- 1Division of Infectious Diseases, VA Greater Los Angeles Healthcare System, Los Angeles, CA, United States
- 2Departments of Medicine, and Microbiology, Immunology, and Molecular Genetics, David Geffen School of Medicine, University of California, Los Angeles, CA, United States
- 3Research Service, VA Greater Los Angeles Healthcare System, Los Angeles, CA, United States
- 4Department of Medicine, David Geffen School of Medicine, University of California, Los Angeles, CA, United States
The virulence mechanisms required for infection and evasion of immunity by pathogenic Leptospira species remain poorly understood. A number of L. interrogans surface proteins have been discovered, lying at the interface between the pathogen and host. Among these proteins, the functional properties of the Lig (leptospiral immunoglobulin-like domain) proteins have been examined most thoroughly. LigA, LigB, and LigC contain a series of, 13, 12, and 12 closely related domains, respectively, each containing a bacterial immunoglobulin (Big) -like fold. The multidomain region forms a mostly elongated structure that exposes a large surface area. Leptospires wield the Lig proteins to promote interactions with a range of specific host proteins, including those that aid evasion of innate immune mechanisms. These diverse binding events mediate adhesion of L. interrogans to the extracellular matrix, inhibit hemostasis, and inactivate key complement proteins. These interactions may help L. interrogans overcome the physical, hematological, and immunological barriers that would otherwise prevent the spirochete from establishing a systemic infection. Despite significant differences in the affinities of the LigA and LigB proteins for host targets, their functions overlap during lethal infection of hamsters; virulence is lost only when both ligA and ligB transcription is knocked down simultaneously. Lig proteins have been shown to be promising vaccine antigens through evaluation of a variety of different adjuvant strategies. This review serves to summarize current knowledge of Lig protein roles in virulence and immunity and to identify directions needed to better understand the precise functions of the Lig proteins during infection.
Introduction
Leptospiral immunoglobulin-like (Lig) domain proteins play key roles in the pathogenesis mechanisms of the spirochetes responsible for leptospirosis, an infectious disease that is re-emerging worldwide. The widespread dissemination of leptospires is related to their ability to colonize the renal tubules of a wide variety of mammalian hosts. Some mammals are reservoir hosts that do not exhibit signs of disease but remain colonized for extended periods of time, during which they shed the spirochetes in their urine and contaminate the surrounding environment. Leptospires may remain viable for extended periods of time in moist soil or freshwater until infecting a new host. Infection is initiated through penetration of skin abrasions and mucous membranes and widespread hematogenous dissemination. The ensuing host inflammatory response results in either clearance of infection, selective colonization of the renal tubules, or multiorgan system failure and death.
To date, 64 leptospiral species have been described, which are distributed into 4 clades: P1, P2, S1, and S2 (1). The clade designation is preferred to the older pathogen, intermediate, and saprophytic designations because the virulence status of many of these species is unknown. Clades P1 and P2 include species known to cause human infections (1). L. interrogans is the most prevalent cause of severe human infection and the subject of most studies on the molecular basis of leptospiral pathogenesis. We are only now beginning to unravel the mechanisms by which L. interrogans infects susceptible hosts and causes disease. Key to L. interrogans survival in the host during the early phases of infection is evasion of the innate immune response. Elimination of L. interrogans requires recognition of microbe-associated molecular patterns (MAMPs) by pattern recognition receptors (PRRs) including members of the Toll-like receptor (TLR) and NOD-like receptor (NLR) families (2). Leptospires must evade the bactericidal effects of complement (3), avoid becoming entrapped in fibrin mesh, and escape capture by neutrophil extracellular traps (NETs), phagocytic activity, and antimicrobial components (4). By the time the humoral immune response produces antibodies targeting L. interrogans, the spirochetes have disseminated to various organs including the kidneys, liver, and other organs and triggered the release of pro-inflammatory cytokines (5).
Among the potential leptospiral surface proteins that have been identified as adhesins based on in vitro adherence studies, the Lig proteins stand out in terms of the range of evidence supporting their role in virulence. Not only have the Lig proteins been found to adhere strongly to a surprising variety of host ligands, indicating a unique level of multifunctionality, gain of function adherence studies have been reported using the normally non-adherent saprophyte, L. biflexa (6–8). Some of the host ligands targeted by the Lig proteins indicate a role for the Lig proteins in evasion of innate immunity. The Lig proteins are strongly upregulated in response to host temperature and osmolarity conditions (9–11), indicating a role in infection of the mammalian host. Virulence is lost when transcription of both ligA and ligB is knocked down, indicating that expression of at least one lig gene is required for infection. The Lig proteins are some of the earliest proteins identified by the humoral immune response to leptospirosis and are among the strongest immunogens. Purified recombinant Lig proteins function as potent immunoprotective antigens in animal models of leptospirosis. This review will describe what is known about the role of the Lig family of proteins in the molecular pathogenesis of L. interrogans. Here we present an integrated model of the role of Lig proteins in leptospiral virulence and immunity. This is the first focused review on the Lig proteins that we are aware of.
Discovery and Description
The ligA gene was first described by Palaniappan et al. in an equine strain of L. interrogans serovar Pomona, subtype kennewicki (12). The gene was found by screening a bacteriophage expression library with serum from a mare after a leptospiral infection that had induced abortion. Sequencing of one positive clone revealed a large open reading frame encoding a protein with a calculated molecular mass of ~130 kDa. They noted a series of tandem 90 amino acid imperfect repeat domains, each predicted to fold into an immunoglobulin-like domain. This observation suggested a virulence function for LigA because the well-characterized bacterial adhesins Yersinia invasin and E. coli intimin also harbored multiple Ig-like domains. The remaining members of the lig gene family were described in a study published a year later (13) using pooled sera from leptospirosis patients to identify the ligA, ligB, and ligC genes in L. interrogans and L. kirschneri expression libraries.
Lipoprotein signal peptide sequences present at the amino termini of the Lig proteins suggested that these were exported proteins. Lipidation occurred during incubation of E. coli expressing LigA in tritiated palmitate (14). Export to the leptospiral surface has been demonstrated by whole cell and thin section immunoelectron microscopy (13). Surface exposure has been confirmed by susceptibility of LigA of intact L. interrogans to proteinase K digestion (14) and by immunofluorescence microscopy using LigB antiserum (10). Taken together, this evidence indicates that the Lig proteins are exported to the outer membrane in a lipidated form by the localization of lipoprotein (Lol) pathway and then translocated to the leptospiral surface (15).
Selective extracellular release of LigA but not LigB, into the culture supernatant was observed with L. kirschneri and L. interrogans (10, 11). Released LigA was in a truncated form, suggesting involvement of proteolysis in its release. Soluble LigA levels were minimal with L. kirschneri at exponential growth phase in EMJH (standard growth medium) and accumulated in stationary phase as cellular LigA levels decreased (10). With L. interrogans, levels of both cellular and soluble LigA increased considerably when sodium chloride was added to achieve the osmolarity of mammalian tissues. However, when the osmolarity was increased with sucrose or glucose, soluble LigA was not detected despite the increased levels of cellular LigA (11). This raises the possibility that glucose, a component of blood, inhibits proteolysis of LigA in the bloodstream. Soluble LigA may have immunomodulatory properties that aid survival of Leptospira during infection, as demonstrated with proteins released from the surface of other bacteria (16).
LigA, LigB, and LigC contain a series of 13, 12, and 12 immunoglobulin-like (Ig-like) fold domains, respectively, belonging to the Big_2 domain protein family PF02368 (Figure 1). Big_2 protein domains are widely distributed in nature including many bacterial species, including host cell binding proteins such as the E. coli intimin host cell-adhesion molecule (17) and phage tail proteins (18). The first six and part of the seventh Ig-like domains of LigA and LigB are nearly identical between these two proteins, while the remaining domains are unique to each protein. While all the Lig protein Ig-like domains belong to the Big_2 protein family and their sequences are related, they are not “repeats” and share only a moderate degree of amino acid sequence identity (Figure 2). Adjacent Ig-like domains are connected by three amino acid “linkers” that separate domains and may enable them to flex relative to one another (Figure 2). As shown in Figure 1, the 12 Ig-like domains of LigB are followed by two putative Big_5 domains that are part of a large carboxyterminal extension (CTE) of 772 amino acids that is not present in LigA. These two Big_5 domains are identified by a sequence search of the Pfam database version 33.1 (https://pfam.xfam.org/) (20) using the LigB CTE as the query sequence. LigC is organized similarly to LigB in that both proteins contain 12 Ig-like domains followed by two Big_5 domains as part of a large CTE. The amino acid sequence identity of the LigB and LigC CTEs is 51% (13).
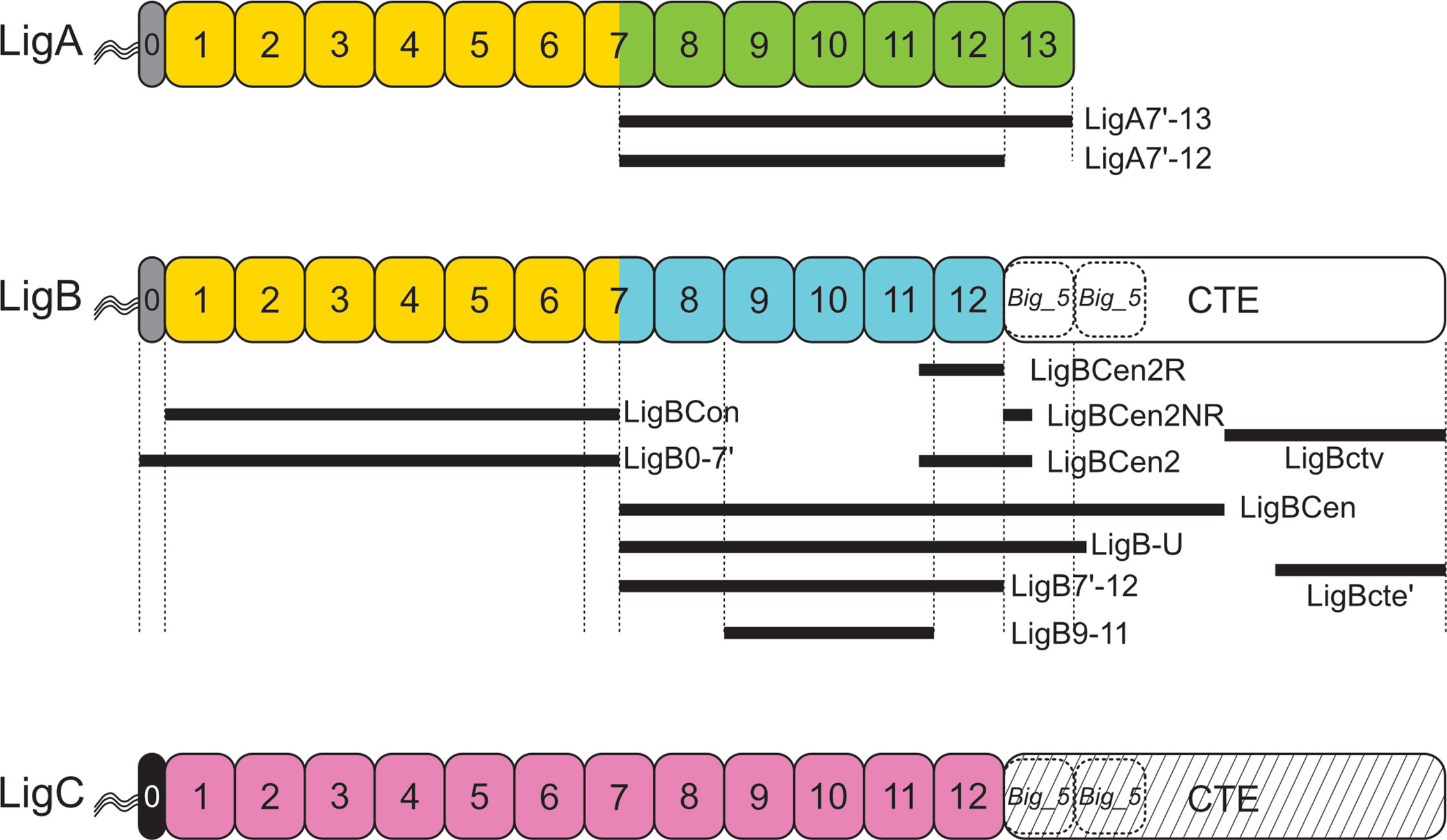
Figure 1 Leptospiral Immunoglobulin-Like (Lig) Protein Immunoglobulin-like Domain Structure. Domain structures of the three Lig proteins are shown. LigA, LigB, and LigC contain a series of 13, 12, and 12 Big_2 domains, respectively. The first six Big_2 domains and part of the seventh domain of LigA and LigB have a high percentage of sequence identity as indicated by yellow domains. The sequences of the remaining Big_2 domains differ between LigA (green) and LigB (blue). The Big_2 domains of LigC are shown in pink. The Big_2 domains of LigB and LigC are followed by a C-terminal extension (CTE) predicted to harbor Big_5 domains (dashed rectangle). The “0” segment between the amino terminus of the mature proteins and Big_2 domain 1 is indicated with gray or black shading. All three Lig proteins are thought to have triacylated amino-terminal cysteines. Lig fragments are shown with black lines, and their amino acid coordinates are: LigA7’-13 (631–1224), LigBCon (47-630), LigBCen (631-1417), LigBCen2R (1014-1124), LigBCen2NR (1120-1165), LigBCen2 (1014-1165), LigBCen2NR (1014-1124), LigBCen2NR (1120-1165), LigB7’-12 (631-1124), LigB-U (631-1257), LigBctv (1418-1889), LigBcte’ (1630-1890). Lig fragment nomenclature may differ from that used in the original studies.
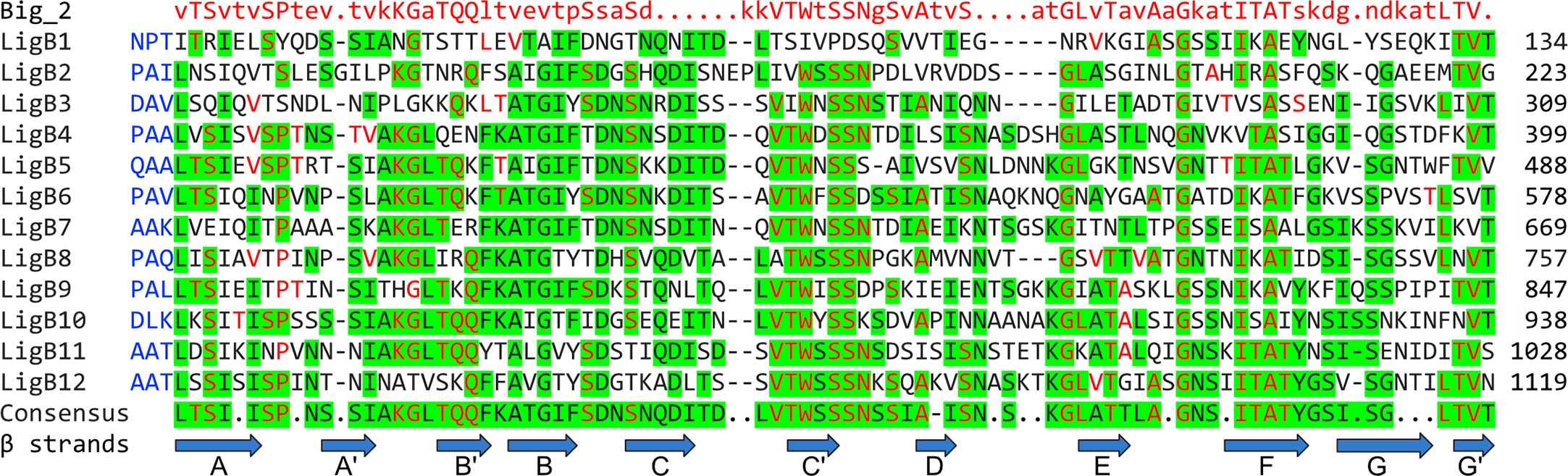
Figure 2 Leptospiral Immunoglobulin-Like B (LigB) Domain Alignment. Alignment of the 12 Big_2 domains of L. interrogans serovar Copenhageni strain Fiocruz L1-130 is shown, green highlights indicate regions of consensus among the domains. Red font indicates agreement with the Big_2 domain consensus sequence shown in the top row. Blue font indicates sequence of 3 amino acid linkers between LigB domains. Amino acid position of the terminal amino acid in each domain is provided on the right. Blue arrows indicate regions of beta sheet in the LigB12 solution structure (19).
The large CTEs of LigB and LigC are likely to be important for the structure and function of these proteins. A complete understanding of LigB structure and how the mature protein is integrated into the leptospiral outer membrane is lacking without a better understanding of the structure and function of the CTE.
