- Mechanisms of Peripheral Tolerance Unit, San Raffaele Telethon Institute for Gene Therapy (SR-Tiget), IRCCS San Raffaele Scientific Institute, Milan, Italy
The non-classical HLA-G is a well-known immune-modulatory molecule. In physiological condition, HLA-G surface expression is restricted to the maternal–fetal interface and to immune-privileged adult tissues, whereas soluble forms of HLA-G are detectable in various body fluids. HLA-G can be de novo expressed in pathological conditions including tumors, chronic infections, or after allogeneic transplantation. HLA-G exerts positive effects modulating innate and adaptive immune responses and promoting tolerance, or detrimental effects inducing immune escape mechanisms. HLA-G locus, in contrast to classical HLA class I gene, is highly polymorphic in the non-coding 3′ untranslated region (UTR) and in the 5′ upstream regulatory region (5′ URR). Variability in these regions influences HLA-G expression by modifying mRNA stability or allowing posttranscriptional regulation in the case of 3′ UTR or by sensing the microenvironment and responding to specific stimuli in the case of HLA-G promoter regions (5′ URR). The influence of genetic variations on the expression of HLA-G makes it an attractive biomarker to monitor disease predisposition and progression, or response to therapy. Here, we summarize the current knowledge, efforts, and obstacles to generate a general consensus on the correlation between HLA-G genetic variability, protein expression, and disease predisposition. Moreover, we discuss perspectives for future investigation on HLA-G genotype/expression in association with disease predisposition and progression.
Introduction
HLA-G, a non-classical HLA class I molecule, was first described to play a critical role in maintaining fetal–maternal tolerance (1). Later, it has been shown that HLA-G modulates innate and adaptive immune responses and promotes tolerance in different clinical settings. HLA-G function is favorable during pregnancy or after transplantation since it protects from rejection, and in autoimmune disease as it prevents autoreactive responses, or it is detrimental when expressed by tumors or during chronic infections, inducing immune escape mechanisms (2).
Because the HLA-G gene has a limited number of polymorphisms within the coding region, relatively few distinct molecules are coded. Nevertheless, seven different HLA-G isoforms have been described: four membrane-bound (HLA-G1 to -G4), and three soluble (HLA-G5 to -G7) (3–5).
The magnitude of HLA-G gene and protein expression is controlled by polymorphisms in the promoter [5′-upstream regulatory region (5′ URR)] and in the 3′ untranslated region (3′ UTR), and several association studies between these polymorphic sites and disease predisposition, response to therapy, and/or HLA-G protein expression have been reported. However, results from these studies often have been weak and inconclusive (6–10). Here, we summarize efforts to generate a general consensus on the correlation between HLA-G genetic variability, protein expression, and disease predisposition. Moreover, we highlight and discuss limits hampering the possibility to define a unique framework in the correlation between HLA-G genetic and disease predisposition or HLA-G genetic and protein expression, or HLA-G genetic/protein expression and disease predisposition.
HLA-G Haplotypes
The HLA-G gene has 74 alleles encoding for 24 different full-length proteins, and four null alleles encoding for truncated form of the protein (IPD78/IMGT/HLA; March 2020). HLA-G locus, similar to other classical HLA class I locus, is composed of eight exons and seven introns, but it presents a stop codon in exon 6, resulting in a short cytoplasmic tail (4) and in an extended 3′ UTR, mainly composed by the exon 8 (11). Since HLA-G discovery in 1987 (12), HLA-G locus has been accurately analyzed, and the variability detected at the HLA-G regulatory regions (e.g., 3′ UTR and 5′ URR) is relatively higher than that observed in the coding region (13, 14).
The first identified and most studied polymorphism of the HLA-G locus is a 14-base-pair insertion/deletion (14-bp INS/DEL) landing in the 3′ UTR (15). More detailed and large genetic studies identified 16 additional single-nucleotide polymorphisms (SNPs) in the HLA-G 3′ UTR, of which only nine—the 14-bp INS/DEL polymorphism, +3003 C/G, +3010 G/C, +3027 C/A, +3035 C/T, +3142 C/G, +3187 A/G, +3196 C/G, and +3227 G/A—were recognized as true polymorphisms (13). The discovery that some of these polymorphisms are in strong linkage disequilibrium allowed the identification of 41 3′ UTR haplotypes, designated from UTR-1 to UTR-41, with only nine UTRs accounting for more than 95% of all haplotypes worldwide (13, 14, 16, 17).
The HLA-G locus presents also several variations in the 5′ URR and in the coding region. SNPs in these regions are in linkage disequilibrium, and a limited number of haplotypes, clustering in few families, have been identified and studied, alone or in combination with 3′ UTR alleles (18–20). In detail, the analysis of 35 SNPs within the 5′ URR revealed 64 different haplotypes (named PROMO), of which only nine representing the 95% of alleles worldwide, clustering in four major groups (PROMO 010101, 010102, 0103, and 010104) (13, 20, 21). Similarly, 81 variations were identified in the coding region, the majority landing in introns, arranging in 93 different haplotypes, of which only 11 having a frequency higher than 1% (13), including a null allele G*0105N encoding for a non-functional protein (22, 23). Interestingly, when the 5′ URR, the coding region, and the 3′ UTR haplotypes have been combined in the “extended haplotypes,” it was clear that, also in this case, among the 200 haplotypes identified, the majority of them was scarcely represented (13). Moreover, 5′ URR, coding region, and 3′ UTR haplotypes are in linkage disequilibrium; thus, a given PROMO haplotype is preferentially associated with one specific 3′ UTR and coding region haplotype (11, 20, 21).
HLA-G Genetic Footprint and Correlation With Disease Course
HLA-G protein levels can be associated with specific genotypes; thus, a number of studies have been performed trying to correlate HLA-G haplotypes with disease susceptibility and morbidity or to use them as a predictive factor for response to therapy or in transplantation outcome (24).
The most studied HLA-G variation is the 14-bp INS/DEL polymorphism. In the field of pregnancy, there is a quite good consensus on the association of the 14-bp INS/INS genotype with recurrent pregnancy loss (RPL); however, a number of caveats have been identified in these correlations, including the heterogeneity of the studies, the sample characteristics, and non-comparable measure of the genotypes (23–25). A recent meta-analysis considering only women of European countries not only corroborated the association of 14-bp INS/INS genotype and RPL, but also highlighted and confirmed that the discrepancies observed in previous studies could be due to ethnic diversity of the cohort analyzed (26).
