- Department of Ecology, University of São Paulo, São Paulo, Brazil
Wolbachia is a maternally transmitted bacterium that lives inside arthropod cells. Historically, it was viewed primarily as a parasite that manipulates host reproduction, but more recently it was discovered that Wolbachia can also protect Drosophila species against infection by RNA viruses. Combined with Wolbachia’s ability to invade insect populations due to reproductive manipulations, this provides a way to modify mosquito populations to prevent them transmitting viruses like dengue. In this review, we discuss the main advances in the field since Wolbachia’s antiviral effect was discovered 12 years ago, identifying current research gaps and potential future developments. We discuss that the antiviral effect works against a broad range of RNA viruses and depends on the Wolbachia lineage. We describe what is known about the mechanisms behind viral protection, and that recent studies suggest two possible mechanisms: activation of host immunity or competition with virus for cellular resources. We also discuss how association with Wolbachia may influence the evolution of virus defense on the insect host genome. Finally, we investigate whether the antiviral effect occurs in wild insect populations and its ecological relevance as a major antiviral component in insects.
Introduction
Wolbachia pipientis is a maternally transmitted alphaproteobacterium that lives obligatorily within the cytoplasm of arthropod cells (1). Until recently it was viewed primarily as a parasite that manipulates host reproduction, most commonly by inducing cytoplasmic incompatibility (2, 3). Cytoplasmic incompatibility allows Wolbachia to invade insect populations by causing embryonic mortality when uninfected females mate with infected males, thus conferring a selective advantage to infected females (4, 5). In 2008, two studies discovered that Wolbachia can protect Drosophila melanogaster against RNA viruses (6, 7). Subsequently, it was discovered that Wolbachia can block dengue virus replication in mosquitoes (8, 9). These findings provided a new way in which Wolbachia can be used to control human arboviruses, since previous attempts relied on using cytoplasmic incompatibility as a transgene driver, or reduction of mosquito longevity by a virulent Wolbachia strain. Wolbachia lineages from different insects that were transferred to the mosquito Aedes aegypti can limit the replication of arboviruses such as Dengue virus (DENV), Chikungunya virus (CHIKV), Yellow Fever virus (YFV), Zika virus (ZIKV) and West Nile virus (WNV) (9–12). Wolbachia can spread quickly through mosquito populations by cytoplasmic incompatibility (13–15), and large field trials have been successful in reducing dengue prevalence in human populations (16, 17).
In this Mini Review, we discuss the main advances in the field since the Wolbachia antiviral effect was discovered 12 years ago, current research gaps, and potential future developments. First, we address the generality of the antiviral effect and how it depends on Wolbachia lineage and on virus identity. Second, we discuss the possible mechanisms of antiviral protection. Third, we discuss how association with Wolbachia may influence the evolution of virus defense on the insect host genome. Finally, we discuss the virus blocking ecological relevance by addressing if it occurs in wild insect populations.
Generality: Different Viruses and Different Wolbachia Lineages
After the first studies showing that Wolbachia protects flies and mosquitoes against RNA viruses (6–8) and its potential to control insect-born human diseases (8–10, 14), there was a great interest in the area. Many studies conducted on mosquitoes tested for their vector competence and revealed that Wolbachia reduces infection and, in some cases, the dissemination and transmission of diseases such as dengue, chikungunya, yellow fever, zika, and West Nile fever (Table 1). In flies, Wolbachia protects mostly against Flock House virus (FHV), and Drosophila C virus (DCV). However, DCV is not commonly found in wild Drosophila populations (41) and there is limited information on protection against viruses that are common in nature, such as Nora (6) and Kallithea virus (36) (Table 1). Although many studies report Wolbachia protection against different viruses, there are a few cases in which Wolbachia provides no protection or even increases the host susceptibility to viral infection (Table 1). Furthermore, only three studies investigated Wolbachia protection against DNA viruses (6, 36, 40) and none found evidence of protection (Table 1). Therefore, Wolbachia protection in insects is a general phenomenon only against RNA viruses.
The level of protection against viruses varies among Wolbachia strains and depends on their density within the host (22, 42). It is common to transfer high density strains into new hosts, such as mosquitoes, to test for protection against viruses (Figure 1A). Thus, protection generally occurs in host-Wolbachia interactions that are not natural, but artificial (43). For example, the virulent strain wMelPop, originally isolated from D. melanogaster (44, 45), protects against different viruses in Aedes aegypti (Table 1). However, wMelPop is a strain that was identified only in laboratory and there is no record of it in nature. Other Wolbachia strains commonly used in experiments that have broad protection against viruses are wMel, wMelCS, both isolated from D. melanogaster, wAu, isolated from D. simulans, wAlbB, isolated from Aedes albopictus, and wStri, isolated from the planthooper Laodelphax striatellus (Table 1). Martinez and colleagues investigated antiviral protection in many Wolbachia strains originated from different Drosophila species after transfer into the same genetic background of D. simulans. Interestingly, they found that protection is not determined by host genotype, but by Wolbachia strain (23). All these studies showing that different strains protect different hosts against many RNA viruses were conducted in the laboratory, and there is still little evidence of the Wolbachia antiviral effect in nature (see last section below).
