, and cell polarity, motility, differentiation, transformation, and signal transduction, as well as the innate/adaptive immunity (56, 57). In the human immune system, galectins play a crucial role in regulating the homeostasis of the microenvironment. Galectin-1 (Gal-1), galectin-3 (Gal-3), and galectin-9 (Gal-9) are the three most exciting representatives of their abilities to mediate immunosuppression. They are perhaps the best understood as an immune checkpoint molecule, and its role in suppressing host immune surveillance is precise, especially Gal-9, as they could induce several kinds of immune cells apoptosis by RAS signaling or calcium-calpain-caspase-1 pathway (58, 59).
Gal-9
Gal-9 is a 36 or 39 kDa tandem-repeat galectin with two non-identical carbohydrate recognition domains connected by a linker of variable (60, 61). In recent years, Gal-9 is gradually considered as a checkpoint molecule and as a marker for identifying the therapeutic potency of MSCs (58, 62). The gal-9-mediated immunosuppressive mechanism in MSCs may promote T cells apoptosis or suppress T cells' activities. Once Gal-9 binds to the ligand TIM-3 on the surface of T cells, the downstream pathways NF-κB and AKT will be activated and promote Th cells apoptosis (63, 64). Therefore, the binding of Gal-9 with proper ligands could improve immune tolerance and could be further used in patients with transplant rejection and autoimmune diseases (16, 65, 66). When ADSCs cocultured with activated PBMC, not only Gal-9 but also the secretion of some inflammatory cytokine, such as IL-1β, IL-1α, TNF-α, and IFN-γ were further increased (5). Besides, the increased expression of Gal-9 was associated with STAT and JNK pathways (5, 60, 67, 68). These results indicated that Gal-9 was an anti-inflammatory mediator and could be induced by the inflammatory environment. Inhibiting Gal-9 expression by retrovirus-based approach or blocking the Gal-9/TIM-3 pathway with inhibitors could effectively decrease the immunoregulatory abilities of MSCs (60, 62, 69), indicating that MSCs could target T cells through the surface expression of Gal-9. Thus, many studies have focused on MSCs-mediated antiproliferative effects on T cells. A study by Fan et al. (67) revealed that umbilical cord-derived mesenchymal stem cells (UCB-MSCs) could functionally inhibit the proliferation of CD4+ T cells, the differentiation of T cells into Th1 and Th17 cells, and the maturation of DC cells, thereby suppressing the antigen-presenting capacities. Besides, Zhou et al. found that MSCs could inhibit the proliferation of CD4+ T cells and CD8+ T cells, and even promote apoptosis of both CD4+ and CD8+ T cells. Furthermore, they also found that MSCs could promote the formation of Treg cells (50). These regulatory effects on T cells may be related to the expression of Gal-9 expressed on MSCs (5, 63, 67). Further animal studies showed similar results from in-vitro studies before, under the PBMC co-coculture with recombinant Gal-9, Th1 cells were inhibited while Th2-derived cytokines are predominant (66, 70, 71). We speculate that different expression levels of TIM-3 expressed in Th1 and Th2 cells may lead to the opposite outcome. Gal-9 expressed by MSCs may play a negative role in T cell activity. The mechanism may promote the apoptosis of Th1, Th2, Th17, and CD8+ T cells, then promote the formation of Treg cells and eventually lead to a tolerant microenvironment, in which cellular immunity is completely dysfunction, and humoral immunity is a partial disability.
In addition to T cells, TIM-3 was identified on the macrophage. It is well-known that MSCs exert their immunomodulatory effects by promoting polarization of pro-inflammatory macrophages (M1-macrophage) into an anti-inflammatory macrophage (M2-macrophage). Interestingly, Gal-9 also plays a vital role in this process. To our surprise, no studies have been conducted to investigate whether Gal-9 expressed by MSCs mediates macrophage reprogramming. In addition to in vitro results, the results of in LPS-induced preeclampsia-like Rats model also indicated that Gal-9 exerted a positive effect on the M2-macrophage polarization (72, 73). Interestingly, once some macrophages reprogrammed into M2-macrophages, the exosomes secreted by these reprogrammed M2-macrophages would synergistically accelerate the reprogramming process of the remaining macrophages into M2 subtype (74). Macrophages are the bridge between the innate and adaptive immune systems. Once macrophages are switched into M2-macrophages, the capacities of the innate and adaptive immune systems will also be affected directly and indirectly. Currently, it is unknown whether Gal-9 on MSCs mediates the polarization of macrophages. Further studies should be conducted to clarify whether Gal-9 on MSCs could mediate M2-macrophage reprogramming. It is worth noting that Gal-9 plays a pivotal role in M2-macrophage polarization, which may reveal the mechanism of immunosuppression of MSCs. When a large number of macrophages are reprogramming into M2-macrophages, the number of antigen-presenting cells (APCs) and macrophages as a clearance function will be insufficient, resulting in the dysfunction of innate and adaptive immune systems. Subsequently, Th and CLT cells will not normally differentiate due to the lack of antigen stimulation. Besides, M2-macrophages will secrete some anti-inflammatory cytokines, such as IL-10, IL-4, and TGF-β, which will turn T and B cells into a regulative phenotype. Therefore, the key mechanism of immunosuppression of MSCs in the inflammatory microenvironment is the Gal-9-mediated macrophage reprogramming (71).
Like Gal-1 and Gal-3, Gal-9 is also secreted into the supernatant (16, 75–77). Although the amount of Gal-9 in the supernatant was negatively associated with the strength of GVHD, TIM-3+ T cells do not correlate with transplantation rejection (16). Therefore, the formation of Treg cells may not be caused by Gal-9 on MSCs, but the polarized macrophages regulated by MSCs most likely cause it.
Gal-1 and Gal-3
Unlike Gal-9, the immunomodulatory properties of Gal-1 on MSC are still under debate. Although Gal-1 on the surface of MSCs could trigger apoptosis of activated T cells in vitro, there is still a lack of in vivo studies to show that Gal-1 deficiency on MSCs could downregulate the immunosuppressive capacities of MSCs (78, 79). Gal-1 may not be the essential part of directly mediating the immunosuppressive properties of MSCs. However, it may still be involved in regulating the other functions of MSCs, such as motility and differentiation. A study by Yun et al. revealed that Gal-1 could promote MSCs' motility by modulating NF-κB and Smad2/3 pathways (80). Other studies have also shown that Gal-1 on the surface of MSCs could promote angiogenesis and tumor progression (81–83). The biological activities of Gal-1 could promote not only repair and regeneration but also tumor metastasis in the tumor microenvironment (84, 85). Besides, Gal-3 has been reported to have similar functions (86–88). However, compared to Gal-1, Gal-3 is more similar to Gal-9 in immunosuppression properties. A study by Liu et al. showed that Gal-3 knockdown significantly abolished the inhibitory effect of MSCs on activated peripheral blood mononuclear cells (PBMCs) (77). Moreover, the Gal-3 secreted by UCB-MSCs showed a positive effect on the infarcted myocardium (89). Unlike Gal-9, the expression of Gal-1 and Gal-3 in equine bone marrow-derived stem cells (BM-MSCs) is impaired under inflammatory conditions (90). Galectins play different roles in the systematic immune system and local microenvironment. Galectins, especially Gal-1 and Gal-3 instead of Gal-9, have shown strong immunomodulatory effects in vitro, but opposite results in vivo. Future clinical studies should focus on explaining why galectins have such different effects in vivo and in vitro and the development of MSCs in the treatment of inflammatory diseases.
Programmed Death-Ligand 1 (PD-L1)
The binding of co-stimulatory molecules such as the ligand of the B7 family CTLA-4/B7-1 to receptors of the CD28 family such as CD28/CD86 is vital for controlling inflammation. Programmed cell death protein 1 (PD-1), also known as CD279, belongs to the CD28/B7 superfamily. PD-1 is mainly expressed on T cells, NK cells, macrophages, DCs, and B cells. PD-L1, also known as B7-H1 (CD274), and PD-L2, also known as B7-DC or CD273, are both ligands of PD-1. PD-L1 is expressed both on non-hematopoietic stem cells and specific subpopulations of hematopoietic stem cells (91–93). In contrast, PD-L2 is expressed primarily inactivated antigen-presenting cells (APCs), such as DCs and macrophages. Still, it could be induced on the surface or cytoplasm of tumor cells and other immunoregulatory cells such as MSCs (31, 94). PD-1 could not only bind to PD-L1 and PD-L2 but also bind to CD80 and RGMb (94). Similar to the CTLA-4/B7 model, PD-L1 generally has a higher expression level than PD-L2 (95). The binding affinity of PD-L1 for PD-1 and PD-L2 for PD-1 are similar (Kd values; 10 nM), but it is important to note that PD-L1 but not PD-L2 had a delayed interaction reminiscent. The striking differences were observed at the level of the association and dissociation characteristics, and the expression level of PD-L1 is significantly higher than PD-L2 on a wide range of human and mouse cells, so PD-L1 is the main negative immune-checkpoint in the programmed cells death protein family (94–96).
Targeting the PD-1/PD-L1 pathway plays a vital role in T cells' homeostasis, and molecular therapeutic drugs such as Nivolumab and Pembrolizumab have been widely used clinically to target PD-1 and PD-L1 and showed promising effects in PD-1+ or PD-L1+ carcinoma (97–99). In the tumor microenvironment, once PD-1/PD-L1 interacts, T cells would receive negative signals transmitted from PD-L1 expressed on the surface of tumor cells, thereby promote the development of Treg cells and eventually develop the characteristics of tumor immune escape (100).
When PD-L1 was found to be highly expressed on MSCs (101), the role of PD-L1 attracted much attention. Studies have also found that PD-1 expressed on MSCs is essential for maintaining MSCs stem cell properties (102). Furthermore, it is interesting that PD-L1 and PD-L2 were found to be secreted into the supernatant by some type of MSCs, such as human BM-MSCs and Tonsil-derived MSCs (5, 91). Many studies that focused on the immunosuppressive mechanism of MSCs mediated by the PD-1/PD-L1 pathway have obtained similar results to the conclusion in the field of tumors.
