- 1Department of Blood Transfusion, The First Affiliated Hospital, Zhejiang University School of Medicine, Hangzhou, China
- 2Department of Pathology and Shenzhen Institute of Research and Innovation, The University of Hong Kong, Hong Kong, Hong Kong, SAR China
- 3Chongqing International Institute for Immunology, Chongqing, China
- 4Department of Blood Transfusion, Shaoxing People’s Hospital (Shaoxing Hospital, Zhejiang University School of Medicine), Shaoxing, China
- 5State Key Laboratory for Diagnosis and Treatment of Infectious Diseases, National Clinical Research Center for Infectious Diseases, Collaborative Innovation Center for Diagnosis and Treatment of Infectious Diseases, The First Affiliated Hospital, Zhejiang University School of Medicine, Hangzhou, China
- 6School of Basic Medical Science, Zhejiang Chinese Medical University, Hangzhou, China
Coronavirus disease 2019 (COVID-19), caused by the novel severe acute respiratory syndrome coronavirus 2 (SARS-CoV-2), is a serious infectious disease that has led to a global pandemic with high morbidity and mortality. High-affinity neutralizing antibody is important for controlling infection, which is closely regulated by follicular helper T (Tfh) cells. Tfh cells play a central role in promoting germinal center reactions and driving cognate B cell differentiation for antibody secretion. Available studies indicate a close relationship between virus-specific Tfh cell-mediated immunity and SARS-CoV-2 infection progression. Although several lines of evidence have suggested that Tfh cells contribute to the control of SARS-CoV-2 infection by eliciting neutralizing antibody productions, further studies are needed to elucidate Tfh-mediated effector mechanisms in anti-SARS-CoV-2 immunity. Here, we summarize the functional features and roles of virus-specific Tfh cells in the immunopathogenesis of SARS-CoV-2 infection and in COVID-19 vaccines, and highlight the potential of targeting Tfh cells as therapeutic strategy against SARS-CoV-2 infection.
Introduction
Severe acute respiratory syndrome coronavirus 2 (SARS-CoV-2), an emerging and acute novel coronavirus mainly transmitted via the respiratory tract, has rapidly caused pandemic-level cases of coronavirus disease 2019 (COVID-19), which has a high morbidity and mortality worldwide (1–5). Globally, as of 22 June 2021, there have been 178,503,429 confirmed cases of COVID-19, including 3,872,457 deaths from 195 countries and 28 regions according to the World Health Organization (WHO) report (6). SARS-CoV-2 is a serious threat to human health and life worldwide.
