- 1Guelph Research and Development Center, Agriculture and Agri-Food Canada, Guelph, ON, Canada
- 2Department of Pathobiology, University of Guelph, Guelph, ON, Canada
The impact of antibiotic use for growth promotion in livestock and poultry production on the rise of antimicrobial resistance (AMR) in bacteria led to the ban of this practice in the European Union in 2006 and a restriction of antimicrobial use (AMU) in animal agriculture in Canada and the United States of America. There is a high risk of infectious diseases such as necrotic enteritis due to Clostridium perfringens, and colibacillosis due to avian pathogenic Escherichia coli in antimicrobial-free broiler chickens. Thus, efficient and cost-effective methods for reducing AMU, maintaining good poultry health and reducing public health risks (food safety) are urgently needed for poultry production. Several alternative agents, including plant-derived polyphenolic compounds, have been investigated for their potential to prevent and control diseases through increasing poultry immunity. Many studies in humans reported that plant flavonoids could modulate the immune system by decreasing production of pro-inflammatory cytokines, T-cell activation, and proliferation. Fruits, especially berries, are excellent sources of flavonoids while being rich in nutrients and other functionally important molecules (vitamins and minerals). Thus, fruit byproducts or wastes could be important resources for value-added applications in poultry production. In the context of the circular economy and waste reduction, this review summarizes observed effects of fruit wastes/extracts on the general health and the immunity of poultry.
Introduction
Poultry products are quickly becoming one of the most-consumed sources of protein around the world (1), making them very important to the global food supply. Globally, poultry meat demand and production are expected to continue rising more rapidly than any other types of meat. Current estimates predict that by 2026, poultry meat will account for 45% of global meat consumption. The increase in poultry production is especially apparent in developing countries, due to poultry’s status as an inexpensive and healthy meat with few cultural or religious barriers (2). Poultry products include meat from broiler chickens and turkeys, eggs from layers, and live chicks often exported to other countries, all of which are important parts of the agricultural sector. Unfortunately, as with any animals kept at high density, poultry are prone to infectious diseases. Farmers have a vested interest in keeping their flocks disease-free and growing faster and larger to meet consumers’ demands. Thus, antibiotics have been used for disease prevention and/or growth promotion in meat-type poultry farms. Due to the selection for and spread of antimicrobial resistance (AMR) genes among several bacterial species of food safety and public health concern, antimicrobial use (AMU) in animal agriculture is becoming increasingly restricted (3). Additionally, poultry pathogens carrying AMR genes can compromise the birds’ health due to increased difficulty in treating diseases associated with them.
Bacterial diseases, such as necrotic enteritis (NE), will continue to negatively impact poultry health and producers’ profits if they become harder to control in the future. As mentioned above, with many countries (including the United States and Canada) withdrawing, restricting, or banning AMU for growth promotion in poultry, producers are searching for new ways to protect their flocks from bacterial diseases that have thus far been managed with low doses of preventative antibiotics. There have been many proposed alternatives to antibiotics where vaccines are not available, including antimicrobial peptides, organic acids, enzymes, metals, clay, bacteriophages, prebiotics, probiotics, and phytochemicals (4). Some of these approaches are able to improve the growth of the chickens. For example, enzymes added to feed improve the digestibility and availability of nutrients in the feed, subsequently improving feed efficiency and growth. Other alternatives, such as antimicrobial peptides, modulate or restrict the population of gut microbiota (including potential pathogens), and increase the overall flock health by reducing the frequency of diseases. The administration of probiotics (Lactobacillus species or others) in the chickens’ diets has shown some success in both increasing growth rates and preventing diseases. Because the productivity of the chickens and their resistance to diseases are both important and linked to multitude of factors, there is no simple solution for replacing antibiotics. It is likely that none of the alternative approaches mentioned above alone would be effective enough, thus a combination of approaches would be more effective.
Current poultry feeds are formulated to meet the nutritional requirements of chickens, but more research is needed to determine if these feeds adequately support the bird’s immune system, or if additional ingredients are needed to prevent immune deficiencies. Ideally, boosting their immune function should help the birds to be more resistant to infectious diseases. Vaccines have been used in poultry production to prevent diseases; however, most of them target viral pathogens such as Marek’s disease virus, Newcastle disease virus, infectious bronchitis virus, and infectious bursal disease virus (5). Longer-lived layer hens typically receive almost all above mentioned vaccines, in addition to those against encephalomyelitis, fowlpox, and laryngotracheitis depending on production conditions and requirements (5). This vaccination schedule is standard in most areas of North America, but it can be modified to suit the surrounding disease environment.
Phytochemicals, particularly those found in certain fruit extracts, are high in antioxidant compounds, and have been shown to improve the immune systems and general health in humans (6, 7). It stands to reason, then, that fruits and their extracts could help prevent diseases by stimulating the immune system in poultry such as broiler chickens. The use of fruit wastes, whole fruits, and their derivatives as supplements to improve immunity is an attractive solution for both poultry farmers and fruit producers. Many studies have been conducted on fruits and their various products (juices, pomaces, extracts, and seeds) with regards to boosting immunity in humans (6, 8–10). Fewer studies have been done on their effects in poultry, or other livestock. Certain fruit products, such as cranberry and blueberry pomaces, have been reported to have antimicrobial activities against a variety of bacterial species (11, 12). Grape seed extracts have demonstrated effects on blood metabolites, the immune system, and overall productivity of broilers (13). Considering that fruit byproducts present an economical and value-added opportunity, they are worthy of further investigation as possible cost-effective alternative agents in poultry production. The application of optimized fruit products, along with proper husbandry and good antimicrobial stewardship, may contribute to healthier and more profitable poultry flocks, as well as decrease the need for antibiotics and therefore the spread of AMR. This review will focus primarily on the application of fruits, particularly berries, and their extracts to enhance poultry immunity.
Immune System of Poultry
The avian immune system is complex and plays a critical role in protecting birds from infectious and metabolic diseases. Similar to the mammalian immune system, the avian immune system provides both innate and adaptive responses. The innate immune response is the first line of defense, responding non-selectively to a wide range of pathogens by phagocytizing and chemically attacking them. The adaptive immune system, consisting of both cellular and humoral immunity, is capable of launching a specific response to previously encountered pathogens. The adaptive immune response and its memory properties can be harnessed using vaccines to provide long-term immunity for birds against specific diseases. A detailed description of the avian immune system has been reported elsewhere (14) and will not be provided here.
A few factors pertinent to poultry farming must be noted. Mainly, broiler chickens live about six weeks, and have several immunological differences from laying hens, which live for 1-2 years. Since the bursa of Fabricius, the main site of B-cell development in birds, disappears by seven months of age, the bursa is always present in broilers but may be absent in older laying hens. Therefore, the bursa and bursa-body weight ratio has been used to assess relative immune health in broilers only (15). Another important practical difference is that broilers are vaccinated against a few prevalent and deadly diseases as stated above whereas layers are vaccinated against many more diseases (5). Heterophils, present throughout the lives of both types of birds, are important cells of the innate immune system, analogous to mammalian neutrophils and involved in the initial acute response to most pathogens (16). Unfortunately, some intracellular bacteria have evolved ways of avoiding phagocytosis and lysis by heterophils. In poultry, Staphylococcus aureus in particular has shown this ability which is troublesome as it can greatly impact the production of both broiler and layer flocks (17).
Cytokines are a diverse group of proteins or peptides secreted by cells to help direct both innate and adaptive immune responses. Cytokine levels are an indicator of the robustness of the immune system. Some cytokines in chickens can be used to evaluate stress levels, infections, or other immune functions. For example, chronically stressed chickens display elevated levels of interleukin-2 (IL-2) and granulocyte colony stimulating factor (G-CSF) in spleen tissue, and IL-1β, IL-2, IL-18, interferon gamma (IFN-γ), and IL-6 in thymic tissue (18). Chickens orally challenged with Salmonella enterica serovar Typhimurium showed high levels of IL-1β, IL-8, and other cytokines in intestinal and liver tissues, but not in the spleen (19). IL-10 plays an important role in the ceca, and has higher expression in birds susceptible to Eimeria tenella (20). A co-infection with both Eimeria spp. and C. perfringens has been shown to downregulate the production of IFN-α, IFN-γ, IL-1β, IL-2, IL-12, IL-13, IL-17 and transforming growth factor-beta 4 (TGF-β4) (21). Additionally, transcript analysis showed T helper 1 (Th1) interleukins such as IFN-γ, IL-2, and IL-12p40 (primarily mediators of cellular immunity) and T helper 2 (Th2) interleukins such as IL-4, IL-5, and IL-10 (primarily mediators of humoral immunity) were upregulated in response to challenge with infectious bursal disease virus in four-week old white leghorn chicks (22). Cytokine responses vary depending on the chicken line, the type of tissue assayed, the type of infection (bacterial, parasitic, viral), and the post-infection time (23, 24). Specifically, Ross broiler chickens showed significantly more expression of avian β-defensins in response to NE when compared to Cobb broilers (23). It is worthwhile to note that different broiler lines can have different immune responses to the same challenge conditions, even if this particular reaction may not be the same across all types of infections. Although a few recent studies have been conducted on cytokine modulation in response to fruit supplementation in chickens, more research and better standardization could elucidate quantitative effects of fruit byproducts on the immune system.