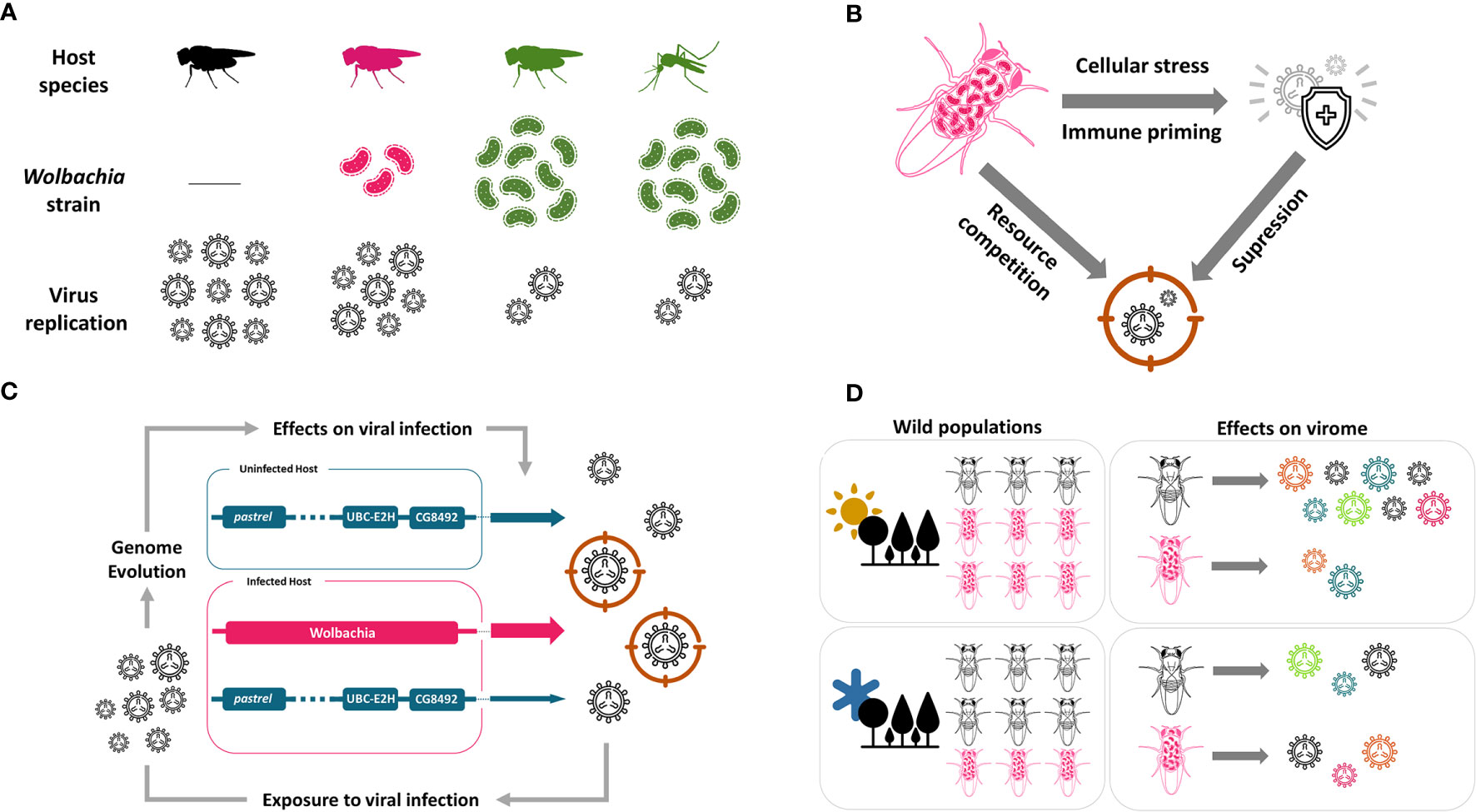
Figure 1 Wolbachia antiviral effect in insects. (A) Wolbachia protects insects against RNA viruses. The protection is dependent on Wolbachia density, which varies between strains. Strains can be experimentally transferred to new hosts, such as mosquitoes. (B) Wolbachia can activate host immune system in some cases, but the mechanism of defense can also be related to competition with virus for cellular resources. The specific mechanism is not yet known. (C) Host immune response fight against virus, but its action and evolution are slowed down in the presence of Wolbachia. Colored arrows and their width represent genome and its participation in antiviral effect, respectively. (D) Environmental conditions, as temperature, determine Wolbachia antiviral response. In hot climate, Wolbachia may have a more important role protecting the host, and this can lead to higher Wolbachia prevalence on hot climate regions. But it is not yet known if Wolbachia reduces the virome in wild insect populations. This figure is made in conjunction with icons provided by thenounproject.com. The icons are: “Bacteria” by farra nugraha; “Virus” by KonKapp; “Immune System” by Bartama Graphic; “Immunity” by Timofey Rostilov; “Forest” by ProSymbols; “Sun” by Alice Design; and, “Cold” by Landan Lloyd.
Another issue is that most studies that test for virus protection by Wolbachia are carried out using only the adult stage. So far, only Graham et al. (40) tested for viral protection in larval stages of Spodoptera exempta, and we still have no information of protection on pupae. Moreover, these results may be affected by the inoculation method in the laboratory. All studies in flies use systemic infection (stabbing or microinjection), while in mosquitoes some studies use oral infection besides microinjection. Although methods such as microinjection allow greater viral dose precision, we know that in nature insects acquire many pathogens by feeding (46, 47). Therefore, although there is a general pattern of protection against viruses in laboratory studies, there are some limitations on the methods used. Further studies testing Wolbachia’s antiviral protection in insect host using methods that approximate of how infections occur in nature, such as oral infection (46, 47), are essential to understanding the dynamics between Wolbachia and viruses in wild populations.
Wolbachia infects about 50% of all insect species (48), and we can hypothesize that the antiviral protection may be one of the reasons for Wolbachia being so widely spread among arthropods. However, studies on Wolbachia’s viral protection are still limited to flies and mosquitoes, with the exception of one study on a Lepidoptera host (40) and one study on a Hemiptera host (33). Thus, more studies on different insect families are essential to test if the antiviral effect also occurs in other insects, and how likely it may be one of the main reasons for the high prevalence of Wolbachia in natural insect populations.
The Possible Mechanisms
Since the discovery of Wolbachia antiviral protection different mechanisms of action have been proposed, but up to now, there is no consensus on the underlying mechanism [reviewed by Lindsey et al (49)]. Current studies work on two main hypotheses to explain Wolbachia interference in viral replication: the activation of host immunity and competition with virus for cellular resources (Figure 1B).
