- Department of Hematology, Transplantology and Internal Medicine, Medical University of Warsaw, Warsaw, Poland
During recent years considerable progress has been made in the treatment of multiple myeloma. However, despite the current improvements in the prognosis of this malignancy, it always ends with relapse, and therefore new therapy approaches for destroying resistant cancer cells are needed. Presently, there is great hope being placed in the use of immunotherapy against refractory/relapsed multiple myeloma which is unresponsive to any other currently known drugs. The most promising one is CAR-T cell therapy which has already shown tremendous success in treating other malignancies such as acute lymphoblastic leukaemia (ALL) and could potentially be administered to multiple myeloma patients. CAR-T cells equipped with receptors against BCMA (B-cell maturation antigen), which is a surface antigen that is highly expressed on malignant cells, are now of great interest in this field with significant results in clinical trials. Furthermore, CAR-T cells with other receptors and combinations of different strategies are being intensively studied. However, even with CAR-T cell therapy, the majority of patients eventually relapse, which is the greatest limitation of this therapy. Serious adverse events such as cytokine release syndrome or neurotoxicity should also be considered as possible side effects of CAR-T cell therapy. Here, we discuss the results of CAR-T cell therapy in the treatment of multiple myeloma, where we describe its main advantages and disadvantages. Additionally, we also describe the current results that have been obtained on using combinations of CAR-T cell therapies with other drugs for the treatment of multiple myeloma.
Introduction
Multiple myeloma (MM) remains an incurable haematological malignancy although substantial progress in the treatment has occurred in recent years (1). The introduction of immunomodulatory drugs (IMiDs) such as thalidomide, lenalidomide, and pomalidomide has resulted in significant improvements for the long-term survival and the quality of life of MM patients (2, 3). The other drugs, namely proteasome inhibitors (bortezomib, carfilzomib, ixazomib), immune checkpoint inhibitors, and monoclonal antibodies have contributed substantially to improve the prognosis of MM patients (2, 4). Apart from these agents, another way of treatment is autologous stem-cell transplantation (ASCT), which is prescribed for patient populations with limited comorbidities and in good overall condition (5). Despite the progress made, MM generally progresses towards relapse/refractory to the available therapies (6). This creates an unmet need for new types of treatments, especially those which are based on a distinct mechanism of action. During recent years many new ideas have appeared and one of the most promising is chimeric antigen receptor T cell (CAR-T) therapy, which has already succeeded in the treatment of other haematological malignancies such as acute lymphoblastic leukaemia (ALL) and diffuse large B cell lymphoma and was approved by the FDA and EMA (7). In this review, our goal is to summarize the results, present challenges, and to describe new ideas for CAR-T cell treatment in refractory/relapsed multiple myeloma patients.
Multiple Myeloma
Multiple myeloma (MM) is one of the most common malignancies of the hematopoietic system (15%) and accounts for approximately 1% of all neoplasms. Multiple myeloma typically affects elderly patients with a median age of 70 and with 63% of them being older than 65 (8). Although the prognosis in this disease is now much better than a few years ago, there are individual differences in survival that range from progression-free survival of more than 15 years in some patients (9), while approximately 15% of patients reach median survival of less than 2 years (10). The differences in survival outcomes occur because MM is a molecularly very heterogeneous disease (11). Additionally, the heterogeneity of this disease makes it improbable to develop a single molecularly targeted therapy (12). While progress can be made by searching for such a therapy for each distinct subset of MM, another way to approach the problem is to focus on cell surface targets that are common to all or a majority of MM cases. However, the main problem is that only a small fraction of antigens are common to myeloma cells while not being simultaneously expressed in other healthy tissues. Immunotherapies that include monoclonal antibodies, bi-specific T-cell engagers (BiTE), and CAR-T cell therapy are examples of such an approach with outstanding results in some cases.
Chimeric Antigen Receptor T Cell Therapy
Chimeric antigen receptor T (CAR-T) cells are genetically engineered by the introduction of a construct coding for a receptor that is specific for target neoplastic cells. Hence, these cells are redirected to the selected target molecules on the surface of cancer cells. Specific CAR-T cell binding to targeted cancer cells triggers their death without major histocompatibility complex restriction (13). This approach provides the opportunity to overcome tumor escape mechanisms that can lead to cancer cell survival, such as i) the down-regulation of MHC molecules, ii) the processing of aberrant antigens, and iii) the creation of an immunosuppressive milieu which deactivates T cells (14). That being mentioned, it seems logical that this novel technique should find its application especially in tumors that rely on immunoregulatory mechanisms, and a good example of such malignancy is multiple myeloma.
CARs consist of four different elements: the recognition domain, the extracellular spacer, costimulatory elements, and the activating endodomain (15). The recognition domain [most common is a single-chain variable fragment—scFv (16)] binds to its ligand on the cell surface (signal 1), where the extracellular spacer provides the optimal space between the two cells to allow activation of the CAR-T cells and not suppression. Once binding to the ligand has occurred, the costimulatory molecule (CD28 or 4-1BB) becomes activated and initiates the response for signal 2; the onset of signal 2 is vital for optimal CAR-T cell activation and the prevention of anergy. The propagation of signals 1 and 2 are then transmitted to the activating domain (CD3-zeta) where both signals are forwarded to the CAR-T cell for activation (17).
The most important factor that determines the success of CAR-T therapy is the selection of target antigen. The first requirement in the process of designing CARs is to verify that the selected molecule is uniformly and sufficiently expressed on tumor cells. The second one is the absence or limited expression of the selected molecule on the normal cell surface. Otherwise, CAR-T cell therapy could lead to the debulking of the tumor but also to serious side effects with the deterioration of related normal cells (18). And that is exactly the main problem with multiple myeloma because it presents exceptional heterogeneity that effectively constricts the search for new antigens (19). However, some targets are now being used in clinical trials with promising results. One of them is CD19, with impressive results in the treatment of acute lymphoblastic leukaemia (20–23). Other antigens being under investigation are CD44v6, CD70, CD56, CD38, CD138, signaling lymphocyte-activating molecule F7 SLAMF7 (CS1), K light chain, NKG2D, and the most promising one about which we are particularly focused on in this review: B-cell maturation antigen (BCMA) (13, 15) (Figure 1).
