- 1Department of Pediatrics, A. Nocivelli Institute for Molecular Medicine, ASST Spedali Civili of Brescia, Brescia, Italy
- 2Department of Molecular and Translational Medicine, A. Nocivelli Institute for Molecular Medicine, University of Brescia, Brescia, Italy
- 3A. Nocivelli Institute for Molecular Medicine, ASST Spedali Civili of Brescia, Brescia, Italy
- 4Section of General Pathology, Department of Medicine, University of Verona, Verona, Italy
- 5Department of Molecular and Translational Medicine, University of Brescia, Brescia, Italy
- 6Laboratory of Human Genetics of Infectious Diseases, Necker Branch, INSERM UMR 1163, Paris, France
- 7University of Paris, Imagine Institute, Paris, France
- 8St. Giles Laboratory of Human Genetics of Infectious Diseases, Rockefeller Branch, The Rockefeller University, New York, NY, United States
- 9Pediatric Hematology and Immunology Unit, Necker Hospital for Sick Children, AP-HP, Paris, France
- 10Howard Hughes Medical Institute, New York, NY, United States
- 11Center for the Study of Primary Immunodeficiencies, Necker Hospital for Sick Children, AP-HP, Paris, France
- 12Department of Clinical and Experimental Sciences, Department of Pediatrics, A. Nocivelli Institute for Molecular Medicine, University of Brescia, Brescia, Italy
Interferon-γ receptor 1 (IFNγR1) deficiency is one of the inborn errors of IFN-γ immunity underlying Mendelian Susceptibility to Mycobacterial Disease (MSMD). This molecular circuit plays a crucial role in regulating the interaction between dendritic cells (DCs) and T lymphocytes, thus affecting DCs activation, maturation, and priming of T cells involved in the immune response against intracellular pathogens. We studied a girl who developed at the age of 2.5 years a Mycobacterium avium infection characterized by disseminated necrotizing granulomatous lymphadenitis, and we compared her findings with other patients with the same genetic condition. The patient carried a heterozygous 818del4 mutation in the IFNGR1 gene responsible of autosomal dominant (AD) partial IFNγR1 deficiency. During the acute infection blood cells immunophenotyping showed a marked reduction in DCs counts, including both myeloid (mDCs) and plasmacytoid (pDCs) subsets, that reversed after successful prolonged antimicrobial therapy. Histology of her abdomen lymph node revealed a profound depletion of tissue pDCs, as compared to other age-matched granulomatous lymphadenitis of mycobacterial origin. Circulating DCs depletion was also observed in another patient with AD partial IFNγR1 deficiency during mycobacterial infection. To conclude, AD partial IFNγR1 deficiency can be associated with a transient decrease in both circulating and tissular DCs during acute mycobacterial infection, suggesting that DCs counts monitoring might constitute a useful marker of treatment response.
Introduction
Interferon-γ receptor 1 (IFNγR1) deficiency was discovered in 1996 as the first genetic etiology of the Mendelian Susceptibility to Mycobacterial Disease (MSMD) (1, 2). MSMD is a rare inherited primary immunodeficiency due to impaired IFN-γ-mediated immunity that leads to selective predisposition to clinical disease caused by weakly virulent mycobacteria, such as bacillus Calmette-Guerin (BCG) vaccines and non-tuberculous environmental mycobacteria (EM) (3). This condition also exposes to higher risk of infection from the more virulent Mycobacterium tuberculosis, Candida spp., Salmonella, and, more rarely, to other intra-macrophagic bacteria, fungi, or parasites. Mutations in the IFNGR1 gene account for the altered cell surface expression of the high affinity ligand-binding chain of the receptor and impair the cellular response to IFNγ. IFNGR1 mutations result in five distinct clinical phenotypes depending on the pattern of inheritance (dominant or recessive), the impact of the mutation (complete or partial defect) and the expression of the mutant allele. Patients with autosomal recessive (AR) complete IFNγR1 deficiency display the most severe clinical phenotype with life-threatening disseminated infections starting in early childhood (3). In this form, the majority are null mutations that prevent the production of the receptor; alternatively, in-frame deletions and missense mutations are reported to cause the production of IFNγR1 that, altered in its extracellular domain, in unable to bind the ligand (3). It has been hypothesized that high levels of serum IFNγ may be responsible for the high rate of graft rejection observed in these patients after hematopoietic stem cell transplantation (HSCT), that currently represents the only curable treatment (4). In patients with AR partial IFNγR1 deficiency, homozygous mutations include specifically the I87T, V63G, and M1K mutations: in leukocytes the receptor is expressed but not responsive to high concentrations of IFNγ. The clinical outcome of infections and the survival are favorable (5). Finally, the autosomal dominant (AD) form of partial IFNγR1 deficiency includes heterozygous mutations in the cytoplasmic domain of the receptor leading to truncated molecule that accumulates at the cell surface, binds IFNγ, but cannot transduce the signal. Typically, these patients manifest mycobacterial disease at an older age (late childhood/adulthood) and are successfully treated with prolonged antimicrobial treatment (6, 7). The susceptibility to mycobacteria and to other intracellular opportunistic pathogens is also common to autosomal defects in IFNGR2, IL12B, IL12RB1, STAT1, ISG15, IRF8 genes, or X-linked mutations in NEMO and CYBB genes, or in the recently described signal peptide peptidase-like 2 A (SPPL2A), IL12RB2, and IL23R deficiencies, TYK2 deficiency, or syndromic forms due to RORγ/RORγT and JAK1 deficiencies (3, 8–10). We herein report the immunological study of a pediatric patient with AD partial IFNγR1 deficiency who onset with disseminated mycobacterial disease that associated with defective dendritic cells (DCs) counts.
Materials and Methods
H&E and Immunohistochemistry
Four μm tissue sections from formalin-fixed and paraffin-embedded lymph nodes from the patient (PT) and two age-matched children were used for Hematoxylin and Eosin staining (H&E) and immunohistochemical staining to CD3 (clone LN10, Leica Biosystems), CD20 (clone L26, Leica Biosystems), and CD303 (clone 124B3.13, Dendritics) antigens. Briefly, after appropriate antigen retrival, antibodies were revealed using Novolink Polymer (Leica Biosystems) followed by Diaminobenzidine (DAB) and counterstained with hematoxylin.