Targeting PD-1/PD-L1 is of great significance for the immunosuppressive properties of MSCs on T cells. When the PD-L1 signaling on MSC was blocked, the immunosuppressive capacities of MSCs were significantly eliminated (5, 103). A series of studies on the PD-1/PD-L1 interaction between MSCs and T cells showed that MSCs had different regulatory effects on different phenotypes of T cells. The secretion of inflammatory cytokine IL-17 by Th17 is suppressed by MSCs-mediated PD-1/PD-L1 interaction (40, 104). Interestingly, there was also found that MSCs inhibited the differentiation of T cells into Th17 cells but did not affect the production of IL-17 by mature Th17 cells (40). Although the mechanism is still unknown, we hypothesize that the mature Th17 will reduce PD-L1 expression. Both Th1 and Th2 cells are essential to the immune system; Th1 cells are involved in cellular immunity, while Th2 cells are responsible for humoral immunity. MSCs could inhibit the Th1 cells while promoting Th2 cell polarization (105). However, both Th1 and Th2 cells seemed to be induced toward the apoptotic pathways in different degrees (6). After MSCs educated t cells, the quality and quantity of Treg cells increased significantly, which may be caused by direct cell-to-cell communication between T cells with MSCs or the secretion of IL-10 and TGF-β by MSCs (31, 106–108). Some studies have shown that the PD-1/PD-L1 interaction only promoted T cells apoptosis but was not related to the elevated levels of immunosuppressive cytokines, such as TGF-β and IL-10. In other words, PD-1/PD-L1 interaction does not promote the formation of Treg cells. Different research results require more experimental confirmation. PD-L1 expressed on MSCs is not stably expressed. It has been shown that inflammatory cytokines such as IFN-γ and TNF-α were associated with the elevated level of PD-L1 on MSCs and then affected the immunosuppressive properties of MSCs (91). Besides, other soluble factors such as granulocyte-macrophage colony-stimulating factor (GM-CSF) and polyinosinic-polycytidylic acid could also increase the PD-L1 expression (100, 104). In conclusion, in an inflammatory microenvironment, pro-inflammatory factors could increase the expression of PD-L1 on the surface of MSCs, eventually, lead irreversible hypoergia and cell death, and promote the formation of Treg cells with more immunosuppressive capacities (91, 109, 110). However, knocking-down the expression of PD-L1 on MSCs or blocking the PD-1/PD-L1 signaling pathway will result in the loss of the immunosuppressive function of T cells (5, 111).
In addition to T cells, the PD-1/PD-L1-mediated signaling pathway is also involved in the regulation of other types of immune cells. Studies have shown that MSCs could inhibit the activation, proliferation, and immune function of microglial cells (111) and suppress the proliferation, differentiation, migration, and immunoglobulin-secreting properties of B cells (34).
As mentioned above, the PD-1/PD-L1 interaction is essential for controlling T cells homeostasis between MSCs and T cells. Still, few results have focused on other immune cells, especially APCs (e.g., DCs) and macrophages. It is well-known that MSCs regulate DCs differentiation and macrophages polarization, and the PD-1/PD-L1 signaling pathway mediates the immunosuppress capability of APCs. Due to the crucial role of APCs in the innate and adaptive systems and in regulating immune cell differentiation, the role of APCs in inflammatory diseases is more important than adaptive immune cells. More attention should be paid to the immunosuppressive capacities of APCs mediated by PD-1/PD-L1 in the future.
T-Cell Immunoglobulin and ITIM Domain (TIGIT)
TIGIT, also known as Vstm3, VSig9, or WUCAM, is a newly identified co-inhibitory receptor by Yu et al. (112). TIGIT was found to be expressed in a variety of immune cells, including NK cells, memory T cells, M2-macrophages (M2), Treg cells, tumor-infiltrating lymphocytes (TILs), and individual immunoregulatory cells (e.g., tumor cells and MSCs) (113–119). In the tumor microenvironment, the highly expressed TIGIT in TILs may cause local immune tolerance and promote cancer metastasis and progression by modulating the activation of NK cells, DCs, and T cells.
TIGIT is part of a ligand/receptor network, and the structure of the network is complicated. In humans, two TIGIT interact with two CD155 to form a unique tetramer structure, known as the “lock-and-key interaction” by Stengel et al. (120). Herein, TIGIT could be replaced by CD226, and CD112 could replace CD155. Besides, CD96 or CD112R may also compete with those ligands (121). In the “lock-key” structure, TIGIT could compete with CD226, a co-stimulatory molecule of this network, to bind to high-affinity receptors such as CD155 and PVR, or weakly interact with CD112 (120, 122) (Figure 2), or even block the interaction between CD226 and CD155 in a dose-dependent manner (123–125). Because TIGIT could not only bind to PVR with about 100-fold higher affinity than CD226 but also disrupt the homodimerization of CD226, thus eventually block the interaction of CD226 and its ligands (112, 126). Furthermore, both PVR and CD112 have bidirectional roles in immune activation and inhibition, which is similar to the CD28/CTLA-4-CD80/CD86 family.
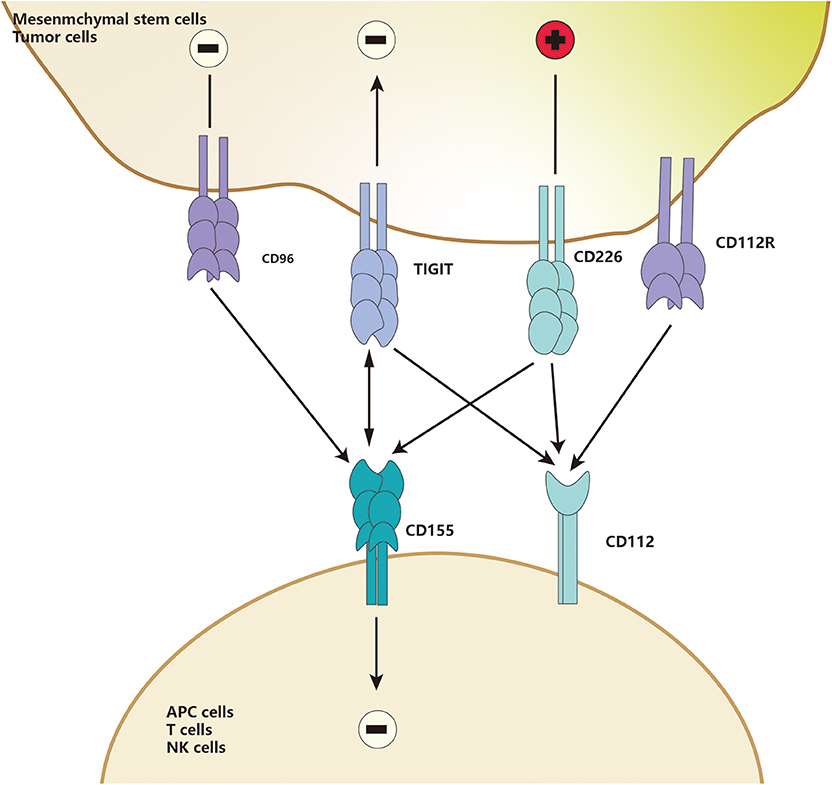
Figure 2. TIGIT and CD226 are detected in MSCs. CD155 and CD112 are detected in APCs. Upon binding, induce co-costimulatory or co-inhibitory signals for CD226 and TIGIT, respectively, CD112 could also deliver an inhibitory signal when engaged by TIGIT.
As an essential immune checkpoint, TIGIT is generally considered as a receptor to mediate intracellular signaling transduction. It was reported that the ligand CD155, also called PVR, had an ITIM motif at the cytoplasmic tail, which could be phosphorylated and trigger activation of downstream wnt/β-catenin pathway (112, 127). TIGIT/PVR seems to be a bidirectional pathway, which is widely involved in immunosuppressive responses of different immune cells, such as monocytes, macrophages, DCs, T cells, and NK cells (112, 124, 128). CD155 was found to be mainly expressed on APCs (112, 119, 129, 130). When macrophages are exposed to LPS or specific pro-inflammatory cytokines, the expression of PVR on macrophages increases significantly. When TIGIT-FC binds to PVR, macrophages polarize to be tolerant M2-macrophages to increase the anti-inflammatory cytokines such as IL-10 and TGF-β and decrease the pro-inflammatory cytokines such as IL-1β and TNF-α (129). A study by Noguchi et al. (119) showed that overexpression of TIGIT in porcine aortic endothelial cells (PAOECs) suppressed M1-macrophage polarization. Interestingly, TIGIT was found to be expressed only on M2-macrophages but not M1 macrophage, suggesting that TIGIT expression promotes M2-polarization (119). Surface TIGIT may likely bind to PVR on other cells and eventually affect other immune cells, such as T cells and NK cells. Meanwhile, the complex inhibitory effects of TIGIT on T cells has attracted widespread attention. TIGIT directly suppresses T cells proliferation and switches them into a tolerant phenotype, called regulatory T cells. These regulatory T cells are then activated to inhibit the secretion of pro-inflammatory cytokine and increase secretion of anti-inflammatory cytokines, eventually blocking the co-stimulatory signal of CD226 through the mechanism (Figure 2) mentioned above. The immunosuppressive properties of TIGIT are close to the TIGIT-educated APCs phenotypes, such as macrophages and DCs (112, 123, 124, 130).
In last year, we found that TIGIT could also be expressed on ADSCs. Although TIGIT has recently become a new immune checkpoint for cancer immunity (113, 121, 131), few studies have reported on TIGIT expressed by MSCs. CD226 and its ligand CD155 have been reported to be associated with impaired NK cells and T Cells function, while MSCs have the same functions. Moreover, MSCs also express CD226 and TIGIT. So does TIGIT mediate the immunosuppression of MSCs on NK and T cells? More experiments are needed. In some special conditions, such as platelet lysate, the decreased expression of PVR and Nectin-2 in MSCs will weaken the inhibitory capacities of MSCs to T cells and NK cells, decrease PGE2 secretion, and increase IL-6 and IL-8 secretion (33, 132). Besides, NK cells showed a significant decrease in DNAM-1 expression when co-cultured with BM-MSC or hADSCs (133). It is unclear whether TIGIT mediates the immunosuppressive effects of MSCs.
Chemokine Receptor
CXCR4 (CD184)
CXCR4, also known as CD184, is one of the most crucial chemokine receptors and the main factor influencing MSCs homing, in which these were circulating MSCs migrated to the target tissues and interacted with the ligand stromal cell-derived factor-1 (SDF-1) (134). Under the interaction with SDF-1, CXCR4+ cells will migrate to the target site according to the gradient of SDF-1.
