- Fish Immunology and Pathology Laboratory, Animal Health Research Centre (CISA-INIA), Madrid, Spain
Probiotics have been defined as live microorganisms that when administered in adequate amounts confer health benefits to the host. The use of probiotics in aquaculture is an attractive bio-friendly method to decrease the impact of infectious diseases, but is still not an extended practice. Although many studies have investigated the systemic and mucosal immunological effects of probiotics, not all of them have established whether they were actually capable of increasing resistance to different types of pathogens, being this the outmost desired goal. In this sense, in the current paper, we have summarized those experiments in which probiotics were shown to provide increased resistance against bacterial, viral or parasitic pathogens. Additionally, we have reviewed what is known for fish probiotics regarding the mechanisms through which they exert positive effects on pathogen resistance, including direct actions on the pathogen, as well as positive effects on the host.
History and Definition of Probiotics in Aquaculture
The term “probiotic” comes from the Latin word “pro” (for) and the Greek word “bios” (life) meaning “for life” (1) and it refers to microbial feed additives which confer a health benefit to the host organism through the modulation of intestinal microbiota. This first definition provided the basis of differentiating probiotics from antibiotics. The term “probiotics” was first proposed by Lilly and Stillwell (2) as “substances secreted by a micro-organism that stimulate the growth of another organism”, being substances microbially produced “factors”. Later on, Parker (3) was the first who defined probiotics as “organisms and substances which contribute to intestinal microbial balance”. As new findings emerged, the definition of “probiotic” was modified over the years. In 1989, Fuller defined probiotics as “live microbial feed supplements which beneficially affect the host animal by improving its intestinal microbial balance” (4), where the use of live microorganisms is emphasized, and the use of the word “substances” is removed, avoiding confusion. To accommodate the immunostimulatory effect of probiotics, Naidu et al. (5) modified the concept of probiotics as “microbial dietary adjuvants that beneficially affect the host physiology by modulating mucosal and systemic immunity, as well as improving nutritional and microbial balance in the intestinal tract”. Since then, many variations to the definition have still been proposed (6). The Food and Agriculture Organization of the United Nations/World Health Organization (FAO/WHO) integrated all these definitions and stated that probiotics are “live microorganisms, which when administered in adequate amounts confer a health benefit on the host” (7).
The first reported use of probiotics in aquaculture was in 1986 by Kosaza who evaluated the use of Bacillus toyoi spores as feed additives to increase the growth rate of yellowtail, Seriola quinqueradiata (8). But it was not until the late 1990s that research on probiotics became prominent in aquaculture. Given the fact that the aquatic animals constantly interact with their surrounding water environment, Moriarty (9) widened the definition of probiotics, also considering them as microbial “water additives”. Later on, Verschuere et al. (10) put forward the concept of aquaculture probiotics, proposing a broader application of the term as “live microbial adjuncts which have a beneficial effect on the host by modifying the host-associated or ambient microbial community by ensuring improved use of the feed or enhancing its nutritional value, by enhancing the host response towards diseases, or by improving the quality of its environment”. This definition allowed a wider application of the term “probiotic” by involving the aquatic environment.
The outmost desired goal of a probiotic is to have a positive effect on the general health status of the fish, thus increasing its resistance to pathogens. This can be achieved through different mechanisms, reviewed in the current paper, that cover direct interference with pathogens as well as effects on the host. Additionally, we have summarized those probiotics that have been shown to confer resistance against different types of pathogens, including bacteria, viruses and parasites.
Source and Selection Criteria of Probiotics in Aquaculture
In the last decades, several microorganisms have been experimentally identified, characterized and applied in aquacultured species as probiotics. Probiotics tested for these species include a wide range of bacteria (Gram-negative or Gram-positive), yeasts, microalgae and bacteriophages which have been added to the water or included as feed supplements (11–17). Nevertheless, the list of probiotics commercially available for use in aquaculture is much more limited (18).
The source from where the probiotic microorganism is obtained varies greatly, including for example, the intestine of healthy fish, water of rearing environment, sediments of culture tanks, other animals or fermented food products (19). Because the main principle of a probiotic is to establish a relation with beneficial and harmful bacteria usually present in fish intestine, the gastrointestinal tract (20, 21) and the mucus (22) of aquatic animals are usually the most common sources to isolate microorganisms which can be used as potential probiotics. Although the probiotics could also have an origin outside the host, host-derived microorganisms are preferred given that microbiota living in healthy hosts can be considered part of the natural defense system, being beneficial to the host in multiple ways (23, 24). Furthermore, probiotics indigenous to the environment are able to survive spontaneously and function physiologically at their optimum level (21). It has to be taken into account, that, in contrast to terrestrial animals, the gastrointestinal microbiota of aquatic species is strongly dependent on the external environment due to the continuous water flow through the digestive tract. Hence, most of bacteria that colonize the tract are transient and could vary if the environmental conditions change (25).
In recent years, a large number of scientific works have been published regarding the screening, selection and characterization of fish probiotic bacterial strains (26–35). Potential candidates isolated from different sources are subjected to screening through multiple steps in order to assess their potential as ideal probiotics. Their safety (10) and lack of pathogenicity (36) have to be demonstrated as an essential first step. Thereafter, a successful probiotic candidate should meet certain criteria. Merrifield et al. (37) proposed an extended list of criteria, classifying them as either essential or favorable. As new findings emerged over the last decades, additional criteria have been added (12, 14). Taking all of this into account, the essential criteria to consider a microorganism as a suitable probiotic are the following: not being pathogenic, not only with regards to the host species, but also with regards to aquatic animals in general and human consumers; being free of plasmid-encoded antibiotic resistance genes; having the ability to tolerate a wide range of pH (low acidic to high alkaline) and high concentration (>2.5%) of bile salts. On the other hand, the merely favorable characteristics include: being able to adhere to and/or grow well within the intestinal mucus; being able to colonize the intestinal epithelial surface; being registered for use as a feed additive; displaying advantageous growth characteristics (e.g. short lag period, a short doubling time and/or growth at host rearing temperatures); exhibiting a broad spectrum of antagonistic activity against one or more key pathogens; producing relevant extracellular digestive enzymes or vitamins; being indigenous to the host or the rearing environment; remaining viable under normal storage conditions and being robust enough to survive industrial processes; having good sensorial properties, fermentative action, tolerance towards freeze-drying and viability in feed during packaging and storing process; having a beneficial effect on the growth, stimulation of immunity and protection of fish against various pathogenic bacteria. Although it is unlikely to find a candidate that will fulfill all of these characteristics, the more of these characteristics are fulfilled, the more likely it will be a promising probiotic. However, the main driver to select potential probiotics among different candidates has been their inhibitory activity against target pathogens in vitro (10, 28, 30, 38–40) or in vivo (11, 14, 15, 21, 41).
Mechanisms of Interference of Probiotics With Pathogens
Production of Inhibitory Substances
The antagonistic action or the inhibition of a variety of pathogens is one of the most important sought properties for potential probiotics. Probiotic microorganisms often have the capacity to produce substances which have bacteriostatic or bactericidal impact on pathogenic microbes, such as lysozymes, proteases, siderophores, hydrogen peroxide or bacteriocins (9, 42–50). For example, a compound named indole (2,3-benzopyrrole) with potent inhibitory activity against bacteria and fungus has been identified in some probiotic bacteria (51, 52). Similarly, some microorganisms produce volatile fatty acids (acetic, butyric, lactic and propionic acid) and organic acid, decreasing the gastrointestinal lumen’s pH, thereby preventing the proliferation of opportunistic pathogens (10, 47, 53–55).
In aquaculture, some candidate probiotics have been shown to produce antibacterial substances that inhibit the growth of harmful microbes and maintain intestinal microecological balance (43, 49). Thus, several probiotics used in aquaculture have been documented to exert direct antibacterial activities against known pathogens (14, 17). On the other hand, while knowledge on antiviral activity of probiotics has increased in recent years (14, 17), the exact mechanism of action through which these probiotic bacteria produce their antiviral effects remains still unknown. Yet, some studies performed in vitro revealed that the inhibition of viruses can occur through the action of extracellular enzymes secreted by the bacteria (14, 17). Finally, only a few studies have been reported the antifungal properties of fish probiotics (14, 17).
Competition for Nutrients and Available Energy
All organisms, including bacteria, require a continuous source of nutrients for survival, growth and proliferation. Probiotics consume the available nutrients, thus, competition for nutrients is one of the mechanisms through which probiotics can inhibit pathogens (56). In fact, the survival of a microorganism will mainly depend on its potential to compete for nutrients and energy with other microorganisms in the same environment (10).
Among nutrients, iron is recognized to be the most important element, as it is an essential cofactor for important cellular processes, being required for DNA replication, oxygen transport, protection against oxidative stress, enzyme activity and energy generation (57). Thus, the majority of bacteria need iron for their growth, but the amount of iron available in animal tissues and body fluids is very limited. As a consequence, the competition for this nutrient between pathogenic bacteria and the host is a very well-known process (58). Siderophores are ferric ion specific chelators that are able to dissolve precipitated iron or extract it from iron complexes under iron-stressed conditions, making it available for bacterial growth (59). Siderophores are produced by several bacteria and fungus (59). Thus, the ability to produce siderophores is a favorable characteristic of a microorganism to be considered as a potential probiotic, in an iron-limited environment, as the probiotic would sequester ferric ion making it unavailable for the growth of pathogenic bacteria (60).
Siderophore production of fish probiotic strains has been investigated to some extent (61). Thus, for example, Smith and Davey (62) and Gram et al. (63) reported the inhibition of the growth of Aeromonas salmonicida and Vibrio anguillarum, respectively, under iron-limited conditions by Pseudomonas fluorescens. Similarly, Lazado et al. (64) showed the capacity of two bacterial isolates (GP21 Pseudomonas sp.; GP12 Psychrobacter sp.) obtained from the intestinal tract of Atlantic cod (Gadus morhua) to release siderophores, showing antagonistic activity against V. anguillarum and A. salmonicida. Also, the probiotic effect of a strain of Vibrio sp. has been associated with its capacity to compete for iron with a pathogenic strain of Vibrio sp. in seabass larvae (Dicentrarchus labrax) (65).
Competition for Colonization of Mucosal Surfaces
The mucosal surface of fish is continuously interacting with the microbiota and the external media in an aquatic environment. In this context, pathogens invade the host through these mucosal surfaces, colonizing them and eventually spreading throughout the host and causing disease (66). Thus, most pathogenic bacteria need to attach to the mucosal layer of the host gastrointestinal tract (or other mucosal tissues) to exert a harmful effect and develop an infection (67).
In this sense, the ability of a microorganism to colonize and adhere to the epithelial surface, interfering with the pathogen’s adhesion is a favorable characteristic for the selection of candidate probiotics (37). In fact, competition for adhesion receptors with pathogens can be considered as an essential probiotic characteristic (68). Probiotics occupy the binding sites of the intestinal mucosa, forming a physical barrier, preventing the attachment of pathogenic microorganisms. Attachment of probiotics may be non-specific, based on physicochemical agents, or specific, based on the interaction of surface receptors on the adherent bacteria to receptor molecules on epithelial cells (19, 69). The mechanism through which a probiotic competes for adhesion sites is referred to as “competitive exclusion” (17).
Various authors have reported the ability of candidate fish probiotics to adhere to the host gastrointestinal tract and to interfere with pathogenic bacteria (66, 70–72). The interference of four potential probiotics (members of Vibrionaceae and Pseudomonodaceae families, as well as Micrococcus genus) with the pathogens Listonella anguillarum and Vibrio harveyi through competition for adhesion to the skin, gill and intestinal mucus of gilthead seabream (Sparus aurata) and Senegalese sole (Solea senegalensis) was demonstrated in vitro by Chabrillón et al. (73, 74). Furthermore, the in vivo probiotic potential of one of the selected candidates (Pdp11, Vibrionaceae) was assessed by oral administration and its ability to reduce the mortality after a challenge in gilthead seabream and sole against L. anguillarum and V. harveyi demonstrated, highlighting the relevance of this probiotic capacity. Another in vitro study investigated the potential of two candidate probiotic bacteria (GP21 and GP12) to adhere to primary cultures of epithelial cells obtained from different segments of the intestine and to interfere with the adhesion of two pathogens, V. anguillarum and A. salmonicida subsp. salmonicida in Atlantic cod. The study concluded that the adhesion of probiotics is segment-specific and the interference with the pathogen adhesion is dependent on both the source of epithelial cells and the mechanism through which the probiotic adheres to the epithelial cells (70). Through an in vivo study, Divya et al. (75) also confirmed the ability of three probiotic strains (Bacillus coagulans, Bacillus mesentericus and Bifidobacterium infantis) to colonize the gut of rosy barb (Puntius conchonius). This probiotic administration significantly changed the proportion of the gut microflora, decreasing the level of pathogenic strains. Vine et al. (72) reported the in vitro suppression of bacterial pathogen growth (Aeromonas hydrophila and Vibrio alginolyticus) as a consequence of their displacement by different probiotic candidate isolates that adhered to the intestinal mucus of spotted grunter (Pomadasys commersonnii). Similarly, the capacity of endogenous microbiota, e.g. Lactobacilli, to compete with pathogens for adhesion sites on the intestinal surface has also been established (76).
From a practical point of view, whether the applied probiotic is able to colonize the gut and for how long is a key issue to establish its administration regime (administration route, concentration and time of administration) and to provide farmers with a specific protocol with beneficial effects on fish health.
Disruption of Quorum Sensing
Quorum sensing (QS) is the regulation of gene expression in response to fluctuations in cell-population density (77). QS is a regulatory mechanism by which the majority of bacteria communicate with each other and response collectively. To this end, bacteria synthesize and secrete small chemical signal molecules called auto-inducers whose concentration can be recognized by other bacteria, and in this way, perceive the surrounding cell density. Gram-negative bacteria secrete acyl-homoserine lactones (AHLs) as auto-inducers, while Gram-positive bacteria use oligopeptides. Both Gram-negative and Gram-positive bacteria can produce autoinducer-2 (AI-2). When a critical threshold concentration is achieved, the QS induces or represses the expression of genes involved in specific physiological functions (77, 78), including luminescence, virulence, motility, sporulation and biofilm formation (79–83).
As pathogenicity is controlled by QS, inhibiting this mechanism is a good strategy to control microbial pathogens. Thus, the disruption of QS is considered a potential anti-infective strategy in aquaculture (17, 84, 85). Quorum quenching (QQ), the disruption of QS, can be performed by molecule antagonists (86) or degrading enzymes (87). Thus, the QQ microorganisms can be used as potential quenchers of quorum-sensing-regulated functions in pathogenic bacteria (88, 89), acting as an alternative to antibiotics in the control of infections in aquatic systems. In aquaculture, QQ has also been demonstrated as an alternative to antibiotic control of infections (90, 91). In this context, probiotic bacteria with QQ capacities would be on one hand efficacious to control antibiotic-resistant pathogens while having other beneficial effects on the host (92). Along this line, searching for probiotics isolated from the intestinal microbiota of olive flounder (Paralichthys olivaceus), Zhang et al. (93) identified AHL lactonase (FiaL) in the genome of Flaviramulus ichthyoenteri. This FiaL degraded some signals used by different fish pathogens such as A. hydrophila, Edwardsiella tarda, Vibrio salmonicida and V. anguillarum; revealing a great potential of F. ichthyoenteri as a fish probiotic. Other studies reported the ability of some microorganisms to produce QS antagonists, such as halogenated furanones, which are produced by the marine red alga Delisea pulchra (94). These compounds were reported to protect Brachionus, Artemia, and rainbow trout (Oncorhynchus mykiss) from the negative effects of pathogenic Vibrio species (95, 96).
Other probiotic bacteria such as Lactobacillus, Bifidobacterium and Bacillus cereus strains degrade the signal molecules of pathogenic bacteria by enzymatic secretion or production of autoinducer antagonists (76). Thus, Bacillus sp. QSI-1 has been shown to significantly reduced the pathogenicity of A. hydrophila in Carassius auratus gibelio (84), zebrafish (Danio rerio) (79) and goldfish (Carassius aurata) (97) by degrading AHLs. Likewise, Ren et al. (98) reported the inhibition of growth and virulence of A. hydrophila by Bacillus subtilis involving QS. Another Bacillus species, Bacillus licheniformis, protects against A. hydrophila in zebrafish through QQ (99). In rainbow trout, Delshad et al. (100) established the QQ activity of different isolates (B. cereus, Bacillus thuringiensis, Stenotrophomonas moltiphilia, Enterobacter hormaechei subsp. hormaechei and Citrobacter gillenii), regulating the virulence of Yersinia ruckeri.