Sequencing of the IFNGR1 Gene
Informed consent for hematological, immunological, and genetic tests was obtained from the patient's parents. The investigation protocol was approved by the local ethic committee of the Asst Spedali Civili of Brescia. We adhered to standard biosecurity and institutional safety procedures. The IFNGR1 gene was analyzed by Sanger sequencing by using standard techniques. DNA was isolated from whole blood using QIAamp DNA Blood Mini Kit (Qiagen). IFNGR1 gene was amplified by PCR and products were sequenced using BigDye Terminator Kit (Applied Biosystems). Sequences were analyzed with 310 Genetic Analyzer (Applied Biosystems).
Expression of IFNγR1 and Phosphorylation of STAT1 on Monocytes by Flow Cytometry Assay
IFNγR1 cell-surface expression was assessed on monocytes (CD14+ cells) by using a standard flow cytometry protocol that requested a staining of 100 μl of fresh whole blood with PE-conjugated anti-human IFNγR1 mAb (BD Biosciencies). Blood samples were acquired using a FACSCalibur flow cytometer (Becton Dickinson, San Diego, Calif) and analyzed by the FlowJo software version 7.5 Software (TreeStar, Ashland, Ore). Cells were fixed and permeabilized, following the manufacturer's instructions (BD Biosciences). Similarly, analysis of STAT1 phosphorylation was performed on 100 μl of fresh whole blood that was left unstimulated or stimulated with IFN-γ (1,000 U/ml for 30 min). Blood cells were fixed and permeabilized, following the manufacturer's instructions (Becton Dickinson) and intracellular staining was performed using a PE-conjugated anti-human pSTAT1-Tyr-701 mAb (BD Pharmigen). Blood samples were acquired using a FACSCalibur flow cytometer and analysis of monocytes (CD14+ cells) pSTAT1 expression performed by FlowJo software.
Cytokine Detection
Peripheral blood mononuclear cells (PBMCs) were obtained from EDTA-treated whole blood of the patient and healthy control by the standard density centrifugation procedure, in Ficoll Hypaque. Cytokine production was measured in the supernatants after 24 h of incubation by ELISA using matched paired Abs specific for IFNα (Mabtech) and CXCL10 (R&D systems).
Immunophenotype Analysis by Flow Cytometry
Whole blood (100 or 200 ml) was stained for immunophenotypic analysis using a combination of monoclonal antibodies (mAb) (Becton Dickinson, San Diego, Calif), according to standard multiparametric flow cytometry protocols, in order to identify different lymphocytes and DCs subsets. Blood samples were acquired using a FACSCantoII flow cytometer (Becton Dickinson) and analyses were performed with the FlowJo software version 8.8.7 (TreeStar, Ashland, Ore). Immunological data were compared to the reference values of the “A. Nocivelli” Institute for Molecular Medicine of Brescia that performed the analysis, based on a database obtained from a pool of age-matched healthy subjects.
Statistical Analysis
Statistical significance of immunological studies was analyzed by non-parametric two-side Mann– Whitney U-test with 95% confidence bounds. For all analyses p < 0.05 was considered statistically significant. Statistical analyses were performed using GraphPad Prism Version 5.0 (GraphPad Software, San Diego, CA).
Results
Disseminated Mycobacterial Infection in Partial Dominant IFNγR1 Deficiency
A 2.5-year-old female (P1) was admitted to the hospital because of 3 weeks of daily fever (over 38°C) associated with fatigue, vomiting and diarrhea. She was born in Italy from Pakistani non-consanguineous parents; her past medical history was uneventful, and the family history was unremarkable. She had not received BCG vaccine, nor had never been to Pakistan. At admission, her blood tests revealed microcytic anemia (Hb 6.5 g/dl, MCV 60.9 fl, RDW 19.4%), raised C-reactive protein (CRP 125 mg/L, range <5 mg/L) and procalcitonin (9.2 ng/ml, range <0.1 ng/ml), leukocytosis (WBC 21,600 cells/mmc) with neutrophilia (ANC 17,170 cells/mmc). She had normal lymphocyte count (ALC 3,230 cells/mmc), and monocyte count at the lower range (AMC 230 cells/mmc). On physical examination, she had mild spleen enlargement. The abdomen ultrasound and the computed tomography-scan revealed a spleen length of 8 cm [mean of normal age-related spleen size from 6.8 to 7.5 cm (11)] with multiple dishomogenous areas, slight hepatomegaly, without focal lesions, and multiple mediastinal and mesenteric lymph nodes showing internal colliquation. The infectious disease screening included blood cultures, Brucella, Rickettsia, Bartonella, Coxiella, Leishmania, Toxoplasma, Plasmodium, Herpesviruses, Epstein-Barr virus, and HIV serology, all tested negative. The Mantoux tuberculin skin test read after 48 h resulted on induration of 5 mm, while the QuantiFERON-TB Gold tested negative. She underwent a laparoscopic biopsy of an abdomen lymph node (20 × 14 mm) that showed a necrotizing granulomatous lymphadenitis with focal colliquation and the Mycobacterium avium was detected by PCR assay. The patient was commenced with the association therapy of clarithromycin, ethambutol and rifabutin, the latter discontinued after 6 months because of an acute drug-related uveitis. The dual therapy with clarithromycin and ethambutol was continued for 18 months. The patient fully recovered. At onset, routine immunological tests revealed slightly elevated levels of serum immunoglobulin (Ig) M (IgG 1,220 mg/dl -range 462–1,710 mg/dl-, IgA 167 mg/dl -range 27–173 mg/dl-, IgM 465 mg/dl -range 62–257 mg/dl-), and IgE (575 range KU/L -range <140 KU/L-), normal neutrophil respiratory burst assay, and normal bone marrow aspirate. The analysis of the lymphocytes subsets displayed a prevalence of effector T and B cells, an increased proportion of activated HLA-DR+ T cells, and reduction of NK cell percentages (CD16+CD56+ 2.7%/90 cells/mmc, normal range 5–28.4%/100–1,000 cells/mmc) (Table 1).
