- 1Microbiome Study Group, School of Health Sciences Dr. Paulo Prata, São Paulo, Brazil
- 2Microbiology Program, Institute of Biosciences, Humanities and Exact Sciences (IBILCE), São Paulo State University (UNESP), São Paulo, Brazil
- 3Barretos Cancer Hospital, São Paulo, Brazil
- 4Food Engineering and Technology Department, Institute of Biosciences, Humanities and Exact Sciences, São Paulo State University (UNESP), São José do Rio Preto, Brazil
Hashimoto thyroiditis (HT) is the most common autoimmune disease worldwide, characterized by chronic inflammation and circulating autoantibodies against thyroid peroxidase and thyroglobulin. Patients require hormone replacement with oral levothyroxine, and if untreated, they can develop serious adverse health effects and ultimately death. There is a lot of evidence that the intestinal dysbiosis, bacterial overgrowth, and increased intestinal permeability favor the HT development, and a thyroid–gut axis has been proposed, which seems to impact our entire metabolism. Here, we evaluated alterations in the gut microbiota in Brazilian patients with HT and correlated this data with dietary habits, clinical data, and systemic cytokines and zonulin concentrations. Stool samples from 40 patients with HT and 53 controls were analyzed using real-time PCR, the serum cytokine levels were evaluated by flow cytometry, zonulin concentrations by ELISA, and the dietary habits were recorded by a food frequency questionnaire. We observed a significant increase (p < 0.05) in the Bacteroides species and a decrease in Bifidobacterium in samples of patients with HT. In addition, Lactobacillus species were higher in patients without thyroid hormone replacement, compared with those who use oral levothyroxine. Regarding dietary habits, we demonstrated that there are significant differences in the consumption of vegetables, fruits, animal-derived proteins, dairy products, saturated fats, and carbohydrates between patients and control group, and an inverse correlation between animal-derived protein and Bacteroides genus was detected. The microbiota modulation by diet directly influences the inflammatory profile due to the generated microbiota metabolites and their direct or indirect action on immune cells in the gut mucosa. Although there are no differences in systemic cytokines in our patients with HT, we detected increased zonulin concentrations, suggesting a leaky gut in patients with HT. These findings could help understand the development and progression of HT, while further investigations to clarify the underlying mechanisms of the diet–microbiota–immune system axis are still needed.
Introduction
The Hashimoto thyroiditis (HT) is an organ-specific autoimmune disease and one of the most common diseases worldwide (1). HT is characterized by chronic inflammation, with tertiary lymphoid follicles development and increased concentrations of circulating autoantibodies against thyroid peroxidase (anti-TPO) and thyroglobulin (anti-Tg) (2). The thyroid parenchyma is replaced by the lymphocytes infiltration, inducing organ enlargement, gland fibrosis, and destruction (3). The progressive thyrocyte depletion leads to decreased thyroid hormone function and clinical hypothyroidism, a condition marked by a reduced metabolic activity in several tissues. The disease is correlated with decreased cardiovascular contractility, coronary artery disease, infertility, dementia, neurosensory and musculoskeletal changes, and reduced colonic activity and orocecal time transit (4, 5). Patients require hormone replacement with oral levothyroxine, and if untreated, they can develop serious adverse health effects and ultimately death (6, 7).
Hashimoto thyroiditis has become a global public health concern, and the worldwide prevalence reaches 10–12%, affecting 10 times more women, and the peak incidence is between 30 and 50 years old (5, 6). In the United States of America, HT affects ~4% of women aged 18–24 years and 21% of women older than 74 years (6). In Europe, the prevalence varies between 0.2 and 5.3% in the general population, and 7.5% of women in the United Kingdom presented elevated thyroid-stimulating hormone (TSH) concentrations (8). In Brazil, the prevalence of elevated TSH in adult women in Rio de Janeiro was 12.3%, reaching 19.1% among those over 70 years old. In the metropolitan region of São Paulo, the HT prevalence reached 8.0%, and in an elderly population, the prevalence was 6.5 and 6.1% for women and men, respectively (9). The economic impact can be significant taking into account the direct effects of the disease, with the HT side effects, which range from depression and to a greater propensity to develop differentiated thyroid cancer and extra-thyroidal cancers (5, 10–13).
Although the HT etiology remains unknown, epidemiological studies suggest that HT is caused by an interaction between genetic and environmental factors (14). The genetic predisposition plays a crucial role in the loss of tolerance to self-antigens and loci linked to immune-modifying genes such as human leukocyte antigens (HLA class I and II) and cytotoxic-T-lymphocyte-associated protein 4 (CTLA-4) could be involved in the autoimmune process. The interactions between these loci and environmental factors might influence disease phenotype and severity (3). The environmental factors that may be involved in the HT triggering include excessive iodine consumption; selenium, iron, zinc, and vitamin D deficiencies; bacterial infections, such as Helicobacter pylori and Yersinia enterocolitica; viral infections, such as hepatitis C, Epstein–Barr virus, and enteroviruses; smoking; pollutants; and chemical agents, and also, factors such as stress, climate, age, and gender (15). More recently, it is hypothesized that gut microbiota might play an important role in triggering HT (16).
There is a lot of evidence that the intestinal dysbiosis, bacterial overgrowth, and increased intestinal permeability (leaky gut) favor HT development, and a thyroid–gut axis has been proposed which seems to impact our entire metabolism (17, 18). The commensal gut bacteria influence the innate, cellular, and humoral immunity by interacting with epithelial cells and mucosal immune cells via pattern recognition receptors. Depending on the generated metabolites, microbiota can activate a proinflammatory or anti-inflammatory program (19). Moreover, gut microbiota can affect the thyroid hormone concentrations by controlling iodine uptake, degradation, and enterohepatic recycling of these hormones, and the levothyroxine bioavailability (L-thyroxine). There is a prominent effect of minerals on microbiota–host interactions, mainly by zinc, selenium, and iron. In addition, the microbiota may play role in thyroid disorders by influencing neurotransmitters, the hypothalamus–pituitary axis, the dopamine production, and consequently the TSH secretion (17).
The short-chain fatty acids (SCFAs), metabolites from anaerobic microbiota fermentation, function as an energy source for enterocytes and, together with thyroid hormones, mainly T3, induce enterocyte differentiation, and strengthen the epithelial barrier integrity (20). In addition to SCFAs, the microbiota produce secondary bile acids in the colon, which present systemic effects and interfere with TSH levels (21). Secondary bile acids can regulate type 2 iodothyronine deiodinase (D2) in the gut, molecules involved in thyroid metabolism, and the lipopolysaccharides (LPS) derived from Gram-negative bacteria inhibits intestinal D2 and hepatic D1, and decreases the expression of thyroid hormones in the liver (22).
In animal models, the association between the gut microbiota and thyroid functions has been proposed since 1970s. The depleted microbiota in antibiotic-treated rats induced a decrease in thyroid functions, when evaluated by radioactive iodine uptake (23). In the same line, the germ-free mice present an increase in TSH levels when compared with the conventionally raised mice (22).
In humans, few studies have been carried out in order to understand the relationship between intestinal microbiota and HT. Some recent studies have demonstrated the existence of intestinal dysbiosis in patients with HT and showed an increase in the Bacteroides, Escherichia-Shigella, and Parasutterella genera and a decrease in the Bifidobacterium, Lactobacillus, Prevotella, and Dialister genera in the gut microbiota in patients with HT (23). Another study showed an increase in the relative abundance of Blautia, Roseburia, Ruminococcus, Romboutsia, Dorea, Fusicatenibacter, and Eubacterium genera and a decrease in Bacteroides, Faecalibacterium, Prevotella, and Lachnoclostridium genera in the gut microbiota in patients with HT (24).