It is well-known that the up-regulation of CXCR4 may enhance MSCs' migration. Under the induction of SDF-1, CXCR4+ MSCs could migrate to the target site for repair. Similarly, the expression level of CXCR4 on MSCs is related to the local microenvironment. SDF-1 pretreatment could promote the expression of CXCR4 in MSCs (135). Hypoxia conditions or other oxidative stress conditions have the same effect as SDF-1 pretreatment (136–138). Since the inflammatory response promotes the secretion of SDF-1, MSCs may express more CXCR4 when the human body is in an inflammatory state. Besides, MSCs with higher CXCR4 expression were considered to have more robust tissue-regeneration capacities, and this could be proved by transfecting CXCR4 into MSCs or using other methods further to increase CXCR4 expression levels (139, 140). Furthermore, CXCR4 transfection did not affect the biological characteristics and vitality of BM-MSCs (141) but enhanced the targeted recruitment and survival of transplanted MSCs (142). Is the establishment of MSCs overexpressing CXCR4 a great way to improve clinical stem cell transplantation, especially autologous fat transplantation? Focusing on the immunoregulation of MSCs, CXCR4 also plays a vital role in inflammatory diseases. Nan et al. revealed that CXCR4 overexpression might reduce colon inflammation in rats while co-transfecting with IL-35 (143). On the one hand, MSCs with high-level CXCR4 have more robust immunomodulatory capacities by reducing the number of DCs and Th17 cells and increasing the number of M2-macrophages and Treg cells (143). On the other hand, CXCR4 deficiency suppresses the immunomodulatory and homing abilities of MSCs (144). These lead to the downregulation of inflammatory cytokines IL-6 and TNF-α and up-regulation of anti-inflammatory IL-10 (140, 143).
Although CXCR4 has been proved to play a crucial role in the regenerative capacities of MSCs, it is unclear how CXCR4 educates the immune cells phenotype switch (145). Which role does directly with immune cells play in this process?
CD200
CD200, a member of the transmembrane surface immunoglobulin superfamily, expressed on various types of cells, such as T cells, B cells, vascular endothelial cells, and APCs as well as MSCs (28, 101, 146, 147). Besides, the ligand CD200R is expressed on myeloid cells, particularly monocytes and macrophages.
Interestingly, the level of CD200 on MSCs may increase due to the inflammatory environment or hypoxic conditions (148, 149). Bruno Delorme discovered that CD200 could be expressed on cultured and expanded BM-MSCs, and realized that CD200 expression might be related to the immunoregulatory properties of MSCs (150). Recent studies have further shown that CD200 has been associated with reducing infiltration of inflammatory cells, expansion frequency of myeloid progenitor cells, and improving inflammation (149, 151). Another mechanism may be related to promoting the release of anti-inflammatory factors, such as TGF-β and IL-4. Thus, inhibiting CD200 expression by shRNA or by antagonist-mediated neutralization is effectively to suppress the immunoregulatory properties of MSCs (152, 153). However, immunosuppression properties depend on the CD200/CD200R pathway and are also related to macrophages. Once educated by CD200+ MSCs, macrophages would lose their pro-inflammatory properties and begin to polarize into tolerant M2-macrophages (4, 154). Whether CD200/CD200R mediates other immunosuppressive effects on other immune cells is still unknown.
In general, the CD200/CD200R pathway not only promotes the osteogenesis and immunosuppressive properties of MSCs and the secretion of anti-inflammatory cytokines by MSCs but also educates the pro-inflammatory immune cells to polarize into an anti-inflammatory phenotype.
Perspective
To date, these refractory inflammatory diseases have plagued researchers for many years. MSCs are one of the most crucial seed cells in regenerative medicine and have strong immunoregulatory abilities. So far, there are two main pathways to achieve immunosuppressive properties of MSCs, namely paracrine soluble factors, and receptor-mediated direct cell-to-cell communication. Soluble factors include HGF, TGF-β, PGE2, IDO, IL-10, NO, HO-1, HLA-G and exosomes, and exosomes are considered to be the foremost effective means to achieve immunosuppressive properties of MSCs. However, we believe that direct cell-to-cell communication is essential for the immunosuppressive properties of MSCs. In preclinical studies, the successful promotion of MSCs-mediated immune cells phenotype switch has excited all investigators in the field. Therefore, the use of MSCs mediated by multiple cell surface receptors has the potential to overcome certain refractory immune diseases, such as GVHD, diabetic ulcer, and autoimmune diseases. Still, most researches remain in the fundamental stage, and there are a few animal experiments (Table 1). However, the application of MSCs in clinical treatment must be careful; the safety and effectiveness of these MSCs in clinical treatment must be ensured. Before the clinical usage of MSCs, it is necessary to understand the underlying mechanisms of action and the immunosuppressive properties of MSCs.
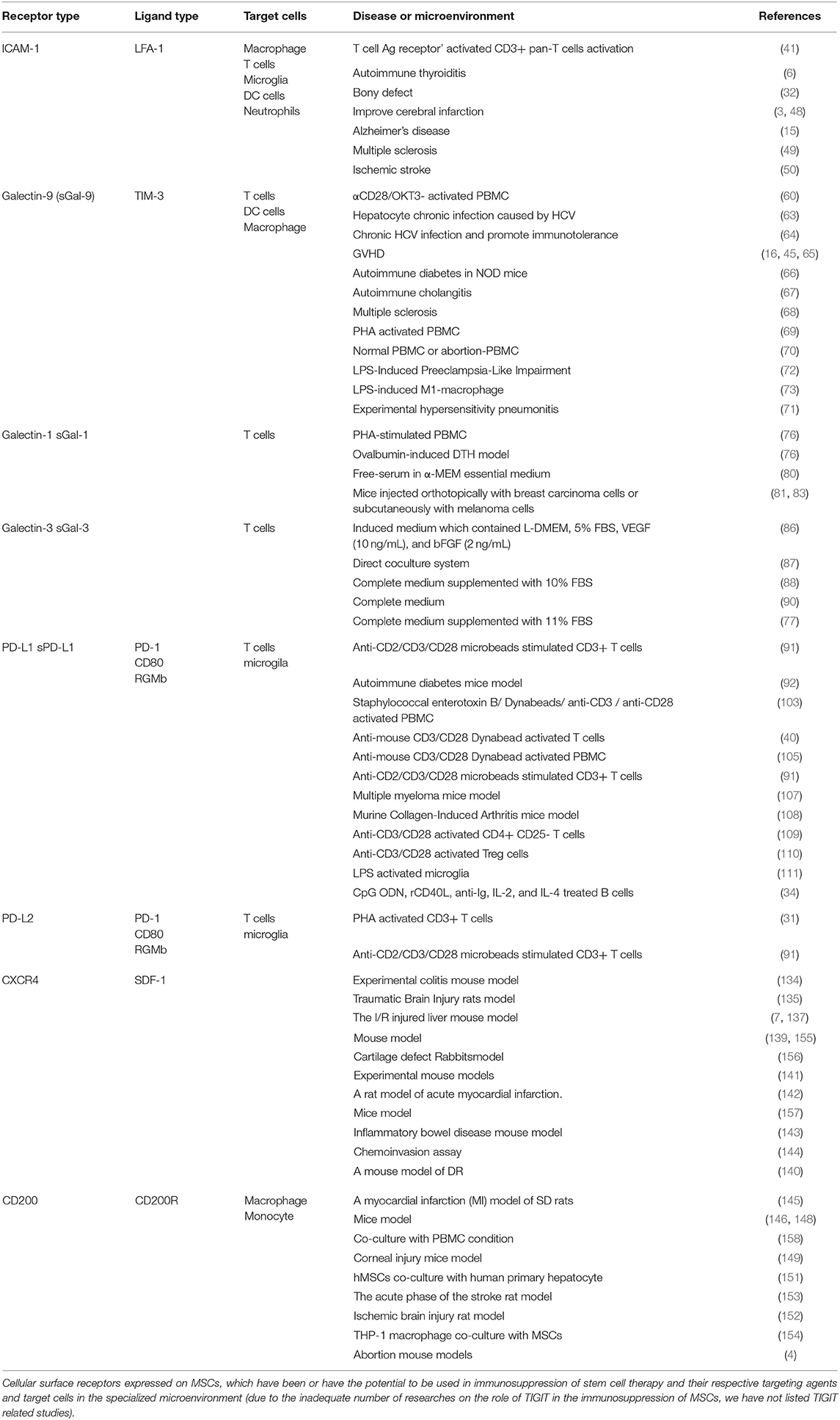
Table 1. Cell surface receptor expressed on mesenchymal stem cells that in the inflammatory microenvironment, which has been reported to have the potential to treat refractory disease by regulating the activity of different immune cells.
Surface molecules contribute to the immunosuppressive properties of MSCs and regulate the phenotype and activity of immune cells (Figure 3). Different surface molecules contribute to the immunosuppressive properties of MSCs in different stages indicated that similar to CXCR4, ICAM-1 facilitated the migration of MSCs to target cells at the site of injury. Subsequently, more MSCs will pool together, and then the co-inhibitory molecules on the MSCs will interact with immune cells directly, eventually regulate the microenvironment. These results indicated that transforming MSCs to a special status by altering the expression of surface molecules on MSCs might be beneficial for immunoregulatory properties. However, so far, most studies have used immune cells and MSCs co-culture system in vitro. Still, this method could not reflect the actual development of microenvironment and is limited in practical clinical applications. However, how to transform MSCs to express more molecules that are conductive to immunosuppression, and to maintain the capacities of stem cells, the safety and effectiveness of clinical application are the main issues we need to face in the future.
