- 1Laboratory of Biological Systems, Department of Health Sciences, University of Guadalajara (UDG), Ameca, Mexico
- 2Department of Health Sciences—Disease as an Individual Process, University of Guadalajara (UDG), Tonalá, Mexico
With the appearance of the SARS-CoV-2 virus in December 2019, all countries in the world have implemented different strategies to prevent its spread and to intensively search for effective treatments. Initially, severe cases of the disease were considered in adult patients; however, cases of older school-age children and adolescents who presented fever, hypotension, severe abdominal pain and cardiac dysfunction, positive for SARS-CoV-2 infection, have been reported, with increased pro-inflammatory cytokines and tissue damage, condition denominated multisystemic inflammatory syndrome (MIS-C); The emerging data from patients with MIS-C have suggested unique characteristics in the immunological response and also clinical similarities with other inflammatory syndromes, which can support as a reference in the search for molecular mechanisms involved in MIS-C. We here in propose that oxidative stress (OE) may play a very important role in the pathophysiology of MIS-C, such as occurs in Kawasaki disease (KD), severe COVID-19 in adults and other processes with characteristics of vascular damage similar to MIS- C, for which we review the available information that can be correlated with possible redox mechanisms.
Introduction
In December 2019, with the recent emergence of the new coronavirus, severe acute respiratory syndrome coronavirus 2 (SARS-CoV-2) has started to appear in China (1). Its subsequent spread globally has forced drastic lifestyle changes around the world after being declared a pandemic by the World Health Organisation (WHO) (2). This virus causes a complicated infectious disease called coronavirus disease 2019 (COVID-19) (3), which has become a global health threat. Until August 2021, COVID‐19 has caused the death of 4,517, 240 individuals worldwide, with more than 217,000,000 cumulative confirmed cases (2).
SARS-CoV-2 and COVID-19
SARS-CoV-2
Coronaviruses (CoVs) are a family of enveloped ribonucleic acid (RNA) viruses that cause infections that are transmitted mainly through the respiratory and fecal-oral routes. This family of viruses is distinguished by the large size of its genome (4); CoVs are classified into four genera based on phylogeny: group 1 or alpha-CoV, group 2 or beta-CoV, group 3 or gamma-CoV and group 4 or delta-CoV (5).
The SARS-CoV-2 is a recently identified beta-coronavirus with a size genome of 29.9 kb, encoding at least 29 proteins, four of which are structural proteins: the spike (S), membrane (M), envelope (E) and nucleocapsid (N) proteins (6, 7).
SARS-CoV-2 Infection and Clinical Aspects of COVID-19
The SARS-CoV-2 is transmitted by droplets generated during coughing and sneezing by infected people without adequate personal protection and hygiene, regardless of whether they are symptomatic or not (8, 9). In SARS-CoV-2 infection, the virus enters the host cells using the S protein through an interaction with host cell receptors (ACE2) and the processing of S protein by endogenous transmembrane serine protease 2 (TMPRSS2), leading to endocytosis and the release of viral RNA, which is replicated and translated into viral proteins to facilitate viral replication (8) (Figure 1).
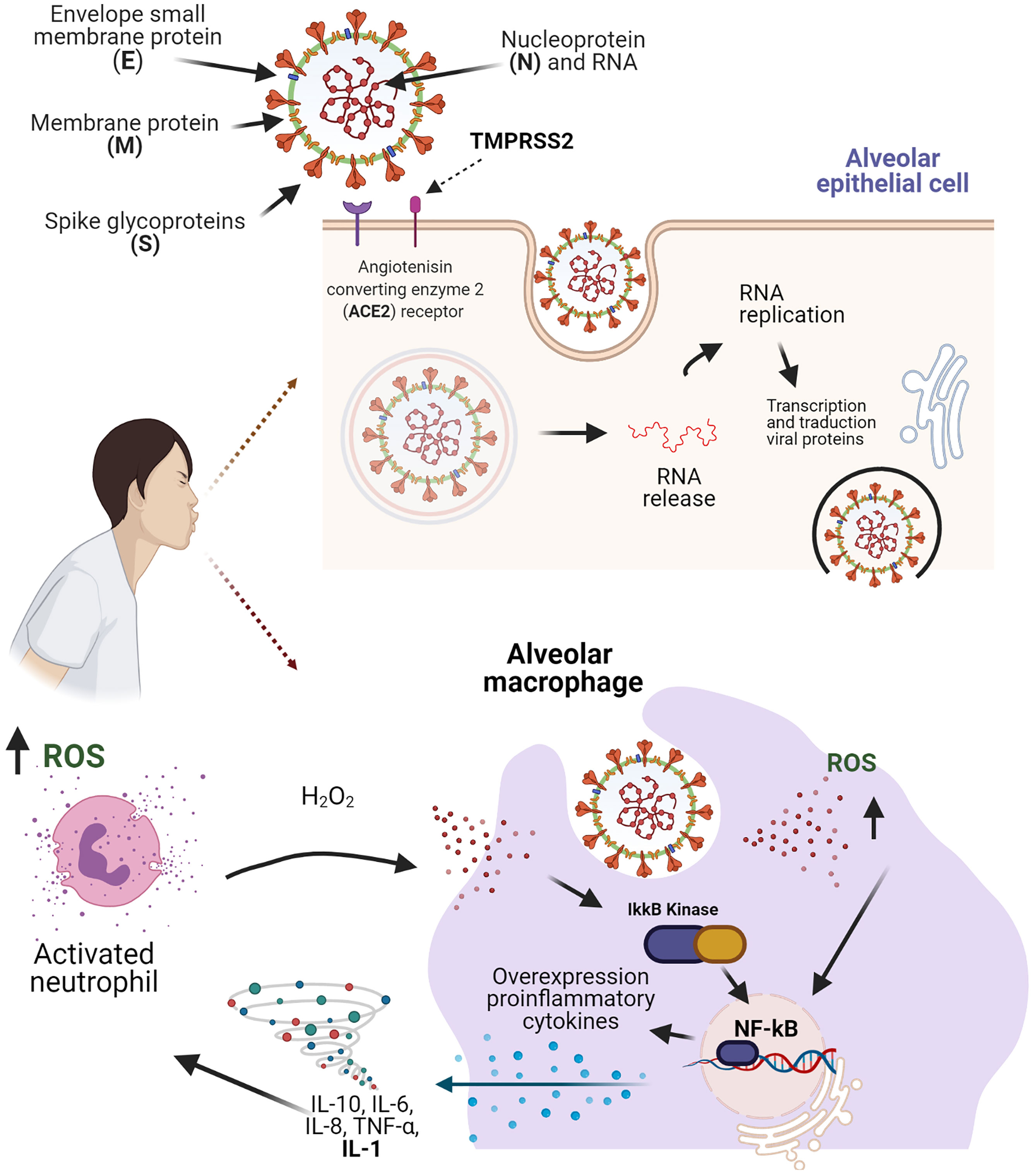
Figure 1 Structure of the SARS-CoV-2 virus and molecular mechanisms in the inflammation process and oxidative stress. The binding of the S protein to a receptor ACER2 and the co-receptor TMPRSS2, allows the SARS-CoV-2 entry the alveolar epithelial cell. The recognition of SARS-CoV-2 in the macrophages, leads the accumulation of ROS and facilitates the activation of the NF-kB pathway, which results in an expression of pro-inflammatory cytokines that promote neutrophil activation. Figure created with BioRender, ©biorender.com.