Modulation of the Poultry Immune System
Immune response can be evaluated in a variety of ways, such as quantifying leukocyte populations, cytokine responses, antibody concentrations, inflammation markers, phagocytic capabilities, and the masses of various immune organs. A multitude of strategies have been employed to enhance the immune and inflammatory responses in poultry. The most successful of these strategies have been vaccinations, which take advantage of the memory property of the adaptive immune system. However, there are many diseases for which no vaccine currently exists, or existing vaccines are in need of improvement. Many provide suboptimal protection, require adjuvants, and may be tedious and expensive to administer (25). Vaccines against bacterial diseases, such as NE, have lagged behind, due to the complexity of these diseases and the counterincentive of efficient and cost-effective antibiotics. Some new vaccines have been developed using attenuated Salmonella as a vector, but these must be administered via oral gavage and are therefore time-consuming in a large-scale regular production cycle (26).
Besides vaccinations to incite immunity against a specific pathogen, immunostimulating products or compounds to increase general non-specific immunity, have been also suggested as alternative solutions in poultry. These products may have pleiotropic actions on the host’s immune system. Accordingly, probiotics have been proposed as immunomodulators, to reduce pro-inflammatory cytokine expression in the gut, and increase the IgA secretion and the integrity of the gut epithelial barrier, and the serum antibody levels to certain antigens (4, 27–30). Probiotics have been specifically shown to decrease the expression of IL-12 and IFN-γ resulting from subsequent infection with S. Typhimurium (31). Another study reported that different strains of Lactobacillus can have different effects on spleen and caecal tonsil mononuclear cells: specifically that L. acidophilus induces primarily Th1 cytokines, while L. salivarius is capable of inducing more anti-inflammatory cytokines (32). Antimicrobial peptides (specifically rabbit sacculus rotundus antimicrobial peptides) have also been employed, which act both directly on bacteria and as immunostimulants leading to increased IgA levels, mast cell populations and intraepithelial lymphocyte counts (33). However, antimicrobial peptides are not an ideal solution due to their high production costs and the ability of bacteria to eventually develop resistance against them. Heterophil levels, and subsequently pro-inflammatory cytokine levels, have been artificially increased by orally administering ulvan, an extract of sea lettuce (Ulva armoricana), a proposed agonist of Toll-Like Receptor (TLR) 2 and 4 (34).
Fruit Byproducts: Processing and Properties
Fruit byproducts are complex mixtures made from processing whole fruit, seeds, juices, or pomace using a variety of techniques. Additional processing of these byproducts can result in the generation of fruit extracts, generally by using a liquid solvent to extract and concentrate valuable chemical components. These processing techniques could include freeze drying, extrusion, separation of fractions via solvent/water extraction, or dialysis to prepare pure extracts. The most abundant phenolic compounds present in fruits relevant to this paper can be found in Table 1 (35), although this does not represent an exhaustive list, as it omits trace compounds and non-phenolic compounds thought to have effects on bacteria. Fruit pomaces and extracts can contain flavonols (such as quercetin, kaempferol, myricetin and isorhamnetin), flavonoids (such as anthocyanins), flavanols (such as catechins, epicatechins, and procyanidins), flavanones, proanthocyanidins, or gallocatechins (prodelphinidins), some of which are involved in the process of fruit browning (36, 37). The non-phenolic contents such as dietary fibers, proteins, oligosaccharides, vitamins, and minerals, contain different chemical constituents depending on the species or subspecies of fruit, extraction process, and the nature of the solvent used. Freeze-drying and solvent extraction methods were applied in combination to organic cranberry and wild blueberry pomaces, after which their polyphenolic profiles were analyzed using a combination of the Glories method and the polyvinylpolypyrrolidone (PVVP) method, and high levels of polyphenols, particularly anthocyanins as well as antioxidants, were found (38). Alternatively, using high temperatures and water as a solvent when extracting grape byproducts resulted in extracting more flavonoids and procyanidins, whereas an organic solvent provided larger polymeric molecules (39). A processing method consisting of base hydrolysis followed by extraction with diethyl ether and ethyl acetate produced the highest concentration of phenolic compounds when used on apple pomace (40). Another study found that extracting pomegranate peel powder with equal volumes of ethanol and water, with a solid:solvent ratio of 1:10, for a period of 24-48 hours, was the most effective method of extracting polyphenols from pomegranates (41). The amount of polyphenolics shown in Figure 1 (35) and their composition in the fruit varies widely even within the same species, as shown by analyzing 31 different cultivars of grapes from around the world (42).
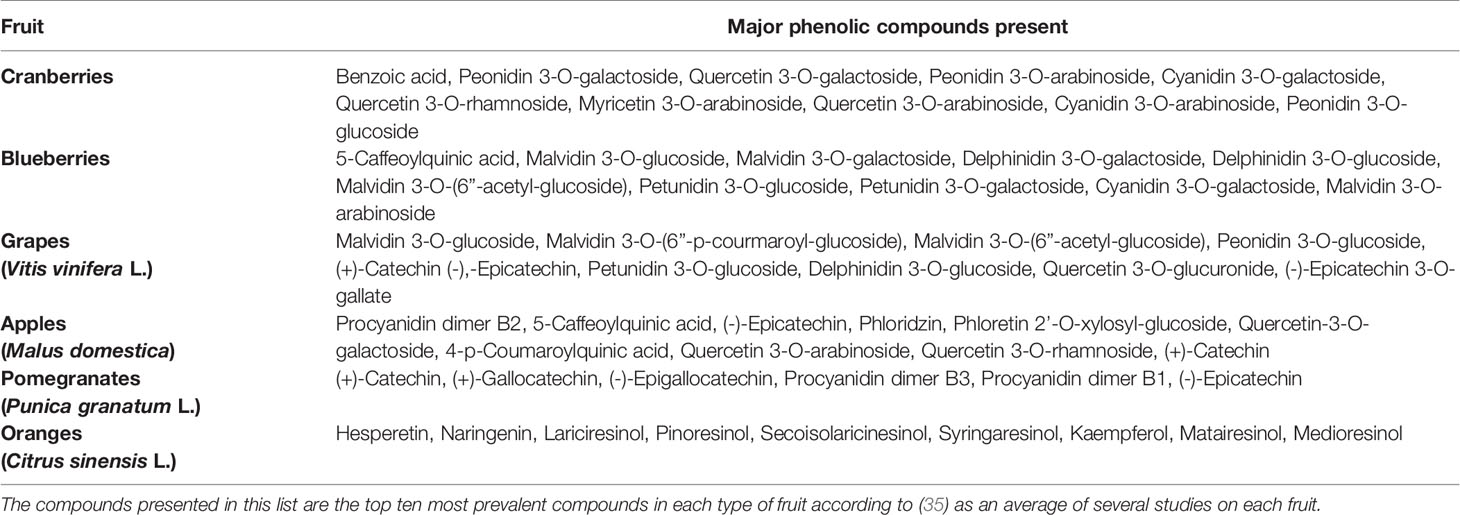
Table 1 The major phenolic compounds found in cranberries, blueberries, grapes, apples, pomegranates, and oranges.
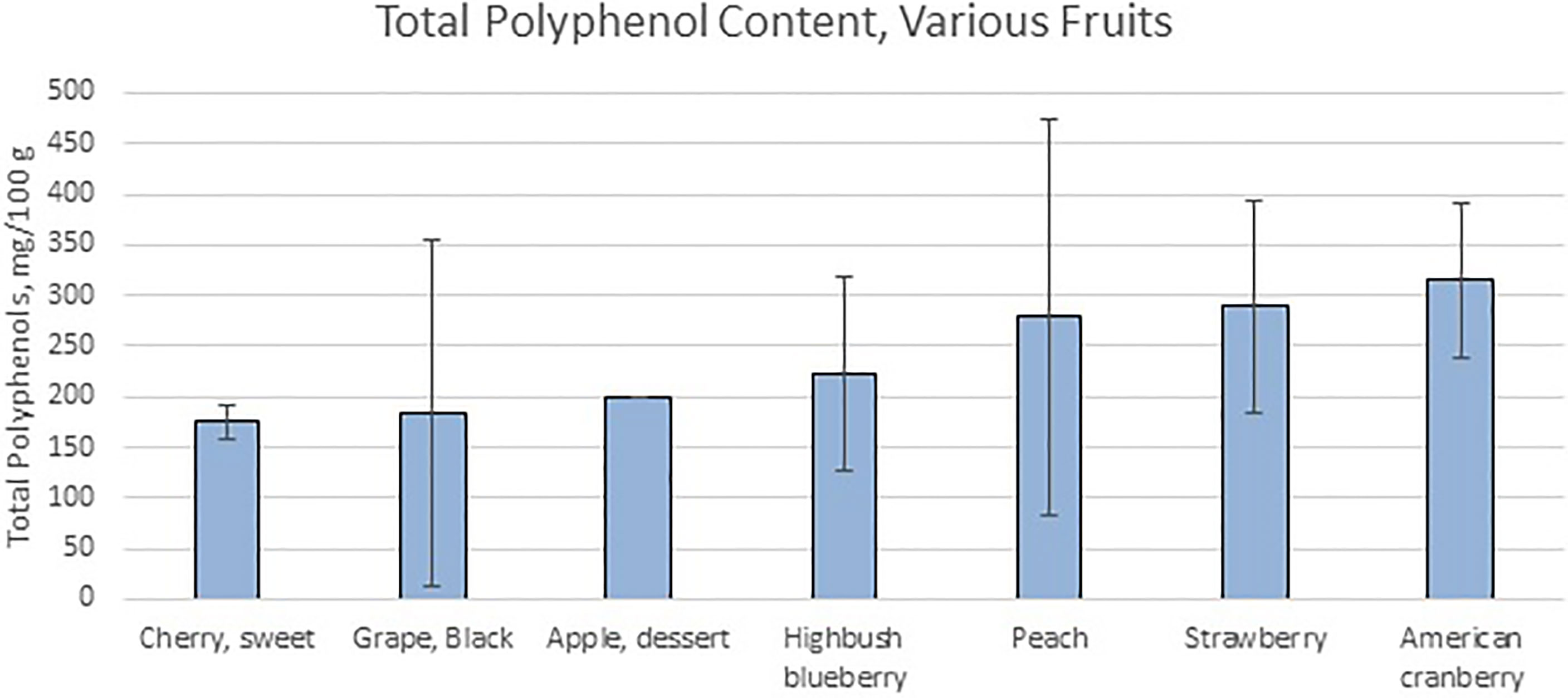
Figure 1 Total Polyphenol Content of Various Fruits shows the values of total polyphenol measured via Folin assay by various research groups. Data from (35).