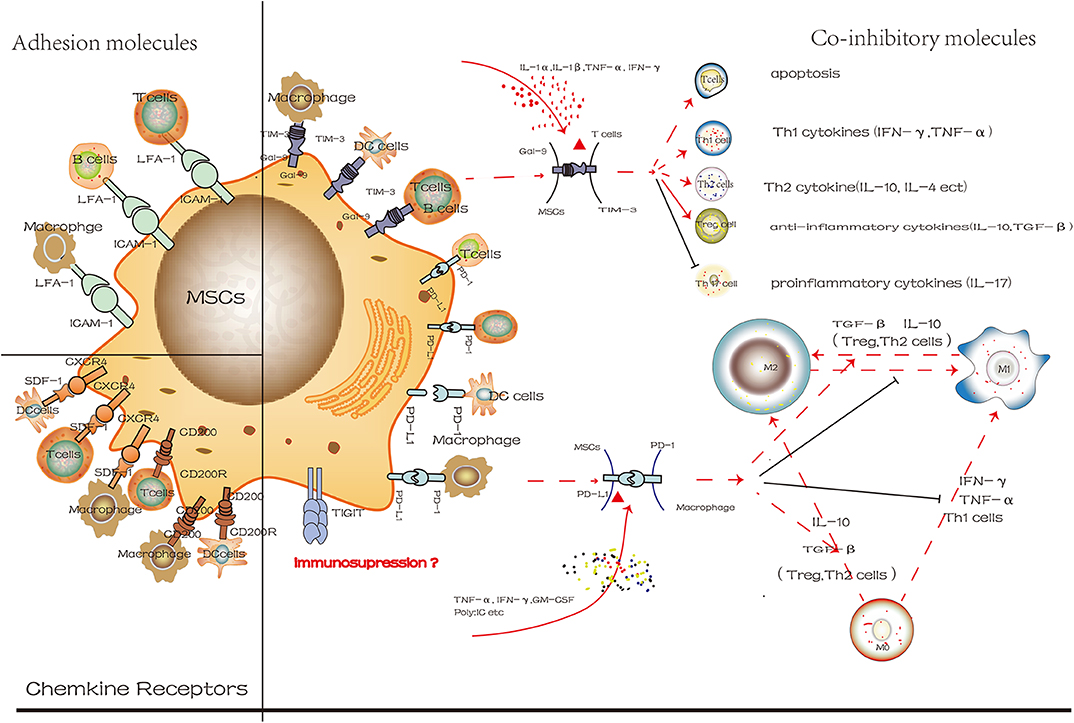
Figure 3. The different degree of cell surface molecules on MSCs in the inflammatory microenvironment is attractive targets for cellular surface receptor targeting therapies. These molecules mainly include co-inhibitory molecules, chemokine receptors and adhesion molecules. And we use Gal-9/TIM-3 and PD-L1/PD-1 to exhibit its mechanism in control of immune cells phenotypic change (red line represents positive effect while the black line represents the negative effect).
Author Contributions
SL, SG, YZ, and QS designed this review. SL and FL collected literatures and conducted the analysis of data. SL and SG drafted and revised the manuscript. FL and BJ wrote the manuscript. All authors contributed to review the manuscript, read, and approved the final manuscript.
Funding
This study was supported by the National Natural Science Foundation of China (Grant No. 51872332).
Conflict of Interest
The authors declare that the research was conducted in the absence of any commercial or financial relationships that could be construed as a potential conflict of interest.
References
1. Shin TH, Kim HS, Choi SW, Kang KS. Mesenchymal stem cell therapy for inflammatory skin diseases: clinical potential and mode of action. Int J Mol Sci. (2017) 18:244. doi: 10.3390/ijms18020244
2. Salami F, Tavassoli A, Mehrzad J, Parham A. Immunomodulatory effects of mesenchymal stem cells on leukocytes with emphasis on neutrophils. Immunobiology. (2018) 223:786–91. doi: 10.1016/j.imbio.2018.08.002
3. Takizawa N, Okubo N, Kamo M, Chosa N, Mikami T, Suzuki K, et al. Bone marrow-derived mesenchymal stem cells propagate immunosuppressive/anti-inflammatory macrophages in cell-to-cell contact-independent and-dependent manners under hypoxic culture. Exp Cell Res. (2017) 358:411–20. doi: 10.1016/j.yexcr.2017.07.014
4. Li YH, Zhang D, Xu L, Dong L, Zheng J, Lin YK, et al. Cell-cell contact with proinflammatory macrophages enhances the immunotherapeutic effect of mesenchymal stem cells in two abortion models. Cell Mol Immunol. (2019) 16:908–20. doi: 10.1038/s41423-019-0204-6
5. Zhou KJ, Guo S, Tong S, Sun Q, Li F, Zhang XW, et al. Immunosuppression of human adipose-derived stem cells on T cell subsets via the reduction of NF-kappaB activation mediated by PD-L1/PD-1 and Gal-9/TIM-3 pathways. Stem Cells Dev. (2018) 27:1191–202. doi: 10.1089/scd.2018.0033
6. Ma SF, Chen XH, Wang LH, Wei Y, Ni YQ, Chu YN, et al. Repairing effects of ICAM-1-expressing mesenchymal stem cells in mice with autoimmune thyroiditis. Exp Ther Med. (2017) 13:1295–302. doi: 10.3892/etm.2017.4131
7. Zhang CY, Zhu Y, Wang J, Hou LS, Li W, An HJ. CXCR4-overexpressing umbilical cord mesenchymal stem cells enhance protection against radiation-induced lung injury. Stem Cells Int. (2019) 2019:2457082. doi: 10.1155/2019/2457082
8. Ni HQ, Yang S, Siaw-Debrah F, Hu JN, Wu K, He ZB, et al. Exosomes derived from bone mesenchymal stem cells ameliorate early inflammatory responses following traumatic brain injury. Front Neurosci. (2019) 13:14. doi: 10.3389/fnins.2019.00014
9. Zhang Z, Huang S, Wu S, Qi J, Li W, Liu S, et al. Clearance of apoptotic cells by mesenchymal stem cells contributes to immunosuppression via PGE2. EBioMedicine. (2019) 45:341–50. doi: 10.1016/j.ebiom.2019.06.016
10. Bulati M, Miceli V, Gallo A, Amico G, Carcione C, Pampalone M, et al. The immunomodulatory properties of the human amnion-derived mesenchymal stromal/stem cells are induced by INF-gamma produced by activated lymphomonocytes and are mediated by cell-to-cell contact and soluble factors. Front Immunol. (2020) 11:54. doi: 10.3389/fimmu.2020.00054
11. He JG, Li BB, Zhou L, Yan D, Xie QL, Zhao W. Indoleamine 2,3-dioxgenase-transfected mesenchymal stem cells suppress heart allograft rejection by increasing the production and activity of dendritic cells and regulatory T cells. J Investig Med. (2020) 68:728–37. doi: 10.1136/jim-2019-001160
12. Wang HL, Zheng RQ, Chen QH, Shao J, Yu JQ, Hu SL. Mesenchymal stem cells microvesicles stabilize endothelial barrier function partly mediated by hepatocyte growth factor (HGF). Stem Cell Res Ther. (2017) 8:211. doi: 10.1186/s13287-017-0662-7
13. Perlee D, van Vught LA, Scicluna BP, Maag A, Lutter R, Kemper EM, et al. Intravenous infusion of human adipose mesenchymal stem cells modifies the host response to lipopolysaccharide in humans: a randomized, single-blind, parallel group, placebo controlled trial. Stem Cells. (2018) 36:1778–88. doi: 10.1002/stem.2891
14. Cui GY, Bai J, Miao LY, Lin DY, Liu H, Li YL, et al. Placenta-derived mesenchymal stem cells with HLA-G positive expression induce Treg in vitro. Zhongguo Ying Yong Sheng Li Xue Za Zhi. (2018) 34:396–400. doi: 10.12047/j.cjap.5621.2018.090
15. Kim JY, Kim DH, Kim JH, Lee D, Jeon HB, Kwon SJ, et al. Soluble intracellular adhesion molecule-1 secreted by human umbilical cord blood-derived mesenchymal stem cell reduces amyloid-beta plaques. Cell Death Different. (2012) 19:680–91. doi: 10.1038/cdd.2011.140
16. Li YM, Shi YY, Li Y, Yan L, Tang JT, Bai YJ, et al. Soluble Tim-3 and Gal-9 are associated with renal allograft dysfunction in kidney transplant recipients: a cross-sectional study. Int Immunopharmacol. (2018) 55:330–5. doi: 10.1016/j.intimp.2018.01.008
17. Philipp D, Suhr L, Wahlers T, Choi YH, Paunel-Gorgulu A. Preconditioning of bone marrow-derived mesenchymal stem cells highly strengthens their potential to promote IL-6-dependent M2b polarization. Stem Cell Res Ther. (2018) 9:286. doi: 10.1186/s13287-018-1039-2
18. Fan XL, Zeng QX, Li X, Li CL, Xu ZB, Deng XQ, et al. Induced pluripotent stem cell-derived mesenchymal stem cells activate quiescent T cells and elevate regulatory T cell response via NF-κB in allergic rhinitis patients. Stem Cell Res Ther. (2018) 9:170. doi: 10.1186/s13287-018-0896-z
19. Ciccocioppo R, Cangemi GC, Kruzliak P, Gallia A, Betti E, Badulli C, et al. Ex vivo immunosuppressive effects of mesenchymal stem cells on Crohn's disease mucosal T cells are largely dependent on indoleamine 2,3-dioxygenase activity and cell-cell contact. Stem Cell Res Ther. (2015) 6:137. doi: 10.1186/s13287-015-0122-1
20. Friedenstein AJ, Chailakhjan RK, Lalykina KS. The development of fibroblast colonies in monolayer cultures of guinea-pig bone marrow and spleen cells. Cell Tissue Kinet. (1970) 3:393–403. doi: 10.1111/j.1365-2184.1970.tb00347.x
21. Dominici M, Le Blanc K, Mueller I, Slaper-Cortenbach I, Marini FC, Krause DS, et al. Minimal criteria for defining multipotent mesenchymal stromal cells. The International Society for Cellular Therapy position statement. Cytotherapy. (2006) 8:315–7. doi: 10.1080/14653240600855905
22. Qin HL, Zhu XH, Zhang B, Zhou L, Wang WY. Clinical evaluation of human umbilical cord mesenchymal stem cell transplantation after angioplasty for diabetic foot. Exp Clin Endocrinol Diabetes. (2016) 124:497–503. doi: 10.1055/s-0042-103684
23. Ding G, Liu Y, Wang W, Wei FL, Liu DY, Fan ZP, et al. Allogeneic periodontal ligament stem cell therapy for periodontitis in swine. Stem Cells. (2010) 28:1829–38. doi: 10.1002/stem.512
24. Wang C, Tanjaya J, Shen J, Lee S, Bisht B, Pan HC, et al. Peroxisome proliferator-activated receptor-γ knockdown impairs bone morphogenetic protein-2-induced critical-size bone defect repair. Am J Pathol. (2019) 189:648–64. doi: 10.1016/j.ajpath.2018.11.019