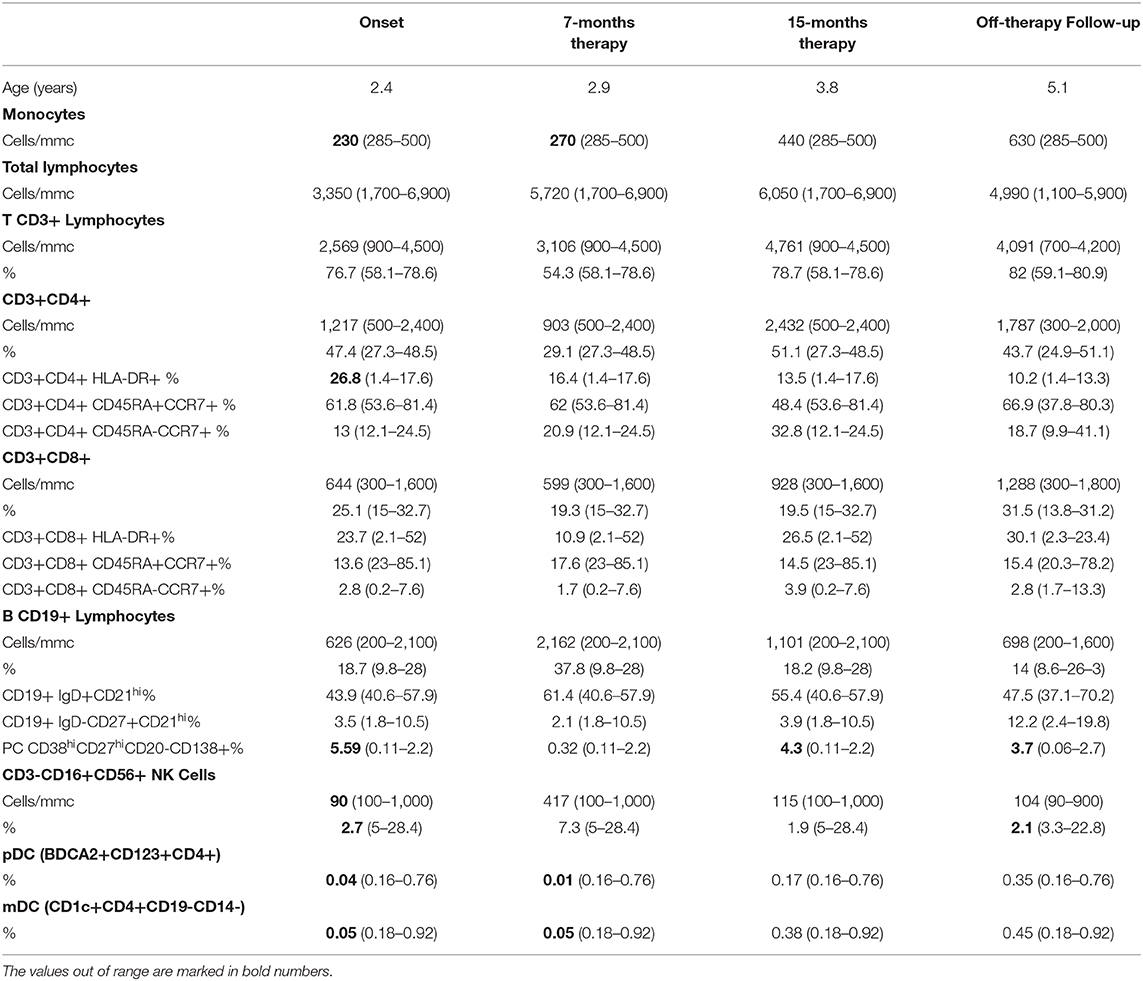
Table 1. The immunophenotype of the patient P1 heterozygous for 818del4 IFNGR1 mutation at the onset of the disease and during follow-up.
IFNGR1 gene sequencing revealed a heterozygous small deletion in the exon 6, the c.819_822delTAAT (p.N274Hfs*2). This mutation is already reported as responsible of AD partial IFNγR1 deficiency (7). The flow cytometry analysis of IFNγR1 expression on patient's monocytes showed that the receptor was present at a higher level on cell's surface (Figures 1A,B), while STAT1 phosphorylation (pY-701) following stimulation with IFNγ was markedly reduced, as compared to the healthy control (Figures 1C,D).