Humans who are immune-naive to SARS-CoV-2 are considered to be a major factor for the COVID-19 pandemic worldwide, and high-affinity neutralizing antibodies are especially essential for the control and clearance of SARS-CoV-2 infection (7–10). Several studies have reported sustained antibody responses in patients with SARS-CoV-2 infection, in which specific antibody titers are increased along with the progression of infection (11–13) (Figure 1). Notably, the titers of specific antibodies against SARS-CoV-2 are usually low in the first week. When the high cumulative seroconversion rate occurs between 2 and 3 weeks after symptom onset, the titers of neutralizing antibodies are significantly decreased in the early convalescent phase, with the titers of neutralizing antibodies not detectable in some patients, which indicate that several weeks may be needed to generate antibodies against SARS-CoV-2 (12–17). These findings suggest that further studies are needed to explore the production and function of neutralizing antibody inSARS-CoV-2 infection.
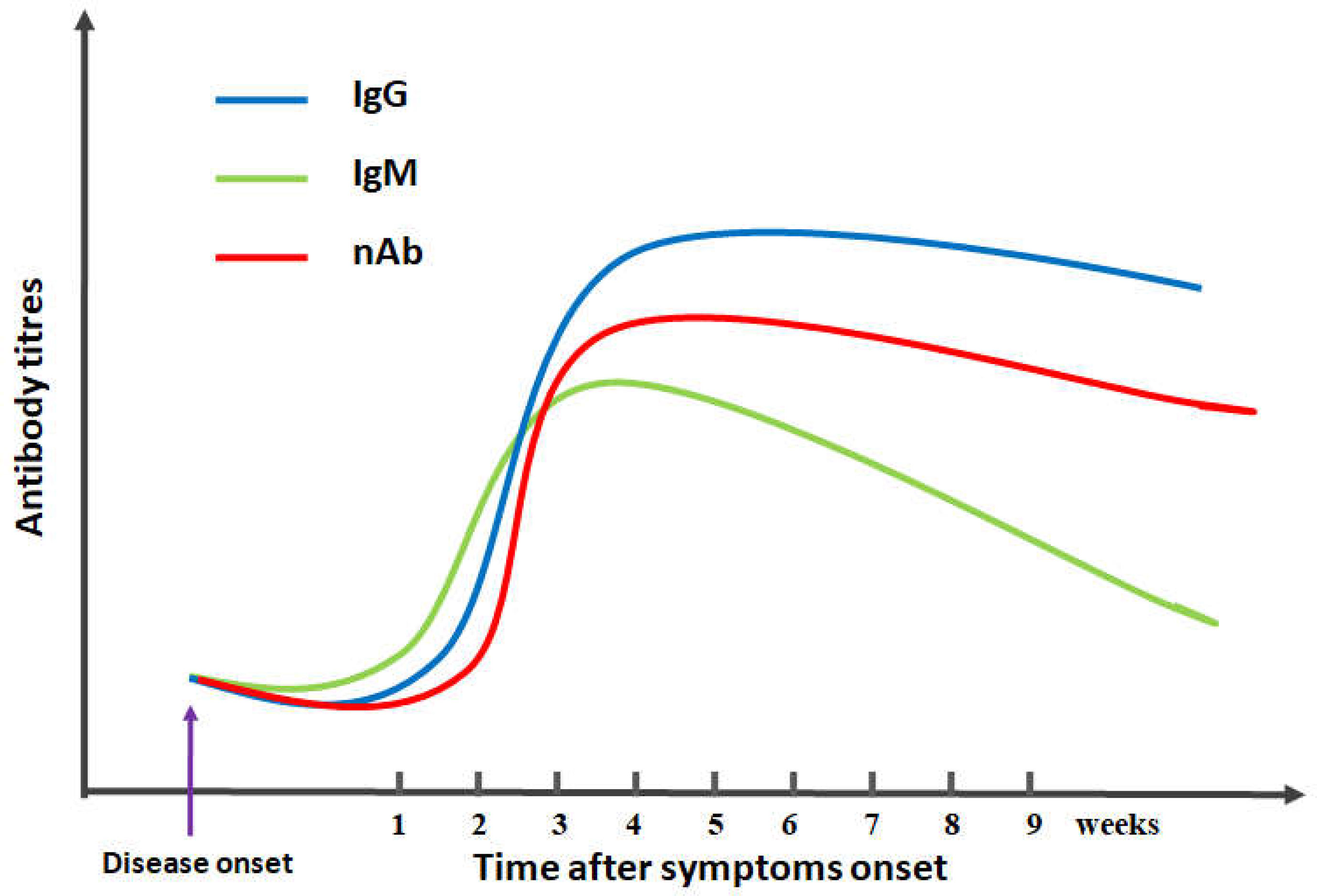
Figure 1 Schematic diagram of antibody kinetics in COVID-19 patients. IgG/IgM/nAb indicates IgG antibodies/IgM antibodies/neutralizing antibodies, respectively.
Antibody responses are closely correlated with CD4+T cell subsets that play important roles in the control of viral infections, including T helper (Th) 1 (Th1), Th2, and Th17 cells and follicular helper T (Tfh) cells (18, 19). Among CD4+Th cell subsets, naive CD4+T cells differentiated into Tfh cells can promote humoral immunity by mediating the interaction between T cells and B cells, which are essential for the control of viral infections and vaccine responses (19–21). Tfh cells, as a novel CD4+T cell subset, are characterized by the high expression of CXC chemokine receptor 5 (CXCR5), inducible T cell costimulator (ICOS), programmed cell death protein 1 (PD-1), B-cell lymphoma 6 (Bcl-6), and interleukin-21 (IL-21) in both mice and humans and can usually initiate B cells to differentiate into plasma cells that produce high-affinity antibodies to neutralize the virus, such as lymphocytic choriomeningitis virus (LCMV), influenza virus and hepatitis B virus (22–25). Loss of Tfh cell function can result in primary immunodeficiencies characterized by impaired humoral immunity, including COVID-19 infection, autosomal-dominant hyper IgE caused by STAT3 deficiency and common variable immunodeficiency (21, 25, 26). However, the roles and function features of Tfh cells in SARS-CoV-2 infection remain largely unclear (19, 20). Here, we will discuss the characteristics and functions of Tfh cells in the immunopathogenesis of SARS-CoV-2 infection and in COVID-19 vaccine responses, as well as their implications in eliciting effective immunity against SARS-CoV-2 infection.
