- Department of Medical Microbiology, School of Biology and Basic Medical Science, Medical College of Soochow University, Suzhou, China
Salmonella spvC gene, encoding a phosphothreonine lyase on host mitogen-activated protein kinases, facilitates systemic infection of Salmonella while the precise mechanisms remain elusive. Autophagy and pyroptosis dependent on the activation of inflammasomes, as parts of innate immune response, contribute to host defense against Salmonella infection. Recently, we reported that spvC could inhibit pyroptosis. To explore the effect of spvC on autophagy and the relationship between its function in pyroptosis and autophagy, infection models of macrophages J774A.1 and epithelial HeLa cells co-cultured with Salmonella Typhimurium wild type, spvC deletion, site-directed mutant which lacks phosphothreonine lyase activity, or complemented strain were established. The levels of LC3 turnover and Beclin 1 of J774A.1 cells were determined by western blot. Confocal laser scanning microscopy was used to visualize the autophagic flux after being transfected with mRFP-GFP-LC3 plasmid in HeLa cells. Results showed that SpvC inhibited autophagosome formation through its phosphothreonine lyase activity. Additionally, analysis of nucleotide-binding oligomerization domain, leucine-rich repeat and pyrin domain-containing 3 (NLRP3) and NLR with CARD domain-containing 4 (NLRC4) in J774A.1 cells indicated that spvC decreased the protein levels of NLRP3 and NLRC4, which were significantly changed by autophagy inhibitor Bafilomycin A1. Together, our observations reveal a novel mechanism of spvC in Salmonella pathogenesis and host inflammatory response via inhibiting autophagy and NLRP3 as well as NLRC4. These pathways and their subversion by diverse pathogen virulence determinants are expected to throw light on the design of anti-infective agents.
Introduction
Salmonella is a facultative intracellular pathogen that causes a serious threat to global public health. Centers for Disease Control and Prevention estimates approximately 1.35 million infections, 26,500 hospitalizations, and 420 deaths caused by Salmonella in the United States every year (1). Among 2600 Salmonella enterica serovars, Salmonella enterica serovar typhimurium (S. Typhimurium) is one of the most common isolates causing infection with a broad range of hosts (2). Therefore, it will be clinically important to develop new strategies to control S. Typhimurium infection. In particular, pathogenesis of S. Typhimurium requires the action of multiple virulence factors. SpvB and SpvC, virulence factors encoded within the Salmonella plasmid virulence (spv) operon, are responsible for pathogenicity of S. Typhimurium (3). Previously we reported a novel contribution of spvB to Salmonella pathogenesis through interfering with intracellular iron homeostasis (4). spvC, another essential factor of Salmonella virulence determinant encoding phosphothreonine lyase, suppresses intestinal inflammation and aggravates systemic dissemination through mitogen-activated protein kinase (MAPK) signaling pathway (5, 6). However, the underlying mechanisms have been only partly illuminated.
Intracellular pathogens invade mammalian host cells in membrane bound vesicles called phagosomes. Of note, autophagy is a process whereby a double-membrane structure (autophagosome) engulfs unnecessary invading pathogens and delivers them to the lysosome for degradation. But the pathogens have developed several survival mechanisms to prevent this degradation event (7). S. Typhimurium, including its effectors, has evolved to block host signaling cascades or even create favorable conditions for self-replication and survival by virtue of autophagy through specific mechanisms, so as to resist the host defense (8, 9). It has been reported that spvC is responsible for the anti-inflammatory effect of S. Typhimurium to facilitate bacterial dissemination, and the host can eliminate intracellular bacteria by autophagy, which can inhibit the further spread of bacteria. In light of this, we hypothesize that autophagy is also involved in spvC-mediated infection while the precise mechanism remains obscure.
Innate immune recognition is initiated by pattern-recognition receptors (PRRs), of which nucleotide-binding domain and leucine-rich repeat receptors (NLRs) function in the recognition of danger signals introduced into the host cell cytosol. Nucleotide-binding oligomerization domain, leucine-rich repeat and pyrin domain-containing 3 (NLRP3) responds to a diverse range of stimuli, including pathogens, microbial toxins, etc. NLRP3 plays a pivotal role in regulating live-or-die cell-fate decisions (10, 11). Inhibition of the NLRP3 inflammasome using MCC950 enhances host protection against B. cereus-induced infection (12). Besides, NLR with CARD domain-containing 4 (NLRC4) in epithelium is sufficient to protect against S. Typhimurium invasion (13). Assembly of the NLRP3 and NLRC4 inflammasomes leads to caspase 1-dependent/independent release of the pro-inflammatory cytokines IL-1β and IL-18, as well as to gasdermin D-mediated pyroptosis (14, 15). NLRP3 recruited by NLRC4 had been considered distinct inflammasome scaffolds in response to S. Typhimurium infection (16). Moreover, NLRP3 could be activated by flagellin under conditions of suboptimal NAIP/NLRC4 activation in S. Typhimurium infected macrophages (17). Our recent research revealed that spvC inhibits NLRP3 and NLRC4-associated pyroptosis against S. Typhimurium (18). Reports showed autophagy machinery constitutes a key cellular monitoring system that prevents excessive NLRP3 inflammasome activation (19, 20). However, more thorough investigation is required to shed light on the fundamental mechanisms underlying autophagy regulated NLRP3 and NLRC4 mediated by spvC.
Herein, both macrophages J774A.1 and epithelial HeLa cells were co-cultured with S. Typhimurium wild type, spvC deletion, site-directed mutant which lacks phosphothreonine lyase activity or complemented strain. We report a novel contribution of spvC to S. Typhimurium pathogenesis through the inhibition of host autophagy via its phosphothreonine lyase activity which affects the protein level of NLRP3 and NLRC4.
Materials and Methods
Bacterial Strains and Culture Conditions
S. Typhimurium wild type strain (STM-WT) was kindly supplied by Professor Qian Yang (Nanjing Agricultural University, Nanjing, China). STM-WT, spvC deletion mutant (STM-ΔspvC), spvC site-directed mutant (STM-ΔspvC/pspvC K136A) which lacks phosphothreonine lyase activity and spvC complemented strain (STM-ΔspvC/pspvC) were grown to log phase at 37°C in Luria Bertani (LB, Hangwei, China) broth overnight. STM-ΔspvC/pspvC K136A and STM-ΔspvC/pspvC were cultured in the media with 100 μg/ml ampicillin (Sigma, USA).
Construction of Mutant Strains
STM-ΔspvC was constructed with λRed recombination system basically as previously described (21) and the corresponding plasmids were gifts from Professor Daoguo Zhou (Purdue University, West Lafayette, USA). STM-ΔspvC/pspvC K136A mutation was constructed by overlap PCR. STM-ΔspvC/pspvC K136A and STM-ΔspvC/pspvC was complemented with STM-ΔspvC using pBAD/gIII expression system (22). spvC deletion mutant, site-directed mutant and complemented strain were identified by PCR and sequencing.