In autoimmunity and cancer, results on the association of 14-bp INS/DEL genotypes with disease development or with response to therapy reported contradictory and/or inconclusive results. In the context of type 1 diabetes (T1D), the analysis revealed the association of the 14-bp DEL/DEL genotype with the development and the early onset of the disease (27, 28). Conversely, in Crohn's disease, a high frequency of the 14-bp INS allele has been associated with an increased risk of early disease onset (29). A meta-analysis performed considering 11 case–control studies from different autoimmune diseases showed the absence of a direct correlation between 14-bp INS/DEL genotypes and susceptibility to autoimmune diseases, including systemic lupus erythematosus, rheumatoid arthritis multiple sclerosis, ulcerative colitis, Crohn's disease, idiopathic dilated cardiomyopathy, pemphigus vulgaris, and non-segmental vitiligo (7). The discrepancies observed might be attributed to the etiological mechanisms of the autoimmune disease in which gene-to-gene and gene-to-environment interactions are involved (see below), to the age of disease onset, and to the sample sizes.
A meta-analysis carried out to solve the 14-bp INS/DEL genotype association with cancer, including a large sample size, a wide variety of cancer types, and a more diverse sample population, overall revealed that HLA-G 14-bp INS/DEL polymorphism is significantly associated with the cancer susceptibility (30). However, these results are inconsistent with previous meta-analysis (8, 9, 24, 31), which concluded the absence of relationship between the HLA-G 14-bp INS/DEL polymorphism and the risk of cancer. Despite the positive correlation between 14-bp INS/DEL genotype and cancer susceptibility, some weaknesses of the latter study, linked to sample size, ethnicity, types of cancer, and sources of controls, have been reported. Indeed, stratified analysis accounting for the abovementioned variables failed to find a significant risk association (30).
In the context of allogeneic hematopoietic stem cell transplantation (HSCT), it has been shown that patients with better outcome carried 14-bp DEL/DEL and 14-bp INS/DEL genotypes, suggesting 14-bp INS/DEL genotype as potential biomarker of transplantation outcome (32, 33). Overall, the association between 14-bp INS/DEL genotypes and disease susceptibility provided some positive, but not conclusive, association. This might be attributed to a number of reasons, including high variability in the etiology of the diseases analyzed and to the relative limited number of patients enrolled in single correlation studies.
The discovery of 3′ UTR haplotypes and genotypes prompted investigators to reconcile the heterogeneity of the results obtained in studies on the association between 14-bp INS/DEL and diseases. We and other groups reported the protective role of specific UTRs in preventing RPL, with UTR-1, UTR-3, and UTR-4 present at low frequency in women with RPL (34–36). These studies indicate that analysis of 3′ UTR provided an improvement beyond the use of 14-bp INS/DEL genotypes in the association with pregnancy. UTR-1, UTR-3, and UTR-4 indeed contain 14-bp DEL, but they differ from other UTRs for additional specific SNPs (13, 14, 16, 17). Similar results were reported in T1D, showing that UTR-3 is present at low frequency in patients (37). In the context of allogeneic HSCT, only a weak association of the UTR-2, containing the 14-bp INS, with protection from acute graft-versus-host disease was reported, and the authors indicated that the study of the entire 3′ UTR, copamred to the sole analysis of the 14-bp INS/DEL, did not improve the prediction of transplant outcome (38).
Thus, far, few groups have investigated the impact of polymorphisms landing in the PROMO or the coding region of HLA-G with disease predisposition, and the most significant association has been found with the G*0105N null allele that is present with high frequency in RPL (19, 39, 40) and preeclampsia (41, 42). In celiac disease (CD), starting from the demonstration that 14-bp INS confers an increased risk factor for the disease in conjunction with the HLA-DQ2.5 (43), an extended analysis, including polymorphisms located in the PROMO and 3′ UTRs, showed the association of the haplotype containing PROMO 010102a and UTR-2 with CD susceptibility (44).
In conclusion, these results indicate that the correlation between HLA-G genotypes, and specifically those containing the 14-bp INS allele, and pregnancy loss or susceptibility to specific types of cancer has been achieved. Drawing conclusion on the association between HLA-G genotypes and susceptibility for other diseases is highly difficult because of the heterogeneity of the pathogenesis of the diseases analyzed, the age of disease onset, the cohorts of patients and controls used, and, in some cases, the limited number of case–control included in the study, disabling the statistical analysis.
Association HLA-G Genotype/Phenotype
Several groups integrated the knowledge on HLA-G genetic with the detection of the HLA-G protein, both as soluble (s) (HLA-G5 and shed-HLA-G1) and as membrane-bound (HLA-G1) form, with the aim to use HLA-G as biomarker of disease predisposition and progression, or response to therapy.
In 2001, Rebmann et al. (45) reported the first evidence that HLA-G genotype influences the amount of sHLA-G in circulation. The study performed in healthy individuals defined some alleles (G*01013 and G*0105N) being associated with low levels, and other (G*01041) with high levels of sHLA-G. Later, the identification of HLA-G 3′ UTR polymorphic sites prompted investigators to correlate HLA-G genetic variation at 3′ UTR and protein expression. The presence of the 14-bp INS allele has been associated with lower HLA-G production for most HLA-G5 and shed-HLA-G1 in plasma or serum, and HLA-G1 in trophoblast samples [reviewed in Carosella et al. (2)]. However, the presence of the 14-bp sequence leads to alternative splicing and the generation of a more stable mRNA associated with high HLA-G1 expression in trophoblast cell lines (46).
Correlation studies performed in autoinflammatory and autoimmune diseases revealed that the 14-bp INS allele was associated with low plasma levels of sHLA-G in Crohn's disease patients (29). In relapsing–remitting multiple sclerosis, the 14-bp DEL/DEL genotype correlated to high levels of HLA-G in cerebrospinal fluid; however, it did not associate with disease duration and clinical symptoms (47). On the same line, in chronic lymphocytic leukemia, the 14-bp DEL allele has been associated with high levels of sHLA-G and HLA-G1, but only sHLA-G correlated with patient survival, possibly as a consequence of the high metalloproteinase activity involved in shedding HLA-G1 (48). A comprehensive analysis, performed to correlate HLA-G genotype/phenotype and disease outcome, showed a link between the 14-bp INS/INS genotype with pretransplant low sHLA-G levels and severe adverse events after HSCT (49). Similarly, the 14-bp DEL haplotype correlated with high sHLA-G serum levels and reduced episodes of heart transplant rejection (50). Despite the variety of the disease investigated, 14-bp INS and DEL alleles have been confirmed to be associated with low and high HLA-G, respectively, and in most cases high HLA-G with positive outcome of the disease.
More recently, investigators have studied the correlation of HLA-G 3′ UTR and protein expression. Haplotypes and diplotypes containing UTR-1 have been associated with high levels of plasma sHLA-G, those containing UTR-5 and UTR-7 with low levels of sHLA-G, and finally, alleles containing UTR-2, UTR-3, UTR-4, and UTR-6 with medium levels of sHLA-G (51). These results were confirmed in other biological fluids as the highest levels of HLA-G in seminal plasma have been detected in the presence of homozygosis for UTR-1 and UTR-3 (52). Our group defined the association of specific UTRs with the expression of HLA-G1 in a specific subset of tolerogenic DC, termed DC-10, inducible in vitro in the presence of IL-10 (53) and present in vivo (54). We showed higher frequency of UTR-2, UTR-5, and UTR-7 haplotypes and diplotypes in donors with DC-10 expressing low HLA-G1 and of UTR-3 in donors expressing high HLA-G1 (55). More recently, we confirmed that the UTR-3 haplotype is associated with high levels of HLA-G1 on circulating DC-10 (Amodio et al., submitted).