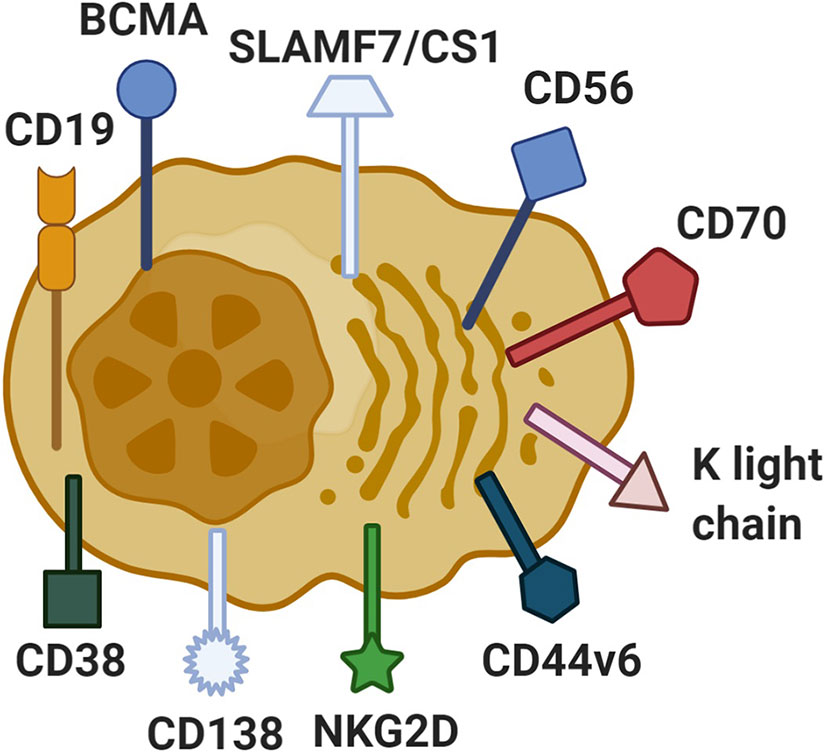
Figure 1 Antigens on multiple myeloma cells used as a target in CAR-T cell treatment. In the process of producing CAR-T cells, the main aim is to find the appropriate antigen which could be characterized by high expression levels on tumor cells and no expression on other healthy cells. The most known antigens used in the refractory/relapsed multiple myeloma CAR-T cell treatment are CD138, CD70, NKG2D, kappa light chain, CD19, SLAMF7/CS1, CD44v6, CD56, CD38, and BCMA. Created with BioRender.com.
The manufacturing of CAR-T cells involves the collection of patients’ T cells using apheresis as the first step. Once the T-cells are collected, they are further purified and activated with cytokines in vitro. Then they are transduced with viral vectors (retrovirus, lentivirus) containing a genetic construct coding for CAR. The efficiency of the transduction is in the range reaching up to 30% depending on the technique. After this, genetically engineered cells are further expanded, aliquoted, and stored frozen. In the meantime, the patient is receiving lymphocyte depleting chemotherapy to avoid rejection and enable the expansion of modified autologous CAR-T-cells. The procedure ends with the infusion of CAR-T cells to the patient in a specific dosing regimen (13).
First-Generation B-Cell Maturation Antigen-Targeted Chimeric Antigen Receptor T Cell
As mentioned, the crucial problem in designing functional CAR-T cells is the selection of the appropriate antigen. Its expression on target cells should be uniform and preferably it should not be present on healthy cells. The problem is that the majority of antigens present on neoplastic cells are also expressed on some other normal cells.
The best known and explored target for CAR-T cells so far is CD19. This is an antigen of normal B cells that is also present on B cell leukemias and lymphomas (24, 25). But when it comes to multiple myeloma, which is also B cell neoplasia, CD19 is present only on a subset of malignant cells (26). Therefore, there is a risk that anti CD19 CAR-T cells would not eliminate myeloma, although it is also hypothesized that CD19+ component has myeloma stem cell functionality (27). Also, attempts to use anti-CD19 CAR-T cells in MM gave inconsistent results and will be reviewed later. This is why the search for other, more commonly expressed antigens on myeloma cells was undertaken. Among a few such candidates, B-cell maturation antigen (BCMA) exhibited the most favorable characteristics. Carpenter et al. showed that BCMA is present almost on all multiple myeloma cells and on none of 34 different tissues as well as on CD34+ hematopoietic stem cells (28). On the other hand, its expression was also found in normal plasma cells and mRNA for its production was noticed in the trachea and gastrointestinal organs which was proven to be associated with B cells in lamina propria and Peyer’s patches (28).
BCMA (CD269) is a member of the TNF receptor superfamily and is present on plasma cells and a small fraction of B cells (29). It binds B-cell activating factor (BAFF) or a proliferation-inducing ligand (APRIL) (30). Although it is particularly found on the cell surface, it can be also released into the serum when it is cleaved from the cell surface by γ-secretase. Patients with high infiltration of bone marrow with MM cells show elevated concentration levels of soluble BCMA in serum; this observation has proven to be particularly useful for the evaluation of CAR-T therapy (31).
Carpenter et al. conducted a few preclinical studies in which they have shown that transduced T cells demonstrated interesting features. CAR-T cells collected from patients could efficiently kill cells that possessed BCMA antigen on its surface even when soluble BCMA was added to the cells’ environment. These in vitro conditions reflect the bone marrow environment where BCMA is present on the cell surface and in the soluble form (28).
Human clinical trials on CAR-T cells in MM were first conducted with the same anti-BCMA CAR-T cells that were reported in the previous paragraph (28). Ali et al. selected 12 patients with a median of seven lines of previous treatments and administered to them increasing numbers of CAR-T cells 0.3 × 106 cells/kg through 1 × 106, 3 × 106 up to the highest dose of 9 × 106 cells/kg. The highest dose (9 × 106 cells/kg) demonstrated the best clinical efficacy but also the most intensive adverse effects (32). All 12 patients experienced toxicities and each of them had cytopenias (mostly transient and attributed to conditioning therapy except in the case of two patients whose symptoms were protracted) that were easily managed. Another adverse event was cytokine release syndrome (CRS) with the greatest intensity in patients receiving the highest dose. CRS was characterized by fever, tachycardia, hypotension, hypoxemia, dyspnoea, and in one patient even with delirium. These symptoms were alleviated with the use of anti-IL 6 antibody tocilizumab and vasopressors. Some patients had elevated serum creatine phosphokinase levels with muscle weakness. Because of the BCMA presence on the normal plasma cells, the level of polyclonal serum antibodies dropped and some patients required intravenous immunoglobulin infusions to avoid infections (32). The best response achieved was with a patient whose bone marrow was 80% infiltrated with MM cells and for 28 weeks he had no detectable IgG lambda in the serum. Eventually, the patient relapsed which could be attributed to a small subset of MM cells which did not express BCMA on its surface and therefore were not selected by anti-BCMA CAR-T cell therapy (32). A few other interesting observations have been made after CAR-T cell infusion. Before CAR-T cell infusion the median CD8+/CD4+ T cell ratio was 1.1 but two weeks following the treatment CD8 positive cells became the main population of T cells. Moreover, these cytotoxic cells expressed new antigens such as PD-1 on their surface which are associated with senescence, exhaustion, and a decrease in proliferation ability. Summarizing, not only the loss or lack of antigen could contribute to eventual relapse but also loss of the efficient CAR-T cells (32).
In the continuation of this trial, Brudno et al. treated the next 24 patients with different dosages of CAR-T cells ranging from 0.3 × 106 cells/kg, 1 × 106, 3 × 106 to 9 × 106 cells/kg (33). Of the 10 patients who received 0.3–3 × 106 cells/kg, only two showed partial or better responses to the treatment. Among the other 16 patients (two of them were treated a second time after the first clinical trial (32)) treated with 9 × 106 cells/kg, the majority experienced partial or better responses but unfortunately the better the response was, the more intensive the adverse events were. Some of the responses exhibited symptoms of cytokine release syndrome (CRS) that was treated with tocilizumab, the others showed cytopenias with the most frequent being thrombocytopenia which was managed with the thrombopoetin agonist eltrombopag and prednisone. What was particularly interesting was the fact that patients with grades 3 and 4 of CRS also had higher numbers of MM cells in the bone marrow (33).