The severity is highly variable among individuals infected with SARS-CoV-2, ranging from asymptomatic cases up to severe respiratory disease with extrapulmonary findings (10–12).
Initially, people over 65 years of age represented a population at high risk of complications, with approximately 50% of hospitalizations and up to 80% of deaths caused by COVID-19 (13), whereas the pediatric population was considered less affected in frequency and severity (14). However, in late April 2020, the first reports of a childhood multisystem inflammatory condition associated to SARS-CoV-2 infection were published in countries such as the United Kingdom, Italy and the United States (US) (15, 16). “Inflammatory Multisystem Syndrome Temporally Associated with SARS-CoV-2 Infection” (PIMS-TS) in Europe and Multisystem Inflammatory Syndrome in Children (MIS-C) in the US (17).
MIS-C
The MIS-C is a systemic inflammation that comprises the heart, lungs, kidneys, brain, skin, eyes and gastrointestinal organs; the diagnostic criteria are shown in Table 1. The onset of symptoms begins at least 2 weeks after SARS-CoV2 infection (17); some are shared with other pathological entities such as toxic shock syndrome (TSS), macrophage activation syndrome (MAS) and Kawasaki disease (KD) (20, 21). Therefore, the clinical manifestations, the role of SARS-CoV-2 infection and the pathophysiological mechanisms of MIS-C are still unknown. In this sense, it is still uncertain whether the symptoms are due to post-infectious hyper-inflammation, autoinflammatory or autoimmune disease (17, 21, 22).
Laboratory findings include lymphopenia and reduced to normal thrombocytes (21). In the acute phase, there is an increase in inflammatory cytokines and other molecules, such as interleukin-1β (IL-1β), IL-6, IL-8, tumor necrosis factor-α (TNF-α), IL-10, IL-17, interferon-γ (IFN-γ), IL-2 receptor agonist, C-reactive protein (CRP) and ferritin. Additionally, markers of myocardial dysfunction and injury are also increased, including N-terminal pro B-type natriuretic peptide (NT-proBNP) and troponin. In contrast to KD, in MIS-C, there is evidence of a procoagulant state establishment due to raised fibrinogen and D-dimer levels and low platelet count (17).
During the acute phase, there is lymphopenia of helper (CD4+), cytotoxic (CD8+) and γδ T cells. The count of total neutrophils, monocyte, dendritic cell and natural killer cells remains normal, despite the expression of molecules associated with their function changes. High levels of IL-8 could be related to increased neutrophil activation, which in turn could influence T and B lymphocyte responses. In contrast, molecules involved in antigen presentation have decreased expression on macrophages and dendritic cells, suggesting impaired function (23).
Oxidative Stress and COVID-19
The OS is an imbalance between the production of RS and the endogenous antioxidant defense, indicating an imbalance of the pro-oxidant-antioxidant balance in favor of pro-oxidants (24). Two types of reactive species are distinguished: reactive oxygen species (ROS) and reactive nitrogen species (RNS) (25) both types of RS are highly reactive, and their interaction with macromolecules can lead to permanent modifications and altered cell signaling events (26).
OS has been implicated in different pathologies as a triggering factor of the pathophysiological process, such as kidney disease (27), diabetes (28), rheumatic autoimmune disease (24, 29) In addition to its role in these chronic diseases, the production of ROS plays an important role in the defense mechanisms against infectious diseases such as hepatitis B, C and D, herpes, influenza, tuberculosis and leprosy (30–33). However, excessive ROS production increases the inflammatory response and in turn can increase cellular injury induced by viral infection (34). Evidence suggests that OS is involved in acute bronchiolitis caused by respiratory syncytial virus, considering elevated plasma levels of oxidized glutathione (GSSG) and the antioxidant enzyme glutathione peroxidase (GPx) as biomarkers of severity in these patients (35).
Most viral infections, after the invasion of host tissues, hijack cellular functions to initiate viral replication; however, the immune system can limit viral spread through the recognition of innate elements in the infected cells, followed by the activation of defense mechanisms to eradicate the infection via the involvement of cells such as macrophages, epithelial cells, dendritic cells and their pattern recognition receptors (36). In SARS-CoV2 infection, in addition to the participation of these cells, it has been described that mast cells (MC) play a fundamental role in inflammation and severity of patients, through the release of histamine by MC caused by the recognition of the virus and subsequent IL-1 secretion (37).
Some of these pathways increase the activity of type I IFN and inflammatory cytokines (38). After viral replication, there is an increase in the levels of TNF-α, IFN-γ and IL-4, which leads to the activation of cellular pro-inflammatory pathways (39). Importantly, in addition to promoting cytotoxicity, ROS and RNS can initiate and amplify inflammatory pathways through the regulation of gene expression via nuclear factor kappa B (NF-κB) (40). Moreover, the presence of ROS, such as hydrogen peroxide (H2O2), usually functions as a stimulus for the activation of inflammatory responses (41, 42).
The NF-κB pathway is essential to regulate multiple cellular processes, especially inflammatory responses, apoptosis and the differentiation of immune system cells. Several factors can activate the NF-κB pathway, for example TNFα, lipopolysaccharide (LPS) and IL-1 (43). The activation of this transcriptional factor during viral infections has been previously evaluated, demonstrating its role in processes such as promoting viral replication and regulating the host immune response (44). The hyperactivation of this transcription factor is an important key for the progression of severe cases of COVID-19, since in these critically ill patients, the levels of pro-inflammatory cytokines and ROS are significantly higher (45).
In severe cases of COVID-19, there is a significant decrease in oxygen saturation (less than 90%), which produces significant hypoxic changes (46, 47). This events also lead to the development of OS just as the reduction in oxygen saturation enhances the formation of ROS such as superoxide and peroxide (H2O2) that, in turn, cause changes in membrane lipids, nucleic acids and other cellular structures (48). For this reason, this pathway NF-κB could be a key element to understand the role of OS in severe cases of COVID-19 through increasing the inflammatory process (49). Likewise, it is known that during SARS-Cov-2 infection, local and systemic inflammation is generated mediated by pro-inflammatory cytokines, mainly by IL-1, a cytokine with a wide spectrum of biological activities: activation of adhesion molecules, endothelial dysfunction, stimulation in the secretion of TNF and IL-6, as well as an increase in nitric oxide and the release of prostaglandins and thromboxane A2 (50), the latter highly related to the development of OS and endothelial damage. On the other hand, it has been proposed that the suppression of the IL-1 and IL-6 response could have therapeutic effects in the management of patients with COVID-19, through an approach directed at IL-37 and IL-38 (51).