In conclusion, these results indicate a general consensus on the association between 14-bp INS and DEL allele and low and high expression of HLA-G, either soluble or membrane-bound isoforms, respectively. However, the 14-bp INS allele encodes for a transcript with a 92-bp deletion leading to a more stable mRNA fragment than that generated by the 14-bp DEL (56), suggesting that 14-bp INS might be also associated with high levels of HLA-G expression. Correlation studies including additional variations in the 3′ UTR improved the correlation between HLA-G genetic and protein expression partially solving the mRNA stability issue. Moreover, HLA-G protein expression is driven by genetic variations in the 3′ UTRs, but also by those landing in the promoter region; thus, variability of the microenvironment associated with specific disease could affect the HLA-G protein expression.
Intracellular and Extracellular Mechanisms Regulating HLA-G Expression
Genetic variations in the 3′ UTR, which contain several target sites for microRNAs (miRNAs), regulate at post-transcriptional level the HLA-G expression. Being miRNA cell-specific, this regulation may affect the expression of HLA-G at cell and tissue levels. Six miRNAs have been reported to regulate HLA-G expression: miR-148a, miR-148b, miR-152, miR-133a, miR-628-5p, and miR-548q (57). The direct effect of these miRNAs in HLA-G protein expression has been mainly demonstrated in vitro, using cell lines (Figure 1). However, several indirect evidences prompted investigators to correlate the presence of specific miRNA with HLA-G protein expression in vivo. In placenta, miR-148a and miR-152 are poorly expressed, whereas the HLA-G mRNA levels are high (58). Since miR-148a and miR-152 down-regulate HLA-G1 protein expression in cell lines, a possible inverse relationship between these molecules in placenta has been postulated (58). Similarly, miR-133 reduced HLA-G protein expression in trophoblast cell lines (59), and its low expression in primary colorectal cancer samples, in which the HLA-G levels are high (60), suggested a possible inverse correlation of these molecules. As anticipated avobe, thus far direct evidence of the role of specific miRNA on the expression of HLA-G in vivo is scanty.
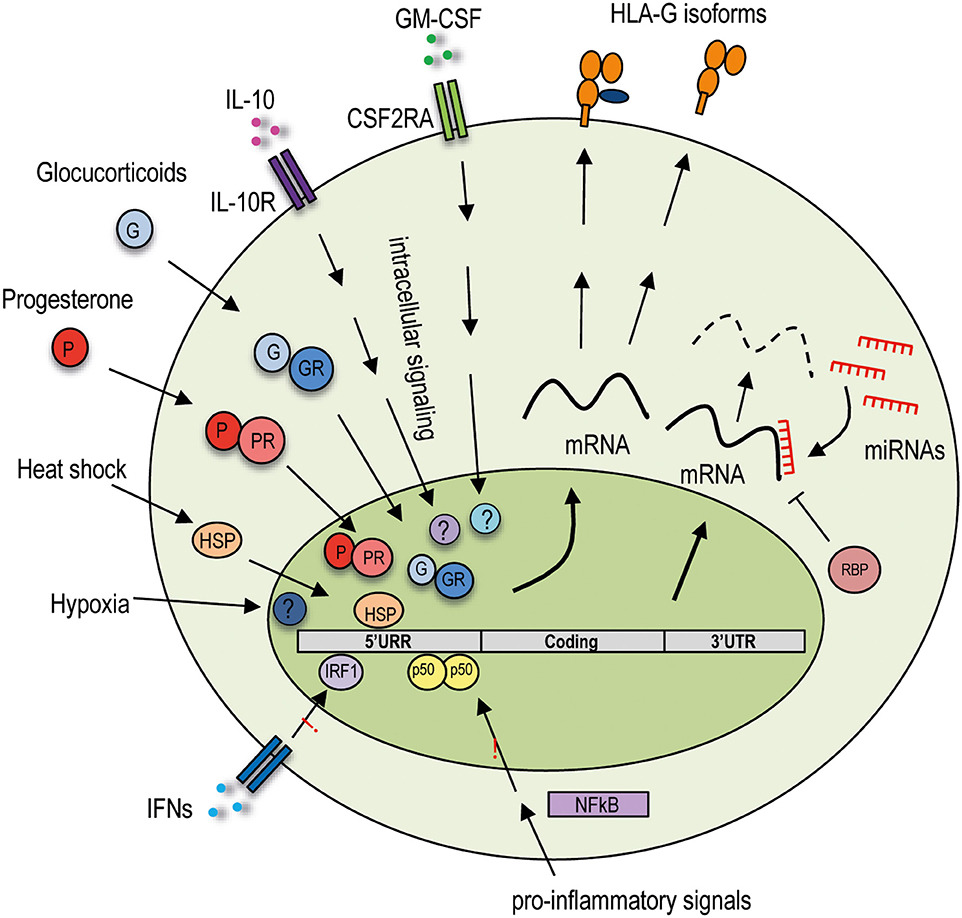
Figure 1. Extracellular and intracellular regulatory mechanisms of HLA-G expression. Variability in the HLA-G promoter region influences HLA-G expression by sensing and responding to the extracellular signals. Variations in the 3′ UTR region may modify mRNA stability or allow posttranscriptional regulation. HLA-G is not responsive to proinflammatory signals acting on the NF-κB pathway and to IFN-mediated stimulation. The HLA-G promoter region is unique among the HLA class I genes as it interacts with specific transcription factors activated by extracellular stimuli induced by hypoxia and heat shock, hormones such as glucocorticoids and progesterone, and cytokines including IL-10 and GM-CSF. HLA-G expression is posttranscriptionally regulated by genetic variations in the 3′ UTR, which contain several target sites for miRNAs and can bind specific RNA-binding proteins. These different regulations concur in the induction or inhibition of the expression of the HLA-G protein, which by alternative splicing of the mRNA can be produced in different isoforms: membrane-bound or soluble. 5′ URR, 5′ upstream regulatory region; 3′ UTR, 3′ untranslated region; CSF2RA, colony-stimulating factor 2 receptor subunit alpha; IL-10R, IL-10 receptor; IFNs, interferons; GR, glucocorticoid receptor; PR, progesterone receptor; HSP, heat shock protein, IRF-1, interferon regulatory factor 1; NF-κB, nuclear factor κ-light-chain-enhancer of activated B cells; RBP, RNA-binding proteins; miRNAs, microRNAs.