Moreover, the level of soluble serum BCMA decreased substantially after treatment with CAR-T cells, while during progression its concentration started to increase. This observation leads to the conclusion that soluble BCMA could be used as a predictive biomarker for CAR-T cell therapy response and also for early progression (33).
Second-Generation B-Cell Maturation Antigen-Targeted Chimeric Antigen Receptor T Cell (Idecabtagene Vicleucel)
After promising results in applying first-generation CAR-T cells, a search was initiated for determinants of better responses in MM patients. Several experiments have shown a significant positive correlation between peak CAR-T cell blood levels and clinical responses (34, 35). Furthermore, patients with better responses to treatment have been proven to have increased CAR-T cells that persist in the blood over a prolonged period of time (32, 33). Friedman et al. reasoned that the type of costimulatory molecule that is incorporated in the engineering of CAR-T cells could substantially affect both serum peak levels and persistence of CAR-T cells in blood. With that concept in mind, they designed T cells with chimeric antigen receptors but instead of CD28 as a costimulatory domain, as utilized in the previous preclinical and clinical experiments, they used another molecule—4-1BB (CD137) (36). Furthermore, an additional reason for changing the costimulatory domain was the high intensity and kinetics of CAR-T cell activation when CD28 is utilized. This high intensity and kinetics of CAR-T cell activation leads to the initiation of early-onset CRS. Moreover, T-cells with CD28 produced increased levels of Th-2 cytokines which mitigated their ability to kill cancer cells (37, 38) (Figure 2).
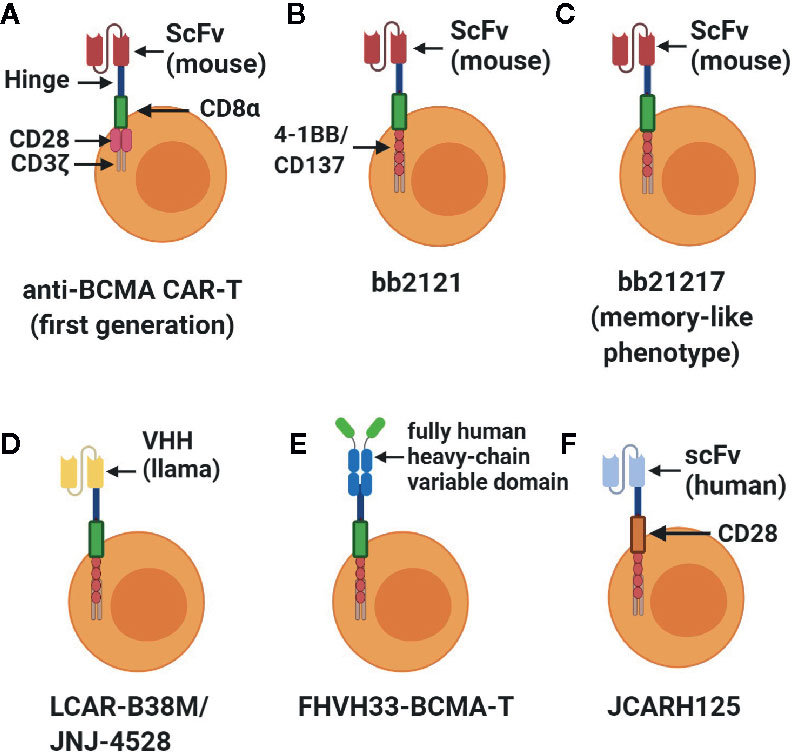
Figure 2 Different constructs of CARs targeting BCMA. BCMA is an antigen that is the most studied in CAR-T cells for relapsed/refractory MM. In use are the following types of anti-BCMA CAR-T cells (A) first generation of anti-BCMA CAR-T cells which consists of scFv as a binding molecule, hinge, transmembrane molecule (CD8α), costimulatory molecule (CD28), and an activating domain (CD3ζ); (B) the main change in the second generation of anti-BCMA CAR-T cells named bb2121 (Indecabtagene Vicleucel) was a new costimulatory molecule (4-1BB/CD137); (C) bb21217 differed from bb2121 with a memory-like phenotype of the former; (D) LCAR38M/JNJ-4528 are identical cells possessing single variable fragment of heavy chains (llama) instead of scFv (mouse) as a binding domain which can bind two different epitopes of BCMA; (E) FHVH33-BCMA-T as a binding domain was used with a fully human heavy-chain variable domain; (F) in the JCAR125 (Orvacabtagene Autoleucel) binding domain consists of a human scFv and a transmembrane domain costimulatory molecule (CD28). Created with BioRender.com.
bb2121
Raje et al. conducted a multicenter, open-label phase I trial with newly designed anti-BCMA CAR-T cells known as bb2121 which exhibited desired characteristics in preclinical experiments (39). They selected 33 patients with refractory/relapsed MM with a median age of 60 years and divided treatment into two phases. The first phase was with a dose escalation (50, 150, 450, and 800 × 106 cells; 21 patients) in which the main aim was to evaluate the maximum tolerated dose (MTD). The second phase was with a dose expansion (150 to 450 × 106 cells; 12 patients) where patients were treated with the estimated MTD to collect additional data (40). Both groups of patients were pre-treated with lymphodepleting therapy using fludarabine 30 mg/m2 and cyclophosphamide 300 mg/m2 over three days: –5, –4, and –3 prior to cell infusion. According to this and previous experiments, it seems unlikely that chemotherapy contributes to the outcomes because there were no responses in patients administered with subtherapeutic doses of anti-BCMA CAR-T cells (32). All but one patient received autologous stem cell transplantation previously (39).
The most frequent side effect of CAR-T cell treatment was cytopenia, especially neutropenia which occurred in 85% of patients and lasting a median of 10.9 months. Additionally, 75% of patients showed symptoms of CRS which occurred typically on the second day after infusion and persisted for 5 days on the average. Analysis of the severity of CRS showed a correlation between CRS score and the dose of the cells, CRP serum level, TNF alfa, ferritin level, and BCMA expression on MM cells. What is more, the peak presence of CAR-T cells in the blood was significantly higher in patients with a score of 3 and 4 on the scale of CRS. Twenty-one percent of patients received tocilizumab, and 12% were given corticosteroid to alleviate the symptoms of CRS. Furthermore, what is important is that these treatments did not affect CAR-T efficacy. Another side effect of the therapy was infections (42%), but they were easily controlled with antimicrobial agents (39).
The objective response rate (ORR) in all patients was 85%, and median PFS was 11.8 months. Forty-five percent achieved a complete response, and there was a significant effect of the dose of infused cells on the duration and frequency of response. Very good partial response (VGPR) occurred only in patients receiving at least 150 × 106 CAR-T cells. Persistence of CAR-T in the host was improved compared to previous trials with 96, 86, 57, and 20% CAR-T cells after 1, 3, 6, and 12 months after infusion, respectively. In this study, 4-1BB instead of CD28 was used as a costimulatory domain. Another interesting remark was that the level of BCMA expression did not influence the patient’s response to CAR-T cell therapy (39). This could be because for anti-BCMA CAR-T cells only 222 BCMA molecules per cell were sufficient to induce cytotoxicity in in vitro experiments. Normally, MM cells exhibit far higher expression of this antigen on its surface (36).