A recent publication focused on the isolation of autochthonous AHL degrading bacteria from the gastrointestinal tract of different fish species. Thus, Ghanei-Motlagh et al. (101) isolated several strains with beneficial QQ AHL-degrading and probiotic activities for the first time in Asian seabass (Lates calcarifer). Vadassery and Pillay (92) also focused at isolating AHL degrading bacteria from the gastrointestinal tract of Nile tilapia (Oerocrhomis niloticus). Among the isolated strains, Enterococcus faecium contained an autoinducer inactivation homolog gene with the ability to degrade N-AHL (N-acyl homoserine lactone) produced by the fish pathogen A. hydrophila.
Immunomodulatory Effects of Probiotics
Probiotics have been shown to have the capacity to increase innate and adaptive immunity of fish, being the effects exerted on the fish innate immune system the main desirable characteristics of candidate probiotics (102). Probiotics can influence both the systemic and the local immunity of the host when they are administered i) orally or through the rearing water, or ii) as live or as dead cells (102). In some studies, the immunomodulatory effect of probiotics was attributed to the release of cytokines, key regulators in orchestrating the immune response in fish, which include interleukins (ILs), tumor necrosis factors (TNFs), interferons (IFNs), transforming growth factors (TGF) and chemokines from immune cells such as lymphocytes, granulocytes, macrophages, mast cells, epithelial cells, and dendritic cells (DCs) (103, 104). In this review, we report some of the immunomodulatory effects that probiotics have been shown to exert on the mucosal immune system.
As mentioned above, fish are constantly interacting with their surrounding water environment. In this sense, mucosal tissues are strategically located in areas where environmental pathogens enter the body. Thus, the mucosal immune system has a pivotal role in the defense mechanism against pathogens and thus considered as a very active immunological site (105). The mucosal surfaces of the fish include the epithelia and the mucosa-associated lymphoid tissues (MALTs). The main MALTs in teleost fish include: GALT (gut-associated lymphoid tissue), SALT (skin-associated lymphoid tissue), GIALT (gill-associated lymphoid tissue) and NALT (nasopharynx-associated lymphoid tissue). All teleost MALTs have common features: i) the presence of a mucus layer, that envelops the majority of the epithelia and consisting mainly of high molecular weight glycoproteins called mucins secreted by the epithelial globet cells. This mucus layer acts as a physical and chemical barrier preventing the entry of pathogens; ii) the presence of innate and adaptive immune components, such as cytokines or immunoglobulins (Igs), among many others; iii) the transport of antibodies across the epithelium by the polymeric Ig receptor (pIgR); and iv) the presence of a complex and diverse commensal bacterial community (microbiota) that plays a critical role in maintaining the host’s physiology homeostasis. In contrast to mammals, the intestinal immune system of fish lacks lymphoid tissue aggregates such as the Peyer’s patches, instead they have a diffuse GALT, and the inductive and effector sites of the lamina propria can hence not be distinguished from each other. However, similar to higher vertebrates, GALT contains mucosal immune cells such as lymphocytes, plasma cells, granulocytes and macrophages present in the epithelium or distributed in the lamina propria (105–107) and these potentiate this mucosal tissue as an active immune organ.
Many studies have demonstrated that probiotic supplementation influences the GALT by modulating gut morphology and the population of intestinal immune cells as well as their physiological activities (102, 108). In addition, probiotics could also manipulate the richness and diversity of the commensal gut microbiota, which in turn may interact with pathogens to influence their success in the intestine. However, to date, despite the great advances made in this field in the past years, there are still many gaps regarding our understanding of how microbiota composition influences mucosal responses in teleosts.
Lactic acid bacteria (LAB) and Bacillus spp. are among the most commonly used probiotic candidates in aquaculture (108, 109). Thus, several effects in the gut immune system have been reported upon LAB administration in different fish species. For example, the administration of Lactobacillus rhamnosus in Nile tilapia resulted in increased villous height in the proximal and mid intestine as well as increased intraepithelial lymphocytes numbers and acidophilic granulocytes (110). In an earlier study performed in rainbow trout, the co-administration of Lactococcus lactis subsp. lactis, Leuconostoc mesenteroides and Lactobacillus sakei resulted in an enhanced phagocytic activity of gut leukocytes (111). Other direct effects on the gut immune system that have been observed in LAB-fed fish include: stimulation of pro-inflammatory cytokines such as IL-1, IL-6, IL-2, TNF-α and IFN-γ and also anti-inflammatory cytokines such as IL-10 and TGF-β; increased gene expression of immune-related genes such as MHC II or IgM; increased presence of T cells; increased mucin-secreting goblet cell numbers; increased total Ig concentration (112–117). Certainly, all these probiotic immune effects vary depending on the types of LAB administered and on the host species, but in general are mostly immunostimulatory pro-inflammatory effects. It is interesting to note that, in contrast, the effects of probiotics on the gut immune system in mammals are mostly anti-inflammatory (118). In mammals, probiotics have been seen to provoke anti-inflammatory effects indirectly by maintaining or repairing epithelial barriers, enhancing the production of short chain fatty acids with anti-inflammatory properties or by inducing the synthesis of antimicrobial peptides that influence inflammation resolution in the mucosa. Also in mammals, probiotics bind innate immune receptors and trigger pathways that affect the production of both pro- and anti-inflammatory cytokines. Despite the capacity to induce both types of cytokines, the overall balance is generally anti-inflammatory. Hence, although the reason for this discrepancy between the effects that probiotics have on inflammation in fish and mammals is currently unknown, it seems obvious that the immunomodulatory properties of a given probiotic are not only dependent on the inherent features of the microorganism used but also on the complexity of the immune system of the host.
Regarding Bacillus spp., numerous investigations have demonstrated their efficacy and potency as probiotics in aquaculture (109). In an overview, Bacillus probiotics have been shown to have the capacity to modulate some innate immune responses such as phagocytic and lysozyme activity, respiratory burst, antiprotease and peroxidase, superoxide dismutase and myeloperoxidase through effects on different some immunocompetent cell populations. These probiotics have also been shown to generate changes in the physiology of immune cells, for example, increasing of neutrophil adherence capacity, neutrophil migration and plasma bactericidal activity that in the end can result in the improvement of immune effector functions such as enhancement in complement activity, Ig production and cell cytotoxicity (119–121). All these immune-stimulatory effects exerted by Bacillus occur in the GALT, although further research work is needed to understand the detailed mechanisms.
The mucus is a key element of mucosal immunity, thus, some studies have focused on determining how probiotics affect the mucus layer in different ways. Hence, for example, Cerezuela and collaborators extensively studied the effects of diets enriched with two different probiotics, Shewanella putrefaciens and Bacillus sp. on the skin mucus of gilthead seabream (122). Both probiotics were shown to significantly alter the carbohydrate composition of the mucus, its IgM content and its enzymatic activity, with some differences depending on the probiotic used. In the case of Nile tilapia, feed supplementation with Lactobacillus plantarum (123) or Bacillus licheniformis (124) was shown to increase the enzymatic activity of the skin mucus, whereas diets containing different Bacillus strains significantly augmented its nitric oxide (NO) and IgM content, and lysozyme and alkaline phosphatase activity (125). The protein profile of the skin mucus was also altered in Crucian carp (Carassius auratus gibelio) fed Bacillus cereus (126) or Lactobacillus acidophilus (127). Despite their relevance, the number of studies that have investigated the effects of probiotics on the intestinal mucus is much more reduced. In this sense, some studies have reported a significant increase in the number of goblet cells in the intestinal mucosa (125, 128) or an increased IgM content of the intestinal mucus (128) in response to a prolongued administration of probiotics.
Finally, it has to be mentioned that many other studies have addressed the systemic immune effects of fish probiotics, but due to length restrictions of this review, we will not refer to them in depth. Most of these studies have been focused on describing increased serum IgM levels (129), increased humoral innate immune parameters or transcriptional changes in systemic immune tissues (reviewed in 129–131) upon probiotic treatment.
Additional Effects of Probiotics on Fish
Production of Beneficial Substances
Probiotics can also produce some substances with beneficial effects, which are useful to the host for feed conversion, growth performance and immunity. Thus, the capability of a microorganism to produce extracellular enzymes, such as proteases, amylases, cellulases, phytases, chitinases, lipases, etc., is also a desirable characteristic of a probiotic candidate. Fish produce a wide range of endogenous enzymes such as those listed above (132–135), however, their quantity and activity are not adequate for a complete metabolism of the ingested materials from feed. Thus, enzymes secreted by permanent gut endosymbionts and potential probiotics are essential from a nutritional perspective (136), contributing to the digestive process of the host. In recent years, the capacity of several fish probiotic strains to produce extracellular enzymes has been extensively investigated (12, 16). For example, Dawood et al. (137) reported that Lactobacillus plantarum significantly enhanced amylase, lipase and protease activity of Nile tilapia. Supplementation of olive flounder with this probiotic (L. plantarum) as well as with Bacillus sp. increased several enzyme activities such as amylase, trypsin and lipase (138). Significant increase of theses enzymes, together with proteases was also reported in carp (Cyprinus carpio) after the administration of Lactobacillus casei in combination with β-glucan and mannan oligosaccharide (139). Other LAB, Lactobacillus bulgaricus and Lactobacillus acidophilus, together with Citrobacter were reported to increase amylase, trypsin and alkaline phosphatase in rainbow trout (140). Tarkhani et al. (141) described the increase of intestinal digestive enzyme activities of Caspian roach (Rutilus caspicus) after the administration of E. faecium. Despite of the reported results, the actual contribution of these enzymes to the fish metabolism is still not well understood.
In general, fish do not produce any vitamins and endosymbionts/probiotics are the primary producers of vitamins, making them available to the host. Thus, many probiotics have been shown to supply vitamins, fatty acids and essential amino acids to the host (45, 111, 142, 143). Besides bacterial probiotics, many strains of yeast have been used as dietary supplements in a number of fish species (144). Interestingly, yeasts can produce polyamines, which enhance intestinal maturation (145). Therefore, considering the provision of vital nutrients such as fatty acids, biotin and vitamins, probiotics might be also considered as a complementary food source (10).
Promotion of Growth Performance
As probiotics contribute to improve the feed consumption and nutrient’s uptake, they also have positive effects on the host growth rate (146). Thus, probiotics often lead to an enhanced growth performance, as well as an increased survival rate.
Lactobacillus is the most studied genus of bacteria regarding its effects on growth performance. Dietary administration of L. plantarum enhanced growth parameters of several fish species (carp, Nile tilapia, brown trout, Salmo trutta caspius; 123, 137, 147–150). Furthermore, the combination of L. plantarum with other probiotics and natural immunostimulants was also shown to increase of growth rate in different fish. Thus, Alishahi et al. (151) reported an increase in the weight gain of carp after dietary administration of a combination of L. plantarum with L. bulgaricus. The growth performance of Nile tilapia was increased after administration of L. plantarum together with the fungus Cordyceps militaris (152), and the catfish (Pangasius bocourti) with artichoke (153) or with Bacillus velezensis (154). L. lactis is another probiotic whose positive effect on growth performance has been reported in several farmed fish species, when administered alone (155–158); in combination with immunostimulants, such as β-glucan and mannan oligosaccharide (139); or other Lactobacillus (117). The ability to increase the growth rate has been demonstrated for other species of Lactobacillus, such as Lactobacillus delbrueckii (159), L. rhamnosus (117, 160), L. bulgaricus, L. acidophilus (138), and for other bacteria species, such as Citrobacter in combination with L. bulgaricus and L. acidophilus (140), Pediococcus (161, 162) and Enterococcus (141, 163). For example, Asian seabass increased its growth after the administration of a commercial probiotic consisting in Lactobacillus spp., E. faecium, B. subtilis and Saccharomyces cerevisiae. Streptoccocus faecium in combination with L. acidophilus and S. cerevisiae was also reported to act as a growth promoter for Nile tilapia (164, 165).
The role of Bacillus probiotics as growth promoters has been reported in several farmed fish species. Thus, dietary administration of B. subtilis enhances growth of Nile tilapia (164, 166), carp (167) and grass carp, Ctenopharyngodon idella (168). B. subtilis has been administered in combination with L. lactis and increase the growth of rohu, Labeo rohita (169), as well as Peidococcus acidilactici in rainbow trout (170). Growth of catfish was increased due to the administration of Bacillus amyloliquefaciens and Bacillus pumilus (171). Similarly, B. coagulans enhanced the growth of carp (172) and B. licheniformis functioned as a growth promoter in tilapia (173).
Established Effects of Probiotics on Pathogen Resistance
The use of probiotics in aquaculture is still faced with a lot of controversies and skepticism. However, the capacity of probiotics to increase the resistance of fish against different types of pathogens, including bacteria, viruses and parasites, has been widely demonstrated experimentally by higher survival rates upon pathogen challenge in probiotic-treated fish when compared to controls (15, 56, 108). Thus, in this section, we briefly review all the published data concerning increased resistance of aquacultured fish to bacteria, viruses or parasites upon probiotic treatment. The main information regarding these studies has been also summarized in Table 1 (bacteria), Table 2 (viruses) and Table 3 (parasites).
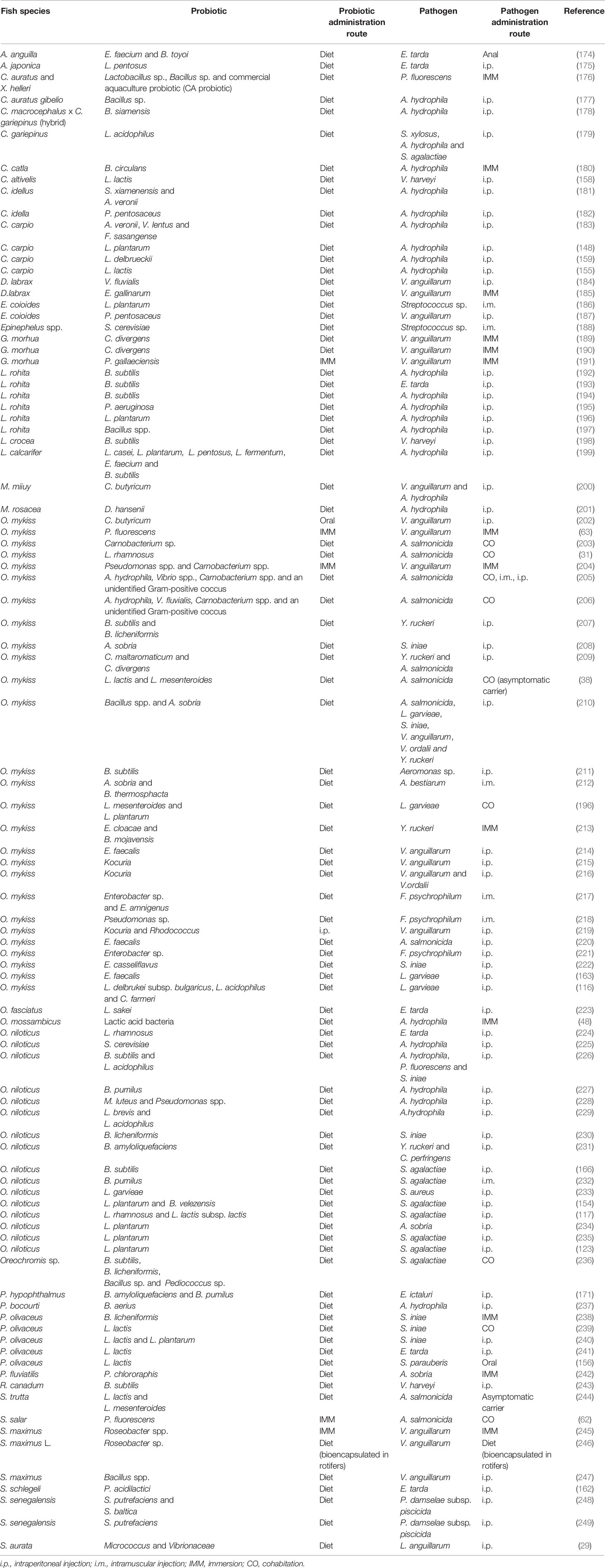
Table 1 Probiotics assayed in vivo in aquacultured species which have shown to confer significant resistance against bacterial pathogens.