The first hypothesis is that Wolbachia can directly activate innate immunity of the host prior to virus infection (immune priming), interfering with virus replication. The presence of the bacterium in host cells leads to cellular stress, including oxidative stress that activates host immune pathways (50). Wolbachia preactivates mosquito innate immunity by the oxidative stress, upregulating Toll pathway genes, known to be responsible for protection against dengue virus (8, 9, 50). Immune effector genes upregulation in A. aegypti suggests that the protection due to immunity priming is responsible for the viral interference (8, 9). However, the upregulation in the immune pathway genes is variable in different species and it seems to be influenced by the time of host-Wolbachia coevolution. For instance, there is no upregulation on Toll or IMD genes by Wolbachia in its native host Aedes fluviatilis, but other immune-related genes are indeed modulated, as oxidative stress-related genes (51). The generation of oxygen reactive species itself is an example of immune response that vary between novel and native host, ranging from triggering oxidative stress to redox homeostasis restoration [reviewed by Zug and Hammerstein (52)]. But there is evidence that Wolbachia-induced oxidative stress is involved in virus blocking both in transinfected mosquito and Drosophila with a natural Wolbachia infection (50, 53).
The second hypothesis is that resources shared by Wolbachia and the virus can represent a limitation for development of the latter when they are co-infecting their host. As discussed in the previous section, Wolbachia protects mainly against RNA viruses which depends on specific cellular resources, the integrity of intracellular membranes for replication, and the host translation apparatus for virus protein production (49). Any disturbance caused by Wolbachia on these cellular components presumably interferes with virus replication. For instance, depletion, reduction, or modification of certain host lipids affect virus replication (54, 55). In particular for cholesterol, providing or restoring its intracellular traffic recover virus replication in a Wolbachia-infected host, indicating both the role of cholesterol in virus development and Wolbachia interference in host lipid availability (55, 56). In another recent example, it was found that Wolbachia and virus have antagonistic effect in the host expression of prat2, a gene involved in nucleotide synthesis (57).
Additionally, several approaches have shown that antiviral protection occurs in host bearing high density of Wolbachia, with no detectable protection is host with low symbiont density (22, 24). The same result is obtained in experimental manipulation of Wolbachia density with antibiotics (58). The control of symbiont density is dependent on the symbiont genotype and, in the case of Wolbachia strains isolated from D. melanogaster, the genetic basis of density determination has been assigned to the Octomom region which presents several duplications, or a deletion of the entire region, in high-density symbionts (59–61). However, one recent study with controlled genetic background showed an intriguing example of Wolbachia with no antiviral action in A. aegypti, even in relatively high density (62). Other than density, host development stage and temperature seem to modulate Wolbachia antiviral properties (61, 63).
The mechanism behind Wolbachia antiviral protection became an active area of research. New experimental approaches, such as the forward genetic screens applicable on genetically intractable bacteria (61), are extremely promising to pursue this question. One example of how recent experimental advances can bring progress to long standing questions is the case of cytoplasmic incompatibility caused by Wolbachia. Cytoplasmic incompatibility has been studied since 1971, yet only recently its mechanism was uncovered (1, 64, 65). The cytoplasmic incompatibility is controlled by two phage WO genes, cifAwMel and cifBwMel, present in the Wolbachia genome (66). Similar advances are likely to figure out the specific antiviral mechanism in the following years.
Influence on Evolution of Host “INTRINSIC” Immunological Resistance Mechanisms
Although Wolbachia confers viral protection to insects, natural insect populations have other means to fight against viruses (67, 68). Insects usually rely on the mechanisms of RNA interference, apoptosis, NF-κB pathways and translation control from its innate immune system to get along viral pathogens (69). Nevertheless, the population’s ability to resist the plethora of viruses present in nature lies on its standing genetic variation on these mechanisms or the sudden appearance of beneficial mutations (70). However, in the presence of Wolbachia, the extended mutualistic genotype could mask or even substitute host’s intrinsic mechanisms of antiviral defenses, shifting its adaptive landscape (71) (Figure 1C). Some recent experimental evolution studies have addressed how the presence of Wolbachia can alter the evolution of intrinsic antiviral mechanisms in insects.
In a pioneer study, Martins and colleagues used an experimental evolution approach in which Drosophila melanogaster populations were subjected to continuous DCV injections for a few generations (72). Compared with control populations that were not exposed to the virus, infected populations showed increased survival after DCV infection, and also increased survival after infection by cricket paralysis virus (CrPV) and FHV (72). This increased resistance to viral infection was associated with three candidate genes on the fly’s genome - pastrel, Ubc-E2H and CG8492 (72). In another experimental evolution study, Martinez and colleagues directly tested how the presence of Wolbachia can alter evolution of intrinsic antiviral mechanisms (71). They focused on a polymorphism of the gene pastrel that explains most of the variation on DCV resistance in D. melanogaster populations (73, 74). They infected populations with and without Wolbachia for nine generations. Resistance to DCV and the frequency of the resistant pastrel allele increased in all populations exposed to the virus compared with virus-free control populations (71). Most interestingly, the frequency of the resistant pastrel allele after nine generations was lower in Wolbachia infected populations than in the symbiont-free populations. After experimentally removing Wolbachia, the populations that had Wolbachia during the selection experiment was much less resistant to the virus than the Wolbachia-free populations. This experiment shows that the presence of Wolbachia resulted in weaker selection on the host intrinsic antiviral defenses, making the host addicted to the protection caused by the symbiont (71). Another study showed that DCV infection selected for a particular Wolbachia strain that enhances survival and fecundity in the presence of DCV (75). Finally, Faria and colleagues showed that intrinsic antiviral defenses can replace symbiont protection (72, 76). They used previously selected populations for increased virus resistance (72), and removed the symbiont from these populations. They first observed a severe drop in survival after DCV infection, but resistance significantly increased in subsequent generations reaching the same levels as seen in the presence of Wolbachia after 20 generations (76).
These studies show that Wolbachia can change the strength of selection on host antiviral mechanisms, leading to evolutionary addiction (71, 72, 75, 76). Because Wolbachia prevalence varies in natural populations, this may be one mechanism that maintains genetic variation in intrinsic antiviral resistance in populations (76). One interesting interplay is that different Drosophila clades respond differently to viral infections (77), therefore, variation in resistance and susceptibility of hosts could be mirrored by the success and establishment of Wolbachia in some clades but not others in nature (78). In addition, it would be remarkably interesting to investigate how the presence of Wolbachia in some clades may affect the evolution of host-shifts by viruses (79).