While the main drawback of this trial was a small study group, it compared favourably to other salvage regimens for refractory and relapsed MM such as pomalidomide plus dexamethasone with a response of 31% (41) and BCMA directed antibody with a response of 60% (42).
During ASCO20 (American Society of Clinical Oncology Annual Meeting 2020), Munshi presented a multicenter, single-arm phase II trial with Indecabtagene Vicleucel for R/R MM. Enrolled patients (n = 140) were already pretreated with ≥3 prior regimens (Imbeds, PIs, anti-CD38 mAbs) and refractory to their last regimen per International Myeloma Working Group (IMWG) criteria (43). After leukapheresis, 88% of patients were administered with bridging therapy ≥14 days before lymphodepletion, which was conducted with cyclophosphamide 300 mg/m2 and fludarabine 30 mg/m2 on days −5, −4, and −3. Eventually, 128 patients received CAR-T cells and among them, four patients were treated with 150 × 106 cells, 70 patients with 300 × 106 cells, and 54 patients with 450 × 106 cells. At data cut-off (16 Oct 2019) ORR was 73% and median PFS 8.6 months. Additionally, these values were significantly higher as the dose of CAR-T cells increased. Cytopenias occurred in 97% and CRS in 84% of patients but were most frequent within a group of higher CAR-T cell doses. Persistence and peak exposure was higher in responders when compared to non-responders (44).
bb21217
There is evidence indicating that the persistence of CAR-T cells post-infusion is a determinant of the duration of the response. Other studies have shown that memory-like T cells represent a more potent and persistent phenotype of CAR-T cells compared to the unselected type. Therefore, enriching CAR-T cells in memory-like phenotype could lead to enhanced and prolonged responses. Such a change in phenotype of T cells may be reached by the utilization of phosphoinositide 3-kinase inhibitor bb007 during the ex vivo culture of CAR-T bb2121 cells. The product of this procedure named bb21217 after showing efficacy in preclinical studies was used in first-in-human study CRB-402 (45).
LCAR-B38M
Zhao et al. designed dual epitope-binding CAR-T cells and utilized them in the first-in-human study named LEGEND-2, which was a multicenter on-label study conducted in China. LCAR-B38M cells have been created to bind to two different epitopes on BCMA target antigens on MM cells. This was intended to confer increased avidity of the cell binding to the target. Consequently, the dose of CAR-T cells could be lowered which was anticipated to decrease the frequency of the adverse events. Fifty-seven patients with refractory/relapsed MM and a median age of 54 were enrolled. All of them received conditioning therapy on days −5, −4, and −3 before infusion which consisted only of one agent—cyclophosphamide in a dose of 300 mg/m2 (the rationale for changing lymphodepletion protocol compared to other trials was safety). Also, the treatment regimen was distinct when compared to previous experiments. Patients having received a median dose of 0.5 × 106 cells/kg split into three doses (20% + 30% + 50% of total dose over 7 days). ORR in this study was 88% (50 out of 57 patients exhibited a clinical response), CR occurred in 68% of patients, VGPR in 9%, and PR in 14%. The median duration of response at cut-off day was 14 months and PFS was 15 months. BCMA expression did not correlate with the response to the therapy. Although decreased doses of cells were administered, the adverse events occurred in the majority of patients. Ninety-one percent of patients developed pyrexia, 90% CRS, 49% thrombocytopenia, and 47% leukopenia. Stage 1 CRS occurred in 47%, stage 2 in 35% and stage 3 in 7% of patients. One patient developed grade 1 neurotoxicity: aphasia, agitation, and seizure-like behavior (46).
At the ASCO20 Meeting, Berdeja presented the results of the CARTITUDE-1 study with a median follow-up of 9 months when phase 1b was complete with n = 29. The CAR-T cells that were used were identical to LCAR-B38M cells used in the Chinese study. The median dose of the CAR-T cells was 0.73 ×106 cells/kg administered in a singular infusion. The ORR for patients was 100%, where 76% had a stringent complete response. Further, 21% of the patients had VGPRs, and 3% had PR. This study showed that after 6 months 22/28 patients had a level of JNJ-4528 cells that was below the level of quantification, suggesting that the response did not correlate with CAR-T persistence in the peripheral blood (47, 48).
In another study, scientists investigated whether lymphodepletion therapy influences CAR-T cell function. They created anti-BCMA CAR-T cells with 4-1BB as a costimulatory domain and divided 25 patients into three cohorts as follows: cohort 1 with a range of infused cells: 1–5 × 108 without lymphodepleting agents, cohort 2 with a range 1–5 × 107 CART-BCMA cells preceded by cyclophosphamide 1.5 g/m2 and cohort 3 with a range 1–5 × 108 CART-BCMA cells plus cyclophosphamide 1.5 g/m2. Percentage of responders in groups 1, 2, and 3 were as follows: 44, 20, and 64%. The longest response to therapy lasted for 32 months. Notable was the fact that BCMA expression on MM cells was significantly lower after CAR-T cell therapy (49).
FHVH33-CD8BBZ
FHVH33-CD8BBZ designed by Lam et al. is a type of CAR-T cells which instead of a scFv possess a fully human heavy-chain variable domain (FHVH) which is connected to the 4-1BB costimulatory domain to increase the persistence of CAR-T cells in the host. The fully human heavy-chain variable domain is less immunogenic than scFv and the use of this receptor should lead to less severe adverse events. This could be especially advantageous in the case of a second transplantation. Moreover, genes used for FHVH creation are smaller than scFv which results in better expression of CARs on T cells. This feature could be particularly useful when it comes to designing CAR-T cells that target two antigens simultaneously (50). Mikkilineni et al. were conducting the first clinical trial of FHVH33-CD8BBZ with encouraging results. Twelve patients were divided into 3 groups with a different dosing regimen of 0.75, 1.5, and 3 × 106 cells/kg. Ten patients out of 12 reached objective responses and CRS occurred in 11 patients. These results, while based on 12 patients only, have shown that the efficacy of CAR-T cells with FHVH could be reached at a far lower dosing regimen in comparison to products based on scFv (51).
JCARH125
EVOLVE is an open-label phase I/II study in which a CAR-T cell product named Orvacabtagene Autoleucel (JCARH125) was administered to 62 patients. These CAR-T cells differed from other types of anti-BCMA CAR-T cells in that their binding domain is fully human with low affinity for soluble BCMA. In this study, patients with at least ≥3 prior therapies including Imbeds, PIs, ASCT, and anti-CD38 mAbs with lack of response to the last administered regimen were infused with 300 × 106, 450 × 106, or 600 × 106 CAR-T cells. ORR was 92%, CR occurred in 36%, VGPR in 32% and PR in 24% of patients (52).
Other Promising Target Antigens In Chimeric Antigen Receptor T Cell Therapy Against Multiple Myeloma
Taking into consideration that the main cause of relapse MM after CAR-T cell therapy is the loss of BCMA antigen, it is the basis of rationale to search for other antigens that could overcome this problem (53).