The Phenotypes and Functions of Tfh Cells
Tfh cells can help B cells generate high-affinity antibodies, long-lived plasma cells, and memory B cells through functional markers (20, 21). The markers of Tfh cells are important to identify Tfh cells and their distinct subsets in the lymphoid tissue and circulation, which commonly include chemokine receptor CXCR5, transcription factor Bcl-6, PD-1, CD40 ligand (CD40L), and ICOS in humans and mice (25, 27–29). Moreover, the phenotypes of Tfh cells are associated with different stages of immune responses (30, 31). In secondary lymphoid organs, naïve CD4+T cells are differentiated into Tfh cells with the upregulation of CXCR5 and downregulation of CC-chemokine receptor 7 (CCR7), which are mediated by antigen-specific conventional dendritic cells (DCs) or monocyte-derived DCs (28, 32, 33). The increased CXCR5 and decreased CCR7contribute to the migration of Tfh cells toward CXC-chemokine ligand 13 (CXCL13)-enriched B lymphoid follicles in the germinal center (GC) (28, 34). The specific transcription factor Bcl-6 is selectively expressed in Tfh cells but is highly expressed in CXCR5hiCCR7low/-Tfh cells in human and mouse GCs (34–37). The IL-21 cytokine is highly and specifically secreted by Tfh cells, which promotes the proliferation of Tfh cells and helps B cell differentiation and antibody secretion, which is characteristic of Tfh cells (38–42). ICOS deficiency significantly reduces GC reactions and Tfh cells in mice and humans, which indicates that ICOS expressed in Tfh cells is essential for the differentiation and maintenance of Tfh cells, GC formation, B cell differentiation and antibody responses (43–45). ICOS, as a key costimulatory molecule, can also induce the secretion of IL-21 in Tfh cells (45–47). HighPD-1 expression on Tfh cells can significantly promote the differentiation and activity of Tfh cells (48–50). Collectively, Tfh cells are commonly identified as having three phenotypes: canonical GC Tfh cells with PD-1++ and ICOS++Bcl-6+CCR7-CXCR5++CD4+T cells, precursor-Tfh (Pre-Tfh) cells characterized as PD-1+ICOS+Bcl-6lowCCR7lowCXCR5+CD4+T cells, and memory Tfh cells similar to Pre-Tfh cells in lymphoid tissue (36, 50–52). In GC, Tfh cells are responsible for regulating B cell differentiation into memory B cells and plasma cells, controlling the selection of high-affinity antibody production and the development of long-term humoral immunity (53–56).
Circulating Tfh (cTfh) cells in the peripheral blood are usually composed of two distinctive phenotypes: effector memory Tfh cells (PD-1+ICOS+CCR7lowBCL-6-CXCR5+CD4+T cells) and central memory Tfh cells (PD-1-ICOS-CCR7highBCL-6-CXCR5+CD4+T cells) (32, 57, 58). Additionally, based on the expression of CXCR3 and CCR6, cTfh cells are further divided into three subsets: Tfh1 (CXCR3+CCR6-), Tfh2 (CXCR3-CCR6-), Tfh17 (CXCR3-CCR6+), and Tfh1/17 (CXCR3+CCR6+) cells, which share the signature transcription factors and cytokines of Th1 (T-bet and IFN-γ), Th2 (GATA3, IL-4, IL-5 and IL-13), Th17 (RORγt, IL-17 and IL-22) cells, respectively (32, 58, 59). cTfh2 and cTfh17 cells can induce B cell differentiation and antibody secretion and regulate immunoglobulin (Ig) isotype switching. cTfh1 cells are commonly considered not to be a helper for B cells, but ICOS+PD-1highCCR7lowcTfh1 cells effectively regulate B cell differentiation and induce antibody responses (59–65). These studies display functionally distinct cTfh cell subsets based on ICOS, PD-1, and CCR7 expression, as well as CXCR3 and CCR6. Moreover, these novel subsets are different from Th1, Th2 and Th17 cells but share some of their characteristics. Additionally, Tfh-like cells have also been identified in non-lymphoid tissues, including the synovium of arthritis, skin and salivary glands of patients, which commonly express low or undetectable CXCR5 and Bcl-6 and high PD-1, ICOS, OX40 and IL-21 compared to Tfh cells in secondary lymphoid organs, which also express tissue-specific chemokine receptors, including CCR2, CCR5, CX3C-chemokinereceptor 1 (CX3CR1) and CXCR4 (52, 66–71). Recently, Tfh13 cells, a novel Tfh cell subset that secretes IL-4 and IL-13, were shown to be responsible for IgE production in human and mouse allergies and to highly express the transcription factors Bcl-6 and GATA3 (72–74). Current studies indicate that distinct phenotypes of Tfh cells are critical for B cell differentiation and high-affinity antibody production (Table 1). Interestingly, follicular regulatory T (Tfr) cells are considered a subset of Foxp3+Treg cells in the GC that are initiated from Foxp3+/- precursors but not from Tfh cells (75–78). Tfr cells share canonical Tfh cell molecules, including CXCR5, Bcl-6, PD-1 and ICOS, as well as Treg cell molecules, including CD25, Foxp3, Blimp-1 and CTLA-4 (79–82). Importantly, Tfr cells, similar to Treg cells, play a critical role in immunosuppression, rather than Tfh cells, which can limit GC responses and suppress the activation of Tfh cells and B cells within GCs through inhibitory molecules, including CTLA-4, PD-1, IL-10 and TGF-β secretion. The balance of Tfh/Tfr cells is essential to maintain immune homeostasis and mediate humoral immunity (63, 66, 82–85).