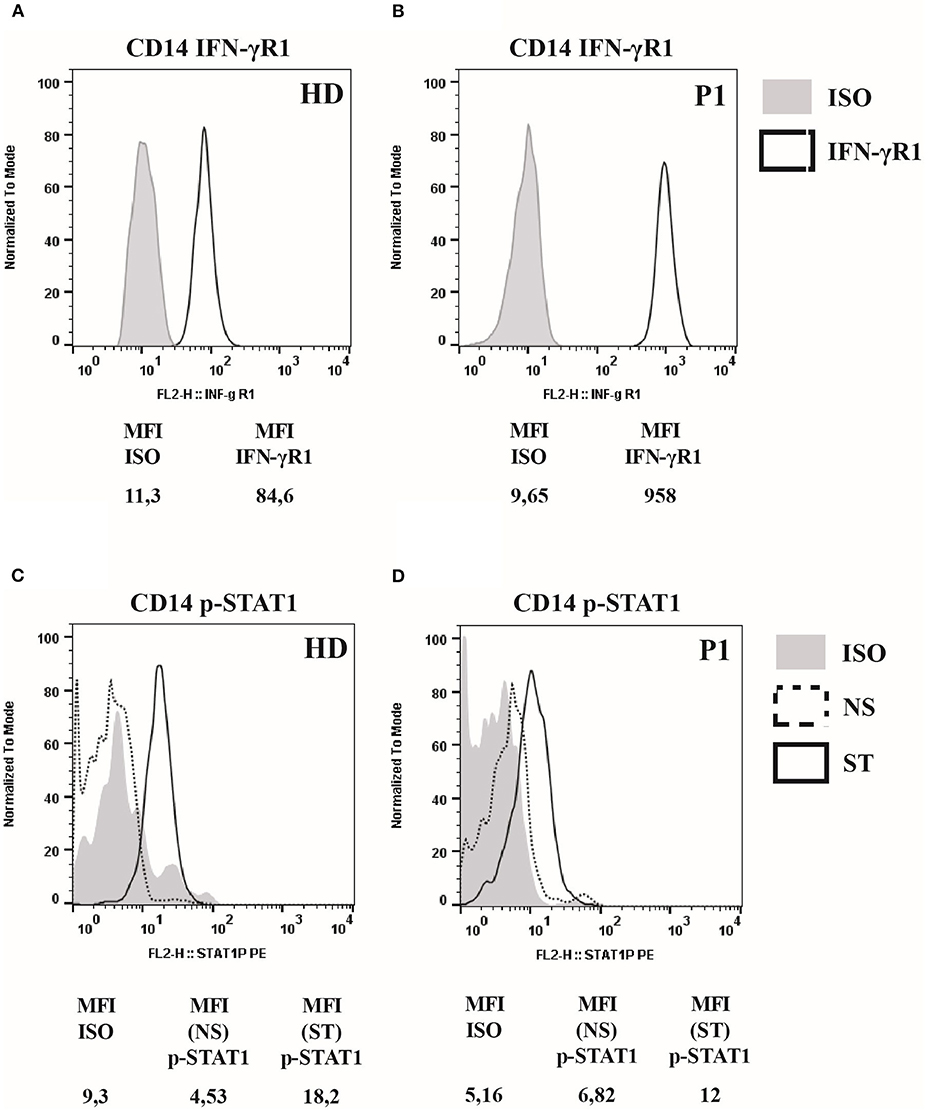
Figure 1. IFNγR1 cell-surface expression and activity in cells of patient heterozygous for 818del4 IFNGR1 mutation. (A) The expression of IFNγR1 molecules (measured as Mean Fluorescence Intensity, MFI) at the surface of CD14-positive mononuclear blood cells, in the patient (P1) and a healthy donor (HD), by flow cytometry assay with a mouse antibody specific for human IFNγR1, and an isotype control. (B) The p-STAT1 level expression (measured as MFI), to analyze IFNγR1-mediated signaling, is detected by flow cytometry assay with a mouse antibody specific for human p-STAT1, and an isotype control, after incubation with IFN-γ (1,000 UI/ml) for 30 min, or medium alone, in CD14-positive mononuclear blood cells in the patient (P1) and a healthy donor (HD).
Study of DCs Counts Revealed a Depletion of Circulating and Tissular Subsets
Analysis of DCs was performed based on specific cell surface markers. Myeloid/classical DCs (mDCs) express typical myeloid antigens (CD11c, CD13, CD33, CD11b) with the majority of them expressing CD1c/BDCA1, while plasmacytoid DCs (pDCs) express CD123, CD303/BDCA2, CD304/BDCA4, and produces IFNα after activation with CpG or viral infection (12–16). At the time of P1 mycobacterial infection diagnosis, we observed a marked reduction of pDCs equal to 0.04%/143 cells/mmc (normal range 0.16–0.76%) and of mDCs equal to 0.05%/179 cells/mmc (normal range 0.18–0.92%) (Table 1). Monitoring of DCs subsets during the infection treatment showed that the percentage of DCs progressively increased, reverting to the normal levels by the end of the treatment (18 months) and remaining normal in the following months (Figure 2A). In addition, ELISA measurements of IFNα and CXCL10 in 24-h supernatants of PBMCs incubated with CpG or with increasing copies of human Herpes Simplex Virus Type 1 (HSV1) demonstrated lower levels of these cytokines in P1 as compared to the healthy control (Figures 2B,C), which is in accord with the blood depletion of pDCs.
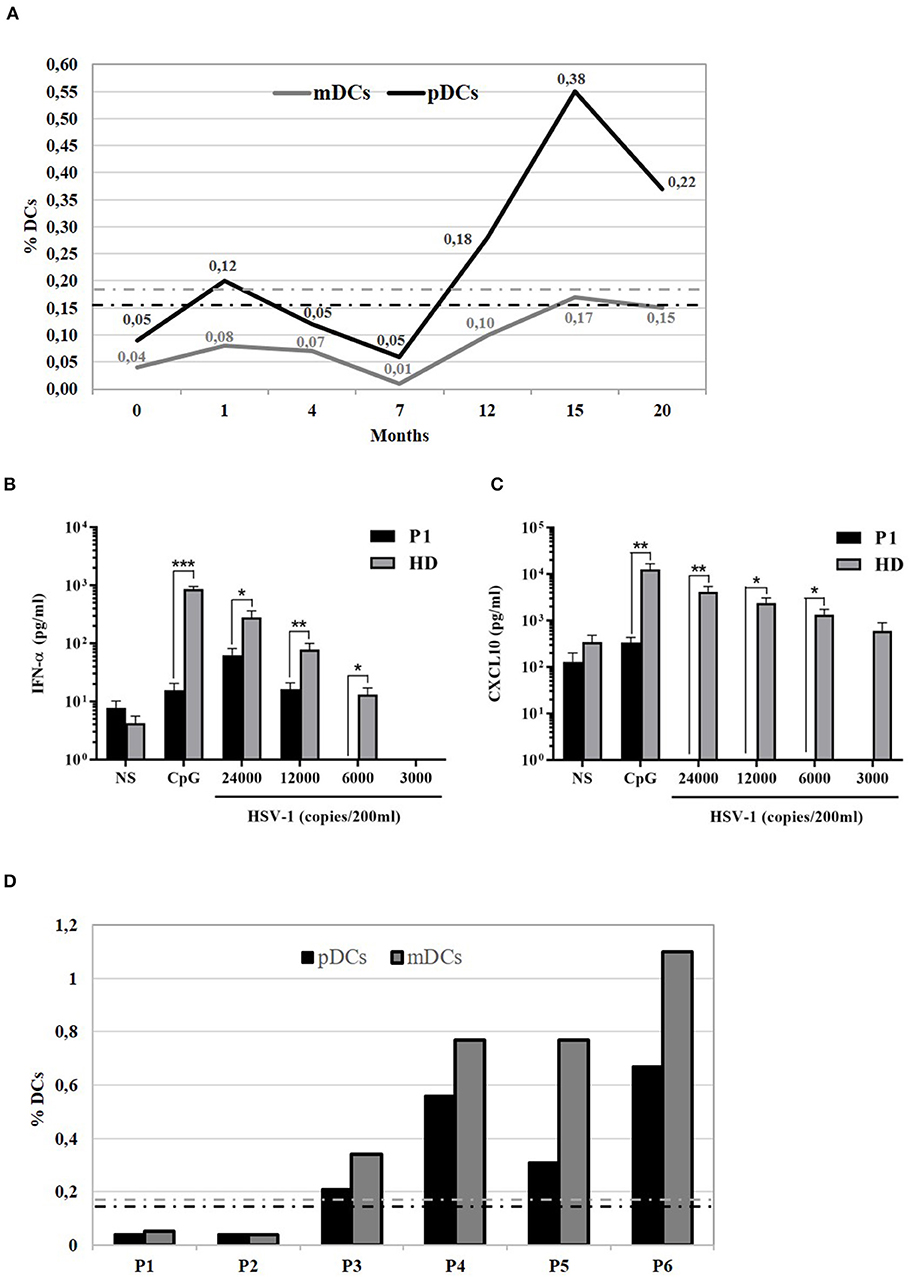
Figure 2. Functional analysis of 818del4 mutated IFNγR1 and study of patient's DCs. (A) Percentage of plasmacytoid dendritic cells (%pDCs) and myeloid dendritic cells (%mDCs) in P1 in timing (months) of therapy and follow-up (the dotted lines indicate the normal values, gray-colored for mDCs and black-colored for pDCs). IFN-α (B) and CXCL10 production (C) are measured in triplicate, by freshly isolated