Cell Culture
J774A.1 cells were purchased from the Procell Life Science & Technology Co.,Ltd. HeLa cells were acquired from National Collection of Authenticated Cell Cultures. Cells were routinely cultured in complete medium that Dulbecco’s modified Eagle medium (HyClone Laboratories, Logan, UT, USA) supplemented with 10% (v/v) fetal bovine serum (Biological Industries, Kibbutz Beit-Haemek, Israel) and in a humidified incubator containing 5% CO2 at 37°C.
Bacterial Infection
J774A.1 cells (1×106/well) and HeLa cells (5×105/well) were seeded in 12-well plates. On the day of infection, S. Typhimurium were diluted 1:100 with LB broth to subculture for 3 h. Both STM-ΔspvC/pspvC K136A and STM-ΔspvC/pspvC were supplemented with 0.2% L-arabinose (Sigma, USA). Bacteria were then washed three times in PBS. The optical density of bacteria was determined by spectrophotometry at 600 nm with viable plate counts before infection. The bacterial suspension was subsequently added to cultured cells at the multiplicity of infection (MOI) described in the figure legends. Fresh medium containing amikacin (100 μg/ml, Sigma, Burlington, MA, USA) was added to kill the extracellular bacteria at 1 hour post infection (hpi). Afterwards, infected cells were washed and subsequently cultured in fresh medium containing amikacin (10 μg/ml) to limit extracellular replication of bacteria. PD0325901 (50 nM, Selleck, USA) functioned as an ERK inhibitor was added to the complete medium mentioned in Cell Culture section 24 h before infection. Cells were pretreated with Bafilomycin A1 (100 nM, Sigma, USA) 2 h before infection to inhibit autophagosome-lysosome fusion. At different time points following infection, cells were processed in the following ways.
Western Blot Analysis
Proteins were extracted using RIPA buffer containing protease inhibitors and phosphatase inhibitors (Beyotime, China). Samples were homogenized on ice, centrifuged for supernatant at 12,000 g for 15 min at 4°C and heated to 100°C for 5 min. Protein extracts resuspended in sample loading buffer were separated by electrophoresis through 12% polyacrylamide gels and transferred to PVDF membranes (Millipore, USA). After blocking with 5% non-fat milk (Sangon Biotech Shanghai Co.,Ltd., China), membranes were incubated with primary antibodies anti-LC3 (4108S, CST, USA; 1: 1,000 dilution), anti-Beclin 1 (3738, CST, USA; 1: 1,000 dilution), anti-NLRP3 (15101S, CST, USA; 1: 1,000 dilution), anti-NLRC4 (ab201792, abcam, UK; 1: 1,000 dilution), anti-GAPDH (BA2913, Boster, China; 1: 1,000 dilution), anti-Tubulin (AF1216, Beyotime, China; 1: 1,000 dilution) and anti-Histone H3 (ab194681, abcam, UK; 1: 1,000 dilution) overnight at 4°C. Membranes were then washed and incubated with the horseradish peroxidase (HRP)-labeled goat anti-rabbit IgG (A0208, Beyotime, China; 1: 3,000 dilution) for 1 h at room temperature. Proteins were visualized using ECL luminescence reagent (Meilunbio, China). The gray-scale values of the bands were determined by Image J launcher broken symmetry software program (National Institutes of Health, Bethesda, MD, USA).
Confocal Laser Scanning Microscopy
For immunofluorescence microscopy, HeLa cells transfected with mRFP-GFP-LC3 plasmid were used to establish the infection model. HeLa cells were collected at 2 hpi, bacteria and cell nucleus were labeled by Hoechst (33258, Beyotime, China; 1: 10,000 dilution). The samples were imaged under a confocal laser scanning microscopy (Fluoview FV1000, Olympus, Japan). GFP-LC3 and mRFP-LC3 punctate dots were counted in more than 100 cells and measured by Image J software program for quantification.
Statistical Analysis
Statistical significance was determined by ANOVA for three or more groups. P < 0.05 was considered to be statistically significant.
Results
spvC Suppresses Autophagy and Increases Intracellular Survival of Salmonella Typhimurium in Macrophages at the Early Stage of Infection
Our previous study revealed that Salmonella plasmid virulence gene spvB impair autophagic flux in infected macrophages for pathogen clearance (23). To determine the role of spvC on host cell autophagy, J774A.1 cells were collected to detect the expression of Microtubule associated protein light chain 3 (LC3) by western blot after being co-cultured with STM-WT, STM-ΔspvC or STM-ΔspvC/pspvC at 2 hpi, 8 hpi, 16 hpi and 24 hpi (Figure 1A). We found an increasing evidence of LC3-II in the early stage of infection (2 hpi and 8 hpi) compared with that in the late stage of infection (16 hpi and 24 hpi). At 2 hpi, higher level of LC3-II was found in STM-ΔspvC infected J774A.1 cells than those in STM-WT or STM-ΔspvC/pspvC infected cells. We next focused on the early stage of infection, and data confirmed that much more LC3-II and Beclin 1 were assessed in macrophages infected with STM-ΔspvC than in those infected with S. Typhimurium carrying spvC (Figure 1B). These data suggest that spvC suppresses autophagy in macrophages at the early stage of infection.