The Differentiation of Tfh Cells
Tfh cell differentiation is regulated by multiple complex factors and stages. Naïve CD4+T cells are primed by binding their T cell receptors with peptide-loaded major histocompatibility complex (MHC) class II (pMHC-II) on professional antigen-presenting cells (APCs), such as DCs and monocytes. Strong TCR signaling and continuous antigenic stimulation play critical roles in favoring Tfh cell differentiation by upregulating BATF to promote Bcl-6 expression (86–90). The early differentiation of Tfh cells is sufficiently initiated by DCs predominantly localized to T cell zones of lymphoid organs, which are considered Pre-Tfh cells that upregulate Bcl-6 and CXCR5 and repress CCR7 expression, and Bcl6+CXCR5+Pre-Tfh cells are attracted by the chemokine CXCL13 (CXCR5 ligand) produced within the B cell follicle zones toward the T-B border (36, 64, 91–94). Pre-Tfh cells migrate to the T-B cell border and interact with cognate B cells to further upregulate Bcl-6, CXCR5, ICOS, PD-1 and IL-21 and downregulate CCR7 expression, which further drives GC-Tfh differentiation and maturation and GC formation. These processes also require available costimulatory molecules and cytokines, including ICOS-ICOSL, OX40-OX40L, PD-1-PD-Ll/2, CD40-CD40L, IL-21, IL-6 and IL-12 cytokines (25, 32, 36, 95–102).
The transcription factor Bcl-6 in CD4+T cells is mostly essential for Tfh differentiation and function, and loss of Bcl-6 represses Tfh differentiation, GC formation, B cell differentiation and antibody responses (34, 35, 43). Bcl6-expressing Tfh cells are also regulated by multiple transcription factors, including positive inductors such as TCF-1 and LEF-1, BATF, NOTCH1/2, and IRF4 and negative regulators such as Blimp-1, FOXO1 and STAT5 (22, 25, 32, 103–110). Some costimulatory molecules expressed on Tfh cells are considered markers of Tfh cells, including ICOS, OX40, PD-1 and CD40L, which can also induce Tfh cell differentiation and maintenance (32, 111, 112). In GC, B cells highly express costimulatory ligands, including ICOSL, CD80, CD86, PD-L1, and PD-L2, which contribute to the maintenance of Tfh cells, and then Tfh cells also mutually promote B cells to differentiate into plasma cells to further produce specific antibodies that mediate humoral immune responses (113, 114). Bcl-6 induces secretion of the cytokine IL-21, which can promote Tfh cell differentiation by upregulating STAT-1 and STAT-3 signals to further induce Bcl-6 expression, and similarly, the cytokine IL-6 plays a critical role in Tfh cell differentiation by upregulating the STAT1/3-Bcl-6 signal axis (56, 85, 115, 116). In addition, Tfh1 cells are characterized by IL-21 and IFN-γ production, and Tfh1 cell differentiation characterized by increased T-bet and Bcl-6 expression is initiated by phosphorylation of STAT1 and STAT4 in CD4+T cells that are induced through IL-12, which is partially inhibited by a high concentration of IL-2 that reduces Bcl-6 expression (85, 115–120). Tfh2 cells are characterized by IL-4 and IL-21 production; Tfh2 cell differentiation is driven by IL-4 but suppressed by IL-6 via STAT3 signaling, and IL-4-secreted Tfh2 cells contribute to humoral immunity (85, 121–123). Tfh17 cells are characterized by IL-21 and IL-17 production; Tfh17 cell differentiation is primed by IL-23, IL-21, ICOS, TGF-β and IL-6, which upregulate Bcl-6 and RORγt expression. Consistent with its well established role in driving B cell response during infection, IL-17 secreted by Tfh17 cells can promote interactions of cognate T-B cells in the GC, inducing the formation of spontaneous GC and Ig isotype class-switching (124–127). However, low doses of IL-2, TGF-β and CTLA-4 promote the development of Tfr cells that play critical roles in inhibiting Tfh cell differentiation and GC responses by activating STAT5, Blimp-1, and Bach2 transcription factors in Tfr cells characterized by CXCR5+Foxp3+CD4+T cells (128–133). Tfr cells can inhibit Tfh cell and plasma cell differentiation by inhibitory molecules, including CTLA-4, IL-10 and TGF-β; conversely, Tfh cells also inhibit the expansion of Tfr cells by the IL-21 cytokine (27, 131–139). This suggests that the balance of Tfh and Tfr cells plays a critical role in regulating B cell differentiation and specific antibody production (140).
Tfh Cells in SARS-CoV-2 Infection and Vaccine
Currently, the SARS-CoV-2 infection pandemic has led to a serious threat to human health worldwide. Neutralizing antibodies of humoral immunity play a critical role in vaccine responses and battles against infectious viruses, including SARS-CoV-2, which is closely associated with Tfh cells differentiation and function (18, 19, 21, 141–144) (Figure 2). The role and function of Tfh cells in the control and clearance of SARS-CoV-2 infection and in the development of new vaccines have been investigated.