Purified Bioactives
Purified, fruit-derived polyphenols, including catechin, epicatechin, gallic acid esters, gallocatechin and epigallocatechin, have demonstrated considerable antimicrobial effects against some pathogens, including Salmonella which is an important foodborne pathogen associated with poultry (12, 43). The health benefits, including antioxidant activities of polyphenols in fruits and spices on humans have been reviewed in detail elsewhere (44). Positive effects on the host’s immune system have been induced using many of these compounds, both as part of a complex mixture and as purified compounds.
Gallic acid, one of the most common phenolic compounds in plants, has been studied extensively and found to have beneficial effects in treating a large range of diseases and malignancies (45). Ellagic acid, a dimerized form of gallic acid in many types of berries, has been found to inhibit aflatoxin production by fungi species (46), decrease colitis symptoms in rats (47), inhibit the growth of several bacteria species including Helicobacter pylori (48), Aeromonas hydrophila (49), Propionibacterium acnes and S. aureus (50). Tannic acid, a polymer of gallic acid, has been shown to act synergistically to decrease minimal inhibitory concentrations (MICs) of oxacillin and cefdinir against S. aureus to less than 0.06 mg/L, although this effect was not as pronounced on blood-agar plates (51). Additionally, another study has proposed that tannic acid decreased the integrity of the bacterial cell wall by attacking peptidoglycan and prevented biofilm formation (52). The anti-inflammatory activity of caffeic acid from cranberries has been reported in murine models of colitis (53). Extractable polyphenols, found in high concentrations in many plants, have the potential to provide a wide range of health benefits. Anti-inflammatory, immunomodulatory, and antimicrobial effects of anthocyanins have been described in human, avian, and murine infection models (54). Anthocyanins are especially prevalent in berries such as grapes and strawberries. Although pure compounds have not been studied extensively in a chicken model, many in vitro and in vivo rat or human evaluations have been conducted. In light of encouraging results, it is worth considering that these compounds could have potential beneficial effects in chickens raised without antibiotic growth promoters.
Bioactive substances in fruit may have synergistic effects when applied as a mixture, as is the case when using whole fruits or pomaces, as opposed to purified or refined extracts which may contain only a few compounds. Although pure compounds are easier to standardize and more concentrated, there may be benefits of using a less processed fruit. Containing a multitude of compounds which could work synergistically, whole fruit pomaces are less likely to induce resistance in bacteria against them. In addition, pomaces have a much lower cost of processing due to fewer purification steps. The preparation of berry pomaces and their chemical compositions have been described to reflect their potential use in poultry feed. Data from these studies reported that berry pomaces and their ethanolic extracts decreased the incidence of NE and coccidiosis when compared to bacitracin, a polypeptide antibiotic traditionally used for NE disease prevention. In addition, the ethanolic extracts induced antimicrobial activities against multiple antibiotic resistant Salmonella serovars isolated from broilers. In the current efforts on developing antibiotic-free and organic broiler chicken production, it seems to be difficult to access the economic benefit of using pomace as feed additive. Proper delivery methods of pomace in feed as well as its stabilization and anticaking strategies would need to be developed further. There is also a lack of knowledge of specific modes of action of fruit pomace in order to determine an effective dose in chicken.
Modes of Action
The pleiotropic effects of fruits pomaces or extracts on immune system may be due to their multiple phenolic and non-phenolic contents. Interestingly, it has been reported that wild birds (Sylvia atricapilla) select their food dependent on the perceived flavonoid content, which when present in the diet were shown to increase the humoral immune response mounted by the birds (55). The presence of such a wealth of compounds, in addition to carbohydrates, proteins, lipids and minerals, make it difficult to establish exact mode of actions of fruits products on immune system (56). Various mechanisms of flavonoids on enzyme function and regulation of gene and protein expression have been reviewed (57). The possibility of flavonoids, including quercetin and pomegranate polyphenols, inducing T regulatory cells through the inhibition of mTOR (mechanistic target of rapamycin) has been explored, albeit in human cancer cells (58). If direct mechanisms can be suggested, indirect mechanisms such as those derived from metabolism of phytonutrients and shaping gut microbiota should not be ignored since gut microbiota play important role in immunity against pathogens (12, 56). Bioactive contents of fruits pomaces and extracts can be efficient free radical scavengers and iron chelators, which can in turn allow them to prevent diseases and combat pathogens (59). In humans, it has been reported that regular consumption of berries significantly reduced oxidative stress and improved the host's resistance against H2O2-induced DNA damage (60, 61). Understanding the mode of action of fruits and their extracts on poultry immunity would enhance the competitiveness and innovation within the food industry by: 1) providing comprehensive information on their use in production; 2) addressing increased public concerns about food security and safety; 3) decreasing the losses associated with poor poultry health while improving performance; and 4) finding a new application for fruit byproducts (wastes), which could be an inexpensive alternative to antibiotics.
Fruit Byproducts in Avian Feed
Pomaces and extracts from various fruits have been investigated in vivo as feed additives in broiler chickens, displayed in Tables 2 through 5, separated by fruit. These tables do not include studies on pure extracts (for example, ellagic acid). Between the quantities of each compound present, and possible synergistic effects of multiple chemicals, the administration of a purified compound versus raw extracts or whole fruit is different enough to make a direct comparison possibly misleading. Not every study listed in these Tables presented data on both the immune system and gut microbiota, therefore some metrics may be absent for certain studies. Studies directly measuring immune parameters would make comparison easier and should be undertaken. For the sake of brevity, only statistically significant results are mentioned within the Tables. Comparisons were made with control birds in each study unless otherwise noted. There are more studies available in the literature on carcass quality, meat preservation, and growth performance using a variety of fruits such as strawberry (78–80), however these were not included as meat quality and economic performance are not the focus of this paper.
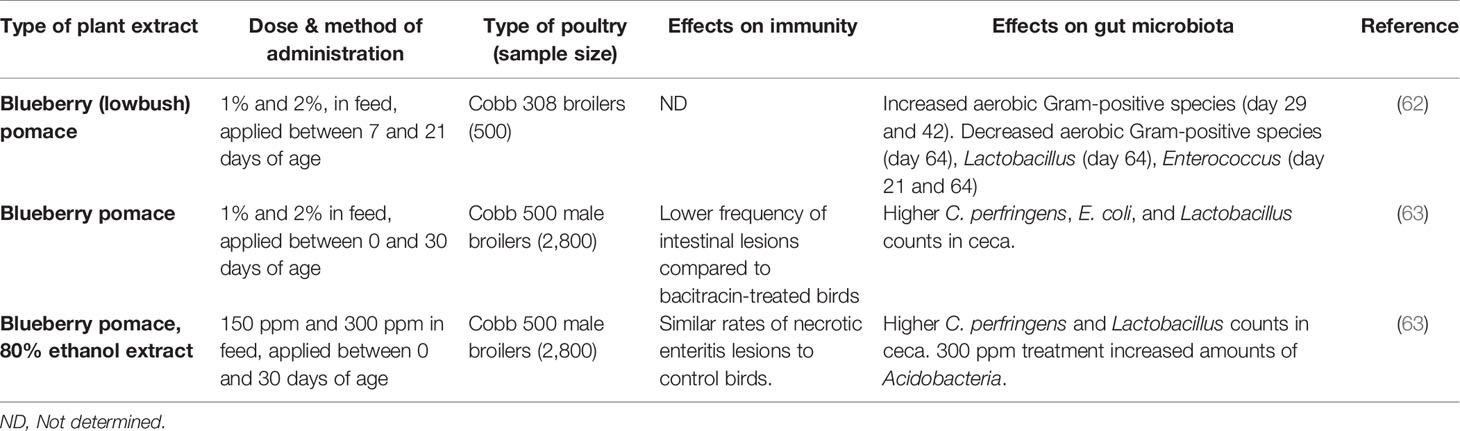
Table 2 A comparison of in vivo feeding studies of blueberry byproducts and extracts in poultry, highlighting significant effects on the immune system and gut microbiota in live chickens.