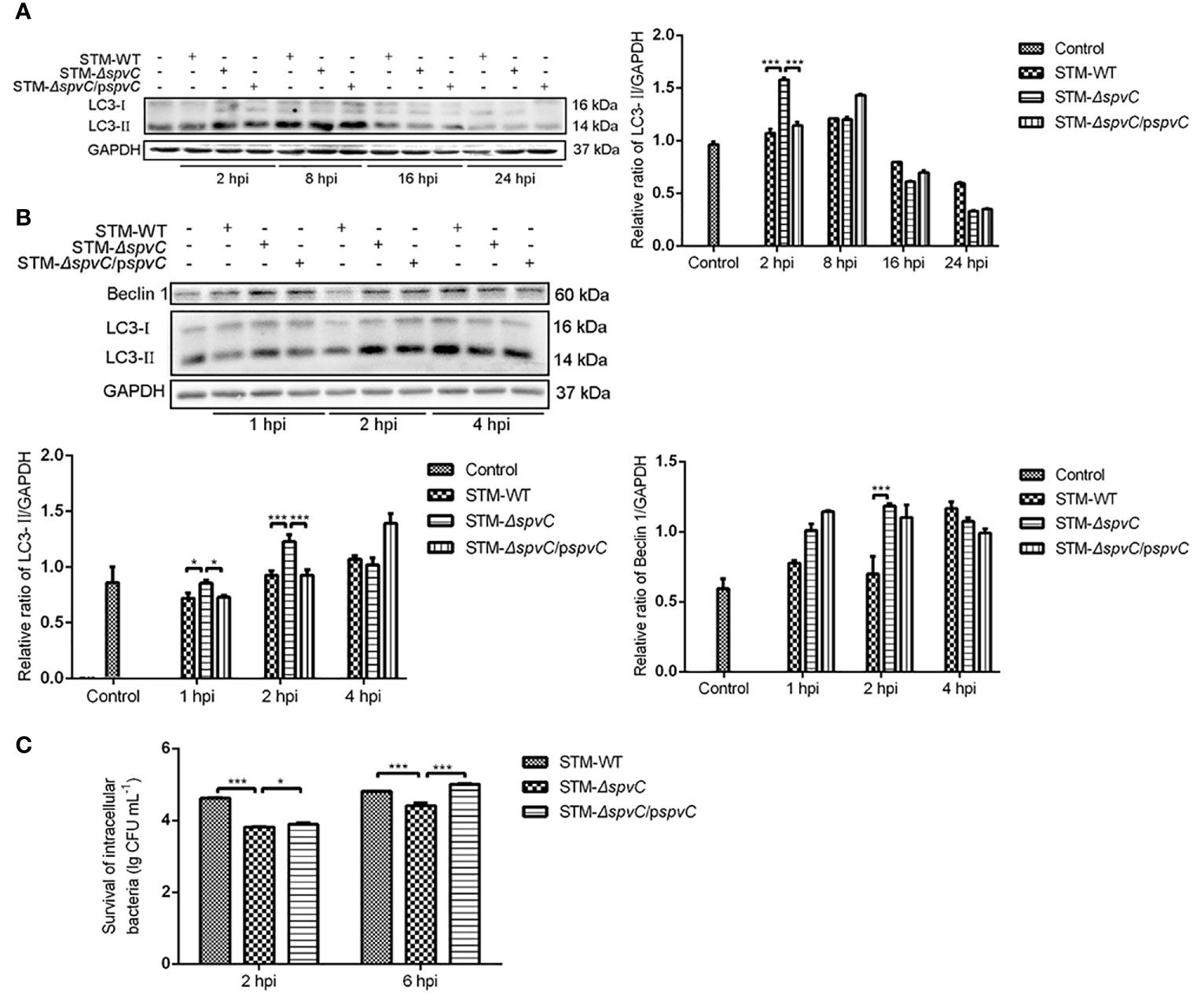
Figure 1 SpvC suppresses autophagy and increases intracellular survival of Salmonella Typhimurium in macrophages at the early stage of infection. (A, B) J774A.1 cells were infected with STM-WT, STM-ΔspvC or STM-ΔspvC/pspvC at an MOI of 10. Cell lysates were analysed by western blot with specific antibodies to LC3 and the control GAPDH at 2 hpi, 8 hpi, 16 hpi and 24 hpi (A), to Beclin 1, LC3 and the control GAPDH at 1 hpi, 2 hpi and 4 hpi (B). (C) Intracellular bacterial loads of J774A. 1 cells were assayed by colony forming units counting. Data were compared by ANOVA. Values are expressed as the means ± S.D., n = 3. Statistically significant differences are indicated. ***P < 0.001; *P < 0.05.
Autophagy is a cellular mechanism involving the degradation of cellular components or intracellular microbes through lysosomal machinery. We next examined the effect of the Salmonella spvC gene on intracellular bacterial loads. In agreement with our previous studies in vivo (18), macrophages J774A.1 infected with STM-ΔspvC showed significantly lower bacterial burden than those infected with STM-WT or STM-ΔspvC/pspvC since 2 hpi in vitro (Figure 1C). The aforementioned results suggest spvC restricts elimination of pathogens in host cells which may be related to its contribution to autophagy, while the underlying mechanism remains elusive.
spvC Inhibits the Formation of Autophagosomes in Host Cells During Salmonella Typhimurium Infection
LC3-II, as the marker of autophagosomes, associates with both the outer and inner membranes of the autophagosomes. The increased protein level of LC3-II in Figures 1A, B indicates that spvC disturbs the number of autophagosomes in S. Typhimurium infected macrophages. Since autophagy is a dynamic process, the raised number of autophagosomes in STM-ΔspvC infected cells may represent either the increased formation of autophagosomes and/or the inhibition in autophagosomal maturation. Of interest, treatment of S. Typhimurium-infected macrophages with Bafilomycin A1, which blocks autophagosome-lysosome fusion, led to the accumulation of autophagosomes in all groups, but the magnitude of the increase was significantly lower in the STM-ΔspvC infected group than STM-WT or STM-ΔspvC/pspvC infected groups (Figure 2A). These data suggest that spvC negatively regulates autophagic activity and intervenes in the formation of autophagosomes in host cells. Furthermore, autophagic flux was morphologically monitored by mRFP-GFP-LC3. Autophagosomes and autolysosomes are labeled with yellow (RFP and GFP merged) and red (RFP only) puncta, respectively, since RFP exhibits more stable fluorescence in acidic compartments while GFP signal quenches for the low pH inside the lysosome (24). HeLa cells transfected with mRFP-GFP-LC3 were infected with different S. Typhimurium strains. More yellow LC3 puncta were visualized in STM-ΔspvC infected cells than those in STM-ΔspvC or STM-ΔspvC/pspvC infected cells at 2 hpi (Figures 2B, C). These results demonstrate that spvC inhibits autophagy by suppressing the formation of autophagosomes.