25. Guo J, Guo S, Wang Y, Yu Y. Promoting potential of adipose derived stem cells on peripheral nerve regeneration. Mol Med Rep. (2017) 16:7297–304. doi: 10.3892/mmr.2017.7570
26. Gao XQ, Usas A, Tang Y, Lu AP, Tan J, Schneppendahl J, et al. A comparison of bone regeneration with human mesenchymal stem cells and muscle-derived stem cells and the critical role of BMP. Biomaterials. (2014) 35:6859–70. doi: 10.1016/j.biomaterials.2014.04.113
27. Chang SH, Park CG. Allogeneic ADSCs induce CD8 T cell-mediated cytotoxicity and faster cell death after exposure to xenogeneic serum or proinflammatory cytokines. Exp Mol Med. (2019) 51:1–10. doi: 10.1038/s12276-019-0231-5
28. Baer PC, Kuci S, Krause M, Kuci Z, Zielen S, Geiger H, et al. Comprehensive phenotypic characterization of human adipose-derived stromal/stem cells and their subsets by a high throughput technology. Stem Cells Dev. (2013) 22:330–9. doi: 10.1089/scd.2012.0346
29. Trounson A, McDonald C. Stem cell therapies in clinical trials: progress and challenges. Cell Stem Cell. (2015) 17:11–22. doi: 10.1016/j.stem.2015.06.007
30. Kabat M, Bobkov I, Kumar S, Grumet M. Trends in mesenchymal stem cell clinical trials 2004–2018: is efficacy optimal in a narrow dose range? Stem Cells Transl Med. (2020) 9:17–27. doi: 10.1002/sctm.19-0202
31. Li H, Wang WW, Wang GY, Hou Y, Xu FH, Liu RR, et al. Interferon-gamma and tumor necrosis factor-alpha promote the ability of human placenta-derived mesenchymal stromal cells to express programmed death ligand-2 and induce the differentiation of CD4(+)interleukin-10(+) and CD8(+)interleukin-10(+)Treg subsets. Cytotherapy. (2015) 17:1560–71. doi: 10.1016/j.jcyt.2015.07.018
32. Li YY, Kong N, Li Z, Tian R, Liu XH, Liu GZ, et al. Bone marrow macrophage M2 polarization and adipose-derived stem cells osteogenic differentiation synergistically promote rehabilitation of bone damage. J Cell Biochem. (2019) 120:19891–901. doi: 10.1002/jcb.29297
33. Abdelrazik H, Spaggiari GM, Chiossone L, Moretta L. Mesenchymal stem cells expanded in human platelet lysate display a decreased inhibitory capacity on T and NK cell proliferation and function. Transplantation. (2012) 94:1128. doi: 10.1097/00007890-201211271-02240
34. Liu OS, Xu JJ, Ding G, Liu DY, Fan ZP, Zhang CM, et al. Periodontal ligament stem cells regulate B lymphocyte function via programmed cell death protein 1. Stem Cells. (2013) 31:1371–82. doi: 10.1002/stem.1387
35. Gray A, Maguire T, Schloss R, Yarmush ML. Identification of IL-1β and LPS as optimal activators of monolayer and alginate-encapsulated mesenchymal stromal cell immunomodulation using design of experiments and statistical methods. Biotechnol Prog. (2015) 31:1058–70. doi: 10.1002/btpr.2103
36. Chamberlain CS, Clements AEB, Kink JA, Choi U, Baer GS, Halanski MA, et al. Extracellular vesicle-educated macrophages promote early achilles tendon healing. Stem Cells. (2019) 37:652–62. doi: 10.1002/stem.2988
37. Ren WH, Hou JF, Yang CG, Wang HJ, Wu ST, Wu YB, et al. Extracellular vesicles secreted by hypoxia pre-challenged mesenchymal stem cells promote non-small cell lung cancer cell growth and mobility as well as macrophage M2 polarization via miR-21–5p delivery. J Exp Clin Cancer Res. (2019) 38:62. doi: 10.1186/s13046-019-1027-0
38. Shi ZZ, Wang Q, Jiang DP. Extracellular vesicles from bone marrow-derived multipotent mesenchymal stromal cells regulate inflammation and enhance tendon healing. J Transl Med. (2019) 17:211. doi: 10.1186/s12967-019-1960-x
39. Németh K, Leelahavanichkul A, Yuen PS, Mayer B, Parmelee A, Doi K, et al. Bone marrow stromal cells attenuate sepsis via prostaglandin E(2)-dependent reprogramming of host macrophages to increase their interleukin-10 production. Nat Med. (2009) 15:42–9. doi: 10.1038/nm.1905
40. Luz-Crawford P, Noel D, Fernandez X, Khoury M, Figueroa F, Carrion F, et al. Mesenchymal stem cells repress Th17 molecular program through the PD-1 pathway. PLoS ONE. (2012) 7:e45272. doi: 10.1371/journal.pone.0045272
41. Ren GW, Zhao X, Zhang LY, Zhang JM, L'Huillier A, Ling WF, et al. Inflammatory cytokine-induced intercellular adhesion molecule-1 and vascular cell adhesion molecule-1 in mesenchymal stem cells are critical for immunosuppression. J Immunol. (2010) 184:2321–8. doi: 10.4049/jimmunol.0902023
42. Guo J, Zhang H, Xia J, Hou J, Wang Y, Yang T, et al. Interleukin-1β induces intercellular adhesion molecule-1 expression, thus enhancing the adhesion between mesenchymal stem cells and endothelial progenitor cells via the p38 MAPK signaling pathway. Int J Mol Med. (2018) 41:1976–82. doi: 10.3892/ijmm.2018.3424
43. Li Y, Feng C, Gao MQ, Jin MY, Liu TY, Yuan Y, et al. MicroRNA-92b-5p modulates melatonin-mediated osteogenic differentiation of bone marrow mesenchymal stem cells by targeting ICAM-1. J Cell Mol Med. (2019) 23:6140–53. doi: 10.1111/jcmm.14490
44. Rüster B, Göttig S, Ludwig RJ, Bistrian R, Müller S, Seifried E, et al. Mesenchymal stem cells display coordinated rolling and adhesion behavior on endothelial cells. Blood. (2006) 108:3938–44. doi: 10.1182/blood-2006-05-025098
45. Tang B, Li X, Liu Y, Chen X, Li X, Chu Y, et al. The therapeutic effect of ICAM-1-overexpressing mesenchymal stem cells on acute graft-versus-host disease. Cell Physiol Biochem. (2018) 46:2624–35. doi: 10.1159/000489689
46. Anbarasan C, Bavanilatha M, Latchumanadhas K, Ajit Mullasari. S. ICAM-1 molecular mechanism and genome wide SNP's association studies. Indian Heart J. (2015) 67:282–7. doi: 10.1016/j.ihj.2015.03.005
47. Lawson C, Wolf S. ICAM-1 signaling in endothelial cells. Pharmacol Rep. (2009) 61:22–32. doi: 10.1016/S1734-1140(09)70004-0
48. Tatebayashi K, Takagi T, Fujita M, Doe N, Nakagomi T, Matsuyama T, et al. Adipose-derived stem cell therapy inhibits the deterioration of cerebral infarction by altering macrophage kinetics. Brain Res. (2019) 1712:139–50. doi: 10.1016/j.brainres.2019.01.037
49. Benvenuto F, Voci A, Carminati E, Gualandi F, Mancardi G, Uccelli A, et al. Human mesenchymal stem cells target adhesion molecules and receptors involved in T cell extravasation. Stem Cell Res Ther. (2015) 6:245. doi: 10.1186/s13287-015-0222-y
50. Cheng Z, Wang LP, Qu MJ, Liang HB, Li WL, Li YF, et al. Mesenchymal stem cells attenuate blood-brain barrier leakage after cerebral ischemia in mice. J Neuroinflamm. (2018) 15:135. doi: 10.1186/s12974-018-1153-1
51. Ren G, Roberts AI, Shi Y. Adhesion molecules. Cell Adhesion Migrat. (2011) 5:20–2. doi: 10.4161/cam.5.1.13491
52. Ren GW, Zhang LY, Zhao X, Xu GW, Zhang YY, Roberts AI, et al. Mesenchymal stem cell-mediated immunosuppression occurs via concerted action of chemokines and nitric oxide. Cell Stem Cell. (2008) 2:141–50. doi: 10.1016/j.stem.2007.11.014
53. Ren GW, Su JJ, Zhang LY, Zhao X, Ling WF, L'Huillie A, et al. Species variation in the mechanisms of mesenchymal stem cell-mediated immunosuppression. Stem Cells. (2009) 27:1954–62. doi: 10.1002/stem.118
54. Grigorian A, Torossian S, Demetriou M. T-cell growth, cell surface organization, and the galectin-glycoprotein lattice. Immunol Rev. (2009) 230:232–46. doi: 10.1111/j.1600-065X.2009.00796.x
55. Thijssen VLJL, Poirier F, Baum LG, Griffioen AW. Galectins in the tumor endothelium: opportunities for combined cancer therapy. Blood. (2007) 110:2819–27. doi: 10.1182/blood-2007-03-077792