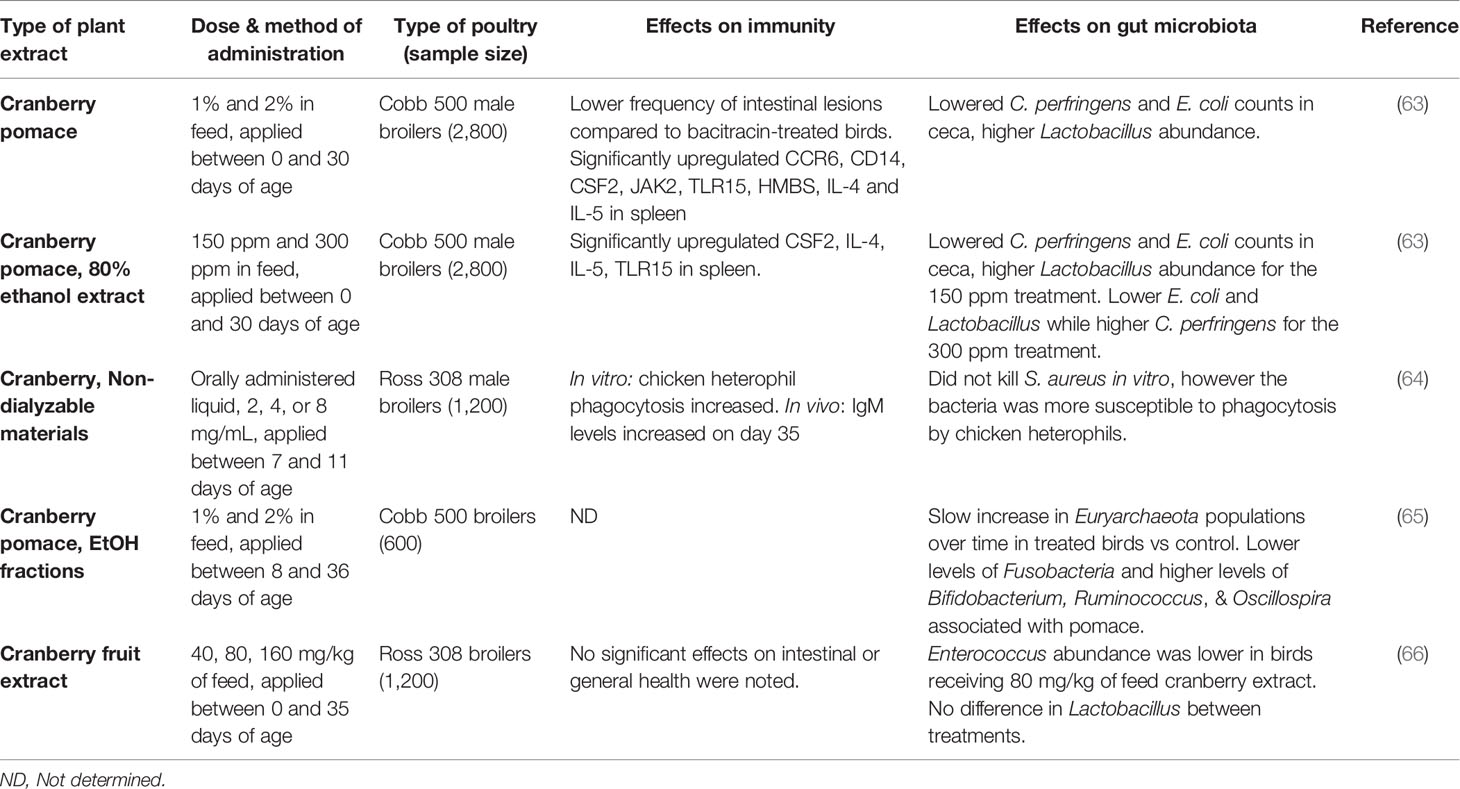
Table 3 A comparison of in vivo feeding studies of cranberry byproducts and extracts in poultry, highlighting significant effects on the immune system and gut microbiota in live chickens.
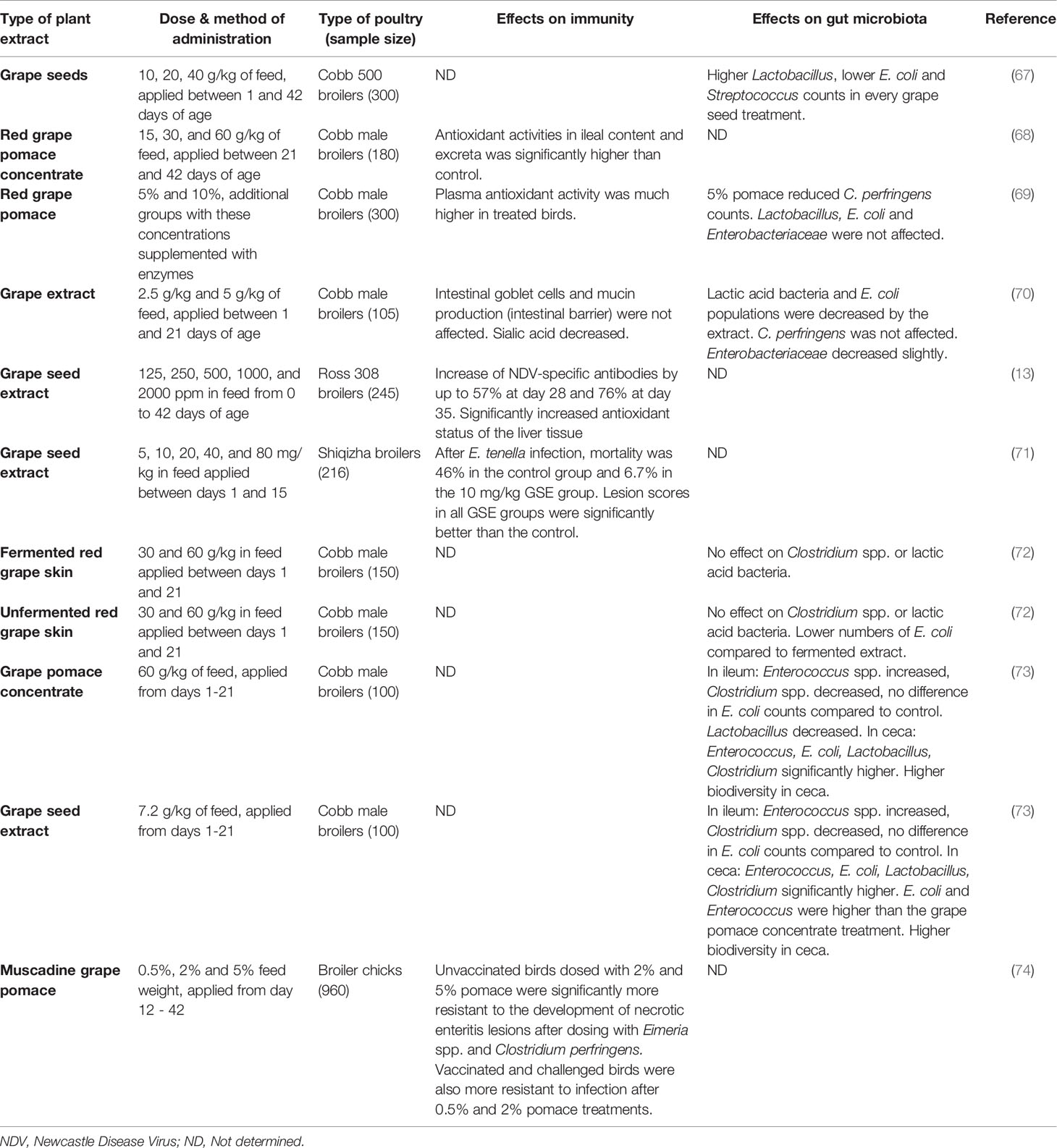
Table 4 A comparison of in vivo feeding studies of grape byproducts and extracts in poultry, highlighting significant effects on the immune system and gut microbiota in live chickens.