On the basis of this background and the fact that there are no studies evaluating the gut microbiota in Brazilian patients with HT, the aim of this study was to evaluate the presence of some specific bacteria in stool samples of patients with HT and to correlate these data with dietary habits, clinical data, and systemic cytokines and zonulin concentrations.
Materials and Methods
Patients With HT and Control Subjects
Patients diagnosed with HT, with increased TSH and decreased free thyroxine levels (FT4), associated or not associated with elevated anti-TPO and anti-Tg antibodies, were enrolled for this study. The Barretos Cancer Hospital Ethics Committee (Process number 1,359/2017) approved this study, and all participants signed the informed consent form.
About 40 patients with HT aged 23–82 years (mean age ± SD = 48.9 ± 13.3 years) were enrolled. Fifty-three healthy controls aged 18–79 years (mean age ± SD = 45.6 ± 16.7 years) were recruited for the study. Exclusion criteria, for both groups, include the use of anti-inflammatories, immunosuppressant drugs, antibiotics, and vaccination in the last 30 days, with gastrointestinal surgeries, inflammatory bowel diseases, and chronic diarrheas.
After the informed consent, patients and controls answered a food frequency questionnaire (FFQ), containing questions about frequency and consumption of vegetables, fruits, animal-derived proteins, dairy products, carbohydrates, and saturated fat. After that, the peripheral blood was collected, and stool samples were collected within 3–5 days. Table 1 summarizes demographic data and clinical parameters in patients with HT.
DNA Extraction From Stool Samples and Real-Time PCR
DNA was obtained from 200 mg of stool samples of patients with HT and controls by using QIAamp Fast DNA Stool Mini Kit (QIAGEN Inc., Hilden, Germany), according to the instructions of the manufacturer. The composition of the gut microbiota was determined by specific primers for Bacteroides (Bac), Bifidobacterium (Bif), Clostridium coccoides (Ccoc), Clostridium coccoides–Eubacterium rectale (CIEub), Clostridium leptum (Clept), Lactobacillus (Lac), Prevotella (Prev), and Roseburia (Ros). The primers were described previously and designed by using the 16S rRNA gene sequences from the Ribosomal Database Project (25). PCR was performed by using 7.5 μl of Power SYBR Green PCR Master Mix (Applied Biosystems, Life Technologies, Carlsbad, CA, USA), 4.0 μl of ultrapure water (Uniscience Corporation, Hialeah, FL, USA), 1.0 μl of each primer (2 μM), and 1.5 μl of DNA (5 ng). The normalization was performed by dividing the DNA copy numbers obtained for target primers (Bac, Bif, Ccoc, CIEub, Clept, Lac, Prev, and Ros) and for the copy numbers of universal primer (Univ). The relative abundance was quantified by using cycle threshold (Ct) values and was expressed in relative expression units (REUs) per 200 mg of stool (26).
Cytokine Serum Quantification by Flow Cytometer
Approximately 8.5 ml of peripheral blood were collected from patients with HT and healthy individuals in gel tube with clot activator (BD Vacutainer SST II Advance, BD Biosciences, Franklin Lakes, NJ, USA). After collection, samples were incubated at room temperature for 1 h and centrifuged at 1.372 × g for 5 min. The supernatant was withdrawn, and the serum was used for cytokine quantification that was performed by cytometric bead array assay (Human Th1/Th2/Th17 Kit, BD Biosciences, San Jose, CA, USA). The levels of interleukin (IL)-2, IL-4, IL-6, IL-10, IL-17A, interferon-gamma (IFN-γ), and tumor necrosis factor (TNF) were detected by a flow cytometer (FACSCanto™ II, BD Biosciences, Franklin Lakes, NJ, USA). Results were analyzed by BDFCAP array™ software and were expressed in pg/ml.
Zonulin Serum Quantification by Sandwich-ELISA
After peripheral blood collection, samples were incubated in gel tube with clot activator for 50 min and then centrifuged at 1.372 × g for 5 min. The serum samples were used for zonulin quantification by using the Human Zonulin ELISA Kit (Elabscience, Bethesda, MD, USA). Standards and samples were added to plates precoated with specific antibodies to human zonulin and incubated for 1 h at 37°C. Then, a biotinylated detection antibody specific for human zonulin and avidin–horseradish peroxidase conjugate was added and incubated for 30 min. Unbound and free molecules were washed away. The substrate solution was added to each well and incubated for 15 min. When the enzyme–substrate reaction was blocked by stop solution, the color turned to yellow. The optical density was measured in a spectrophotometer at 450 nm. The standard curve was constructed, and the zonulin concentrations were calculated by converting the obtained optical density in ng/ml.
Statistical Analysis
The data extracted from the surveys on dietary habits of patients with HT and controls were analyzed by the Pearson chi-square test, the REUs of the gut microbiota were analyzed by the non-parametric Mann–Whitney U-test, and the serum concentrations of cytokines and zonulin were performed by unpaired t-test with the Welch's correction. Correlations of the gut microbiota, clinical data, cytokines, and zonulin concentrations were performed by the Spearman's rank correlation coefficient. Values of p < 0.05 were considered statistically significant.
Results
Increased Bacteroides and Decreased Bifidobacterium in the Gut Microbiota in Patients
To analyze the composition of the gut microbiota in patients with HT, we investigated some bacterial species in stool samples by real-time PCR. We detected an increase in the REUs of Bacteroides species in the samples derived from patients (median Bac: 2,344 REU/200 mg stool; p < 0.0001) compared with control subjects (median Bac: 221.3 REU/200 mg stool) (Figure 1A). A significant decrease in the REUs of Bifidobacterium species was observed in the stool samples isolated from patients with HT (median Bif : 70.86 REU/200 mg stool; p = 0.005) when compared with controls (median Bif : 830.6 REU/200 mg stool) (Figure 1B). There are no significant differences (p > 0.05) in the REU/200 mg stool obtained from Clostridium coccoides (median Ccoc: 43.51), Clostridium coccoides-Eubacteria rectale (median CIEub: 48.03), Clostridium leptum (median Clept: 1.060), Lactobacillus (median Lac: 0.974), Prevotella (median Prev: 12.53), and Roseburia species (median: 385.8) in stool samples of patients with HT, when compared with the healthy individuals (median Ccoc: 51.21; CIEub: 78.04; Clept: 1,762; Lac: 4.933; Prev: 0.530; and Ros: 635.9, respectively) (Figures 1C–H).