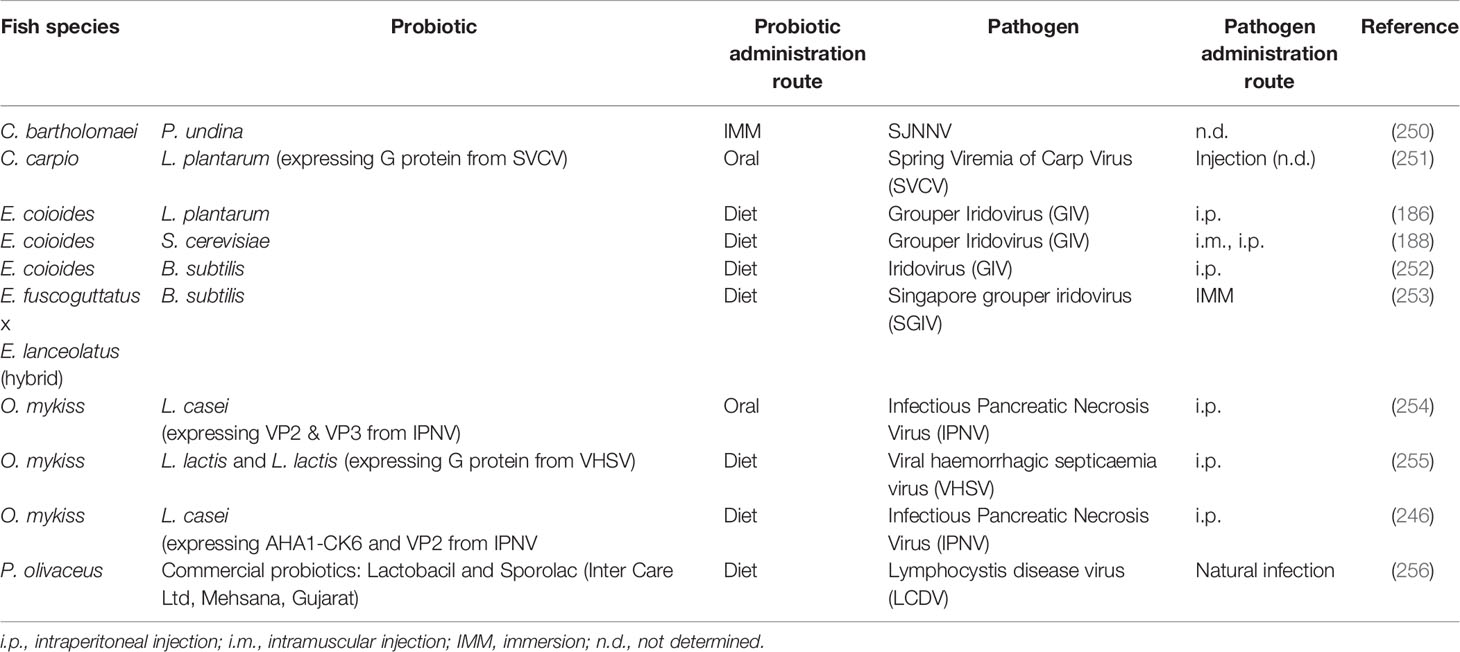
Table 2 Probiotics assayed in vivo in aquacultured species which have shown to confer significant resistance against viral pathogens.

Table 3 Probiotics assayed in vivo in aquacultured species which have shown to confer significant resistance against parasites.
Probiotics Providing Resistance to Bacterial Pathogens
Most of the investigations in the literature that have studied pathogen resistance conferred by probiotics have studied it in relation to bacterial pathogens.
As mentioned above, the most commonly used probiotic species in aquaculture include genera Lactobacillus and Bacillus (108, 260). In all the studies summarized in Table 1, apart from these probiotic species, there are other Gram-positive bacteria frequently used that include genera Carnobacterium, Lactococcus, Leuconostoc, Pediococcus, Enterococcus, Clostridium, Micrococcus, Rhodococcus and Kocuria. Regarding the Gram-negative bacteria, Pseudomonas, Aeromonas, Shewanella, Enterobacter, Citrobacter, Roseobacter, Vibrio and Flavobacterium have also been tested with positive effects. Regarding yeast, the genera Saccharomyces is the most commonly used. Interestingly, in numerous investigations the mixture of different probiotic candidates, mainly LAB or Bacillus spp. together or with other species (see Table 1) resulted in higher disease resistance against bacteria (also reviewed in 108). Indeed, as mentioned throughout the review, the combination of different probiotics and other immunostimulants resulted in higher positive effects on the host, not only regarding pathogen resistance but also on growth performance or in the immune response.
In almost all studies described in Table 1, the probiotic candidates were administered along with the diet. Lactobacillus spp. constitutes one of the probiotics for which antibacterial activity has been more frequently shown. Thus, the dietary supplementation of L. rhamnosus increased disease resistance of rainbow trout against A. salmonicida (31); Nile tilapia against E. tarda (224) and, in combination with L. lactis, also increased disease resistance of Nile tilapia against Streptococcus agalactiae (117). In case of dietary inclusion of L. plantarum, it has also been shown that significantly increased disease resistance of common carp and L. rohita against A. hydrophila (148, 196); rainbow trout against Lactoccocus garvieae (261); Epinephelus coioides against Streptococcus sp. (186) and Nile tilapia against Aeromonas sobria (234). Other bacteria species of genera Lactobacillus, such as L. pentosus, L. acidophilus, L. fermentum, L. delbrueckii or L. casei have also been studied as probiotic candidates improving disease resistance against a variety of bacterial pathogens, when administered with the diet alone or in combinations (see Table 1). Regarding Lactococcus spp., it has been shown that diet supplementation of these probiotic species also led to the improvement disease resistance of common carp against A. hydrophila (155); Chromileptes altivelis against V. harveyi (158); rainbow trout against A. salmonicida (38); olive flounder against E. tarda (241), S. iniae (239, 240) and also against Streptococcus parauberis (156); Nile tilapia against Staphylococcus aureus (233); Oreochromis mossambicus against A. hydrophila (48) and brown trout against A. salmonicida (244). In many other studies, Carnobacterium spp. were the selected microorganisms to be investigated as probiotic candidates and dietary administration of these bacteria species resulted in enhanced disease resistance of Atlantic cod against V. anguillarum (189, 190); and rainbow trout against Y. ruckeri and/or A. salmonicida (203, 209). Also, in some investigations, the mixture of these bacteria together with A. hydrophila and Vibrio spp., resulted in increased resistance of rainbow trout against A. salmonicida (203, 206, 262). Similar results were also revealed in numerous investigations where Bacillus spp. appears as the selected probiotic agent to study the control of fish disease (Table 1). For example, dietary supplementation of B. subtilis and B. licheniformis significantly increased disease resistance of rainbow trout against Y. ruckeri (207). Likewise, rainbow trout fed Bacillus spp. and A. sobria showed enhanced disease resistance against S. iniae (210), and L. rohita fed B. subtilis showed enhanced disease protection against E. tarda (193) and A. hydrophila (194, 197). In case of fish-fed with Aeromonas spp., increased disease resistance against S. iniae and A. salmonicida were shown (206, 208, 210, 262). Another study worth mentioning is that of Gong et al. (182), that isolated a new Pediococcus pentosaceus strain (SL001) which exhibited a wide antimicrobial spectrum against fish pathogens, including A. hydrophila, Aeromonas veronii, A. sobria, E. tarda, L. garvieae, and Plesiomonas shigelloide. Less frequent are the studies that used yeasts as probiotic agents; however, we found a few of them in the literature in which dietary supplementation with S. cerevisiae significantly increased the disease resistance of Ephinephelus spp. against streptococcosis (188) or Nile tilapia against A. hydrophila (225); in the same way, fish-fed Debaryomyces hansenii presented increased disease resistance against A. hydrophila (201). The effect of dietary inclusion of other selected probiotics, such as Clostridium butyricum or Enterobacter cloacae, to control fish disease against a variety of bacterial pathogens, such as vibriosis or yersiniosis, respectively, are also summarized in Table 1.
Probiotics Providing Resistance to Viral Pathogens
Some studies searching for bacteria with antiviral activity have been carried out in fish, especially during the 80s and 90s (Table 2). In 1988, Kamei et al. performed a plaque reduction assay to screen the antiviral activity of bacteria isolated from fresh water salmonid hatcheries against infectious hematopoietic necrosis virus (IHNV). The results showed that different Pseudomonas spp. and Aeromonas spp. strains produced a 90% plaque reduction (263). In 1997, Maeda et al. performed a natural infection of the yellow jack (Carangoides bartholomaei) larvae with Sima-aji Neuro Necrosis Virus (SJNNV), reporting that the bacterial strain Pseudoalteromonas undina VKM-124 showed an inhibitory activity towards SJNNV (250), consequently increasing the survival rate of the yellow jack larvae. In another study, carried out by Son et al., the dietary administration of the probiotic L. plantarum enhanced disease resistance of the grouper E. coioides against grouper iridovirus (GIV). Interestingly, fish fed L. plantarum also showed enhanced growth and innate immune responses, such as respiratory burst or plasma lysozyme activity among other effects (186). In a similar way, Chiu et al., found that the dietary administration of the yeast probiotic, S. cerevisiae P13 isolated from fermented peaches, enhanced disease resistance of E. coioides against GIV (188). Two years later, Liu et al., observed a similar effect in fish supplemented with B. subtilis E20 isolated from fermented boiled soybeans and then infected with GIV (252). Decreased fish mortality and increased survival rate were observed. In both studies, the administered probiotics also enhanced the innate immune responses (respiratory burst, plasma lysozyme activity, phagocytosis activity and alternative complement activity). Also, dietary supplementation with commercial probiotic named Lactobacil, individually or mixed with Sporolac, in olive flounder naturally infected with lymphocystis disease virus (LCDV) enhanced disease resistance (256). In another study, hulong grouper fed B. subtilis 7k were significantly strengthened in innate immune functions when compared with those fed with control diets. Moreover, B. subtilis 7k supplementation inhibited infection by Singapore grouper iridovirus (SGIV) (253).
In other cases, probiotic strains have been used as vectors to administer viral antigens to the host. Through this strategy, probiotics would exert all their beneficial effects and be at the same time vaccine vectors. Along this line, a study carried out by Min et al. with rainbow trout orally immunized with Lactobacillus-expressing the VP2 and VP3 protein of the infectious pancreatic necrosis virus (IPNV) resulted in reduced viral loads, as analyzed by real-time RT-PCR after IPNV challenge (254). Likewise, oral immunization of rainbow trout with recombinant L. lactis NZ3900 expressing the G gene of viral hemorrhagic septicemia virus (VHSV) resulted in a significant reduction of viral loads and decreased fish mortality after viral challenge (255). Increased resistance to IPNV was also detected in rainbow trout orally immunized with recombinant L. casei expressing the viral antigens (264). Similar results were observed in common carp orally immunized with recombinant L. plantarum expressing the G protein of spring viremia of carp virus (SVCV).
Probiotics Providing Resistance to Parasites
Although parasitic infections often provoke lower mortalities than viral and bacterial pathogens, they adversely affect animal health, with an enormous impact on aquaculture from an economic point of view. Despite this, not many studies have investigated the capacity of probiotics to confer resistance against different types of parasites (Table 3). In one of these studies, Pieters et al. demonstrated that the oral administration of A. sobria GC2 and Brochothrix thermosphacta BA211 to rainbow trout conferred increased resistance against Ichthyophthirius multifiliis (Ich), with the A. sobria GC2 strain being more effective in its protecting role (212). In a similar study, dietary supplementation with B. subtilis spores expressing Clonorchis sinensis paramyosin protected grass carp from cercaria infection (257). In another study, catfish exposed to L. plantarum showed a reduced infection with Saprolegnia parasitica (259). Finally, in Yanuhar et al. (258), probiotic formulations containing a mixture of Bacillus spp., Lactobacillus sp. and Nitrosomonas sp., were administered at different doses to Koi carp and resulted in increased disease protection against Myxobolus sp., in terms of gill tissue damage reduction. Further investigations are needed in fish to explore additional effects of probiotic treatment on the resistance to parasitic infections.
General Conclusions
The use of probiotics in aquaculture is a promising approach to increase fish health status and reduce the impact of infectious diseases. Reports in the past years, have broadly established that there is a wide range of probiotic microorganisms that can produce beneficial effects to the host, including immunostimulatory effects, increased resistance to pathogens, stimulation of growth, increased digestion or even improved water quality. Despite all these studies, the use of probiotics in aquaculture is not as extended as would be expected taking into account the effort that has been devoted to this research field in the past years. In this sense, we thought of importance to gather in a specific review all direct evidence of increased protection to pathogens conferred by probiotic administration. As visualized in this review, most of the efforts have been directed to establish how probiotics can protect against bacterial infections, but much less is known regarding their antiviral or antiparasitic effects. Nevertheless, probiotic application is a dynamic research field in the sense that there is a continuous search for new probiotic candidates that have even more beneficial effects. Despite the numerous reports on fish probiotics, the mechanisms through which these probiotics exert their effects has not yet been clarified in many cases. Other practical and safety issues also need to be addressed to convince farmers that probiotics are safe and eco-friendly alternatives to chemotherapy. Thus, for each probiotic candidate, the best administration regime should be established, determining for how long these probiotics should be administered to colonize the mucosal surfaces and obtain optimal results. Finally, the safety of each candidate has to be established beyond doubt, including a determination of the antibiotic resistance and a confirmation that any resistance will not be transferred to surrounding microorganisms. Hopefully, all these studies focused on providing insights on the mechanisms of action of probiotics, practical administration issues and safety will contribute to stimulate the regular use of probiotics in aquaculture.
Author Contributions
RS, CT, and PD-R collected information and wrote the manuscript with help and contributions from FD and NN-O. All authors contributed to the article and approved the submitted version.
Funding
This work was supported by the European Research Council (ERC Consolidator Grant 2016 725061 TEMUBLYM), by the Spanish Ministry of Science, Innovation and Universities (project AGL2017-85494-C2-1-R) and by the Comunidad de Madrid (grant 2016-T1/BIO-1672).
Conflict of Interest
The authors declare that the research was conducted in the absence of any commercial or financial relationships that could be construed as a potential conflict of interest.
References
2. Lilly DM, Stillwell RH. Probiotics: growth-promoting factors produced by microorganisms. Science (1965) 147(3659):747–8. doi: 10.1126/science.147.3659.747
4. Fuller R. Probiotics in man and animals. J Appl Bacteriol (1989) 66(5):365–78. doi: 10.1111/j.1365-2672.1989.tb05105.x
5. Naidu A, Bidlack W, Clemens R. Probiotic spectra of lactic acid bacteria (LAB). Crit Rev Food Sci Nutr (1999) 39(1):13–126. doi: 10.1080/10408699991279187
6. Gram L, Ringø E. Prospects of fish probiotics. In Biology of Growing Animals, vol. 2, pp. 379–417. Amsterdam, The Netherlands: Elsevier. (2005). p. 3–504.
7. Food and Agricultural Organization (FAO), World Health Organization (WHO). Health and nutritional properties of probiotics in food including powder milk with live lactic acid bacteria. A joint FAO/WHO expert consultation. Cordoba, Argentina. Rome, Italy: FAO and WHO (2001). Available at: https://www.who.int/foodsafety/publications/fs_management/probiotics/en/index.html.