Another pathway that correlates OS with SARS-CoV2 infection is the Nrf-2 factor (nuclear factor erythroid-derived 2-related factor 2), which regulates approximately 250 genes involved in cell homeostasis, including antioxidant proteins and numerous cytoprotective proteins (52). The role of Nrf2 in inflammation, immunity and aging is well known, and it has also been shown that the severity of COVID-19 infection is inversely associated with the expression of Nrf2 (53). Studies have shown that the use of Nrf2 activators suppresses the inflammatory response and generates antiviral effects that can help reduce viral replication, improving the prognosis of patients with hyper-inflammation with COVID-19 (54, 55).
Both NF-κB and Nrf2 are regulated by oxidative factors: the blocking of Nrf2 is associated with increased oxidative and nitrosative stress, leading to amplification of cytokine production, and the NF-κB factor is readily activated in oxidative environments (56) (Figure 1). For this reason, some authors consider that the Nrf2 and NF-κB pathways are involved in the development and progress of inflammatory pathology in COVID-19, and the different treatments aimed at this signaling pathway offer a tool in the management of the patients (57).
The involvement of OS in the progression and severity of COVID-19 has not been fully demonstrated; however, a study showed the increase of high-sensitivity CRP (hs-CRP), a marker of OS, in patients that died after COVID-19 disease (58). The administration of antioxidants supports the hypothesis that the re-establishment of redox conditions in cells could be a suitable approach for the concomitant treatment of multiple diseases associated with OS. Moreover, recent studies have shown that vitamin D supplementation, as adjuvant therapy, improves the prognosis of patients with COVID-19; in some cases supplemented with vitamin D and B12, oxygen requirements decreased compared with the non-supplemented group (58, 59).
Influence of OS on the Pathophysiology of MIS-C
The pathophysiology of MIS-C is not exactly known, and OS could play an important role: first, OS regulates the NF-κB, which in turn regulates the immune response and inflammation in several viral infections (17, 20). Second, previous studies have shown that OS is involved in the acute stage of KD, caused by an overproduction of ROS for activated inflammatory cells (60). Although MIS-C has clinical similarities and cytokine profiles comparable to KD and TSS, recent studies suggest that there are differences in the type of activated cells during the immune response, observing a specific expansion of activated T lymphocytes that express the Vβ21.3 T cell receptor β chain variable region in CD4 and CD8 cells, something that differs in KD, TSS patients or SARS-COV-2 (61). In addition, the antibody response in MIS-C compared to adults with severe COVID-19 presents important differences, for example, a study showed that patients with MIS-C produced predominantly specific IgG antibodies (Abs) for the S protein but not for the N protein and in addition to a reduced neutralizing activity, while adult patients with COVID-19 presented Abs anti-S IgG, IgM and IgA, as well as Abs anti-N IgG, these results suggested that the immunological profile in MIS-C presents certain differential characteristics of COVID-19 in adults (62). Similarly, it has been reported that patients with MIS-C present an immunological profile that is characterized by strong activation of CX3CR1 + CD8 + T cells of vascular patrolling however this activation decreases with time and is correlated with clinical improvement of patients, which only occurs in patients with MIS-C and not in pediatric patients with COVID-19 without MIS-C (63). Despite these specific differences between COVID-19 in adults, pediatric patients without MIS-C versus MIS-C, there are similar immunological characteristics between MIS-C and severely ill adult patients with COVID-19, in which it has been shown that the redox state is strongly altered, observing an increase in lipid peroxidation, and a deficit of some antioxidants (vitamin C, glutathione), a situation that could be similar in patients with MIS-C (64).
The pathophysiology of COVID-19 in adults includes endothelitis and lymphocytic endothelitis in organs such as the lung, heart, kidney and liver (65), this endothelial dysfunction has been associated with an increase in the hormone that regulates vascular tone (endthelin-1) and changes in the coagulation; despite the differences in the immune response between COVID-19 in adults and MIS-C (previously mentioned), there are similar events such as endothelitis, which have been proposed to describe the pathophysiology in MIS-C, identifying the presence of IgG and IgA autoantibodies that recognize endothelial cells, promoting damage and contributing to endothelial dysfunction and multisystem inflammation typical of MIS-C (66, 67). In patients with atherosclerosis, hypertension, endotoxic shock or infectious diseases, an increase in endothelin-1 has been described, which is attributed pro-inflammatory activities, platelet aggregation and also plays a role in the increase in the expression of adhesion molecules of leukocytes (68), causing endothelial cell injury; this endothelial dysfunction is also consistent and is related to mortality in patients with COVID-19 (69), in severe patients with MIS-C endothelial dysfunction could explain its clinical presentation (Figure 2).