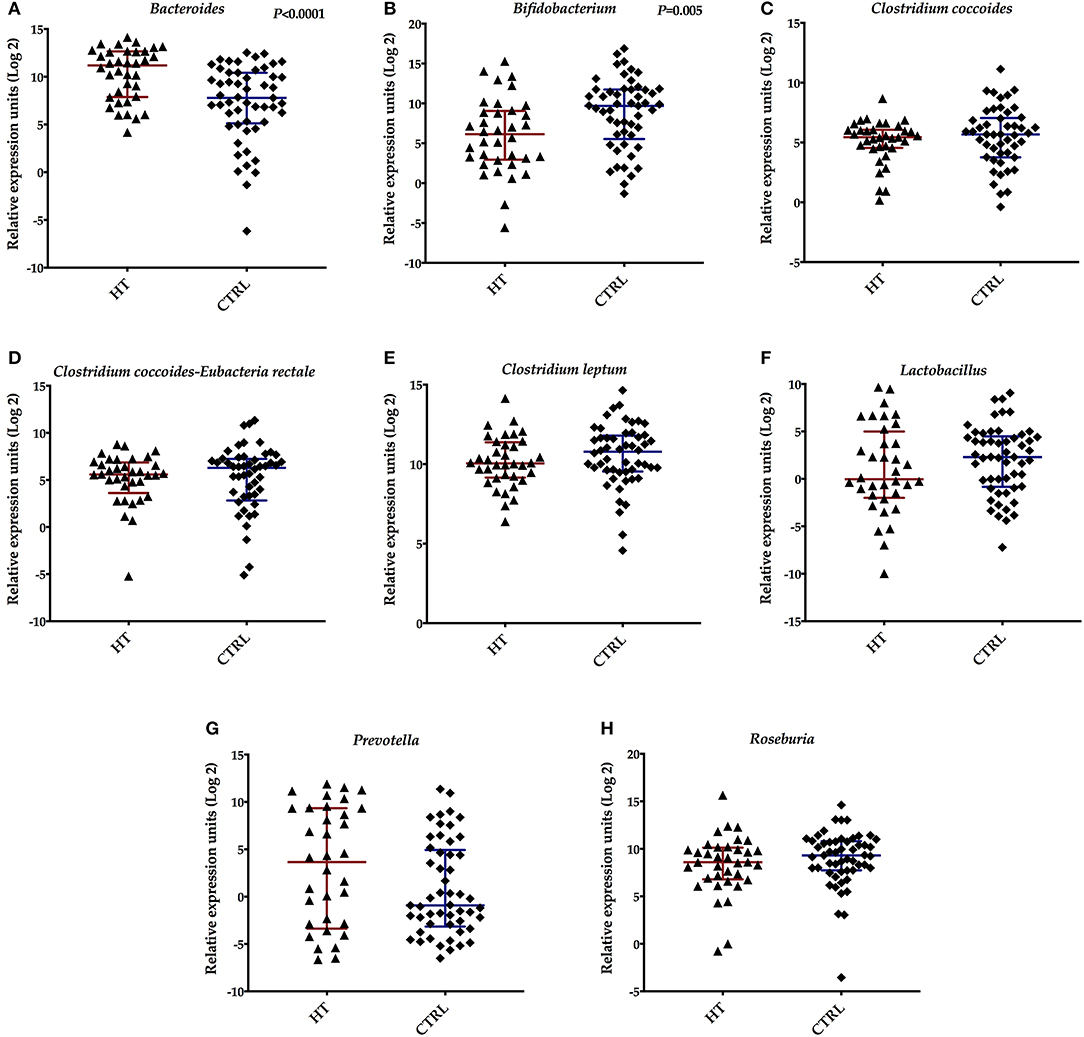
Figure 1. Relative expression units (REU) of the intestinal microbiota found in stool samples from patients with Hashimoto thyroiditis (HT) and healthy controls (CTRL). (A) Bacteroides, (B) Bifidobacterium, (C) Clostridium coccoides, (D) Clostridium coccoides-Eubacterium-rectale, (E) Clostridium leptum, (F) Lactobacillus, (G) Prevotella, and (H) Roseburia species. Bars represent the median with interquartile range of REU per 200 mg of stool.
Dietary Habits and Correlations With the Gut Microbiota
To evaluate the dietary habits from patients and controls, we applied an FFQ regarding the frequency of consumption of vegetables, fruits, animal-derived proteins, dairy products, saturated fats, and carbohydrates. The interviewed subjects reported the daily ingestion of vegetables [patients (HT) = 59%; controls (C) = 53.3%; fruits (HT = 38.5%; C = 43.3%), proteins (HT = 51.3%; C = 30%), dairy products (HT = 48.7%; C = 60%), saturated fats (HT = 17.9%; C = 16.7%), and carbohydrates (HT = 38.5%; C = 43.3%)]. When we compared the dietary habits between patients with HT and controls, we observed a significant difference (p < 0.05) in the consumption of vegetables, fruits, proteins, dairy products, saturated fats, and carbohydrates. The results concerning the dietary habits from patients and controls were shown in Table 2.
In order to detect correlations between diet and gut microbiota composition in patients with HT, we used the consumption frequencies and the REUs of Bacteroides, Bifidobacterium, Clostridium coccoides, Clostridium coccoides-Eubacteria rectale, Clostridium leptum, Lactobacillus, Prevotella, and Roseburia. We found an inverse correlation (p = 0.002; r = −0.61) between animal-derived protein consumption by patients and the REUs of Bacteroides species.
Correlations Between the Gut Microbiota and Clinical Data
To evaluate the connection between gut microbiota composition and clinical parameters, we correlated the REUs of Bacteroides, Bifidobacterium, Clostridium coccoides, Clostridium coccoides-Eubacteria rectale, Clostridium leptum, Lactobacillus, Prevotella, and Roseburia with concentrations of TSH, FT4, TPO, and Tg autoantibodies. We detected positive correlations among the REUs of Clostridium coccoides (p = 0.023; r = 0.40) and Clostridium coccoides–Eubacteria rectale (p = 0.010; r = 0.45) and in the TSH levels (Figure 2A). The REUs of Roseburia species inversely correlated (p = 0.015; r = −0.49) with FT4 levels (Figure 2B). We also found a positive correlation between Clostridium coccoides (p = 0.040; r = 0.30) and disease duration (Figure 2C). There are no correlations (p > 0.05) among gut microbes and TPO and Tg autoantibodies. Besides that, we observed significant differences in the REUs of Lactobacillus between patients who did thyroid hormone replacement therapy (N = 28) and those who did not (N = 8) (median Lac LT4: 0.8065 REU/200 mg stool; median Lac NR: 47.73; p = 0.020; Figure 2D).
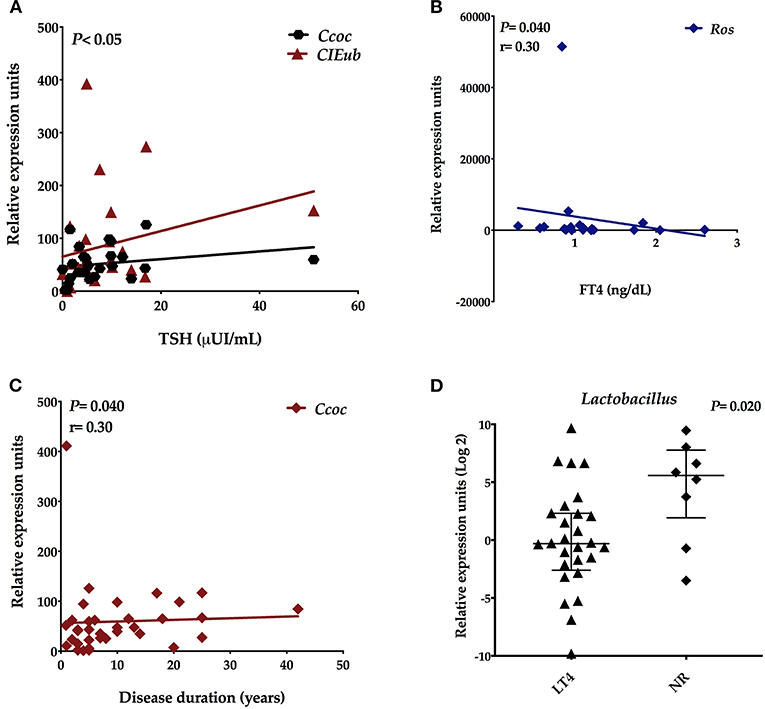
Figure 2. Spearmans' correlation between the relative expression units (REU) of the intestinal microbiota and clinical data. (A) REU of Clostridium coccoides and Clostridium coccoides-Eubacterium-rectale with TSH concentrations, (B) REU of Roseburia with FT4 levels, (C) REU of Clostridium coccoides with disease duration, and (D) REU of Lactobacillus species in treated patients (LT4) and patients with no hormone replacement (NR).