56. Chou FC, Chen HY, Kuo CC, Sytwu HK. Role of galectins in tumors and in clinical immunotherapy. Int J Mol Sci. (2018) 19:430. doi: 10.3390/ijms19020430
57. Arikawa T, Matsukawa A, Watanabe K, Sakata K-M, Seki M, Nagayama M, et al. Galectin-9 accelerates transforming growth factor beta 3-induced differentiation of human mesenchymal stem cells to chondrocytes. Bone. (2009) 44:849–57. doi: 10.1016/j.bone.2009.01.365
58. Ruvolo PP. Galectins as regulators of cell survival in the leukemia niche. Adv Biol Regul. (2019) 71:41–54. doi: 10.1016/j.jbior.2018.09.003
59. Kashio Y, Nakamura K, Abedin MJ, Seki M, Nishi N, Yoshida N, et al. Galectin-9 induces apoptosis through the calcium-calpain-caspase-1 pathway. J Immunol. (2003) 170:3631–6. doi: 10.4049/jimmunol.170.7.3631
60. Gieseke F, Kruchen A, Tzaribachev N, Bentzien F, Dominici M, Muller I. Proinflammatory stimuli induce galectin-9 in human mesenchymal stromal cells to suppress T-cell proliferation. Eur J Immunol. (2013) 43:2741–9. doi: 10.1002/eji.201343335
61. Mushtaq Z, Krishnan R, Prasad KP, Bedekar MK, Kumar AP. Molecular cloning, characterization and expression profiling of galectin-9 gene from Labeo rohita (Hamilton, 1822). Fish Shellf Immunol. (2018) 76:287–92. doi: 10.1016/j.fsi.2018.02.037
62. Ungerer C, Quade-Lyssy P, Radeke HH, Henschler R, Koenigs C, Koehl U, et al. Galectin-9 is a suppressor of T and B cells and predicts the immune modulatory potential of mesenchymal stromal cell preparations. Stem Cells Dev. (2014) 23:755–66. doi: 10.1089/scd.2013.0335
63. Ji XJ, Ma CJ, Wang JM, Wu XY, Niki T, Hirashima M, et al. HCV-infected hepatocytes drive CD4+CD25+Foxp3+regulatory T-cell development through the Tim-3/Gal-9 pathway. Eur J Immunol. (2013) 43:458–67. doi: 10.1002/eji.201242768
64. Zhuo Y, Zhang Y-F, Wu H-J, Qin L, Wang Y-P, Liu AM, et al. Interaction between Galectin-9/TIM-3 pathway and follicular helper CD4(+) T cells contributes to viral persistence in chronic hepatitis C. Biomed Pharmacother. (2017) 94:386–93. doi: 10.1016/j.biopha.2017.07.134
65. Veenstra RG, Taylor PA, Zhou Q, Panoskaltsis-Mortari A, Hirashima M, Flynn R, et al. Contrasting acute graft-versus-host disease effects of Tim-3/galectin-9 pathway blockade dependent upon the presence of donor regulatory T cells. Blood. (2012) 120:682–90. doi: 10.1182/blood-2011-10-387977
66. Chou F-C, Shieh S-J, Sytwu H-K. Attenuation of Th1 response through galectin-9 and T-cell Ig mucin 3 interaction inhibits autoimmune diabetes in NOD mice. Eur J Immunol. (2009) 39:2403–11. doi: 10.1002/eji.200839177
67. Fan JY, Tang XJ, Wang Q, Zhang ZY, Wu SF, Li WC, et al. Mesenchymal stem cells alleviate experimental autoimmune cholangitis through immunosuppression and cytoprotective function mediated by galectin-9. Stem Cell Res Ther. (2018) 9:237. doi: 10.1186/s13287-018-0979-x
68. Steelman AJ, Smith R, Welsh CJ, Li JR. Galectin-9 protein is up-regulated in astrocytes by tumor necrosis factor and promotes encephalitogenic T-cell apoptosis. J Biol Chem. (2013) 288:23776–87. doi: 10.1074/jbc.M113.451658
69. Kim SN, Lee HJ, Jeon MS, Yi T, Song SU. Galectin-9 is involved in immunosuppression mediated by human bone marrow-derived clonal mesenchymal stem cells. Immune Netw. (2015) 15:241–51. doi: 10.4110/in.2015.15.5.241
70. He MZ, Jiang M, Zhou Y, Li FF, Yang MT, Fan Y, et al. Impaired Gal-9 dysregulates the PBMC-induced Th1/Th2 imbalance in abortion-prone matings. J Immunol Res. (2018) 2018:9517842. doi: 10.1155/2018/9517842
71. Arikawa T, Saita N, Oomizu S, Ueno M, Matsukawa A, Katoh S, et al. Galectin-9 expands immunosuppressive macrophages to ameliorate T-cell-mediated lung inflammation. Eur J Immunol. (2010) 40:548–58. doi: 10.1002/eji.200939886
72. Li ZH, Wang LL, Liu H, Muyayalo KP, Huang XB, Mor G, et al. Galectin-9 alleviates LPS-induced preeclampsia-like impairment in rats via switching decidual macrophage polarization to M2 subtype. Front Immunol. (2019) 9:3142. doi: 10.3389/fimmu.2018.03142
73. Zhang W, Zhang YT, He YX, Wang XY, Fang Q. Lipopolysaccharide mediates time-dependent macrophage M1/M2 polarization through the Tim-3/Galectin-9 signalling pathway. Exp Cell Res. (2019) 376:124–32. doi: 10.1016/j.yexcr.2019.02.007
74. Kim H, Wang SY, Kwak G, Yang Y, Kwon IC, Kim SH. Exosome-guided phenotypic switch of M1 to M2 macrophages for cutaneous wound healing. Adv Sci. (2019) 6:1900513. doi: 10.1002/advs.201900513
75. Kadri T, Lataillade JJ, Doucet C, Marie A, Ernou I, Bourin P, et al. Proteomic study of galectin-1 expression in human mesenchymal stem cells. Stem Cells Dev. (2005) 14:204–12. doi: 10.1089/scd.2005.14.204
76. Lepelletier Y, Lecourt S, Renand A, Arnulf B, Vanneaux V, Fermand JP, et al. Galectin-1 and semaphorin-3A are two soluble factors conferring T-cell immunosuppression to bone marrow mesenchymal stem cell. Stem Cells Dev. (2010) 19:1075–9. doi: 10.1089/scd.2009.0212
77. Liu GY, Xu Y, Li Y, Wang LH, Liu YJ, Zhu DL. Secreted galectin-3 as a possible biomarker for the immunomodulatory potential of human umbilical cord mesenchymal stromal cells. Cytotherapy. (2013) 15:1208–17. doi: 10.1016/j.jcyt.2013.05.011
78. Fajka-Boja R, Urbán VS, Szebeni GJ, Czibula Á, Blaskó A, Kriston-Pál É, et al. Galectin-1 is a local but not systemic immunomodulatory factor in mesenchymal stromal cells. Cytotherapy. (2016) 18:360–70. doi: 10.1016/j.jcyt.2015.12.004
79. Najar M, Raicevic G, Boufker HI, Stamatopoulos B, De Bruyn C, Meuleman N, et al. Modulated expression of adhesion molecules and galectin-1: role during mesenchymal stromal cell immunoregulatory functions. Exp Hematol. (2010) 38:922–32. doi: 10.1016/j.exphem.2010.05.007
80. Yun SP, Lee SJ, Jung YH, Han HJ. Galectin-1 stimulates motility of human umbilical cord blood-derived mesenchymal stem cells by downregulation of smad2/3-dependent collagen 3/5 and upregulation of NF-κB-dependent fibronectin/laminin 5 expression. Cell Death Dis. (2014) 5:e1049. doi: 10.1038/cddis.2014.3
81. Szebeni GJ, Kriston-Pal E, Blazso P, Katona RL, Novak J, Szabo E, et al. Identification of galectin-1 as a critical factor in function of mouse mesenchymal stromal cell-mediated tumor promotion. PLoS ONE. (2012) 7:e41372. doi: 10.1371/journal.pone.0041372
82. Takigawa H, Kitadai Y, Shinagawa K, Yuge R, Higashi Y, Tanaka S, et al. Mesenchymal stem cells induce epithelial to mesenchymal transition in colon cancer cells through direct cell-to-cell contact. Neoplasia. (2017) 19:429–38. doi: 10.1016/j.neo.2017.02.010
83. Burns JS, Kristiansen M, Kristensen LP, Larsen KH, Nielsen MO, Christiansen H, et al. Decellularized matrix from tumorigenic human mesenchymal stem cells promotes neovascularization with galectin-1 dependent endothelial interaction. PLoS ONE. (2011) 6:e21888. doi: 10.1371/journal.pone.0021888
84. Chan J, O'Donoghue K, Gavina M, Torrente Y, Kennea N, Mehmet H, et al. Galectin-1 induces skeletal muscle differentiation in human fetal mesenchymal stem cells and increases muscle regeneration. Stem Cells. (2006) 24:1879–91. doi: 10.1634/stemcells.2005-0564
85. Ding Z, Liu XG, Ren XH, Zhang QL, Zhang TT, Qian Q, et al. Galectin-1-induced skeletal muscle cell differentiation of mesenchymal stem cells seeded on an acellular dermal matrix improves injured anal sphincter. Discov Med. (2016) 21:331–40.