8. Kozasa M. Toyocerin (Bacillus toyoi) as growth promotor for animal feeding. Microbiol-Aliments-Nutr (1986) 4:121–35.
9. Moriarty D. Control of luminous Vibrio species in penaeid aquaculture ponds. Aquaculture (1998) 164(1-4):351–8. doi: 10.1016/S0044-8486(98)00199-9
10. Verschuere L, Rombaut G, Sorgeloos P, Verstraete W. Probiotic bacteria as biological control agents in aquaculture. Microbiol Mol Biol Rev (2000) 64(4):655–71. doi: 10.1128/mmbr.64.4.655-671.2000
11. Amenyogbe E, Chen G, Wang Z, Huang J, Huang B, Li H. The exploitation of probiotics, prebiotics and synbiotics in aquaculture: present study, limitations and future directions: a review. Aquac Int (2020) 28:1017–41. doi: 10.1007/s10499-020-00509-0
12. Ray AK. The advancement of probiotics research and its application in fish farming industries. Res Vet Sci (2017) 115:66–77. doi: 10.1016/j.rvsc.2017.01.016
13. Caipang C, Suharman I, Avillanosa A, Bargoyo V. Host-derived Probiotics for Finfish Aquaculture. In: IOP Conf Ser: Earth Environ Sci. Bristol, UK: IOP Publishing (2020). 430:012026. doi: 10.1088/1755-1315/430/1/012026
14. Chauhan A, Singh R. Probiotics in aquaculture: a promising emerging alternative approach. Symbiosis (2019) 77(2):99–113. doi: 10.1007/s13199-018-0580-1
15. Hoseinifar SH, Sun Y-Z, Wang A, Zhou Z. Probiotics as means of diseases control in aquaculture, a review of current knowledge and future perspectives. Front Microbiol (2018) 9:2429. doi: 10.3389/fmicb.2018.02429
16. Ringø E, Doan HV, Lee S, Song SK. Lactic acid bacteria in shellfish: possibilities and challenges. Rev Fish Sci Aquac (2020) 28(2):139–69. doi: 10.1080/23308249.2019.1683151
17. Zorriehzahra MJ, Delshad ST, Adel M, Tiwari R, Karthik K, Dhama K, et al. Probiotics as beneficial microbes in aquaculture: an update on their multiple modes of action: a review. Vet Q (2016) 36(4):228–41. doi: 10.1080/01652176.2016.1172132
18. Hasan KN, Banerjee G. Recent studies on probiotics as beneficial mediator in aquaculture: review. J Basic Appl Zool (2020) 81:53. doi: 10.1186/s41936-020-00190-y
19. Shefat SHT. Probiotic strains used in aquaculture. Int Res J Microbiol (2018) 7:43–55. doi: 10.14303/irjm.2018.023
20. Lazado CC, Caipang CMA, Estante EG. Prospects of host-associated microorganisms in fish and penaeids as probiotics with immunomodulatory functions. Fish Shellfish Immunol (2015) 45(1):2–12. doi: 10.1016/j.fsi.2015.02.023
21. Sharifuzzaman S, Austin B. Probiotics for disease control in aquaculture. In: Diagnosis and Control of Diseases of Fish and Shellfish. New Jersey (USA): John Wiley & Sons Inc (2017). p. 189–222. doi: 10.1002/9781119152125.ch8
22. Tapia-Paniagua S, Díaz-Rosales P, León-Rubio J, de La Banda IG, Lobo C, Alarcón F, et al. Use of the probiotic Shewanella putrefaciens Pdp11 on the culture of Senegalese sole (Solea senegalensis, Kaup 1858) and gilthead seabream (Sparus aurata L.). Aquac Int (2012) 20(6):1025–39. doi: 10.1007/s10499-012-9509-5
23. Gomez D, Sunyer JO, Salinas I. The mucosal immune system of fish: the evolution of tolerating commensals while fighting pathogens. Fish Shellfish Immunol (2013) 35(6):1729–39. doi: 10.1016/j.fsi.2013.09.032
24. Sharifuzzaman S, Al-Harbi A, Austin B. Characteristics of growth, digestive system functionality, and stress factors of rainbow trout fed probiotics Kocuria SM1 and Rhodococcus SM2. Aquaculture (2014) 418:55–61. doi: 10.1016/j.aquaculture.2013.10.006
25. Martínez-Cruz P, Ibáñez AL, Monroy-Hermosillo OA, Ramírez-Saad HC. Use of probiotics in aquaculture. ISRN Microbiol (2012) 916845:1–13. doi: 10.5402/2012/916845
26. Alonso S, Castro MC, Berdasco M, de la Banda IG, Moreno-Ventas X, de Rojas AH. Isolation and partial characterization of lactic acid bacteria from the gut microbiota of marine fishes for potential application as probiotics in aquaculture. Probiotics Antimicrob Proteins (2019) 11(2):569–79. doi: 10.1007/s12602-018-9439-2
27. Aly SM, Abd-El-Rahman AM, John G, Mohamed MF. Characterization of some bacteria isolated from Oreochromis niloticus and their potential use as probiotics. Aquaculture (2008) 277(1-2):1–6. doi: 10.1016/j.aquaculture.2008.02.021
28. Balcázar JL, Vendrell D, de Blas I, Ruiz-Zarzuela I, Muzquiz JL, Girones O. Characterization of probiotic properties of lactic acid bacteria isolated from intestinal microbiota of fish. Aquaculture (2008) 278(1-4):188–91. doi: 10.1016/j.aquaculture.2008.03.014
29. Chabrillón M, Arijo S, Díaz-Rosales P, Balebona MC, Moriñigo MA. Interference of Listonella anguillarum with potential probiotic microorganisms isolated from farmed gilthead seabream (Sparus aurata, L.). Aquac Res (2006) 37(1):78–86. doi: 10.1111/j.1365-2109.2005.01400.x
30. Gatesoupe FJ. The use of probiotics in aquaculture. Aquaculture (1999) 180(1-2):147–65. doi: 10.1016/S0044-8486(99)00187-8
31. Nikoskelainen S, Ouwehand A, Salminen S, Bylund G. Protection of rainbow trout (Oncorhynchus mykiss) from furunculosis by Lactobacillus rhamnosus. Aquaculture (2001) 198(3-4):229–36. doi: 10.1016/S0044-8486(01)00593-2
32. Muthukumar P, Kandeepan C. Isolation, identification and characterization of probiotic organisms from intestine of fresh water fishes. Int J Curr Microbiol Appl Sci (2015) 4:607–16.
33. Sica MG, Brugnoni LI, Marucci PL, Cubitto MA. Characterization of probiotic properties of lactic acid bacteria isolated from an estuarine environment for application in rainbow trout (Oncorhynchus mykiss, Walbaum) farming. Antonie Van Leeuwenhoek (2012) 101(4):869–79. doi: 10.1007/s10482-012-9703-5
34. Thankappan B, Ramesh D, Ramkumar S, Natarajaseenivasan K, Anbarasu K. Characterization of Bacillus spp. from the gastrointestinal tract of Labeo rohita—towards to identify novel probiotics against fish pathogens. App Biochem Biotechnol (2015) 175(1):340–53. doi: 10.1007/s12010-014-1270-y
35. Wanka KM, Damerau T, Costas B, Krueger A, Schulz C, Wuertz S. Isolation and characterization of native probiotics for fish farming. BMC Microbiol (2018) 18(1):119. doi: 10.1186/s12866-018-1260-2
36. Chythanya R, Karunasagar I, Karunasagar I. Inhibition of shrimp pathogenic vibrios by a marine Pseudomonas I-2 strain. Aquaculture (2002) 208(1-2):1–10. doi: 10.1016/S0044-8486(01)00714-1
37. Merrifield DL, Dimitroglou A, Foey A, Davies SJ, Baker RT, Bøgwald J, et al. The current status and future focus of probiotic and prebiotic applications for salmonids. Aquaculture (2010) 302(1-2):1–18. doi: 10.1016/j.aquaculture.2010.02.007
38. Balcázar JL, De Blas I, Ruiz-Zarzuela I, Vendrell D, Gironés O, Muzquiz JL. Enhancement of the immune response and protection induced by probiotic lactic acid bacteria against furunculosis in rainbow trout (Oncorhynchus mykiss). FEMS Immunol Med Microbiol (2007) 51(1):185–93. doi: 10.1111/j.1574-695X.2007.00294.x
39. Chahad Bourouni O, El Bour M, Mraouna R, Abdennaceur H, Boudabous A. Preliminary selection study of potential probiotic bacteria from aquacultural area in Tunisia. Ann Microbiol (2007) 57(2):185. doi: 10.1007/BF03175205
40. Medina M, Sotil G, Flores V, Fernández C, Sandoval N. In vitro assessment of some probiotic properties and inhibitory activity against Yersinia ruckeri of bacteria isolated from rainbow trout Oncorhynchus mykiss (Walbaum). Aquac Rep (2020) 18:100447. doi: 10.1016/j.aqrep.2020.100447
41. Hai NV. The use of probiotics in aquaculture. J Appl Microbiol (2015) 119(4):917–35. doi: 10.1111/jam.12886
42. Chen D-D, Yao Y-Y, Cui Z-W, Zhang X-Y, Guo X, Zhou Y-Y, et al. Comparative study of the immunoprotective effect of two grass carp-sourced Bacillus subtilis spore-based vaccines against grass carp reovirus. Aquaculture (2019) 504:88–95. doi: 10.1016/j.aquaculture.2019.01.055
43. Gao X-Y, Liu Y, Miao L-L, Li E-W, Hou T-T, Liu Z-P. Mechanism of anti-Vibrio activity of marine probiotic strain Bacillus pumilus H2, and characterization of the active substance. AMB Express (2017) 7(1):23. doi: 10.1186/s13568-017-0323-3
44. Muñoz-Atienza E, Gómez-Sala B, Araújo C, Campanero C, del Campo R, Hernández PE, et al. Antimicrobial activity, antibiotic susceptibility and virulence factors of lactic acid bacteria of aquatic origin intended for use as probiotics in aquaculture. BMC Microbiol (2013) 13(1):15. doi: 10.1186/1471-2180-13-15
45. Panigrahi A, Azad IS. Microbial intervention for better fish health in aquaculture: the Indian scenario. Fish Physiol Biochem (2007) 33(4):429–40. doi: 10.1007/s10695-007-9160-7
46. Servin AL. Antagonistic activities of lactobacilli and bifidobacteria against microbial pathogens. FEMS Microbiol Rev (2004) 28(4):405–40. doi: 10.1016/j.femsre.2004.01.003
47. Tinh NT, Dierckens K, Sorgeloos P, Bossier P. A review of the functionality of probiotics in the larviculture food chain. Mar Biotechnol (NY) (2008) 10(1):1–12. doi: 10.1007/s10126-007-9054-9
48. Vijayabaskar P, Somasundaram ST. Isolation of bacteriocin producing lactic acid bacteria from fish gut and probiotic activity against common fresh water fish pathogen Aeromonas hydrophila. Biotechnol (2008) 7(1):124–48. doi: 10.3923/biotech.2008.124.128
49. Xu HM, Rong YJ, Zhao MX, Song B, Chi ZM. Antibacterial activity of the lipopetides produced by Bacillus amyloliquefaciens M1 against multidrug-resistant Vibrio spp. isolated from diseased marine animals. App Microbiol Biotechnol (2014) 98(1):127–36. doi: 10.1007/s00253-013-5291-1
50. Zapata A, Lara-flores M. Antimicrobial activities of lactic acid bacteria strains isolated from Nile tilapia intestine (Oreochromis niloticus). J Biol Life Sci (2013) 4:164–71. doi: 10.5296/jbls.v4i1.2408
51. Gibson LF. Bacteriocin activity and probiotic activity of Aeromonas media. J App Microbiol (1999) 85(1):243–8. doi: 10.1111/j.1365-2672.1998.tb05304.x
52. Lategan MJ, Booth W, Shimmon R, Gibson LF. An inhibitory substance produced by Aeromonas media A199, an aquatic probiotic. Aquaculture (2006) 254(1):115–24. doi: 10.1016/j.aquaculture.2005.11.019
53. Faramarzi M, Kiaalvandi S, Iranshahi F. The effect of probiotics on growth performance and body composition of common carp (Cyprinus carpio). J Anim Vet Adv (2011) 10:2408–13. doi: 10.3923/javaa.2011.2408.2413
54. Ma Y-X, Li L-Y, Li M, Chen W, Bao P-Y, Yu Z-C, et al. Effects of dietary probiotic yeast on growth parameters in juvenile sea cucumber, Apostichopus japonicus. Aquaculture (2019) 499:203–11. doi: 10.1016/j.aquaculture.2018.09.043
55. Rengpipat S, Phianphak W, Piyatiratitivorakul S, Menasveta P. Effects of a probiotic bacterium on black tiger shrimp Penaeus monodon survival and growth. Aquaculture (1998) 167(3):301–13. doi: 10.1016/S0044-8486(98)00305-6
56. Ringø E, Zhou Z, Vecino JLG, Wadsworth S, Romero J, Krogdahl Å, et al. Effect of dietary components on the gut microbiota of aquatic animals. A never-ending story? Aquac Nutr (2016) 22(2):219–82. doi: 10.1111/anu.12346
57. Cairo G, Bernuzzi F, Recalcati S. A precious metal: iron, an essential nutrient for all cells. Genes Nutr (2006) 1(1):25–40. doi: 10.1007/BF02829934
58. Skaar EP. The battle for iron between bacterial pathogens and their vertebrate hosts. PloS Pathog (2010) 6(8):e1000949. doi: 10.1371/journal.ppat.1000949
59. Khan A, Singh P, Srivastava A. Synthesis, nature and utility of universal iron chelator – Siderophore: a review. Microbiol Res (2018) 212-213:103–11. doi: 10.1016/j.micres.2017.10.012
60. Parmanand BA, Kellingray L, Le Gall G, Basit AW, Fairweather-Tait S, Narbad A. A decrease in iron availability to human gut microbiome reduces the growth of potentially pathogenic gut bacteria; an in vitro colonic fermentation study. J Nutr Biochem (2019) 67:20–7. doi: 10.1016/j.nutbio.2019.01.010
61. Sugita H, Mizuki H, Itoi S. Diversity of siderophore-producing bacteria isolated from the intestinal tracts of fish along the Japanese coast. Aquac Res (2011) 43:481–8. doi: 10.1111/j.1365-2109.2011.02851.x
62. Smith P, Davey S. Evidence for the competitive exclusion of Aeromonas salmonicida from fish with stress-inducible furunculosis by a fluorescent pseudomonad. J Fish Dis (1993) 16(5):521–4. doi: 10.1111/j.1365-2761.1993.tb00888.x
63. Gram L, Melchiorsen J, Spanggaard B, Huber I, Nielsen TF. Inhibition of Vibrio anguillarum by Pseudomonas fluorescens AH2, a possible probiotic treatment of fish. App Environ Microbiol (1999) 65(3):969–73. doi: 10.1128/AEM.65.3.969-973.1999
64. Lazado C, Caipang CM, Rajan B, Brinchmann M, Kiron V. Characterization of GP21 and GP12: two potential probiotic bacteria isolated from the gastrointestinal tract of Atlantic cod. Probiotics Antimicrob Proteins (2010) 2:126–34. doi: 10.1007/s12602-010-9041-8
65. Gatesoupe F-J, Infante J-LZ, Cahu C, Quazuguel P. Early weaning of seabass larvae, Dicentrarchus labrax: the effect on microbiota, with particular attention to iron supply and exoenzymes. Aquaculture (1997) 158(1):117–27. doi: 10.1016/S0044-8486(97)00179-8
66. Li M, Xi B, Qin T, Chen K, Xie J. Isolation and characterization of AHL-degrading bacteria from fish and pond sediment. J Oceanol Limnol (2019) 37(4):1460–7. doi: 10.1007/s00343-019-8137-6
67. Adams CA. The probiotic paradox: live and dead cells are biological response modifiers. Nutr Res Rev (2010) 23(1):37–46. doi: 10.1017/S0954422410000090
69. Salminen S, Isolauri E, Salminen E. Clinical uses of probiotics for stabilizing the gut mucosal barrier: successful strains and future challenges. Antonie van Leeuwenhoek (1996) 70(2):347–58. doi: 10.1007/BF00395941
70. Lazado CC, Caipang CMA, Brinchmann MF, Kiron V. In vitro adherence of two candidate probiotics from Atlantic cod and their interference with the adhesion of two pathogenic bacteria. Vet Microbiol (2011) 148(2):252–9. doi: 10.1016/j.vetmic.2010.08.024
71. Pan X, Wu T, Zhang L, Song Z, Tang H, Zhao Z. In vitro evaluation on adherence and antimicrobial properties of a candidate probiotic Clostridium butyricum CB2 for farmed fish. J Appl Microbiol (2008) 105(5):1623–9. doi: 10.1111/j.1365-2672.2008.03885.x
72. Vine NG, Leukes WD, Kaiser H, Daya S, Baxter J, Hecht T. Competition for attachment of aquaculture candidate probiotic and pathogenic bacteria on fish intestinal mucus. J Fish Dis (2004) 27(6):319–26. doi: 10.1111/j.1365-2761.2004.00542.x
73. Chabrillón M, Arijo S, Díaz-Rosales P, Balebona MC, Moriñigo MA. Interference of Listonella anguillarum with potential probiotic microorganisms isolated from farmed gilthead seabream (Sparus aurata, L.). Aquac Res (2005) 37(1):78–86. doi: 10.1111/j.1365-2109.2005.01400.x
74. Chabrillón M, Rico RM, Arijo S, Díaz-Rosales P, Balebona MC, Moriñigo MA. Interactions of microorganisms isolated from gilthead sea bream, Sparus aurata L., on Vibrio harveyi, a pathogen of farmed Senegalese sole, Solea senegalensis (Kaup). J Fish Dis (2005) 28(9):531–7. doi: 10.1111/j.1365-2761.2005.00657.x
75. Divya KR, Isamma A, Ramasubramanian V, Sureshkumar S, Arunjith TS. Colonization of probiotic bacteria and its impact on ornamental fish Puntius conchonius. J Environ Biol (2012) 33(3):551–5.