SLAMF7/CS1
Signaling Lymphocytic Activation Family Member 7 (SLAMF7) is a robust marker of premalignant cells of MGUS and malignant cells in MM. The healthy cells that exhibit expression of SLAMF7 are: plasma cells, NK, and some CD8+ T cells, activated monocytes, and dendritic cells, and activated B cells (54, 55). This relative lack of specificity compared to BCMA requires changes in the treatment procedure. Therefore, SLAMF7 could be potentially used as a target of CAR-T cells only when associated with suicidal gene neighbouring CAR-T construct whose expression could be initiated on demand. An example of such a gene is inducible caspase 9 which is activated with rimiducid. This strategy aims to protect the patient from cytopenias in the case of severe adverse events (56). A functional antibody against SLAMF7 known as elotuzumab is already used in the treatment of MM, which confirms that it is a valuable target (57, 58). In a preclinical study, Wang et al. designed two CAR-T cell models. One targeted BCMA molecule whereas the second one was binding with SLAMF7. The effector function of both cells was similar although in a mouse model anti-SLAMF7 CAR-T cells proved to have better antitumor efficacy (59). Several SLAMF7-based CAR-T cells have entered clinical trial phases.
CD19
Garfall et al. presented a study in which another type of CAR-T cell was used that uses CD19 as target molecule instead of BCMA. These cells are currently utilized in the management of acute lymphoblastic leukaemia with success (7, 21). The main difference between BCMA and CD19 is its profile of expression—CD19 was proposed to be present on the surface of myeloma stem cells whereas BCMA is present both on myeloma cells and normal plasma cells (60). The authors decided to infuse patients with a history of previous autologous stem cell transplantation (ASCT) with CAR-T cells expressing receptor targeting CD19 (CTL019) following high-dose melphalan conditioning therapy and second ASCT. Two out of 10 patients after ASCT + CTL019 compared with the first ASCT showed significantly longer PFS. The authors of this study indicate two possible reasons for such low efficacy of the therapy. First, due to safety reasons, the combination of CTL019 and ASCT required a 10-fold dose reduction of the former compared to other studies of CTL019. Therefore, the CAR-T cell engraftment within the patient’s bone marrow was impaired and consequently CD19+ cells were not sufficiently targeted. The second possible explanation is the inter-patient heterogeneity in the case of CD19+ expression on myeloma stem cells. Authors suspect that the immunophenotype of this subset of myeloma cells not can only vary between patients but also change over time in individuals (61) (Table 1).
Bi-Specific Chimeric Antigen Receptor T Cells
In vitro experiments in which a mixture of two populations of CAR-T cells was administered (one population consisted of CD19 CAR-T cells and the second one of CD20 CAR-T cells) ended with unsatisfactory results. This is probably because of the different proliferation rates in these two populations which led to selective expansion of one population at the expense of the second one (62). One way to avoid competition between two populations of CAR-T cells is bi-specific CAR-T cells which express two receptors for two different target antigens on one T cell. In theory, it should allow CAR-T cells to debulk tumors containing both or one of the targeted molecules.
B-Cell Maturation Antigen + Transmembrane Activator and Calcium-Modulator and Cyclophilin Ligand
A proliferation-inducing ligand (APRIL) is a natural ligand for both BCMA and transmembrane activator and calcium-modulator and cyclophilin ligand (TACI). According to Lee et al., TACI expression on human MM is limited to 78%, whereas BCMA was found on cells from all 50 tested patients. They have created bi-specific CAR-T cells which apart from BCMA targeted also TACI on MM cells. Reported results showed the efficacy of this approach in an in vivo model of tumor escape where BCMA+TACI− and BCMA−TACI+ cells were equally killed by CAR-T cells, whereas scFv bearing CAR-T cells against BCMA only was insufficient to prevent the outgrowth of BCMA-negative tumor (63).
B-Cell Maturation Antigen + G Protein-Coupled Receptor Class C Group 5 Member D
G protein-coupled receptor class C group 5 member D (GPRC5D) was another antigen used for the creation of bi-specific CAR-T cells with BCMA. De Larrea et al. showed that in mice not only parallel infusion of anti-BCMA and anti-GPRC5D CAR-T cells could result in preventing tumor escape but also a single bicistronic vector encoding two 4-1BB-containing CARs has a significant efficacy and additionally avoids some problems with a parallel production of both CAR-T containing cells (64).
Chimeric Antigen Receptor T Cell With Other Agents
γ-Secretases Inhibitors
As mentioned before, the main obstacle that makes patients vulnerable to tumor relapse is the loss of the target antigen. A possible strategy to avoid at least some of the relapses could be the administration of inhibitors of γ-secretases, the enzymes responsible for BCMA loss from MM cell surface. Treatment of MM in NOD/SCID/gamma c−/− mice has a notable impact on the antitumor efficacy of anti-BCMA CAR-T cells alone. Furthermore, the antigen cleavage from the cell surface is decreased leading to less soluble antigen accumulation in the bone marrow which could improve antigen recognition by CAR-T cells and consequently MM cells being killed. The FDA-approved clinical trial (NCT03502577) has been initiated where gamma-secretase inhibitors are combined with anti-BCMA CAR-T cells (65).
Lenalidomide
Wang et al. showed increased in vitro and in vivo effect of lenalidomide addition to genetically engineered T cells. Lenalidomide added during in vitro transduction and expansion of CAR-T cells increased cytotoxicity, memory maintenance, Th1 cytokine production, and immune synapse formation. Moreover, the addition of in vivo lenalidomide improved the persistence of anti-CS1 CAR-T cells in the host. These observations led to the design of a clinical trial combining lenalidomide and anti-CS1 CAR-T cells (59).
PD-1 Inhibitor
Bernabei et al. demonstrated the efficacy of PD-1 inhibitor combination with anti-BCMA CAR-T cells. In the clinical study, they administered pembrolizumab, PD-1 inhibitor to five patients with relapsed MM after treatment with CAR-T cells. This combination proved to be efficient in inducing re-expansion of T cells in relapsed patients. However, re-expansion occurred infrequently and serious adverse events were evident (66).
Summary And Future Directions
Previous studies have shown great efficacy of CAR-T cell therapy in MM in the context of remissions, even in a population of refractory/relapsed MM patients after many lines of treatment. It seems that the results of treatment are improving with the development of the new CAR constructs. Still, the main problem constitutes relapses of disease and shortness of remissions. Bearing in mind that relapses are frequently developed by MM cells possessing target antigens, it appears that future studies should be focused on improvements in the durability of response by prolonging the efficacy of CAR-T cells in the patient. That could be reached by modification of CAR constructs (especially in the costimulatory domain), use of different growth factors, and by avoiding CAR-T cell exhaustion (lower strength of binding to the antigen or decreasing intensiveness of activation signal; a combination of CAR-T cells with checkpoint inhibitors). Studies of CAR-T cells should be accompanied by studies of the biology of the cells that are responsible for relapses after CAR-T cell treatment. The study of the cells causing relapses can play a critical role in the search for new target antigens. Similar to transplantations, it seems that the combination of CAR-T cells with other drugs currently used in MM (new immunomodulatory drugs, proteasome inhibitors, or monoclonal antibodies) is the future of the field. Additionally, the conception of conditioning—CAR-T cell administration-consolidation/maintenance with new drugs or even a combination of CAR-T cell therapy with autologous/allogeneic transplantation requires deep consideration.