Systemic Cytokine Profile in Patients With HT Was Similar to Controls
To investigate whether the alterations of intestinal microbiota impacted on systemic cytokine profile in patients with HT, we analyzed the serum concentrations of IL-2, IL-4, IL-6, IL-10, IL-17A, IFN-γ, and TNF by flow cytometer. Significant differences in these cytokines were not detected (p > 0.05) (mean ± SE for IL-2: 0.3369 ± 0.0638 pg/ml; IL-4: 0.4081 ± 0.0542 pg/ml; IL-6: 1.121 ± 0.1599 pg/ml; IL-10: 0.3192 ± 0.0333 pg/ml; IL-17A: 0.5835 ± 0.2388 pg/ml; IFN-γ: 0.4473 ± 0.1023 pg/ml; and TNF: 1.154 ± 0.1090 pg/ml) when compared with controls (mean ± SE for IL-2: 0.3800 ± 0.0527 pg/ml; IL-4: 0.3791 ± 0.0541 pg/ml; IL-6: 1.375 ± 0.2492 pg/ml; IL-10: 0.2884 ± 0.0414 pg/ml; IL-17A: 0.3981 ± 0.1985 pg/ml; IFN-γ: 0.3438 ± 0.0951 pg/ml; and TNF: 1.317 ± 0.1711 pg/ml) (Figures 3A–G).
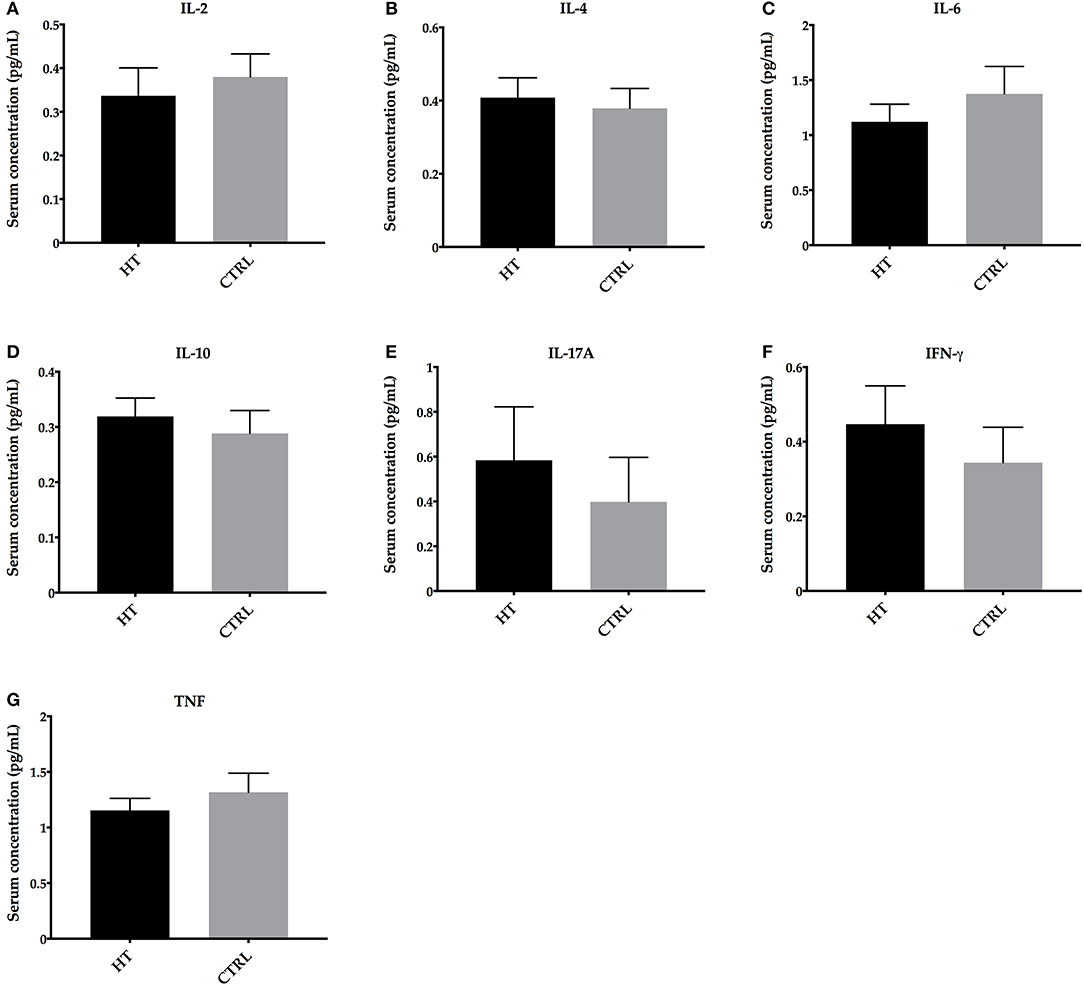
Figure 3. Cytokine profile in HT patients and control subjects (CTRL). Serum concentrations of (A) IL-2, (B) IL-4, (C) IL-6, (D) IL-10, (E) IL-17A, (F) IFN-γ, and (G) TNF. Statistical analyses were performed by Mann-Whitney. Significance was set at P < 0.05.
Detection of Increased Intestinal Permeability in Patients With HT
In order to find out whether patients with HT were presented with a leaky gut, since alterations in the gut microbiota were detected, we evaluated the serum zonulin concentrations in patients with HT and controls. The zonulin serum concentrations were significantly increased (p = 0.002) in samples of patients with HT (mean ± SE: 30.92 ± 2.36 ng/ml) when compared with controls (mean ± SE: 19.01 ± 2.98 pg/ml) (Figure 4A). Besides that, the zonulin concentrations positively correlated with systemic IFN-γ levels (p = 0.042; r = 0.52; Figure 4B) and inversely correlated with IL-2 concentrations (p = 0.042; r = −0.51) in patients with HT (Figure 4B).