Importance in Wild Populations
The Wolbachia antiviral effects were intensely studied in the last decade because of its importance in the field of public health. However, their ecological importance in wild populations has rarely being addressed. Around 50% of insect species may carry one or more strains of Wolbachia (48), meaning that almost 3 million insect species are infected. Therefore, Wolbachia may be a major component of antiviral defenses in nature (43). But just recently some studies started to test if Wolbachia can confer protection against viruses in wild insect populations. The antiviral effects of Wolbachia may mean that in nature it is frequently a mutualist that protects its host against infection. This may explain why Wolbachia strains that do not cause cytoplasmic incompatibility and have no obvious phenotypic effect can invade and be maintained in populations (80). Theory predicts that cytoplasmic incompatibility can only invade when local infection frequencies becomes sufficiently high to offset imperfect maternal transmission and infection costs (81, 82). However, recent data suggested that Wolbachia can spread from arbitrarily low frequencies (80). In this scenario, there appears to be a fitness advantage for the host caused by Wolbachia in natural populations (83). This fitness advantage may be Wolbachia antiviral effects. This is expected by the studies carried out in the laboratory showing the antiviral effect, but just now some studies started to test this in wild populations. It is interesting to notice that Wolbachia can also protect insects against bacteria and entomopathogenic fungi (84–86), and this can also add to the possible mutualistic effect in natural populations.
Drosophila flies have been used as the main model to study insect virus interactions, but until recently we knew extraordinarily little about the virus community that infect wild Drosophila populations. This is changing rapidly with recent studies using metagenomic approaches (87). In 2015, Webster and colleagues used metagenomic techniques in more than 2000 wild collect Drosophila melanogaster flies and discovered more than 20 new viruses (41). They found a high prevalence of virus infection with more than 30% of the wild collected individuals carrying a virus. There was also large variation in prevalence among the 17 sampled locations across the world. Because Wolbachia prevalence in these locations varied from 1.6% to 98% - with a mean of about 50% - they tested for associations between the prevalence of Wolbachia and the different viruses among and within populations. They could not find any association, indicating that Wolbachia is not an important determinant of virus incidence in the wild (41). However, as pointed by the authors, they had a small sample size per population resulting in low statistical power to detect an association. In addition, they looked only on the effect of Wolbachia on prevalence, but Wolbachia can also be influencing virus titer on infected flies.
In 2018, Shi and colleagues tested the effect of Wolbachia on viral abundance on six D. melanogaster populations sampled in Australia (88). They first sequenced total transcriptome of pools of Wolbachia-infected and Wolbachia-free lines to estimate viral abundance. Despite finding high RNA virus’ abundance in all pools, they did not find any Wolbachia protective effect. They also sequenced the transcriptome of individual Wolbachia-infected and Wolbachia-free flies from one location, but again did not find any Wolbachia protective effect (88). These results should be interpreted with caution as well, since they sequenced only 122 flies in the pools, plus 40 individual flies. Given the large variation among pools in viral abundance and in the prevalence that varied from two to five viruses per pool, the statistical power to detect an effect was low. Additionally, they did not sequence wild collected flies, but F1 or F3 of laboratory cultured lines that were kept at 19°C. Unfortunately, it was discovered, very recently, that the antiviral effect of the Wolbachia strain wMel in D. melanogaster depends on temperature (63). The strong protection observed when flies develop from egg to adult at 25°C is greatly reduced or disappear when flies develop at 18°C (63). Therefore, the development conditions used by Shi et al. may have masked any possible Wolbachia protective effect.
Interestingly, the recent study on the effect of temperature on the Wolbachia antiviral effect (63) offers a hint on this puzzle. It is interesting that the Wolbachia antiviral effect observed at high development temperature is extremely reduced when flies develop at low temperatures. This was observed with different genotypes of D. melanogaster, different Wolbachia lineages, and different viruses, suggesting this is a general phenomenon (63). These results suggest that in nature the mutualistic effect of virus protection will vary geographically and seasonally depending on climate, and this will result in the prevalence of Wolbachia being higher in tropical regions (Figure 1D). This is indeed what is observed in nature, where the frequency of Wolbachia is generally higher in populations from tropical regions (89). This pattern, although only a correlative suggestion, indicates that the antiviral protection may be the mutualistic effect in natural populations responsible for the widespread success of Wolbachia.
Conclusions
Since the Wolbachia antiviral effect in insects was discovered 12 years ago (6, 7), researchers have intensely studied this phenomenon. Wolbachia has even been successfully used to control the prevalence of human arboviruses, such as dengue, in mosquito populations (16, 17, 90). We learned a lot about the basic biology of the host-Wolbachia-virus interaction, but there are still many knowledge gaps. We now know the antiviral effect depends on Wolbachia strain, with only high-density strains having the antiviral effect. However, it is still unknown whether the antiviral effect occurs in insect species other than mosquitoes, flies and a planthopper. Importantly, the specific mechanism underlying antiviral protection has not been fully elucidated; upregulation of the host immune system or competition between Wolbachia and RNA viruses inside the host cell for some yet unknown resource necessary for virus replication are likely hypothesis (49, 52, 56). We have also learned that Wolbachia can alter the intensity of selection on host antiviral defenses, making the host more dependent on the symbiont for protection (71). We still do not know if the antiviral effect occurs in natural populations of insects and if it is the major mutualistic effect responsible for the extremely high prevalence of Wolbachia in insects. If it does, Wolbachia may be a major component of antiviral defense in nature.
Author Contributions
AP, CC, MM, and RC wrote the paper. All authors contributed to the article and approved the submitted version.
Funding
Funding for this work was provided by São Paulo Research Foundation (FAPESP) (2013/25991-0, 2015/08307-3, 2018/01295-8 and 2019/03997-2), CNPq (154568/2018-0, 307015/2015-7 and 307447/2018-9), and a Newton Advanced Fellowship from the Royal Society.
Conflict of Interest
The authors declare that the research was conducted in the absence of any commercial or financial relationships that could be construed as a potential conflict of interest.
Acknowledgments
The authors thank Camila Beraldo and Murillo Rodrigues for suggestions on the manuscript.