Conclusions
Given the fact that MM is the second most frequent cancer in haematological practice, the need for new treatment strategies is considerable. The most promising trials include CAR-T cells targeted against BCMA antigen but use of other antigens like CS1 or CD19 could also lead to a better prognosis for refractory/relapsed MM patients. Although a few CAR-T cell products are currently registered by the FDA in the US for the treatment of other haematological malignancies, we are still waiting for the next phases of clinical trials regarding the use of CAR-T cells in multiple myeloma refractory/relapsed patients. Observations of the use of CAR-T cells in other malignancies have shown that a similar strategy will be probably common in the future in a matter of a few years. However, we should not forget about the potentially serious side effects of this therapy. Only selected MM patients can benefit from CAR-T treatment, and therefore, studies are needed to identify these groups. Furthermore, the majority of patients relapse after a relatively short time, so also studies regarding methods of blocking mechanisms of tumor escape are necessary (61) (Table 2).
Author Contributions
All authors contributed to the article and approved the submitted version.
Conflict of Interest
The authors declare that the research was conducted in the absence of any commercial or financial relationships that could be construed as a potential conflict of interest.
References
1. Medical Masterclass C., Firth J. Haematology: multiple myeloma. Clin Med (Lond) (2019) 19(1):58–60. doi: 10.7861/clinmedicine.19-1-58
2. Soekojo CY, Ooi M, Mel S, Chng WJ. Immunotherapy in Multiple Myeloma. Cells (2020) 9(3):1–3. doi: 10.3390/cells9030601
3. Naymagon L, Abdul-Hay M. Novel agents in the treatment of multiple myeloma: a review about the future. J Hematol Oncol (2016) 9(1):52. doi: 10.1186/s13045-016-0282-1
4. Okazuka K, Ishida T. Proteasome inhibitors for multiple myeloma. Jpn J Clin Oncol (2018) 48(9):785–93. doi: 10.1093/jjco/hyy108
5. Koniarczyk HL, Ferraro C, Miceli T. Hematopoietic Stem Cell Transplantation for Multiple Myeloma. Semin Oncol Nurs (2017) 33(3):265–78. doi: 10.1016/j.soncn.2017.05.004
6. Robak P, Drozd I, Szemraj J, Robak T. Drug resistance in multiple myeloma. Cancer Treat Rev (2018) 70:199–208. doi: 10.1016/j.ctrv.2018.09.001
7. Pehlivan KC, Duncan BB, Lee DW. CAR-T Cell Therapy for Acute Lymphoblastic Leukemia: Transforming the Treatment of Relapsed and Refractory Disease. Curr Hematol Malig Rep (2018) 13(5):396–406. doi: 10.1007/s11899-018-0470-x
8. Palumbo A, Anderson K. Multiple myeloma. N Engl J Med (2011) 364(11):1046–60. doi: 10.1056/NEJMra1011442
9. Barlogie B, Mitchell A, van Rhee F, Epstein J, Morgan GJ, Crowley J. Curing myeloma at last: defining criteria and providing the evidence. Blood (2014) 124(20):3043–51. doi: 10.1182/blood-2014-07-552059
10. Pawlyn C, Morgan GJ. Evolutionary biology of high-risk multiple myeloma. Nat Rev Cancer (2017) 17(9):543–56. doi: 10.1038/nrc.2017.63
11. Bolli N, Avet-Loiseau H, Wedge DC, Van Loo P, Alexandrov LB, Martincorena I, et al. Heterogeneity of genomic evolution and mutational profiles in multiple myeloma. Nat Commun (2014) 5:2997. doi: 10.1038/ncomms3997
12. Lohr JG, Stojanov P, Carter SL, Cruz-Gordillo P, Lawrence MS, Auclair D, et al. Widespread genetic heterogeneity in multiple myeloma: implications for targeted therapy. Cancer Cell (2014) 25(1):91–101. doi: 10.1016/j.ccr.2013.12.015
13. Mikkilineni L, Kochenderfer JN. Chimeric antigen receptor T-cell therapies for multiple myeloma. Blood (2017) 130(24):2594–602. doi: 10.1182/blood-2017-06-793869
14. Kershaw MH, Teng MWL, Smyth MJ, Darcy PK. Supernatural T cells: genetic modification of T cells for cancer therapy. Nat Rev Immunol (2005) 5(12):928–40. doi: 10.1038/nri1729
15. Rotolo A, Caputo V, Karadimitris A. The prospects and promise of chimeric antigen receptor immunotherapy in multiple myeloma. Br J Haematol (2016) 173(3):350–64. doi: 10.1111/bjh.13976
16. Sadelain M, Brentjens R, Riviere I. The basic principles of chimeric antigen receptor design. Cancer Discovery (2013) 3(4):388–98. doi: 10.1158/2159-8290.CD-12-0548
17. Zhang C, Liu J, Zhong JF, Zhang X. Engineering CAR-T cells. Biomark Res (2017) 5:22. doi: 10.1186/s40364-017-0102-y
18. Srivastava S, Riddell SR. Engineering CAR-T cells: Design concepts. Trends Immunol (2015) 36(8):494–502. doi: 10.1016/j.it.2015.06.004
19. Kuehl WM, Bergsagel PL. Molecular pathogenesis of multiple myeloma and its premalignant precursor. J Clin Invest (2012) 122(10):3456–63. doi: 10.1172/JCI61188
20. Malard F, Mohty M. Acute lymphoblastic leukaemia. Lancet (2020) 395(10230):1146–62. doi: 10.1016/S0140-6736(19)33018-1
21. Maude SL, Teachey DT, Porter DL, Grupp SA. CD19-targeted chimeric antigen receptor T-cell therapy for acute lymphoblastic leukemia. Blood (2015) 125(26):4017–23. doi: 10.1182/blood-2014-12-580068
22. Lee DW, Kochenderfer JN, Stetler-Stevenson M, Cui YK, Delbrook C, Feldman SA, et al. T cells expressing CD19 chimeric antigen receptors for acute lymphoblastic leukaemia in children and young adults: a phase 1 dose-escalation trial. Lancet (2015) 385(9967):517–28. doi: 10.1016/S0140-6736(14)61403-3
23. Park JH, Riviere I, Gonen M, Wang X, Senechal B, Curran KJ, et al. Long-Term Follow-up of CD19 CAR Therapy in Acute Lymphoblastic Leukemia. N Engl J Med (2018) 378(5):449–59. doi: 10.1056/NEJMoa1709919
24. Porter DL, Levine BL, Kalos M, Bagg A, June CH. Chimeric antigen receptor-modified T cells in chronic lymphoid leukemia. N Engl J Med (2011) 365(8):725–33. doi: 10.1056/NEJMoa1103849
25. Kochenderfer JN, Wilson WH, Janik JE, Dudley ME, Stetler-Stevenson M, Feldman SA, et al. Eradication of B-lineage cells and regression of lymphoma in a patient treated with autologous T cells genetically engineered to recognize CD19. Blood (2010) 116(20):4099–102. doi: 10.1182/blood-2010-04-281931