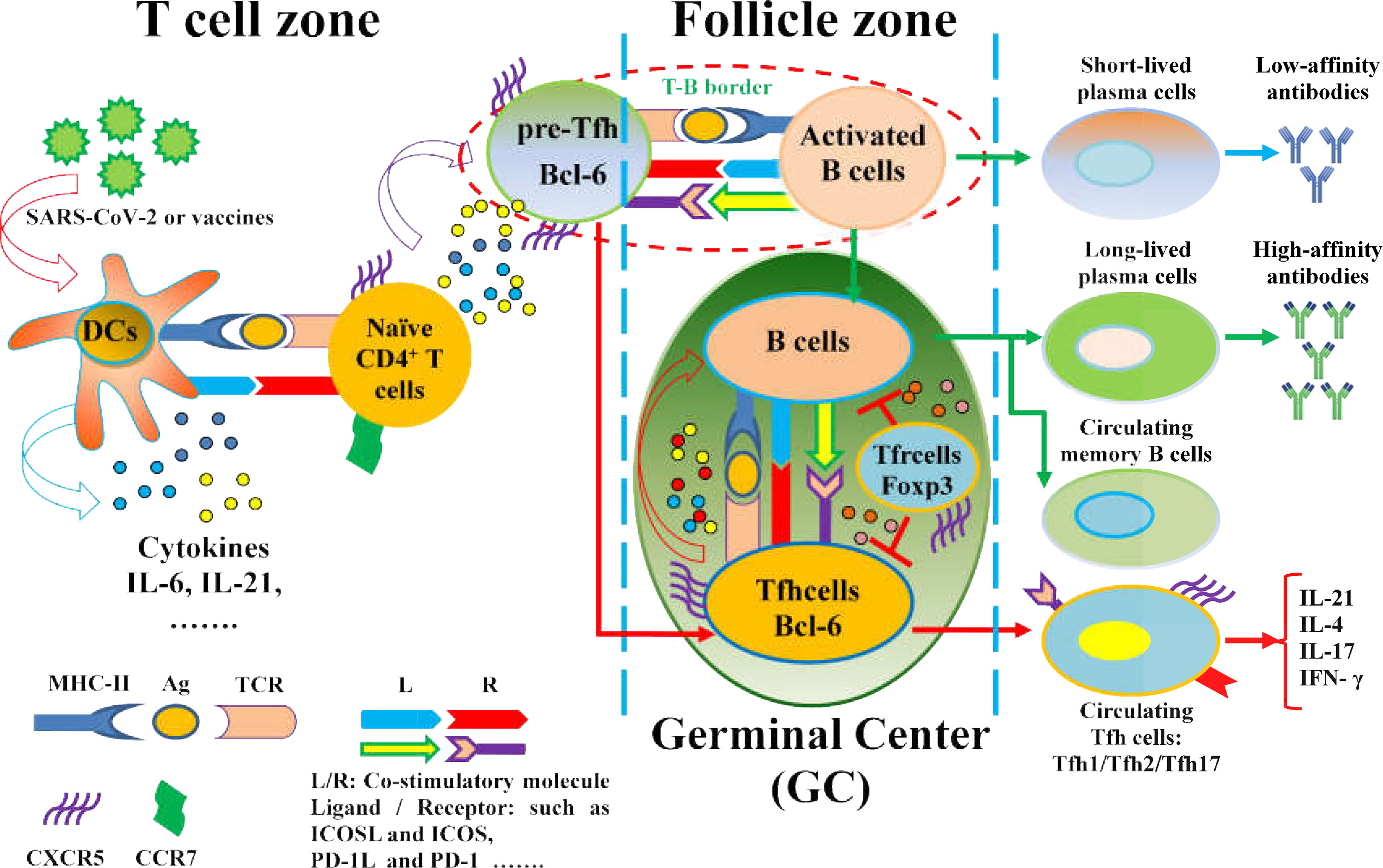
Figure 2 The regulation of Tfh cell differentiation and function in SARS-CoV-2 infection and vaccines. Naïve CD4+ T cells are driven by APCs (DCs) upon exposure to SARS-CoV-2 or virus antigens, which are activated toward antigen-specific Pre-Tfh cells with upregulation of CXCR5 and Bcl-6 and downregulation of CCR7 under the interaction of MHC-II molecules on DCs and cognate TCR on CD4+T cells, as well as the expression of costimulatory molecules and cytokine production. Pre-Tfh cells interact with activated B cells at the T-B border in the follicle zone, which further differentiate into various Tfh cell subsets that migrate to the GC, where Tfh cells promote B cell differentiation and specific antibody production. However, the loss of GC structures reduces Bcl-6+Tfh cells in severe COVID-19 patients. Notably, SARS-CoV-2-specific Tfh cells are expanded in mild and asymptomatic patients with COVID-19.Moreover, vaccines can efficiently induce Tfh cell differentiation, GC formation, and protective antibody responses.