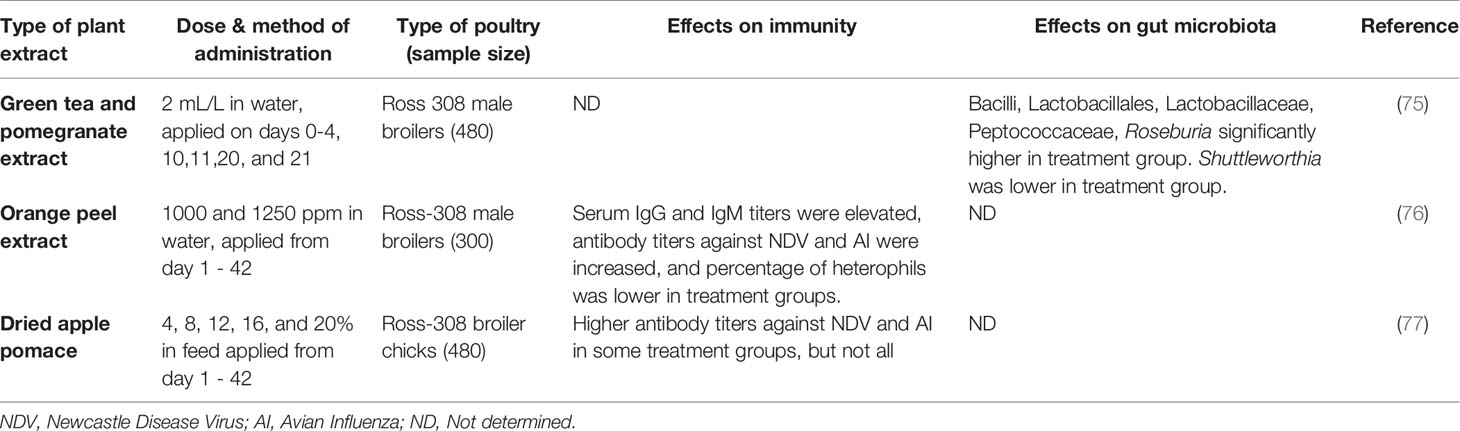
Table 5 A comparison of in vivo feeding studies of various other fruit byproducts and extracts in poultry, highlighting significant effects on the immune system and gut microbiota in live chickens.
Berries
A summary of studies performed on blueberry products can be found in Table 2, and cranberry products in Table 3. Although the immune-modifying compounds of lowbush blueberry pomace were not investigated, the pomace was found to significantly decrease concentrations of blood metabolites such as serum glucose and triglycerides in 21-day old slow growth broilers raised on pasture (62). It is worth noting that this study applied the blueberry pomace treatment for two weeks in 7-day old chicks, before transferring them to a pasture-style environment at 21 days of age. A study with non-dialyzable materials containing high-molecular weight compounds of cranberry juice enhanced phagocytic functions of chicken heterophils against S. aureus, as well as some humoral response in broilers (64). A subsequent study by the same group on cranberry pomace feeding during a longer administration period found similar effects on lowering levels of serum glucose and triglycerides while increasing blood serum iron level (65). A recent study evaluated different types of cranberry and blueberry products, including pomaces and their ethanolic extracts, and found promising results with regards to the intestinal health of the birds as stated above (63). The blood metabolites in this study once again agreed with previous studies, namely that triglycerides were significantly lower in fruit-treated birds, although iron was not significantly affected. They also found significant differences in cytokine gene expression in chickens fed cranberry products. Notably, the modulated cytokines included mostly Th2 cytokines, which help to regulate antibody-mediated immune responses via Th2 cells. Analysis of immunoglobulin levels as well as liver and bursal gene expression, revealed that dietary cranberry products increased the serum IgY level, modulated the innate immune and suppressed proinflammatory cytokines in 21-day old broilers (81).
Grapes
A summary of studies performed on grape products can be found in Table 4. When grape pomace concentrate was compared to grape seed extract (73), the levels of extractable polyphenols were standardized between the two different products, however the results between the two still differed, suggesting that there are other factors influencing microbiota besides exclusively polyphenols. Studies that measured the level of polyphenolic compounds in the blood plasma or ileal digesta consistently found that the concentration of polyphenols was higher in the tissues of treated birds vs untreated birds. This could contribute to a health benefit for consumers who eat these meats, as these compounds are regarded to have health benefits for humans in small quantities (82). Higher levels of polyphenols generally lead to higher levels of antioxidants, and therefore a greater resistance to oxidative stresses. Although all of the studies agreed on this, they did not agree on many other parameters: whether certain species of bacteria in the ileum and ceca of the birds were increased or decreased, and whether antibody or resistance levels were increased or not significantly affected. With the large variety of grapes available, all containing different levels of polyphenolics and other compounds, it can be difficult to compare two different studies that used different fruits – especially since many of the studies did not name the cultivar of grape used, the locality it was harvested in, or even whether the grape was white or red. The disparity between different cultivars of grapes, especially when grouped by geographical origin, is significant (42).
Apples, Pomegranates, and Other Fruits
Table 5 contains a summary of studies performed on other fruits, including apples, pomegranates, and oranges. Apple is an important commercial crop for many countries. In poultry it has been suggested that apple byproducts could be included up to 5% in diets for broilers and up to 10% for laying hens diet to reduce oxidative stress (83). It has been reported that inclusion of up to 12% dry apple pomace in diet increased antibody levels against some pathogenic virus in poultry (77). However, this group also found that inclusion levels of 12 – 20% lowered the feed intake of birds, which lead them to suggest this may have been caused by the high fiber content of this food source.
Pomegranates have also been used in feed and drinking water. The addition of pomegranate rind extract and green tea to broilers’ water during critical periods in development showed a significant increase in the population of bacilli, specifically Lactobacilli, in the caeca (75). Another study compared pomegranate peel, pomegranate peel extract, and purified α-tocopheryl acetate, and found a significantly higher antioxidant activity in most of the treated groups, with the exception of the birds treated with the lowest concentration of pomegranate peel (84). These authors also reported that the pomegranate peel extract and the α-tocopheryl increased the amount of polyunsaturated fatty acids in the breast meat. Supplementation with pomegranate peel extract or α-tocopheryl did not impact the performance of the birds, however the pomegranate peel decreased the body weight gain, feed intake, and feed efficiency of the birds, overall making it the least desirable treatment (85).
A study on orange peel extracts added to water found that broilers vaccinated against various diseases had elevated antibody titers against these diseases in a dose-dependent manner (76). Although this cannot be directly compared to studies on other fruits, due to the dissimilarity in both the species of fruit and the method of administration, it is worth noting this positive result.
Potential Side Effects
Although there are many positive effects of plant-derived phenolic materials, some of them demonstrated negative effects as well. For example, the sensory profile of meat from chickens fed 5% grape seed meal was found to have a less sweet and more metallic flavor, while being more stringy, but overall the diet did not significantly impact the meat acceptability (86). A study on various pure extracts in poultry feed determined that anthocyanins from cherries added to poultry feed significantly increased the daily feed intake, but resulted in a slightly lower average weight, which translates to a lower feed efficiency (87). The addition of pomegranate peel into the feed has been shown to impair body weight gain, feed intake, and feed efficiency (85). Adding more than 5% dried apple pomace to feed caused birds to lose weight (83). It is therefore not advisable to feed these additives in high concentrations without fully discerning the effects on both the living chicken and the carcass or meat quality.
Conclusion
Immune stimulation and modulation are becoming increasingly necessary in the fight against pathogens. Several options for immunomodulation have been explored, and each has its own advantages and disadvantages. While there are some substantial limitations of studying and implementing fruit by-products as feed additives, there are also many potential benefits for the immune system, thereby increasing resistance to disease. This makes fruit wastes not only inexpensive alternatives to traditional antibiotics, but value-added and environmentally friendly products that promote the circular economy. Many types of fruit contain high levels of desirable polyphenolic and antioxidant compounds. However, a balance must be struck between minimal processing (inexpensive, large volumes produced, low levels of many compounds) and high levels of processing (expensive, small volumes produced, high levels of a few targeted compounds). More studies are needed on the effects of these byproducts and their associated extracts. Additionally, the type of chicken, farm setup, feed composition, vaccination schedule, treatment schedule and dosage, as well as the parameters being evaluated need to be standardized in order for studies to be comparable. There has not yet been a study that evaluates every parameter of a certain product (immune robustness, growth performance, feed conversion ratio, resistance to disease, gut microflora, meat nutrition, sensory profile, and shelf-life). Once more is known about one type of fruit waste, comparisons to other types may be made and the most effective can be determined. This review indicates the potential of fruit products as feed supplements to enhance the immunity in birds, providing information for future investigations to develop them in poultry feeding strategies.
Author Contributions
MSD conceived the works and provided resources. TH found available literatures and wrote the paper. MSD, PB and SS provided guidelines, reviewed and edited the manuscript. All authors contributed to the article and approved the submitted version.
Funding
The research has been funded through the A-base program by Agriculture and Agri-Food Canada (PSS #2574, J-001788).
Conflict of Interest
The authors declare that the research was conducted in the absence of any commercial or financial relationships that could be construed as a potential conflict of interest.
Acknowledgments
We thank Michelle Bargel, Canadian Agriculture Library for her assistance.
References
1. Campos M. Poultry is King of Proteins in IPC Outlook: International Poultry Council (2019). Available at: https://www.internationalpoultrycouncil.com/ipc-on-the-press/ipc-press-releases/poultry-is-king-of-proteins-in-ipc-outlook.
2. OECD/FAO. Commodity Snapshots: Meat. Oecd-FAO Agricultural Outlook for 2017-2026. Paris: OECD Publishing (2017) p. 110–21.