Structure
The Lig protein domains feature the immunoglobulin-like fold, which was initially described for an Fab’ fragment of human immunoglobulin in 1973 (21). The subsequent discovery of the immunoglobulin fold in numerous proteins functionally unrelated to the immunoglobulins led to the description of the immunoglobulin superfamily, which is defined by its characteristic fold rather than its primary sequence (22). The classic Ig-fold comprises 70 to 100 amino acid residues divided into two stacked β sheets with a total of at least seven and as many as ten anti-parallel β strands (23). The Ig-fold is divided into subtypes based on their topology and sequence (24). A comparison of the first 52 known structures of the fold revealed that in most cases strands A, B, and E were located in the first β sheet and strands C, F, and G in the second sheet (24). Depending on the subtype, the first β sheet may also have strand D, and the second sheet may have one, two, or all of strands A’, C’, and C’’ (23). Ig-like domains within each subtype share a hydrophobic core comprising several hydrophobic residues that have their side chains directed toward the interior of the domain, primarily from strands B, C, E, and F. These proteins are found in all domains of life and both inside and outside the cell (24).
The solution structures of domains LigA4 and LigB12 were solved by NMR (19, 25, 26). Both domains adopt a similar Ig-like fold; however, their structures differ from the classic two-layer sandwich described for other Ig-like domains, such as the D2 domain of invasin from Yersinia pseudotuberculosis (Figure 3A) (27). Figure 3A shows the structure of LigB12. The Ig-like fold of both LigA4 and LigB 12 consists of 10 β-strands divided into one large β sheet, sheet 2 (β strands C’, F, and G), and two smaller sheets, 1a (strands A, B, and C) and 1b (strands B’, D, and E), with β strand B/B’ split between sheets 1a and 1b (Figure 3B). β sheets 1b and 2 form the β sandwich, while sheet 1a extends beyond the rest of the domain to leave both of its surfaces solvent-exposed (Figure 3A). The residues directed toward the hydrophobic core are highly conserved among the Lig Ig-like domains (26). The Ig-like domains of LigA and LigB lack the cysteines that connect the β sheets of some Ig domains with a disulfide bridge to make the core more compact (26).
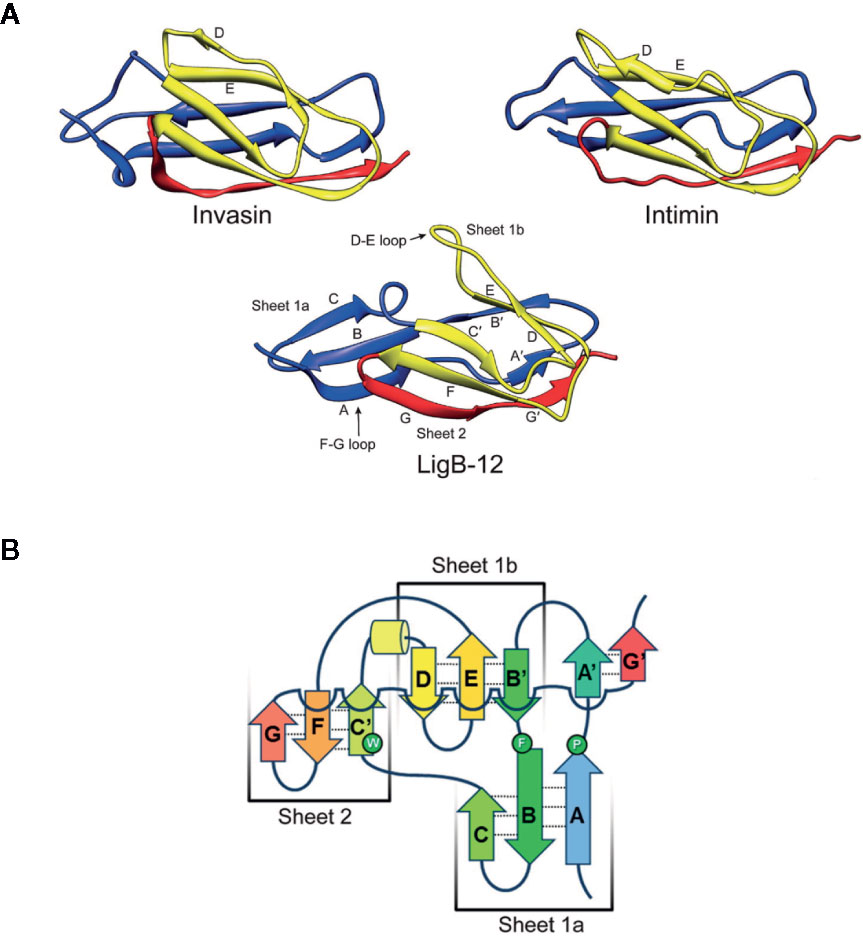
Figure 3 Comparison of Leptospiral Immunoglobulin-Like B (LigB) Domain 12 and Invasin Domain 2 Structures. (A) The most stable NMR solution structures of LigB12 (PDB entry: 2MOG) from L. interrogans serovar Pomona type kennewicki strain JEN4 (26), and the D2 domain of invasion (PDB entry: 1CWV) from Yersinia pseudotuberculosis (27) are presented showing the immunoglobulin-like fold. LigB12 is composed of a β sandwich with ten β strands distributed over the two layers, with the more extensive divided into two smaller sheets. Invasin is composed of a β sandwich with ten β strands in two sheets. Note the strands extending from the amino- and carboxy-termini of the domain, which serve as linkages between domains. The images were obtained from the RCSB Protein Data Bank (28). (B) The secondary structure of LigB12 is represented as a topology diagram (26). Images from Ptak et al., 2014 (https://pubs.acs.org/doi/10.1021/bi500669u) (26), with permission from the American Chemical Society.
To obtain the low-resolution structure of the 12 LigB domains in series, a series of LigB polypeptides comprising a sliding window of five consecutive Ig-like domains covering all 12 LigB Ig-like domains were subjected to small angle X-ray scattering (SAXS) (29). The tandem Ig-like domains of LigB adopts an elongated conformation without being fully extended. Kinks occur at domains 2 and 7, and the structure beyond domain 7 forms a gentle spiral. Interdomain interactions between neighboring domains may occur via a salt bridge between lysine and aspartate residues in adjacent domains (26). The lysine and aspartate residues are found at the same position in most of the Ig-like domains of LigA and LigB. On the other hand, only three of the twelve domains of LigC have lysine at the equivalent position, although 11 of the 12 have the aspartate residue. Salt bridges are unlikely to contribute significantly to the kinks in the extended structure of the Lig proteins since the location of paired lysine and arginine residues in adjacent domains do not correlate with the location of the kinks. However, the salt bridges likely hinder rotation of neighboring domains. The low sequence identity between adjacent Lig Ig-like domains may be necessary to prevent interdomain misfolding following its biosynthesis (30).
LigA and LigB are calcium-binding proteins (31). An early study showed that LigBCen2, which comprised LigB12 plus extra amino acids at its amino- and carboxy termini, bound to calcium (32). It was shown later that individual Ig-like domains LigA9 and LigA10 bound to calcium, confirming that the residues involved in calcium binding were contained entirely within the Ig-like domains (31). LigA9, LigA10, and LigBCen2 formed homodimers in the absence of calcium (31). The Lig proteins were the first examples of the new class of Ig-like domains that bind calcium (33). LigA4, LigBCen2, and LigBCen2R (comprising LigB12 and an N-terminal extension, Figure 1) also bound with high affinity to Ca2+, and a chemical shift was observed in NMR experiments upon the addition of Ca2+ (19, 25, 32). However, this observation was not confirmed in a separate NMR study that showed no chemical shift of LigB12 when CaCl2 or EGTA were added (26).
Evolution and Relatedness
Whole-genome sequencing reveals that >90% leptospiral species in clades P1 and P2 have at least one member of the lig gene family (1). In Clade P1 species, the ligB gene is most consistently present, while the ligA and ligC genes are only present in selected species. The version of Lig protein present in Clade P2 species has been classified as LigC (34), though the phylogenetic tree of the Lig proteins in Figure 4 shows that it is distinct from the LigC proteins found in L. interrogans and other Clade P1 species. Species in Clades S1 and S2 appear to lack lig genes. This suggests that an ancestral lig gene, similar in structure to ligB and ligC, was acquired after divergence of P and S clades and prior to divergence of Clades P1 and P2. Presumably, after divergence of Clade P1, duplication of this ancestral lig gene led to the formation of ligB and ligC. Subsequently, ligA was derived from ligB by gene duplication with loss of the carboxy-terminal region, as suggested by McBride et al. (36). The sequences of all three lig genes exhibit evidence of horizontal gene flow and recombinational events between lig genes of related Leptospira strains (36). Additional studies are needed to obtain a more accurate understanding of lig gene distribution and relatedness among leptospiral species.
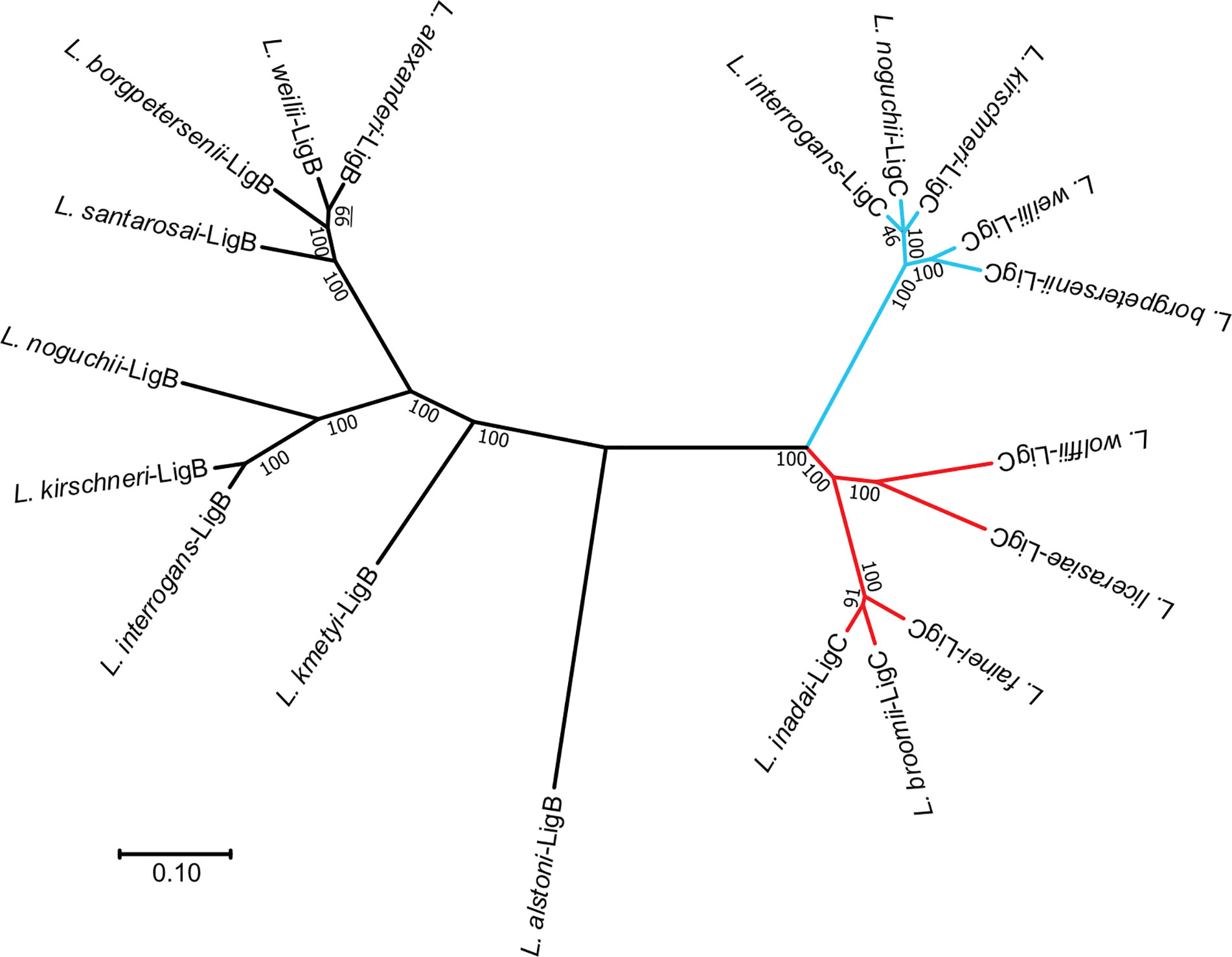
Figure 4 Phylogenetic Tree of Leptospiral Immunoglobulin-Like B (LigB) and LigC. An unrooted phylogenetic tree of the LigB and LigC proteins is shown. Sequences were aligned with MUSCLE (35), and the tree was generated with the Neighbor Joining distance algorithm. Branch lengths represent the distance between nodes. Scale bar shows the distance for a 10% difference between sequences. Bootstrap values from 1,000 replicates are shown. LigB branches are shown in black. Branches for clade P1 LigC proteins are indicated in light blue. Clade P2 LigC branches are colored red. Clade P1 sequences were obtained from L. .interrogans sv Lai str 56601 (AAN50976, AAN50273), L. kirschneri sv Grippotyphosa str. RM52 (EJO71150), L. kirschneri sv Cynopteri str 3522CT (EPG39682), L. noguchii sv Panama str CZ214T (EQA72229, EQA70476), L. alstoni sv Pingchang str 80-412 (EQA78456, EQA70476), L. weilii str Ecochallenge (EMY15141, EMY13008), L. alexanderi sv Manhao 3, str L 60T (EQA60934), L. borgpetersenii sv Javanica str UI 09931 (EPG5 EQA784568006, EPG56034), L. santarosai sv Shermani str 1342KT (EPG84276), and L. kmetyi sv Malaysia str Bejo-Iso9 (EQA54247). Clade P2 sequences were obtained from L. fainei sv Hurstbridge str BUT6T (EPG73553), L broomii sv Hurstbridge str 5399T (EQA44381), L. wolffii sv Khorat str Khorat-H2T (EPG64420), L. licerasiae sv Varillal str VAR 010 (EIE02109), and L. .inadai sv Lyme str 10T (EQA38254).
In L. interrogans serovar Copenhageni strain Fiocruz L1-130 and L. kirschneri serovar Grippotyphosa strain RM52, the regions immediately upstream of the ligA and ligB coding regions are identical. In the Fiocruz L1-130 strain, the region of identity begins -266 nucleotides upstream of the ligA and ligB start codons and extends to position +1890 encoding the part of the seventh Ig-like domain with only minor coding differences between ligA and ligB (13). Inclusion of E. coli-like -35 and -10 RNA polymerase binding elements upstream of the transcriptional start site at position -175 as mapped by 5’ RACE (9) and confirmed by differential RNAseq designed to identify primary transcripts (37) suggests that ligA and ligB are transcriptionally co-regulated. Maintenance of this high degree of gene identity between ligA and ligB implies a gene conversion process involving replacement of this segment of one gene by the other. Gene conversion is likely facilitated by the arrangement of ligA and ligB as tandem genes on some leptospiral chromosomes.
Virulence and Gene Regulation
There were clues that the Lig proteins were involved in pathogenesis even before the tools for the genetic manipulation of L. interrogans became available. The initial study on ligA described it as a gene that was induced during infection (12). LigA could not be detected in lysates of L. interrogans growing in vitro, yet antibody against LigA was detected in sera from infected mares, suggesting that expression of ligA was infection-associated. This was confirmed by reactivity of LigA antiserum with kidney sections from infected hamsters (12). Loss of virulence observed with multiple culture passage of L. interrogans is correlated by a reduction of LigA and LigB expression (13, 38). Subsequently it was found that LigA and LigB expression increased in vitro under growth conditions encountered in the host, when various salts were added to the culture medium to attain physiological osmolarity (10, 11) or when the culture temperature was increased to 37°C (9, 39).
There is signification post-transcriptional regulation of lig gene expression. Figure 5 shows the predicted stem-loop structure that sequesters the ribosome-binding site and start codon of the lig genes (9). Deletion mutations that remove a portion of one strand of the stem increase ternary complex formation at the ribosome binding site in vitro and increase expression of a lig’-’lacZ translational fusion in E. coli (9). Mutations that disrupt base pairing in the stem also elevate expression of the fusion, and compensatory mutations that restore base pairing without restoring the wild-type sequence exhibit near wild-type levels of fusion expression. Elevation of the incubation temperature causes expression of the translational fusion to increase. These results support the existence of an “RNA thermometer” strategy for regulating lig gene expression, which has been identified in a number of bacterial pathogens, such as Listeria monocytogenes with lifestyles that include phases inside and outside of the mammalian host (40). Transcript levels of ligA and ligB were higher at 37°C than at 30°C (9) and higher during growth in a dialysis membrane chamber model in the rat compared to growth in vitro at 30°C (41). Transcript levels of ligB were also higher during the bacteremia of hamster infection (42). The simplest explanation for the effect of temperature on lig transcript levels is that the increased translation at the higher temperature protected the lig transcripts from degradation by RNases. Direct measurement of lig mRNA stability is required to test this hypothesis. It should be noted that the ligB gene is followed immediately by four small open reading frames, predicted to encode lipoproteins, that appear to be cotranscribed and coregulated with ligB in the same operon (11, 39).