86. Wan SY, Zhang TF, Ding Y. Galectin-3 enhances proliferation and angiogenesis of endothelial cells differentiated from bone marrow mesenchymal stem cells. Transplant Proceed. (2011) 43:3933–8. doi: 10.1016/j.transproceed.2011.10.050
87. Li YJ, Xu X, Wang LH, Liu GJ, Li YQ, Wu XB, et al. Senescent mesenchymal stem cells promote colorectal cancer cells growth via galectin-3 expression. Cell Biosci. (2015) 5:21. doi: 10.1186/s13578-015-0012-3
88. Gao Q, Xia Y, Liu L, Huang L, Liu Y, Zhang X, et al. Galectin-3 enhances migration of minature pig bone marrow mesenchymal stem cells through inhibition of RhoA-GTP activity. Sci Rep. (2016) 6:26577. doi: 10.1038/srep26577
89. Peng Y, Chen BQ, Zhao JL, Peng ZY, Xu WF, Yu GL. Effect of intravenous transplantation of hUCB-MSCs on M1/M2 subtype conversion in monocyte/macrophages of AMI mice. Biomed Pharmacother. (2019) 111:624–30. doi: 10.1016/j.biopha.2018.12.095
90. Reesink HL, Sutton RM, Shurer CR, Peterson RP, Tan JS, Su J, et al. Galectin-1 and galectin-3 expression in equine mesenchymal stromal cells (MSCs), synovial fibroblasts and chondrocytes, and the effect of inflammation on MSC motility. Stem Cell Res Ther. (2017) 8:243. doi: 10.1186/s13287-017-0691-2
91. Davies LC, Heldring N, Kadri N, Le Blanc. K. Mesenchymal stromal cell secretion of programmed death-1 ligands regulates T cell mediated immunosuppression. Stem Cells. (2017) 35:766–76. doi: 10.1002/stem.2509
92. Ben Nasr M, Tezza S, D'Addio F, Mameli C, Usuelli V, Maestroni A, et al. PD-L1 genetic overexpression or pharmacological restoration in hematopoietic stem and progenitor cells reverses autoimmune diabetes. Sci Transl Med. (2017) 9:eaam7543. doi: 10.1126/scitranslmed.aam7543
93. Ahmad SM, Borch TH, Hansen M, Andersen MH. PD-L1-specific T cells. Cancer Immunol Immunother. (2016) 65:797–804. doi: 10.1007/s00262-015-1783-4
94. Pai SI, Zandberg DP, Strome SE. The role of antagonists of the PD-1:PD-L1/PD-L2 axis in head and neck cancer treatment. Oral Oncol. (2016) 61:152–8. doi: 10.1016/j.oraloncology.2016.08.001
95. Ghiotto M, Gauthier L, Serriari N, Pastor S, Truneh A, Nunes JA, et al. PD-L1 and PD-L2 differ in their molecular mechanisms of interaction with PD-1. Int Immunol. (2010) 22:651–60. doi: 10.1093/intimm/dxq049
96. Keir ME, Butte MJ, Freeman GJ, Sharpel AH. PD-1 and its ligands in tolerance and immunity. Annu Rev Immunol. (2008) 26:677–704. doi: 10.1146/annurev.immunol.26.021607.090331
97. Herbst RS, Baas P, Kim DW, Felip E, Perez-Gracia JL, Han JY, et al. Pembrolizumab versus docetaxel for previously treated, PD-L1-positive, advanced non-small-cell lung cancer (KEYNOTE-010): a randomised controlled trial. Lancet. (2016) 387:1540–50. doi: 10.1016/S0140-6736(15)01281-7
98. Reck M, Rodriguez-Abreu D, Robinson AG, Hui RN, Csoszi T, Fulop A, et al. Pembrolizumab versus chemotherapy for PD-L1-positive non-small-cell lung cancer. N Engl J Med. (2016) 375:1823–33. doi: 10.1056/NEJMoa1606774
99. Westin JR, Chu FL, Zhang M, Fayad LE, Kwak LW, Fowler N, et al. Safety and activity of PD1 blockade by pidilizumab in combination with rituximab in patients with relapsed follicular lymphoma: a single group, open-label, phase 2 trial. Lancet Oncol. (2014) 15:69–77. doi: 10.1016/S1470-2045(13)70551-5
100. Yang MQ, Liu PP, Wang KF, Glorieux C, Hu YM, Wen SJ, et al. Chemotherapy induces tumor immune evasion by upregulation of programmed cell death ligand 1 expression in bone marrow stromal cells. Mol Oncol. (2017) 11:358–72. doi: 10.1002/1878-0261.12032
101. Camilleri ET, Gustafson MP, Dudakovic A, Riester SM, Garces CG, Paradise CR, et al. Identification and validation of multiple cell surface markers of clinical-grade adipose-derived mesenchymal stromal cells as novel release criteria for good manufacturing practice-compliant production. Stem Cell Res Ther. (2016) 7:107. doi: 10.1186/s13287-016-0370-8
102. Liu Y, Jing H, Kou XX, Chen CD, Liu DW, Jin Y, et al. PD-1 is required to maintain stem cell properties in human dental pulp stem cells. Cell Death Different. (2018) 25:1350–60. doi: 10.1038/s41418-018-0077-8
103. Chinnadurai R, Copland IB, Patel SR, Galipeau J. IDO-independent suppression of T cell effector function by IFN-gamma-licensed human mesenchymal stromal cells. J Immunol. (2014) 192:1491–501. doi: 10.4049/jimmunol.1301828
104. Cho KA, Park M, Kim YH, Ryu KH, Woo SY. Poly I:C primes the suppressive function of human palatine tonsil-derived MSCs against Th17 differentiation by increasing PD-L1 expression. Immunobiology. (2017) 222:394–8. doi: 10.1016/j.imbio.2016.08.012
105. Zhu J, Wen H, Ju X, Bi R, Zuo W, Wu X. Clinical significance of programmed death ligand-1 and intra-tumoral CD8+ T lymphocytes in ovarian carcinosarcoma. PLoS ONE. (2017) 12:e0170879. doi: 10.1371/journal.pone.0170879
106. Sun L, Wang QQ, Chen B, Zhao YY, Shen B, Wang XL, et al. Human gastric cancer mesenchymal stem cell-derived IL15 contributes to tumor cell epithelial-mesenchymal transition via upregulation tregs ratio and PD-1 expression in CD4(+)T cell. Stem Cells Dev. (2018) 27:1203–14. doi: 10.1089/scd.2018.0043
107. Chen DD, Tang P, Liu LX, Wang F, Xing HZ, Sun L, et al. Bone marrow-derived mesenchymal stem cells promote cell proliferation of multiple myeloma through inhibiting T cell immune responses via PD-1/PD-L1 pathway. Cell Cycle. (2018) 17:858–67. doi: 10.1080/15384101.2018.1442624
108. Yan ML, Liu X, Dang QJ, Huang H, Yang F, Li Y. Intra-articular injection of human synovial membrane-derived mesenchymal stem cells in murine collagen-induced arthritis: assessment of immunomodulatory capacity in vivo. Stem Cells Int. (2017) 2017:9198328. doi: 10.1155/2017/9198328
109. Yan ZD, Zhuansun YX, Liu GR, Chen R, Li JG, Ran PX. Mesenchymal stem cells suppress T cells by inducing apoptosis and through PD-1/B7-H1 interactions. Immunol Lett. (2014) 162:248–55. doi: 10.1016/j.imlet.2014.09.013
110. Yan ZD, Zhuansun YX, Chen R, Li JG, Ran PX. Immunomodulation of mesenchymal stromal cells on regulatory T cells and its possible mechanism. Exp Cell Res. (2014) 324:65–74. doi: 10.1016/j.yexcr.2014.03.013
111. Wu WJ, Lan Q, Lu H, Xu J, Zhu AH, Fang WF, et al. Human amnion mesenchymal cells negative co-stimulatory molecules PD-L1 expression and its capacity of modulating microglial activation of CNS. Cell Biochem Biophys. (2014) 69:35–45. doi: 10.1007/s12013-013-9763-9
112. Yu X, Harden K, Gonzalez LC, Francesco M, Chiang E, Irving B, et al. The surface protein TIGIT suppresses T cell activation by promoting the generation of mature immunoregulatory dendritic cells. Nat Immunol. (2009) 10:48–57. doi: 10.1038/ni.1674
113. Pauken KE, Wherry EJ. TIGIT and CD226: tipping the balance between costimulatory and coinhibitory molecules to augment the cancer immunotherapy toolkit. Cancer Cell. (2014) 26:785–7. doi: 10.1016/j.ccell.2014.11.016
114. Zhou XM, Li WQ, Wu YH, Han L, Cao XG, Yang XM, et al. Intrinsic expression of immune checkpoint molecule TIGIT could help tumor growth in vivo by suppressing the function of NK and CD8(+) T cells. Front Immunol. (2018) 9:2821. doi: 10.3389/fimmu.2018.02821
115. Zhang Q, Bi JC, Zheng XD, Chen YY, Wang H, Wu WY, et al. Blockade of the checkpoint receptor TIGIT prevents NK cell exhaustion and elicits potent anti-tumor immunity. Nat Immunol. (2018) 19:723. doi: 10.1038/s41590-018-0132-0
116. Sun HY, Huang Q, Huang M, Wen H, Lin RY, Zheng MJ, et al. Human CD96 correlates to natural killer cell exhaustion and predicts the prognosis of human hepatocellular carcinoma. Hepatology. (2019) 70:168–83. doi: 10.1002/hep.30347
117. Zhang C, Lin R, Li Z, Yang S, Bi X, Wang H, et al. Immune exhaustion of T cells in alveolar echinococcosis patients and its reversal by blocking checkpoint receptor TIGIT in a murine model. Hepatology. (2020) 71:1297–15. doi: 10.1002/hep.30896
118. Josefsson SE, Huse K, Kolstad A, Beiske K, Pende D, Steen CB, et al. T cells expressing checkpoint receptor TIGIT are enriched in follicular lymphoma tumors and characterized by reversible suppression of T-cell receptor signaling. Clin Cancer Res. (2018) 24:870–81. doi: 10.1158/1078-0432.CCR-17-2337
119. Noguchi Y, Maeda A, Lo PC, Takakura C, Haneda T, Kodama T, et al. Human TIGIT on porcine aortic endothelial cells suppresses xenogeneic macrophage-mediated cytotoxicity. Immunobiology. (2019) 224:605–13. doi: 10.1016/j.imbio.2019.07.008
120. Stengel KF, Harden-Bowles K, Yu X, Rouge L, Yin JP, Comps-Agrar L, et al. Structure of TIGIT immunoreceptor bound to poliovirus receptor reveals a cell-cell adhesion and signaling mechanism that requires cis-trans receptor clustering. Proc Natl Acad Sci USA. (2012) 109:5399–404. doi: 10.1073/pnas.1120606109
121. Manieri NA, Chiang EY, Grogan JL. TIGIT: a key inhibitor of the cancer immunity cycle. Trends Immunol. (2017) 38:20–8. doi: 10.1016/j.it.2016.10.002
122. Joller N, Kuchroo VK. Tim-3, Lag-3, and TIGIT. Curr Top Microbiol Immunol. (2017) 410:127–56. doi: 10.1007/82_2017_62
123. Lozano E, Joller N, Cao YH, Kuchroo VK, Hafler DA. The CD226/CD155 interaction regulates the proinflammatory (Th1/Th17)/anti-inflammatory (Th2) balance in humans. J Immunol. (2013) 191:3673–80. doi: 10.4049/jimmunol.1300945