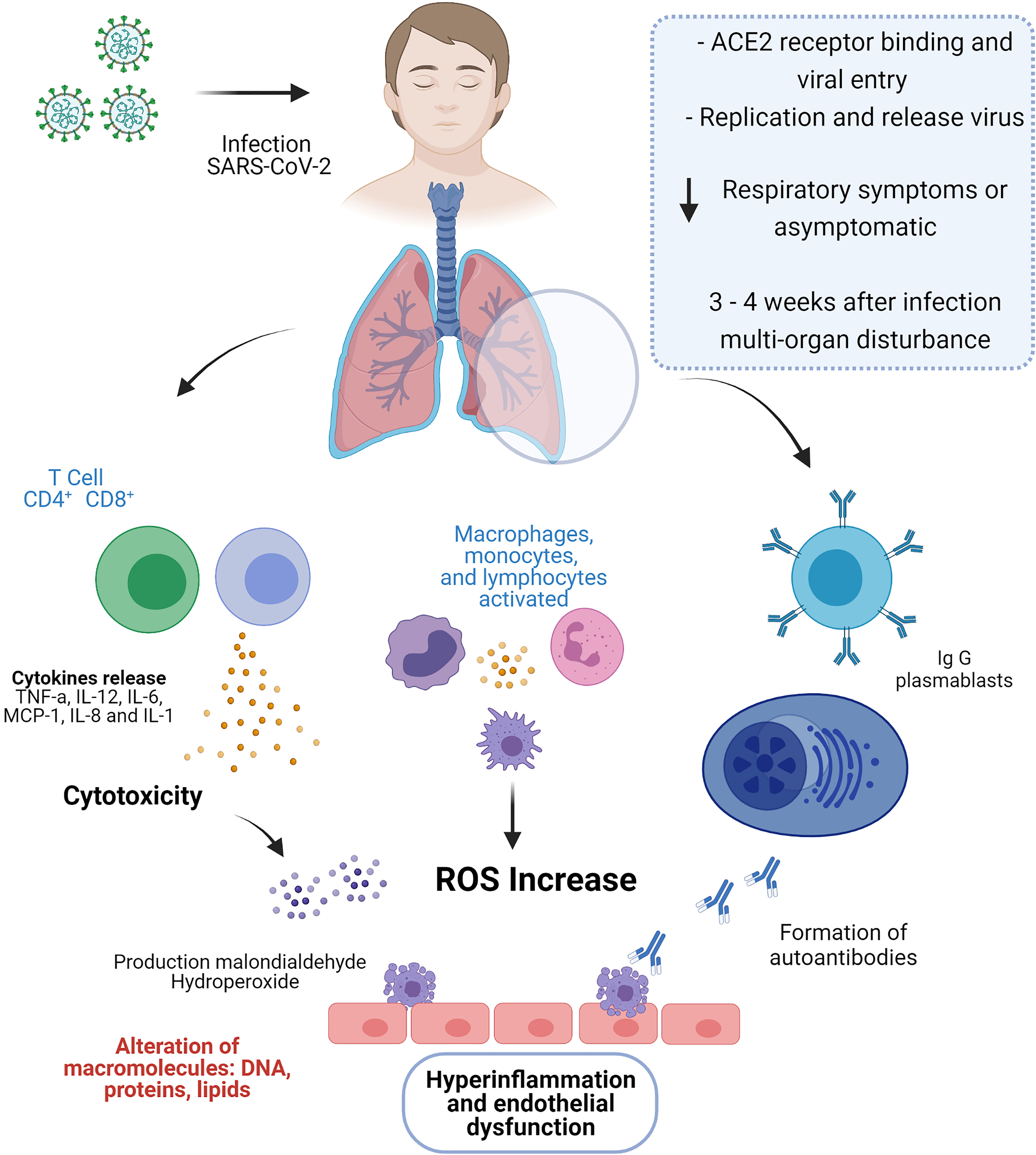
Figure 2 In SARS-CoV-2 infection, the binding of the receptor ACE2 with the virus leads to entry into the host cell and its subsequent replication and release. During the active phase of COVID-19, MIS-C patients were asymptomatic or with mild respiratory symptoms; however, three to four weeks after exposure to SARS-CoV-2, children develop a multi-organ disturbance. MIS-C is characterized by strong activation of T lymphocytes leading to the release of a cytokine storm and increased cytotoxic activity. Furthermore, T cells, together with monocytes and macrophages, promote oxidative stress by increasing reactive oxygen species, which alter macromolecules such as DNA, proteins and lipids. Additionally, the presence of autoantibodies that recognize endothelial cells, promoting damage and contributing to endothelial dysfunction and multisystem inflammation typical of MIS-C. Figure created with BioRender, ©biorender.com.
As mentioned previously, MIS-C has been associated due to its clinical characteristics with other inflammatory syndromes (TSS and KD), for which some common pathways and divergences are mentioned that may explain the participation of OS in MIS-C. It has been proposed that platelet count can help differentiate KD and MIS-C, since KD patients are characterized by normal/high platelet counts while MIS-C patients commonly present with lower platelet counts (70) which leads us to reinforce our hypothesis that OS could be involved in this process, as occurs in immune thrombocytopenia (IT), in which multiple lines of evidence suggest that OS participates in the pathogenesis of this disorder, observing elevation of lipid peroxidation and the reduction of antioxidant capacity, as well as the use of antioxidants improves the prognosis in these patients (71, 72). It has also been described that patients with acute MIS-C present increased binding of antibodies to antigens associated with the development of the endothelium and the heart and other common targets with autoimmunity compared to healthy controls (73). Likewise, the possibility is raised that in patients with severe MIS-C, there is the formation of autoantibodies, which bind to endothelial cells, which contribute to endothelial dysfunction and multisystem inflammation characteristic of these patients (74). Although there is no unifying hypothesis on how ET-1 affects this process in patients with MIS-C, its significant role in the progression of the pathology is not ruled out, such as occurs in KD where the levels of immunoreactive endothelin (iET) in the blood are increased as a result of associated vascular endothelial damage (75). The biosynthesis and release of ET-1 are regulated at the transcriptional level by different factors such as the kinase p38MAP, NF-κB, PKC/ERK and JNK/c-Jun, which in turn are activated in the presence of OS (76), which may reinforce the possible participation of OS in the pathogenesis of MIS-C, considering the similarities with other diseases with a characteristic of endothelial damage.
It is evident that in inflammatory syndromes there is an exacerbated and uncontrolled immune response, such as occurs with MIS-C; Therefore, prior knowledge of these syndromes can support the description of the pathophysiology in MIS-C, for example in the systemic inflammatory response syndrome (SIRS), there is an over-performance of cellular immune response, similar to what happens In patients with MIS-C, likewise, the participation of EO in SIRS is well known, reports report that in patients with a diagnosis of SIRS there are increases in lipoperoxidation, as well as decreased antioxidant capacity compared to patients without SIRS (77, 78). Likewise, the use of antioxidant nutrients has demonstrated clinical and n SIRS (79).
Similar to SIRS, there is evidence of OS involvement in KD, Some OS biomarkers, such as reactive oxygen metabolites (ROM), were increased in patients with KD naïve to treatment and favorably decreased in cases responding to treatment, in contrast to non-responding patients (80). It has also been described that there is a late increase in plasma levels of malondialdehyde and hydroperoxide after acute disease in patients with KD (81).
On the other hand, antioxidant markers are decreased in patients with KD in acute stages, such as the antioxidant power (82). Also, there is evidence of an association between the presence of manganese superoxide dismutase (MnSOD) rs5746136 polymorphism and susceptibility to KD (83). Other studies have proposed the use of antioxidant substances as adjuvant therapy for these patients; in particular, berberine exerts a protective effect against KD-induced damage of human coronary artery endothelial cells through the decrease of OS markers (84).
Although KD, SIRS, TSS, and MIS-C have differences, these diseases can provide a model for studying the pathogenesis of MIS-C, especially since KD, TSS, and SIRS are believed to be triggered by viral infections, sepsis, and can evolve to systemic inflammation and vasculitis (85).
Conclusions
The disorder MIS-C is a systemic hyper-inflammation developed by some children after SARS-CoV-2 infection. It shares clinical features and molecular mechanisms with other pathological entities, such as KD. Like KD, the exacerbated immune response in MIS-C could be associated with OS development via NF-κB, Nrf2, endothelin-1, and others pathways. However, more studies are required to clarify the participation of OS in the pathogenesis of MIS-C.