PBMCs after stimulation for 24 h with 6 mg/ml CpG, HSV-1 (copies/200 ml), or medium alone, in the patient (P1) and a healthy donor (HD). Statistical analysis by non-parametric test shows a significant difference (*p < 0.05, **p < 0.01, ***p < 0.001). (D) Plasmacytoid dendritic cells (%pDCs) and myeloid dendritic cells (%mDCs) are analyzed in other patients with mycobacterial infection: P2 has AD partial IFNγR1 deficiency and had acute mycobacterial infection during the evaluation of flow cytometry, while P3 has AD partial IFNγR1 deficiency but blood was collected when he was free of infection; P4, P5, and P6 presented a mycobacterial infection without any identified predisposing genetic cause (the dotted lines indicate the normal values, gray-colored for mDCs and black-colored for pDCs).
Next, we compared DCs subsets in other two patients (P2 and P3) with AD partial IFNγR1 deficiency, in P2 during a mycobacterial infection, while P3 was free of acute mycobacterial infection at the time the blood sample was collected. We also analyzed DCs in three other patients that had a mycobacterial cervical lymphadenopathy but still without any evidence of genetic mutations associated with MSMD. We detected a low number of both pDCs and mDCs subsets in P2, while DCs counts were normal in all the other investigated patients (Figure 2D). Finally, in P1, we studied DCs maturation by flow cytometry: the analysis of HLA-DR, CD80, CD83, and CD86 markers revealed that, after 24 h of in vitro culture with LPS, DCs exhibited a normal pattern of maturation (Figure 3).
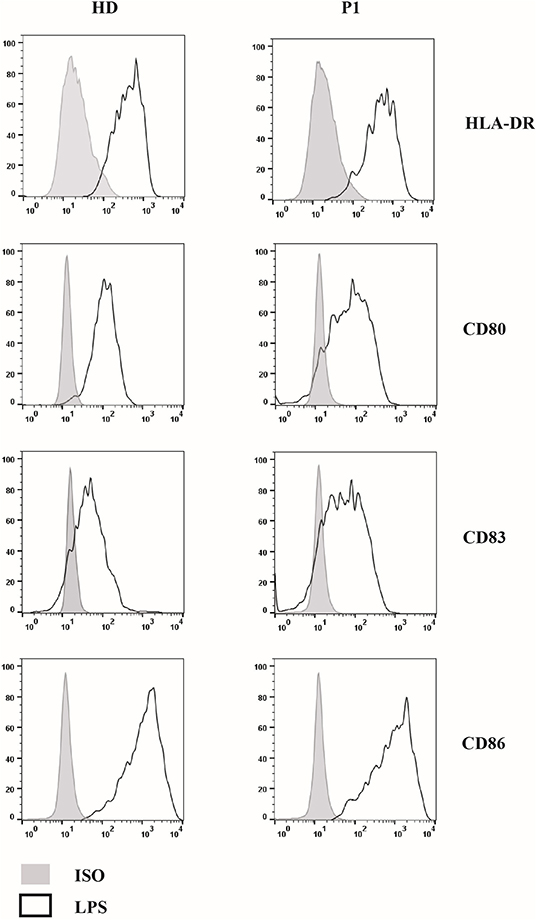
Figure 3. Study of 818del4 mutated DCs maturation pattern. DCs are cultured for 24 h with LPS for both the patient (P1) and a healthy donor (HD) and the expression of DCs maturation markers (HLA-DR, CD80, CD83, CD86) are analyzed by flow cytometry, together with an isotype control (PE- or PerCP- IgG): a similar pattern of maturation is present in both the patient and the healthy subject.
The Study of the Mycobacteria-Infected Lymph Node Biopsy Revealed pDCs Depletion
In P1, the analysis of the lymph node biopsy revealed a granulomatous necrotizing lymphadenitis with BDCA2+ plasmacytoid dendritic cells reduction (Figures 4A–E), as compared with other age-matched granulomatous lymphadenitis of mycobacterial origin derived from control subjects (Figures 4F–L); the distribution of CD20+ B-cells and CD3+ T-cells of the nodal parenchyma was normal.