3. Manyi-Loh C, Mamphweli S, Meyer E, Okoh A. Antibiotic Use in Agriculture and its Consequential Resistance in Environmental Sources: Potential Public Health Implications. Molecules (2018) 23(4):795. doi: 10.3390/molecules23040795
4. Gadde U, Kim WH, Oh ST, Lillehoj HS. Alternatives to Antibiotics for Maximizing Growth Performance and Feed Efficiency in Poultry: A Review. Anim Health Res Rev (2017) 18:26–45. doi: 10.1017/S1466252316000207
5. Stewart-Brown B. Vaccination Program for Broilers. Merck Veterinary Manual. Kenilworth, NJ: Merck & Co., Inc. (2015).
6. Ma L, Sun Z, Zeng Y, Luo M, Yang J. Molecular Mechanism and Health Role of Functional Ingredients in Blueberry for Chronic Disease in Human Beings. Int J Mol Sci (2018) 19(9):2785. doi: 10.3390/ijms19092785
7. Veldhoen M, Veiga-Fernandes H. Feeding Immunity: Skepticism, Delicacies and Delights. Nat Immunol (2015) 16(32):215–19. doi: 10.1038/ni.3100
8. Lee SH, Lillehoj HS, Cho SM, Chun HK, Park HJ, Lim CI, et al. Immunostimulatory Effects of Oriental Plum (Prunus salicina lindl.). Comp Immunol Microbiol Infect Dis (2009) 32(5):407–17. doi: 10.1016/j.cimid.2007.12.001
9. Saeed M, Naveed M, BiBi J, Kamboh AA, Arain MA, Shah QA, et al. The Promising Pharmacological Effects and Therapeutic/Medicinal Applications of Punica granatum l. (Pomegranate) as a Functional Food in Humans and Animals. Recent Pat Inflammation Allergy Drug Discovery (2018) 12(1):24–38. doi: 10.2174/1872213x12666180221154713
10. Yang X, Liu T, Chen B, Wang F, Yang Q, Chen X. Grape Seed Proanthocyanidins Prevent Irradiation-Induced Differentiation of Human Lung Fibroblasts by Ameliorating Mitochondrial Dysfunction. Sci Rep (2017) 7(1):62. doi: 10.1038/s41598-017-00108-9
11. Yin Lau AT, Barbut S, Ross K, Diarra MS, Balamurugan S. The Effect of Cranberry Pomace Ethanol Extract on the Growth of Meat Starter Cultures, Escherichia coli O157:H7, Salmonella enterica Serovar enteritidis and Listeria monocytogenes. LWT (2019) 115:108452. doi: 10.1016/j.lwt.2019.108452
12. Das Q, Islam MR, Marcone MF, Warriner K, Diarra MS. Potential of Berry Extracts to Control Foodborne Pathogens. Food Control (2017) 73:650–62. doi: 10.1016/j.foodcont.2016.09.019
13. Farahat MH, Abdallah FM, Ali HA, Hernandez-Santana A. Effect of Dietary Supplementation of Grape Seed Extract on the Growth Performance, Lipid Profile, Antioxidant Status and Immune Response of Broiler Chickens. Animal (2017) 11(5):771–77. doi: 10.1017/S1751731116002251
14. Kaiser P, Balic A. The Avian Immune System. In: Scanes CG, editor. Sturkie’s Avian Physiology, Sixth ed. San Diego: Academic Press (2015). p. 403–18. doi: 10.1016/B978-0-12-407160-5.00017-8
15. Raji AA, Mohammed B, Oladele SB, Saidu L, Jibril AH, Cazaban C. Bursa Body Index as a Visual Indicator for the Assessment of Bursa of Fabricius. J Vet Med Anim Health (2017) 9:32–8. doi: 10.5897/jvmah2016.0456
16. Genovese KJ, He H, Swaggerty CL, Kogut MH. The Avian Heterophil. Dev Comp Immunol (2013) 41:334–40. doi: 10.1016/j.dci.2013.03.021
17. Lowder BV, Guinane CM, Ben Zakour NL, Weinert LA, Conway-Morris A, Cartwright RA, et al. Recent Human-to-Poultry Host Jump, Adaptation, and Pandemic Spread ofStaphylococcus aureus. Proc Nat Aca Sci (2009) 106:19545–50. doi: 10.1073/pnas.0909285106
18. Zaytsoff SJM, Lyons SM, Garner AM, Uwiera RRE, Zandberg WF, Abbott DW, et al. Host Responses to Clostridium perfringens Challenge in a Chicken Model of Chronic Stress. Gut Pathog (2020) 12. doi: 10.1186/s13099-020-00362-9
19. Withanage GSK, Kaiser P, Wigley P, Powers C, Mastroeni P, Brooks H, et al. Rapid Expression of Chemokines and Proinflammatory Cytokines in Newly Hatched Chickens Infected With Salmonella enterica Serovar typhimurium. Infect Immun (2004) 72:2152–59. doi: 10.1128/iai.72.4.2152-2159.2004
20. Rothwell L, Young JR, Zoorob R, Whittaker CA, Hesketh P, Archer A, et al. Cloning and Characterization of Chicken IL-10 and its Role in the Immune Response to Eimeria maxima. J Immunol (2004) 173:2675–82. doi: 10.4049/jimmunol.173.4.2675
21. Park SS, Lillehoj HS, Allen PC, Park DW, FitzCoy S, Bautista DA, et al. Immunopathology and Cytokine Responses in Broiler Chickens Coinfected With Eimeria maxima and Clostridium perfringens With the Use of an Animal Model of Necrotic Enteritis. Avian Dis (2008) 52:14–22. doi: 10.1637/7997-041707-Reg
22. Liu H, Zhang M, Han H, Yuan J, Li Z. Comparison of the Expression of Cytokine Genes in the Bursal Tissues of the Chickens Following Challenge With Infectious Bursal Disease Viruses of Varying Virulence. Virol J (2010) 7:364. doi: 10.1186/1743-422x-7-364
23. Hong YH, Song W, Lee SH, Lillehoj HS. Differential Gene Expression Profiles of β-Defensins in the Crop, Intestine, and Spleen Using a Necrotic Enteritis Model in 2 Commercial Broiler Chicken Lines. Poult Sci (2012) 91:1081–8. doi: 10.3382/ps.2011-01948
24. Oh ST, Lillehoj HS. The Role of Host Genetic Factors and Host Immunity in Necrotic Enteritis. Avian Pathol (2016) 45:313–6. doi: 10.1080/03079457.2016.1154503
25. Perrie Y, Mohammed AR, Kirby DJ, McNeil SE, Bramwell VW. Vaccine Adjuvant Systems: Enhancing the Efficacy of Sub-Unit Protein Antigens. Int J Pharm (2008) 364:272–80. doi: 10.1016/j.ijpharm.2008.04.036
26. Kulkarni RR, Parreira VR, Sharif S, Prescott JF. Oral Immunization of Broiler Chickens Against Necrotic Enteritis With an Attenuated Salmonella Vaccine Vector Expressing Clostridium perfringens Antigens. Vaccine (2008) 26:4194–203. doi: 10.1016/j.vaccine.2008.05.079
27. Lee KW, Lillehoj HS, Jeong W, Jeoung HY, An DJ. Avian Necrotic Enteritis: Experimental Models, Host Immunity, Pathogenesis, Risk Factors, and Vaccine Development. Poult Sci (2011) 90:1381–90. doi: 10.3382/ps.2010-01319
28. Lee KW, Lee SH, Lillehoj HS, Li GX, Jang SI, Babu US, et al. Effects of Direct-Fed Microbials on Growth Performance, Gut Morphometry, and Immune Characteristics in Broiler Chickens. Poult Sci (2010) 89:203–16. doi: 10.3382/ps.2009-00418
29. Lee KW, Li G, Lillehoj HS, Lee SH, Jang SI, Babu US, et al. Bacillus subtilis-Based Direct-Fed Microbials Augment Macrophage Function in Broiler Chickens. Res Vet Sci (2011) 91:e87–91. doi: 10.1016/j.rvsc.2011.01.018
30. Haghighi HR, Gong J, Gyles CL, Hayes MA, Sanei B, Parvizi P, et al. Modulation of Antibody-Mediated Immune Response by Probiotics in Chickens. Clin Diagn Lab Immunol (2005) 12:1387–92. doi: 10.1128/CDLI.12.12.1387-1392.2005
31. Haghighi HR, Abdul-Careem MF, Dara RA, Chambers JR, Sharif S. Cytokine Gene Expression in Chicken Cecal Tonsils Following Treatment With Probiotics and Salmonella Infection. Vet Microbiol (2008) 126:225–33. doi: 10.1016/j.vetmic.2007.06.026
32. Brisbin JT, Gong J, Parvizi P, Sharif S. Effects of Lactobacilli on Cytokine Expression by Chicken Spleen and Cecal Tonsil Cells. Clin Vaccine Immunol (2010) 17:1337–43. doi: 10.1128/CVI.00143-10
33. Liu T, She R, Wang K, Bao H, Zhang Y, Luo D, et al. Effects of Rabbit Sacculus Rotundus Antimicrobial Peptides on the Intestinal Mucosal Immunity in Chickens. Poult Sci (2008) 87:250–4. doi: 10.3382/ps.2007-00353