Further Research
Further research is needed to support confirming whether OS plays an important role in the physiopathology of MIS-C, studies should be established focused on the search for new specific biomarkers on OS in these patients.
Author Contributions
Conceptualization of the study, OG-M and SS-F. Literature search, GV-R, IL-P, and JM-B. Writing—original draft preparation, GV-R, IL-P, OG-M, and SS-F. Writing—review and editing, JM-B, OG-M, and SS-F. All authors contributed to the article and approved the submitted version.
Conflict of Interest
The authors declare that the research was conducted in the absence of any commercial or financial relationships that could be construed as a potential conflict of interest.
Publisher’s Note
All claims expressed in this article are solely those of the authors and do not necessarily represent those of their affiliated organizations, or those of the publisher, the editors and the reviewers. Any product that may be evaluated in this article, or claim that may be made by its manufacturer, is not guaranteed or endorsed by the publisher.
References
1. Li T, Lu H, Zhang W. Clinical Observation and Management of COVID-19 Patients. Emerging Microbes Infect (2020) 9(1):687–90. doi: 10.1080/22221751.2020.1741327
3. Zhu N, Zhang DY, Wang WL, Li X, Yang B, Song J, et al. A Novel Coronavirus From Patients With Pneumonia in China, 2019. N Engl J Med (2020) 382(8):727–33. doi: 10.1056/NEJMoa2001017
4. Masters PS. The Molecular Biology of Coronaviruses. Adv Virus Res 66 (2006) 66:193–292. doi: 10.1016/S0065-3527(06)66005-3
5. De-Groot RJ, Baker SC, Baric R, Enjuanes L, Gorbalenya AE, Holmes KV, et al. Family Coronaviridae. In: Virus Taxonomy: Ninth Report of the International Committee on Taxonomy of Viruses. San Diego, CA: Academic Press (2012). p. 806–8.
6. Wu F, Zhao S, Yu B, Chen YM, Wang W, Song ZG, et al. A New Coronavirus Associated With Human Respiratory Disease in China. Nature (2020) 579(7798):265–9. doi: 10.1038/s41586-020-2008-3
7. Kim D, Lee JY, Yang JS, Kim JW, Kim VN, Chang H. The Architecture of SARS-CoV-2 Transcriptome. Cell (2020) 181(4):914–21.e10. doi: 10.1016/j.cell.2020.04.011
8. Rothe C, Schunk M, Sothmann P, Bretzel G, Froeschl G, Wallrauch C, et al. Transmission of 2019- Ncov Infection From an Asymptomatic Contact in Germany. N Engl J Med (2020) 382(10):970–1. doi: 10.1056/NEJMc2001468
9. Xu J, Li Y, Gan F, Du Y, Yao Y. Salivary Glands: Potential Reservoirs for COVID-19 Asymptomatic Infection. J Dental Res (2020) 99(8):989. doi: 10.1177/0022034520918518
10. Satturwar S, Fowkes M, Farver C, Wilson AM, Eccher A, Girolami I, et al. Postmortem Findings Associated With SARS-CoV-2: Systematic Review and Meta-Analysis. Am J Surg Pathol (2021) 45(5):587–603. doi: 10.1097/PAS.0000000000001650
11. Chu J, Yang N, Wei Y, Yue H, Zhang F, Zhao J, et al. Clinical Characteristics of 54 Medical Staff With COVID-19: A Retrospective Study in a Single Center in Wuhan, China. J Med Virol (2020) 92(7):807–13. doi: 10.1002/jmv.25793
12. Lin L, Jiang X, Zhang Z, Huang S, Zhang Z, Fang Z, et al. Gastrointestinal Symptoms of 95 Cases With SARS-CoV-2 Infection. Gut (2020) 69:997–1001. doi: 10.1136/gutjnl-2020-321013
13. Centers for Disease Control and Prevention. Underlying Medical Conditions Associated With High Risk for Severe COVID-19: Information for Healthcare Providers. Available at: https://www.cdc.gov/coronavirus/2019-ncov/hcp/clinical-care/underlyingconditions.html (Accessed March 29, 2021).
14. Huang C, Wang Y, Li X, Ren L, Zhao J. Clinical Features of Patients Infected With 2019 Novel Coronavirus in Wuhan, China. Lancet (2020) 395:497–506. doi: 10.1016/S0140-6736(20)30183-5
15. Riphagen S, Gomez X, Gonzalez-Martinez C, Wilkinson N, Theocharis P. Hyperinflammatory Shock in Children During COVID-19 Pandemic. Lancet (2020) 395(10237):1607–8. doi: 10.1016/S0140-6736(20)31094-1
16. Verdoni L, Mazza A, Gervasoni A, Martelli L, Ruggeri M, Ciuffreda M, et al. An Outbreak of Severe Kawasaki-Like Disease at the Italian Epicentre of the SARS-CoV-2 Epidemic: An Observational Cohort Study. Lancet (2020) 395(10239):1771–8. doi: 10.1016/S0140-6736(20)31103-X
17. Carter MJ, Shankar-Hari M, Tibby SM. Paediatric Inflammatory Multisystem Syndrome Temporally-Associated With SARS-CoV-2 Infection: An Overview. Intensive Care Med (2021) 47(1):90–93. doi: 10.1007/s00134-020-06273-2
18. Royal College of Pediatrics and Child Health (UK). COVID-19 - Guidance for Management of Children Admitted to Hospital. Available at: https://www.rcpch.ac.uk/resources/covid-19-guidance-management-children-admitted-hospital (Accessed March 29, 2021).
19. Centers for Disease Control and Prevention. MIS-C and COVID-19. Available at: https://www.cdc.gov/mis-c/ (Accessed March 29, 2021).
20. Jones VG, Mills M, Suarez D, Hogan CA, Yeh D, Segal JB, et al. COVID-19 and Kawasaki Disease: Novel Virus and Novel Case. Hosp Pediatr (2020) 10(6):537–40. doi: 10.1542/hpeds.2020-0123
21. Hoste L, Van Paemel R, Haerynck F. Multisystem Inflammatory Syndrome in Children Related to COVID-19: A Systematic Review. Eur J Pediatr (2021) 180(7):1–16. doi: 10.1007/s00431-021-03993-5
22. Tung-Chen Y, Algora-Martín A, Rodríguez-Roca S, Díaz de Santiago A. COVID-19 Multisystemic Inflammatory Syndrome in Adults: A Not to be Missed Diagnosis. BMJ Case Rep (2021) 14(4):e241696. doi: 10.1136/bcr-2021-241696
23. Carter MJ, Fish M, Jennings A, Doores KJ, Wellman P, Seow J, et al. Peripheral Immunophenotypes in Children With Multisystem Inflammatory Syndrome Associated With SARS-CoV-2 Infection. Nat Med (2020) 26:1701–7. doi: 10.1038/s41591-020-1054-6
24. Anand Kumar K, Humairafarooqi. Evaluation of the Effect of Hydrogen Peroxide (H2O2) on Haemoglobin and the Protective Effect of Glycine. Int J Sci &Tecnhnoledge (2014) 2(2):36–41.