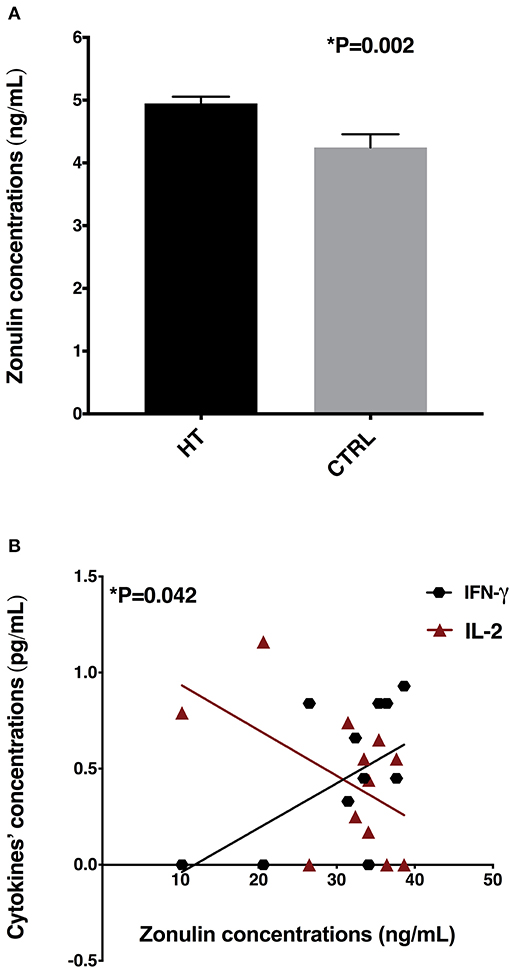
Figure 4. Zonulin concentrations and correlations with systemic cytokine levels. (A) Serum zonulin concentrations in HT patients and controls (CTRL), (B) Correlations among zonulin levels with IFN-γ and IL-2 concentrations.
Discussion
There is a lot of evidence that alterations in the gut microbiota are associated with autoimmune diseases development (16, 27–29). Researchers suggested that the molecular mimicry, bystander T-cell activation, post-translational modification of luminal proteins by altered microbiota, a shift to proinflammatory milieu in the gut mucosa, intestinal dysbiosis, bacterial overgrowth, and leaky gut could contribute to autoimmunity and HT trigger in genetically susceptible individuals (17, 18, 30, 31). The commensal microbiota play an important role in our physiological processes, influencing the mucosal innate and adaptive immunity and host metabolism, including carbohydrate digestion and fermentation, vitamin synthesis, hormones production and secretion, secondary bile acid synthesis, absorption of micronutrients, including iron, zinc, and iodine, which directly affect thyroid function (22, 32). In the present study, we investigated the alterations in the gut microbiota in Brazilian patients with HT and correlated these data with dietary habits, clinical data, and systemic cytokines and zonulin concentrations.
There are four studies that compared the gut microbiota from patients with euthyroid and hypothyroid HT in Chinese population, and researchers detected intestinal dysbiosis when compared with healthy controls (23, 24, 33, 34). Ishaq et al. (23) investigated the microbial composition in 29 patients with HT and 12 healthy controls by PCR-DGGE, real-time PCR, and 16S pyrosequencing (17, 19). Authors showed a reduction in Firmicutes and Bacteroidetes, and increased Proteobacteria and Cyanobacteria phyla in HT samples. Findings of the DGGE analysis showed a different fingerprint between patients and controls and the predominance of pathogenic microbes, such as Bacteroides uniformis, B. pyogenes, B. vulgates, Shigella dysenteriae, B. intestinalis, Escherichia coli, Sporomusa ovate, Shigella flexneri, and Bacillus species. By real-time PCR, authors observed a significant decrease in copy numbers of Bifidobacterium and Lactobacillus, and an increase in E. coli in patients with HT. By the 16S pyrosequencing, researchers detected a predominance of Bacteroides, Escherichia–Shigella, and Parasutterella genera, and a reduction in Prevotella and Dialister genera in patients with HT (23).
Another study examined the intestinal microbiota in 28 patients with euthyroid and 16 controls by 16S sequencing and showed that Firmicutes/Bacteroidetes ratio was increased and the Lachnospiraceae family was prevalent in stool of patients with HT. The abundance of Bacteroides, Faecalibacterium, Prevotella, and Lachnoclostridium genera was lower in patients with HT, and Blautia, Ruminococcus, Roseburia, Fusicatenibacter, Romboutsia, Dorea, and Eubacterium genera were higher in samples derived from patients with HT. Furthermore, the authors reported positive correlations between Firmicutes members and anti-TPO or anti-Tg autoantibodies, while Bacteroidetes members inversely correlated with these antibodies. The abundance of Alloprevotella positively correlated with FT4 levels, while Romboutsia negatively correlated with TSH concentrations (24).
In a more recent cross-sectional work, Liu et al. (33) investigated the gut microbiota in 45 patients with euthyroid, 18 patients with HT hypothyroid, and 34 controls by 16S sequencing. Compared with healthy controls, intestinal microbiota richness and diversity were significantly decreased in subjects with HT, with predominance of Bacteroidetes phylum members, Prevotella and Phascolarctobacterium species. In patients with euthyroid, some microbes, such as Lachnospiraceae incertae sedis, Lactonifactor, Alistipes, and Subdoligranulum genus, were enriched in stool samples. The authors concluded that there is a different microbiota profile in patients with diverse thyroid function, and they suggest that Phascolarctobacterium genus could be involved in HT progression in humans (33). Phascolarctobacterium species, mainly Phascolarctobacterium faecium, can produce acetate and propionate and influence the metabolic status of the host (35).
In accordance with the previous study, significant differences in richness and diversity of the gut microbiota were observed in 52 patients with primary hypothyroidism, compared with 40 healthy controls. The abundance of Bacteroidetes phylum members, Veillonella and Paraprevotella were significantly decreased, while Neisseria and Rheinheimera were increased in patients with hypothyroid. Veillonella and Paraprevotella genera positively correlated with FT3 and FT4 levels and were inversely associated with TSH concentrations. Researchers also showed that mRNA from enzymes involved in SCFAs production was decreased in the gut, and LPS serum levels were increased, suggesting a leaky gut in these patients. Additionally, mice that received fecal microbiota transplantation from patients with hypothyroid presented lower serum total thyroxine levels, decreased tight junction mRNA in the colon, and increased serum LPS (34).
There are neither any studies evaluating the gut microbiota in Brazilian patients with HT, nor are these data correlating with dietary habits, clinical data, and cytokines and zonulin concentrations. Here, we evaluated the gut microbiota in 40 Brazilian patients with HT and 53 healthy controls, and we observed a HT-associated microbiota imbalance, with an increase in Bacteroides and a significant decrease in Bifidobacterium genus. Our data concerning the decreased abundance of Bifidobacterium are in agreement with real-time PCR results from Ishaq et al. (23). Bifidobacterium is the first microbial colonizer of the intestines in newborns, and it plays key roles in immune system maturation and dietary metabolism (36). Some Bifidobacterium strains are considered probiotic because of their beneficial effects, and some studies demonstrated the beneficial role of Bifidobacterium species in inflammatory conditions (37, 38). The discrepancies in relation to other studies and between studies may be due to different methodologies or even due to geographic location, lifestyle, and dietary habits.
In our study, we also observed significant differences in Lactobacillus abundance between patients who realized thyroid hormone replacement therapy and those who did not. Lactobacillus are acid-lactic producing bacteria and belong to the Firmicutes phylum, the second most prevalent in the human gut (39). Recently, a study showed that Lactobacillus promote gut barrier integrity by producing L-Ornithine from arginine amino acid (40). We suggest that this increase in Lactobacillus in patients with no hormone replacement could be associated with the small intestinal bacterial overgrowth (SIBO) in patients with HT (41). Lauritano et al. studied 50 patients with HT and 40 controls and found that 54% of patients presented SIBO, in contrast with the control group (4). A clinical trial conducted by Yao et al. (42) demonstrated that the intestinal microbiota in patients with hypothyroidism, randomly divided to receive L-thyroxine (N = 49) or no treatment (N = 68), did not show significant differences with respect to alpha diversity. However, the relative abundance of Enterococcus and Odoribacter genera varied slightly depending on the dosage of L-thyroxine (42).