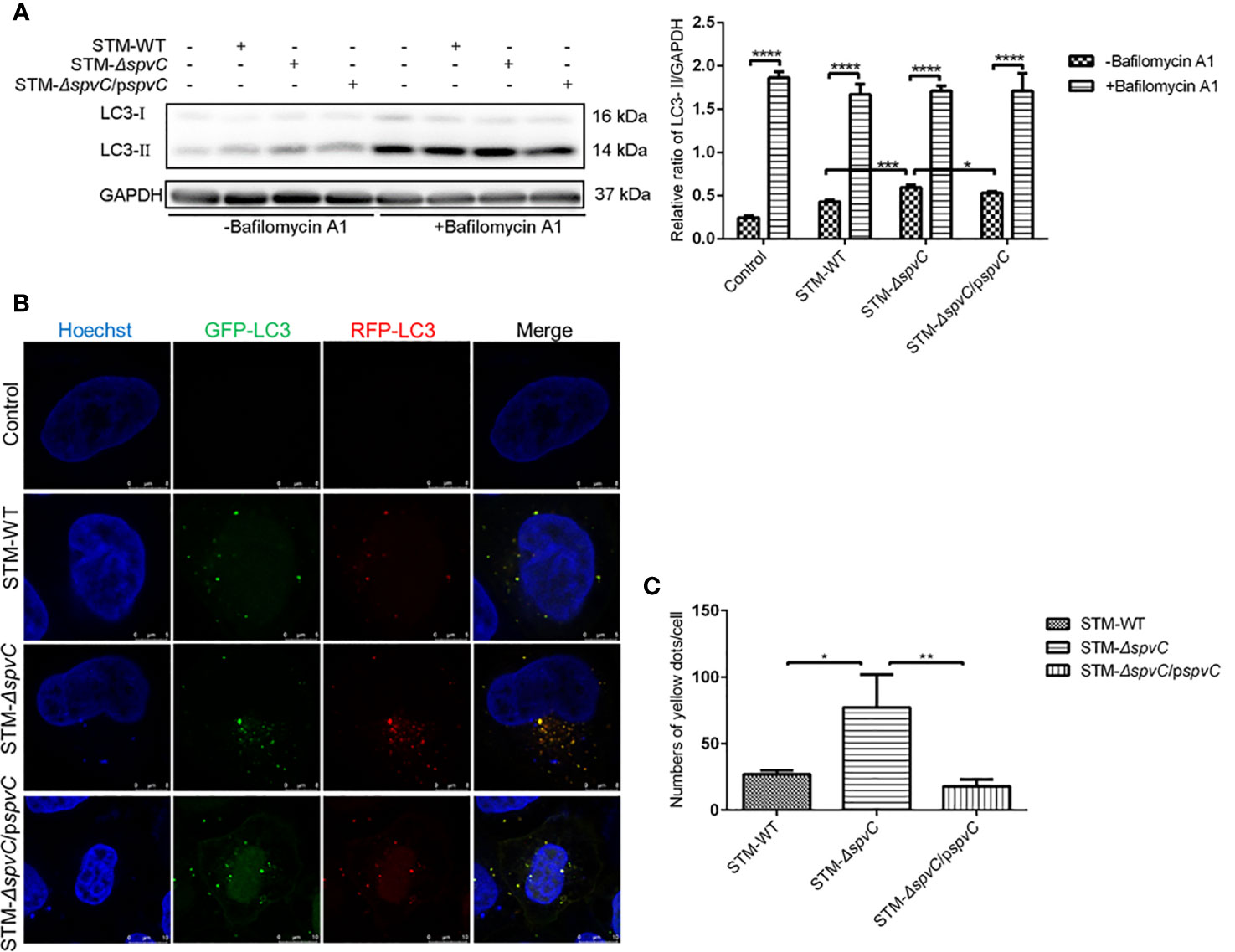
Figure 2 SpvC inhibits the formation of autophagosomes in host cells during Salmonella Typhimurium infection. (A) J774A.1 cells were infected with STM-WT, STM-ΔspvC or STM-ΔspvC/pspvC at an MOI of 10 after being pretreated with Bafilomycin A1. Cell lysates were analysed by western blot with specific antibodies to LC3 and the control GAPDH at 2 hpi. n = 3. (B, C) HeLa cells stability expressing mRFP-GFP-LC3 were used to establish an infection model. (B) LC3 puncta were visualized at 2 hpi by CLSM. Cell nucleus were labeled by Hoechst. (C) Punctate LC3 dots were measured by Image J. Number of punctate dots was enumerated in at least 100 cells. Scale bar, 5 µm. Data were compared by ANOVA. Values are expressed as the means ± S.D. and statistically significant differences are indicated. *P < 0.05; **P < 0.01; ***P < 0.001; ****P < 0.0001.
spvC Phosphothreonine Lyase Activity Is Critical for Inhibiting Autophagy in Salmonella Typhimurium Infection
Previous literature reported that SpvC is a Salmonella effector with phosphothreonine lyase activity on host MAPK (6). To investigate whether the enzymatic activity of SpvC is involved in its effect on autophagy, J774A.1 cells were co-cultured with STM-WT, STM-ΔspvC, site-directed mutant STM-ΔspvC/pspvC K136A which lacks phosphothreonine lyase activity and STM-ΔspvC/pspvC, respectively. As expected, both STM-ΔspvC and STM-ΔspvC/pspvC K136A gave rise to elevated levels of LC3-II in their infected macrophages compared with S. Typhimurium carrying spvC (Figure 3A). Concomitantly, levels of Beclin 1 were in line with the changing trend of LC3-II (Figure 3B). These results reveal that SpvC suppresses autophagy in macrophages through its phosphothreonine lyase activity.
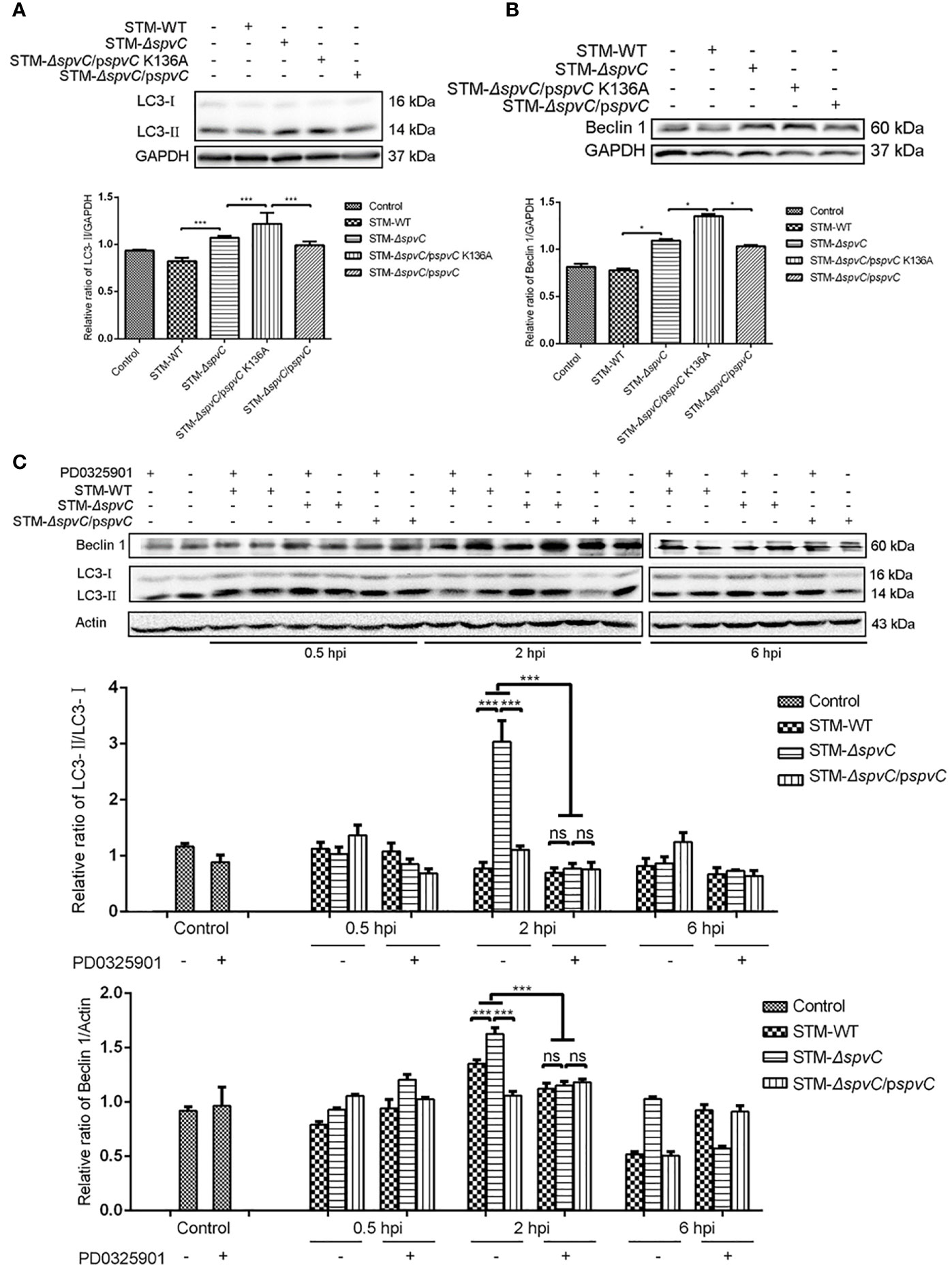
Figure 3 SpvC phosphothreonine lyase activity is critical for inhibiting autophagy in Salmonella Typhimurium infection. (A, B) J774A.1 cells were infected with STM-WT, STM-ΔspvC, STM-ΔspvC/pspvC or STM-ΔspvC/pspvC K136A at an MOI of 10 for 2 h. Cell lysates were analysed by western blot with specific antibodies to LC3 (A), Beclin 1 (B) and the control GAPDH. (C) HeLa cells were infected with STM-WT, STM-ΔspvC or STM-ΔspvC/pspvC at an MOI of 100 after being pretreated with PD0325901. Cell lysates were analysed by western blot with specific antibodies to LC3, Beclin 1 and the control Actin at 0.5 hpi, 2 hpi and 6 hpi. Data were compared by ANOVA. Values are expressed as the means ± S.D., n = 3. Statistically significant differences are indicated. ***P < 0.001; *P < 0.01; ns, not significant.
ERK is an essential component in MAPK signaling pathway. To further elucidate the relationship between the effect of SpvC on autophagy and its phosphothreonine lyase activity on MAPK, HeLa cells were pretreated with ERK inhibitor PD0325901 before co-cultured with STM-WT, STM-ΔspvC or STM-ΔspvC/pspvC. In line with the results obtained in Figure 1B, significantly more LC3-II and Beclin 1 were determined in HeLa cells infected with STM-ΔspvC than those in cells infected with S. Typhimurium carrying spvC at 2 hpi. The conversion from LC3-I to LC3-II also correlates well with the number of autophagosomes (24). PD0325901 only decreased the expression of LC3-II and Beclin 1 in STM-ΔspvC infected cells rather than those infected with STM-WT or STM-ΔspvC/pspvC. Additionally, there was no significant difference of the expression of LC3-II and Beclin 1 among STM-WT, STM-ΔspvC or STM-ΔspvC/pspvC infected cells after PD0325901 treatment, which indicates that ablation of ERK signaling pathway virtually eliminates the inhibition of spvC on autophagy (Figure 3C). Collectively, these data indicate that phosphothreonine lyase activity of SpvC is required to inhibit the formation of autophagosomes.