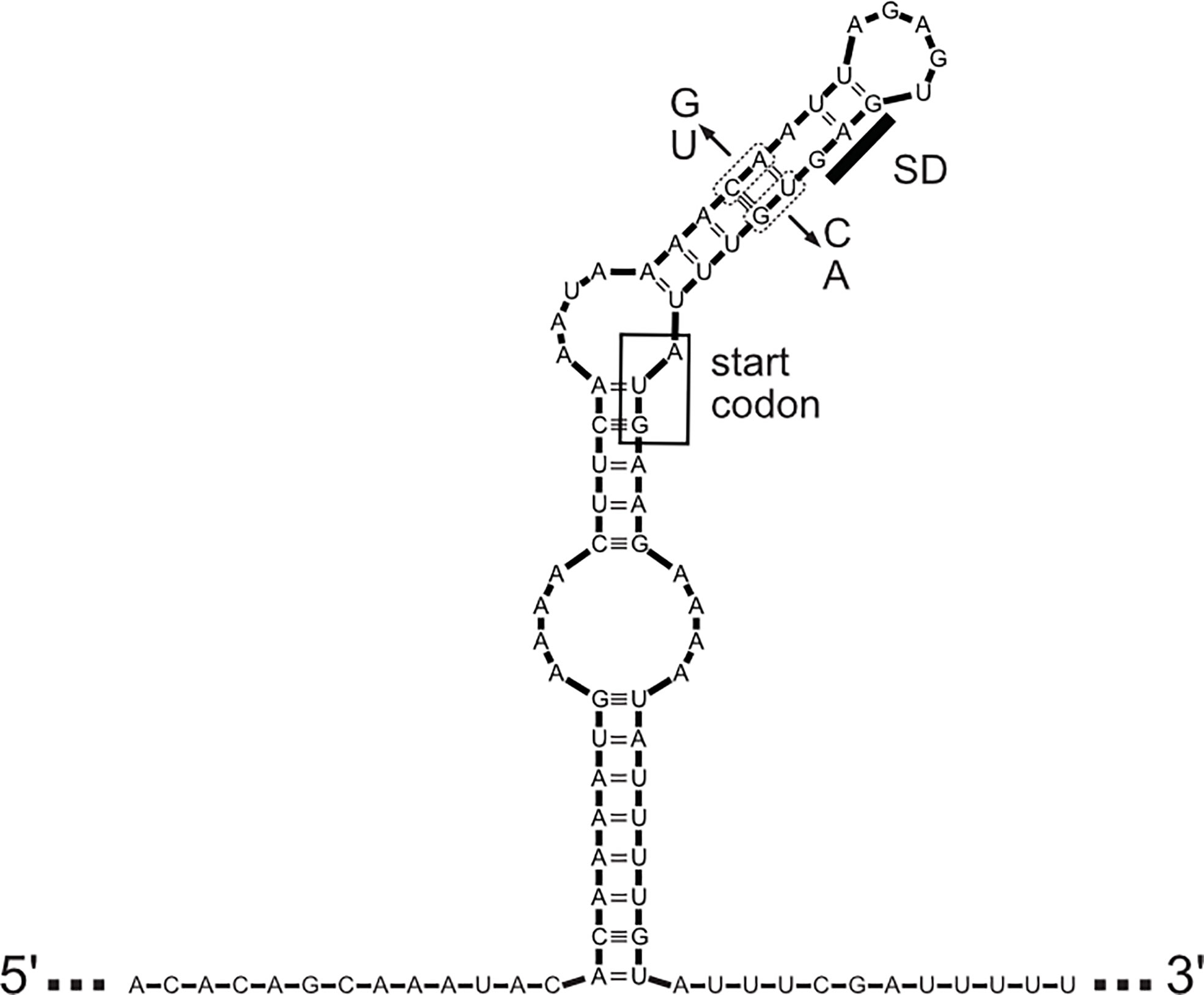
Figure 5 Predicted Structure of Leader Sequence. The predicted secondary structure of the mRNA leader sequence encompassing the coding sequences of ligA and ligB of L. interrogans sv Copenhageni str Fiocruz is shown. The Shine-Dalgarno sequence (SD) and AUG start codon (inside the box) are sequestered by the stem-loop structure. Location of mutations used to either disrupt or restore base pairing and test effects on expression are shown (see text for details).
The requirement of ligB for virulence was examined directly in two studies involving L. interrogans. A ligB mutant was generated in the Fiocruz L1-130 strain by targeted mutagenesis of ligB by replacing a segment of the gene with an antibiotic-resistance cassette (43). The mutant retained virulence by intraperitoneal inoculation into the hamster model of leptospirosis and caused histopathological damage. Although the ligB mutant also retained the ability to persistently colonize rats, a subtle effect of the mutation on renal colonization cannot be ruled out since the bacterial load in the kidneys was not quantified. Similarly, when hamsters were inoculated with a pool of leptospiral mutants carrying transposon insertions, the fitness of ligB mutant remained unaltered in blood, kidney, and liver (44). Because the hamsters were inoculated intraperitoneally in both studies, an effect of the ligB mutation on infection by a natural route of infection cannot be ruled out. Additionally, this study did not rule out the possibility that ligA was compensating for the loss of ligB.
A more recent study provided evidence that expression of either ligA or ligB is required for virulence (45). An artificial transcriptional repressor of the lig genes was constructed by using a Transcription Activator-Like Effector (TALE) and inserted into a transposon harbored on a suicide plasmid. The plasmid was transferred to the L495 strain of L. interrogans by conjugation, and the transposon was allowed to randomly insert into the chromosome. LigA and LigB levels were diminished in three transconjugants, two of which were avirulent following intraperitoneal inoculation into hamsters. Blood and kidneys were culture negative at death, indicating that expression of either ligA or ligB was required not only for virulence but also for renal colonization. These results support the conclusion that LigA and LigB have overlapping essential functions in virulence. It is important to note that the ligC gene is intact in L. interrogans serovar Manilae, strain L495, suggesting a distinct, non-redundant function for LigC. A ligC transposon insertion mutant in the strain L495 retained virulence (46).
Interactions With the Host Extracellular Matrix
The extracellular matrix (ECM) is a complex mixture of fibrous proteins, proteoglycans, and glycoproteins that are found in the spaces between cells and provide for their support. Together with cell adhesion proteins, they are the glue that holds tissues together. They also provide tracks along which cells migrate during development, immune response, tissue repair, and other processes requiring cell movement.
Some of the earliest studies of leptospiral attachment found that L. interrogans adhered to the ECM and to the individual ECM components fibronectin, type I collagen, and laminin and that the level of adherence was correlated with leptospiral virulence (47, 48), a characteristic correlated with Lig protein expression (13). Over 40 leptospiral proteins have been identified that bind to selected extracellular matrix components (49, 50). For most of these putative ECM adhesins, evidence comes primarily from in vitro studies that examine interactions between purified recombinant proteins and ECM components. The biological significance of some of these interactions has been questioned (51) because it seems unlikely that such a large number of adhesins would have redundant roles in the interaction with the extracellular matrix in vivo.
The designation of LigA and LigB as ECM binding proteins is based on a range of evidence. The binding of LigA and LigB to purified fibronectin and other components of the ECM has been examined in vitro with purified recombinant Lig proteins. Because full-length Lig proteins are difficult to express in E. coli, shorter fragments of LigA and LigB that collectively spanned both proteins were used in these studies. High-affinity binding to fibronectin was observed with a fragment harboring the carboxyterminal Ig-like domains of LigA (LigA7’-13, Figure 1) and LigB (LigB7’-12, LigBCen, Figure 1) (32, 52, 53). Binding affinity was highest when the first Big_5 domain was included with LigB7’-12 (LigB-U, Figure 1) (52). The N-terminal Ig-like domains common to LigA and LigB did not bind fibronectin (52, 54). A weak binding site with a dissociation constant in the micromolar range was also detected in the C-terminal half of the CTE of LigB in two independent studies (LigBcte’ and LigBctv, Figure 1) (52, 54). The binding site in the CTE was narrowed down to an 11 amino acid peptide containing a critical Leu-Ile-Pro-Ala-Asp sequence, which attached to the 15th type III module of fibronectin (55). Differential binding activities for extracellular matrix proteins were observed for LigA and LigB. The affinity of LigA7’-13 for fibronectin was weaker than that of LigB7’-12 (apparent Kd of 126 nM vs. 73 nM) (52), although similar affinities of LigA7’-13 and LigB7’-12 for the gelatin-binding domain of fibronectin were observed by another group (53). LigB7’-12 also attached with moderate affinity to collagen I, collagen IV, and laminin, whereas binding of LigA7’-13 to the collagen molecules was poor and to laminin undetectable (52). Finally, LigBCon and LigBCen had moderate affinity for lung elastin, whereas LigBctv adherence to elastin was undetectable (56). Adherence of LigA7’-13 to elastin was not examined. In gain-of-function studies with the poorly adhesive L. biflexa, heterologous expression of LigA and LigB on the surface of this organism significantly enhanced its ability to adhere to fibronectin and laminin (57). However, production of LigA or LigB did not increase attachment of L. biflexa to elastin, collagen I, or collagen IV, despite the host molecules being bound with moderate affinity by recombinant LigB.
L. interrogans adheres to a variety of cultured mammalian cells, including MDCK canine kidney cells (58, 59). Adhesion to MDCK cells is inhibited if L. interrogans is premixed with fibronectin prior to addition of leptospires to the monolayer, suggesting that adherence of L. interrogans to MDCK cells relies in part on fibronectin (54). LigBCen and LigBctv (Figure 1) attached to MDCK cells in a dose-dependent manner, although binding of LigBctv was weaker. This result is consistent with the stronger affinity of LigBCen over LigBctv for fibronectin. Binding of both LigBCen and LigBctv were reduced in MDCK cells in which fibronectin expression was knocked down with siRNA, further supporting the notion that fibronectin serves a receptor for LigB-mediated adhesion of L. interrogans to MDCK cells. Moreover, LigBCen and LigBctv inhibited adherence of L. interrogans when the MDCK cells were pretreated with the proteins (54). However, an L. interrogans ligB mutant adhered to MDCK cells as well as the wild-type strain (43). The discrepancy between the lack of effect of the ligB mutation on adherence and the inhibitory activity of LigB protein segments on adherence may be explained by the presence of additional adhesins that target the same host protein. It is likely that the LigB proteins prebound to MDCK cells block other fibronectin-binding adhesins expressed by L. interrogans, such as LigA. In general, interpretation of experiments whose goal is to assign adhesin activity to a microbial surface protein by deploying it an inhibitor are complicated by the presence of additional surface proteins targeting the same host receptor. In this case, gain-of-function experiments with L. biflexa expressing the Lig proteins are helpful. In fact, L. biflexa producing LigA adhered to MDCK cells better than L. biflexa alone (7). Surprisingly, surface expression of LigB did not enhance adherence of L. biflexa to MDCK cells. This result is possibly due to proteolysis of LigB in L. biflexa leaving behind a slightly smaller version of LigB as its major species, as revealed in Western blots (7). The lost segment of LigB may have contained the fibronectin-binding sequence in LigBctv (54).
Fibronectin-binding proteins mediate bacterial adhesion to and invasion of host cells (60). For example, binding of fibronection by Staphylococcus aureus fibronectin-binding proteins enables over a 100-fold increase of intracellular bacteria within epithelial and endothelial cells (61, 62). Similarly, L. biflexa expressing LigB is taken up by mouse macrophages and exhibits enhanced binding to fibronectin compared with L. biflexa lacking LigB (38). Virulent L. interrogans invades the murine monocyte-macrophage-like cell line J774A.1 and induces apoptosis (63). A small population of leptospires that escaped killing also multiplied in a vacuole of mouse macrophages (64). L. interrogans injected into zebrafish embryos also invade macrophages, which subsequently traffic to the kidneys (65). However, a ligB mutant was able to infect mouse macrophages as well as wild-type L. interrogans, whereas an lmb216 mutant was significantly impaired in invading macrophages (38), which calls into question whether LigB is significantly involved in macrophage invasion.
Evasion of Innate Immunity Including Complement
Leptospires must fight off a number of components of host innate immune system to establish an infection. During the initial encounter with the host, the spirochetes immediately encounter physical barriers, antimicrobial peptides, and other elements of the innate immune system. They must also deal with the cellular elements of innate immunity, including neutrophils and macrophages. During dissemination in the bloodstream, leptospires must evade the bactericidal activity of complement. Leptospires that colonize the proximal tubular lumen of the kidney also encounter complement due to local production by the kidney (66, 67). The three complement pathways, classical, lectin-binding, and alternative, are triggered by different processes that converge on the cleavage of C3 into C3a and C3b near the surface of microbes. The covalent binding of C3b to the microbial surface leads to direct killing of the target microbe through assembly of C5 convertase and assembly of the lethal membrane attack complex. Indirect killing occurs because C3b is an opsonin that is recognized by specific receptors on phagocytes, which are attracted by the anaphylatoxins C3a and C5a released by cleavage of C3 and C5 by the respective convertases.
Sensitivity of Leptospira species to serum killing is related to their pathogenicity. The noninvasive saprophyte Leptospira biflexa is rapidly killed in 40% normal human serum (NHS) within 5 min (68). On the other hand, pathogenic leptospiral strains exhibit various degrees of resistance to serum killing (69). Serum resistance of pathogenic leptospires is likely due to complement resistance mechanisms. Additional studies are needed to determine which complement pathways are responsible for controlling L. interrogans infections. The survival of one L. interrogans strain with intermediate levels of resistance to normal human serum was enhanced in serum deficient for the complement component C1s, suggesting that serum resistant strains of L. interrogans are equipped to resist the classical complement pathway (69). Human sera that test negative for Leptospira-specific antibodies with the microscopic agglutination test contain IgG that binds to Leptospira strains (69, 70). Therefore, the classical complement pathway may be triggered on the surface of L. interrogans by nonspecific IgG in individuals never exposed to Leptospira previously.
Pathogenic leptospires incubated in normal human serum capture the complement regulators factor H, FHL-1, FHR-1, and C4b-binding protein (C4BP) (68, 69). The principal role of these complement regulators is to prevent complement activation on host-cell surfaces by acting as a cofactor for the protease factor I, which cleaves C3b and C4b, components of the alternative and classical pathways, respectively. They also accelerate disassembly of the convertases. Except for FHL-1, the complement regulators captured by L. interrogans retained cofactor activity for factor I, which cleaves C3b and C4b. In contrast, L. biflexa bound negligible amounts of factor H and C4BP. To examine the role of the Lig proteins in the capture of complement regulators, separate plasmids carrying the ligA and ligB genes were transformed into L. biflexa. Heterologous production of LigA or LigB on the surface of L. biflexa resulted in enhanced survival in normal human serum (8, 71). The transformed strains captured factor H and C4BP from human serum and cleaved C3b and C4b when added along with the substrates to the Lig-producing L. biflexa pre-incubated with human serum or purified complement regulators (8). Membrane attack complex (MAC) deposition on the surface was also reduced in the transformants, although it remained greater than the level of MAC on L. interrogans. Recombinant LigA7'-13 and LigB7'-12 also bound to factor H and C4BP, which retained the cofactor activities leading to proteolysis of C3b and C4b, respectively. Although LigB0-7’ was also shown to bind both complement regulators at room temperature (72), a later study showed that attachment of LigB0-7’ to C4BP during incubation at physiologic temperature was barely detectable (73). The Lig proteins also attached to factor H related-1 (FHR-1) and factor H like-1 (FHL-1) proteins (72). These results suggest that L. interrogans uses LigA and LigB to capture host complement regulators to defend itself against complement.
Additional studies provided direct evidence that LigB inhibits complement pathways. A segment of LigB that hinders complement activity was identified with complement-dependent hemolytic assays (71). LigB9-11 that was preincubated with human serum inhibited lysis of antibody-sensitized sheep erythrocytes by human complement, indicating that LigB inhibits the classical complement pathway. C4BP binds with high affinity to LigB9-10 (73), indicating that C4BP is at least partially responsible for LigB-mediated resistance to the classical pathway. Additional high-affinity sites for C4BP were mapped to LigA7-8 and LigA10-11. Similarly, LigB9-11 preincubated with human serum suppressed lysis of rabbit erythrocytes by human complement, indicating that LigB inhibited the alternative complement pathway as well, presumably by acquiring factor H from the host.