An additional layer of posttranscriptional regulation of HLA-G protein expression is mediated by a specific RNA-binding protein (RBP) (Figure 1), the heterogeneous nuclear ribonucleoprotein R (HNRNPR), which binds the 3′ UTR of the transcripts, stabilizes them, and allows HLA-G1 expression in transduced cell lines (61). More recently, a distinct and unique region in the 3′ UTR of HLA-G has been identified, but neither miRNAs nor RBPs seem to bind to this site and to control HLA-G1 expression in cell lines (62). Thus, additional studies are warranted to define the relevance of this sequence into the regulation of HLA-G protein expression in vivo.
The HLA-G protein expression is regulated not only by allelic variability and posttranscriptional regulation, but also by specific regulatory regions present in the HLA-G promoter. Nucleotide variability in the promoter region may indeed influence HLA-G protein levels by modifying transcription factor–binding affinity. In the HLA-G locus, the enhancer A (EnhA) region, which allows the interaction with nuclear factor k-light-chain-ehnancer of activated B cells (NF-κB) family of transcription factors, binds only p50/p50 homodimers (63). Moreover, the binding of interferon regulatory factor 1 and 2 (IRF-1 and IRF-2) to interferon-stimulated response element in the HLA-G promoter is not present in HLA-G (64, 65). The presence of this unique HLA-G promoter region indicates that HLA-G expression is not influenced by NF-κB or by interferon α (IFN-α), IFN-β, and IFN-γ (Figure 1).
Despite the unresponsiveness to interferons, HLA-G transcriptional rate is increased by a number of anti-inflammatory cytokine and mediators, as the HLA-G promoter region presents specific regulatory elements. The presence in the HLA-G promoter of a heat shock element allows response to heat shock proteins (HSP) (Figure 1), essential for regulating the state of intracellular folding, assembly, and translocation of proteins, potent modulators of the immune responses, and necessary for placental development. HLA-G transcription is induced upon heat shock in tumor cell lines, via the heat shock transcription factor 1; however, the consequent HLA-G protein expression has not been investigated (66).
Glucocorticoids (dexamethasone) and progesterone, a hormone fundamental for endometrium maintenance and embryo implantation, increase the secretion of soluble HLA-G5 and HLA-G6 by trophoblasts (67–69). The molecular mechanism underlying this protein expression was proposed to be induced via the interaction of progesterone receptor complex binding to a unique progesterone response element (PRE) sequence present in the HLA-G promoter (13) (Figure 1). Classical HLA class I genes have neither a classical PRE nor the unique PRE identified in HLA-G, thus suggesting that progesterone may be involved in cell-specific regulation of the HLA-G protein expression (70, 71).
Hypoxia modulates different processes, and it is associated with induction of HLA-G. Low oxygen increases HLA-G mRNA expression in trophoblasts (72) and in tumor cell lines (73), but its effect on the expression of HLA-G protein is still undefined.
Finally, the HLA-G protein expression can be modulated by granulocyte–macrophage colony-stimulating factor (GM-CSF) in cell lines (74) and by IL-10 in monocytes (75) and DC-10 (53, 55). Although the mechanisms underlying the induction of HLA-G protein expression by the above cytokines have not been completely elucidated (Figure 1), these evidences support the role of HLA-G in promoting an anti-inflammatory and in inducting a protolerogenic microenvironment.
Overall, HLA-G protein expression is driven by specific alleles, and it is regulated by intracellular and extracellular signals. Despite results obtained in cell lines, only putative correlations between the presence of specific miRNAs and HLA-G protein levels in vivo have been suggested. Moreover, is has to be taken into account that the microenvironment (e.g., the presence of specific molecules, hormones and/or cytokines) might affect HLA-G protein expression. These considerations are particularly important when HLA-G genotype/protein association is studied in specific diseases.
Concluding Remarks
The discovery that HLA-G genetic variants represent target of gene expression regulation led to intensive research for the identification of HLA-G genetic association with disease predisposition or progression and HLA-G protein expression. Despite several good genotype/protein correlations have been reported using in vitro model, when these observations have been translated in disease setting, the diagnostic/prognostic relevance of these findings in some cases appeared weak. A number of issue should be considered: (i) in the majority of the studies, conclusions have been based on results from small patient cohorts and in subjects from different ethnicity; (ii) only few studies performed a complete assessment of HLA-G genotyping, protein expression, and diseases outcome; (iii) the mechanisms regulating HLA-G expression can be distinctly active in different diseases; (iv) excluding the well-studied 14-bp INS/DEL polymorphism, there is a high heterogeneity of the genetic variations investigated, hindering the possibility to claim univocal conclusions.
The specific intracellular signaling and the microenvironment characterizing a given disease have to be considered for a proper selection of the polymorphisms to be investigated. As an example, the use of the entire 3′ UTR could be relevant, but other regulatory elements (e.g., the expression of miRNAs) should be investigated in parallel, to have a comprehensive picture; otherwise, the analysis of 14-bp INS/DEL polymorphism could be sufficiently informative. Indeed, the expression of miRNAs can vary among different pathological conditions and in different cells, thus affecting the expression of HLA-G if present. Similarly, the observation that haplotypes comprising 3′ UTR, coding regions and PROMO are mainly defined by the 3′ UTR region suggested that the analysis of PROMO and coding regions of HLA-G gene should be considered for improving a better correlation between HLA-G genetic and disease predisposition if disease-specific mediators are also analyzed in parallel; otherwise, the sole analysis of the 3′ UTR might be sufficient. Finally, in several studies, the presence of HLA-G has been evaluated at mRNA but not at protein levels, being the most reliable indicator of activity. Another important aspect to consider in the analysis HLA-G proteins is the selection of the isoform, the site or cell population to be evaluated. The recent discovery in renal carcinoma cells of new HLA-G transcripts, characterized by previously not described intron retention or exon skipping events, which cannot be recognized by the available antibodies (76) increases the complexity in the field. However, whether these transcripts encode for novel isoforms, are specifically produced by cancer cells, or have regulatory functions, remain to be defined. Based on these premises, it can be envisaged that a more sophisticated selection of the parameters to be investigated is critically important to improve the HLA-G genotype/expression/disease association studies. We propose that the following steps should be taken into account: the selection of the HLA-G genetic variations (e.g., 14-bp INS/DEL or 3′ UTRs, the coding region and/or the PROMO), which might depend on the type of diseases under consideration, on the tissue or the specific subset of cells analyzed; the possibility to analyze in parallel other specific parameters (e.g., the expression of miRNAs or RBP, soluble mediators including hormones or cytokines); the selection of the most relevant HLA-G isoform (e.g., soluble HLA-Gs or HLA-G1 or other isoforms) (Figure 2).