124. Lozano E, Dominguez-Villar M, Kuchroo V, Hafler DA. The TIGIT/CD226 axis regulates human T cell function. J Immunol. (2012) 188:3869–75. doi: 10.4049/jimmunol.1103627
125. Levin SD, Taft DW, Brandt CS, Bucher C, Howard ED, Chadwick EM, et al. Vstm3 is a member of the CD28 family and an important modulator of T-cell function. Eur J Immunol. (2011) 41:902–15. doi: 10.1002/eji.201041136
126. Johnston RJ, Comps-Agrar L, Hackney J, Yu X, Huseni M, Yang Y, et al. The immunoreceptor TIGIT regulates antitumor and antiviral CD8(+) T cell effector function. Cancer Cell. (2014) 26:923–37. doi: 10.1016/j.ccell.2014.10.018
127. Dougall WC, Kurtulus S, Smyth MJ, Anderson AC. TIGIT and CD96: new checkpoint receptor targets for cancer immunotherapy. Immunol Rev. (2017) 276:112–20. doi: 10.1111/imr.12518
128. Guillerey C, Harjunpaa H, Carrie N, Kassem S, Teo T, Miles K, et al. TIGIT immune checkpoint blockade restores CD8(+) T-cell immunity against multiple myeloma. Blood. (2018) 132:1689–94. doi: 10.1182/blood-2018-01-825265
129. Chen X, Lu PH, Liu L, Fang ZM, Duan W, Liu ZL, et al. TIGIT negatively regulates inflammation by altering macrophage phenotype. Immunobiology. (2016) 221:48–55. doi: 10.1016/j.imbio.2015.08.003
130. Riquelme P, Haarer J, Kammler A, Walter L, Tomiuk S, Ahrens N, et al. TIGIT(+) iTregs elicited by human regulatory macrophages control T cell immunity. Nat Commun. (2018) 9:2858. doi: 10.1038/s41467-018-05167-8
131. He WL, Zhang H, Han F, Chen XL, Lin R, Wang W, et al. CD155T/TIGIT signaling regulates CD8(+) T-cell metabolism and promotes tumor progression in human gastric cancer. Cancer Res. (2017) 77:6375–88. doi: 10.1158/0008-5472.CAN-17-0381
132. Giuliani M, Bennaceur-Griscelli A, Nanbakhsh A, Oudrhiri N, Chouaib S, Azzarone B, et al. TLR ligands stimulation protects MSC from NK killing. Stem Cells. (2014) 32:290–300. doi: 10.1002/stem.1563
133. DelaRosa O, Sanchez-Correa B, Morgado S, Ramirez C, del Rio B, Menta R, et al. Human adipose-derived stem cells impair natural killer cell function and exhibit low susceptibility to natural killer-mediated lysis. Stem Cells Dev. (2012) 21:1333–43. doi: 10.1089/scd.2011.0139
134. Li X, Lan X, Zhao Y, Wang G, Shi G, Li H, et al. SDF-1/CXCR4 axis enhances the immunomodulation of human endometrial regenerative cells in alleviating experimental colitis. Stem Cell Res Ther. (2019) 10:204. doi: 10.1186/s13287-019-1298-6
135. Deng QJ, Xu XF, Ren J. Effects of SDF-1/CXCR4 on the repair of traumatic brain injury in rats by mediating bone marrow derived mesenchymal stem cells. Cell Mol Neurobiol. (2018) 38:467–77. doi: 10.1007/s10571-017-0490-4
136. Li L, Jaiswal PK, Makhoul G, Jurakhan R, Selvasandran K, Ridwan K, et al. Hypoxia modulates cell migration and proliferation in placenta-derived mesenchymal stem cells. J Thoracic Cardiovasc Surg. (2017) 154:543. doi: 10.1016/j.jtcvs.2017.03.141
137. Jin W, Liang XW, Brooks A, Futrega K, Liu X, Doran MR, et al. Modelling of the SDF-1/CXCR4 regulated in vivo homing of therapeutic mesenchymal stem/stromal cells in mice. PeerJ. (2018) 6:e6072. doi: 10.7717/peerj.6072
138. Barzelay A, Algor SW, Niztan A, Katz S, Benhamou M, Nakdimon I, et al. Adipose-derived mesenchymal stem cells migrate and rescue RPE in the setting of oxidative stress. Stem Cells Int. (2018) 2018:9682856. doi: 10.1155/2018/9682856
139. Bae YK, Kim GH, Lee JC, Seo BM, Joo KM, Lee G, et al. The significance of SDF-1α-CXCR4 axis in in vivo angiogenic ability of human periodontal ligament stem cells. Mol Cells. (2017) 40:386–92. doi: 10.14348/molcells.2017.0004
140. Wang J, Zhang W, He GH, Wu B, Chen S. Transfection with CXCR4 potentiates homing of mesenchymal stem cells in vitro and therapy of diabetic retinopathy in vivo. Int J Ophthalmol. (2018) 11:766–72. doi: 10.18240/ijo.2018.05.08
141. Chen Z, Chen QQ, Du HT, Xu LJ, Wan J. Mesenchymal stem cells and CXC chemokine receptor 4 overexpression improved the therapeutic effect on colitis via mucosa repair. Exp Ther Med. (2018) 16:821–9. doi: 10.3892/etm.2018.6233
142. Tian XQ, Yang YJ, Li Q, Xu J, Huang PS, Xiong YY, et al. Combined therapy with atorvastatin and atorvastatin-pretreated mesenchymal stem cells enhances cardiac performance after acute myocardial infarction by activating SDF-1/CXCR4 axis. Am J Transl Res. (2019) 11:4214. doi: 10.1016/j.jacc.2018.08.401
143. Nan Z, Fan H, Tang Q, Zhang M, Xu M, Chen Q, et al. Dual expression of CXCR4 and IL-35 enhances the therapeutic effects of BMSCs on TNBS-induced colitis in rats through expansion of Tregs and suppression of Th17 cells. Biochem Biophys Res Commun. (2018) 499:727–34. doi: 10.1016/j.bbrc.2018.03.043
144. Wynn RF, Hart CA, Corradi-Perini C, O'Neill L, Evans CA, Wraith JE, et al. A small proportion of mesenchymal stem cells strongly expresses functionally active CXCR4 receptor capable of promoting migration to bone marrow. Blood. (2004) 104:2643–5. doi: 10.1182/blood-2004-02-0526
145. Wu SZ, Li YL, Huang W, Cai WF, Liang JL, Paul C, et al. Paracrine effect of CXCR4-overexpressing mesenchymal stem cells on ischemic heart injury. Cell Biochem Funct. (2017) 35:113–23. doi: 10.1002/cbf.3254
146. Zhu YZ, Yang YX, Zhang YL, Hao GL, Liu T, Wang LB, et al. Placental mesenchymal stem cells of fetal and maternal origins demonstrate different therapeutic potentials. Stem Cell Res Ther. (2014) 5:48. doi: 10.1186/scrt436
147. Minas K, Liversidge J. Is the CD200/CD200 receptor interaction more than just a myeloid cell inhibitory signal? Crit Rev Immunol. (2006) 26:213–30. doi: 10.1615/CritRevImmunol.v26.i3.20
148. Pontikoglou C, Langonne A, Ba MA, Varin A, Rosset P, Charbord P, et al. CD200 expression in human cultured bone marrow mesenchymal stem cells is induced by pro-osteogenic and pro-inflammatory cues. J Cell Mol Med. (2016) 20:655–65. doi: 10.1111/jcmm.12752
149. Amouzegar A, Mittal SK, Sahu A, Sahu SK, Chauhan SK. Mesenchymal stem cells modulate differentiation of myeloid progenitor cells during inflammation. Stem Cells. (2017) 35:1532–41. doi: 10.1002/stem.2611
150. Delorme B, Ringe J, Gallay N, Le Vern Y, Kerboeuf D, Jorgensen C, et al. Specific plasma membrane protein phenotype of culture-amplified and native human bone marrow mesenchymal stem cells. Blood. (2008) 111:2631–5. doi: 10.1182/blood-2007-07-099622
151. Wang J, Zhu ZY, Huang Y, Wang P, Luo Y, Gao YT, et al. The subtype CD200-positive, chorionic mesenchymal stem cells from the placenta promote regeneration of human hepatocytes. Biotechnol Lett. (2014) 36:1335–41. doi: 10.1007/s10529-014-1468-7
152. McGuckin CP, Jurga M, Miller AM, Sarnowska A, Wiedner M, Boyle NT, et al. Ischemic brain injury: a consortium analysis of key factors involved in mesenchymal stem cell-mediated inflammatory reduction. Arch Biochem Biophys. (2013) 534:88–97. doi: 10.1016/j.abb.2013.02.005
153. Li Z, Ye H, Cai X, Sun W, He B, Yang Z, et al. Bone marrow-mesenchymal stem cells modulate microglial activation in the peri-infarct area in rats during the acute phase of stroke. Brain Res Bull. (2019) 153:324–33. doi: 10.1016/j.brainresbull.2019.10.001
154. Pietila M, Lehtonen S, Tuovinen E, Lahteenmaki K, Laitinen S, Leskela HV, et al. CD200 positive human mesenchymal stem cells suppress TNF-alpha secretion from CD200 receptor positive macrophage-like cells. PLoS ONE. (2012) 7:e31671. doi: 10.1371/journal.pone.0031671
155. Lim RZL, Li L, Yong EL, Chew N. STAT-3 regulation of CXCR4 is necessary for the prenylflavonoid Icaritin to enhance mesenchymal stem cell proliferation, migration and osteogenic differentiation. Biochim Biophys Acta Gener Subj. (2018) 1862:1680–92. doi: 10.1016/j.bbagen.2018.04.016
156. Wang YZ, Sun XJ, Lv J, Zeng LY, Wei XC, Wei L. Stromal cell-derived factor-1 accelerates cartilage defect repairing by recruiting bone marrow mesenchymal stem cells and promoting chondrogenic differentiation. Tissue Eng Part A. (2017) 23:1160–8. doi: 10.1089/ten.tea.2017.0046
157. Nam H, Kim GH, Bae YK, Jeong DE, Joo KM, Lee K, et al. Angiogenic capacity of dental pulp stem cell regulated by SDF-1α-CXCR4 axis. Stem Cells Int. (2017) 2017:8085462. doi: 10.1155/2017/8085462
Keywords: mesenchymalstemcells, immunosuppression, cellularsurfacemolecules, adhesionreceptors, chemokinereceptors, co-inhibitorymolecules
Citation: Liu S, Liu F, Zhou Y, Jin B, Sun Q and Guo S (2020) Immunosuppressive Property of MSCs Mediated by Cell Surface Receptors. Front. Immunol. 11:1076. doi: 10.3389/fimmu.2020.01076
Received: 28 February 2020; Accepted: 04 May 2020;
Published: 28 July 2020.
Edited by:
Irun R. Cohen, Weizmann Institute of Science, IsraelReviewed by:
Stefania Scala, IRCCS Istituto Nazionale Tumori Napili “G. Pascale”, ItalyStefano Ugel, University of Verona, Italy
Copyright © 2020 Liu, Liu, Zhou, Jin, Sun and Guo. This is an open-access article distributed under the terms of the Creative Commons Attribution License (CC BY). The use, distribution or reproduction in other forums is permitted, provided the original author(s) and the copyright owner(s) are credited and that the original publication in this journal is cited, in accordance with accepted academic practice. No use, distribution or reproduction is permitted which does not comply with these terms.
*Correspondence: Shu Guo, c2d1b0BjbXUuZWR1LmNu