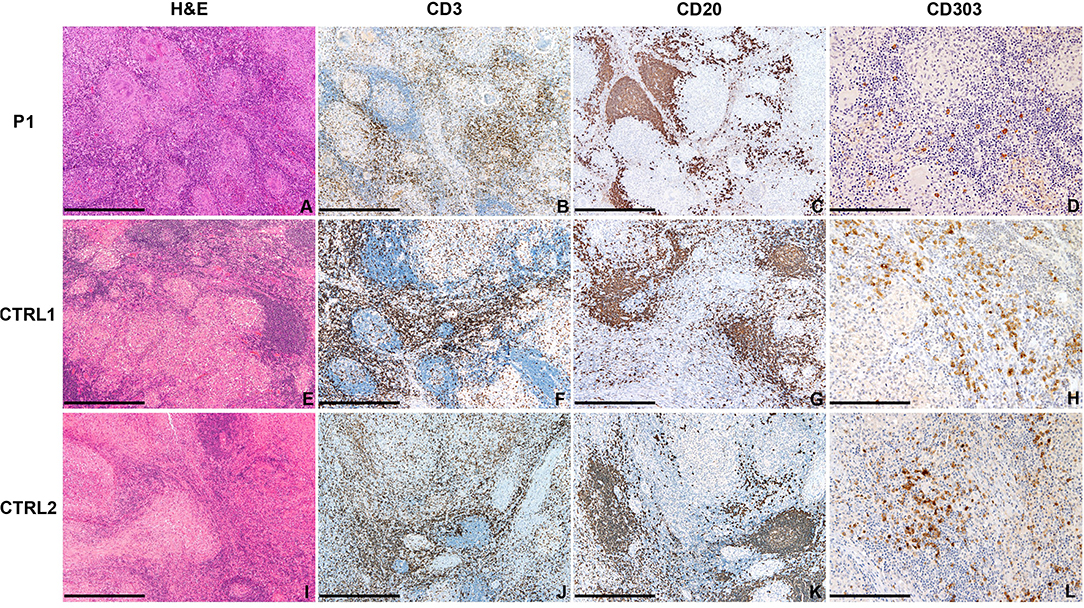
Figure 4. Abdominal lymph node histology. Sections are from lymph nodes of P1 (A–D) and two age-matched control (E–L) with mycobacterial infection, stained as labeled. On H&E, necrotizing granulomas are surrounded by lymphoid cells represented by CD20+ B-cells and CD3+ T-cells. CD303/BDCA2+ pDC are severely reduced as compared to matched controls. Original magnification: 40x (A–C,E–G,I–K; scale bar 500 μm), 100x (D,H,L; scale bar 200 μm).
Discussion
Immature DCs circulating in the peripheral blood can recognize and internalize pathogens for antigen presentation to T lymphocytes. Upon activation, DCs undergo maturation, secrete T cell activating cytokines such as IL-12, and migrate to lymph nodes where they have an essential role in orchestrating the host defense (11–15). In recent years, the identification of patients with DCs deficiency has allowed the investigation of the cellular pathways and genetic regulation of DCs haematopoiesis and their complex role in both innate and adaptive immunity. DCs deficiency (in both myeloid and plasmocytoid subsets) has been reported in patients with heterozygous germline GATA2 mutations, a heterogeneous disorder characterized by familial myelodysplasia/acute myeloid leukemia (MSD/AML), the MonoMac (monocytopenia with Mycobacterium avium complex) syndrome, and/or the Emberger syndrome (primary lymphedema and MSD/AML) (17). In patients with Interferon Regulator Factor (IRF) eight mutations the AR variant causes a complete lack of circulating monocytes and DCs, while the AD variant associates with a selective depletion of CD11c+CD1c+ circulating dendritic cells (18, 19), as reported also in patients with the recently described SPPL2A (signal peptide peptidase-like 2A) deficiency, where a defective IL-12 and IL-23 production by mDCs may disrupt the priming of T lymphocytes (19, 20). Other disorders may display pancytopenia with monocyte and DCs deficiencies, from the WHIM (warts, hypogammaglobulinemia, infections, myelokathexis) syndrome (21), to defects in genes related to DCs development and functions, such as the CD40/CD40L deficiency, the MCH class II deficiency, the Wiskott-Aldrich syndrome, the Pitt-Hopkins Syndrome, or the IRF7 deficiency (22). The activation of DCs depends upon a crosstalk with CD4+ T lymphocytes, enabling the same DCs to prime cytotoxic T lymphocytes, polarize a Th1 T cells response, and regulate the immunity against intracellular pathogens (23–25). In lymph nodes, the T-cell response requires contact-dependent interaction with DCs, in order to activate cytokine production and cellular proliferation (26). Miro et al. investigated the T cell-driven maturation of DCs in a human in vitro model and supported how the secretion of IL-12 by DCs is dependent upon co-stimulatory stimuli, the CD40-CD40L-interaction during a direct cell-cell contact with CD4+ T cells, and the IFNγ production by T cells, in the presence of the antigen (27). Meanwhile, DCs maturation and activation requires T cell response to IL-12 and IFNγ: defect in the IL12β1R receptor prevents IL-12-mediated signaling and impairs both the expression of DCs maturation markers and the DCs production of IL-12. Moreover, the IFNγ receptor response in T cells is required for a functional interaction with DCs, likely through the STAT1-dependent IL-12R β2 expression on T cells via IFNγ signaling (28). Herein, we report the case of a de novo AD partial IFNγR1 deficiency that onset in early childhood as disseminated lymphadenitis due to Mycobacterium avium, well-responding to a treatment with clarithromycin and ethambutol. The acute phase of the mycobacterial infection associated with a marked reduction of both mDCs and pDCs, as measured in the peripheral blood. In addition, lymph node biopsy analysis demonstrated a profound decrease of pDCs also in the lymphoid tissue. It has been reported that DCs are decreased in HIV (29), in the most severe form of dengue fever (30), and in patients with active tuberculosis (TB), where it is associated an impaired IFNα production (31). Particularly, it was observed a more significant reduction of pDCs both in the pulmonary and extrapulmonary forms of TB, while mDCs count was lower in the extrapulmonary form of TB. It has been showed that DC counts can increase during highly active antiretroviral therapy and normalize after anti-tuberculous therapy in responder patients. Similarly, in our patient DCs subsets progressively increased and normalized after the end of the 18-months therapy. In addition, evaluation of DCs maturation in vitro appeared normal, thus suggesting that circulating mDCs and pDCs depletion during the active mycobacterial infection may not be related to abnormal generation of these cell types. Conversely, a disrupted DCs trafficking or increased DCs apoptosis due to the defective IFNγR1-mediated signaling might account for DCs depletion during mycobacterial infection. Further studies will allow to elucidate DCs role in response against mycobacterial disease and evaluate the DC counts as a diagnostic tool to monitor treatment response or to predict development of mycobacterial infection in patients with inborn errors of IFNγ immunity.