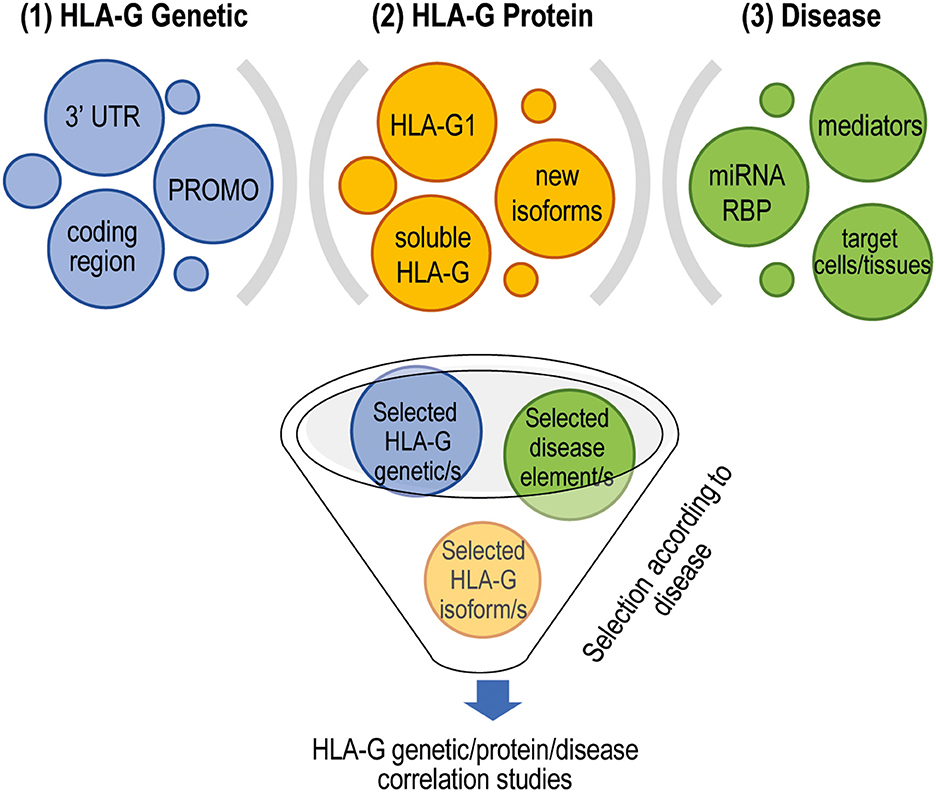
Figure 2. Proper selection of targets to perform HLA-G genetic/protein and disease development association studies. Different layers of complexity, both cell specific and disease specific, have to be taken into account to reliably define the role of HLA-G in disease development. (1) To select the HLA-G polymorphisms to include in the analysis; (2) to include in the analysis the evaluation of disease-specific features such as the expression of specific miRNAs or RBP and the presence of proinflammatory and anti-inflammatory mediators that can modulate the expression of HLA-G, and the target cells or tissues to study that can be affected by the presence of HLA-G; (3) to identify the most appropriate HLA-G isoforms to be investigated. 3′ UTR, 3′ untranslated region; PROMO, variations in the promoter region; miRNAs, microRNAs; RBP, RNA-binding proteins.
In conclusion, up to now a clear and univocal HLA-G genotype/expression/disease association has not been yet identified, with the exception for 14-bp IND/DEL allele. A more specific and “disease-oriented” analysis, meaning the selection of the relevant polymorphisms, isoforms, and regulatory factors that could impact on HLA-G expression, would be more helpful and affordable for better defining the interplay among HLA-G genetic variations, protein expression, and disease predisposition or response to therapy.
Data Availability Statement
The original contributions presented in the study are included in the article.
Author Contributions
GA wrote the manuscript. SG designed and wrote the manuscript. All authors read and approve the final manuscript.
Funding
This work was supported by research funding from the Italian Telethon Foundation (TGT16-21G01).
Conflict of Interest
The authors declare that the research was conducted in the absence of any commercial or financial relationships that could be construed as a potential conflict of interest.
References
1. Kovats S, Main EK, Librach S, Fisher SJ, DeMars. A class I antigen, HLA-G, expressed in human trophoblasts. Science. (1990) 248:220–3. doi: 10.1126/science.2326636
2. Carosella ED, Rouas-Freiss N, Roux DT Le, Moreau P, LeMaoult J. HLA-G. An immune checkpoint molecule. Adv Immunol. (2015) 127:33–144. doi: 10.1016/bs.ai.2015.04.001
3. Fujii T, Ishitani A, Geraghty DE. A soluble form of the HLA-G antigen is encoded by a messenger ribonucleic acid containing intron 4. J Immunol. (1994) 153:5516–24.
4. Ishitani A, Geraghty DE. Alternative splicing of HLA-G transcripts yields proteins with primary structures resembling both class I and class II antigens. Proc Natl Acad Sci U S A. (1992) 89:3947–51. doi: 10.1073/pnas.89.9.3947
5. Paul P, Adrian Cabestre F, Ibrahim EC, Lefebvre S, Khalil-Daher I, Vazeux G, et al. Identification of HLA-G7 as a new splice variant of the HLA-G mRNA and expression of soluble HLA-G5, -G6, and -G7 transcripts in human transfected cells. Hum Immunol. (2000) 61:1138–49. doi: 10.1016/S0198-8859(00)00197-X
6. Larsen MH, Hviid TVF. Human leukocyte antigen-G polymorphism in relation to expression, function, and disease. Hum Immunol. (2009) 70:1026–34. doi: 10.1016/j.humimm.2009.07.015
7. Kim SK, Jeong KH, Kang IJ, Chung JH, Shin MK, Lee MH. Relationship between the HLA-G 14bp insertion/deletion polymorphism and susceptibility to autoimmune disease: a meta-analysis. Genet Mol Res. (2015) 14:15839–47. doi: 10.4238/2015.December.1.35
8. Zhang S, Wang HT. Association between HLA-G 14-bp insertion/deletion polymorphism and cancer risk: a meta-analysis. J BUON. (2014) 19:567–72. Available online at: www.jbuon.com
9. Ge YZ, Ge Q, Li MH, Shi GM, Xu X, Xu LW, et al. Association between human leukocyte antigen-G 14-bp insertion/deletion polymorphism and cancer risk: a meta-analysis and systematic review. Hum Immunol. (2014) 75:827–32. doi: 10.1016/j.humimm.2014.06.004
10. Fan W, Li S, Huang Z, Chen Q. Relationship between HLA-G polymorphism and susceptibility to recurrent miscarriage: a meta-analysis of non-family-based studies. J Assist Reprod Genet. (2014) 31:173–84. doi: 10.1007/s10815-013-0155-2
11. Castelli EC, Mendes-Junior CT, Deghaide NHS, De Albuquerque RS, Muniz YCN, Simes RT, et al. The genetic structure of 3′untranslated region of the HLA-G gene: polymorphisms and haplotypes. Genes Immun. (2010) 11:134–41. doi: 10.1038/gene.2009.74
12. Geraghty DE, Koller BH, Orr HT. A human major histocompatibility complex class I gene that encodes a protein with a shortened cytoplasmic segment. Proc Natl Acad Sci U S A. (1987) 84:9145–9. doi: 10.1073/pnas.84.24.9145
13. Castelli EC, Ramalho J, Porto IOP, Lima THA, Felício LP, Sabbagh A, et al. Insights into HLA-G genetics provided by worldwide haplotype diversity. Front Immunol. (2014) 5:476. doi: 10.3389/fimmu.2014.00476
14. Sabbagh A, Luisi P, Castelli EC, Gineau L, Courtin D, Milet J, et al. Worldwide genetic variation at the 3′ untranslated region of the HLA-G gene: balancing selection influencing genetic diversity. Genes Immun. (2014) 15:95–106. doi: 10.1038/gene.2013.67