Previous reports showed that the frequencies of cTfh cells characterized byCXCR5+ICOS+PD-1+progressively increased up to 20 days from the onset of infection in a case with non-severe convalescent COVID-19, in addition to elevated specific plasma SARS-CoV-2-binding IgM and IgG antibodies (145). Single-cell analysis revealed that expanded frequencies of cTfh cells were found in patients with active COVID-19 disease, as well as a high percentage of specific anti-SARS-CoV-2 antibodies, including IgA and IgG (146). The frequencies of spike (S)-specific cTfh cells (CD3+CD4+CD45RA–CXCR5+) are consistently elicited after S peptide stimulation in convalescent COVID-19 cases and exhibit a clear phenotypic bias to aCCR6+CXCR3-cTfh17 cell phenotype; however, neutralizing activity is inversely correlated with S-specific cTfh17 cell frequencies but positively correlated with S-specific cTfh, cTfh1 (CCR6-CXCR3+) and cTfh2 (CCR6-CXCR3-) cell frequencies (147). Previous reports suggested that expanded CXCR3+cTfh1 cells positively correlated with the neutralizing antibody response against influenza vaccination and live-attenuated yellow fever vaccination (148, 149). A recent study showed that increased frequencies of CCR7lowPD-1+cTfh-effectormemory (em), cTfh1 and cTfh2 cells in CXCR5+CD45RA-CD25-CD4+T cells are significantly increased, as well as high IL-1β and TNF-α, and that the frequencies of cTfh1 cells are associated with SARS-CoV-2-specific IgG/IgM antibodies, although CCR7highPD-1-cTfh-central memory (cm) and cTfh17 cells in CXCR5+CD45RA-CD25-CD4+T cells are decreased, as well as cTfr cells in Treg cells in convalescent patients compared to healthy subjects. Moreover, the frequencies of high cTfh-em, low cTfh-cm and cTfr cells are positively correlated with disease severity (150). These observations indicated that cTfh cell phenotypes can induce potent neutralizing responses against SARS-CoV-2 in COVID-19convalescent patients, which will contribute to antibody-based therapeutics and vaccination design for COVID-19.
Additionally, increased frequencies of virus-specific cTfh cells (CD4+CXCR5+OX40+CD40L+) were observed in acute and convalescent COVID-19 cases, and the frequencies of both SARS-CoV-2-specific cTfh cells and S-specific CCR6+CXCR3-cTfh17 cells were closely associated with low disease severity (151). Longitudinal studies on COVID-19 infection and convalescent subjects indicate that the levels of SARS-CoV-2 antibodies are low and insufficient in humoral immunity response, although the underlying mechanism is poorly understood (11–14). The numbers of CD4+CXCR5+Tfh, ICOS+Tfh, Bcl-6+Tfh and Bcl-6+B cells are decreased in lymph nodes and spleens, which are possibly associated with exclusively abundantTh1 cells, increased Treg cells (but not Tfr cells) and aberrant TNF-α production in COVID-19 lymph nodes in COVID-19 patients, as well as loss of GCs in lymph nodes and spleens from acute and dead COVID-19 patients (26, 152, 153). These data indicated that defective Tfh cell generation and dysregulated humoral immunity provide a possible mechanistic explanation for the limited durability of antibody responses in COVID-19 disease. Furthermore, low frequencies of CD45RA-PD-1+CXCR5+cTfh cells were also observed, but elevated frequencies of activated cTfh (CD38+ICOS+) cells were positively correlated with anti-SARS-CoV-2 IgM and IgG titers in hospitalized COVID-19 patients (154). These findings indicated that activated cTfh cells may be more reflective of recent antigen encounter and emigration from the GCs. Additionally, a single-cell transcriptomic analysis revealed that increased proportions of cytotoxic cTfh cells in hospitalized COVID-19 patients early in the illness are negatively correlated with the IgG levels of anti-spike protein antibodies to SARS-CoV-2, although the total SARS-reactive cTfh cells show a positive correlation with anti-spike antibody levels in hospitalized COVID-19 patients but not in non-hospitalized COVID-19 patients, which provided insights into cytotoxic cTfh cells in the distinct disease severities of COVID-19 patients (155). Moreover, reduced cTfh and PD-1+cTfh and increased exhausted TIM‐3+cTfh cell frequencies are significantly observed, but the correlations between cTfh cells and anti-SARS-CoV-2IgM and IgG titers were not analyzed in hospitalized COVID-19 patients (156). These results indicated that cytotoxic cTfh and exhausted cTfh cells may inhibit specific anti-SARS-CoV-2 antibody production, which plays a critical role in severe SARS-CoV-2 infection (157). In a recent cohort study of COVID-19 patients within six months of recovery, the CXCR5+CD4+cTfh cell frequencies were significantly higher in COVID-19 patients in the long-term clinically recovered (20∼26 weeks) cohort (LCR) than in those in the short-time clinically recovered (4∼9 weeks) cohort (SCR). However, the frequencies of cTfh cells in both the LCR and SCR cohorts were lower than those in the healthy donor cohort (HD). Moreover, three cTfh subsets were similar between the LCR and HD cohorts; cTfh1 cell frequencies in the SCR cohort were shown to be significantly low, but cTfh2 and cTfh17 subsets were found to be high compared with the LCR and HD cohorts (158). Virus-specific Tfh cell frequencies, memory B cell responses, and serum CXCL13 levels were not different between asymptomatic or mild symptomatic COVID-19 patients. In contrast, COVID-19 patients with moderate or severe disease exhibited vigorous virus-specific GC B cell responses and Tfh cell responses. Moreover, potent virus-specific Th1 and CD8+T cell responses were observed in asymptomatic or mildly symptomatic patients but not in severely symptomatic patients. These data suggest that asymptomatic and mild patients have weak and transient SARS-CoV-2 antibody responses (159).