spvC Down-Regulates NLRP3 and NLRC4 in an Autophagy Related Manner
Previous studies have reported that SpvC exerts as an anti-inflammatory effector in systemic infection of Salmonella (5). Our earlier research has showed that spvC inhibits pyroptosis of host cells and it could also modulate NLRP3 and NLRC4-associated inflammatory response against S. Typhimurium. Of note, various literatures suggest that autophagy, a cellular waste removal and rejuvenation process, serves a crucial role as a macrophage-intrinsic negative regulator of NLRP3 inflammasome (25). In order to explore whether the autophagic response contributed to the effect of spvC on NLRP3 and NLRC4, we first co-cultured macrophages J774A.1 with STM-WT, STM-ΔspvC, or STM-ΔspvC/pspvC to investigate the dynamic function of spvC on NLRP3 and NLRC4. Western blot analysis showed the increasing evidence of NLRP3 and NLRC4 at 8 hpi due to the absence of spvC (Figures 4A, B), which suggests that spvC down-regulates NLRP3 and NLRC4.
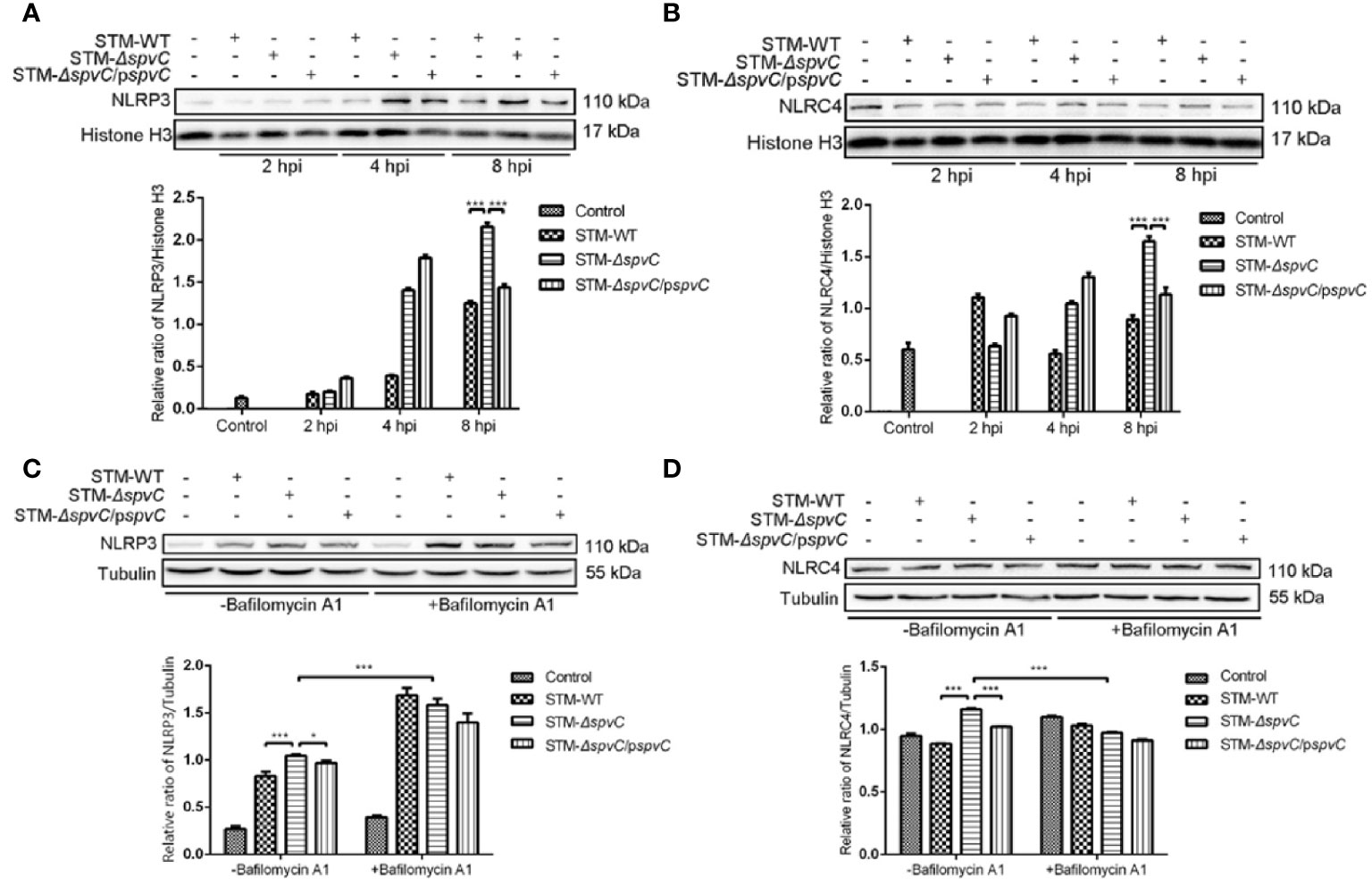
Figure 4 spvC down-regulates NLRP3 and NLRC4 in an autophagy related manner. (A, B) J774A.1 cells were infected with STM-WT, STM-ΔspvC and STM-ΔspvC/pspvC at an MOI of 10. Cell lysates were analysed by western blot with specific antibodies to NLRP3 (A), NLRC4 (B) and the control Histone H3 at 2 hpi, 4 hpi and 8 hpi. (C, D) J774A.1 cells were infected with STM-WT, STM-ΔspvC and STM-ΔspvC/pspvC at an MOI of 10 at 8 hpi after being pretreated with Bafilomycin A1. Cell lysates were analysed by western blot with specific antibodies to NLRP3 (C), NLRC4 (D) and the control Tubulin. Data were compared by ANOVA. Values are expressed as the means ± S.D., n = 3. Statistically significant differences are indicated. ***P < 0.001; *P < 0.01.
Next, we generated the infection model pretreated with Bafilomycin A1. As previously mentioned, western blot analysis exhibited that in the absence of Bafilomycin A1, the levels of NLRP3 and NLRC4 in cells infected with STM-ΔspvC were significantly higher than that in cells infected with Salmonella carrying spvC. After Bafilomycin A1 treatment to inhibit autophagosome-lysosome fusion, the levels of NLRP3 and NLRC4 significantly changed though differences among three groups were still observed (Figures 4C, D). The results indicate that the effect of spvC on NLRP3 and NLRC4 is closely related to autophagy, but other factors are also involved in this process.
Discussion
S. Typhimurium is not only a leading cause of human morbidity and mortality worldwide, but also a model pathogen for investigating the mechanisms of host-bacterium interactions (26). It is well known that macrophages, the professional phagocytes in host innate immune system, play a pivotal role in the clearance of S. Typhimurium. Autophagy is an important component of the innate immune system in host anti-bacterial defense, which is known to target a population of Salmonella for degradation and restrict Salmonella replication (27, 28). Beclin 1 interacts with several cofactors (e.g., Atg14L, HMGB1, IP3R and PINK) to promote the formation of Beclin 1-Vps34-Vps15 core complexes, thereby inducing autophagy (9). LC3, a mammalian homolog of yeast Atg8, is known to serve as a widely used marker for autophagosomes. To assess a possible correlation between autophagy and spvC, we extended these studies by monitoring the time course of autophagy in macrophages during 24 h. Data showed that spvC restrains autophagy at the early stage of infection (2 hpi). Concomitantly, much more bacteria were counted in macrophages infected with S. Typhimurium carrying spvC at 2 hpi and 6 hpi, suggesting that spvC gene restricts elimination of pathogens in host cells which may related to autophagy.