References
1. Werren JH. Biology of Wolbachia. Annu Rev Entomol (1997) 42:587–609. doi: 10.1146/annurev.ento.42.1.587
2. Bourtzis K, Nirgianaki A, Markakis G, Savakis C. Wolbachia infection and cytoplasmic incompatibility in Drosophila species. Genetics (1996).
3. Yen JH, Barr AR. The etiological agent of cytoplasmic incompatibility in Culex pipiens. J Invertebr Pathol (1973). doi: 10.1016/0022-2011(73)90141-9
4. Werren JH, Baldo L, Clark ME. Wolbachia: Master manipulators of invertebrate biology. Nat Rev Microbiol (2008). doi: 10.1038/nrmicro1969
5. Turelli M, Hoffmann AA. Rapid spread of an inherited incompatibility factor in California Drosophila. Nature (1991). doi: 10.1038/353440a0
6. Teixeira L, Ferreira Á, Ashburner M. The bacterial symbiont Wolbachia induces resistance to RNA viral infections in Drosophila melanogaster. PloS Biol (2008). doi: 10.1371/journal.pbio.1000002
7. Hedges LM, Brownlie JC, O’Neill SL, Johnson KN. Wolbachia and virus protection in insects. Sci (80- ) (2008). doi: 10.1126/science.1162418
8. Bian G, Xu Y, Lu P, Xie Y, Xi Z. The endosymbiotic bacterium Wolbachia induces resistance to dengue virus in Aedes aegypti. PloS Pathog (2010) 6:e1000833. doi: 10.1371/journal.ppat.1000833
9. Moreira LA, Iturbe-Ormaetxe I, Jeffery JA, Lu G, Pyke AT, Hedges LM, et al. A Wolbachia Symbiont in Aedes aegypti Limits Infection with Dengue, Chikungunya, and Plasmodium. Cell (2009) 139:1268–78. doi: 10.1016/j.cell.2009.11.042
10. van den Hurk AF, Hall-Mendelin S, Pyke AT, Frentiu FD, McElroy K, Day A, et al. Impact of Wolbachia on Infection with Chikungunya and Yellow FeverViruses in the Mosquito Vector Aedes aegypti. PloS Negl Trop Dis (2012) 6:e1892. doi: 10.1371/journal.pntd.0001892
11. Aliota MT, Peinado SA, Velez ID, Osorio JE. The wMel strain of Wolbachia Reduces Transmission of Zika virus by Aedes aegypti. Sci Rep (2016). doi: 10.1038/srep28792
12. Glaser RL, Meola MA. The native Wolbachia endosymbionts of Drosophila melanogaster and Culex quinquefasciatus increase host resistance to west nile virus infection. PloS One (2010). doi: 10.1371/journal.pone.0011977
13. Hoffmann AA, Montgomery BL, Popovici J, Iturbe-Ormaetxe I, Johnson PH, Muzzi F, et al. Successful establishment of Wolbachia in Aedes populations to suppress dengue transmission. Nature (2011). doi: 10.1038/nature10356
14. Walker T, Johnson PH, Moreira LA, Iturbe-Ormaetxe I, Frentiu FD, McMeniman CJ, et al. The wMel Wolbachia strain blocks dengue and invades caged Aedes aegypti populations. Nature (2011). doi: 10.1038/nature10355
15. Xi Z, Khoo CCH, Dobson SL. Ecology: Wolbachia establishment and invasion in an Aedes Aegypti laboratory population. Sci (80- ) (2005). doi: 10.1126/science.1117607
16. Ryan PA, Turley AP, Wilson G, Hurst TP, Retzki K, Brown-Kenyon J, et al. Establishment of wMel Wolbachia in Aedes aegypti mosquitoes and reduction of local dengue transmission in Cairns and surrounding locations in northern Queensland, Australia. Gates Open Res (2020). doi: 10.12688/gatesopenres.13061.2
17. Anders KL, Indriani C, Tantowijoyo W, Rancès E, Andari B, Prabowo E, et al. Reduced dengue incidence following deployments of Wolbachia-infected Aedes aegypti in Yogyakarta, Indonesia: A quasi-experimental trial using controlled interrupted time series analysis. Gates Open Res (2020). doi: 10.12688/gatesopenres.13122.1
18. Bian G, Joshi D, Dong Y, Lu P, Zhou G, Pan X, et al. Wolbachia invades Anopheles stephensi populations and induces refractoriness to Plasmodium infection. Sci (80- ) (2013). doi: 10.1126/science.1236192
19. Ant TH, Herd CS, Geoghegan V, Hoffmann AA, Sinkins SP. The Wolbachia strain wAu provides highly efficient virustransmission blocking in Aedes aegypti. PloS Pathog (2018) 14:e1006815. doi: 10.1371/journal.ppat.1006815
20. Joubert DA, Walker T, Carrington LB, De Bruyne JT, Kien DHT, Hoang NLT, et al. Establishment of a Wolbachia Superinfection in Aedes aegypti Mosquitoes as a Potential Approach for Future Resistance Management. PloS Pathog (2016) 12:e1005434. doi: 10.1371/journal.ppat.1005434
21. Mousson L, Zouache K, Arias-Goeta C, Raquin V, Mavingui P, Failloux AB. The Native Wolbachia Symbionts Limit Transmission of Dengue Virus inAedes albopictus. PloS Negl Trop Dis (2012) 6:e1989. doi: 10.1371/journal.pntd.0001989
22. Martinez J, Longdon B, Bauer S, Chan YS, Miller WJ, Bourtzis K, et al. Symbionts Commonly Provide Broad Spectrum Resistance to Viruses inInsects: A Comparative Analysis of Wolbachia Strains. PloS Pathog (2014) 10:e1004369. doi: 10.1371/journal.ppat.1004369
23. Martinez J, Tolosana I, Ok S, Smith S, Snoeck K, Day JP, et al. Symbiont strain is the main determinant of variation inWolbachia-mediated protection against viruses across Drosophila species. MolEcol (2017) 26:4072–84. doi: 10.1111/mec.14164
24. Osborne SE, Leong YS, O’Neill SL, Johnson KN. Variation in antiviral protection mediated by different Wolbachiastrains in Drosophila simulans. PloS Pathog (2009) 5:e1000656. doi: 10.1371/journal.ppat.1000656