Curiously, LigB9-11 also binds with high affinity to C3b and C4b (71). The reason for LigB capturing such lethal host proteins is unknown. However, the outer surface protein OspC of Lyme Borrelia blocks formation of C3 convertase by competing with complement factor C2 for binding to C4b (74). Similarly, Efb of Staphylococcus aureus binding to C3b allosterically inhibited its binding to complement factor Bb (75).
The CCP (complement control protein) modules of the complement regulators bound by the Lig proteins were identified using a panel of C4BP mutants lacking single CCP domains in its α chain (73). Deletion of CCP1, CCP2, or CCP3, which are involved in binding C4b, has no effect on binding of LigA7’-13 or LigB7’-12. Instead, removal of CCP7 or CCP8 diminished binding of both Lig proteins (73). Several other bacterial pathogens also interact with C4BP via CCP7 and/or CCP8. LigA7’-13, LigB7’-12, and LigB0-7’ also binds to a fragment of factor H encompassing the six C-terminal CCP domains, CCP15-20 (72). More importantly, two different monoclonal antibodies raised against CCP20 inhibited binding of LigB7’-12 by 50% suggesting that LigB7’-12 targets CCP20 for binding. CCP19-20 is critical for factor H function because CCP20 harbors the site for binding to host cells and CCP19 binds to C3b. A variety of microbes use the same site in CCP20 to bind factor H, including the spirochetes Borrelia burgdorferi and Borrelia hermsii (76–79). It is possible that L. interrogans uses LigB to interact with factor H at the same site in CCP20.
Additional complement resistance mechanisms involve capturing plasminogen and secreting metalloproteases that cleave complement components (80). Plasmin is a tightly controlled protease that plays a central role in the fibrinolytic system by dissolving fibrin clots after wound repair. Plasmin is generated from cleavage of the proenzyme plasminogen by one of several proteases, including urokinase-type plasminogen activator (uPA). L. interrogans exploits plasmin to cleave and inactivate C3b and IgG bound to its surface (81). L. interrogans captures plasminogen, which is converted by exogenously added urokinase-type plasminogen activator (uPA) into plasmin (82). The LigB7’-12 fragment binds with high affinity to plasminogen (KD = 95 nM). LigA7’-13 also binds plasminogen, but with much lower affinity (KD = 1.2 µM) (70). When plasmin is generated from the bound plasminogen by the addition of uPA, not only does plasmin cleave its physiologic substrate, fibrinogen, but it also cleaves C3b, and C5 in vitro. These results suggest that LigA and LigB help L. interrogans resist complement not only by acquiring host complement regulators but also by proteolysis of complement components with a captured host protease.
Modulation of Hemostasis
Hemorrhage is a well-known hallmark of leptospirosis, with a majority of patients manifesting mild bleeding of the skin and mucous membranes to severe pulmonary hemorrhage and gastrointestinal bleeding (83). In a study of 52 consecutive patients with severe leptospirosis in Semarang, Indonesia, 60% of patients had clinical evidence of bleeding (84). At autopsy, widespread hemorrhaging has been described at mucosal surfaces and various organs including the heart, lungs and kidneys (85). Laboratory measures of coagulation (e.g., prothrombin time) and fibrinolysis (e.g., D-dimer levels) are typically abnormal in leptospirosis (83, 84). These changes in hemostatic pathways could be due to either a sepsis-like host response to leptospirosis (i.e., disseminated intravascular coagulation), direct effects of L. interrogans (86–89), or both.
Impaired coagulation and enhanced fibrinolysis are likely to facilitate leptospiral invasion and dissemination in the case of a mucosal or skin wound as the site of initial infection. Local impairment of hemostasis could act as a form of immune evasion by preventing entrapment in fibrin strands. There is in vitro evidence that the Lig proteins could be playing one or more roles in these processes through their interactions with host coagulation factors and platelets. Both LigA and LigB bind to fibrinogen as purified proteins (52, 90) and increased fibrinogen binding when expressed on the surface of L. biflexa (6). The region of LigB responsible for binding was identified to be domains 9-11 (Figure 6), which was found to have high affinity for fibrinogen and fibrinogen fragment D, with KDs of ~28 nM (6). The interaction with fibrinogen fragment D is important because fragment D is responsible for cross-linking fibrin monomers during coagulation. The affinity of LigB domains 9-11 (LigB9-11) for fragment D may be responsible for the ability of LigB9-11 to block thrombin-catalyzed fibrin clot formation (6).
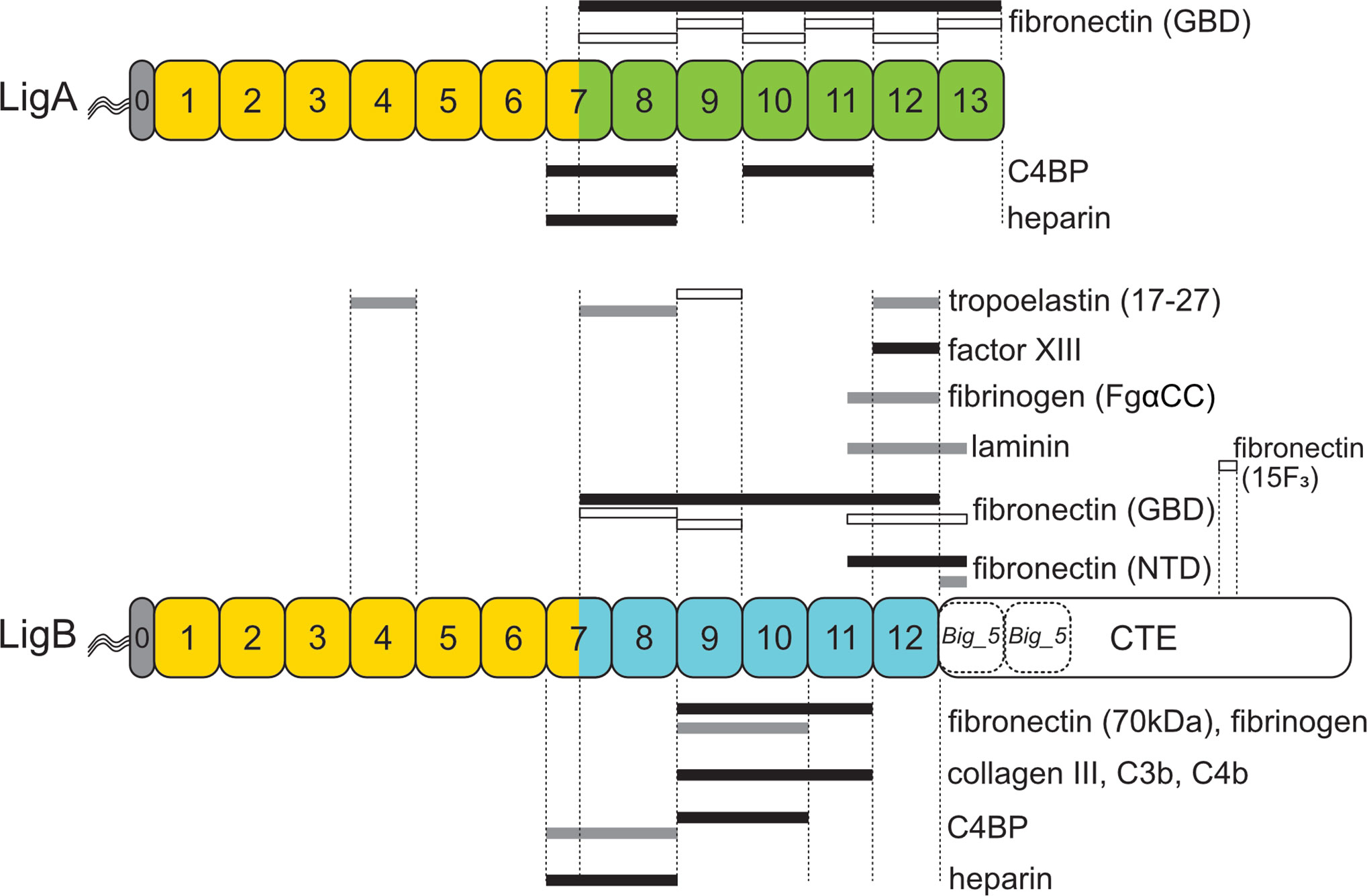
Figure 6 Leptospiral Immunoglobulin-Like B (LigB) Domains Involved in Interactions with Host Proteins. Regions of LigA and LigB involved in interactions with host proteins are shown for heparin (73), C4 binding protein (C4BP) (73), tropoelastin (56), factor XIII (91), fibrinogen (6, 92), laminin (90), collagen III (6), complement components C3b and C4b (71), the gelatin-binding domain (GBD) of fibronectin (53), the amino-terminal domain (NTD) of fibronectin (32, 93), the 70 kDa fragment of fibronectin (6), and the 15th type III module of fibronectin (15F3) (55). The color scheme used for the Lig proteins is identical to that of Figure 1. Nearly identical sequences between LigA and LigB are shown in yellow. The remaining Big_2 domains are shown in green (LigA) and blue (LigB). The C-terminal extensions (CTE) contain putative Big_5 domains (dashed). Black bars indicate high-affinity binding (< 100 nM) to the host target, gray bars indicate moderate affinity (100–800 nM), and open bars indicate low affinity (> 1 µM).
LigB9-11 overlaps with LigBCen2R, a region of LigB comprising a portion of domain 11 and all of domain 12. LigBCen2R bound to the C-terminal αC (FgαCC) domain of fibrinogen, a component of fragment D (92). Like LigB9-11, LigBCen2R inhibited fibrin clot formation, and also blocked platelet adhesion and aggregation (92). Interestingly, a region of LigB similar to LigBCen2 was enriched from among 280,000 phage clones in a screen for heparin binding proteins (94). Another group mapped additional heparin-binding sites to LigB7-8 and LigA7-8 (73). This is relevant to hemostasis because heparin is a host glycosaminoglycan that inhibits clot formation. Subsequently, LigB domain 12 (LigB12) was found to interact not only with the FgαCC domain of fibrinogen but also with factor XIII, which acts to cross-link fibrin to form a more stable clot (91). LigB12 blocked binding of factor XIII to FgαCC and prevented factor XIII-mediated cross-linking of fibrinogen. This discovery suggests a second possible mechanism for LigB to inhibit coagulation, and this study found that like LigB9-11 and LigBCen2R, LigB12 was able to block thrombin-catalyzed fibrin clot formation (91).
Fibrinolysis involves digestion of fibrin clots, primarily by the proteolytic activity of plasmin. Plasminogen has been shown to bind to the leptospiral surface and become activated to plasmin (82). As discussed earlier, the Lig proteins may be responsible for some of the binding of plasminogen to the leptospiral surface. Both LigA and LigB bind plasminogen, and Lig-bound plasminogen can be converted to active plasmin in the presence of urokinase-type plasminogen activator (8). Thus, expression of the Lig proteins on the leptospiral surface provides a way for leptospires to cleave fibrin as a dissemination and/or immune evasion mechanism.
Characterization of Domains Involved in Host Protein Interactions
Deletion studies in vitro with LigB by several groups have narrowed down the contact points of LigB with most host molecules examined to discrete segments within domains 9 through 11 (Figure 6) (6, 71, 73). The 70 kDa N-terminal domain of fibronectin, fibrinogen, collagen III, C3b, and C4b make high-affinity contact with LigB9-11, and C4BP binds tightly to LigB9-10 (Figure 6). At least for fibronectin and fibrinogen, LigB domain 11 contributes less strongly to the interactions. The evidence for this is that LigB9-10 retained moderate affinity for fibronectin and fibrinogen (~150-250 nM), whereas binding to the host proteins by LigB10-11 was not detected (6). On the other hand, domains 9 and 10 are critical for binding activity. In contrast to the effect of removing domain 11 from Lig9-11, removal of the amino-terminal half of domain 9 or even placing a histidine tag (His6) at the amino terminus of LigB9-11 eliminated binding. Removal of domain 10 from LigB7’-10 resulted in weak binding to fibronectin and fibrinogen (6). From these observations, the authors proposed that high-affinity contacts occur between LigB and fibronectin and fibrinogen within domains 9 through 10, with domain 11 serving to enhance binding by affecting the conformation or positioning of the other domains (6). The kinks detected in the LigB structure may be important in interdomain interactions that lead to high-affinity binding with host proteins (29).
Another group has demonstrated through a series of studies that the binding of LigB to fibronectin, fibrinogen, and factor XIII centers on domain 12 (54, 91–93). Although the involvement of LigB12 in binding to fibronectin seems to contradict the findings by Choy et al. at first glance (6), Lin et al. found that the high-affinity binding of LigB12 to the NTD of fibronectin required an additional 47 residues beyond domain 12, extending into the first putative Big_5 domain in the CTE (93). LigB12 missing the additional sequence failed to bind the NTD of fibronectin, which is consistent with the lack of binding to fibronectin observed by Choy et al. with LigB11-12. On the other hand, the observed moderate affinity of LigBCen2R (part of domain 11 and all of domain 12) for fibrinogen (92) is contradicted by the complete lack of binding observed with LigB11-12 (6). A possible reason for the discrepancy is that the 6-histidine tag was placed at the carboxy terminus of LigB11-12 and at the amino terminus of LigBCen2R (6, 92).
Fibronectin binding requires conformational flexibility of the Lig proteins. High-affinity binding of LigBCen2 to the NTD of fibronectin requires Ca2+ (32). The far-UV CD spectrum of LigB Cen2 in the absence of calcium suggests that although the protein is largely a β-sheet structure, the protein is partial unfolded (32). In the presence of calcium, LigBCen2 gains β-sheet structure. The key segment in LigBCen2 for interaction with the NTD of fibronectin lies within the 47-residue segment beyond the C-terminus of LigB12 rather than within LigB12. In the presence of calcium, binding to the NTD by LigBCen2R, which lacks the 47 residues, was not detected (93). The 47 amino acid segment alone, LigBCen2NR, which comprises the initial portion of the first Big_5 domain in the CTE, retained moderate binding affinity for the NTD. Several biophysical experiments indicated that LigBCen2NR was highly disordered and extended. The β-sheet conformation of LigBCen2NR increased considerably upon addition of the NTD of fibronectin, akin to the β-zipper mode of binding described for other fibronectin-binding proteins (95). On the other hand, the NTD did not undergo major conformational changes upon binding LigBCen2NR (93). Further studies are needed to understand the role of calcium in the conformation of LigBCen2NR in relation to the 47-residue extension.
Binding of LigA and LigB may involve multiple domains contacting host proteins in an avidity effect. LigA7’-13 and LigB7’-12 bind with high affinity to the GBD of fibronectin, yet none of the individual domains were able to attach with even moderate affinity (53). Weak binding was detected with domains LigB7’-8, LigB9, and LigBCen2 (Figure 6). Binding was not measurable with LigB10 or LigB11 (53). Weak binding to the GBD was also detected with LigA7’-8 and the individual LigA domains from LigA9 through LigA13. These results suggest that LigA and LigB binding to GBD involves multidomain interactions. In another study, L. interrogans was shown to adhere to elastin and tropoelastin. LigBCon (LigB1-7’) and LigBCen (LigB7’-12 plus half the C-terminal domain) had moderate affinity for tropoelastin, while binding by LigA7’-13 was not detected (56). Deletion studies narrowed down the binding sites for tropoelastin to LigB4 and LigB12, but weaker binding sites were also detected with LigB7’-8 and LigB9 (Figure 6). Thus, interaction of the Lig proteins with fibronectin and tropoelastin may involve multiple Ig-like domains making simultaneous contact with the host proteins.
LigB4 was selected for further analysis since it had the highest binding affinity for tropoelastin. The affinity of LigB4 for tropoelastin was affected by pH changes, although the secondary structure was unaffected. This indicated that an ionic interaction was involved in the LigB-tropoelastin interaction. Since the tropoelastin fragment used for the experiment was positively charged, the sole acidic amino acid conserved in LigB4, LigB7’-8, LigB9, and LigB12 was changed to asparagine in LigB4. This D341N mutation caused reduced affinity of LigB4 for tropoelastin without affecting its secondary structure (56). In the NMR structure of LigB12, the asparate residue corresponding to position 341 is located in the loop between strands B and C at the external edge of sheet 1a, where its side chain is well positioned to interact with a host macromolecule (Figures 2 and 3A).
Amino acid residues in LigB12 important for binding to FgαCC8 were also identified by targeted mutagenesis (91). The D1061A, F1054A, and A1065K mutations decreased binding of LigB12 to FgαCC8. None of the three changes affected the secondary structure of LigB12. All three changes are located in sheet 1a, which extends out from the rest of the structure of LigB12 (Figure 3A). Although the aspartate at position 1061 is conserved among the LigB Ig-like domains, the phenylalanine at position 1054 and alanine at 1065 are not present in the other LigB domains, which do not bind FgαCC8 (Figure 2). Thus, LigB uses sheet 1a of its Ig-fold to interact with tropoelastin and fibrinogen (56, 91).