34. Guriec N, Bussy F, Gouin C, Mathiaud O, Quero B, Le Goff M, et al. Ulvan Activates Chicken Heterophils and Monocytes Through Toll-Like Receptor 2 and Toll-Like Receptor 4. Front Immunol (2018) 9. doi: 10.3389/fimmu.2018.02725
35. Rothwell JA, Pérez-Jiménez J, Neveu V, Medina-Ramon A, M’Hiri N, Garcia Lobato P, et al. Phenol-Explorer 3.0: A Major Update of the Phenol-Explorer Database to Incorporate Data on the Effects of Food Processing on Polyphenol Content. (2013). doi: 10.1093/database/bat070
36. Diarra MS, Hassan YI, Block GS, Drover JCG, Delaquis P, Oomah BD. Antibacterial Activities of a Polyphenolic-Rich Extract Prepared From American Cranberry (Vaccinium macrocarpon) Fruit Pomace Against Listeria spp. LWT (2020) 123:109056. doi: 10.1016/j.lwt.2020.109056
37. Hassan YI, Kosir V, Yin X, Ross K, Diarra MS. Grape Pomace as a Promising Antimicrobial Alternative in Feed: A Critical Review. J Agri Food Chem (2019) 67:9705–18. doi: 10.1021/acs.jafc.9b02861
38. Ross KA, Ehret D, Godfrey D, Fukumoto L, Diarra M. Characterization of Pilot Scale Processed Canadian Organic Cranberry (Vaccinium macrocarpon) and Blueberry (Vaccinium angustifolium) Juice Pressing Residues and Phenolic-Enriched Extractives. Int J Fruit Sci (2017) 17:202–32. doi: 10.1080/15538362.2017.1285264
39. Khanal RC, Howard LR, Prior RL. Procyanidin Content of Grape Seed and Pomace, and Total Anthocyanin Content of Grape Pomace as Affected by Extrusion Processing. J Food Sci (2009) 74:H174–H82. doi: 10.1111/j.1750-3841.2009.01221.x
40. Li W, Yang R, Ying D, Yu J, Sanguansri L, Augustin MA. Analysis of Polyphenols in Apple Pomace: A Comparative Study of Different Extraction and Hydrolysis Procedures. Indus Crops Prod (2020) 147:112250. doi: 10.1016/j.indcrop.2020.112250
41. Venkataramanamma D, Aruna P, Singh RP. Standardization of the Conditions for Extraction of Polyphenols From Pomegranate Peel. J Food Sci Tech (2016) 53:2497–503. doi: 10.1007/s13197-016-2222-z
42. Xia L, Xu C, Huang K, Lu J, Zhang Y. Evaluation of Phenolic Compounds, Antioxidant and Antiproliferative Activities of 31 Grape Cultivars With Different Genotypes. J Food Biochem (2019) 43:e12626. doi: 10.1111/jfbc.12626
43. Puupponen-Pimiä R, Nohynek L, Alakomi HL, Oksman-Caldentey KM. The Action of Berry Phenolics Against Human Intestinal Pathogens. Biofactors (2005) 23:243–51. doi: 10.1002/biof.5520230410
44. Shahidi F, Ambigaipalan P. Phenolics and Polyphenolics in Foods, Beverages and Spices: Antioxidant Activity and Health Effects – a Review. J Func Foods (2015) 18:820–97. doi: 10.1016/j.jff.2015.06.018
45. Kahkeshani N, Farzaei F, Fotouhi M, Alavi SS, Bahramsoltani R, Naseri R, et al. Pharmacological Effects of Gallic Acid in Health and Diseases: A Mechanistic Review. Iran J Basic Med Sci (2019) 22:225–37. doi: 10.22038/ijbms.2019.32806.7897
46. Soni KB, Rajan A, Kuttan R. Reversal of Aflatoxin Induced Liver Damage by Turmeric and Curcumin. Cancer Lett (1992) 66:115–21. doi: 10.1016/0304-3835(92)90223-i
47. Rosillo MA, Sanchez-Hidalgo M, Cárdeno A, Alarcón De La Lastra C. Protective Effect of Ellagic Acid, a Natural Polyphenolic Compound, in a Murine Model of Crohn’s Disease. Biochem Pharmacol (2011) 82:737–45. doi: 10.1016/j.bcp.2011.06.043
48. De R, Sarkar A, Ghosh P, Ganguly M, Karmakar BC, Saha DR, et al. Antimicrobial Activity of Ellagic Acid Against Helicobacter pylori Isolates From India and During Infections in Mice. J Antimicrob Chemother (2018) 73:1595–603. doi: 10.1093/jac/dky079
49. Abuelsaad ASA, Mohamed I, Allam G, Al-Solumani AA. Antimicrobial and Immunomodulating Activities of Hesperidin and Ellagic Acid Against Diarrheic Aeromonas hydrophila in a Murine Model. Life Sci (2013) 93:714–22. doi: 10.1016/j.lfs.2013.09.019
50. Panichayupakaranant P. Antibacterial Activity of Ellagic Acid-Rich Pomegranate Rind Extracts. Planta Med (2010) 7:6. doi: 10.1055/s-0030-1264706
51. Akiyama H, Fujii K, Yamasaki O, Oono T, Iwatsuki K. Antibacterial Action of Several Tannins Against Staphylococcus aureus. J Antimicro Chemother (2001) 48:487–91. doi: 10.1093/jac/48.4.487
52. Dong G, Liu H, Yu X, Zhang X, Lu H, Zhou T, et al. Antimicrobial and Anti-Biofilm Activity of Tannic Acid Against Staphylococcus aureus. Nat Prod Res (2018) 32:2225–28. doi: 10.1080/14786419.2017.1366485
53. Zhang Z, Wu X, Cao S, Wang L, Wang D, Yang H, et al. Caffeic Acid Ameliorates Colitis in Association With Increased Akkermansia Population in the Gut Microbiota of Mice. Oncotarget (2016) 7:31790–9. doi: 10.18632/oncotarget.9306
54. Changxing L, Chenling M, Alagawany M, Jianhua L, Dongfang D, Gaichao W, et al. Health Benefits and Potential Applications of Anthocyanins in Poultry Feed Industry. World Poult Sci J (2018) 74:251–64. doi: 10.1017/S0043933918000053
55. Catoni C, Schaefer HM, Peters A. Fruit for Health: The Effect of Flavonoids on Humoral Immune Response and Food Selection in a Frugivorous Bird. Func Ecol (2008) 22:649–54. doi: 10.1111/j.1365-2435.2008.01400.x
56. Williams AR, Andersen-Civil AIS, Zhu L, Blanchard A. Dietary Phytonutrients and Animal Health: Regulation of Immune Function During Gastrointestinal Infections. J Anim Sci (2020) 98. doi: 10.1093/jas/skaa030
57. González-Gallego J, García-Mediavilla MV, Sánchez-Campos S, Tuñón MJ. Fruit Polyphenols, Immunity and Inflammation. Br J Nutri (2010) 104:S15–27. doi: 10.1017/s0007114510003910
58. Hosseinzade A, Sadeghi O, Naghdipour Biregani A, Soukhtehzari S, Brandt GS, Esmaillzadeh A. Immunomodulatory Effects of Flavonoids: Possible Induction of T CD4+ Regulatory Cells Through Suppression of Mtor Pathway Signaling Activity. Front Immunol (2019) 10:51. doi: 10.3389/fimmu.2019.00051
59. Katalinić V, Možina SS, Skroza D, Generalić I, Abramovič H, Miloš M, et al. Polyphenolic Profile, Antioxidant Properties and Antimicrobial Activity of Grape Skin Extracts of 14 Vitis vinifera Varieties Grown in Dalmatia (Croatia). Food Chem (2010) 119:715–23. doi: 10.1016/j.foodchem.2009.07.019
60. Lin L-C, Kuo Y-C, Chou C-J. Immunomodulatory Proanthocyanidins From Ecdysanthera utilis. J Nat Prod (2002) 65:505–08. doi: 10.1021/np010414l
61. Pérez-Jiménez J, Arranz S, Saura-Calixto F. Proanthocyanidin Content in Foods is Largely Underestimated in the Literature Data: An Approach to Quantification of the Missing Proanthocyanidins. Food Res Int (2009) 42:1381–88. doi: 10.1016/j.foodres.2009.07.002
62. Islam MR, Lepp D, Godfrey DV, Orban S, Ross K, Delaquis P, et al. Effects of Wild Blueberry (Vaccinium angustifolium) Pomace Feeding on Gut Microbiota and Blood Metabolites in Free-Range Pastured Broiler Chickens. Poult Sci (2019) 98:3739–55. doi: 10.3382/ps/pez062
63. Das Q, Islam MR, Lepp D, Tang J, Yin X, Mats L, et al. Gut Microbiota, Blood Metabolites, and Spleen Immunity in Broiler Chickens Fed Berry Pomaces and Phenolic-Enriched Extractives. Front Vet Sci (2020) 7:150. doi: 10.3389/fvets.2020.00150
64. Islam MR, Oomah DB, Diarra MS. Potential Immunomodulatory Effects of non-Dialyzable Materials of Cranberry Extract in Poultry Production. Poult Sci (2016) 96:341–50.. doi: 10.3382/ps/pew302
65. Islam MR, Hassan YI, Das Q, Lepp D, Hernandez M, Godfrey DV, et al. Dietary Organic Cranberry Pomace Influences Multiple Blood Biochemical Parameters and Cecal Microbiota in Pasture-Raised Broiler Chickens. J Func Foods (2020) 72:104053. doi: 10.1016/j.jff.2020.104053