26. Gupta R, Bhascar A, Kumar L, Sharma A, Jain P. Flow cytometric immunophenotyping and minimal residual disease analysis in multiple myeloma. Am J Clin Pathol (2009) 132(5):728–32. doi: 10.1309/AJCP1GYI7EHQYUYK
27. Basak GW, Carrier E. The search for multiple myeloma stem cells: the long and winding road. Biol Blood Marrow Transplant (2010) 16(5):587–94. doi: 10.1016/j.bbmt.2009.10.024
28. Carpenter RO, Evbuomwan MO, Pittaluga S, Rose JJ, Raffeld M, Yang S, et al. B-cell maturation antigen is a promising target for adoptive T-cell therapy of multiple myeloma. Clin Cancer Res (2013) 19(8):2048–60. doi: 10.1158/1078-0432.CCR-12-2422
29. Laabi Y, Gras M, Brouet J, Berger R, Larsen C, Tsapis A. The BCMA gene, preferentially expressed during B lymphoid maturation, is bidirectionally transcribed. Nucleic Acids Res (1994) 22(7):1147–54. doi: 10.1093/nar/22.7.1147
30. Thompson JS, Schneider P, Kalled SL, Wang L, Lefevre EA, Cachero TG, et al. BAFF binds to the tumor necrosis factor receptor-like molecule B cell maturation antigen and is important for maintaining the peripheral B cell population. J Exp Med (2000) 192(1):129–35. doi: 10.1084/jem.192.1.129
31. Sanchez E, Li M, Kitto A, Li J, Wang CS, Kirk DT, et al. Serum B-cell maturation antigen is elevated in multiple myeloma and correlates with disease status and survival. Br J Haematol (2012) 158(6):727–38. doi: 10.1111/j.1365-2141.2012.09241.x
32. Ali SA, Shi V, Maric I, Wang M, Stroncek DF, Rose JJ, et al. T cells expressing an anti-B-cell maturation antigen chimeric antigen receptor cause remissions of multiple myeloma. Blood (2016) 128(13):1688–700. doi: 10.1182/blood-2016-04-711903
33. Brudno JN, Maric I, Hartmann SD, Rose JJ, Wang M, Lam N, et al. T Cells Genetically Modified to Express an Anti-B-Cell Maturation Antigen Chimeric Antigen Receptor Cause Remissions of Poor-Prognosis Relapsed Multiple Myeloma. J Clin Oncol (2018) 36(22):2267–80. doi: 10.1200/JCO.2018.77.8084
34. Kochenderfer JN, Somerville RPT, Lu T, Shi V, Bot A, Rossi J, et al. Lymphoma Remissions Caused by Anti-CD19 Chimeric Antigen Receptor T Cells Are Associated With High Serum Interleukin-15 Levels. J Clin Oncol (2017) 35(16):1803–13. doi: 10.1200/JCO.2016.71.3024
35. Brudno JN, Somerville RPT, Shi V, Rose JJ, Halverson DC, Fowler DH, et al. Allogeneic T Cells That Express an Anti-CD19 Chimeric Antigen Receptor Induce Remissions of B-Cell Malignancies That Progress After Allogeneic Hematopoietic Stem-Cell Transplantation Without Causing Graft-Versus-Host Disease. J Clin Oncol (2016) 34(10):1112–21. doi: 10.1200/JCO.2015.64.5929
36. Friedman KM, Garrett TE, Evans JW, Horton HM, Latimer HJ, Seidel SL, et al. Effective Targeting of Multiple B-Cell Maturation Antigen-Expressing Hematological Malignances by Anti-B-Cell Maturation Antigen Chimeric Antigen Receptor T Cells. Hum Gene Ther (2018) 29(5):585–601. doi: 10.1089/hum.2018.001
37. Milone MC, Fish JD, Carpenito C, Carroll RG, Binder GK, Teachey D, et al. Chimeric receptors containing CD137 signal transduction domains mediate enhanced survival of T cells and increased antileukemic efficacy in vivo. Mol Ther (2009) 17(8):1453–64. doi: 10.1038/mt.2009.83
38. van der Stegen SJ, Hamieh M, Sadelain M. The pharmacology of second-generation chimeric antigen receptors. Nat Rev Drug Discovery (2015) 14(7):499–509. doi: 10.1038/nrd4597
39. Raje N, Bardeja J, Lin Y, Siegel D, Jagannath S, Madduri D, et al. Anti-BCMA CAR T-Cell Therapy bb2121 in Relapsed or Refractory Multiple Myeloma. N Engl J Med (2019) 380(18):1726–37. doi: 10.1056/NEJMoa1817226
40. Iasonos A, O’Quigley J. Dose expansion cohorts in Phase I trials. Stat Biopharm Res (2016) 8(2):161–70. doi: 10.1080/19466315.2015.1135185
41. Miguel JS, Weisel K, Moreau P, Lacy M, Song K, Delforge M, et al. Pomalidomide plus low-dose dexamethasone versus high-dose dexamethasone alone for patients with relapsed and refractory multiple myeloma (MM-003): a randomised, open-label, phase 3 trial. Lancet Oncol (2013) 14(11):1055–66. doi: 10.1016/S1470-2045(13)70380-2
42. Trudel S, Lendvai N, Popat R, Voorhees PM, Reeves B, Libby EN, et al. Targeting B-cell maturation antigen with GSK2857916 antibody-drug conjugate in relapsed or refractory multiple myeloma (BMA117159): a dose escalation and expansion phase 1 trial. Lancet Oncol (2018) 19(12):1641–53. doi: 10.1016/S1470-2045(18)30576-X
43. Rajkumar SV, Dimopoulos MA, Palumbo A, Blade J, Merlini G, Mateos MV, et al. International Myeloma Working Group updated criteria for the diagnosis of multiple myeloma. Lancet Oncol (2014) 15(12):e538–48. doi: 10.1016/S1470-2045(14)70442-5
44. Munshi NC, Anderson LD, Shah N, Jagannath S, Bardeja JG, Lonial S, et al. Idecabtagene vicleucel (ide-cel; bb2121), a BCMA-targeted CAR T-cell therapy, in patients with relapsed and refractory multiple myeloma (RRMM): Initial KarMMa results. J Clin Oncol (2020) 38(15_suppl):8503–3. doi: 10.1200/JCO.2020.38.15_suppl.8503
45. Berdeja JG, Alsina M, Shah ND, Siegel DS, Jagannath S, Madduri D, et al. Updated Results from an Ongoing Phase 1 Clinical Study of bb21217 Anti-Bcma CAR T Cell Therapy. Blood (2019) 134(Supplement_1):927–7. doi: 10.1182/blood-2019-126660
46. Zhao WH, Liu Z, Wang BY, Chen YX, Cao XM, Yang Y, et al. A phase 1, open-label study of LCAR-B38M, a chimeric antigen receptor T cell therapy directed against B cell maturation antigen, in patients with relapsed or refractory multiple myeloma. J Hematol Oncol (2018) 11(1):141. doi: 10.1186/s13045-018-0681-6
47. Berdeja JG, Madduri D, Usmani SZ, Singh I, Zudaire E, Yeh TM, et al. Update of CARTITUDE-1: A phase Ib/II study of JNJ-4528, a B-cell maturation antigen (BCMA)-directed CAR-T-cell therapy, in relapsed/refractory multiple myeloma. J Clin Oncol (2020) 38(15_suppl):8505–5. doi: 10.1200/JCO.2020.38.15_suppl.8505