Data Availability Statement
All datasets presented in this study are included in the article/supplementary materials.
Ethics Statement
The studies involving human participants were reviewed and approved by Comitato Etico Spedali Civili of Brescia. Written informed consent to participate in this study was provided by the participants' legal guardian/next of kin. Written informed consent was obtained from the minor(s)' legal guardian/next of kin for the publication of any potentially identifiable images or data included in this article.
Author Contributions
LD followed the patient and wrote the manuscript. DV, MG, and NT performed the assays. DM performed the immunophenotyping assay. WV and SL performed the histology study of lymph nodes. LD, DV, and RB analyzed all the data and the results. SG, JB, and J-LC participated in the conception of the work and revised the manuscript. RB designed and supervised the whole study. All authors participated in manuscript writing and/or revised the manuscript and approved the final version.
Funding
This work was supported by a grant from Ministero della Salute (RF-2016-02362384) to RB.
Conflict of Interest
The authors declare that the research was conducted in the absence of any commercial or financial relationships that could be construed as a potential conflict of interest.
References
1. Newport MJ, Huxley CM, Huston S, Hawrylowicz CM, Oostra BA, Williamson R, et al. A mutation in the interferon-gamma-receptor gene and susceptibility to mycobacterial infection. N Engl J Med. (1996) 335:1941–9. doi: 10.1056/NEJM199612263352602
2. Dorman SE, Picard C, Lammas D, Heyne K, van Dissel JT, Baretto R, et al. Clinical features of dominant and recessive interferon γ receptor 1 deficiencies. Lancet. (2004) 364:2113–21. doi: 10.1016/S0140-6736(04)17552-1
3. Bustamante J, Boisson-Dupuis S, Abel L, Casanova JL. Mendelian susceptibility to mycobacterial disease: genetic, immunological, and clinical features of inborn errors of IFN-γ immunity. Semin Immunol. (2014) 26:454–70. doi: 10.1016/j.smim.2014.09.008
4. Roesler J, Horwitz ME, Picard C, Bordigoni P, Davies G, Koscielniak E, et al. Hematopoietic stem cell transplantation for complete IFN-γ receptor 1 deficiency: a multi-institutional survey. J Pediatr. (2004) 145:806–12. doi: 10.1016/j.jpeds.2004.08.021
5. Sologuren I, Boisson-Dupuis S, Pestano J, Vincent QB, Fernández-Pérez L, Chapgier A, et al. Partial recessive IFN-R1 deficiency: genetic, immunological and clinical features of 14 patients from 11 kindreds. Hum Mol Genet. (2011) 20:1509–23. doi: 10.1093/hmg/ddr029
6. Holland SM. Treatment of infections in the patient with Mendelian susceptibility to mycobacterial infection. Microbes Infect. (2000) 2:1579–90. doi: 10.1016/S1286-4579(00)01314-9
7. Jouanguy E, Lamhamedi-Cherradi S, Lammas D, Dorman SE, Fondanèche MC, Dupuis S, et al. A human IFNGR1 small deletion hotspot associated with dominant susceptibility to mycobacterial infection. Nat Genet. (1999) 21:370–8. doi: 10.1038/7701
8. Rosain J, Kong XF, Martinez-Barricarte R, Oleaga-Quintas C, Ramirez-Alejo N, Markle J, et al. Mendelian susceptibility to mycobacterial disease: 2014–2018 update. Immunol Cell Biol. (2019) 97:360–67. doi: 10.1111/imcb.12210
9. Boisson-Dupuis S, Ramirez-Alejo N, Li Z, Patin E, Rao G, Kerner G, et al. Tuberculosis and impaired IL-23–dependent IFN- immunity in humans homozygous for a common TYK2 missense variant. Sci Immunol. (2018) 3:aau8714. doi: 10.1126/sciimmunol.aau8714
10. Martínez-Barricarte R, Markle JG, Ma CS, Deenick EK, Ramírez-Alejo N, Mele F, et al. Human IFN- immunity to mycobacteria is governed by both IL-12 and IL-23. Sci Immunol. (2018) 3:aau6759. doi: 10.1126/sciimmunol.aau6759
11. Calle-Toro JS, Back SJ, Viteri B, Andronikou S, Kaplan SL. Liver, spleen, and kidney size in children as measured by ultrasound: a systematic review. J Ultrasound Med. (2020) 39:223–30. doi: 10.1002/jum.15114
12. MacDonald KPA, Munster DJ, Clark GJ, Dzionek A, Schmitz J, Hart DNJ. Characterization of human blood dendritic cell subsets. Blood. (2002) 100:4512–20. doi: 10.1182/blood-2001-11-0097
13. Ziegler-Heitbrock L, Ancuta P, Crowe S, Dalod M, Grau V, Hart DN, et al. Nomenclature of monocytes and dendritic cells in blood. Blood. (2010) 116:e74–80. doi: 10.1182/blood-2010-02-258558
14. Watowich SS, Liu YJ. Mechanisms regulating dendritic cell specification and development. Immunol Rev. (2010) 238:76–92. doi: 10.1111/j.1600-065X.2010.00949.x
15. Reizis B, Bunin A, Ghosh HS, Lewis KL, Sisirak V. Plasmacytoid dendritic cells: recent progress and open questions. Annu Rev Immunol. (2011) 29:163–83. doi: 10.1146/annurev-immunol-031210-101345