There are no studies correlating dietary components and gut microbiota in patients with HT. In our study, we found significant differences in dietary habits between patients with HT and controls regarding the frequency of the consumption of vegetables, fruits, animal-derived proteins, dairy products, saturated fats, and carbohydrates. Furthermore, we found an inverse correlation between animal-derived protein consumption and the abundance of Bacteroides genus. The main role of diet in microbiota modulation and its role in the host metabolism regulation can be exemplified by the establishment of the resident microbiota in early childhood, through the oligosaccharides ingestion from the breast milk, and also by the increase in the microbiota diversity associated with the solid food introduction (43, 44). The gut bacteria overgrowth can be directly affected by the nutrients, and these, in turn, affect both relative and absolute microbiota abundances and bacterial kinetics (45). Alterations in the ingestion of macronutrients, including proteins, carbohydrates, and fats, induce a significant shift in the gut microbiota (46). The microbiota modulation by diet directly influences on the inflammatory profile due to the generated microbiota metabolites and their direct or indirect action on immune cells from the mucosal immune system (47–51). From fermentation of dietary fibers, the intestinal microbes can generate metabolites or SCFAs with anti-inflammatory properties and preserve gut homeostasis (47). In a recent study, Sepahi et al. showed that SCFAs produced by gut microbes in response to dietary fibers influence the expansion on innate lymphoid cells in the gut through G-protein-coupled receptors and can impact the immune responses in the distant tissues depending on the host condition (51). On the other hand, dietary fats are involved in decreased microbiota diversity and richness, which increased intestinal permeability and low-grade systemic inflammation (48).
In the present study, although we demonstrated significant differences in the dietary habits and alterations in the gut microbes in patients with HT, we did not observe significant differences in the cytokine profile in patients when compared with controls. We suggest that this factor can be related to patients' diet, lifestyle, or hormone replacement and disease control. In a recent study, Tabasi et al. (52) evaluated the gut microbiota by real-time PCR and cytokine concentrations in 23 patients with obese hypothyroid and 79 obese control subjects. Researchers reported no significant differences in the gut microbes between patients with hypothyroid and controls. However, similar to our findings, the serum cytokine concentrations, including IL-1β, IL-6, IFN-γ, and TGF-β, were similar in patients and controls (52).
Finally, although there are no differences in systemic cytokines in patients with HT, we detected an increased intestinal permeability, seen by the higher zonulin concentrations in the serum of patients, when compared with controls. Similar to our study, a case–control work investigated the leaky gut in 30 patients with HT (children and adolescents), and 30 patients with congenital hypothyroidism, and reported a significant increase in serum zonulin in patients with HT. Moreover, zonulin levels positively correlated with levothyroxine dose, suggesting a connection between the increased intestinal permeability and disease severity (53). Zonulin is a physiological modulator of intercellular tight junctions, involved in macromolecules trafficking, epithelial and endothelial barrier integrity, and immune tolerance in the gut mucosa (54). Intestinal dysbiosis can activate the zonulin pathway and stimulate their release and allow the traffic of luminal contents through the epithelial barrier. The leaky gut induces inflammatory cytokines release that themselves promote an increased permeability, a vicious circle favoring the entry of antigens derived from diet and gut microbes, triggering the activation of innate and adaptive immunity in the gut mucosa (55). The main factors involved in zonulin release are the bacterial overgrowth and gluten, and the increased intestinal permeability can induce a tolerance breakdown, and then, activated immune cells can remain in the gut mucosa or migrate to distant organs, participating in chronic inflammatory and autoimmune diseases (55–57).
Conclusions
We concluded that there are alterations in the microbiota and intestinal permeability in Brazilian patients with HT. In addition, we suggest that diet might have played an important role in modulating the gut microbiota in patients with HT. These findings could help understand the HT development and progression, while further investigations to clarify the underlying mechanisms of the diet–microbiota–immune system axis are still needed.
Data Availability Statement
The original contributions presented in the study are included in the article/supplementary material, further inquiries can be directed to the corresponding author/s.
Ethics Statement
The studies involving human participants were reviewed and approved by Barretos Cancer Hospital Ethics Committee (Process number 1,359/2017). The patients/participants provided their written informed consent to participate in this study.
Author Contributions
LC and LVVS: enrollment of patients with HT, DNA extraction and quantification, cytokine determination, data acquisition, and manuscript writing. LC, GR, and AB: enrollment of controls and sample collection. AL: PCR real-time experiments and cytokine determination. LDBS: responsible for clinical data from patients with HT. JB: support for blood samples collection. EG: manuscript writing and revision. GO: experimental design, data interpretation, manuscript writing, and revision. All authors contributed to the article and approved the submitted version.
Funding
This study was supported by the Brazilian Governmental Agency, Fundação de Amparo à Pesquisa do Estado de São Paulo (FAPESP Grant numbers #2017/07444-2 and 2021/01035-9), and by the School of Health Sciences from Barretos Dr. Paulo Prata (PAP Grant number #2017).
Conflict of Interest
The authors declare that the research was conducted in the absence of any commercial or financial relationships that could be construed as a potential conflict of interest.
Acknowledgments
We would like to thank the Microbiology Program from Institute of Biosciences, Humanities, and Exact Sciences (IBILCE), São Paulo State University (UNESP), Sao Jose do Rio Preto, Brazil.