However, some pathogens have evolved complex escape mechanisms of autophagy. We have previously shown that spvB blocks initial stage of autophagy and enhanced intracellular bacterial survival (29). In this study, we pretreated the infection model with Bafilomycin A1 which inhibits autophagosome-lysosome fusion, and results showed that spvC blocks the formation of autophagosomes. It is now appreciated that the devoured Salmonella can survive after internalization into professional phagocytes (e.g. macrophages and neutrophils) and nonprofessional cells (e.g. epithelial cells) (30). Consistent with this, morphologically tracked autophagosomes (yellow puncta) and autolysosomes (red puncta) with mRFP-GFP-LC3 tandem construct indicates that S. Typhimurium harboring spvC inhibits the formation of autophagosomes.
As mentioned above, SpvC is a phosphothreonine lyase which exerts anti-inflammatory effects by inactivating dual-phosphorylated MAPK through beta elimination (31). A site-directed mutant STM-ΔspvC/pΔspvC K136A which lacks phosphothreonine lyase activity was constructed. We found that the enzymatic activity of SpvC contributes to down-regulation of autophagy in macrophages. To date, study showed that S. Typhimurium spvC alleviated phospho-ERK1/2 expression in the villus epithelial cells and lamina propria of caeca, but no significant difference in phospho-p38 or phospho-JNK levels in the caeca infected with all strains (5). Based on this, HeLa cells were pretreated with ERK inhibitor PD0325901 before co-cultured with S. Typhimurium. We demonstrated that spvC affects the formation of autophagosomes in an ERK dependent manner. Besides we have proved that spvC inactivates phospho-ERK1/2, phospho-JNK and phospho-p38, leading to the interference of NLRP3 and NLRC4 in S. Typhimurium infected macrophages J774A.1 (18). Given all this, whether JNK and p38 are involved in spvC suppressed autophagy remains to be fully elucidated.
Several studies employing diverse bacterial species have highlighted the tactical interplay between autophagy and NLRP3 or NLRC4 inflammasomes. Therefore, macrophages J774A.1, applied in inflammation related research, were used to firstly valid that spvC down-regulates NLRP3 and NLRC4 at 8 hpi. Activation of NLRP3 inflammasome involves in damage to the mitochondria and the increased production of reactive oxygen species (ROS) (32). Autophagy plays a role in the removal of misfolded proteins, and the clearance of damaged mitochondria and ROS (33). Notably, we demonstrated that alleviated autophagosome formation is closely related to the effect of spvC on NLRP3. On the other hand, type ι interferon-dependent host response performs a negative feedback that represses expression of NLRC4 during Salmonella infection (34). Besides, we have reported that Salmonella spv locus could affect type ι interferon response via inhibiting autophagy in macrophages (35). Thus, we speculate that spvC-inhibited autophagy may be related to NLRC4. Indeed, experimental evidence reveals that the inhibition of autophagosome formation by spvC interferes with the level of NLRC4. Furthermore, MAPK can transmit signals from the cell membrane to the nucleus, which may provide the first signal for transcription of inflammasomes (36). This pathway is independent on the effect of autophagy on NLRP3 and NLRC4. Hence, repression of NLRP3 and NLRC4 by spvC contributes to the alleviation of pyroptosis, subsequently promotes bacterial dissemination in mice (18). Therefore, the role of NLRP3 and NLRC4 regulated by spvC to drive cell fate decisions between autophagy and pytoptosis in Salmonella infection deserves further investigation.