15. Harrison GA, Humphrey KE, Jakobsen IB, Cooper DW. A 14 bp deletion polymorphism in the HLA-G gene. Hum Mol Genet. (1993) 2:2200. doi: 10.1093/hmg/2.12.2200-a
16. Larsen MH, Hylenius S, Andersen AMN, Hviid TVF. The 3′-untranslated region of the HLA-G gene in relation to pre-eclampsia: revisited. Tissue Antigens. (2010) 75:253–61. doi: 10.1111/j.1399-0039.2009.01435.x
17. Castelli EC, Mendes CT, Donadi EA. HLA-G alleles and HLA-G 14 bp polymorphisms in a Brazilian population. Tissue Antigens. (2007) 70:62–68. doi: 10.1111/j.1399-0039.2007.00855.x
18. Hviid TVF, Sørensen S, Morling N. Polymorphism in the regulatory region located more than 1.1 Kilobases 5' to the start site of transcription, the promoter region, and exon 1 of the HLA-G gene. Hum Immunol. (1999) 60:1237–44. doi: 10.1016/S0198-8859(99)00130-5
19. Ober C, Aldrich CL, Chervoneva I, Billstrand C, Rahimov F, Gray HL, et al. Variation in the HLA-G promoter region influences miscarriage rates. Am J Hum Genet. (2003) 72:1425–35. doi: 10.1086/375501
20. Castelli EC, Mendes-Junior CT, Veiga-Castelli LC, Roger M, Moreau P, Donadi EA. A comprehensive study of polymorphic sites along the HLA-G gene: implication for gene regulation and evolution. Mol Biol Evol. (2011) 28:3069–86. doi: 10.1093/molbev/msr138
21. Tan Z, Shon AM, Ober C. Evidence of balancing selection at the HLA-G promoter region. Hum Mol Genet. (2005) 14:3619–28. doi: 10.1093/hmg/ddi389
22. Ober C, Aldrich C, Rosinsky B, Robertson A, Walker MA, Willadsen S, et al. HLA-G1 protein expression is not essential for fetal survival. Placenta. (1998) 19:127–32. doi: 10.1016/S0143-4004(98)90000-5
23. Le Discorde M, Le Danff C, Moreau P, Rouas-Freiss N, Carosella ED. HLA-G*0105N null allele encodes functional HLA-G isoforms1. Biol Reprod. (2005) 73:280–8. doi: 10.1095/biolreprod.104.037986
24. de Almeida BS, Muniz YCN, Prompt AH, Castelli EC, Mendes-Junior CT, Donadi EA. Genetic association between HLA-G 14-bp polymorphism and diseases: a systematic review and meta-analysis. Hum Immunol. (2018) 79:724–35. doi: 10.1016/j.humimm.2018.08.003
25. Meuleman T, Lashley LELO, Dekkers OM, van Lith JMM, Claas FHJ, Bloemenkamp KWM. HLA associations and HLA sharing in recurrent miscarriage: a systematic review and meta-analysis. Hum Immunol. (2015) 76:362–73. doi: 10.1016/j.humimm.2015.02.004
26. Monti M, Lupoli R, Sosa Fernandez LM, Cirillo F, Di Minno MND. Association of human leukocyte antigen-G 14 bp polymorphism with recurrent pregnancy loss in European countries: a meta-analysis of literature studies. Fertil Steril. (2019) 112:577–85.e3. doi: 10.1016/j.fertnstert.2019.05.003
27. Silva HPV, Ururahy MAG, Souza KSC, Loureiro MB, Oliveira YMC, Oliveira GHM, et al. The association between the HLA-G 14-bp insertion/deletion polymorphism and type 1 diabetes. Genes Immun. (2016) 17:13–18. doi: 10.1038/gene.2015.45
28. Gerasimou P, Skordis N, Picolos M, Spyridonidis A, Costeas P. HLA-G 14-bp polymorphism affects the age of onset in Type I diabetes mellitus. Int J Immunogenet. (2016) 43:135–42. doi: 10.1111/iji.12259
29. Zidi I, Yahia H, Ben Bortolotti D, Mouelhi L, Laaribi AB, Ayadi S, et al. Association between sHLA-G and HLA-G 14-bp deletion/insertion polymorphism in Crohn's disease. Int Immunol. (2015) 27:289–96. doi: 10.1093/intimm/dxv002
30. Jiang Y, Lu J, Wu YE, Zhao X, Li L. Genetic variation in the HLA-G 3UTR 14–bp insertion/deletion and the associated cancer risk: evidence from 25 case–control studies. Biosci Rep. (2019) 39:1–13. doi: 10.1042/BSR20181991
31. Li T, Huang H, Liao D, Ling H, Su B, Cai M. Genetic polymorphism in HLA-G 3′UTR 14-bp ins/del and risk of cancer: a meta-analysis of case–control study. Mol Genet Genomics. (2015) 290:1235–45. doi: 10.1007/s00438-014-0985-3
32. Chiusolo P, Bellesi S, Piccirillo N, Giammarco S, Marietti S, De Ritis D, et al. The role of HLA-G 14-bp polymorphism in allo-HSCT after short-term course MTX for GvHD prophylaxis. Bone Marrow Transplant. (2012) 47:120–24. doi: 10.1038/bmt.2011.40
33. Crocchiolo R, Ringden O, Bay JO, Blaise D, Omasic B, Mazzi B, et al. Impact of HLA-G polymorphism on the outcome of allogeneic hematopoietic stem cell transplantation for metastatic renal cell carcinoma. Bone Marrow Transplant. (2018) 53:213–18. doi: 10.1038/bmt.2017.243
34. Amodio G, Canti V, Maggio L, Rosa S, Castiglioni MT, Rovere-Querini P, et al. Association of genetic variants in the 3′UTR of HLA-G with recurrent pregnancy loss. Hum Immunol. (2016) 77:886–91. doi: 10.1016/j.humimm.2016.06.020
35. Michita RT, Zambra FMB, Fraga LR, Sanseverino MTV, Callegari-Jacques SM, Vianna P, et al. A tug-of-war between tolerance and rejection – new evidence for 3′UTR HLA-G haplotypes influence in recurrent pregnancy loss. Hum Immunol. (2016) 77:892–7. doi: 10.1016/j.humimm.2016.07.004
36. Meuleman T, Drabbels J, van Lith JMM, Dekkers OM, Rozemuller E, Cretu-Stancu M, et al. Lower frequency of the HLA-G UTR-4 haplotype in women with unexplained recurrent miscarriage. J Reprod Immunol. (2018) 126:46–52. doi: 10.1016/j.jri.2018.02.002