References
1. Caturegli P, De Remigis A, Rose NR. Hashimoto thyroiditis: clinical and diagnostic criteria. Autoimmun Rev. (2014) 13:391–7. doi: 10.1016/j.autrev.2014.01.007
2. Fröhlich E, Wahl R. Thyroid autoimmunity: role of anti-thyroid antibodies in thyroid and extra-thyroidal diseases. Front Immunol. (2017) 8:521. doi: 10.3389/fimmu.2017.00521
3. Ajjan RA, Weetman AP. The pathogenesis of Hashimoto's thyroiditis: further developments in our understanding. Horm Metab Res. (2015) 47:702–10. doi: 10.1055/s-0035-1548832
4. Lauritano EC, Bilotta AL, Gabrielli M, Scarpellini E, Lupascu A, Laginestra A, et al. Association between hypothyroidism and small intestinal bacterial overgrowth. J Clin Endocrinol Metab. (2007) 92:4180–4. doi: 10.1210/jc.2007-0606
5. Chiovato L, Magri F, Carlé A. Hypothyroidism in context: where we've been and where we're going. Adv Ther. (2019) 36(Suppl. 2):47–58. doi: 10.1007/s12325-019-01080-8
6. Chaker L, Bianco AC, Jonklaas J, Peeters RP. Hypothyroidism. Lancet Lond Engl. (2017) 390:1550–62. doi: 10.1016/S0140-6736(17)30703-1
7. Hiromatsu Y, Satoh H, Amino N. Hashimoto's thyroiditis: history and future outlook. Horm Athens Greece. (2013) 12:12–8. doi: 10.1007/BF03401282
8. Garmendia Madariaga A, Santos Palacios S, Guillén-Grima F, Galofré JC. The incidence and prevalence of thyroid dysfunction in Europe: a meta-analysis. J Clin Endocrinol Metab. (2014) 99:923–31. doi: 10.1210/jc.2013-2409
9. Sgarbi JA, Teixeira PFS, Maciel LMZ, Mazeto GMFS, Vaisman M, Montenegro Junior RM, et al. The Brazilian consensus for the clinical approach and treatment of subclinical hypothyroidism in adults: recommendations of the thyroid Department of the Brazilian Society of Endocrinology and Metabolism. Arq Bras Endocrinol Amp Metabol. (2013) 57:166–83. doi: 10.1590/S0004-27302013000300003
10. Loh HH, Lim LL, Yee A, Loh HS. Association between subclinical hypothyroidism and depression: an updated systematic review and meta-analysis. BMC Psychiatry. (2019) 19:12. doi: 10.1186/s12888-018-2006-2
11. Qin J, Yu Z, Guan H, Shi L, Liu Y, Zhao N, et al. High thyroglobulin antibody levels increase the risk of differentiated thyroid carcinoma. Dis Markers. (2015) 2015:648670. doi: 10.1155/2015/648670
12. Jankovic B, Le KT, Hershman JM. Clinical review: Hashimoto's thyroiditis and papillary thyroid carcinoma: is there a correlation? J Clin Endocrinol Metab. (2013) 98:474–82. doi: 10.1210/jc.2012-2978
13. Prinzi N, Sorrenti S, Baldini E, De Vito C, Tuccilli C, Catania A, et al. Association of thyroid diseases with primary extra-thyroidal malignancies in women: results of a cross-sectional study of 6,386 patients. PLoS ONE. (2015) 10:e0122958. doi: 10.1371/journal.pone.0122958
14. Taylor PN, Albrecht D, Scholz A, Gutierrez-Buey G, Lazarus JH, Dayan CM, et al. Global epidemiology of hyperthyroidism and hypothyroidism. Nat Rev Endocrinol. (2018) 14:301–16. doi: 10.1038/nrendo.2018.18
15. Liontiris MI, Mazokopakis EE. A concise review of Hashimoto thyroiditis (HT) and the importance of iodine, selenium, vitamin D and gluten on the autoimmunity and dietary management of HT patients. Points that need more investigation. Hell J Nucl Med. (2017) 20:51–6. doi: 10.1967/s002449910507
16. Köhling HL, Plummer SF, Marchesi JR, Davidge KS, Ludgate M. The microbiota and autoimmunity: their role in thyroid autoimmune diseases. Clin Immunol. (2017) 183:63–74. doi: 10.1016/j.clim.2017.07.001
17. Fröhlich E, Wahl R. Microbiota and thyroid interaction in health and disease. Trends Endocrinol Metab TEM. (2019) 30:479–90. doi: 10.1016/j.tem.2019.05.008
18. Knezevic J, Starchl C, Tmava Berisha A, Amrein K. Thyroid-gut-axis: how does the microbiota influence thyroid function? Nutrients. (2020) 12:1769. doi: 10.3390/nu12061769
19. Levy M, Kolodziejczyk AA, Thaiss CA, Elinav E. Dysbiosis and the immune system. Nat Rev Immunol. (2017) 17:219–32. doi: 10.1038/nri.2017.7
20. Meng S, Badrinarain J, Sibley E, Fang R, Hodin R. Thyroid hormone and the d-type cyclins interact in regulating enterocyte gene transcription. J Gastrointest Surg Off J Soc Surg Aliment Tract. (2001) 5:49–55. doi: 10.1016/S1091-255X(01)80013-5
21. Song Y, Zhao M, Zhang H, Zhang X, Zhao J, Xu J, et al. Thyroid-stimulating hormone levels are inversely associated with serum total bile acid levels: a cross-sectional study. Endocr Pract Off J Am Coll Endocrinol Am Assoc Clin Endocrinol. (2016) 22:420–6. doi: 10.4158/EP15844.OR
22. Fenneman AC, Rampanelli E, Yin YS, Ames J, Blaser MJ, Fliers E, et al. Gut microbiota and metabolites in the pathogenesis of endocrine disease. Biochem Soc Trans. (2020) 48:915–31. doi: 10.1042/BST20190686
23. Ishaq HM, Mohammad IS, Guo H, Shahzad M, Hou YJ, Ma C. Molecular estimation of alteration in intestinal microbial composition in Hashimoto's thyroiditis patients. Biomed Pharmacother Biomedecine Pharmacother. (2017) 95:865–74. doi: 10.1016/j.biopha.2017.08.101
24. Zhao F, Feng J, Li J, Zhao L, Liu Y, Chen H, et al. Alterations of the gut microbiota in Hashimoto's thyroiditis patients. Thyroid Off J Am Thyroid Assoc. (2018) 28:175–86. doi: 10.1089/thy.2017.0395
25. Larsen N, Vogensen FK, van den Berg FWJ, Nielsen DS, Andreasen AS, Pedersen BK, et al. Gut microbiota in human adults with type 2 diabetes differs from non-diabetic adults. PLoS ONE. (2010) 5:e9085. doi: 10.1371/journal.pone.0009085
26. Rodrigues GSP, Cayres LCF, Gonçalves FP, Takaoka NNC, Lengert AH, Tansini A, et al. Detection of increased relative expression units of Bacteroides and Prevotella, and decreased Clostridium leptum in stool samples from Brazilian rheumatoid arthritis patients: a pilot study. Microorganisms. (2019) 7:413. doi: 10.3390/microorganisms7100413
27. Lerner A, Aminov R, Matthias T. Dysbiosis may trigger autoimmune diseases via inappropriate post-translational modification of host proteins. Front Microbiol. (2016) 7:84. doi: 10.3389/fmicb.2016.00084
28. de Oliveira GLV, Leite AZ, Higuchi BS, Gonzaga MI, Mariano VS. Intestinal dysbiosis and probiotic applications in autoimmune diseases. Immunology. (2017) 152:1–12. doi: 10.1111/imm.12765
29. De Luca F, Shoenfeld Y. The microbiome in autoimmune diseases. Clin Exp Immunol. (2019) 195:74–85. doi: 10.1111/cei.13158
30. Lerner A, Jeremias P, Matthias T. Gut-thyroid axis and celiac disease. Endocr Connect. (2017) 6:R52–8. doi: 10.1530/EC-17-0021
31. Mu Q, Kirby J, Reilly CM, Luo XM. Leaky gut as a danger signal for autoimmune diseases. Front Immunol. (2017) 8:598. doi: 10.3389/fimmu.2017.00598
32. Rooks MG, Garrett WS. Gut microbiota, metabolites and host immunity. Nat Rev Immunol. (2016) 16:341–52. doi: 10.1038/nri.2016.42