Taken together, we identify a novel contribution of the spvC gene to the pathogenesis of Salmonella via impairing the formation of autophagosome, thereby interfering with protein levels of NLRP3 and NLRC4. These findings have important implications for understanding the intricate evolutionary adaptations that shape host-pathogen cross-talk.
Data Availability Statement
The original contributions presented in the study are included in the article/supplementary material. Further inquiries can be directed to the corresponding author.
Ethics Statement
This study was approved by Soochow University Institutional Review Board.
Author Contributions
LTZ, YL, and SW designed the research and wrote the manuscript. LTZ, YL, SG, HY, LLZ, and CW performed the research and conducted the data analysis. YL, RH, and SW supervised the project and edited the manuscript. All authors contributed to the article and approved the submitted version.
Funding
The research leading to these results has received funding from the Natural Science Foundation of China (No. 31970132, No. 81971899), the Suzhou Municipal Science and Technology Foundation (SYS2019031), and the Priority Academic Program Development of Jiangsu Higher Education Institutions (PAPD).
Conflict of Interest
The authors declare that the research was conducted in the absence of any commercial or financial relationships that could be construed as a potential conflict of interest.
References
1. Hurley D, Hoffmann M, Muruvanda T, Allard MW, Brown EW, Martins M, et al. Atypical Salmonella Enterica Serovars in Murine and Human Macrophage Infection Models. Infect Immun (2020) 88(4):e00353-19. doi: 10.1128/IAI.00353-19
2. Kharroubi S, Nasser NA, El-Harakeh MD, Sulaiman AA, Kassem II. First Nation-Wide Analysis of Food Safety and Acceptability Data in Lebanon. Foods (2020) 9(11):1717. doi: 10.3390/foods9111717
3. Käppeli R, Kaiser P, Stecher B, Hardt W-D. Roles of spvB and spvC in S. Typhimurium Colitis Via the Alternative Pathway. Int J Med Microbiol (2011) 301(2):117–24. doi: 10.1016/j.ijmm.2010.08.017
4. Yang SD, Deng QF, Sun LQ, Dong KD, Li YY, Wu SY, et al. Salmonella Effector SpvB Interferes With Intracellular Iron Homeostasis via Regulation of Transcription Factor Nrf2. FASEB J (2019) 33(12):13450–64. doi: 10.1096/fj.201900883RR
5. Haneda T, Ishii Y, Shimizu H, Ohshima K, Iida N, Danbara H, et al. Salmonella Type III Effector SpvC, a Phosphothreonine Lyase, Contributes to Reduction in Inflammatory Response During Intestinal Phase of Infection. Cell Microbiol (2012) 14(4):485–99. doi: 10.1111/j.1462-5822.2011.01733.x
6. Mazurkiewicz P, Thomas J, Thompson JA, Liu M, Arbibe L, Sansonetti P, et al. SpvC Is a Salmonella Effector With Phosphothreonine Lyase Activity on Host Mitogen-Activated Protein Kinases. Mol Microbiol (2008) 67(6):1371–83. doi: 10.1111/j.1365-2958.2008.06134.x
7. Ammanathan V, Mishra P, Chavalmane AK, Muthusamy S, Jadhav V, Siddamadappa C, et al. Restriction of Intracellular Salmonella Replication by Restoring TFEB-Mediated Xenophagy. Autophagy (2019) 16(9):1584–97. doi: 10.1080/15548627.2019.1689770
8. Kang JW, Yan J, Ranjan K, Zhang X, Turner JR, Abraham C. Myeloid Cell Expression of LACC1 Is Required for Bacterial Clearance and Control of Intestinal Inflammation. Gastroenterology (2020) 159(3):1051–67. doi: 10.1053/j.gastro.2020.07.024
9. Jiao Y, Zhang YG, Lin ZJ, Lu R, Xia YL, Meng C, et al. Salmonella Enteritidis Effector AvrA Suppresses Autophagy by Reducing Beclin-1 Protein. Front Immunol (2020) 11:686. doi: 10.3389/fimmu.2020.00686
10. Samir P, Kesavardhana S, Patmore DM, Gingras S, Malireddi RKS, Karki R, et al. DDX3X Acts as a Live-Or-Die Checkpoint in Stressed Cells by Regulating NLRP3 Inflammasome. Nature (2019) 573(7775):590–4. doi: 10.1038/s41586-019-1551-2
11. Xue Y, Enosi Tuipulotu D, Tan WH, Kay C, Man SM. Emerging Activators and Regulators Of Inflammasomes and Pyroptosis. Trends Immunol (2019) 40(11):1035–52. doi: 10.1016/j.it.2019.09.005
12. Jiang H, Gong T, Zhou RB. The Strategies of Targeting the NLRP3 Inflammasome to Treat Inflammatory Diseases. Adv Immunol (2020) 145:55–93. doi: 10.1016/bs.ai.2019.11.003
13. Fattinger SA, Geiser P, Samperio Ventayol P, Di Martino ML, Furter M, Felmy B, et al. Epithelium-Autonomous Naip/Nlrc4 Prevents TNF-Driven Inflammatory Destruction of the Gut Epithelial Barrier in Salmonella-Infected Mice. Mucosal Immunol (2021) 14(3):615–29. doi: 10.1038/s41385-021-00381-y
14. Kay C, Wang R, Kirkby M, Man SM. Molecular Mechanisms Activating the NAIP-NLRC4 Inflammasome: Implications in Infectious Disease, Autoinflammation, and Cancer. Immunol Rev (2020) 297(1):67–82. doi: 10.1111/imr.12906
15. Swanson KV, Deng M, Ting JP. The NLRP3 Inflammasome: Molecular Activation and Regulation to Therapeutics. Nat Rev Immunol (2019) 19(8):477–89. doi: 10.1038/s41577-019-0165-0
16. Qu Y, Misaghi S, Newton K, Maltzman A, Izrael-Tomasevic A, Arnott D, et al. NLRP3 Recruitment by Nlrc4 During Salmonella Infection. J Exp Med (2016) 213(6):877–85. doi: 10.1084/jem.20132234
17. Gram AM, Wright JA, Pickering RJ, Lam NL, Booty LM, Webster SJ, et al. Salmonella Flagellin Activates NAIP/NLRC4 and Canonical NLRP3 Inflammasomes in Human Macrophages. J Immunol (2021) 206(3):631–40. doi: 10.4049/jimmunol.2000382