During acute COVID-19 infection, expanded activated CD38+HLA-DR+PD-1+ICOS+CXCR5+CD4+cTfh cells, CD38+HLA-DR+CXCR3+cTfh1 cells, and activated CD38+HLA-DR+Th1 cells emerged, together with cytotoxic CD8+T cells. The number of activated cTfh1 cells positively correlated with the levels of RBD- and spike-specific antibodies, including IgG, IgA and IgM isotypes (160). These data indicated that activated cTfh cell responses were associated with robust antibody responses elicited during SARS-CoV-2 infection, which may be valuable as potential biomarkers in vaccine clinical trials. Similarly, CD38+HLA-DR+cTfh cells, activated CD4+T cells and cytotoxic CD8+T cells were expanded in COVID-19 patients, and increased CD38+HLA-DR+cTfh cells indicated a recent antigen encounter and emigration from the GC of the patients (161). The frequencies of PD-1+ICOS+cTfh, cytotoxic CD4+T and exhausted T cells were strongly expanded in COVID-19 patients, particularly in severe patients compared to healthy individuals, which suggested that extensive T cell dysfunction was associated with COVID-19 severity (162). In severe COVID-19 patients, the frequencies of CCR6+cTfh cells and CCR4+cTfh cells were expanded, but CCR3+cTfh cells and Th1 cells were low in severe COVID-19 patients compared to healthy individuals (163). The frequencies of PD-1+ICOS+cTfh cells, activated cTfh cells and cytotoxic CD8+T cells were strongly upregulated in COVID-19 patients, particularly in severe patients compared to healthy donors. Moreover, an increase in CD4+CD127-CD25+Treg cells was found in mild patients, and upregulation of CCR4 in activated CD8+T cells indicated enhanced lung homing in severe COVID-19 patients (164). Additionally, in rhesus macaques, SARS-CoV-2 infection induces predominantly GC CXCR3+Tfh cells (but not a PD-1++Foxp3+Tfr cell subset) specific for the SARS-CoV-2 spike and nucleocapsid proteins and produce high titers of antiviral serum IgG and IgM antibodies againstSARS-CoV-2 (165) (Table 2). These data indicated that variable Tfh cell subsets dysregulated the humoral immune responses in COVID-19 patients caused by SARS-CoV-2 infection.
The COVID-19 pandemic continues to spread worldwide, and a safe and protective vaccine is urgently needed to effectuate herd protection and control of SARS-CoV-2. Currently, rapid advances have been made in the design and development of SARS-CoV-2 vaccines, such as inactivated vaccines, DNA vaccines, mRNA vaccines and specific SARS-CoV-2 proteins (166). mRNA-1273 vaccine could significantly induce Th1 and interleukin-21-producing CXCR5+PD−1+ICOS+Tfh cell responses, and elicit robust SARS-CoV-2 neutralizing activity, which provided rapid protection in the upper and lower airways from SARS-CoV-2 infection in Rhesus Macaques (167). When compared to SARS-CoV-2 with recombinant SARS-CoV-2 receptor-binding domain (rRBD) formulated with AddaVax (rRBD-AddaVax) protein vaccine, the SARS-CoV-2 mRNA vaccines encoding RBD and full-length spike protein efficiently induce SARS-CoV-2-specific GC B cell and Tfh cell responses, which promoted specific neutralizing antibody production in vaccinated mice. Interestingly, the rRBD-AddaVax vaccine could elicit high frequencies of IL-4+ Tfh cells (168). In human vaccination, the BNT162b2 mRNA vaccine for SARS-CoV-2 had significantly elicited AIM+CXCR5+CD45RA-CD3+cTfh cell responses, AIM (activation induced marker) cells include CD69+OX40+ or CD69+CD40L+ orCD69+4-1BB+ or OX40+4-1BB+ or CD40L+4-1BB+ or CD40L+OX40+cells, and the frequency of AIM+cTfh cells is positively correlated with anti-Spike-specific IgA and IgG antibody titers (169). These findings have indicated that SARS-CoV-2 mRNA vaccines can effectively promote antigen-specific Tfh cell differentiation, B cell responses and the generation of protective antibodies, which are considered as promising candidates for eliciting high-quality adaptive immune responses to control and clear SARS-CoV-2 infection. Additionally, the specific protein vaccines including SARS-CoV-2 subunit vaccine (NVX-CoV2373) with the full-length spike (S) protein, StriFK-FH002C and Spike (S)/receptor binding domain (RBD) protein subunit vaccine significantly induce specific cTfh cell and GC B cell responses, resulting in high neutralizing antibody titers of SARS-CoV-2 (170–172) (Table 3). Various clinical trials in humans indicate that inactivated SARS-CoV-2 vaccines can induce satisfactory high neutralizing antibody titers that notably reduce the number of patients with severe COVID-19 (173–176). These data suggested that SARS-CoV-2 vaccines can safely and effectively promote humoral immune responses, enhance neutralizing antibody titers, and reduce the incidence and mortality of critically ill patients.