Serodiagnosis
LigA and LigB are potential serodiagnostic antigens. As mentioned above, the lig genes were discovered by probing expression libraries with convalescent sera. LigB seroreactivity is present in 81% of Brazilian patients with leptospirosis for less than 7 days of illness, including many patients who had not yet formed antibodies detectable by whole cell IgM enzyme-linked immunosorbent assay (ELISA) and microagglutionation test (MAT) (96). LigA seroreactivity has also been shown to be present in high percentages of patients with acute leptospirosis in Thailand an average of 7 days after onset of fever (97) and in the Philippines at the time of hospital admission (98). Lig antigens have also been found to be useful serodiagnostic antigens for canine (99) and equine (100, 101) leptospirosis. In a proteome microarray screen comparing KD 2,241 leptospiral proteins (61% of the L. interrogans proteome), LigA7-13 and LigB7-12 had the highest IgG area under the curve (AUC) among all proteins studied for reactivity with sera obtained from patients with acute leptospirosis (at the time of admittance to the hospital) compared to sera from healthy controls in an area of high endemicity for leptospirosis (102). A follow-up proteome microarray study examining reactivity of sera from patients with mild and severe disease (requiring hospitalization) found that the domains shared by LigA and LigB (LigA/B1-6) had the highest accuracy for diagnosis of both forms of infection (103). The high levels of Lig seroreactivity in patients with acute leptospirosis suggests that the Lig proteins are expressed early in infection.
Vaccine Studies
The Lig proteins are among the most promising subunit vaccine candidates for immunoprotection against leptospirosis. At least 21 studies have examined immunoprotection with either recombinant Lig proteins or lig DNA (Table 1). The seminal Lig vaccine study, published in 2004 by Koizumi and Watanabe, provided evidence for immunoprotection from lethality (but not renal colonization) with both LigA and LigB expressed as recombinant proteins in which all of the Ig-like domains were included (14). Unlike most vaccine studies, which involve hamsters, the animal model was 4-week-old C3H/HeJ mice. Hamsters are highly susceptible to overwhelming, disseminated infection, while mice are considerably less susceptible though they may develop renal colonization. C3H/HeJ mice have a defect in TLR4, which impairs their innate immune response to leptospiral lipopolysaccharide (LPS) and increases their susceptibility to leptospiral infection. In addition, this study involved a mouse-adapted L. interrogans serovar Manilae strain that was inoculated by intraperitoneal inoculation at a challenge dose of 106 organisms, 1000-times greater than the ED50 dose.
An important aspect of the 2004 Koizumi and Watanabe study was immunization with recombinant Lig proteins expressed as His6 fusion proteins for purification purposes. Expression of such large (~120 kD), recombinant Lig proteins has proven to be difficult for other laboratories to produce in soluble form. A subsequent vaccine study, published in 2006 by the group at Cornell University, involved expression of recombinant LigA fragments as glutathione-S-transferase (GST) fusion proteins to enhance solubility. Two recombinant LigA fusion proteins were produced; the first protein consisted of the region of LigA largely though not entirely shared with LigB (domains 0-7’, Figure 1), while the second protein consisted of the region unique to LigA (domains 7’-13, Figure 1) (122). Both the shared and unique regions were combined and adsorbed to the adjuvant aluminum hydroxide (Al(OH)3) for immunization purposes. A weakness of the 2006 study was that the serovar Pomona challenge strain required inoculation at a dose of 108 organisms for 50% of hamsters to reach endpoint criteria (ED50). Because hamsters were challenged at the ED50 dose, a statistical difference between groups of control- and LigA-immunized hamsters was not observable based on endpoint criteria alone (Table 1). Nevertheless, the LigA-immunized hamsters had a significant advantage in terms of the severity of renal histopathology.
Subsequently, efforts were made to identify the region of LigA responsible for immunoprotective activity. A study published in 2007 involved immunization with Freund’s adjuvant combined with recombinant His6-tagged LigA expressed in E. coli, purified under denaturing conditions and then refolded by stepwise dialysis. The initial immunization was performed with Complete Freund’s Adjuvant (CFA), a water-in-oil emulsion containing killed mycobacteria (123). Specific mycobacterial cell wall components in CFA, N-glycolyl muramyl dipeptide and the glycolipid trehalose-6, 6 dimycolate, stimulate cellular immunity and antibody production and induce a mixed Th1 and Th17 response (124–126). Subsequent immunizations were performed with incomplete Freund’s adjuvant (IFA), which is CFA without mycobacteria. IFA induces a balanced Th1/Th2 response and produces an antibody response (125, 127). Immunoprotection was demonstrated with a recombinant His6 fusion protein consisting of the carboxyterminal region that is unique to LigA (LigA7’-13) (104). No protection was observed with the aminoterminal domains shared between LigA and LigB and carboxyterminal region of LigB. Subsequently, our group showed that this immunoprotective effect was found to reside primarily in LigA domains 10-13, with a minimum of three domains required for 100% survival of hamsters (109). LigA domains 11–12 were required but insufficient for immunoprotection, requiring combination with a third domain, either 10 or 13, for immunoprotection.
Because of concern for the safety of Freund’s adjuvant, LigA7’-13 was also tested with the adjuvant aluminum hydroxide (Al(OH)3), which is used in several FDA-approved vaccines (128). Aluminum hydroxide is comprised of nanoparticles that form porous aggregates to which the antigen adsorbs (129). Aluminum hydroxide maintains the antigen at a high local concentration in the depot to promote antigen uptake by antigen-presenting cells (128). “Danger” signals released from cells exposed to aluminum hydroxide stimulate APCs, eventually leading to a Th2 response for most antigens (130, 131). In contrast to the results with Freund’s adjuvant, significant immunoprotection against L. interrogans was not observed in four studies with LigA7’-13 adsorbed to aluminum hydroxide despite generation of specific IgG responses (Table 1, Fisher’s Exact Test) (106, 110, 111, 116). Given the uptake and survival of L. interrogans in macrophages in cell culture and in vivo models (64, 65), one possible explanation for the advantage of Freund’s adjuvant over aluminum salts is that immunoprotection from L. interrogans involves Th1 lymphocyte activation of macrophages with intracellular leptospires. In addition, both Th1 and Th17 cells were involved in protective immunity against other predominantly extracellular bacteria in immunoprotection studies (132, 133). One study that did observe immunoprotection with aluminum hydroxide involved mannosylated LigA7’-13 synthesized in the yeast Pichia pastoris (113). Mannoproteins are targeted to DC-SIGN and mannose receptor on the surface of antigen presenting cells (APCs) to enhance antigen presentation (134).
Modern adjuvants known to induce a more robust Th1 response were also tested. Biodegradable particulate systems, such as liposomes and microspheres, enhance delivery of protein antigens to APCs (135, 136). In one study, hamsters were protected from illness following immunization with LigA7’-13 that was packaged in a phosphatidylcholine-based liposome for immunization (106). The animals vaccinated with the liposome formulation had a higher titer of anti-LigA IgG than those vaccinated with aluminum hydroxide as adjuvant, which was not significantly protective (106). Similar protection was obtained by the same group when poly-lactic-co-glycolic acid (PLGA) microspheres were used as carrier for LigA7’-13, although the antibody titer was similar to when aluminum hydroxide was the adjuvant (Table 1, Fisher’s Exact test). In general, PLGA particles cause a Th1 bias in the immune response (136), although splenic Th1 and Th2 cytokine mRNAs were both increased following immunization with the LigA7’-13/PLGA particles relative to transcript levels from hamsters immunized with LigA7’-13/Al(OH)3 (106). In another study, neutral liposomes were mixed with the saponin QS-21 and the TLR4 agonist monophosphoryl lipid A (120, 121). 60% of the immunized hamsters survived challenge, whereas no animals immunized with adjuvant alone survived (121). Altering the physicochemical properties of the liposome such as its surface charge or size may substantially improve performance of liposome-based vaccines (137). On the other hand, LigA7’-13 was not protective when introduced into hamsters using carboxyl multi-walled carbon nanotubes (COOH-MWCNTs), even when CpG oligodeoxynucleotides, a potent TLR9 agonist, were added to the carrier (116). Similarly, CpG oligodeoxynucleotides (ODNs) had no effect as an adjuvant for LigA7’-13 in a separate study (114). Because the sequence of the CpG ODNs was optimized to stimulate mice and human TLR9 (138), and the optimal CpG ODN sequence for stimulating hamster TLR9 is unknown, the negative results do not rule out the possibility of TLR9 promoting immunity to L. interrogans in hamsters. Finally, different polysaccharides have been tested as immune adjuvants with other pathogens (139). Xanthan, an exopolysaccharide of Xanthomonas spp., exhibited strong immunoprotection against L. interrogans when used as an adjuvant for LigA7’-13 (114).
An alternative approach that would be appropriate for immunization of reservoir hosts is to present LigA7’-13 as an oral vaccine. We have shown that hamsters could be moderately protected against intradermal challenge by oral immunization with E. coli expressing a lipidated form of LigA7’-13 (112). Protection from intraperitoneal challenge did not reach statistical significance by Fisher’s Exact test (Table 1). The antigen was expressed from a plasmid encoding the OspA signal peptide fused to LigA7’-13. Triacylated lipopeptides stimulate signaling through TLR1/TLR2 heterodimers (140), and the lipid moiety influences the appearance of specific IgG subtypes in mice orally immunized with Lactobacillus plantarum expressing N-terminal triacylated OspA (141). L. plantarum is being explored as a vehicle to orally deliver leptospiral immunogens (142) in part because of its ability to stimulate innate immune memory via macrophages (143).
Considerable effort has been devoted to development of a LigB vaccine because ligB is found in, if not expressed by, more pathogenic Leptospira species than ligA (34). Neither LigB7’-12 as a recombinant protein with Freund’s or aluminum hydroxide adjuvant nor DNA with aluminum hydroxide was protective (104, 107, 111). In addition, DNA encoding the C-terminal extension of LigB was not protective (111) (Table 1). Immunization with the early Ig-like domains of LigB shared with LigA as recombinant protein has conferred varying degrees of protection. As shown in Table 1, some studies have reported largely negative results (104, 108, 118), another moderate success (107), and a more recent study nearly complete protection (117). The success of the latter study may be accounted for by the use of an intramuscular route of immunization instead of the subcutaneous route used for the other studies (Table 1). However, it should be noted that in a separate study, hamsters immunized with LigB0-7' protein via the intramuscular route were not protected from heterologous challenge (115) (Table 1).
Published Lig vaccine studies have employed a variety of approaches to evaluate protection results including antibody responses, lymphoproliferative responses, histopathology, as well as the number of animals that met endpoint criteria. Periodic acid Schiff staining and a histopathology scoring system are particularly helpful in evaluating the impacts on vaccination on renal histopathology (109). Current approaches to examining cellular immune responses involve measurement of Lig-specific lymphoproliferative responses of splenic cells and determination of cytokine mRNA levels in vaccinated hamsters (105–107). A preliminary determination of the T helper response can be made based on the IgG isotype generated by vaccination. Hamster IgG isotypes involved with immunoprotection have been determined (116, 117, 119, 121), yet the T helper responses associated with the IgG subsets have not been well characterized in hamsters. Experiments in mice may be more informative due to the well-characterized immune system and the available reagents. Statistical evaluation of subtle outcome differences in small sample size (i.e., fewer than 30 animals) studies using binary (pass/fail) endpoints by Fisher’s Exact test is often less sensitive than comparison of survival curves using the Log-rank (Mantel-Cox) test. For example, while the difference between the group immunized with LigBCon vs. the control group of 2/6 vs. 0/6 in the study by Cao et al. (108) is not significant on a simple binary analysis, the survival curves are significantly different at a level of P < 0.05 by Log-rank analysis.
An advantage of a protein-based vaccine against leptospirosis is the potential for broad, cross-protection against multiple species and serovars. Cross-protection with Lig proteins seems possible given their relatively high degree of sequence conservation. For example, we found 96.4% average LigB amino acid sequence identity among eleven different L. interrogans and L. kirschneri serovars (Table 2). Most current leptospiral vaccines consist of inactivated whole bacterial cells and elicit a humoral response primarily against LPS, whose structure differs considerably among leptospiral serovars. As a result, immunity generated by whole cell vaccines is generally serovar specific. For this reason, whole cell vaccines typically contain a combination of serovars prevalent locally (144). In some studies, heterologous protection against unrelated serovars has been observed (145, 146), including cases with altered or depleted LPS (147–149). As discussed in a recent review on leptospirosis vaccines (150), these varying results may also stem from different levels of immunoprotective protein antigens, such as the Lig proteins, whose expression varies according to the culture conditions used to grown the antigen source or challenge strain.
There is some experimental evidence that LigB can mediate heterologous protection. Cross protection was observed in one study with a DNA vaccine encoding the Ig-like domains shared between LigA and LigB (LigB0-7’) from serovar Canicola of L. interrogans (111). DNA vaccines have the potential of stimulating both an antibody and a Th1-biased response (151), which may be an advantage given the evidence for intracellular invasion of macrophages (38, 65). Five of eight hamsters immunized with the DNA with aluminum hydroxide were protected from lethal challenge with serovar Copenhageni, whereas none of six control hamsters survived (P = 0.03). However, these results may not be robust. In a later study only two of five hamsters immunized with the same DNA vaccine were protected versus none of five in the control group (P = 0.44) (115). Homologous challenge with a Canicola strain that would have revealed the maximum possible protective effect with the immunization regimen was not performed in either study. A prime-boost regimen exhibited more promising results in the same study. Prime boost regimens have the potential to broaden the immune response, as demonstrated for influenza vaccination (152). Hamsters were first immunized with the DNA vaccine adsorbed to aluminum hydroxide and then boosted with recombinant LigB0-7’ protein adsorbed to the adjuvant 21 days later. Specific IgG levels were higher in animals subjected to the prime-boost regimen than those that received the DNA vaccine for both immunizations, although lower than the group that received the recombinant protein for both inoculations (115). Only the group receiving the prime-boost was significantly protected from heterologous challenge with the Copenhageni serovar (5 of 6 survivors) (115). None of the five animals immunized with the recombinant protein alone were protected despite the high levels of LigB antibody produced by the immunization.
Critical to any evaluation of any candidate leptospirosis vaccine is its ability to not only prevent overwhelming infection and endpoint criteria such as lethality but also residual renal infection among survivors. Prevention of sublethal infection is particularly important for vaccines intended for animals. LigA7’-13 vaccines have typically revealed sublethal renal infection regardless of adjuvant, as determined by culture, serology, histopathology and/or real-time PCR. Even in studies showing dramatic 100% vs. 0% survival rates in Lig- vs. control-immunized animals, burdens of infection up to ~1 million copies of leptospiral DNA per microgram of hamster tissue DNA have been documented in hamsters surviving infection (109). In contrast, recent studies that have shown protection from lethal infection with domains shared by LigA and LigB as protein (117) or DNA (111) showed culture negativity of kidneys from most survivors in the vaccinated group. The immunological mechanisms necessary for sterilizing immunity are unknown, but several observations suggest that renal production of IFN-γ is involved (153, 154). Mice deficient in IL-10, a negative regulator of IFN-γ production, rapidly clear L. interrogans from their kidneys (155). In addition, a whole-cell serovar Hardjo vaccine that prevents renal colonization in cattle stimulates a potent Th1 response; CD4+ and γδ T cells from the animals proliferate and produce IFN-γ in response to Hardjo antigens (156).
In a structure-based approach, investigators took a rational approach to vaccine design by first considering the low-resolution structure of the tandem Ig-like domains of LigB (29). The extensively accessible surface area involves almost all of the 12 domains, justifying their subsequent effort to generate monoclonal antibodies against the entire length of the 12 Ig-like domains of LigB. Knowledge of the NMR-derived structure of LigB12 (26), which was assumed to be similar across all 12 Ig-like domains, allowed them to finely map the epitopes of bactericidal monoclonal antibodies to specific surfaces of a domain. Surfaces targeted by highly bactericidal monoclonal antibodies were combined to form a single chimeric Ig-like domain. The chimera comprised β strands A, B, B’, and C from domain 10, β strands C’, D, E, and F from domain 7, and β strands G and G’ from domain 7. This “LigB10-B7-B7” chimeric protein completely protected hamsters from lethal challenge with L. interrogans, whereas all hamsters immunized with LigB7, LigB10, or PBS succumbed to the infection. The bacterial loads in the liver, kidney, and bladder of animals immunized with the chimera were orders of magnitude lower than in tissues from animals mock immunized with PBS. No histopathological damage was observed in animals immunized with LigB10-B7-B7. These promising results demonstrate the advantages of structure-based vaccine design for guiding construction of leptospiral vaccines based on recombinant surface proteins.