48. Madduri D, Usmani SZ, Jagannath S, Singh I, Zudaire E, Yeh TM, et al. Results from CARTITUDE-1: A Phase 1b/2 Study of JNJ-4528, a CAR-T Cell Therapy Directed Against B-Cell Maturation Antigen (BCMA), in Patients with Relapsed and/or Refractory Multiple Myeloma (R/R MM). Blood (2019) 134(Supplement_1):577–7. doi: 10.1182/blood-2019-121731
49. Cohen AD, Garfall AL, Stadtmauer EA, Melenhorst JJ, Lacey SF, Lancaster E, et al. B cell maturation antigen-specific CAR T cells are clinically active in multiple myeloma. J Clin Invest (2019) 129(6):2210–21. doi: 10.1172/JCI126397
50. Lam N, Trinklein ND, Buelow B, Patterson GH, Ojha N, Kochenderfer JN. Anti-BCMA chimeric antigen receptors with fully human heavy-chain-only antigen recognition domains. Nat Commun (2020) 11(1):283. doi: 10.1038/s41467-019-14119-9
51. Mikkilineni L, Manasanch EE, Lam N, Vanasse D, Brudno JN, Maric I, et al. T Cells Expressing an Anti-B-Cell Maturation Antigen (BCMA) Chimeric Antigen Receptor with a Fully-Human Heavy-Chain-Only Antigen Recognition Domain Induce Remissions in Patients with Relapsed Multiple Myeloma. Blood (2019) 134(Supplement_1):3230–0. doi: 10.1182/blood-2019-129088
52. Mailankody S, Jakubowiak AJ, Htut M, Costa LJ, Lee K, Ganguly S, et al. Orvacabtagene autoleucel (orva-cel), a B-cell maturation antigen (BCMA)-directed CAR T cell therapy for patients (pts) with relapsed/refractory multiple myeloma (RRMM): update of the phase 1/2 EVOLVE study (NCT03430011). J Clin Oncol (2020) 38(15_suppl):8504–4. doi: 10.1200/JCO.2020.38.15_suppl.8504
53. Timmers M, Roex G, Wang Y, Campillo-Davo D, Van Tendeloo VFI, Chu Y, et al. Chimeric Antigen Receptor-Modified T Cell Therapy in Multiple Myeloma: Beyond B Cell Maturation Antigen. Front Immunol (2019) 10:1613. doi: 10.3389/fimmu.2019.01613
54. Hsi ED, Steinle R, Balasa B, Szmania S, Draksharapu A, Shum BP, et al. CS1, a potential new therapeutic antibody target for the treatment of multiple myeloma. Clin Cancer Res (2008) 14(9):2775–84. doi: 10.1158/1078-0432.CCR-07-4246
55. Cannons JL, Tangye SG, Schwartzberg PL. SLAM family receptors and SAP adaptors in immunity. Annu Rev Immunol (2011) 29:665–705. doi: 10.1146/annurev-immunol-030409-101302
56. Di Stasi A, Tey SK, Dotti G, Fujita Y, Kennedy-Nasser A, Martinez C, et al. Inducible apoptosis as a safety switch for adoptive cell therapy. N Engl J Med (2011) 365(18):1673–83. doi: 10.1056/NEJMoa1106152
57. Friend R, Bhutani M, Voorhees PM, Usmani SZ. Clinical potential of SLAMF7 antibodies - focus on elotuzumab in multiple myeloma. Drug Des Devel Ther (2017) 11:893–900. doi: 10.2147/DDDT.S98053
58. Gogishvili T, Danhof S, Prommersberger S, Rydzek J, Schreder M, Brede C, et al. SLAMF7-CAR T cells eliminate myeloma and confer selective fratricide of SLAMF7(+) normal lymphocytes. Blood (2017) 130(26):2838–47. doi: 10.1182/blood-2017-04-778423
59. Wang X, Walter M, Urak R, Weng L, Huynh C, Lim L, et al. Lenalidomide Enhances the Function of CS1 Chimeric Antigen Receptor-Redirected T Cells Against Multiple Myeloma. Clin Cancer Res (2018) 24(1):106–19. doi: 10.1158/1078-0432.CCR-17-0344
60. Ghosh N, Matsui W. Cancer stem cells in multiple myeloma. Cancer Lett (2009) 277(1):1–7. doi: 10.1016/j.canlet.2008.08.005
61. Garfall AL, Stadtmauer EA, Hwang W, Lacey SF, Melenhorst JJ, Krevvata M, et al. Anti-CD19 CAR T cells with high-dose melphalan and autologous stem cell transplantation for refractory multiple myeloma. JCI Insight (2019) 4(4):9–10. doi: 10.1172/jci.insight.127684
62. Zah E, Lim MY, Silva-Benedict A, Jensen MC, Chen YY. T Cells Expressing CD19/CD20 Bispecific Chimeric Antigen Receptors Prevent Antigen Escape by Malignant B Cells. Cancer Immunol Res (2016) 4(6):498–508. doi: 10.1158/2326-6066.CIR-15-0231
63. Lee L, Draper B, Chaplin N, Philip B, Chin M, Galas-Filipowicz D, et al. An APRIL-based chimeric antigen receptor for dual targeting of BCMA and TACI in multiple myeloma. Blood (2018) 131(7):746–58. doi: 10.1182/blood-2017-05-781351
64. Fernandez de Larrea C, Staehr M, Lopez A, Chen Y, Purdon TJ, Ng KY, et al. Optimal Dual-Targeted CAR Construct Simultaneously Targeting Bcma and GPRC5D Prevents Bcma-Escape Driven Relapse in Multiple Myeloma. Blood (2019) 134(Supplement_1):136–6. doi: 10.1182/blood-2019-126145
65. Pont MJ, Hill T, Cole GO, Abbott JJ, Kelliher J, Salter AI, et al. gamma-Secretase inhibition increases efficacy of BCMA-specific chimeric antigen receptor T cells in multiple myeloma. Blood (2019) 134(19):1585–97. doi: 10.1182/blood.2019000050
66. Bernabei L, Garfall AL, Melenhorst JJ, Lacey SF, Stadtmauer EA, Vogl DT, et al. PD-1 Inhibitor Combinations As Salvage Therapy for Relapsed/Refractory Multiple Myeloma (MM) Patients Progressing after Bcma-Directed CAR T Cells. Blood (2018) 132(Supplement 1):1973–3. doi: 10.1182/blood-2018-99-119514
Keywords: multiple myeloma, CAR-T cells, T lymphocyte, B-cell maturation antigen (BCMA), immunotherapy, cytokine release syndrome
Citation: Jasiński M, Basak GW and Jedrzejczak WW (2021) Perspectives for the Use of CAR-T Cells for the Treatment of Multiple Myeloma. Front. Immunol. 12:632937. doi: 10.3389/fimmu.2021.632937
Received: 24 November 2020; Accepted: 11 January 2021;
Published: 24 February 2021.
Edited by:
Axel Schambach, Hannover Medical School, GermanyReviewed by:
Amorette Barber, Longwood University, United StatesRodabe N. Amaria, University of Texas MD Anderson Cancer Center, United States
Copyright © 2021 Jasiński, Basak and Jedrzejczak. This is an open-access article distributed under the terms of the Creative Commons Attribution License (CC BY). The use, distribution or reproduction in other forums is permitted, provided the original author(s) and the copyright owner(s) are credited and that the original publication in this journal is cited, in accordance with accepted academic practice. No use, distribution or reproduction is permitted which does not comply with these terms.
*Correspondence: Grzegorz W. Basak, Z3J6ZWdvcnouYmFzYWtAd3VtLmVkdS5wbA==