66. Leusink G, Rempel H, Skura B, Berkyto M, White W, Yang Y, et al. Growth Performance, Meat Quality, and Gut Microflora of Broiler Chickens Fed With Cranberry Extract. Poult Sci (2010) 89:1514–23.. doi: 10.3382/ps.2009-00364
67. Abu Hafsa SH, Ibrahim SA. Effect of Dietary Polyphenol-Rich Grape Seed on Growth Performance, Antioxidant Capacity and Ileal Microflora in Broiler Chicks. J Anim Physiol Anim Nutr (Berl) (2018) 102:268–75. doi: 10.1111/jpn.12688
68. Brenes A, Viveros A, Goñi I, Centeno C, Sáyago-Ayerdy SG, Arija I, et al. Effect of Grape Pomace Concentrate and Vitamin E on Digestibility of Polyphenols and Antioxidant Activity in Chickens. Poult Sci (2008) 87:307–16. doi: 10.3382/ps.2007-00297
69. Chamorro S, Viveros A, Rebolé A, Arija I, Romero C, Alvarez I, et al. Addition of Exogenous Enzymes to Diets Containing Grape Pomace: Effects on Intestinal Utilization of Catechins and Antioxidant Status of Chickens. Food Res Int (2017) 96:226–34. doi: 10.1016/j.foodres.2017.02.010
70. Chamorro S, Romero C, Brenes A, Sánchez-Patán F, Bartolomé B, Viveros A, et al. Impact of a Sustained Consumption of Grape Extract on Digestion, Gut Microbial Metabolism and Intestinal Barrier in Broiler Chickens. Food Funct (2019) 10:1444–54. doi: 10.1039/c8fo02465k
71. Wang ML, Suo X, Gu JH, Zhang WW, Fang Q, Wang X. Influence of Grape Seed Proanthocyanidin Extract in Broiler Chickens: Effect on Chicken Coccidiosis and Antioxidant Status. Poult Sci (2008) 87:2273–80. doi: 10.3382/ps.2008-00077
72. Nardoia M, Romero C, Brenes A, Arija I, Viveros A, Ruiz-Capillas C, et al. Addition of Fermented and Unfermented Grape Skin in Broilers’ Diets: Effect on Digestion, Growth Performance, Intestinal Microbiota and Oxidative Stability of Meat. Animal (2020) 14:1371–81. doi: 10.1017/s1751731119002933
73. Viveros A, Chamorro S, Pizarro M, Arija I, Centeno C, Brenes A. Effects of Dietary Polyphenol-Rich Grape Products on Intestinal Microflora and Gut Morphology in Broiler Chicks. Poult Sci (2011) 90:566–78. doi: 10.3382/ps.2010-00889
74. McDougald LR, Hofacre C, Mathis G, Fuller L, Hargrove JL, Greenspan P, et al. Enhancement of Resistance to Coccidiosis and Necrotic Enteritis in Broiler Chickens by Dietary Muscadine Pomace. Avian Dis (2008) 52:646–51. doi: 10.1637/8306-041508-reg.1
75. Perricone V, Comi M, Giromini C, Rebucci R, Agazzi A, Savoini G, et al. Green Tea and Pomegranate Extract Administered During Critical Moments of the Production Cycle Improves Blood Antiradical Activity and Alters Cecal Microbial Ecology of Broiler Chickens. Animals (2020) 10:785. doi: 10.3390/ani10050785
76. Pourhossein Z, Qotbi AAA, Seidavi A, Laudadio V, Centoducati G, Tufarelli V. Effect of Different Levels of Dietary Sweet Orange (Citrus sinensis) Peel Extract on Humoral Immune System Responses in Broiler Chickens. Anim Sci J (2015) 86:105–10. doi: 10.1111/asj.12250
77. Aghili AH, Toghyani M, Tabeidian SA. Effect of Incremental Levels of Apple Pomace and Multi Enzyme on Performance, Immune Response, Gut Development and Blood Biochemical Parameters of Broiler Chickens. Int J Recycl Organic Waste Agri (2019) 8:321–34. doi: 10.1007/s40093-019-00305-8
78. Saha J, Debnath M, Saha A, Ghosh T, Sarkar PK. Response Surface Optimisation of Extraction of Antioxidants From Strawberry Fruit, and Lipid Peroxidation Inhibitory Potential of the Fruit Extract in Cooked Chicken Patties. J Sci Food Agri (2011) 91:1759–65. doi: 10.1002/jsfa.4374
79. Banaszkiewicz T, Białek A, Tokarz A, Kaszperuk K. Effect of Dietary Grape and Pomegranate Seed Oil on the Post-Slaughter Value and Physicochemical Properties of Muscles of Broiler Chickens. Acta Sci Pol Technol Aliment (2018) 17:199–209. doi: 10.17306/j.afs.0563
80. Gungor E, Erener G. Effect of Dietary Raw and Fermented Sour Cherry Kernel (Prunus cerasus l.) on Growth Performance, Carcass Traits, and Meat Quality in Broiler Chickens. Poult Sci (2020) 99:301–09. doi: 10.3382/ps/pez490
81. Das Q, Tang J, Yin X, Ross K, Warriner K, Marcone MF, et al. Organic Cranberry Pomace and its Ethanolic Extractives as Feed Supplement in Broiler: Impacts on Serum Ig Titers, Liver and Bursal Immunity. Poult Sci (2020). doi: 10.1016/j.psj.2020.09.044
82. Chen Y, Wen J, Deng Z, Pan X, Xie X, Peng C. Effective Utilization of Food Wastes: Bioactivity of Grape Seed Extraction and its Application in Food Industry. J Func Foods (2020) 73:104113. doi: 10.1016/j.jff.2020.104113
83. Azizi M, Seidavi AR, Ragni M, Laudadio V, Tufarelli V. Practical Applications of Agricultural Wastes in Poultry Feeding in Mediterranean and Middle East Regions. Part 1: Citrus, Grape, Pomegranate and Apple Wastes. World Poult Sci J (2018) 74:489–98. doi: 10.1017/s0043933918000478
84. Saleh H, Golian A, Kermanshahi H, Mirakzehi MT. Effects of Dietary α-Tocopherol Acetate, Pomegranate Peel, and Pomegranate Peel Extract on Phenolic Content, Fatty Acid Composition, and Meat Quality of Broiler Chickens. J Appl Anim Res (2017) 45:629–36. doi: 10.1080/09712119.2016.1248841
85. Saleh H, Golian A, Kermanshahi H, Mirakzehi MT. Antioxidant Status and Thigh Meat Quality of Broiler Chickens Fed Diet Supplemented With α-Tocopherolacetate, Pomegranate Pomace and Pomegranate Pomace Extract. Ital J Anim Sci (2018) 17:386–95. doi: 10.1080/1828051x.2017.1362966
86. Francesch A, Cartañà M. The Effects of Grape Seed in the Diet of the Penedes Chicken, on Growth and on the Chemical Composition and Sensory Profile of Meat. Br Poult Sci (2015) 56:477–85. doi: 10.1080/00071668.2015.1062842
87. Csernus B, Biró S, Babinszky L, Komlósi I, Jávor A, Stündl L, et al. Effect of Carotenoids, Oligosaccharides and Anthocyanins on Growth Performance, Immunological Parameters and Intestinal Morphology in Broiler Chickens Challenged With Escherichia coli Lipopolysaccharide. Animals (2020) 10:347. doi: 10.3390/ani10020347
Keywords: cranberry, blueberry, grape, other fruits, byproducts, poultry, immune stimulation
Citation: Hasted T-L, Sharif S, Boerlin P and Diarra MS (2021) Immunostimulatory Potential of Fruits and Their Extracts in Poultry. Front. Immunol. 12:641696. doi: 10.3389/fimmu.2021.641696
Received: 14 December 2020; Accepted: 21 April 2021;
Published: 17 May 2021.
Edited by:
Guan Yang, University of Florida, United StatesReviewed by:
Kyung-Woo Lee, Konkuk University, South KoreaAli Nazmi, Vanderbilt University Medical Center, United States
Copyright © 2021 Patrick Boerlin, Shayan Sharif and Her Majesty the Queen in Right of Canada, as represented by the Minister of Agriculture and Agri-Food Canada for the contribution of Moussa S. Diarra and Teri-Lyn Hasted. This is an open-access article distributed under the terms of the Creative Commons Attribution License (CC BY). The use, distribution or reproduction in other forums is permitted, provided the original author(s) and the copyright owner(s) are credited and that the original publication in this journal is cited, in accordance with accepted academic practice. No use, distribution or reproduction is permitted which does not comply with these terms.
*Correspondence: Moussa Sory Diarra, bW91c3NhLmRpYXJyYUBjYW5hZGEuY2E=