Conclusions
Leptospira speciesbelonging to the P1 and P2 clades contain one or more members of the family of surface-exposed, virulence proteins containing a series of Ig-like domains: LigA, LigB, and LigC. The various drivers, characteristics, functions, and impacts of the Lig proteins covered in this review are summarized in Figure 7. As indicated by studies involving simultaneous transcriptional knockdown of ligA and ligB, some or all of the roles played by these proteins appear to be required for infection of hamsters. While expression of LigA and LigB is strongly induced by osmolar and thermal conditions found in the mammalian host, little is known about the regulatory pathways controlling their expression. The Lig proteins interact with a range of host proteins and appear to play multiple roles in host invasion and evasion of innate immunity, including complement resistance and hemostasis. Despite the surface accessibility along the length of the Ig-like domains, almost all host protein interactions are confined to the C-terminal Ig-like domains that differ between LigA and LigB. The structure and function of the putative Big_5 domains in the LigB C-terminal extension remain to be investigated beyond their involvement in fibronectin binding. LigB is a logical target for vaccine development because its gene is present in most pathogenic species of Leptospira. However, protective immunity accompanied by dramatic reductions in the burden of infection in the kidneys has been achieved in few studies. Future success of vaccine development with LigB will require a better understanding of the cellular immune mechanisms associated with immunoprotection. While the Lig proteins have been examined extensively since their discovery nearly two decades ago, their potential as vaccines and serodiagnostic antigens for prevention and diagnosis of leptospirosis remain to be fully realized.
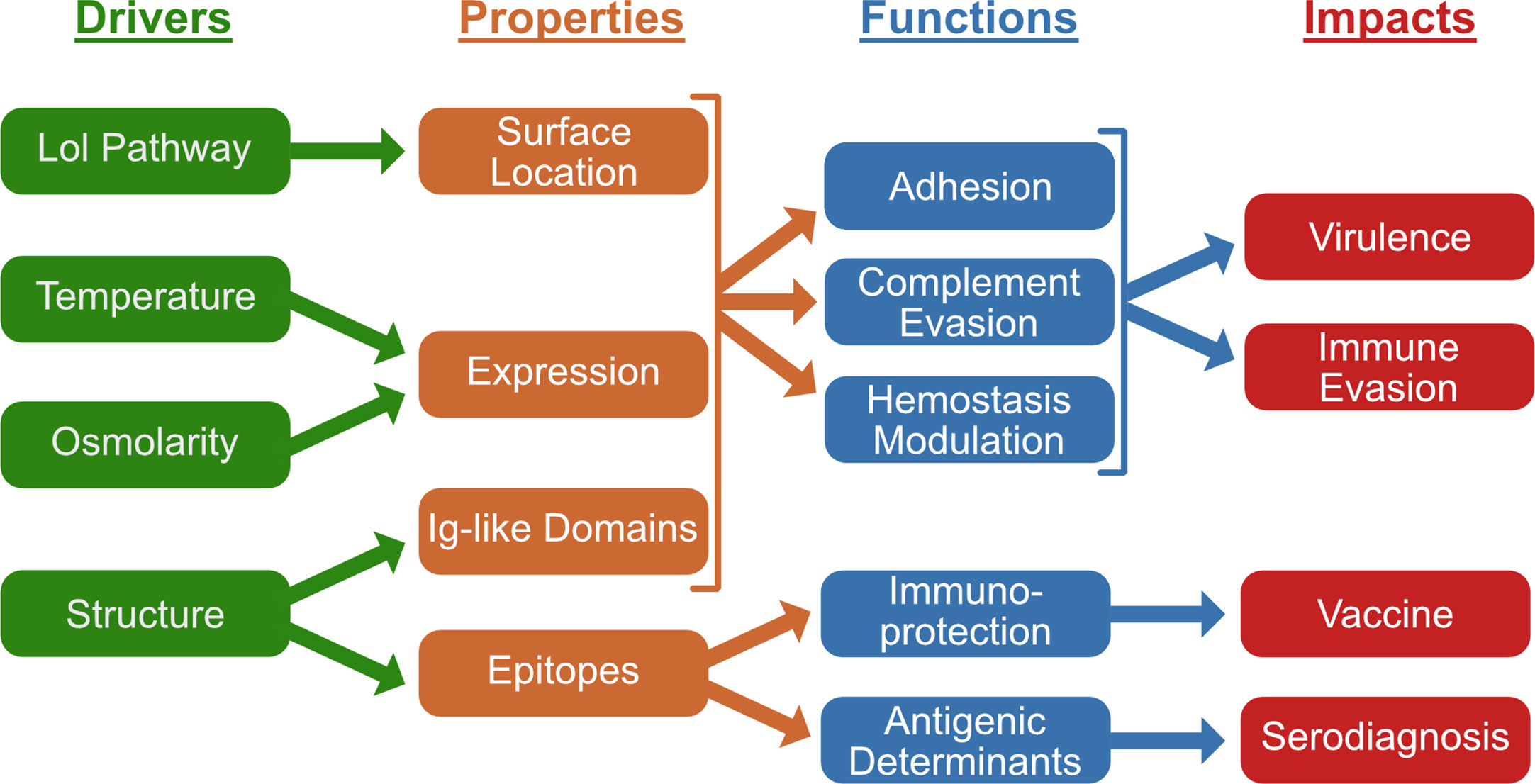
Figure 7 Summary of Leptospiral Immunoglobulin-Like (Lig) Protein Drivers, Charateristics, Functions, and Impacts. Lig proteins are exported to the leptospiral surface as lipoproteins via the localization of lipoprotein (Lol) pathway. Expression is stimulated by temperature and osmolarity. Their immunoglobulin (Ig)-like domains are responsible for various functions including leptospiral adhesion, complement evasion and modulation of hemostasis. These functions contribute to leptospiral virulence and immune evasion. Lig protein epitopes have been found to contain immunoprotective vaccine and serodiagnostic determinants.
Author Contributions
DH and JM conceived the review, appraised the literature, and wrote the manuscript. All authors contributed to the article and approved the submitted version.
Funding
The work was supported by a Veterans Affairs Merit Award and a National Institutes of Health grant R21AI128560 to DH.
Conflict of Interest
The authors have relevant intellectual property related to the Lig proteins.
References
1. Vincent AT, Schiettekatte O, Goarant C, Neela VK, Bernet E, Thibeaux R, et al. Revisiting the taxonomy and evolution of pathogenicity of the genus Leptospira through the prism of genomics. PloS Negl Trop Dis (2019) 13:e0007270. doi: 10.1371/journal.pntd.0007270
2. Werts C. Interaction of Leptospira with the innate immune system. Curr Top Microbiol Immunol (2018) 415:163–87. doi: 10.1007/82_2017_46
3. Fraga TR, Isaac L, Barbosa AS. Complement evasion by pathogenic Leptospira. Front Immunol (2016) 7:623. doi: 10.3389/fimmu.2016.00623
4. Scharrig E, Carestia A, Ferrer MF, Cedola M, Pretre G, Drut R, et al. Neutrophil extracellular traps are involved in the innate immune response to infection with Leptospira. PloS Negl Trop Dis (2015) 9:e0003927. doi: 10.1371/journal.pntd.0003927
5. Cagliero J, Villanueva S, Matsui M. Leptospirosis pathophysiology: into the storm of cytokines. Front Cell Infect Microbiol (2018) 8:204. doi: 10.3389/fcimb.2018.00204
6. Choy HA, Kelley MM, Croda J, Matsunaga J, Babbitt JT, Ko AI, et al. The multifunctional LigB adhesin binds homeostatic proteins with potential roles in cutaneous infection by pathogenic Leptospira interrogans. PloS One (2011) 6:e16879. doi: 10.1371/journal.pone.0016879
7. Figueira CP, Croda J, Choy HA, Haake DA, Reis MG, Ko AI, et al. Heterologous expression of pathogen-specific genes ligA and ligB in the saprophyte Leptospira biflexa confers enhanced adhesion to cultured cells and fibronectin. BMC Microbiol (2011) 11:129. doi: 10.1186/1471-2180-11-129
8. Castiblanco-Valencia MM, Fraga TR, Breda LC, Vasconcellos SA, Figueira CP, Picardeau M, et al. Acquisition of negative complement regulators by the saprophyte Leptospira biflexa expressing LigA or LigB confers enhanced survival in human serum. Immunol Lett (2016) 173:61–8. doi: 10.1016/j.imlet.2016.03.005
9. Matsunaga J, Schlax PJ, Haake DA. Role for cis-acting RNA sequences in the temperature-dependent expression of the multiadhesive Lig proteins in Leptospira interrogans. J Bacteriol (2013) 195:5092–101. doi: 10.1128/JB.00663-13
10. Matsunaga J, Sanchez Y, Xu X, Haake DA. Osmolarity, a key environmental signal controlling expression of leptospiral proteins LigA and LigB and the extracellular release of LigA. Infect Immun (2005) 73:70–8. doi: 10.1128/IAI.73.1.70-78.2005
11. Matsunaga J, Lo M, Bulach DM, Zuerner RL, Adler B, Haake DA. Response of Leptospira interrogans to physiologic osmolarity: relevance in signaling the environment-to-host transition. Infect Immun (2007) 75:2864–74. doi: 10.1128/IAI.01619-06
12. Palaniappan RU, Chang YF, Jusuf SS, Artiushin S, Timoney JF, McDonough SP, et al. Cloning and molecular characterization of an immunogenic LigA protein of Leptospira interrogans. Infect Immun (2002) 70:5924–30. doi: 10.1128/iai.70.11.5924-5930.2002
13. Matsunaga J, Barocchi MA, Croda J, Young TA, Sanchez Y, Siqueira I, et al. Pathogenic Leptospira species express surface-exposed proteins belonging to the bacterial immunoglobulin superfamily. Mol Microbiol (2003) 49:929–45. doi: 10.1046/j.1365-2958.2003.03619.x
14. Koizumi N, Watanabe H. Leptospiral immunoglobulin-like proteins elicit protective immunity. Vaccine (2004) 22:1545–52. doi: 10.1016/j.vaccine.2003.10.007
15. Haake DA, Zuckert WR. Spirochetal lipoproteins in pathogenesis and immunity. Curr Top Microbiol Immunol (2018) 415:239–71. doi: 10.1007/82_2017_78
16. Herwald H, Cramer H, Morgelin M, Russell W, Sollenberg U, Norrby-Teglund A, et al. M protein, a classical bacterial virulence determinant, forms complexes with fibrinogen that induce vascular leakage. Cell (2004) 116:367–79. doi: 10.1016/s0092-8674(04)00057-1
17. Kelly G, Prasannan S, Daniell S, Fleming K, Frankel G, Dougan G, et al. Structure of the cell-adhesion fragment of intimin from enteropathogenic Escherichia coli. Nat Struct Biol (1999) 6:313–8. doi: 10.1038/7545
18. Fraser JS, Yu Z, Maxwell KL, Davidson AR. Ig-like domains on bacteriophages: a tale of promiscuity and deceit. J Mol Biol (2006) 359:496–507. doi: 10.1016/j.jmb.2006.03.043
19. Mei S, Zhang J, Zhang X, Tu X. Solution structure of a bacterial immunoglobulin-like domain of the outer membrane protein (LigB) from Leptospira. Proteins (2015) 83:195–200. doi: 10.1002/prot.24723
20. El-Gebali S, Mistry J, Bateman A, Eddy SR, Luciani A, Potter SC, et al. The Pfam protein families database in 2019. Nucleic Acids Res (2019) 47:D427–D32. doi: 10.1093/nar/gky995
21. Poljak RJ, Amzel LM, Avey HP, Chen BL, Phizackerley RP, Saul F. Three-dimensional structure of the Fab’ fragment of a human immunoglobulin at 2,8-Â resolution. Proc Natl Acad Sci USA (1973) 70:3305–10. doi: 10.1073/pnas.70.12.3305
22. Halaby DM, Mornon JP. The immunoglobulin superfamily: an insight on its tissular, species, and functional diversity. J Mol Evol (1998) 46:389–400. doi: 10.1007/pl00006318
23. Bodelon G, Palomino C, Fernandez LA. Immunoglobulin domains in Escherichia coli and other enterobacteria: from pathogenesis to applications in antibody technologies. FEMS Microbiol Rev (2013) 37:204–50. doi: 10.1111/j.1574-6976.2012.00347.x
24. Halaby DM, Poupon A, Mornon J. The immunoglobulin fold family: sequence analysis and 3D structure comparisons. Protein Eng (1999) 12:563–71. doi: 10.1093/protein/12.7.563
25. Mei S, Zhang J, Zhang X, Tu X. Solution structure of leptospiral LigA4 Big domain. Biochem Biophys Res Commun (2015) 467:288–92. doi: 10.1016/j.bbrc.2015.09.170
26. Ptak CP, Hsieh CL, Lin YP, Maltsev AS, Raman R, Sharma Y, et al. NMR solution structure of the terminal immunoglobulin-like domain from the Leptospira host-interacting outer membrane protein, LigB. Biochemistry (2014) 53:5249–60. doi: 10.1021/bi500669u
27. Hamburger ZA, Brown MS, Isberg RR, Bjorkman PJ. Crystal structure of invasin: a bacterial integrin-binding protein. Science (1999) 286:291–5. doi: 10.1126/science.286.5438.291
28. Sehnal D, Rose AS, Koča J, Burley SK, Velankar S. Mol*: towards a common library and tools for web molecular graphics. In: Proceedings of the Workshop on Molecular Graphics and Visual Analysis of Molecular Data. Goslar, DEU: Eurographics Association (2018). p. 29–33.