37. de Albuquerque RS, Mendes-Junior CT, Lucena-Silva N, da Silva CLL, Rassi DM, Veiga-Castelli LC, et al. Association of HLA-G 3' untranslated region variants with type 1 diabetes mellitus. Hum Immunol. (2016) 77:358–64. doi: 10.1016/j.humimm.2016.02.001
38. Sizzano F, Testi M, Zito L, Crocchiolo R, Troiano M, Mazzi B, et al. Genotypes and haplotypes in the 3′ untranslated region of the HLA-G gene and their association with clinical outcome of hematopoietic stem cell transplantation for beta-thalassemia. Tissue Antigens. (2012) 79:326–32. doi: 10.1111/j.1399-0039.2012.01862.x
39. Pfeiffer KA. The HLA-G genotype is potentially associated with idiopathic recurrent spontaneous abortion. Mol Hum Reprod. (2001) 7:373–8. doi: 10.1093/molehr/7.4.373
40. Aldrich CL. HLA-G genotypes and pregnancy outcome in couples with unexplained recurrent miscarriage. Mol Hum Reprod. (2001) 7:1167–72. doi: 10.1093/molehr/7.12.1167
41. Hylenius S, Andersen AMN, Melbye M, Hviid TVF. Association between HLA-G genotype and risk of pre-eclampsia: A case-control study using family triads. Mol Hum Reprod. (2004) 10:237–46. doi: 10.1093/molehr/gah035
42. Aldrich C, Verp MS, Walker MA, Ober C. A null mutation in HLA-G is not associated with preeclampsia or intrauterine growth retardation. J Reprod Immunol. (2000) 47:41–8. doi: 10.1016/S0165-0378(00)00052-8
43. Fabris A, Segat L, Catamo E, Morgutti M, Vendramin A, Crovella S. HLA-G 14 bp deletion/insertion polymorphism in celiac disease. Am J Gastroenterol. (2011) 106:139–44. doi: 10.1038/ajg.2010.340
44. Catamo E, Zupin L, Segat L, Celsi F, Crovella S. HLA-G and susceptibility to develop celiac disease. Hum Immunol. (2015) 76:36–41. doi: 10.1016/j.humimm.2014.12.006
45. Rebmann V, Van Der Ven K, Päßler M, Pfeiffer K, Krebs D, Grosse-Wilde H. Association of soluble HLA-G plasma levels with HLA-G alleles. Tissue Antigens. (2001) 57:15–21. doi: 10.1034/j.1399-0039.2001.057001015.x
46. Svendsen SG, Hantash BM, Zhao L, Faber C, Bzorek M, Nissen MH, et al. The expression and functional activity of membrane-bound human leukocyte antigen-G1 are influenced by the 3'-untranslated region. Hum Immunol. (2013) 74:818–27. doi: 10.1016/j.humimm.2013.03.003
47. Rizzo R, Bortolotti D, Fredj N, Ben Rotola A, Cura F, Castellazzi M, et al. Role of HLA-G 14bp deletion/insertion and +3142C>G polymorphisms in the production of sHLA-G molecules in relapsing-remitting multiple sclerosis. Hum Immunol. (2012) 73:1140–6. doi: 10.1016/j.humimm.2012.08.005
48. Rizzo R, Audrito V, Vacca P, Rossi D, Brusa D, Stignani M, et al. HLA-G is a component of the chronic lymphocytic leukemia escape repertoire to generate immune suppression: Impact of the HLA-G 14 base pair (rs66554220) polymorphism. Haematologica. (2014) 99:888–96. doi: 10.3324/haematol.2013.095281
49. Waterhouse M, Duque-Afonso J, Wäsch R, Bertz H, Finke J. Soluble HLA-G molecules and HLA-G 14-base pair polymorphism after allogeneic hematopoietic cell transplantation. Transplant Proc. (2013) 45:397–401. doi: 10.1016/j.transproceed.2012.05.073
50. Twito T, Joseph J, Mociornita A, Rao V, Ross H, Delgado DH. The 14-bp deletion in the HLA-G gene indicates a low risk for acute cellular rejection in heart transplant recipients. J Hear Lung Transplant. (2011) 30:778–82. doi: 10.1016/j.healun.2011.01.726
51. Martelli-Palomino G, Pancotto JA, Muniz YC, Mendes-Junior CT, Castelli EC, Massaro JD, et al. Polymorphic sites at the 3' untranslated region of the HLA-G gene are associated with differential hla-g soluble levels in the brazilian and french population. PLoS ONE. (2013) 8:e71742. doi: 10.1371/journal.pone.0071742
52. Craenmehr MHC, Haasnoot GW, Drabbels JJM, Spruyt-Gerritse MJ, Cao M, van der Keur C, et al. Soluble HLA-G levels in seminal plasma are associated with HLA-G 3′UTR genotypes and haplotypes. HLA. (2019) 94:339–46. doi: 10.1111/tan.13628
53. Gregori S, Tomasoni D, Pacciani V, Scirpoli M, Battaglia M, Magnani F, et al. Differentiation of type 1 T regulatory cells (Tr1) by tolerogenic DC-10 requires the IL-10 – dependent ILT4 / HLA-G pathway. Blood. (2010) 116:935–44. doi: 10.1182/blood-2009-07-234872
54. Comi M, Avancini D, Santoni de Sio F, Villa M, Uyeda MJ, Floris M, et al. Coexpression of CD163 and CD141 identifies human circulating IL-10-producing dendritic cells (DC-10). Cell Mol Immunol. (2019) 17:95–107. doi: 10.1038/s41423-019-0218-0
55. Amodio G, Comi M, Tomasoni D, Gianolini ME, Rizzo R, Lemaoult J, et al. HLA-G expression levels influence the tolerogenic activity of human DC-10. Haematologica. (2015) 100:548–57. doi: 10.3324/haematol.2014.113803
56. Rousseau P, Le Discorde M, Mouillot G, Marcou C, Carosella ED, Moreau P. The 14 bp deletion-insertion polymorphism in the 3′ UT region of the HLA-G gene influences HLA-G mRNA stability. Hum Immunol. (2003) 64:1005–10. doi: 10.1016/j.humimm.2003.08.347
57. Poras I, Yaghi L, Martelli-Palomino G, Mendes CT, Muniz YCN, Cagnin NF, et al. Haplotypes of the HLA-G 3′ untranslated region respond to endogenous factors of HLA-G+ and HLA-G- cell lines differentially. PLoS ONE. (2017) 12:e0169032. doi: 10.1371/journal.pone.0169032