76. Brown M. Modes of Action of Probiotics: Recent Developments. J Anim Vet Adv (2011) 10:1895–900. doi: 10.3923/javaa.2011.1895.1900
77. Miller MB, Bassler BL. Quorum sensing in bacteria. Ann Rev Microbiol (2001) 55(1):165–99. doi: 10.1146/annurev.micro.55.1.165
78. Defoirdt T. Virulence mechanisms of bacterial aquaculture pathogens and antivirulence therapy for aquaculture. Rev Aquac (2014) 6(2):100–14. doi: 10.1111/raq.12030
79. Chu W, Zhou S, Zhu W, Zhuang X. Quorum quenching bacteria Bacillus sp. QSI-1 protect zebrafish (Danio rerio) from Aeromonas hydrophila infection. Sci Rep (2014) 4:5446. doi: 10.1038/srep05446
80. Fuqua WC, Winans SC, Greenberg EP. Quorum sensing in bacteria: the LuxR-LuxI family of cell density-responsive transcriptional regulators. J Bacteriol (1994) 176(2):269. doi: 10.1128/jb.176.2.269-275.1994
81. Gram L, Ravn L, Rasch M, Bruhn JB, Christensen AB, Givskov M. Food spoilage—interactions between food spoilage bacteria. Int J Food Microbiol (2002) 78(1-2):79–97. doi: 10.1016/s0168-1605(02)00233-7
82. Whitehead NA, Barnard AM, Slater H, Simpson NJ, Salmond GP. Quorum-sensing in Gram-negative bacteria. FEMS Microbiol Rev (2001) 25(4):365–404. doi: 10.1111/j.1574-6976.2001.tb00583.x
83. Winson MK, Camara M, Latifi A, Foglino M, Chhabra SR, Daykin M, et al. Multiple N-acyl-L-homoserine lactone signal molecules regulate production of virulence determinants and secondary metabolites in Pseudomonas aeruginosa. Proc Natl Acad Sci USA (1995) 92(20):9427–31. doi: 10.1073/pnas.92.20.9427
84. Chu W, Lu F, Zhu W, Kang C. Isolation and characterization of new potential probiotic bacteria based on quorum-sensing system. J App Microbiol (2011) 110(1):202–8. doi: 10.1111/j.1365-2672.2010.04872.x
85. Defoirdt T, Boon N, Bossier P, Verstraete W. Disruption of bacterial quorum sensing: an unexplored strategy to fight infections in aquaculture. Aquaculture (2004) 240(1-4):69–88. doi: 10.1016/j.aquaculture.2004.06.031
86. Givskov M, de Nys R, Manefield M, Gram L, Maximilien R, Eberl L, et al. Eukaryotic interference with homoserine lactone-mediated prokaryotic signalling. J Bacteriol (1996) 178(22):6618–22. doi: 10.1128/jb.178.22.6618-6622.1996
87. Roy V, Adams BL, Bentley WE. Developing next generation antimicrobials by intercepting AI-2 mediated quorum sensing. Enzyme Microb Technol (2011) 49(2):113–23. doi: 10.1016/j.enzmictec.2011.06.001
88. Dong Y-H, Wang L-H, Zhang L-H. Quorum-quenching microbial infections: mechanisms and implications. Philos Trans R Soc Lond B Biol Sci (2007) 362(1483):1201–11. doi: 10.1098/rstb.2007.2045
89. Kalia VC. Quorum sensing inhibitors: an overview. Biotechnol Adv (2013) 31(2):224–45. doi: 10.1016/j.biotechadv.2012.10.004
90. Cam DTV, Nhan DT, Ceuppens S, Hao NV, Dierckens K, Wille M, et al. Effect of N-acyl homoserine lactone-degrading enrichment cultures on Macrobrachium rosenbergii larviculture. Aquaculture (2009) 294(1):5–13. doi: 10.1016/j.aquaculture.2009.05.015
91. Defoirdt T, Boon N, Sorgeloos P, Verstraete W, Bossier P. Alternatives to antibiotics to control bacterial infections: luminescent vibriosis in aquaculture as an example. Trends Biotechnol (2007) 25(10):472–9. doi: 10.1016/j.tibtech.2007.08.001
92. Vadassery DH, Pillai D. Quorum quenching potential of Enterococcus faecium QQ12 isolated from gastrointestinal tract of Oreochromis niloticus and its application as a probiotic for the control of Aeromonas hydrophila infection in goldfish Carassius auratus (Linnaeus 1758). Braz J Microbiol (2020) 51(3):1333–43. doi: 10.1007/s42770-020-00230-3
93. Zhang Y, Liu J, Tang K, Yu M, Coenye T, Zhang X-H. Genome analysis of Flaviramulus ichthyoenteri Th78 T in the family Flavobacteriaceae: insights into its quorum quenching property and potential roles in fish intestine. BMC Genomics (2015) 16(1):38. doi: 10.1186/s12864-015-1275-0
94. Manefield M, de Nys R, Naresh K, Roger R, Givskov M, Peter S, et al. Evidence that halogenated furanones from Delisea pulchra inhibit acylated homoserine lactone (AHL)-mediated gene expression by displacing the AHL signal from its receptor protein. Microbiology (1999) 145(2):283–91. doi: 10.1099/13500872-145-2-283
95. Defoirdt T, Crab R, Wood TK, Sorgeloos P, Verstraete W, Bossier P. Quorum sensing-disrupting brominated furanones protect the gnotobiotic brine shrimp Artemia franciscana from pathogenic Vibrio harveyi, Vibrio campbellii, and Vibrio parahaemolyticus isolates. Appl Environ Microbiol (2006) 72(9):6419–23. doi: 10.1128/AEM.00753-06
96. Rasch M, Buch C, Austin B, Slierendrecht WJ, Ekmann KS, Larsen JL, et al. An inhibitor of bacterial quorum sensing reduces mortalities caused by vibriosis in rainbow trout (Oncorhynchus mykiss, Walbaum). Syst Appl Microbiol (2004) 27(3):350–9. doi: 10.1078/0723-2020-00268
97. Zhou S, Zhang A, Yin H, Chu W. Bacillus sp. QSI-1 modulate quorum sensing signals reduce Aeromonas hydrophila level and alter gut microbial community structure in fish. Front Cell Infect Microbiol (2016) 6:184. doi: 10.3389/fcimb.2016.00184
98. Ren Y, Li S, Wu Z, Zhou C, Zhang D, Chen X. The influences of Bacillus subtilis on the virulence of Aeromonas hydrophila and expression of luxS gene of both bacteria under co-cultivation. Curr Microbiol (2017) 74(6):718–24. doi: 10.1007/s00284-017-1236-8
99. Chen B, Peng M, Tong W, Zhang Q, Song Z. The quorum quenching bacterium Bacillus licheniformis T-1 protects zebrafish against Aeromonas hydrophila infection. Probiotics Antimicrob Proteins (2020) 12(1):160–71. doi: 10.1007/s12602-018-9495-7
100. Torabi Delshad S, Soltanian S, Sharifiyazdi H, Haghkhah M, Bossier P. Identification of N-acyl homoserine lactone-degrading bacteria isolated from rainbow trout (Oncorhynchus mykiss). J Appl Microbiol (2018) 125(2):356–69. doi: 10.1111/jam.13891
101. Ghanei-Motlagh R, Mohammadian T, Gharibi D, Menanteau-Ledouble S, Mahmoudi E, Khosravi M, et al. Quorum quenching properties and probiotic potentials of intestinal associated bacteria in Asian sea bass Lates calcarifer. Mar Drugs (2020) 18(1):23. doi: 10.3390/md18010023
102. Lazado CC, Caipang CMA. Mucosal immunity and probiotics in fish. Fish Shellfish Immunol (2014) 39(1):78–89. doi: 10.1016/j.fsi.2014.04.015
103. Azad MAK, Sarker M, Wan D. Immunomodulatory effects of probiotics on cytokine profiles. BioMed Res Int (2018) 8063647:10. doi: 10.1155/2018/8063647
104. Savan R, Sakai M. Genomics of fish cytokines. Comp Biochem Physiol Part D Genomics Proteomics (2006) 1(1):89–101. doi: 10.1016/j.cbd.2005.08.005
105. Salinas I, Zhang Y-A, Sunyer JO. Mucosal immunoglobulins and B cells of teleost fish. Dev Comp Immunol (2011) 35(12):1346–65. doi: 10.1016/j.dci.2011.11.009
106. Rombout JHWM, Abelli L, Picchietti S, Scapigliati G, Kiron V. Teleost intestinal immunology. Fish Shellfish Immunol (2011) 31(5):616–26. doi: 10.1016/j.fsi.2010.09.001
107. Salinas I, Parra D. Fish mucosal immunity: intestine. In: Mucosal Health in Aquaculture. Cambridge, Massachusetts (USA): Academic Press (2015). p. 135–70. doi: 10.1016/B978-0-12-417186-2.00006-6
108. Ringø E, Van Doan H, Lee SH, Soltani M, Hoseinifar SH, Harikrishnan R, et al. Probiotics, lactic acid bacteria and bacilli: interesting supplementation for aquaculture. J Appl Microbiol (2020) 129(1):116–36. doi: 10.1111/jam.14628
109. Soltani M, Ghosh K, Hoseinifar SH, Kumar V, Lymbery AJ, Roy S, et al. Genus Bacillus, promising probiotics in aquaculture: aquatic animal origin, bio-active components, bioremediation and efficacy in fish and shellfish. Rev Fish Sci Aquac (2019) 27(3):331–79. doi: 10.1080/23308249.2019.1597010
110. Pirarat N, Pinpimai K, Endo M, Katagiri T, Ponpornpisit A, Chansue N, et al. Modulation of intestinal morphology and immunity in Nile tilapia (Oreochromis niloticus) by Lactobacillus rhamnosus GG. Res Vet Sci (2011) 91(3):e92–7. doi: 10.1016/j.rvsc.2011.02.014
111. Balcázar JL, Vendrell D, de Blas I, Ruiz-Zarzuela I, Gironés O, Muzquiz JL. Immune modulation by probiotic strains: Quantification of phagocytosis of Aeromonas salmonicida by leukocytes isolated from gut of rainbow trout (Oncorhynchus mykiss) using a radiolabelling assay. Comp Immunol Microbiol Infect Dis (2006) 29(5):335–43. doi: 10.1016/j.cimid.2006.09.004
112. Picchietti S, Mazzini M, Taddei AR, Renna R, Fausto AM, Mulero V, et al. Effects of administration of probiotic strains on GALT of larval gilthead seabream: Immunohistochemical and ultrastructural studies. Fish Shellfish Immunol (2007) 22(1):57–67. doi: 10.1016/j.fsi.2006.03.009
113. Picchietti S, Fausto AM, Randelli E, Carnevali O, Taddei AR, Buonocore F, et al. Early treatment with Lactobacillus delbrueckii strain induces an increase in intestinal T-cells and granulocytes and modulates immune-related genes of larval Dicentrarchus labrax (L.). Fish Shellfish Immunol (2009) 26(3):368–76. doi: 10.1016/j.fsi.2008.10.008
114. Salinas I, Abelli L, Bertoni F, Picchietti S, Roque A, Furones D, et al. Monospecies and multispecies probiotic formulations produce different systemic and local immunostimulatory effects in the gilthead seabream (Sparus aurata L.). Fish Shellfish Immunol (2008) 25(1):114–23. doi: 10.1016/j.fsi.2008.03.011
115. Shabirah A, Rosidah R, Mulyani Y, Lili W. Effect of types isolated lactic acid bacteria on hematocrit and differential leukocytes fingerling common carp (Cyprinus carpio L.) infected with Aeromonas hydrophila bacteria. World News Nat Sci (2019) 24:22–35.