25. Amuzu HE, Tsyganov K, Koh C, Herbert RI, Powell DR, McGraw EA. Wolbachia enhances insect-specific flavivirus infection in Aedes aegypti mosquitoes. Ecol Evol (2018) 8:5441–54. doi: 10.1002/ece3.4066
26. Blagrove MSC, Arias-Goeta C, Failloux A-B, Sinkins SP. Wolbachia strain wMel induces cytoplasmic incompatibility and blocks dengue transmission in Aedes albopictus. Proc Natl Acad Sci (2012) 109:255–60. doi: 10.1073/pnas.1112021108
27. Fraser JE, De Bruyne JT, Iturbe-Ormaetxe I, Stepnell J, Burns RL, Flores HA, et al. Novel Wolbachia-transinfected Aedes aegypti mosquitoes possess diverse fitness and vector competence phenotypes. PloS Pathog (2017) 13:e1006751. doi: 10.1371/journal.ppat.1006751
28. Hussain M, Lu G, Torres S, Edmonds JH, Kay BH, Khromykh AA, et al. Effect of Wolbachia on Replication of West Nile Virus in a MosquitoCell Line and Adult Mosquitoes. J Virol (2013)87:851–8. doi: 10.1128/JVI.01837-12
29. Ye YH, Carrasco AM, Dong Y, Sgrò CM, McGraw EA. The effect of temperature on Wolbachia-mediated dengue virusblocking in Aedes aegypti. Am J Trop Med Hyg (2016)94:812–9. doi: 10.4269/ajtmh.15-0801
30. Rancès E, Ye YH, Woolfit M, McGraw EA, O’Neill SL. The relative importance of innate immune priming inWolbachia-mediated dengue interference. PloS Pathog (2012) 8:e1002548. doi: 10.1371/journal.ppat.1002548
31. Unckless RL, Jaenike J. Maintenance of a male-killing wolbachia in drosophila innubila by male-killing dependent and male-killing independent mechanisms. Evol (N Y) (2012) 66:678–89. doi: 10.1111/j.1558-5646.2011.01485.x
32. Cattel J, Martinez J, Jiggins F, Mouton L, Gibert P. Wolbachia-mediated protection against viruses in the invasive pest Drosophila suzukii. Insect Mol Biol (2016) 25:595–603. doi: 10.1111/imb.12245
33. Gong JT, Li Y, Li TP, Liang Y, Hu L, Zhang D, et al. Stable Introduction of Plant-Virus-Inhibiting Wolbachia intoPlanthoppers for Rice Protection. Curr Biol (2020)30:4837–45. doi: 10.1016/j.cub.2020.09.033
34. Altinli M, Soms J, Ravallec M, Justy F, Bonneau M, Weill M, et al. Sharing cells with Wolbachia: the transovarian vertical transmission of Culex pipiens densovirus. Environ Microbiol (2019). doi: 10.1111/1462-2920.14511
35. Skelton E, Rancès E, Frentiu FD, Kusmintarsih ES, Iturbe-Ormaetxe I, Caragata EP, et al. A native Wolbachia endosymbiont does not limit dengue virus infection in the mosquito Aedes notoscriptus (Diptera: Culicidae). J Med Entomol (2016). doi: 10.1093/jme/tjv235
36. Palmer WH, Medd NC, Beard PM, Obbard DJ. Isolation of a natural DNA virus of Drosophila melanogaster, and characterisation of host resistance and immune responses. PloS Pathog (2018). doi: 10.1371/journal.ppat.1007050
37. Mousson L, Martin E, Zouache K, Madec Y, Mavingui P, Failloux AB. Wolbachia modulates Chikungunya replication in Aedesalbopictus. Mol Ecol (2010)19:1953–64. doi: 10.1111/j.1365-294X.2010.04606.x
38. Longdon B, Fabian DK, Hurst GDD, Jiggins FM. Male-killing Wolbachia do not protect Drosophila bifasciata againstviral infection. BMC Microbiol (2012)12:1–6. doi: 10.1186/1471-2180-12-S1-S8
39. Tsai K-H, Huang C-G, Wu W-J, Chuang C-K, Lin C-C, Chen W-J. Parallel infection of Japanese encephalitis virus and Wolbachia within cells of mosquito salivary glands. J Med Entomol (2006) 43:752–6. doi: 10.1093/jmedent/43.4.752
40. Graham RI, Grzywacz D, Mushobozi WL, Wilson K. Wolbachia in a major African crop pest increases susceptibility toviral disease rather than protects. Ecol Lett (2012) 15:993–1000. doi: 10.1111/j.1461-0248.2012.01820.x
41. Webster CL, Waldron FM, Robertson S, Crowson D, Ferrari G, Quintana JF, et al. The discovery, distribution, and evolution of viruses associated with drosophila melanogaster. PloS Biol (2015). doi: 10.1371/journal.pbio.1002210
42. Martinez J, Ok S, Smith S, Snoeck K, Day JP, Jiggins FM. Should Symbionts Be Nice or Selfish? Antiviral Effects of Wolbachia Are Costly but Reproductive Parasitism Is Not. PloS Pathog (2015). doi: 10.1371/journal.ppat.1005021
43. Zug R, Hammerstein P. Bad guys turned nice? A critical assessment of Wolbachia mutualisms in arthropod hosts. Biol Rev Camb Philos Soc (2015). doi: 10.1111/brv.12098
44. Min KT, Benzer S. Wolbachia, normally a symbiont of Drosophila, can be virulent, causing degeneration and early death. Proc Natl Acad Sci USA (1997). doi: 10.1073/pnas.94.20.10792
45. Carrington LB, Leslie J, Weeks AR, Hoffmann AA. The popcorn wolbachia infection of drosophila melanogaster: Can selection alter wolbachia longevity effects? Evol (N Y) (2009). doi: 10.1111/j.1558-5646.2009.00745.x
46. Gupta V, Stewart CO, Rund SSC, Monteith K, Vale PF. Costs and benefits of sublethal Drosophila C virus infection. J Evol Biol (2017). doi: 10.1111/jeb.13096
47. Siva-Jothy JA, Prakash A, Vasanthakrishnan RB, Monteith KM, Vale PF. Oral bacterial infection and shedding in Drosophilamelanogaster. J Vis Exp (2018) 135:e57676. doi: 10.3791/57676