58. Kalotra V, Lall M, Verma IC, Kaur A, Kaur A. The HLA-G 14 bp insertion/deletion polymorphism and its association with soluble HLA-G levels in women with recurrent miscarriages. HLA. (2018) 91:167–74. doi: 10.1111/tan.13198
59. Wang X, Li B, Wang J, Lei J, Liu C, Ma Y, et al. Evidence that miR-133a causes recurrent spontaneous abortion by reducing HLA-G expression. Reprod Biomed Online. (2012) 25:415–24. doi: 10.1016/j.rbmo.2012.06.022
60. Ye SR, Yang H, Li K, Dong DD, Lin XM, Yie SM. Human leukocyte antigen G expression: as a significant prognostic indicator for patients with colorectal cancer. Mod Pathol. (2007) 20:375–83. doi: 10.1038/modpathol.3800751
61. Reches A, Nachmani D, Berhani O, Duev-Cohen A, Shreibman D, Ophir Y, et al. HNRNPR Regulates the expression of classical and nonclassical MHC class I proteins. J Immunol. (2016) 196:4967–76. doi: 10.4049/jimmunol.1501550
62. Reches A, Berhani O, Mandelboim O. A unique regulation region in the 3′ UTR of HLA-G with a promising potential. Int J Mol Sci. (2020) 21:900. doi: 10.3390/ijms21030900
63. Gobin SJP, Van Den Elsen PJ. Transcriptional regulation of the MHC class Ib genes HLA-E, HLA-F and HLA-G. Hum Immunol. (2000) 61:1102–7. doi: 10.1016/S0198-8859(00)00198-1
64. Gobin SJ, van Zutphen M, Woltman AM, van den Elsen PJ. Transactivation of classical and nonclassical HLA class I genes through the IFN-stimulated response element. J Immunol. (1999) 163:1428–34. Available online at: http://www.ncbi.nlm.nih.gov/pubmed/10415043
65. Dias FC, Bertol BC, Poras I, Souto BM, Mendes-Junior CT, Castelli EC, et al. The genetic diversity within the 1.4 kb HLA-G 5′ upstream regulatory region moderately impacts on cellular microenvironment responses. Sci Rep. (2018) 8:5652. doi: 10.1038/s41598-018-24009-7
66. Ibrahim EC, Morange M, Dausset J, Carosella ED, Paul P. Heat shock and arsenite induce expression of the nonclassical class I histocompatibility HLA-G gene in tumor cell lines. Cell Stress Chaperones. (2000) 5:207–18. doi: 10.1379/1466-1268(2000)005<0207:HSAAIE>2.0.CO;2
67. Akhter A, Faridi RM, Das V, Pandey A, Naik S, Agrawal S. In vitro up-regulation of HLA-G using dexamethasone and hydrocortisone in first-trimester trophoblast cells of women experiencing recurrent miscarriage. Tissue Antigens. (2012) 80:126–35. doi: 10.1111/j.1399-0039.2012.01884.x
68. Moreau P, Faure O, Lefebvre S, Ibrahim EG, O'Brien M, Gourand L, et al. Glucocorticoid hormones upregulate levels of HLA-G transcripts in trophoblasts. Transplant Proc. (2001) 33:2277–80. doi: 10.1016/S0041-1345(01)01990-X
69. Yie SM, Li LH, Li GM, Xiao R, Librach CL. Progesterone enhances HLA-G gene expression in JEG-3 choriocarcinoma cells and human cytotrophoblasts in vitro. Hum Reprod. (2006) 21:46–51. doi: 10.1093/humrep/dei305
70. Yie SM, Xiao R, Librach CL. Progesterone regulates HLA-G gene expression through a novel progesterone response element. Hum Reprod. (2006) 21:2538–44. doi: 10.1093/humrep/del126
71. Ivanova-todorova E, Mourdjeva M, Kyurkchiev D, Bochev I, Stoyanova E, Dimitrov R, et al. HLA-G expression Is up-regulated by progesterone in mesenchymal stem cells. Am J Reprod Immunol. (2009) 62:25–33. doi: 10.1111/j.1600-0897.2009.00707.x
72. Nagamatsu T, Fujii T, Yamashita T, Miki A, Kanai T, Kusumi M, et al. Hypoxia does not reduce HLA-G expression on extravillous cytotrophoblasts. J Reprod Immunol. (2004) 63:85–95. doi: 10.1016/j.jri.2004.07.001
73. Mouillot G, Marcou C, Zidi I, Guillard C, Sangrouber D, Carosella ED, et al. Hypoxia modulates HLA-G gene expression in tumor cells. Hum Immunol. (2007) 68:277–85. doi: 10.1016/j.humimm.2006.10.016
74. Onno M, Le Friec G, Pangault C, Amiot L, Guilloux V, Drénou B, et al. Modulation of HLA-G antigens expression in myelomonocytic cells. Hum Immunol. (2000) 61:1086–94. doi: 10.1016/S0198-8859(00)00191-9
75. Moreau P, Adrian-Cabestre F, Menier C, Guiard V, Gourand L, Dausset J, et al. IL-10 selectively induces HLA-G expression in human trophoblasts and monocytes. Int Immunol. (1999) 11:803–11. doi: 10.1093/intimm/11.5.803
Keywords: HLA-G, immune regulation, autoimmune diseases, pregnancy, cancer, HLA-G polymorphisms
Citation: Amodio G and Gregori S (2020) HLA-G Genotype/Expression/Disease Association Studies: Success, Hurdles, and Perspectives. Front. Immunol. 11:1178. doi: 10.3389/fimmu.2020.01178
Received: 07 April 2020; Accepted: 13 May 2020;
Published: 08 July 2020.
Edited by:
Wei-Hua Yan, Wenzhou Medical University, ChinaReviewed by:
Tomomi Toubai, Yamagata University, JapanSandeep Kumar Dhanda, St. Jude Children's Research Hospital, United States
Copyright © 2020 Amodio and Gregori. This is an open-access article distributed under the terms of the Creative Commons Attribution License (CC BY). The use, distribution or reproduction in other forums is permitted, provided the original author(s) and the copyright owner(s) are credited and that the original publication in this journal is cited, in accordance with accepted academic practice. No use, distribution or reproduction is permitted which does not comply with these terms.
*Correspondence: Silvia Gregori, Z3JlZ29yaS5zaWx2aWFAaHNyLml0