29. Hsieh CL, Ptak CP, Tseng A, Suguiura IMS, McDonough SP, Sritrakul T, et al. Extended low-resolution structure of a Leptospira antigen offers high bactericidal antibody accessibility amenable to vaccine design. Elife (2017) 6. doi: 10.7554/eLife.30051
30. Borgia MB, Borgia A, Best RB, Steward A, Nettels D, Wunderlich B, et al. Single-molecule fluorescence reveals sequence-specific misfolding in multidomain proteins. Nature (2011) 474:662–5. doi: 10.1038/nature10099
31. Raman R, Rajanikanth V, Palaniappan RU, Lin YP, He H, McDonough SP, et al. Big domains are novel Ca2+-binding modules: evidences from Big domains of Leptospira immunoglobulin-like (Lig) proteins. PloS One (2010) 5:e14377. doi: 10.1371/journal.pone.0014377
32. Lin YP, Raman R, Sharma Y, Chang YF. Calcium binds to leptospiral immunoglobulin-like protein, LigB, and modulates fibronectin binding. J Biol Chem (2008) 283:25140–9. doi: 10.1074/jbc.M801350200
33. Dominguez DC, Guragain M, Patrauchan M. Calcium binding proteins and calcium signaling in prokaryotes. Cell Calcium (2015) 57:151–65. doi: 10.1016/j.ceca.2014.12.006
34. Fouts DE, Matthias MA, Adhikarla H, Adler B, Amorim-Santos L, Berg DE, et al. What makes a bacterial species pathogenic?: Comparative genomic analysis of the genus Leptospira. PloS Negl Trop Dis (2016) 10:e0004403. doi: 10.1371/journal.pntd.0004403
35. Edgar RC. MUSCLE: multiple sequence alignment with high accuracy and high throughput. Nucleic Acids Res (2004) 32:1792–7. doi: 10.1093/nar/gkh340
36. McBride AJ, Cerqueira GM, Suchard MA, Moreira AN, Zuerner RL, Reis MG, et al. Genetic diversity of the leptospiral immunoglobulin-like (Lig) genes in pathogenic Leptospira spp. Infect Genet Evol (2009) 9:196–205. doi: 10.1016/j.meegid.2008.10.012
37. Zhukova A, Fernandes LG, Hugon P, Pappas CJ, Sismeiro O, Coppee JY, et al. Genome-wide transcriptional start site mapping and sRNA identification in the pathogen Leptospira interrogans. Front Cell Infect Microbiol (2017) 7:10. doi: 10.3389/fcimb.2017.00010
38. Toma C, Murray GL, Nohara T, Mizuyama M, Koizumi N, Adler B, et al. Leptospiral outer membrane protein LMB216 is involved in enhancement of phagocytic uptake by macrophages. Cell Microbiol (2014) 16:1366–77. doi: 10.1111/cmi.12296
39. Lo M, Bulach DM, Powell DR, Haake DA, Matsunaga J, Paustian ML, et al. Effects of temperature on gene expression patterns in Leptospira interrogans serovar Lai as assessed by whole-genome microarrays. Infect Immun (2006) 74:5848–59. doi: 10.1128/IAI.00755-06
40. Loh E, Righetti F, Eichner H, Twittenhoff C, Narberhaus F. RNA thermometers in bacterial pathogens. Microbiol Spectr (2018) 6. doi: 10.1128/microbiolspec.RWR-0012-2017
41. Caimano MJ, Sivasankaran SK, Allard A, Hurley D, Hokamp K, Grassmann AA, et al. A model system for studying the transcriptomic and physiological changes associated with mammalian host-adaptation by Leptospira interrogans serovar Copenhageni. PloS Pathog (2014) 10:e1004004. doi: 10.1371/journal.ppat.1004004
42. Matsui M, Soupe ME, Becam J, Goarant C. Differential in vivo gene expression of major Leptospira proteins in resistant or susceptible animal models. Appl Environ Microbiol (2012) 78:6372–6. doi: 10.1128/AEM.00911-12
43. Croda J, Figueira CP, Wunder EA Jr., Santos CS, Reis MG, Ko AI, et al. Targeted mutagenesis in pathogenic Leptospira species: disruption of the LigB gene does not affect virulence in animal models of leptospirosis. Infect Immun (2008) 76:5826–33. doi: 10.1128/IAI.00989-08
44. Lourdault K, Matsunaga J, Haake DA. High-throughput parallel sequencing to measure fitness of Leptospira interrogans transposon insertion mutants during acute infection. PloS Negl Trop Dis (2016) 10:e0005117. doi: 10.1371/journal.pntd.0005117
45. Pappas CJ, Picardeau M. Control of gene expression in Leptospira spp. by transcription activator-like effectors demonstrates a potential role for LigA and LigB in Leptospira interrogans virulence. Appl Environ Microbiol (2015) 81:7888–92. doi: 10.1128/AEM.02202-15
46. Murray GL, Morel V, Cerqueira GM, Croda J, Srikram A, Henry R, et al. Genome-wide transposon mutagenesis in pathogenic Leptospira species. Infect Immun (2009) 77:810–6. doi: 10.1128/IAI.01293-08
47. Ito T, Yanagawa R. Leptospiral attachment to four structural components of extracellular matrix. Nihon Juigaku Zasshi (1987) 49:875–82. doi: 10.1292/jvms1939.49.875
48. Ito T, Yanagawa R. Leptospiral attachment to extracellular matrix of mouse fibroblast (L929) cells. Vet Microbiol (1987) 15:89–96. doi: 10.1016/0378-1135(87)90133-7
49. Vieira ML, Fernandes LG, Domingos RF, Oliveira R, Siqueira GH, Souza NM, et al. Leptospiral extracellular matrix adhesins as mediators of pathogen-host interactions. FEMS Microbiol Lett (2014) 352:129–39. doi: 10.1111/1574-6968.12349
50. Murray GL. The molecular basis of leptospiral pathogenesis. Curr Top Microbiol Immunol (2015) 387:139–85. doi: 10.1007/978-3-662-45059-8_7
51. Adler B. Pathogenesis of leptospirosis: cellular and molecular aspects. Vet Microbiol (2014) 172:353–8. doi: 10.1016/j.vetmic.2014.06.015
52. Choy HA, Kelley MM, Chen TL, Moller AK, Matsunaga J, Haake DA. Physiological osmotic induction of Leptospira interrogans adhesion: LigA and LigB bind extracellular matrix proteins and fibrinogen. Infect Immun (2007) 75:2441–50. doi: 10.1128/IAI.01635-06
53. Lin YP, McDonough SP, Sharma Y, Chang YF. The terminal immunoglobulin-like repeats of LigA and LigB of Leptospira enhance their binding to gelatin binding domain of fibronectin and host cells. PloS One (2010) 5:e11301. doi: 10.1371/journal.pone.0011301
54. Lin YP, Chang YF. The C-terminal variable domain of LigB from Leptospira mediates binding to fibronectin. J Vet Sci (2008) 9:133–44. doi: 10.4142/jvs.2008.9.2.133
55. Lin YP, Greenwood A, Yan W, Nicholson LK, Sharma Y, McDonough SP, et al. A novel fibronectin type III module binding motif identified on C-terminus of Leptospira immunoglobulin-like protein, LigB. Biochem Biophys Res Commun (2009) 389:57–62. doi: 10.1016/j.bbrc.2009.08.089
56. Lin YP, Lee DW, McDonough SP, Nicholson LK, Sharma Y, Chang YF. Repeated domains of Leptospira immunoglobulin-like proteins interact with elastin and tropoelastin. J Biol Chem (2009) 284:19380–91. doi: 10.1074/jbc.M109.004531
57. Figueira CP, Croda J, Choy HA, Haake DA, Reis MG, Ko AI, et al. Heterologous expression of pathogen-specific genes ligA and ligB in the saprophyte Leptospira biflexa confers enhanced adhesion to cultured cells and extracellular matrix components. BMC Microbiol (2011) 11:129. doi: 10.1186/1471-2180-11-129
58. Thomas DD, Higbie LM. In vitro association of leptospires with host cells. Infect Immun (1990) 58:581–5. doi: 10.1128/IAI.58.3.581-585.1990
59. Tsuchimoto M, Niikura M, Ono E, Kida H, Yanagawa R. Leptospiral attachment to cultured cells. Zentralbl Bakteriol Mikrobiol Hyg A (1984) 258:268–74. doi: 10.1016/s0176-6724(84)80044-9
60. Hymes JP, Klaenhammer TR. Stuck in the middle: fibronectin-binding proteins in gram-positive bacteria. Front Microbiol (2016) 7:1504. doi: 10.3389/fmicb.2016.01504
61. Sinha B, Francois PP, Nusse O, Foti M, Hartford OM, Vaudaux P, et al. Fibronectin-binding protein acts as Staphylococcus aureus invasin via fibronectin bridging to integrin α5β1. Cell Microbiol (1999) 1:101–17. doi: 10.1046/j.1462-5822.1999.00011.x
62. Massey RC, Kantzanou MN, Fowler T, Day NP, Schofield K, Wann ER, et al. Fibronectin-binding protein A of Staphylococcus aureus has multiple, substituting, binding regions that mediate adherence to fibronectin and invasion of endothelial cells. Cell Microbiol (2001) 3:839–51. doi: 10.1046/j.1462-5822.2001.00157.x
63. Merien F, Baranton G, Perolat P. Invasion of Vero cells and induction of apoptosis in macrophages by pathogenic Leptospira interrogans are correlated with virulence. Infect Immun (1997) 65:729–38. doi: 10.1128/IAI.65.2.729-738.1997
64. Toma C, Okura N, Takayama C, Suzuki T. Characteristic features of intracellular pathogenic Leptospira in infected murine macrophages. Cell Microbiol (2011) 13:1783–92. doi: 10.1111/j.1462-5822.2011.01660.x
65. Davis JM, Haake DA, Ramakrishnan L. Leptospira interrogans stably infects zebrafish embryos, altering phagocyte behavior and homing to specific tissues. PloS Negl Trop Dis (2009) 3:e463. doi: 10.1371/journal.pntd.0000463
66. Welch TR, Beischel LS, Witte DP. Differential expression of complement C3 and C4 in the human kidney. J Clin Invest (1993) 92:1451–8. doi: 10.1172/JCI116722
67. Noris M, Remuzzi G. Overview of complement activation and regulation. Semin Nephrol (2013) 33:479–92. doi: 10.1016/j.semnephrol.2013.08.001
68. Meri T, Murgia R, Stefanel P, Meri S, Cinco M. Regulation of complement activation at the C3-level by serum resistant leptospires. Microb Pathog (2005) 39:139–47. doi: 10.1016/j.micpath.2005.07.003
69. Barbosa AS, Abreu PA, Vasconcellos SA, Morais ZM, Goncales AP, Silva AS, et al. Immune evasion of leptospira species by acquisition of human complement regulator C4BP. Infect Immun (2009) 77:1137–43. doi: 10.1128/IAI.01310-08
70. Castiblanco-Valencia MM, Fraga TR, Pagotto AH, Serrano SM, Abreu PA, Barbosa AS, et al. Plasmin cleaves fibrinogen and the human complement proteins C3b and C5 in the presence of Leptospira interrogans proteins: A new role of LigA and LigB in invasion and complement immune evasion. Immunobiology (2016) 221:679–89. doi: 10.1016/j.imbio.2016.01.001
71. Choy HA. Multiple activities of LigB potentiate virulence of Leptospira interrogans: inhibition of alternative and classical pathways of complement. PloS One (2012) 7:e41566. doi: 10.1371/journal.pone.0041566
72. Castiblanco-Valencia MM, Fraga TR, Silva LB, Monaris D, Abreu PA, Strobel S, et al. Leptospiral immunoglobulin-like proteins interact with human complement regulators factor H, FHL-1, FHR-1, and C4BP. J Infect Dis (2012) 205:995–1004. doi: 10.1093/infdis/jir875
73. Breda LC, Hsieh CL, Castiblanco Valencia MM, da Silva LB, Barbosa AS, Blom AM, et al. Fine mapping of the interaction between C4b-binding protein and outer membrane proteins LigA and LigB of pathogenic Leptospira interrogans. PloS Negl Trop Dis (2015) 9:e0004192. doi: 10.1371/journal.pntd.0004192
74. Caine JA, Lin YP, Kessler JR, Sato H, Leong JM, Coburn J. Borrelia burgdorferi outer surface protein C (OspC) binds complement component C4b and confers bloodstream survival. Cell Microbiol (2017) 19. doi: 10.1111/cmi.12786
75. Chen H, Ricklin D, Hammel M, Garcia BL, McWhorter WJ, Sfyroera G, et al. Allosteric inhibition of complement function by a staphylococcal immune evasion protein. Proc Natl Acad Sci USA (2010) 107:17621–6. doi: 10.1073/pnas.1003750107
76. Meri T, Amdahl H, Lehtinen MJ, Hyvarinen S, McDowell JV, Bhattacharjee A, et al. Microbes bind complement inhibitor factor H via a common site. PloS Pathog (2013) 9:e1003308. doi: 10.1371/journal.ppat.1003308
77. Rossmann E, Kraiczy P, Herzberger P, Skerka C, Kirschfink M, Simon MM, et al. Dual binding specificity of a Borrelia hermsii-associated complement regulator-acquiring surface protein for factor H and plasminogen discloses a putative virulence factor of relapsing fever spirochetes. J Immunol (2007) 178:7292–301. doi: 10.4049/jimmunol.178.11.7292
78. Kraiczy P, Hellwage J, Skerka C, Kirschfink M, Brade V, Zipfel PF, et al. Immune evasion of Borrelia burgdorferi: mapping of a complement-inhibitor factor H-binding site of BbCRASP-3, a novel member of the Erp protein family. Eur J Immunol (2003) 33:697–707. doi: 10.1002/eji.200323571
79. Kraiczy P, Skerka C, Brade V, Zipfel PF. Further characterization of complement regulator-acquiring surface proteins of Borrelia burgdorferi. Infect Immun (2001) 69:7800–9. doi: 10.1128/IAI.69.12.7800-7809.2001
80. Barbosa AS, Isaac L. Strategies used by Leptospira spirochetes to evade the host complement system. FEBS Lett (2020). doi: 10.1002/1873-3468.13768
81. Vieira ML, de Morais ZM, Vasconcellos SA, Romero EC, Nascimento AL. In vitro evidence for immune evasion activity by human plasmin associated to pathogenic Leptospira interrogans. Microb Pathog (2011) 51:360–5. doi: 10.1016/j.micpath.2011.06.008
82. Vieira ML, Vasconcellos SA, Goncales AP, de Morais ZM, Nascimento AL. Plasminogen acquisition and activation at the surface of Leptospira species lead to fibronectin degradation. Infect Immun (2009) 77:4092–101. doi: 10.1128/IAI.00353-09
83. Chierakul W, Tientadakul P, Suputtamongkol Y, Wuthiekanun V, Phimda K, Limpaiboon R, et al. Activation of the coagulation cascade in patients with leptospirosis. Clin Infect Dis (2008) 46:254–60. doi: 10.1086/524664
84. Wagenaar JF, Goris MG, Partiningrum DL, Isbandrio B, Hartskeerl RA, Brandjes DP, et al. Coagulation disorders in patients with severe leptospirosis are associated with severe bleeding and mortality. Trop Med Int Health (2010) 15:152–9. doi: 10.1111/j.1365-3156.2009.02434.x
85. Arean VM. The pathologic anatomy and pathogenesis of fatal human leptospirosis (Weil’s disease). Am J Pathol (1962) 40:393–423.
86. Vieira ML, Herwald H, Nascimento A. The interplay between host haemostatic systems and Leptospira spp. infections. Crit Rev Microbiol (2020) 46:121–35. doi: 10.1080/1040841X.2020.1735299
87. Vieira ML, Naudin C, Morgelin M, Romero EC, Nascimento AL, Herwald H. Modulation of hemostatic and inflammatory responses by Leptospira spp. PloS Negl Trop Dis (2016) 10:e0004713. doi: 10.1371/journal.pntd.0004713
88. Vieira ML, de Andrade SA, Morais ZM, Vasconcellos SA, Dagli ML, Nascimento AL. Leptospira infection interferes with the prothrombinase complex assembly during experimental leptospirosis. Front Microbiol (2017) 8:500. doi: 10.3389/fmicb.2017.00500
89. Fernandes LG, de Morais ZM, Vasconcellos SA, Nascimento AL. Leptospira interrogans reduces fibrin clot formation by modulating human thrombin activity via exosite I. Pathog Dis (2015) 73. doi: 10.1093/femspd/ftv001
90. Lin YP, Chang YF. A domain of the Leptospira LigB contributes to high affinity binding of fibronectin. Biochem Biophys Res Commun (2007) 362:443–8. doi: 10.1016/j.bbrc.2007.07.196
91. Hsieh CL, Chang E, Tseng A, Ptak C, Wu LC, Su CL, et al. Leptospira immunoglobulin-like protein B (LigB) binds to both the C-terminal 23 amino acids of fibrinogen αC domain and factor XIII: Insight into the mechanism of LigB-mediated blockage of fibrinogen α chain cross-linking. PloS Negl Trop Dis (2016) 10:e0004974. doi: 10.1371/journal.pntd.0004974
92. Lin YP, McDonough SP, Sharma Y, Chang YF. Leptospira immunoglobulin-like protein B (LigB) binding to the C-terminal fibrinogen αC domain inhibits fibrin clot formation, platelet adhesion and aggregation. Mol Microbiol (2011) 79:1063–76. doi: 10.1111/j.1365-2958.2010.07510.x
93. Lin YP, Greenwood A, Nicholson LK, Sharma Y, McDonough SP, Chang YF. Fibronectin binds to and induces conformational change in a disordered region of leptospiral immunoglobulin-like protein B. J Biol Chem (2009) 284:23547–57. doi: 10.1074/jbc.M109.031369
94. Ching AT, Favaro RD, Lima SS, Chaves Ade A, de Lima MA, Nader HB, et al. Leptospira interrogans shotgun phage display identified LigB as a heparin-binding protein. Biochem Biophys Res Commun (2012) 427:774–9. doi: 10.1016/j.bbrc.2012.09.137
95. Schwarz-Linek U, Werner JM, Pickford AR, Gurusiddappa S, Kim JH, Pilka ES, et al. Pathogenic bacteria attach to human fibronectin through a tandem β-zipper. Nature (2003) 423:177–81.