48. Weinert LA, Araujo-Jnr EV, Ahmed MZ, Welch JJ. The incidence of bacterial endosymbionts in terrestrial arthropods. Proc R Soc B Biol Sci (2015) 282:20150249. doi: 10.1098/rspb.2015.0249
49. Lindsey ARI, Bhattacharya T, Newton ILG, Hardy RW. Conflict in the intracellular lives of endosymbionts and viruses: A mechanistic look at Wolbachia-mediated pathogen-blocking. Viruses (2018) 10:1–29. doi: 10.3390/v10040141
50. Pan X, Zhou G, Wu J, Bian G, Lu P, Raikhel AS, et al. Wolbachia induces reactive oxygen species (ROS)-dependent activationof the Toll pathway to control dengue virus in the mosquito Aedes aegypti.Proc Natl Acad Sci USA (2012) 109:23–31. doi: 10.1073/pnas.1116932108
51. Caragata EP, Pais FS, Baton LA, Silva JBL, Sorgine MHF, Moreira LA. The transcriptome of the mosquito Aedes fluviatilis (Diptera:Culicidae), and transcriptional changes associated with its native Wolbachiainfection. BMC Genomics (2017)18:1–19. doi: 10.1186/s12864-016-3441-4
52. Zug R, Hammerstein P. Wolbachia and the insect immune system: What reactive oxygen species can tell us about the mechanisms of Wolbachia-host interactions. Front Microbiol (2015). doi: 10.3389/fmicb.2015.01201
53. Wong ZS, Brownlie JC, Johnson KN. Oxidative stress correlates with Wolbachia-mediated antiviralprotection in Wolbachia-Drosophila associations. Appl EnvironMicrobiol (2015) 81:3001–5. doi: 10.1128/AEM.03847-14
54. Molloy JC, Sommer U, Viant MR, Sinkins SP. Wolbachia modulates lipid metabolism in Aedes albopictus mosquito cells. Appl Environ Microbiol (2016) 82:3109–20. doi: 10.1128/AEM.00275-16
55. Geoghegan V, Stainton K, Rainey SM, Ant TH, Dowle AA, Larson T, et al. Perturbed cholesterol and vesicular trafficking associated withdengue blocking in Wolbachia-infected Aedes aegypti cells. NatCommun (2017) 8:1–10. doi: 10.1038/s41467-017-00610-8
56. Caragata EP, Rancès E, Hedges LM, Gofton AW, Johnson KN, O’Neill SL, et al. Dietary Cholesterol Modulates Pathogen Blocking byWolbachia. PloS Pathog (2013) 9:e1003459. doi: 10.1371/journal.ppat.1003459
57. Lindsey AR, Bhattacharya T, Hardy R, Newton IL. Wolbachia and virus alter the host transcriptome at the interface of nucleotide metabolism pathways. bioRxiv (2020) 1–38. doi: 10.1101/2020.06.18.160317
58. Lu P, Bian G, Pan X, Xi Z. Wolbachia induces density-dependent inhibition to dengue virus in mosquito cells. PloS Negl Trop Dis (2012) 6:1–8. doi: 10.1371/journal.pntd.0001754
59. Chrostek E, Marialva MSP, Esteves SS, Weinert LA, Martinez J, Jiggins FM, et al. Wolbachia Variants Induce Differential Protection to Viruses in Drosophila melanogaster: A Phenotypic and Phylogenomic Analysis. PloS Genet (2013). doi: 10.1371/journal.pgen.1003896
60. Chrostek E, Teixeira L. Mutualism Breakdown by Amplification of Wolbachia Genes. PloS Biol (2015). doi: 10.1371/journal.pbio.1002065
61. Duarte EH, Carvalho A, Verde UDC, Lisboa U. Regulation of Wolbachia proliferation by the amplification and deletion of an addictive genomic island. bioRxiv (2020). doi: 10.1101/2020.09.08.288217
62. Fraser JE, O’Donnell TB, Duyvestyn JM, O’Neill SL, Simmons CP, Flores HA. Novel phenotype of Wolbachia strain wPip in Aedes aegypti challenges assumptions on mechanisms of Wolbachia-mediated dengue virus inhibition. PloS Pathog (2020) 16:1–21. doi: 10.1371/journal.ppat.1008410
63. Chrostek E, Martins NE, Marialva MS, Teixeira L. Wolbachia-conferred antiviral protection is determined by developmental temperature. bioRxiv (2020). doi: 10.1101/2020.06.24.169169
64. Le Page DP, Metcalf JA, Bordenstein SR, On J, Perlmutter JI, Shropshire JD, et al. Prophage WO genes recapitulate and enhance Wolbachia-induced cytoplasmic incompatibility. Nature (2017) 543:243–7. doi: 10.1038/nature21391
65. Yen JH, Barr AR. New hypothesis of the cause of cytoplasmic incompatibility in Culexpipiens L. [31]. Nature (1971) 232:657–8. doi: 10.1038/232657a0
66. Shropshire JD, Bordenstein SR. Two-by-one model of cytoplasmic incompatibility: Synthetic recapitulation by transgenic expression of cifa and cifb in drosophila. PloS Genet (2019). doi: 10.1371/journal.pgen.1008221
67. Palmer WH, Varghese FS, Van Rij RP. Natural variation in resistance to virus infection in dipteran insects. Viruses (2018). doi: 10.3390/v10030118
68. Cogni R, Cao C, Day JP, Bridson C, Jiggins FM. The genetic architecture of resistance to virus infection in Drosophila. Mol Ecol (2016). doi: 10.1111/mec.13769
69. Marques JT, Imler JL. The diversity of insect antiviral immunity: Insights from viruses. Curr Opin Microbiol (2016). doi: 10.1016/j.mib.2016.05.002
70. Duxbury EML, Day JP, Vespasiani DM, Thüringer Y, Tolosana I, Smith SCL, et al. Host-pathogen coevolution increases genetic variation in susceptibility to infection. Elife (2019). doi: 10.7554/eLife.46440
71. Martinez J, Cogni R, Cao C, Smith S, Illingworth CJR, Jiggins FM. Addicted? Reduced host resistance in populations with defensive symbionts. Proc R Soc B Biol Sci (2016). doi: 10.1098/rspb.2016.0778