18. Zuo LL, Zhou LT, Wu CY, Wang YL, Li YY, Huang R, et al. Salmonella spvC Gene Iinhibits Pyroptosis and Intestinal Inflammation to Aggravate Systemic Infection in Mice. Front Microbiol (2020) 11:562491. doi: 10.3389/fmicb.2020.562491
19. Zhou RB, Yazdi AS, Menu P, Tschopp J. A Role for Mitochondria in NLRP3 Inflammasome Activation. Nature (2011) 469(7329):221–5. doi: 10.1038/nature09663
20. Crother TR, Porritt RA, Dagvadorj J, Tumurkhuu G, Slepenkin AV, Peterson EM, et al. Autophagy Limits Inflammasome During Chlamydia Pneumoniae Infection. Front Immunol (2019) 10:754. doi: 10.3389/fimmu.2019.00754
21. Song J, Dong HQ, Ma C, Zhao BY, Shang GD. Construction and Functional Characterization of an Integrative Form Lambda Red Recombineering Escherichia Coli Strain. FEMS Microbiol Lett (2010) 309(2):178–83. doi: 10.1111/j.1574-6968.2010.02036.x
22. Szeliova D, Krahulec J, Safranek M, Liskova V, Turna J. Modulation of Heterologous Expression From Pbad Promoter in Escherichia Coli Production Strains. J Biotechnol (2016) 236:1–9. doi: 10.1016/j.jbiotec.2016.08.004
23. Chu YY, Yang YR, Li Y, Ye Y, Yan J, Wang T, et al. A Salmonella Enterica Conjugative Plasmid Impairs Autophagic Flux in Infected Macrophages. Microbes Infect (2014) 16(7):553–61. doi: 10.1016/j.micinf.2014.05.001
24. Mizushima N, Yoshimori T, Levine B. Methods in Mammalian Autophagy Research. Cell (2010) 140(3):313–26. doi: 10.1016/j.cell.2010.01.028
25. Zhong ZY, Elsa S, Michael K. Autophagy, NLRP3 Inflammasome and Auto-Inflammatory/Immune Diseases. Clin Exp Rheumatol (2016) 34(4 Suppl 19):12–6.
26. Keestra-Gounder AM, Tsolis RM, Baumler AJ. Now You See Me, Now You Don’t: The Interaction of Salmonella With Innate Immune Receptors. Nat Rev Microbiol (2015) 13(4):206–16. doi: 10.1038/nrmicro3428
27. Wu S, Shen YR, Zhang S, Xiao YQ, Shi SR. Salmonella Interacts With Autophagy to Offense or Defense. Front Microbiol (2020) 11:721. doi: 10.3389/fmicb.2020.00721
28. Xu Y, Zhou P, Cheng S, Lu QH, Nowak K, Hopp AK, et al. A Bacterial Effector Reveals the V-Atpase-Atg16l1 Axis That Initiates Xenophagy. Cell (2019) 178(3):552–66.e20. doi: 10.1016/j.cell.2019.06.007
29. Chu YY, Gao S, Wang T, Yan J, Xu GM, Li YY, et al. A Novel Contribution of spvB to Pathogenesis of Salmonella Typhimurium by Inhibiting Autophagy in Host Cells. Oncotarget (2016) 7(7):8295–309. doi: 10.18632/oncotarget.6989
30. Boada-Romero E, Martinez J, Heckmann BL, Green DR. The Clearance of Dead Cells by Efferocytosis. Nat Rev Mol Cell Biol (2020) 21(7):398–414. doi: 10.1038/s41580-020-0232-1
31. Li HT, Xu H, Zhou Y, Zhang J, Long CZ, Li SQ, et al. The Phosphothreonine Lyase Activity of a Bacterial Type III Effector Family. Science (2007) 315(5814):1000–3. doi: 10.1126/science.1138960
32. Zhong ZY, Liang S, Sanchez-Lopez E, He F, Shalapour S, Lin XJ, et al. New Mitochondrial DNA Synthesis Enables NLRP3 Inflammasome Activation. Nature (2018) 560(7717):198–203. doi: 10.1038/s41586-018-0372-z
33. Wu AG, Zhou XG, Qiao G, Yu L, Tang Y, Yan L, et al. Targeting Microglial Autophagic Degradation in Nlrp3 Inflammasome-Mediated Neurodegenerative Diseases. Ageing Res Rev (2020) 65:101202. doi: 10.1016/j.arr.2020.101202
34. Akhade AS, Atif SM, Lakshmi BS, Dikshit N, Hughes KT, Qadri A, et al. Type 1 Interferon-Dependent Repression of NLRC4 and Ipla2 Licenses Down-Regulation Of Salmonella Flagellin Inside Macrophages. Proc Natl Acad Sci USA (2020) 117(47):29811–22. doi: 10.1073/pnas.2002747117
35. Wang LD, Li YL, Liu YH, Zuo LL, Li YY, Wu SY, et al. Salmonella Spv Locus Affects Type I Interferon Response and the Chemotaxis of Neutrophils via Suppressing Autophagy. Fish Shellfish Immunol (2019) 87:721–9. doi: 10.1016/j.fsi.2019.02.009
Keywords: Salmonella, spvC, autophagy, NLRP3, NLRC4
Citation: Zhou L, Li Y, Gao S, Yuan H, Zuo L, Wu C, Huang R and Wu S (2021) Salmonella spvC Gene Inhibits Autophagy of Host Cells and Suppresses NLRP3 as Well as NLRC4. Front. Immunol. 12:639019. doi: 10.3389/fimmu.2021.639019
Received: 08 December 2020; Accepted: 28 June 2021;
Published: 14 July 2021.
Edited by:
Isabelle Vergne, UMR5089 Institut de Pharmacologie et de Biologie Structurale (IPBS), FranceReviewed by:
Jere W. McBride, University of Texas Medical Branch at Galveston, United StatesShourong Shi, Poultry Institute, Chinese Academy of Agricultural Sciences, China
Michiel Van Der Vaart, Leiden University, Netherlands
Copyright © 2021 Zhou, Li, Gao, Yuan, Zuo, Wu, Huang and Wu. This is an open-access article distributed under the terms of the Creative Commons Attribution License (CC BY). The use, distribution or reproduction in other forums is permitted, provided the original author(s) and the copyright owner(s) are credited and that the original publication in this journal is cited, in accordance with accepted academic practice. No use, distribution or reproduction is permitted which does not comply with these terms.
*Correspondence: Shuyan Wu, d3VzaHV5YW5Ac3VkYS5lZHUuY24=
†Present address: Song Gao, Department of Central Laboratory, Liaocheng People’s Hospital, Liaocheng, China Lingli Zuo, Medical Research Center, The People’s Hospital of Suzhou New District, Suzhou, China
‡These authors have contributed equally to this work