25. Noiri E, Addabbo F, Goligorsky MS. Reactive Oxygen and Nitrogen Species, Oxidative and Nitrosative Stress, and Their Role in the Pathogenesis of Acute Kidney Injury. In: Miyata T, Eckardt KU, Nangaku M. editors. Studies on Renal Disorders. Oxidative Stress in Applied Basic Research and Clinical Practice. Humana Press (2011). doi: 10.1007/978-1-60761-857-7_9
26. Forman HJ, Ursini F, Maiorino M. An Overview of Mechanisms of Redox Signaling. J Mol Cell Cardiol (2014) 73:2–9. doi: 10.1016/j.yjmcc.2014.01.018
27. Su H, Wan C, Song A, Qiu Y, Xiong W, Zhang C. Oxidative Stress and Renal Fibrosis: Mechanisms and Therapies. Adv Exp Med Biol (2019) 1165:585–604. doi: 10.1007/978-981-13-8871-2_29
28. Ighodaro OM. Molecular Pathways Associated With Oxidative Stress in Diabetes Mellitus. BioMed Pharmacother (2018) 108:656–62. doi: 10.1016/j.biopha.2018.09.058
29. Winrow VR, Winyard PG, Morris CJ, Blake DR. Free Radicals in Inflammation: Second Messengers and Mediators of Tissue Destruction. Br Med Bull (1993) 49(3):506–22. doi: 10.1093/oxfordjournals.bmb.a072627
30. Pereira-Suárez AL, Alvarado-Navarro A, Barrietos-García JG, Estrada-Chávez C, Muñoz-Valle JF, Fafutis-Morris M. Differential Expression of Solute Carrier Family 11a Member 1 and Inducible Nitric Oxide Synthase 2 in Skin Biopsies From Leprosy Patients. Indian J Dermatol Venereology Leprology (2015) 81(6):594–9. doi: 10.4103/0378-6323.168345
31. Shastri MD, Shukla SD, Chong WC, Dua K, Peterson GM, Patel RP, et al. Role of Oxidative Stress in the Pathology and Management of Human Tuberculosis. Oxid Med Cell Longevity (2018) 2018:7695364. doi: 10.1155/2018/7695364
32. Ivanov AV, Valuev-Elliston VT, Ivanova ON, Kochetkov SN, Starodubova ES, Bartosch B, et al. Oxidative Stress During HIV Infection: Mechanisms and Consequences. Oxid Med Cell Longevity (2016) 2016:8910396. doi: 10.1155/2016/8910396
33. Zhang Z, Rong L, Li YP. Flaviviridae Viruses and Oxidative Stress: Implications for Viral Pathogenesis. Oxid Med Cell Longevity (2019) 2019:1409582. doi: 10.1155/2019/1409582
34. de la Fuente M, Miquel J. An Update of the Oxidation-Inflammation Theory of Aging: The Involvement of the Immune System in Oxi-Inflamm-Aging. Curr Pharm Des (2009) 15(26):3003–26. doi: 10.2174/138161209789058110
35. Moreno-Solís G, Dela Torre-Aguilar MJ, Torres-Borrego J, Llorente-Cantarero FJ, Fernández-Gutiérrez F, Gil-Campos M, et al. Oxidative Stress and Inflamatory Plasma Biomarkers in Respiratory Syncytial Virus Bronchiolitis. Clin Respir J (2017) 11(6):839–46. doi: 10.1111/crj.12425
36. Maarouf M, Rai KR, Goraya MU, Chen JL. Immune Ecosystem of Virus-Infected Host Tissues. Int J Mol Sci (2018) 19(5):1379. doi: 10.3390/ijms19051379
37. Conti P, Caraffa A, Tetè G, Gallenga CE, Ross R, Kritas SK, et al. Mast Cells Activated by SARS-CoV-2 Release Histamine Which Increases IL-1 Levels Causing Cytokine Storm and Inflammatory Reaction in COVID-19. J Biol Regulators Homeostatic Agents (2020) 34(5):1629–32. doi: 10.23812/20-2EDIT
38. Koyama S, Ishii KJ, Coban C, Akira S. Innate Immune Response to Viral Infection. Cytokine (2008) 43(3):336–41. doi: 10.1016/j.cyto.2008.07.009
39. Mogensen TH, Paludan SR. Molecular Pathways in Virus-Induced Cytokine Production. Microbiol Mol Biol Rev MMBR (2001) 65(1):131–50. doi: 10.1128/MMBR.65.1.131-150.2001
40. DeDiego ML, Nieto-Torres JL, Regla-Nava JA, Jimenez-Guardeño JM, Fernandez-Delgado R, Fett C, et al. Inhibition of NF-κb-Mediated Inflammation in Severe Acute Respiratory Syndrome Coronavirus-Infected Mice Increases Survival. J Virol (2014) 88(2):913–24. doi: 10.1128/JVI.02576-13
41. Schreck R, Rieber P, Baeuerle PA. Reactive Oxygen Intermediates as Apparently Widely Used Messengers in the Activation of the NF-Kappa B Transcription Factor and HIV-1. EMBO J (1991) 10(8):2247–58. doi: 10.1002/j.1460-2075.1991.tb07761.x
42. Chen Y, Zhou Z, Min W. Mitochondria, Oxidative Stress and Innate Immunity. Front Physiol (2018) 9:3389. doi: 10.3389/fphys.2018.01487
43. Staal FJ, Roederer M, Herzenberg LA, Herzenberg LA. Intracellular Thiols Regulate Activation of Nuclear Factor Kappa B and Transcription of Human Immunodeficiency Virus. Proc Natl Acad Sci USA (1990) 87(24):9943–7. doi: 10.1073/pnas.87.24.9943
44. Sun SC. The Non-Canonical NF-κb Pathway in Immunity and Inflammation. Nat Rev Immunol (2017) 17(9):545–58. doi: 10.1038/nri.2017.52
45. Carcaterra M, Caruso C. Alveolar Epithelial Cell Type II as Main Target of SARS-CoV-2 Virus and COVID-19 Development via NF-Kb Pathway Deregulation: A Physio-Pathological Theory. Med Hypotheses (2021) 146:110412. doi: 10.1016/j.mehy.2020.110412
46. Xu Z, Shi L, Wang Y, Zhang J, Huang L, Zhang C, et al. Pathological Findings of COVID-19 Associated With Acute Respiratory Distress Syndrome. Lancet Respir Med (2020) 8(4):420–2. doi: 10.1016/S2213-2600(20)30076-X
47. Vaporidi K, Akoumianaki E, Telias I, Goligher EC, Brochard L, Georgopoulos D. Respiratory Drive in Critically Ill Patients. Pathophysiology and Clinical Implications. Am J Respir Crit Care Med (2020) 201(1):20–32. doi: 10.1164/rccm.201903-0596SO