72. Martins NE, Faria VG, Nolte V, Schlötterer C, Teixeira L, Sucena É, et al. Host adaptation to viruses relies on few genes with different cross-resistance properties. Proc Natl Acad Sci USA (2014). doi: 10.1073/pnas.1400378111
73. Magwire MM, Fabian DK, Schweyen H, Cao C, Longdon B, Bayer F, et al. Genome-Wide Association Studies Reveal a Simple Genetic Basis of Resistance to Naturally Coevolving Viruses in Drosophila melanogaster. PloS Genet (2012). doi: 10.1371/journal.pgen.1003057
74. Cao C, Cogni R, Barbier V, Jiggins FM. Complex coding and regulatory polymorphisms in a restriction factor determine the susceptibility of Drosophila to viral infection. Genetics (2017). doi: 10.1534/genetics.117.201970
75. Faria VG, Martins NE, Magalhães S, Paulo TF, Nolte V, Schlötterer C, et al. Drosophila Adaptation to Viral Infection through Defensive Symbiont Evolution. PloS Genet (2016). doi: 10.1371/journal.pgen.1006297
76. Faria VG, Martins NE, Schlötterer C, Sucena É. Readapting to DCV infection without Wolbachia: Frequency changes of Drosophila antiviral alleles can replace endosymbiont protection. Genome Biol Evol (2018). doi: 10.1093/gbe/evy137
77. Longdon B, Hadfield JD, Day JP, Smith SCL, McGonigle JE, Cogni R, et al. The Causes and Consequences of Changes in Virulence following Pathogen Host Shifts. PloS Pathog (2015). doi: 10.1371/journal.ppat.1004728
78. Mateos M, Castrezana SJ, Nankivell BJ, Estes AM, Markow TA, Moran NA. Heritable endosymbionts of Drosophila. Genetics (2006) 174:363–76. doi: 10.1534/genetics.106.058818
79. Pimentel AC, Beraldo CS, Cogni R. Host-shift as the cause of emerging infectious diseases: Experimental approaches using Drosophila -virus interactions. Genet Mol Biol (2021) 44:e20200197. doi: 10.1590/1678-4685-gmb-2020-0197
80. Kriesner P, Hoffmann AA, Lee SF, Turelli M, Weeks AR. Rapid Sequential Spread of Two Wolbachia Variants in Drosophilasimulans. PloS Pathog (2013) 9:e1003607. doi: 10.1371/journal.ppat.1003607
81. Hoffmann AA, Turelli M. Unidirectional incompatibility in Drosophila simulans: inheritance, geographic variation and fitness effects. Genetics (1988). doi: 10.1093/genetics/119.2.435
82. Hoffmann AA, Hercus M, Dagher H. Population dynamics of the Wolbachia infection causing cytoplasmic incompatibility in Drosophila melanogaster. Genetics (1998).
83. Harcombe W, Hoffmann AA. Wolbachia effects in Drosophila melanogaster: In search of fitness benefits. J Invertebr Pathol (2004). doi: 10.1016/j.jip.2004.07.003
84. Panteleev DY, Goryacheva II, Andrianov BV, Reznik NL, Lazebny OE, Kulikov AM. The endosymbiotic bacterium Wolbachia enhances the nonspecific resistance to insect pathogens and alters behavior of Drosophila melanogaster. Russ J Genet (2007). doi: 10.1134/S1022795407090153
85. Ye YH, Woolfit M, Rancès E, O’Neill SL, McGraw EA. Wolbachia-Associated Bacterial Protection in the Mosquito Aedes aegypti. PloS Negl Trop Dis (2013). doi: 10.1371/journal.pntd.0002362
86. Pan X, Pike A, Joshi D, Bian G, McFadden MJ, Lu P, et al. The bacterium Wolbachia exploits host innate immunity to establish a symbiotic relationship with the dengue vector mosquito Aedes aegypti. ISME J (2018). doi: 10.1038/ismej.2017.174
87. Zhang YZ, Shi M, Holmes EC. Using Metagenomics to Characterize an Expanding Virosphere. Cell (2018). doi: 10.1016/j.cell.2018.02.043
88. Shi M, White VL, Schlub T, Eden JS, Hoffmann AA, Holmes EC. No detectable effect of Wolbachia wMel on the prevalence and abundance of the RNA virome of Drosophila melanogaster. Proc R Soc B Biol Sci (2018). doi: 10.1098/rspb.2018.1165
89. Kriesner P, Conner WR, Weeks AR, Turelli M, Hoffmann AA. Persistence of a Wolbachia infection frequency cline in Drosophila melanogaster and the possible role of reproductive dormancy. Evol (N Y) (2016). doi: 10.1111/evo.12923
Keywords: antiviral, Wolbachia, insects, arboviruses, evolution, wild populations, review, endosymbiont
Citation: Pimentel AC, Cesar CS, Martins M and Cogni R (2021) The Antiviral Effects of the Symbiont Bacteria Wolbachia in Insects. Front. Immunol. 11:626329. doi: 10.3389/fimmu.2020.626329
Received: 05 November 2020; Accepted: 14 December 2020;
Published: 29 January 2021.
Edited by:
Luc Swevers, National Centre of Scientific Research Demokritos, GreeceReviewed by:
Zhiyong Xi, Michigan State University, United StatesIoannis Eleftherianos, George Washington University, United States
Copyright © 2021 Pimentel, Cesar, Martins and Cogni. This is an open-access article distributed under the terms of the Creative Commons Attribution License (CC BY). The use, distribution or reproduction in other forums is permitted, provided the original author(s) and the copyright owner(s) are credited and that the original publication in this journal is cited, in accordance with accepted academic practice. No use, distribution or reproduction is permitted which does not comply with these terms.
*Correspondence: Rodrigo Cogni, cm9kcmlnb2NvZ25pQGdtYWlsLmNvbQ==