116. Mohammadian T, Nasirpour M, Tabandeh MR, Heidary AA, Ghanei-Motlagh R, Hosseini SS. Administrations of autochthonous probiotics altered juvenile rainbow trout Oncorhynchus mykiss health status, growth performance and resistance to Lactococcus garvieae, an experimental infection. Fish Shellfish Immunol (2019) 86:269–79. doi: 10.1016/j.fsi.2018.11.052
117. Xia Y, Lu M, Chen G, Cao J, Gao F, Wang M, et al. Effects of dietary Lactobacillus rhamnosus JCM1136 and Lactococcus lactis subsp. lactis JCM5805 on the growth, intestinal microbiota, morphology, immune response and disease resistance of juvenile Nile tilapia, Oreochromis niloticus. Fish Shellfish Immunol (2018) 76:368–79. doi: 10.1016/j.fsi.2018.03.020
118. Lescheid DW. Probiotics as regulators of inflammation: a review. Funct Food Health Dis (2014) 4(7):299–311. doi: 10.31989/ffhd.v4i7.2
119. Di J, Chu Z, Zhang S, Huang J, Du H, Wei Q. Evaluation of the potential probiotic Bacillus subtilis isolated from two ancient sturgeons on growth performance, serum immunity and disease resistance of Acipenser dabryanus. Fish Shellfish Immunol (2019) 93:711–9. doi: 10.1016/j.fsi.2019.08.020
120. Li J, Wu Z-B, Zhang Z, Zha J-W, Qu S-Y, Qi X-Z, et al. Effects of potential probiotic Bacillus velezensis K2 on growth, immunity and resistance to Vibrio harveyi infection of hybrid grouper (Epinephelus lanceolatus♂ × E. fuscoguttatus♀). Fish Shellfish Immunol (2019) 93:1047–55. doi: 10.1016/j.fsi.2019.08.047
121. Soltani M, Kane A, Taheri-Mirghaed A, Pakzad K, Hosseini-Shekarabi P. Effect of the probiotic, Lactobacillus plantarum on growth performance and haematological indices of rainbow trout (Oncorhynchus mykiss) immunized with bivalent streptococcosis/lactococcosis vaccine. Iran J Fish Sci (2019) 18(2):283–95. doi: 10.22092/ijfs.2018.117757
122. Cerezuela R, Guardiola FA, Cuesta A, Esteban MA. Enrichment of gilthead seabream (Sparus aurata L.) diet with palm fruit extract andn probiotics: effects on skin mucosal immunity. Fish Shellfish Immunol (2016) 49:100–9. doi: 10.1016/j.fsi.2015.12.028
123. Van Doan H, Hoseinifar SH, Tapingkae W, Seel-Audom M, Jaturasitha S, Dawood MA, et al. Boosted growth performance, mucosal and serum immunity, and disease resistance Nile tilapia (Oreochromis niloticus) fingerlings using corncob-derived xylooligosaccharide and Lactobacillus plantarum CR1T5. Probiotics Antimicrob Proteins (2020) 12(2):400–11. doi: 10.1007/s12602-019-09554-5
124. Gobi N, Vaseeharan B, Chen J-C, Rekha R, Vijayakumar S, Anjugam M, et al. Dietary supplementation of probiotic Bacillus licheniformis Dahb1 improves growth performance, mucus and serum immune parameters, antioxidant enzyme activity as well as resistance against Aeromonas hydrophila in tilapia Oreochromis mossambicus. Fish Shellfish Immunol (2018) 74:501–8. doi: 10.1016/j.fsi.2017.12.066
125. Kuebutornye FKA, Wang Z, Lu Y, Abarike ED, Sakyi MW, Li Y, et al. Effects of three host-associated Bacillus species on mucosal immunity and gut health of Nile tilapia, Oreochromis niloticus and its resistance against Aeromonas hydrophila infection. Fish Shellfish Immunol (2020) 97:83–95. doi: 10.1016/j.fsi.2019.12.046
126. Jiang Y, Zhou S, Chu W. The effects of dietary Bacillus cereus QSI-1 on skin mucus proteins profile and immune response in Crucian Carp (Carassius auratus). Fish Shellfish Immunol (2019) 89:319–25. doi: 10.1016/j.fsi.2019.04.014
127. Hosseini M, Miandare HK, Hoseinifar SH, Yarahmadi P. Dietary Lactobacillus acidophilus modulated skin mucus protein profile, immune and appetite genes expression in gold fish (Carassius auratus gibelio). Fish Shellfish Immunol (2016) 59:149–54. doi: 10.1016/j.fsi.2016.10.026
128. Zhang DX, Kang YH, Zhan S, Zhao ZL, Jin SN, Chen C, et al. Effect of Bacillus velezensis on Aeromonas veronii-induced intestinal mucosal barrier function damage and inflammation in crucian carp (Carassius auratus). Front Microbiol (2019) 15:2663(10). doi: 10.3389/fmicb.2019.02663
129. Nayak SK. Probiotics and immunity: a fish perspective. Fish Shellfish Immunol (2010) 29(1):2–14. doi: 10.1016/j.fsi.2010.02.017
130. Gómez GD, Balcázar JL. A review on the interactions between gut microbiota and innate immunity of fish. FEMS Immunol Med Microbiol (2008) 52(2):145–54. doi: 10.111/j.1574-695X.2007.00343.x
131. Dawood MAO, Abo-Al-Ela HG, Hasan MT. Modulation of transcriptomic profile in aquatic animals: probiotics, prebiotics and symbiotic scenarios. Fish Shellfish Immunol (2020) 97:268–82. doi: 10.1016/j.fsi.2019.12.054
132. Alarcón F, Martinez T, Díaz M, Moyano F. Characterization of digestive carbohydrase activity in the gilthead seabream (Sparus aurata). Hydrobiologia (2001) 445(1-3):199–204. doi: 10.1023/A:1017521900442
133. German DP, Horn MH, Gawlicka A. Digestive enzyme activities in herbivorous and carnivorous prickleback fishes (Teleostei: Stichaeidae): ontogenetic, dietary, and phylogenetic effects. Physiol Biochem Zool (2004) 77(5):789–804. doi: 10.1086/422228
134. Krogdahl Å, Hemre GI, Mommsen T. Carbohydrates in fish nutrition: digestion and absorption in postlarval stages. Aquac Nutr (2005) 11(2):103–22. doi: 10.1111/j.1365-2095.2004.00327.x
135. Ray A, Ghosh K, Ringø E. Enzyme-producing bacteria isolated from fish gut: a review. Aquac Nutr (2012) 18(5):465–92. doi: 10.1111/j.1365-2095.2012.00943.x
136. Banerjee SP, Dora KC, Chowdhury S. Detection, partial purification and characterization of bacteriocin produced by Lactobacillus brevis FPTLB3 isolated from freshwater fish. J Food Sci Technol (2013) 50(1):17–25. doi: 10.1007/s13197-011-0240-4
137. Dawood MA, Magouz FI, Salem MF, Abdel-Daim HA. Modulation of digestive enzyme activity, blood health, oxidative responses and growth-related gene expression in GIFT by heat-killed Lactobacillus plantarum (L-137). Aquaculture (2019) 505:127–36. doi: 10.1016/j.aquaculture.2019.02.053
138. Jang WJ, Lee JM, Hasan MT, Lee B-J, Lim SG, Kong I-S. Effects of probiotic supplementation of a plant-based protein diet on intestinal microbial diversity, digestive enzyme activity, intestinal structure, and immunity in olive flounder (Paralichthys olivaceus). Fish Shellfish Immunol (2019) 92:719–27. doi: 10.1016/j.fsi.2019.06.056
139. Mohammadian T, Nasirpour M, Tabandeh MR, Mesbah M. Synbiotic effects of β-glucan, mannan oligosaccharide and Lactobacillus casei on growth performance, intestine enzymes activities, immune-hematological parameters and immune-related gene expression in common carp, Cyprinus carpio: an experimental infection with Aeromonas hydrophila. Aquaculture (2019) 511:634197. doi: 10.1016/j.aquaculture.2019.06.011
140. Mohammadian T, Nasirpour M, Tabandeh MR, Heidary AA, Ghanei-Motlagh R, Hosseini SS. Administrations of autochthonous probiotics altered juvenile rainbow trout Oncorhynchus mykiss health status, growth performance and resistance to Lactococcus garvieae, an experimental infection. Fish Shellfish Immunol (2019) 86:269–79. doi: 10.1016/j.fsi.2018.11.052
141. Tarkhani R, Imani A, Hoseinifar SH, Moghanlou KS, Manaffar R. The effects of host-associated Enterococcus faecium CGMCC1.2136 on serum immune parameters, digestive enzymes activity and growth performance of the Caspian roach (Rutilus rutilus caspicus) fingerlings. Aquaculture (2020) 519:734741. doi: 10.1016/j.aquaculture.2019.734741
142. Sugita H, Miyajima C, Deguchi Y. The vitamin B12-producing ability of the intestinal microflora of freshwater fish. Aquaculture (1991) 92:267–76. doi: 10.1016/0044-8486(91)90028-6
143. Sugita H, Takahashi J, Deguchi Y. Production and consumption of biotin by the intestinal microflora of cultured freshwater fishes. Biosci Biotechnol Biochem (1992) 56(10):1678–9. doi: 10.1271/bbb.56.1678
144. Navarrete P, Tovar-Ramírez D. Use of yeasts as probiotics in fish aquaculture. In: Hernandez-Vergara MP, Perez-Castro CI, editors. Sustainable Aquaculture Techniques 1. London, UK: IntechOpen (2014). p. 135–72. doi: 10.5772/57196
145. Wang X, Li H, Zhang X, Li Y, Ji W, Xu H. Microbial flora in the digestive tract of adult penaeid shrimp (Penaeus chinensis). J Ocean Univ Qingdao (2000) 30:493–8.
146. Nath S, Matozzo V, Bhandari D, Faggio C. Growth and liver histology of Channa punctatus exposed to a common biofertilizer. Nat Prod Res (2019) 33(11):1591–8. doi: 10.1080/14786419.2018.1428586
147. Hamdan AM, El-Sayed AFM, Mahmoud MM. Effects of a novel marine probiotic, Lactobacillus plantarum AH 78, on growth performance and immune response of Nile tilapia (Oreochromis niloticus). J Appl Microbiol (2016) 120(4):1061–73. doi: 10.1111/jam.13081
148. Soltani M, Abdy E, Alishahi M, Mirghaed AT, Hosseini-Shekarabi P. Growth performance, immune-physiological variables and disease resistance of common carp (Cyprinus carpio) orally subjected to different concentrations of Lactobacillus plantarum. Aquac Int (2017) 25(5):1913–33. doi: 10.1007/s10499-017-0164-8
149. Yu L, Zhai Q, Zhu J, Zhang C, Li T, Liu X, et al. Dietary Lactobacillus plantarum supplementation enhances growth performance and alleviates aluminum toxicity in tilapia. Ecotoxicol Environ Saf (2017) 143:307–14. doi: 10.1016/j.ecoenv.2017.05.023
150. Zhai Q, Wang H, Tian F, Zhao J, Zhang H, Chen W. Dietary Lactobacillus plantarum supplementation decreases tissue lead accumulation and alleviates lead toxicity in Nile tilapia (Oreochromis niloticus). Aquac Res (2017) 48(9):5094–103. doi: 10.1111/are.13326
151. Alishahi M, Tulaby Dezfuly Z, Mohammadian T, Mesbah M. Effects of two probiotics, Lactobacillus plantarum and Lactobacillus bulgaricus on growth performance and intestinal lactic acid bacteria of Cyprinus Carpio. Iran J Vet Med (2018) 12(3):207–18. doi: 10.22059/IJVM.2018.235444.1004816
152. Van Doan H, Hoseinifar SH, Dawood MAO, Chitmanat C, Tayyamath K. Effects of Cordyceps militaris spent mushroom substrate and Lactobacillus plantarum on mucosal, serum immunology and growth performance of Nile tilapia (Oreochromis niloticus). Fish Shellfish Immunol (2017) 70:87–94. doi: 10.1016/j.aquaculture.2018.03.019
153. Van Doan H, Doolgindachbaporn S, Suksri A. Effect of Lactobacillus plantarum and Jerusalem artichoke (Helianthus tuberosus) on growth performance, immunity and disease resistance of Pangasius catfish (Pangasius bocourti, Sauvage 1880). Aquac Nutr (2016) 22(2):444–56. doi: 10.1111/anu.12263
154. Van Doan H, Hoseinifar SH, Khanongnuch C, Kanpiengjai A, Unban K, Srichaiyo S. Host-associated probiotics boosted mucosal and serum immunity, disease resistance and growth performance of Nile tilapia (Oreochromis niloticus). Aquaculture (2018) 491:94–100. doi: 10.1016/j.aquaculture.2018.03.019
155. Feng J, Chang X, Zhang Y, Yan X, Zhang J, Nie G. Effects of Lactococcus lactis from Cyprinus carpio L. as probiotics on growth performance, innate immune response and disease resistance against Aeromonas hydrophila. Fish Shellfish Immunol (2019) 93:73–81. doi: 10.1016/j.fsi.2019.07.028
156. Nguyen TL, Park C-I, Kim D-H. Improved growth rate and disease resistance in olive flounder, Paralichthys olivaceus, by probiotic Lactococcus lactis WFLU12 isolated from wild marine fish. Aquaculture (2017) 471:113–20. doi: 10.1016/j.aquaculture.2017.01.008
157. Nguyen TL, Chun W-K, Kim A, Kim N, Roh HJ, Lee Y, et al. Dietary probiotic effect of Lactococcus lactis WFLU12 on low-molecular-weight metabolites and growth of olive flounder (Paralichythys olivaceus). Front Microbiol (2018) 9:2059. doi: 10.3389/fmicb.2018.02059
158. Sun Y, He M, Cao Z, Xie Z, Liu C, Wang S, et al. Effects of dietary administration of Lactococcus lactis HNL12 on growth, innate immune response, and disease resistance of humpback grouper (Cromileptes altivelis). Fish Shellfish Immunol (2018) 82:296–303. doi: 10.1016/j.fsi.2018.08.039
159. Zhang C-N, Zhang J-L, Guan W-C, Zhang X-F, Guan S-H, Zeng Q-H, et al. Effects of Lactobacillus delbrueckii on immune response, disease resistance against Aeromonas hydrophila, antioxidant capability and growth performance of Cyprinus carpio Huanghe var. Fish Shellfish Immunol (2017) 68:84–91. doi: 10.1016/j.fsi.2017.07.012
160. Sewaka M, Trullas C, Chotiko A, Rodkhum C, Chansue N, Boonanuntanasarn S, et al. Efficacy of synbiotic Jerusalem artichoke and Lactobacillus rhamnosus GG-supplemented diets on growth performance, serum biochemical parameters, intestinal morphology, immune parameters and protection against Aeromonas veronii in juvenile red tilapia (Oreochromis spp.). Fish Shellfish Immunol (2019) 86:260–8. doi: 10.1016/j.fsi.2018.11.026
161. Ashouri G, Soofiani NM, Hoseinifar SH, Jalali SAH, Morshedi V, Van Doan H, et al. Combined effects of dietary low molecular weight sodium alginate and Pediococcus acidilactici MA18/5M on growth performance, haematological and innate immune responses of Asian sea bass (Lates calcalifer) juveniles. Fish Shellfish Immunol (2018) 79:34–41. doi: 10.1016/j.fsi.2018.05.009
162. Rahimnejad S, Guardiola FA, Leclercq E, Ángeles Esteban M, Castex M, Sotoudeh E, et al. Effects of dietary supplementation with Pediococcus acidilactici MA18/5M, galactooligosaccharide and their synbiotic on growth, innate immunity and disease resistance of rockfish (Sebastes schlegeli). Aquaculture (2018) 482:36–44. doi: 10.1016/j.aquaculture.2017.09.020
163. Baños A, Ariza JJ, Nuñez C, Gil-Martínez L, García-López JD, Martínez-Bueno M, et al. Effects of Enterococcus faecalis UGRA10 and the enterocin AS-48 against the fish pathogen Lactococcus garvieae. Studies in vitro and in vivo. Food Microbiol (2019) 77:69–77. doi: 10.1016/j.fm.2018.08.002
164. Xie Z, Guo W, Zhou Y. Dietary administration of Bacillus subtilis HAINUP40 enhances growth, digestive enzyme activities, innate immune responses and disease resistance of tilapia, Oreochromis niloticus. Fish Shellfish Immunol (2017) 60:326–33. doi: 10.1016/j.fsi.2016.12.003
165. Lara-Flores M, Olvera-Novoa MA, Guzmán-Méndez BE, López-Madrid W. Use of the bacteria Streptococcus faecium and Lactobacillus acidophilus, and the yeast Saccharomyces cerevisiae as growth promoters in Nile tilapia (Oreochromis niloticus). Aquaculture (2003) 216(1-4):193–201. doi: 10.1016/S0044-8486(02)00277-6
166. Liu H, Wang S, Cai Y, Guo X, Cao Z, Zhang Y, et al. Dietary administration of Bacillus subtilis HAINUP40 enhances growth, digestive enzyme activities, innate immune responses and disease resistance of tilapia, Oreochromis niloticus. Fish Shellfish Immunol (2017) 60:326–33. doi: 10.1016/j.fsi.2016.12.003
167. Bisht A, Singh UP, Pandey N. Bacillus subtilis as a potent probiotic for enhancing growth in fingerlings of common carp (Cyprinus carpio Linnaeus). Indian J Fish (2012) 59(3):103–7.
168. Guo X, Chen D-D, Peng K-S, Cui Z-W, Zhang X-J, Li S, et al. Identification and characterization of Bacillus subtilis from grass carp (Ctenopharynodon idellus) for use as probiotic additives in aquatic feed. Fish Shellfish Immunol (2016) 52:74–84. doi: 10.1016/j.fsi.2016.03.017
169. Abareethan M, Amsath A. Characterization and evaluation of probiotic fish feed. Int J Pure Appl Zool (2015) 3(2):148–53.