48. Trentadue R, Fiore F, Massaro F, Papa F, Iuso A, Scacco S, et al. Induction of Mitochondrial Dysfunction and Oxidative Stress in Human Fibroblast Cultures Exposed to Serum From Septic Patients. Life Sci (2012) 91(7-8):237–43. doi: 10.1016/j.lfs.2012.06.041
49. Tisoncik JR, Korth MJ, Simmons CP, Farrar J, Martin TR, Katze MG. Into the Eye of the Cytokine Storm. Microbiol Mol Biol Rev (2012) 76:16–32. doi: 10.1128/MMBR.05015-11
50. Conti P, Caraffa A, Gallenga CE, Ross R, Kritas SK, Frydas I, et al. Coronavirus-19 (SARS-CoV-2) Induces Acute Severe Lung Inflammation via IL-1 Causing Cytokine Storm in COVID-19: A Promising Inhibitory Strategy. J Biol Regulators Homeostatic Agents (2020) 34(6):1971–5. doi: 10.23812/20-1-E
51. Conti P, Ronconi G, Caraffa A, Gallenga CE, Ross R, Frydas I, et al. Induction of Pro-Inflammatory Cytokines (IL-1 and IL-6) and Lung Inflammation by Coronavirus-19 (COVI-19 or SARS-CoV-2): Anti-Inflammatory Strategies. J Biol Regulators Homeostatic Agents (2020) 34(2):327–31. doi: 10.23812/CONTI-E
52. O’Connell MA, Hayes JD. The Keap1/Nrf2 Pathway in Health and Disease: From the Bench to the Clinic. Biochem Soc Trans (2015) 43:687–9. doi: 10.1042/BST20150069
53. Liu J, Li S, Liu J, Liang B, Wang X, Wang H, et al. Longitudinal Characteristics of Lymphocyte Responses and Cytokine Profiles in the Peripheral Blood of SARS–CoV–2 Infected Patients. E Bio Med (2020) 55:102763. doi: 10.2139/ssrn.3539682
54. Olagnier D, Farahani E, Thyrsted J, Cadanet J, Herengt A, Idorn M, et al. Identification of SARS-CoV2-Mediated Suppression of NRF2 Signaling Reveals a Potent Antiviral and Anti–Inflammatory Activity of 4–Octyl-Itaconate and Dimethyl Fumarate. Res Sq (2020) 11(1):4938. doi: 10.21203/rs.3.rs-31855/v1
55. McCord JM, Hybertson BM, Cota–Gomez A, Gao B. Nrf2 Activator PB125® as a Potential Therapeutic Agent Against COVID-19. bioRxiv (2020) 9(2):518. doi: 10.1101/2020.05.16.099788
56. Ganesh Yerra V, Negi G, Sharma SS, Kumar A. Potential Therapeutic Effects of the Simultaneous Targeting of the Nrf2 and NF-KappaB Pathways in Diabetic Neuropathy. Redox Biol (2013) 1:394–7. doi: 10.1016/j.redox.2013.07.005
57. Bhandari R, Khanna G, Kaushik D, Kuhad A. Divulging the Intricacies of Crosstalk Between NF-Kb and Nrf2-Keap1 Pathway in Neurological Complications of COVID-19. Mol Neurobiol (2021) 58(7):1–15. doi: 10.1007/s12035-021-02344-7
58. Chen L, Liu HG, Liu W, Liu J, Liu K, Shang J, et al. Zhonghuajie He Hehu Xi Zazhi = Zhonghuajiehe He Huxizazhi. = Chin J tuberculosis Respir Dis (2020) 43(0):E005. doi: 10.3760/cma.j.issn.1001-0939.2020.0005
59. Trentadue R, Fiore F, Massaro F, Papa F, Iuso A, Scacco S, et al. Induction of Mitochondrial Dysfunction and Oxidative Stress in Human Fibroblast Cultures Exposed to Serum From Septic Patients. Life Sci (2012) 91(7-8):237–43. doi: 10.1016/j.lfs.2012.06.041
60. Takahashi K, Oharaseki T, Naoe S, Wakayama M, Yokouchi Y. Neutrophilic Involvement in the Damage to Coronary Arteries in Acute Stage of Kawasaki Disease. PediatrInt (2005) 47:305–10. doi: 10.1111/j.1442-200x.2005.02049.x
61. Moreews M, Le Gouge K, Khaldi-Plassart S, Pescarmona R, Mathieu AL, Malcus C, et al. Polyclonal Expansion of TCR Vbeta 21.3+ CD4+ and CD8+ T Cells Is a Hallmark of Multisystem Inflammatory Syndrome in Children. Sci Immunol (2021) 6(59):eabh1516. doi: 10.1126/sciimmunol.abh1516
62. Weisberg SP, Connors T, Zhu Y, Baldwin M, Lin W-H, Wontakal S, et al. Antibody Responses to SARS-CoV2 Are Distinct in Children With MIS-C Compared to Adults With COVID-19. medRxiv: Preprint Server Health Sci (2020) 2020.07.12.20151068. doi: 10.1101/2020.07.12.20151068
63. Vella LA, Giles JR, Baxter AE, Oldridge DA, Diorio C, Kuri-Cervantes L, et al. Deep Immune Profiling of MIS-C Demonstrates Marked But Transient Immune Activation Compared to Adult and Pediatric COVID-19. Sci Immunol (2021) 6(57):eabf7570. doi: 10.1126/sciimmunol.abf7570
64. Pincemail J, Cavalier E, Charlier C, Cheramy-Bien JP, Brevers E, Courtois A, et al. Oxidative Stress Status in COVID-19 Patients Hospitalized in Intensive Care Unit for Severe Pneumonia. A Pilot Study Antioxidants (Basel Switzerland) (2021) 10(2):257. doi: 10.3390/antiox10020257
65. Varga Z, Flammer AJ, Steiger P, Haberecker M, Andermatt R, Zinkernagel AS, et al. Endothelial Cell Infection and Endotheliitis in COVID-19. Lancet (London England) (2020) 395(10234):1417–8. doi: 10.1016/S0140-6736(20)30937-5
66. Consiglio CR, Cotugno N, Sardh F, Pou C, Amodio D, Rodriguez L, et al. The Immunology of Multisystem Inflammatory Syndrome in Children With COVID-19. Cell (2020) 183(4):968–81.e7. doi: 10.1016/j.cell.2020.09.016
67. Gruber CN, Patel RS, Trachtman R, Lepow L, Amanat F, Krammer F, et al. Mapping Systemic Inflammation and Antibody Responses in Multisystem Inflammatory Syndrome in Children (MIS-C). Cell (2020) 183(4):982–95.e14. doi: 10.1016/j.cell.2020.09.034
68. Freeman BD, Machado FS, Tanowitz HB, Desruisseaux MS. Endothelin-1 and Its Role in the Pathogenesis of Infectious Diseases. Life Sci (2014) 118(2):110–9. doi: 10.1016/j.lfs.2014.04.021