16. Collin M, Mcgovern N, Haniffa M. Human dendritic cell subsets. Immunology. (2013) 154:3–20. doi: 10.1111/imm.12888
17. Wlodarski MW, Hirabayashi S, Pastor V, Starý J, Hasle H, Masetti R, et al. Prevalence, clinical characteristics, and prognosis of GATA2-related myelodysplastic syndromes in children and adolescents. Blood. (2016) 127:1387–97. doi: 10.1182/blood-2015-09-669937
18. Bigley V, Maisuria S, Cytlak U, Jardine L, Care MA, Green K, et al. Biallelic interferon regulatory factor 8 mutation: a complex immunodeficiency syndrome with dendritic cell deficiency, monocytopenia, and immune dysregulation. J Allergy Clin Immunol. (2018) 141:2234–48. doi: 10.1016/j.jaci.2017.08.044
19. Hambleton S, Salem S, Bustamante J, Bigley V, Boisson-Dupuis S, Azevedo J, et al. IRF8 mutations and human dendritic-cell immunodeficiency. N Engl J Med. (2011) 365:127–38. doi: 10.1056/NEJMoa1100066
20. Kong XF, Martinez-Barricarte R, Kennedy J, Mele F, Lazarov T, Deenick EK, et al. Disruption of an antimycobacterial circuit between dendritic and helper T cells in human SPPL2a deficiency. Nat Immunol. (2018) 19:973–85. doi: 10.1038/s41590-018-0178-z
21. Dotta L, Notarangelo LD, Moratto D, Kumar R, Porta F, Soresina A, et al. Long-term outcome of whim syndrome in 18 patients: high risk of lung disease and HPV-related malignancies. J Allergy Clin Immunol Pract. (2019) 7:1568–77. doi: 10.1016/j.jaip.2019.01.045
22. Bigley V, arge D, Collin M. Dendritic cell analysis in primary immunodeficiency. Curr Opin Allergy Clin Immunol. (2016) 16:530–40. doi: 10.1097/ACI.0000000000000322
23. Caux C, Massacrier C, Vanbervliet B, Dubois B, Kooten C Van, Durand I, et al. Activation of human dendritic cells through CD40 cross-linking. J Exp Med. (1994) 180:1263–72. doi: 10.1084/jem.180.4.1263
24. de Jong EC, Smits HH, Kapsenberg ML. Dendritic cell-mediated T cell polarization. Springer Semin Immunopathol. (2005) 26:289–307. doi: 10.1007/s00281-004-0167-1
25. Behrens G, Li M, Smith CM, Belz GT, Mintern J, Carbone FR, et al. Helper T cells, dendritic cells and CTL Immunity. Immunol Cell Biol. (2004) 82:84–90. doi: 10.1111/j.1440-1711.2004.01211.x
26. Mempel TR, Henrickson SE, Von Andrian UH. T-cell priming by dendritic cells in lymph nodes occurs in three distinct phases. Nature. (2004) 427:154–9. doi: 10.1038/nature02238
27. Miro F, Nobile C, Blanchard N, Lind M, Filipe-Santos O, Fieschi C, et al. T cell-dependent activation of dendritic cells requires IL-12 and IFN-γ signaling in T cells. J Immunol. (2006) 177:3625–34. doi: 10.4049/jimmunol.177.6.3625
28. Afkarian M, Sedy JR, Yang J, Jacobson NG, Cereb N, Yang SY, et al. T-bet is a STATI-induced regulator for IL-12R expression in naïve CD4 + T cells. Nat Immunol. (2002) 3:549–57. doi: 10.1038/ni794
29. Finke JS, Shodell M, Shah K, Siegal FP, Steinman RM. Dendritic cell numbers in the blood of HIV-1 infected patients before and after changes in antiretroviral therapy. J Clin Immunol. (2004) 24:647–52. doi: 10.1007/s10875-004-6250-5
30. Pichyangkul S, Endy TP, Kalayanarooj S, Nisalak A, Yongvanitchit K, Green S, et al. A blunted blood plasmacytoid dendritic cell response to an acute systemic viral infection is associated with increased disease severity. J Immunol. (2003) 171:5571–8. doi: 10.4049/jimmunol.171.10.5571
Keywords: partial IFNγR1 deficiency, IFNγ, dendritic cells deficiency, mycobacteria, MSMD
Citation: Dotta L, Vairo D, Giacomelli M, Moratto D, Tamassia N, Vermi W, Lonardi S, Casanova J-L, Bustamante J, Giliani S and Badolato R (2020) Transient Decrease of Circulating and Tissular Dendritic Cells in Patients With Mycobacterial Disease and With Partial Dominant IFNγR1 Deficiency. Front. Immunol. 11:1161. doi: 10.3389/fimmu.2020.01161
Received: 01 April 2020; Accepted: 12 May 2020;
Published: 26 June 2020.
Edited by:
Satoshi Okada, Hiroshima University, JapanReviewed by:
Kunihiko Moriya, Tohoku University, JapanCarolina Prando, Pelé Pequeno Príncipe Research Institute, Brazil
Copyright © 2020 Dotta, Vairo, Giacomelli, Moratto, Tamassia, Vermi, Lonardi, Casanova, Bustamante, Giliani and Badolato. This is an open-access article distributed under the terms of the Creative Commons Attribution License (CC BY). The use, distribution or reproduction in other forums is permitted, provided the original author(s) and the copyright owner(s) are credited and that the original publication in this journal is cited, in accordance with accepted academic practice. No use, distribution or reproduction is permitted which does not comply with these terms.
*Correspondence: Laura Dotta, bGF1cmFkb3R0YUBpY2xvdWQuY29t
†These authors have contributed equally to this work