170. Giannenas I, Karamaligas I, Margaroni M, Pappas I, Mayer E, Encarnação P, et al. Effect of dietary incorporation of a multi-strain probiotic on growth performance and health status in rainbow trout (Oncorhynchus mykiss). Fish Physiol Biochem (2015) 41(1):119–28. doi: 10.1007/s10695-014-0010-0
171. Thy HTT, Tri NN, Quy OM, Fotedar R, Kannika K, Unajak S, et al. Effects of the dietary supplementation of mixed probiotic spores of Bacillus amyloliquefaciens 54A, and Bacillus pumilus 47B on growth, innate immunity and stress responses of striped catfish (Pangasianodon hypophthalmus). Fish Shellfish Immunol (2017) 60:391–9. doi: 10.1016/j.fsi.2016.11.016
172. Lin S, Mao S, Guan Y, Luo L, Luo L, Pan Y. Effects of dietary chitosan oligosaccharides and Bacillus coagulans on the growth, innate immunity and resistance of koi (Cyprinus carpio koi). Aquaculture (2012) 342:36–41. doi: 10.1016/j.aquaculture.2012.02.009
173. Han B, Long W-Q, He J-Y, Liu Y-J, Si Y-Q, Tian L-X. Effects of dietary Bacillus licheniformis on growth performance, immunological parameters, intestinal morphology and resistance of juvenile Nile tilapia (Oreochromis niloticus) to challenge infections. Fish Shellfish Immunol (2015) 46(2):225–31. doi: 10.1016/j.fsi.2015.06.018
174. Chang CI, Liu WY. An evaluation of two probiotic bacterial strains, Enterococcus faecium SF68 and Bacillus toyoi, for reducing edwardsiellosis in cultured European eel, Anguilla anguilla L. J Fish Dis (2002) 25:311–5. doi: 10.1046/j.1365-2761.2002.00365.x
175. Lee JS, Cheng H, Damte D, Lee SJ, Kim JC, Rhee MH, et al. Effects of dietary supplementation of Lactobacillus pentosus PL11 on the growth performance, immune and antioxidant systems of Japanese eel Anguilla japonica challenged with Edwardsiella tarda. Fish Shellfish Immunol (2013) 34(3):756–61. doi: 10.1016/j.fsi.2012.11.028
176. Abraham TJ, Mondal S, Babu CS. Effect of commercial aquaculture probiotic and fish gut antagonistic bacterial flora on the growth and disease resistance of ornamental fishes Carassius auratus and Xiphophorus helleri. J Fish Aquat Sci (2008) 25(1):27–30.
177. Zhou S, Xia Y, Zhu C, Chu W. Isolation of marine Bacillus sp. with antagonistic and organic-substances-degrading activities and its potential application as a fish probiotic. Mar Drugs (2018) 16(6):196. doi: 10.3390/md16060196
178. Meidong R, Doolgindachbaporn S, Jamjan W, Sakai K, Tashiro Y, Okugawa Y, et al. A novel probiotic Bacillus siamensis B44v isolated from Thai pickled vegetables (Phak-dong) for potential use as a feed supplement in aquaculture. J Gen Appl Microbiol (2017) 63(4):246–53. doi: 10.2323/jgam.2016.12.002
179. Al-Dohail MA, Hashim R, Aliyu-Paiko M. Evaluating the use of Lactobacillus acidophilus as a biocontrol agent against common pathogenic bacteria and the effects on the haematology parameters and histopathology in African catfish Clarias gariepinus juveniles. Aquac Res (2011) 42(2):196–209. doi: 10.1111/j.1365-2109.2010.02606.x
180. Bandyopadhyay P, Mohapatra PKD. Effect of a probiotic bacterium Bacillus circulans PB7 in the formulated diets: on growth, nutritional quality and immunity of Catla catla (Ham.). Fish Physiol Biochem (2009) 35(3):467–78. doi: 10.1007/s10695-008-9272-8
181. Wu ZQ, Jiang C, Ling F, Wang GX. Effects of dietary supplementation of intestinal autochthonous bacteria on the innate immunity and disease resistance of grass carp (Ctenopharyngodon idellus). Aquaculture (2015) 438:105–14. doi: 10.1016/j.aquaculture.2014.12.041
182. Gong L, He H, Li D, Cao L, Khan TA, Li Y, et al. A new isolate of Pediococcus pentosaceus (SL001) with antibacterial activity against fish pathogens and potency in facilitating the immunity and growth performance of grass carp. Front Microbiol (2019) 10:1384. doi: 10.3389/fmicb.2019.01384
183. Chi C, Jiang B, Yu XB, Liu TQ, Xia L, Wang GX. Effects of three strains of intestinal autochthonous bacteria and their extracellular products on the immune response and disease resistance of common carp, Cyprinus carpio. Fish Shellfish Immunol (2014) 36(1):9–18. doi: 10.1016/j.fsi.2013.10.003
184. Sorroza L, Padilla D, Acosta F, Román L, Grasso V, Vega J, et al. Characterization of the probiotic strain Vagococcus fluvialis in the protection of European sea bass (Dicentrarchus labrax) against vibriosis by Vibrio anguillarum. Vet Microbiol (2012) 155(2-4):369–73. doi: 10.1016/j.vetmic.2011.09.013
185. Sorroza L, Real F, Acosta F, Acosta B, Déniz S, Román L, et al. A probiotic potential of Enterococcus gallinarum against Vibrio anguillarum infection. Fish Pathol (2013) 48(1):9–12. doi: 10.3147/jsfp.48.9
186. Son VM, Chang CC, Wu MC, Guu YK, Chiu CH, Cheng W. Dietary administration of the probiotic, Lactobacillus plantarum, enhanced the growth, innate immune responses, and disease resistance of the grouper Epinephelus coioides. Fish Shellfish Immunol (2009) 26(5):691–8. doi: 10.1016/j.fsi.2009.02.018
187. Huang JB, Wu YC, Chi SC. Dietary supplementation of Pediococcus pentosaceus enhances innate immunity, physiological health and resistance to Vibrio anguillarum in orange-spotted grouper (Epinephelus coioides). Fish Shellfish Immunol (2014) 39(2):196–205. doi: 10.1016/j.fsi.2014.05.003
188. Chiu CH, Cheng CH, Gua WR, Guu YK, Cheng W. Dietary administration of the probiotic, Saccharomyces cerevisiae P13, enhanced the growth, innate immune responses, and disease resistance of the grouper, Epinephelus coioides. Fish Shellfish Immunol (2010) 29(6):1053–9. doi: 10.1016/j.fsi.2010.08.019
189. Gildberg A, Mikkelsen H, Sandaker E, Ringo E. Probiotic effect of lactic acid bacteria in the feed on growth and survival of fry of Atlantic cod (Gadus morhua). Hydrobiologia (1997) 352:279–85. doi: 10.1007/978-94-011-5234-1_27
190. Gildberg A, Mikkelsen H. Effect of supplementing the diet to Atlantic cod (Gadus morhua) fry with lactic acid bacteria and immuno-stimulating peptides during a challenge trial with Vibrio anguillarum. Aquaculture (1998) 167:103–13. doi: 10.1016/s0044-8486(98)00296-8
191. D’Alvise PW, Lillebø S, Prol-Garcia MJ, Wergeland HI, Nielsen KF, Bergh Ø, et al. Phaeobacter gallaeciensis reduces Vibrio anguillarum in cultures of microalgae and rotifers, and prevents vibriosis in cod larvae. PloS One (2012) 7(8):e43996. doi: 10.1371/journal.pone.0043996
192. Kumar R, Mukherjee SC, Prasad KP, Pal AK. Evaluation of Bacillus subtilis as a probiotic to Indian major carp Labeo rohita (Ham.). Aquac Res (2006) 37(12):1215–21. doi: 10.1111/j.1365-2109.2006.01551.x
193. Nayak SK, Swain P, Mukherjee SC. Effect of dietary supplementation of probiotic and vitamin C on the immune response of Indian major carp, Labeo rohita (Ham). Fish Shellfish Immunol (2007) 23:892–6. doi: 10.1016/j.fsi.2007.02.008
194. Kumar R, Mukherjee SC, Ranjan R, Nayak SK. Enhanced innate immune parameters in Labeo rohita (Ham.) following oral administration of Bacillus subtilis. Fish Shellfish Immunol (2008) 24(2):168–72. doi: 10.1016/j.fsi.2007.10.008
195. Giri SS, Sen SS, Sukumaran V. Effects of dietary supplementation of potential probiotic Pseudomonas aeruginosa VSG-2 on the innate immunity and disease resistance of tropical freshwater fish, Labeo rohita. Fish Shellfish Immunol (2012) 32(6):1135–40. doi: 10.1016/j.fsi.2012.03.019
196. Giri SS, Sukumaran V, Oviya M. Potential probiotic Lactobacillus plantarum VSG3 improves the growth, immunity, and disease resistance of tropical freshwater fish, Labeo rohita. Fish Shellfish Immunol (2013) 34(2):660–6. doi: 10.1016/j.fsi.2012.12.008
197. Ramesh D, Vinothkanna A, Rai AK, Vignesh VS. Isolation of potential probiotic Bacillus spp. and assessment of their subcellular components to induce immune responses in Labeo rohita against Aeromonas hydrophila. Fish Shellfish Immunol (2015) 45(2):268–76. doi: 10.1016/j.fsi.2015.04.018
198. Ai Q, Xu H, Mai K, Xu W, Wang J, Zhang W. Effects of dietary supplementation of Bacillus subtilis and fructooligosaccharide on growth performance, survival, non-specific immune response and disease resistance of juvenile large yellow croaker, Larimichthys crocea. Aquaculture (2011) 317(1-4):155–61. doi: 10.1016/j.aquaculture.2011.04.036
199. Lin HL, Shiu YL, Chiu CS, Huang SL, Liu CH. Screening probiotic candidates for a mixture of probiotics to enhance the growth performance, immunity, and disease resistance of Asian seabass, Lates calcarifer (Bloch), against Aeromonas hydrophila. Fish Shellfish Immunol (2017) 60:474–82. doi: 10.1016/j.fsi.2016.11.026
200. Pan X, Wu T, Song Z, Tang H, Zhao Z. Immune responses and enhanced disease resistance in Chinese drum, Miichthys miiuy (Basilewsky), after oral administration of live or dead cells of Clostridium butyrium CB2. J Fish Dis (2008) 31(9):679–86. doi: 10.1111/j.1365-2761.2008.00955.x
201. Reyes-Becerril M, Tovar-Ramírez D, Ascencio-Valle F, Civera-Cerecedo R, Gracia-López V, Barbosa-Solomieu V, et al. Effects of dietary supplementation with probiotic live yeast Debaryomyces hansenii on the immune and antioxidant systems of leopard grouper Mycteroperca rosacea infected with Aeromonas hydrophila. Aquac Res (2011) 42(11):1676–86. doi: 10.1111/j.1365-2109.2010.02762.x
202. Sakai M, Yoshida T, Atsuta S, Kobayashi M. Enhancement of resistance to vibriosis in rainbow trout, Oncorhynchus mykiss (Walbaum), by oral administration of Clostridium butyricum bacterin. J Fish Dis (1995) 18(2):187–90. doi: 10.1111/j.1365-2761.1995.tb00276.x
203. Robertson PAW, O’Dowd C, Burrells C, Williams P, Austin B. Use of Carnobacterium sp as a probiotic for Atlantic salmon (Salmo salar L.) and rainbow trout (Oncorhynchus mykiss, Walbaum). Aquaculture (2000) 185:235–43. doi: 10.1016/S0044-8486(99)00349-X
204. Spanggaard B, Huber I, Nielsen J, Sick EB, Pipper CB, Martinussen T, et al. The probiotic potential against vibriosis of the indigenous microflora of rainbow trout. Environ Microbiol (2001) 3:755–65. doi: 10.1046/j.1462-2920.2001.00240.x
205. Irianto A, Austin B. Use of probiotics to control furunculosis in rainbow trout, Oncorhynchus mykiss (Walbaum). J Fish Dis (2002) 25(6):333–42. doi: 10.1046/j.1365-2761.2002.00375.x
206. Irianto A, Austin B. Use of dead probiotic cells to control furunculosis in rainbow trout, Oncorhynchus mykiss (Walbaum). J Fish Dis (2003) 26(1):59–62. doi: 10.1046/j.1365-2761.2003.00414.x
207. Raida MK, Larsen JL, Nielsen ME, Buchmann K. Enhanced resistance of rainbow trout, Oncorhynchus mykiss (Walbaum), against Yersinia ruckeri challenge following oral administration of Bacillus subtilis and B. licheniformis (BioPlus2B). J Fish Dis (2003) 26(8):495–8. doi: 10.1046/j.1365-2761.2003.00480.x
208. Brunt J, Austin B. Use of a probiotic to control lactococcosis and streptococcosis in rainbow trout, Oncorhynchus mykiss (Walbaum). J Fish Dis (2005) 28:693–701. doi: 10.1111/j.1365-2761.2005.00672.x
209. Kim DH, Austin B. Innate immune responses in rainbow trout (Oncorhynchus mykiss, Walbaum) induced by probiotics. Fish Shellfish Immunol (2006) 21(5):513–24. doi: 10.1016/j.fsi.2006.02.007
210. Brunt J, Newaj-Fyzul A, Austin B. The development of probiotics for the control of multiple bacterial diseases of rainbow trout, Oncorhynchus mykiss (Walbaum). J Fish Dis (2007) 30(10):573–9. doi: 10.1111/j.1365-2761.2007.00836.x
211. Newaj-Fyzul A, Adesiyunz AA, Mutani A, Austin B. Bacillus subtilis AB1 controls Aeromonas infection in rainbow trout (Oncorhynchus mykiss, Walbaum). J Appl Microbiol (2007) 103:1699–706. doi: 10.1111/j.1365-2672.2007.03402.x
212. Pieters N, Brunt J, Austin B, Lyndon AR. Efficacy of in-feed probiotics against Aeromonas bestiarum and Ichthyophthirius multifiliis skin infections in rainbow trout (Oncorhynchus mykiss, Walbaum). J Appl Microbiol (2008) 105(3):723–32. doi: 10.1111/j.1365-2672.2008.03817.x
213. Capkin E, Altinok I. Effects of dietary probiotic supplementations on prevention/treatment of yersiniosis disease. J Appl Microbiol (2009) 106:1147–53. doi: 10.1111/j.1365-2672.2008.04080.x
214. Rodriguez-Estrada U, Satoh S, Haga Y, Fushimi H, Sweetman J. Effects of single and combined supplementation of Enterococcus faecalis, mannan oligosaccharide and polyhydrobutyric acid on growth performance and immune response of rainbow trout Oncorhynchus mykiss. Aquacult Sci (2009) 57:609–17. doi: 10.11233/AQUACULTURESCI.57.609
215. Sharifuzzaman SM, Austin B. Influence of probiotic feeding duration on disease resistance and immune parameters in rainbow trout. Fish Shellfish Immunol (2009) 27:440–5. doi: 10.1016/j.fsi.2009.06.010
216. Sharifuzzaman SM, Austin B. Kocuria SM1 controls vibriosis in rainbow trout (Oncorhynchus mykiss, Walbaum). . J Appl Microbiol (2010) 108(6):2162–70. doi: 10.1111/j.1365-2672.2009.04618.x
217. Burbank DR, Shah DH, LaPatra SE, Fornshell G, Cain KD. Enhanced resistance to coldwater disease following feeding of probiotic bacterial strains to rainbow trout (Oncorhynchus mykiss). Aquaculture (2011) 321(3-4):185–90. doi: 10.1016/j.aquaculture.2011.09.004
218. Korkea-Aho TL, Heikkinen J, Thompson KD, Von Wright A, Austin B. Pseudomonas sp. M174 inhibits the fish pathogen Flavobacterium psychrophilum. J Appl Microbiol (2011) 111(2):266–77. doi: 10.1111/j.1365-2672.2011.05044.x
219. Sharifuzzaman SM, Abbass A, Tinsley JW, Austin B. Subcellular components of probiotics Kocuria SM1 and Rhodococcus SM2 induce protective immunity in rainbow trout (Oncorhynchus mykiss, Walbaum) against Vibrio anguillarum. Fish Shellfish Immunol (2011) 30(1):347–53. doi: 10.1016/j.fsi.2010.11.005
220. Rodriguez-Estrada U, Satoh S, Haga Y, Fushimi H, Sweetman J. Effects of inactivated Enterococcus faecalis and mannan oligosaccharide and their combination on growth, immunity, and disease protection in rainbow trout. N Am J Aquac (2013) 75(3):416–28. doi: 10.1080/15222055.2013.799620