69. Amraei R, Rahimi N. COVID-19, Renin-Angiotensin System and Endothelial Dysfunction. Cells (2020) 9(7):1652. doi: 10.3390/cells9071652
70. Yeo WS, Ng QX. Distinguishing Between Typical Kawasaki Disease and Multisystem Inflammatory Syndrome in Children (MIS-C) Associated With SARS-CoV-2. Med Hypotheses (2020) 144:110263. doi: 10.1016/j.mehy.2020.110263
71. Li X, Wang Z, Qi X, Zhang D. Oxidative Stress and Antioxidant Status in Immune Thrombocytopenia. Blood Disord Transfusion (2017) 8:4. doi: 10.4172/2155-9864.1000392
72. Polat G, Tamer L, Tanriverdi K, Gürkan E, Baslamisli F, Atik U. Levels of Malondialdehyde, Glutathione and Ascorbic Acid in Idiopathic Thrombocytopaenic Purpura. East Afr Med J (2002) 79(8):446–9. doi: 10.4314/eamj.v79i8.8833
73. Gruber CN, Patel RS, Trachtman R, Lepow L, Amanat F, Krammer F, et al. Mapping Systemic Inflammation and Antibody Responses in Multisystem Inflammatory Syndrome in Children (MIS-C). Cell (2020) 183(4):982–995.e14. doi: 10.1016/j.cell.2020.09.034
74. Ramaswamy A, Brodsky NN, Sumida TS, Comi M, Asashima H, Hoehn KB, et al. Post-Infectious Inflammatory Disease in MIS-C Features Elevated Cytotoxicity Signatures and Autoreactivity That Correlates With Severity. medRxiv Preprint Server Health Sci (2021) 2020.12.01.20241364. doi: 10.1101/2020.12.01.20241364
75. Morise T, Takeuchi Y, Takeda R, Karayalcin U, Yachie A, Miyawaki T. Increased Plasma Endothelin Levels in Kawasaki Disease: A Possible Marker for Kawasaki Disease. Angiology (1993) 44(9):719–23. doi: 10.1177/000331979304400908
76. Piechota A, Polańczyk A, Goraca A. Role of Endothelin-1 Receptor Blockers on Hemodynamic Parameters and Oxidative Stress. Pharmacol Rep PR (2010) 62(1):28–34. doi: 10.1016/s1734-1140(10)70240-1
77. Alonso de Vega JM, Díaz J, Serrano E, Carbonell LF. Oxidative Stress in Critically Ill Patients With Systemic Inflammatory Response Syndrome. Crit Care Med (2002) 30(8):1782–6. doi: 10.1097/00003246-200208000-00018
78. Mondal NK, Sorensen EN, Pham SM, Koenig SC, Griffith BP, Slaughter MS, et al. Systemic Inflammatory Response Syndrome in End-Stage Heart Failure Patients Following Continuous-Flow Left Ventricular Assist Device Implantation: Differences in Plasma Redox Status and Leukocyte Activation. Artif Organs (2016) 40(5):434–43. doi: 10.1111/aor.12580
79. Berger MM, Chioléro RL. Antioxidant Supplementation in Sepsis and Systemic Inflammatory Response Syndrome. Crit Care Med (2007) 35(9 Suppl):S584–90. doi: 10.1097/01.CCM.0000279189.81529.C4
80. Yahata T, Hamaoka K. Oxidative Stress and Kawasaki Disease: How Is Oxidative Stress Involved From the Acute Stage to the Chronic Stage? Rheumatology 56(1):6–13. doi: 10.1093/rheumatology/kew044
81. Ishikawa T, Seki K. The Association Between Oxidative Stress and Endothelial Dysfunction in Early Childhood Patients With Kawasaki Disease. BMC Cardiovasc Disord (2018) 18(1):30. doi: 10.1186/s12872-018-0765-9
82. Straface E, Gambardella L, Metere A, Marchesi A, Palumbo G, Cortis E, et al. Oxidative Stress and Defective Platelet Apoptosis in Naïve Patients With Kawasaki Disease. Biochem Biophys Res Commun (2010) 392(3):426–30. doi: 10.1016/j.bbrc.2010.01.040
83. Wu J, Yu M, Huang L, Qian Y, Kong M, Kang Z, et al. Association of MnSOD Gene Polymorphism With Susceptibility to Kawasaki Disease in Chinese Children. Cardiol Young (2021) 31(2):179–85. doi: 10.1017/S104795112000356X
84. Xu M, Qi Q, Men L, Wang S, Li M, Xiao M, et al. Berberine Protects Kawasaki Disease-Induced Human Coronary Artery Endothelial Cells Dysfunction by Inhibiting of Oxidative and Endoplasmic Reticulum Stress. Vasc Pharmacol (2020) 127:106660. doi: 10.1016/j.vph.2020.106660
Keywords: COVID-19, oxidative stress, Multisystem Inflammatory Syndrome in Children, inflammation, cytokines
Citation: Graciano-Machuca O, Villegas-Rivera G, López-Pérez I, Macías-Barragán J and Sifuentes-Franco S (2021) Multisystem Inflammatory Syndrome in Children (MIS-C) Following SARS-CoV-2 Infection: Role of Oxidative Stress. Front. Immunol. 12:723654. doi: 10.3389/fimmu.2021.723654
Received: 11 June 2021; Accepted: 20 September 2021;
Published: 19 October 2021.
Edited by:
Fabrice Cognasse, INSERM U1059 SAnté INgéniérie BIOlogie, FranceReviewed by:
Pio Conti, University of Studies G. d’Annunzio Chieti and Pescara, ItalyAdriana Bonomo, Oswaldo Cruz Foundation (Fiocruz), Brazil
Copyright © 2021 Graciano-Machuca, Villegas-Rivera, López-Pérez, Macías-Barragán and Sifuentes-Franco. This is an open-access article distributed under the terms of the Creative Commons Attribution License (CC BY). The use, distribution or reproduction in other forums is permitted, provided the original author(s) and the copyright owner(s) are credited and that the original publication in this journal is cited, in accordance with accepted academic practice. No use, distribution or reproduction is permitted which does not comply with these terms.
*Correspondence: Sonia Sifuentes-Franco, c2lmdWVudGVzX3NmMDVAaG90bWFpbC5jb20=