96. Croda J, Ramos JG, Matsunaga J, Queiroz A, Homma A, Riley LW, et al. Leptospira immunoglobulin-like proteins as a serodiagnostic marker for acute leptospirosis. J Clin Microbiol (2007) 45:1528–34. doi: 10.1128/JCM.02344-06
97. Srimanote P, Wongdeethai N, Jieanampunkul P, Samonkiert S, Leepiyasakulchai C, Kalambaheti T, et al. Recombinant LigA for leptospirosis diagnosis and LigA among the Leptospira spp. clinical isolates. J Microbiol Methods (2008) 72:73–81. doi: 10.1016/j.mimet.2007.10.012
98. Kitashoji E, Koizumi N, Lacuesta TL, Usuda D, Ribo MR, Tria ES, et al. Diagnostic accuracy of recombinant immunoglobulin-like protein A-based IgM ELISA for the early diagnosis of leptospirosis in the Philippines. PloS Negl Trop Dis (2015) 9:e0003879. doi: 10.1371/journal.pntd.0003879
99. Thome S, Lessa-Aquino C, Ko AI, Lilenbaum W, Medeiros MA. Identification of immunodominant antigens in canine leptospirosis by Multi-Antigen Print ImmunoAssay (MAPIA). BMC Vet Res (2014) 10:288. doi: 10.1186/s12917-014-0288-2
100. Yan W, Saleem MH, McDonough P, McDonough SP, Divers TJ, Chang YF. Development of an enzyme-linked immunosorbent assay using a recombinant LigA fragment comprising repeat domains 4 to 7.5 as an antigen for diagnosis of equine leptospirosis. Clin Vaccine Immunol (2013) 20:1143–9. doi: 10.1128/CVI.00245-13
101. Ye C, Yan W, McDonough PL, McDonough SP, Mohamed H, Divers TJ, et al. Serodiagnosis of equine leptospirosis by enzyme-linked immunosorbent assay using four recombinant protein markers. Clin Vaccine Immunol (2014) 21:478–83. doi: 10.1128/CVI.00649-13
102. Lessa-Aquino C, Borges Rodrigues C, Pablo J, Sasaki R, Jasinskas A, Liang L, et al. Identification of seroreactive proteins of Leptospira interrogans serovar Copenhageni using a high-density protein microarray approach. PloS Negl Trop Dis (2013) 7:e2499. doi: 10.1371/journal.pntd.0002499
103. Lessa-Aquino C, Lindow JC, Randall A, Wunder E, Pablo J, Nakajima R, et al. Distinct antibody responses of patients with mild and severe leptospirosis determined by whole proteome microarray analysis. PloS Negl Trop Dis (2017) 11:e0005349. doi: 10.1371/journal.pntd.0005349
104. Silva EF, Medeiros MA, McBride AJ, Matsunaga J, Esteves GS, Ramos JG, et al. The terminal portion of leptospiral immunoglobulin-like protein LigA confers protective immunity against lethal infection in the hamster model of leptospirosis. Vaccine (2007) 25:6277–86. doi: 10.1016/j.vaccine.2007.05.053
105. Faisal SM, Yan W, Chen CS, Palaniappan RU, McDonough SP, Chang YF. Evaluation of protective immunity of Leptospira immunoglobulin like protein A (LigA) DNA vaccine against challenge in hamsters. Vaccine (2008) 26:277–87. doi: 10.1016/j.vaccine.2007.10.029
106. Faisal SM, Yan W, McDonough SP, Chang YF. Leptospira immunoglobulin-like protein A variable region (LigAvar) incorporated in liposomes and PLGA microspheres produces a robust immune response correlating to protective immunity. Vaccine (2009) 27:378–87. doi: 10.1016/j.vaccine.2008.10.089
107. Yan W, Faisal SM, McDonough SP, Divers TJ, Barr SC, Chang CF, et al. Immunogenicity and protective efficacy of recombinant Leptospira immunoglobulin-like protein B (rLigB) in a hamster challenge model. Microbes Infect (2009) 11:230–7. doi: 10.1016/j.micinf.2008.11.008
108. Cao Y, Faisal SM, Yan W, Chang YC, McDonough SP, Zhang N, et al. Evaluation of novel fusion proteins derived from extracellular matrix binding domains of LigB as vaccine candidates against leptospirosis in a hamster model. Vaccine (2011) 29:7379–86. doi: 10.1016/j.vaccine.2011.07.070
109. Coutinho ML, Choy HA, Kelley MM, Matsunaga J, Babbitt JT, Lewis MS, et al. A LigA three-domain region protects hamsters from lethal infection by Leptospira interrogans. PloS Negl Trop Dis (2011) 5:e1422. doi: 10.1371/journal.pntd.0001422
110. Lucas DS, Cullen PA, Lo M, Srikram A, Sermswan RW, Adler B. Recombinant LipL32 and LigA from Leptospira are unable to stimulate protective immunity against Leptospirosis in the hamster model. Vaccine (2011) 29:3413–8. doi: 10.1016/j.vaccine.2011.02.084
111. Forster KM, Hartwig DD, Seixas FK, Bacelo KL, Amaral M, Hartleben CP, et al. A conserved region of leptospiral immunoglobulin-like A and B proteins as a DNA vaccine elicits a prophylactic immune response against leptospirosis. Clin Vaccine Immunol (2013) 20:725–31. doi: 10.1128/CVI.00601-12
112. Lourdault K, Wang LC, Vieira A, Matsunaga J, Melo R, Lewis MS, et al. Oral immunization with Escherichia coli expressing a lipidated form of LigA protects hamsters against challenge with Leptospira interrogans serovar Copenhageni. Infect Immun (2014) 82:893–902. doi: 10.1128/IAI.01533-13
113. Hartwig DD, Bacelo KL, Oliveira PD, Oliveira TL, Seixas FK, Amaral MG, et al. Mannosylated LigANI produced in Pichia pastoris protects hamsters against leptospirosis. Curr Microbiol (2014) 68:524–30. doi: 10.1007/s00284-013-0505-4
114. Bacelo KL, Hartwig DD, Seixas FK, Schuch R, Moreira Ada S, Amaral M, et al. Xanthan gum as an adjuvant in a subunit vaccine preparation against leptospirosis. BioMed Res Int (2014) 2014:636491. doi: 10.1155/2014/636491
115. Forster KM, Hartwig DD, Oliveira TL, Bacelo KL, Schuch R, Amaral MG, et al. DNA prime-protein boost based vaccination with a conserved region of leptospiral immunoglobulin-like A and B proteins enhances protection against leptospirosis. Mem Inst Oswaldo Cruz (2015) 110:989–95. doi: 10.1590/0074-02760150222
116. Oliveira TL, Bacelo KL, Schuch RA, Seixas FK, Collares T, Rodrigues OE, et al. Immune response in hamsters immunised with a recombinant fragment of LigA from Leptospira interrogans, associated with carrier molecules. Mem Inst Oswaldo Cruz (2016) 111:712–6. doi: 10.1590/0074-02760160214
117. Conrad NL, Cruz McBride FW, Souza JD, Silveira MM, Felix S, Mendonca KS, et al. LigB subunit vaccine confers sterile immunity against challenge in the hamster model of leptospirosis. PloS Negl Trop Dis (2017) 11:e0005441. doi: 10.1371/journal.pntd.0005441
118. Evangelista KV, Lourdault K, Matsunaga J, Haake DA. Immunoprotective properties of recombinant LigA and LigB in a hamster model of acute leptospirosis. PloS One (2017) 12:e0180004. doi: 10.1371/journal.pone.0180004
119. da Cunha CEP, Bettin EB, Bakry A, Seixas Neto ACP, Amaral MG, Dellagostin OA. Evaluation of different strategies to promote a protective immune response against leptospirosis using a recombinant LigA and LigB chimera. Vaccine (2019) 37:1844–52. doi: 10.1016/j.vaccine.2019.02.010
120. Techawiwattanaboon T, Barnier-Quer C, Palaga T, Jacquet A, Collin N, Sangjun N, et al. Reduced renal colonization and enhanced protection by leptospiral factor H binding proteins as a multisubunit vaccine against leptospirosis in hamsters. Vaccines (Basel) (2019) 7. doi: 10.3390/vaccines7030095
121. Techawiwattanaboon T, Barnier-Quer C, Palaga T, Jacquet A, Collin N, Sangjun N, et al. A comparison of intramuscular and subcutaneous administration of LigA subunit vaccine adjuvanted with neutral liposomal formulation containing monophosphoryl lipid A and QS21. Vaccines (Basel) (2020) 8. doi: 10.3390/vaccines8030494
122. Palaniappan RU, McDonough SP, Divers TJ, Chen CS, Pan MJ, Matsumoto M, et al. Immunoprotection of recombinant leptospiral immunoglobulin-like protein A against Leptospira interrogans serovar Pomona infection. Infect Immun (2006) 74:1745–50. doi: 10.1128/IAI.74.3.1745-1750.2006
123. Shah RR, Hassett KJ, Brito LA. Overview of vaccine adjuvants: Introduction, history, and current status. Methods Mol Biol (2017) 1494:1–13. doi: 10.1007/978-1-4939-6445-1_1
124. Chase MW. The cellular transfer of cutaneous hypersensitivity to tuberculin. Proc Soc Exp Biol Med (1945) 59:134–5. doi: 10.3181/00379727-59-15006P
125. Shibaki A, Katz SI. Induction of skewed Th1/Th2 T-cell differentiation via subcutaneous immunization with Freund’s adjuvant. Exp Dermatol (2002) 11:126–34. doi: 10.1034/j.1600-0625.2002.110204.x
126. Shenderov K, Barber DL, Mayer-Barber KD, Gurcha SS, Jankovic D, Feng CG, et al. Cord factor and peptidoglycan recapitulate the Th17-promoting adjuvant activity of mycobacteria through mincle/CARD9 signaling and the inflammasome. J Immunol (2013) 190:5722–30. doi: 10.4049/jimmunol.1203343
127. Gavin AL, Hoebe K, Duong B, Ota T, Martin C, Beutler B, et al. Adjuvant-enhanced antibody responses in the absence of Toll-like receptor signaling. Science (2006) 314:1936–8. doi: 10.1126/science.1135299
128. Shi S, Zhu H, Xia X, Liang Z, Ma X, Sun B. Vaccine adjuvants: Understanding the structure and mechanism of adjuvanticity. Vaccine (2019) 37:3167–78. doi: 10.1016/j.vaccine.2019.04.055
129. HogenEsch H, O’Hagan DT, Fox CB. Optimizing the utilization of aluminum adjuvants in vaccines: you might just get what you want. NPJ Vaccines (2018) 3:51. doi: 10.1038/s41541-018-0089-x
130. Coffman RL, Sher A, Seder RA. Vaccine adjuvants: putting innate immunity to work. Immunity (2010) 33:492–503. doi: 10.1016/j.immuni.2010.10.002
131. Reed SG, Orr MT, Fox CB. Key roles of adjuvants in modern vaccines. Nat Med (2013) 19:1597–608. doi: 10.1038/nm.3409
132. Ross PJ, Sutton CE, Higgins S, Allen AC, Walsh K, Misiak A, et al. Relative contribution of Th1 and Th17 cells in adaptive immunity to Bordetella pertussis: towards the rational design of an improved acellular pertussis vaccine. PloS Pathog (2013) 9:e1003264. doi: 10.1371/journal.ppat.1003264
133. Lin L, Ibrahim AS, Xu X, Farber JM, Avanesian V, Baquir B, et al. Th1-Th17 cells mediate protective adaptive immunity against Staphylococcus aureus and Candida albicans infection in mice. PloS Pathog (2009) 5:e1000703. doi: 10.1371/journal.ppat.1000703
134. Geijtenbeek TB, Gringhuis SI. Signalling through C-type lectin receptors: Shaping immune responses. Nat Rev Immunol (2009) 9:465–79. doi: 10.1038/nri2569
135. Bramwell VW, Perrie Y. Particulate delivery systems for vaccines: What can we expect? J Pharm Pharmacol (2006) 58:717–28. doi: 10.1211/jpp.58.6.0002
136. Brunner R, Jensen-Jarolim E, Pali-Scholl I. The ABC of clinical and experimental adjuvants–a brief overview. Immunol Lett (2010) 128:29–35. doi: 10.1016/j.imlet.2009.10.005
137. De Serrano LO, Burkhart DJ. Liposomal vaccine formulations as prophylactic agents: design considerations for modern vaccines. J Nanobiotechnol (2017) 15:83. doi: 10.1186/s12951-017-0319-9
138. Bauer S, Kirschning CJ, Hacker H, Redecke V, Hausmann S, Akira S, et al. Human TLR9 confers responsiveness to bacterial DNA via species-specific CpG motif recognition. Proc Natl Acad Sci USA (2001) 98:9237–42. doi: 10.1073/pnas.161293498
139. Petrovsky N, Cooper PD. Carbohydrate-based immune adjuvants. Expert Rev Vaccines (2011) 10:523–37. doi: 10.1586/erv.11.30
140. Takeuchi O, Sato S, Horiuchi T, Hoshino K, Takeda K, Dong Z, et al. Cutting edge: Role of Toll-like receptor 1 in mediating immune response to microbial lipoproteins. J Immunol (2002) 169:10–4. doi: 10.4049/jimmunol.169.1.10
141. del Rio B, Seegers JF, Gomes-Solecki M. Immune response to Lactobacillus plantarum expressing Borrelia burgdorferi OspA is modulated by the lipid modification of the antigen. PloS One (2010) 5:e11199. doi: 10.1371/journal.pone.0011199
142. Suphatpahirapol C, Nguyen TH, Tansiri Y, Yingchutrakul Y, Roytrakul S, Nitipan S, et al. Expression of a leptospiral leucine-rich repeat protein using a food-grade vector in Lactobacillus plantarum, as a strategy for vaccine delivery. 3 Biotech (2019) 9:324. doi: 10.1007/s13205-019-1856-8
143. Santecchia I, Vernel-Pauillac F, Rasid O, Quintin J, Gomes-Solecki M, Boneca IG, et al. Innate immune memory through TLR2 and NOD2 contributes to the control of Leptospira interrogans infection. PloS Pathog (2019) 15:e1007811. doi: 10.1371/journal.ppat.1007811
144. Xu Y, Ye Q. Human leptospirosis vaccines in China. Hum Vaccin Immunother (2018) 14:984–93. doi: 10.1080/21645515.2017.1405884
145. Bouvet J, Lemaitre L, Cariou C, Scotto M, Blain C, Oberli F, et al. A canine vaccine against Leptospira serovars Icterohaemorrhagiae, Canicola and Grippotyphosa provides cross protection against Leptospira serovar Copenhageni. Vet Immunol Immunopathol (2020) 219:109985. doi: 10.1016/j.vetimm.2019.109985
146. Rosario LA, Arencibia DF, Suarez YE, Infante JF, Valdes BY, Batista N. Cross-protection among unrelated Leptospira pathogens serovars: an unfinished story. Adv Clin Exp Med (2012) 21:581–9.
147. Lauretti-Ferreira F, Silva PLD, Alcantara NM, Silva BF, Grabher I, Souza GO, et al. New strategies for Leptospira vaccine development based on LPS removal. PloS One (2020) 15:e0230460. doi: 10.1371/journal.pone.0230460
148. Srikram A, Zhang K, Bartpho T, Lo M, Hoke DE, Sermswan RW, et al. Cross-protective immunity against leptospirosis elicited by a live, attenuated lipopolysaccharide mutant. J Infect Dis (2011) 203:870–9. doi: 10.1093/infdis/jiq127
149. Sonrier C, Branger C, Michel V, Ruvoen-Clouet N, Ganiere JP, Andre-Fontaine G. Evidence of cross-protection within Leptospira interrogans in an experimental model. Vaccine (2000) 19:86–94. doi: 10.1016/s0264-410x(00)00129-8
150. Adler B. Vaccines against leptospirosis. Curr Top Microbiol Immunol (2015) 387:251–72. doi: 10.1007/978-3-662-45059-8_10
151. Liu MA. DNA vaccines: an historical perspective and view to the future. Immunol Rev (2011) 239:62–84. doi: 10.1111/j.1600-065X.2010.00980.x
152. Ledgerwood JE, Wei CJ, Hu Z, Gordon IJ, Enama ME, Hendel CS, et al. DNA priming and influenza vaccine immunogenicity: two phase 1 open label randomised clinical trials. Lancet Infect Dis (2011) 11:916–24. doi: 10.1016/S1473-3099(11)70240-7
153. Zuerner RL. Host response to Leptospira infection. Curr Top Microbiol Immunol (2015) 387:223–50. doi: 10.1007/978-3-662-45059-8_9
154. Vernel-Pauillac F, Werts C. Recent findings related to immune responses against leptospirosis and novel strategies to prevent infection. Microbes Infect (2018) 20:578–88. doi: 10.1016/j.micinf.2018.02.001
155. Devlin AA, Halvorsen PJ, Miller JC, Laster SM. Il-10 deficient mice express IFN-γ mRNA and clear Leptospira interrogans from their kidneys more rapidly than normal C57BL/6 mice. Immunobiology (2017) 222:768–77. doi: 10.1016/j.imbio.2017.02.004
Keywords: leptospira, leptospirosis, virulence, vaccine, adhesin, complement resistance, immunoglobulin-like domain, hemostasis
Citation: Haake DA and Matsunaga J (2021) Leptospiral Immunoglobulin-Like Domain Proteins: Roles in Virulence and Immunity. Front. Immunol. 11:579907. doi: 10.3389/fimmu.2020.579907
Received: 03 July 2020; Accepted: 05 November 2020;
Published: 08 January 2021.
Edited by:
Ana Lucia Nascimento, Butantan Institute, BrazilReviewed by:
Yi-Pin Lin, Wadsworth Center, United StatesMônica Larucci Vieira, Federal University of Minas Gerais, Brazil
Copyright © 2021 Haake and Matsunaga. This is an open-access article distributed under the terms of the Creative Commons Attribution License (CC BY). The use, distribution or reproduction in other forums is permitted, provided the original author(s) and the copyright owner(s) are credited and that the original publication in this journal is cited, in accordance with accepted academic practice. No use, distribution or reproduction is permitted which does not comply with these terms.
*Correspondence: David A. Haake, ZGhhYWtlQHVjbGEuZWR1