221. LaPatra SE, Fehringer TR, Cain KD. A probiotic Enterobacter sp. provides significant protection against Flavobacterium psychrophilum in rainbow trout (Oncorhynchus mykiss) after injection by two different routes. Aquaculture (2014) 433:361–6. doi: 10.1016/j.aquaculture.2014.06.022
222. Safari R, Adel M, Lazado CC, Caipang CMA, Dadar M. Host-derived probiotics Enterococcus casseliflavus improves resistance against Streptococcus iniae infection in rainbow trout (Oncorhynchus mykiss) via immunomodulation. Fish Shellfish Immunol (2016) 52:198–205. doi: 10.1016/j.fsi.2016.03.020
223. Harikrishnan R, Kim MC, Kim JS, Balasundaram C, Heo MS. Protective effect of herbal and probiotics enriched diet on haematological and immunity status of Oplegnathus fasciatus (Temminck & Schlegel) against Edwardsiella tarda. Fish Shellfish Immunol (2011) 30(3):886–93. doi: 10.1016/j.fsi.2011.01.013
224. Pirarat N, Kobayashi T, Katagiri T, Maita M, Endo M. Protective effects and mechanisms of a probiotic bacterium Lactobacillus rhamnosus against experimental Edwardsiella tarda infection in tilapia (Oreochromis niloticus). Vet Immunol Immunopathol (2006) 113(3-4):339–47. doi: 10.1016/j.vetimm.2006.06.003
225. Abdel-Tawwab M, Abdel-Rahman AM, Ismael NE. Evaluation of commercial live bakers’ yeast, Saccharomyces cerevisiae as a growth and immunity promoter for Fry Nile tilapia, Oreochromis niloticus (L.) challenged in situ with Aeromonas hydrophila. Aquaculture (2008) 280(1-4):185–9. doi: 10.1016/j.aquaculture.2008.03.055
226. Aly SM, Ahmed YAG, Ghareeb AAA, Mohamed MF. Studies on Bacillus subtilis and Lactobacillus acidophilus, as potential probiotics, on the immune response and resistance of Tilapia nilotica (Oreochromis niloticus) to challenge infections. Fish Shellfish Immunol (2008) 25:128–36. doi: 10.1016/j.fsi.2008.03.013
227. Aly SM, Mohamed MF, John G. Effect of probiotics on the survival, growth and challenge infection in Tilapia nilotica (Oreochromis niloticus). Aquac Res (2008) 39(6):647–56. doi: 10.1111/j.1365-2109.2008.01932.x
228. Abd El-Rhman AM, Khattab YA, Shalaby AM. Micrococcus luteus and Pseudomonas species as probiotics for promoting the growth performance and health of Nile tilapia, Oreochromis niloticus. Fish Shellfish Immunol (2009) 27(2):175–80. doi: 10.1016/j.fsi.2009.03.020
229. Liu W, Ren P, He S, Xu L, Yang Y, Gu Z, et al. Comparison of adhesive gut bacteria composition, immunity, and disease resistance in juvenile hybrid tilapia fed two different Lactobacillus strains. Fish Shellfish Immunol (2013) 35(1):54–62. doi: 10.1016/j.fsi.2013.04.010
230. Han B, Long WQ, He JY, Liu YJ, Si YQ, Tian LX. Effects of dietary Bacillus licheniformis on growth performance, immunological parameters, intestinal morphology and resistance of juvenile Nile tilapia (Oreochromis niloticus) to challenge infections. Fish Shellfish Immunol (2015) 46(2):225–31. doi: 10.1016/j.fsi.2015.06.018
231. Selim KM, Reda RM. Improvement of immunity and disease resistance in the Nile tilapia, Oreochromis niloticus, by dietary supplementation with Bacillus amyloliquefaciens. Fish Shellfish Immunol (2015) 44(2):496–503. doi: 10.1016/j.fsi.2015.03.004
232. Srisapoome P, Areechon N. Efficacy of viable Bacillus pumilus isolated from farmed fish on immune responses and increased disease resistance in Nile tilapia (Oreochromis niloticus): laboratory and on-farm trials. Fish Shellfish Immunol (2017) 67:199–210. doi: 10.1016/j.fsi.2017.06.018
233. Abdelfatah EN, Mahboub HHH. Studies on the effect of Lactococcus garvieae of dairy origin on both cheese and Nile tilapia (O. niloticus). Int J Vet Sci Med (2018) 6(2):201–7. doi: 10.1016/j.ijvsm.2018.11.002
234. Abou-El-Atta ME, Abdel-Tawwab M, Abdel-Razek N, Abdelhakim TM. Effects of dietary probiotic Lactobacillus plantarum and whey protein concentrate on the productive parameters, immunity response and susceptibility of Nile tilapia, Oreochromis niloticus (L.), to Aeromonas sobria infection. Aquac Nutr (2019) 25(6):1367–77. doi: 10.1111/anu.12957
235. Van Doan H, Hoseinifar SH, Naraballobh W, Jaturasitha S, Tongsiri S, Chitmanat C, et al. Dietary inclusion of orange peels derived pectin and Lactobacillus plantarum for Nile tilapia (Oreochromis niloticus) cultured under indoor biofloc systems. Aquaculture (2019) 508:98–105. doi: 10.1016/j.aquaculture.2019.03.067
236. Ng WK, Kim YC, Romano N, Koh CB, Yang SY. Effects of dietary probiotics on the growth and feeding efficiency of red hybrid tilapia, Oreochromis sp., and subsequent resistance to Streptococcus agalactiae. J Appl Aquac (2014) 26(1):22–31. doi: 10.1080/10454438.2013.874961
237. Meidong R, Khotchanalekha K, Doolgindachbaporn S, Nagasawa T, Nakao M, Sakai K, et al. Evaluation of probiotic Bacillus aerius B81e isolated from healthy hybrid catfish on growth, disease resistance and innate immunity of Pla-mong Pangasius bocourti. Fish Shellfish Immunol (2018) 73:1–10. doi: 10.1016/j.fsi.2017.11.032
238. Cha JH, Rahimnejad S, Yang SY, Kim KW, Lee KJ. Evaluations of Bacillus spp. as dietary additives on growth performance, innate immunity and disease resistance of olive flounder (Paralichthys olivaceus) against Streptococcus iniae and as water additives. Aquaculture (2013) 402:50–7. doi: 10.1016/j.aquaculture.2013.03.030
239. Kim D, Beck BR, Heo SB, Kim J, Kim HD, Lee SM, et al. Lactococcus lactis BFE920 activates the innate immune system of olive flounder (Paralichthys olivaceus), resulting in protection against Streptococcus iniae infection and enhancing feed efficiency and weight gain in large-scale field studies. Fish Shellfish Immunol (2013) 35(5):1585–90. doi: 10.1016/j.fsi.2013.09.008
240. Beck BR, Kim D, Jeon J, Lee SM, Kim HK, Kim OJ, et al. The effects of combined dietary probiotics Lactococcus lactis BFE920 and Lactobacillus plantarum FGL0001 on innate immunity and disease resistance in olive flounder (Paralichthys olivaceus). Fish Shellfish Immunol (2015) 42(1):177–83. doi: 10.1016/j.fsi.2014.10.035
241. Beck BR, Lee SH, Kim D, Park JH, Lee HK, Kwon SS, et al. A Lactococcus lactis BFE920 feed vaccine expressing a fusion protein composed of the OmpA and FlgD antigens from Edwardsiella tarda was significantly better at protecting olive flounder (Paralichthys olivaceus) from edwardsiellosis than single antigen vaccines. Fish Shellfish Immunol (2017) 68:19–28. doi: 10.1016/j.fsi.2017.07.004
242. Gobeli S, Goldschmidt-Clermont E, Frey J, Burr SE. Pseudomonas chlororaphis strain JF3835 reduces mortality of juvenile perch, Perca fluviatilis L., caused by Aeromonas sobria. J Fish Dis (2009) 32(7):597–602. doi: 10.1111/j.1365-2761.2009.01021.x
243. Geng X, Dong XH, Tan BP, Yang QH, Chi SY, Liu HY, et al. Effects of dietary chitosan and Bacillus subtilis on the growth performance, non-specific immunity and disease resistance of cobia, Rachycentron canadum. Fish Shellfish Immunol (2011) 31(3):400–6. doi: 10.1016/j.fsi.2011.06.006
244. Balcázar JL, Vendrell D, Blas ID, Ruiz-Zarzuela I, Múzquiz JL. Effect of Lactococcus lactis CLFP 100 and Leuconostoc mesenteroides CLFP 196 on Aeromonas salmonicida infection in brown trout (Salmo trutta). J Mol Microbiol Biotechnol (2009) 17:153–7. doi: 10.1159/000226588
245. Hjelm M, Bergh O, Riaza A, Nielsen J, Melchiorsen J, Jensen S, et al. Selection and identification of autochthonous potential probiotic bacteria from turbot larvae (Scophthalmus maximus) rearing units. Syst Appl Microbiol (2004) 27:360–71. doi: 10.1078/0723-2020-00256
246. Planas M, Pérez-Lorenzo M, Hjelm M, Gram L, Fiksdal IU, Bergh Ø, et al. Probiotic effect in vivo of Roseobacter strain 27-4 against Vibrio (Listonella) anguillarum infections in turbot (Scophthalmus maximus L.) larvae. Aquaculture (2006) 255(1-4):323–33. doi: 10.1016/j.aquaculture.2005.11.039
247. Chen Y, Li J, Xiao P, Li GY, Yue S, Huang J, et al. Isolation and characterization of Bacillus spp. M 001 for potential application in turbot (Scophthalmus maximus L.) against Vibrio anguillarum. Aquac Nutr (2016) 22(2):374–81. doi: 10.1111/anu.12259
248. Díaz-Rosales P, Arijo S, Chabrillón M, Alarcón FJ, Tapia-Paniagua ST, Martínez-Manzanares E, et al. Effects of two closely related probiotics on respiratory burst activity of Senegalese sole (Solea senegalensis, Kaup) phagocytes, and protection against Photobacterium damselae subsp. piscicida. Aquaculture (2009) 293(1-2):16–21. doi: 10.1016/j.aquaculture.2009.03.050
249. De la Banda I, Lobo C, Chabrillón M, León-Rubio JM, Arijo S, Pazos G, et al. Influence of dietary administration of a probiotic strain Shewanella putrefaciens on Senegalese sole (Solea senegalensis, Kaup 1858) growth, body composition and resistance to Photobacterium damselae subsp. piscicida. Aquac Res (2012) 43(5):662–9. doi: 10.1111/j.1365-2109.2011.02871.x
250. Maeda M, Nogami K, Kanematsu M, Hirayama K. The concept of biological control methods in aquaculture. Hydrobiologia (1997) 358:285–90. doi: 10.1023/A:1003126129709
251. Jia S, Zhou K, Pan RH, Wei J, Liu ZM, Xu YG. Oral immunization of carps with chitosan-alginate microcapsule containing probiotic expressing spring viremia of carp virus (SVCV) G protein provides effective protection against SVCV infection. Fish Shellfish Immunol (2020) 105:327–9. doi: 10.1016/j.fsi.2020.07.052
252. Liu M, Zhao LL, Ge JW, Qiao XY, Li YJ, Liu DQ. Immunogenicity of Lactobacillus-expressing VP2 and VP3 of the infectious pancreatic necrosis virus (IPNV) in rainbow trout. Fish Shellfish Immunol (2012) 32(1):196–203. doi: 10.1016/j.fsi.2011.11.015
253. Zhou S, Song DL, Zhou XF, Mao XL, Zhou XF, Wang SL, et al. Characterization of Bacillus subtilis from gastrointestinal tract of hybrid Hulong grouper (Epinephelus fuscoguttatus x E. lanceolatus) and its effects as probiotic additives. Fish Shellfish Immunol (2019) 84:1115–24. doi: 10.1016/j.fsi.2018.10.058
254. Min L, Li-Li Z, Jun-Wei G, Xin-Yuan Q, Yi-Jing L, Di-Qiu L. Immunogenicity of Lactobacillus-expressing VP2 and VP3 of the infectious pancreatic necrosis virus (IPNV) in rainbow trout. Fish Shellfish Immunol (2012) 32(1):196–203. doi: 10.1016/j.fsi.2011.11.015
255. Naderi-Samani M, Soltani M, Dadar M, Taheri-Mirghaed A, Zargar A, Ahmadivand S, et al. Oral immunization of trout fry with recombinant Lactococcus lactis NZ3900 expressing G gene of viral hemorrhagic septicaemia virus (VHSV). Fish Shellfish Immunol (2020) 105:62–70. doi: 10.1016/j.fsi.2020.07.007
256. Harikrishnan R, Balasundaram C, Heo MS. Effect of probiotics enriched diet on Paralichthys olivaceus infected with lymphocystis disease virus (LCDV). Fish Shellfish Immunol (2010) 29(5):868–74. doi: 10.1016/j.fsi.2010.07.031
257. Sun H, Shang M, Tang Z, Jiang H, Dong H, Zhou X, et al. Oral delivery of Bacillus subtilis spores expressing Clonorchis sinensis paramyosin protects grass carp from cercaria infection. Appl Microbiol Biotechnol (2020) 104(4):1633–46. doi: 10.1007/s00253-019-10316-0
258. Yanuhar U, Junirahma NS, Susilowati K, Caesar NR, Musa M. Effects of probiotic treatment on histopathology of Koi carp (Cyprinus carpio) infected by Myxobolus sp. J Phys: Conf Ser (2019) 1374:12051. doi: 10.1088/1742-6596/1374/1/012051
259. Nurhajati J, Atira, Aryantha INP, Kadek Indah DG. The curative action of Lactobacillus plantarum FNCC 226 to Saprolegnia parasitica A3 on catfish (Pangasius hypophthalamus Sauvage). Int Food Res J (2012) 19(4):1723–7.
260. Kuebutornye FKA, Abarike ED, Lu Y. A review on the application of Bacillus as probiotics in aquaculture. Fish Shellfish Immunol (2019) 87:820–8. doi: 10.1016/j.fsi.2019.02.010
261. Vendrell D, Balcázar JL, de Blas I, Ruiz-Zarzuela I, Gironés O, Múzquiz JL. Protection of rainbow trout (Oncorhynchus mykiss) from lactococcosis by probiotic bacteria. Comp Immunol Microb (2008) 31:337–45. doi: 10.1016/j.cimid.2007.04.002
262. Irianto A, Austin B. Use of probiotics to control furunculosis in rainbow trout, Oncorhynchus mykiss (Walbaum). J Fish Dis (2002) 25:333–42. doi: 10.1046/j.1365-2761.2002.00375.x
263. Kamei Y, Yoshimizu M, Ezura Y, Kimura T. Screening of bacteria with antiviral activity from fresh-water salmonid hatcheries. Microbiol Immunol (1988) 32(1):67–73. doi: 10.1111/j.1348-0421.1988.tb01366.x
264. Chen Y, Hua X, Ren X, Duan K, Gao S, Sun J, et al. Oral immunization with recombinant Lactobacillus casei displayed AHA1-CK6 and VP2 induces protection against infectious pancreatic necrosis in rainbow trout (Oncorhynchus mykiss). Fish Shellfish Immunol (2020) 100:18–26. doi: 10.1016/j.fsi.2020.03.001
Keywords: probiotics, pathogen resistance, interference mechanisms, immunomodulatory effect, teleosts
Citation: Simón R, Docando F, Nuñez-Ortiz N, Tafalla C and Díaz-Rosales P (2021) Mechanisms Used by Probiotics to Confer Pathogen Resistance to Teleost Fish. Front. Immunol. 12:653025. doi: 10.3389/fimmu.2021.653025
Received: 13 January 2021; Accepted: 29 March 2021;
Published: 27 April 2021.
Edited by:
Samuel A. M. Martin, University of Aberdeen, United KingdomReviewed by:
Carlo C. Lazado, Fisheries and Aquaculture Research (Nofima), NorwayDaniel Merrifield, University of Plymouth, United Kingdom
Copyright © 2021 Simón, Docando, Nuñez-Ortiz, Tafalla and Díaz-Rosales. This is an open-access article distributed under the terms of the Creative Commons Attribution License (CC BY). The use, distribution or reproduction in other forums is permitted, provided the original author(s) and the copyright owner(s) are credited and that the original publication in this journal is cited, in accordance with accepted academic practice. No use, distribution or reproduction is permitted which does not comply with these terms.
*Correspondence: Patricia Díaz-Rosales, ZGlhei5wYXRyaWNpYUBpbmlhLmVz