33. Liu S, An Y, Cao B, Sun R, Ke J, Zhao D. The composition of gut microbiota in patients bearing Hashimoto's thyroiditis with euthyroidism and hypothyroidism. Int J Endocrinol. (2020) 2020:5036959. doi: 10.1155/2020/5036959
34. Su X, Zhao Y, Li Y, Ma S, Wang Z. Gut dysbiosis is associated with primary hypothyroidism with interaction on gut-thyroid axis. Clin Sci Lond Engl. (1979) (2020) 134:1521–35. doi: 10.1042/CS20200475
35. Wu F, Guo X, Zhang J, Zhang M, Ou Z, Peng Y. Phascolarctobacterium faecium abundant colonization in human gastrointestinal tract. Exp Ther Med. (2017) 14:3122–6. doi: 10.3892/etm.2017.4878
36. O'Callaghan A, van Sinderen D. Bifidobacteria and their role as members of the human gut microbiota. Front Microbiol. (2016) 7:925. doi: 10.3389/fmicb.2016.00925
37. Patole SK, Rao SC, Keil AD, Nathan EA, Doherty DA, Simmer KN. Benefits of Bifidobacterium breve M-16V supplementation in preterm neonates - a retrospective cohort study. PLoS ONE. (2016) 11:e0150775. doi: 10.1371/journal.pone.0150775
38. Saez-Lara MJ, Gomez-Llorente C, Plaza-Diaz J, Gil A. The role of probiotic lactic acid bacteria and bifidobacteria in the prevention and treatment of inflammatory bowel disease and other related diseases: a systematic review of randomized human clinical trials. BioMed Res Int. (2015) 2015:505878. doi: 10.1155/2015/505878
39. Azad MAK, Sarker M, Li T, Yin J. Probiotic species in the modulation of gut microbiota: an overview. BioMed Res Int. (2018) 2018:9478630. doi: 10.1155/2018/9478630
40. Qi H, Li Y, Yun H, Zhang T, Huang Y, Zhou J, et al. Lactobacillus maintains healthy gut mucosa by producing L-Ornithine. Commun Biol. (2019) 2:171. doi: 10.1038/s42003-019-0424-4
41. Patil AD. Link between hypothyroidism and small intestinal bacterial overgrowth. Indian J Endocrinol Metab. (2014) 18:307–9. doi: 10.4103/2230-8210.131155
42. Yao Z, Zhao M, Gong Y, Chen W, Wang Q, Fu Y, et al. Relation of gut microbes and L-thyroxine through altered thyroxine metabolism in subclinical hypothyroidism subjects. Front Cell Infect Microbiol. (2020) 10:495. doi: 10.3389/fcimb.2020.00495
43. Laursen MF, Bahl MI, Michaelsen KF, Licht TR. First foods and gut microbes. Front Microbiol. (2017) 8:356. doi: 10.3389/fmicb.2017.00356
44. Zmora N, Suez J, Elinav E. You are what you eat: diet, health and the gut microbiota. Nat Rev Gastroenterol Hepatol. (2019) 16:35–56. doi: 10.1038/s41575-018-0061-2
45. Korem T, Zeevi D, Suez J, Weinberger A, Avnit-Sagi T, Pompan-Lotan M, et al. Growth dynamics of gut microbiota in health and disease inferred from single metagenomic samples. Science. (2015) 349:1101–6. doi: 10.1126/science.aac4812
46. Kolodziejczyk AA, Zheng D, Elinav E. Diet-microbiota interactions and personalized nutrition. Nat Rev Microbiol. (2019) 17:742–53. doi: 10.1038/s41579-019-0256-8
47. Yap YA, Mariño E. An insight into the intestinal web of mucosal immunity, microbiota, and diet in inflammation. Front Immunol. (2018) 9:2617. doi: 10.3389/fimmu.2018.02617
48. Cândido FG, Valente FX, Grześkowiak ŁM, Moreira APB, Rocha DMUP, Alfenas R de CG. Impact of dietary fat on gut microbiota and low-grade systemic inflammation: mechanisms and clinical implications on obesity. Int J Food Sci Nutr. (2018) 69:125–43. doi: 10.1080/09637486.2017.1343286
49. Zou J, Chassaing B, Singh V, Pellizzon M, Ricci M, Fythe MD, et al. Fiber-mediated nourishment of gut microbiota protects against diet-induced obesity by restoring IL-22-mediated colonic health. Cell Host Microbe. (2018) 23:41–53.e4. doi: 10.1016/j.chom.2017.11.003
50. Matt SM, Allen JM, Lawson MA, Mailing LJ, Woods JA, Johnson RW. Butyrate and dietary soluble fiber improve neuroinflammation associated with aging in mice. Front Immunol. (2018) 9:1832. doi: 10.3389/fimmu.2018.01832
51. Sepahi A, Liu Q, Friesen L, Kim CH. Dietary fiber metabolites regulate innate lymphoid cell responses. Mucosal Immunol. (2020) 15:1–14. doi: 10.1038/s41385-020-0312-8
52. Tabasi M, Eybpoosh S, Sadeghpour Heravi F, Siadat SD, Mousavian G, Elyasinia F, et al. Gut microbiota and serum biomarker analyses in obese patients diagnosed with diabetes and hypothyroid disorder. Metab Syndr Relat Disord. (2020) 24:1–8. doi: 10.21203/rs.3.rs-46107/v1
53. Küçükemre Aydin B, Yildiz M, Akgün A, Topal N, Adal E, Önal H. Children with Hashimoto's thyroiditis have increased intestinal permeability: results of a pilot study. J Clin Res Pediatr Endocrinol. (2020) 12:303–7. doi: 10.4274/jcrpe.galenos.2020.2019.0186
54. Fasano A. Zonulin and its regulation of intestinal barrier function: the biological door to inflammation, autoimmunity, and cancer. Physiol Rev. (2011) 91:151–75. doi: 10.1152/physrev.00003.2008
55. Fasano A. All disease begins in the (leaky) gut: role of zonulin-mediated gut permeability in the pathogenesis of some chronic inflammatory diseases. F1000Research. (2020) 9:1–12. doi: 10.12688/f1000research.20510.1
56. Drago S, El Asmar R, Di Pierro M, Grazia Clemente M, Tripathi A, Sapone A, et al. Gliadin, zonulin and gut permeability: effects on celiac and non-celiac intestinal mucosa and intestinal cell lines. Scand J Gastroenterol. (2006) 41:408–19. doi: 10.1080/00365520500235334
Keywords: intestinal dysbiosis, inflammatory cytokines, dietary habits, gut microbiota, Hashimoto thyroiditis, autoimmune disease, intestinal permeability
Citation: Cayres LCdF, de Salis LVV, Rodrigues GSP, Lengert AvH, Biondi APC, Sargentini LDB, Brisotti JL, Gomes E and de Oliveira GLV (2021) Detection of Alterations in the Gut Microbiota and Intestinal Permeability in Patients With Hashimoto Thyroiditis. Front. Immunol. 12:579140. doi: 10.3389/fimmu.2021.579140
Received: 09 October 2020; Accepted: 04 February 2021;
Published: 05 March 2021.
Edited by:
Yuan Kun Lee, National University of Singapore, SingaporeReviewed by:
Huaxi Yi, Ocean University of China, ChinaZhihong Sun, Inner Mongolia Agricultural University, China
Copyright © 2021 Cayres, de Salis, Rodrigues, Lengert, Biondi, Sargentini, Brisotti, Gomes and de Oliveira. This is an open-access article distributed under the terms of the Creative Commons Attribution License (CC BY). The use, distribution or reproduction in other forums is permitted, provided the original author(s) and the copyright owner(s) are credited and that the original publication in this journal is cited, in accordance with accepted academic practice. No use, distribution or reproduction is permitted which does not comply with these terms.
*Correspondence: Gislane Lelis Vilela de Oliveira, Z2xlbGlzdmlsZWxhQGdtYWlsLmNvbQ==
†These authors have contributed equally to this work