Conclusions
Tfh cells and associated molecules play a critical role in the development of viral infection, and Tfh cell subsets are required for high-quality neutralizing antibodies from B cells to control and clear viruses including SARS-CoV-2, which can effectively promote humoral immune responses. Emerging evidence indicates that functional characterization of Tfh cells and their subsets will provide novel insights into improved vaccine design and therapeutic strategies to prevent and control various viral infections including SARS-CoV-2 infection.
Author Contributions
DC drafted the manuscript and designed the figures and tables. FX, QJ, DJ, YL, DX, and JW revised the manuscript. DC, JX, CW, and LL conceived the topic and revised the manuscript. All authors contributed to the article and approved the submitted version.
Funding
This work was supported by the National Natural Science Foundation of China (Grant Nos. 81871709, 81971994, 82071817 and 91846103), Funding for Chongqing International Institute for Immunology (2020YJC10), Hong Kong Research Grants Council General Research Fund (17113319) and Theme-Based Research Scheme (T12-703/19R), Zhejiang Provincial Key Research and Development Program (Grant No. 2020C03032).
Conflict of Interest
The authors declare that the research was conducted in the absence of any commercial or financial relationships that could be construed as a potential conflict of interest.
Publisher’s Note
All claims expressed in this article are solely those of the authors and do not necessarily represent those of their affiliated organizations, or those of the publisher, the editors and the reviewers. Any product that may be evaluated in this article, or claim that may be made by its manufacturer, is not guaranteed or endorsed by the publisher.
Acknowledgments
We gratefully appreciate the highly qualified native English speaking editors at American Journal Experts (AJE) for providing reputable English language editing service (Verification code:6107-48C6-A470-D97E-899B) for our manuscript.
References
1. Zhu N, Zhang D, Wang W, Li X, Yang B, Song J, et al. A Novel Coronavirus From Patients With Pneumonia in China, 2019. N Engl J Med (2020) 382(8):727–33. doi: 10.1056/NEJMoa2001017
2. Chan JF, Yuan S, Ko k, To KK, Chu H, Yang J, et al. A Familial Cluster of Pneumonia Associated With the 2019 Novel Coronavirus Indicating Person-to-Person Transmission: A Study of a Family Cluster. Lancet (2020) 395(10223):514–23. doi: 10.1016/S0140-6736(20)30154-9
3. Huang C, Wang Y, Li X, Ren L, Zhao J, Hu Y, et al. Clinical Features of Patients Infected With 2019 Novel Coronavirus in Wuhan, China. Lancet (2020) 395(10223):497–506. doi: 10.1016/S0140-6736(20)30183-5
4. Holshue ML, DeBolt C, Lindquist S, Lofy KH, Wiesman J, Bruce H, et al. First Case of 2019 Novel Coronavirus in the United States. N Engl J Med (2020) 382(10):929–36. doi: 10.1056/NEJMoa2001191
5. Zheng S, Fan J, Yu F, Feng B, Lou B, Zou Q, et al. Viral Load Dynamics and Disease Severity in Patients Infected With SARS-CoV-2 in Zhejiang Province, China, January-March 2020: Retrospective Cohort Study. BMJ (2020) 369:m1443. doi: 10.1136/bmj.m1443
6. World Health Organization. Coronavirus (COVID-19) Dashboard. Available at: https://covid19.who.int/ (Accessed 22June 2021).
7. Lai S, Ruktanonchai NW, Zhou L, Prosper O, Luo W, Floyd JR, et al. Effect of non-Pharmaceutical Interventions to Contain COVID-19 in China. Nature (2020) 585(7825):410–3. doi: 10.1038/s41586-020-2293-x
8. Sun K, Wang W, Gao L, Wang Y, Luo K, Ren L, et al. Transmission Heterogeneities, Kinetics, and Controllability of SARS-CoV-2. Sci (New York NY) (2021) 371(6526):eabe2424. doi: 10.1126/science.abe2424
9. Wang W, Wu Q, Yang J, Dong K, Chen X, Bai X, et al. Global, Regional, and National Estimates of Target Population Sizes for Covid-19 Vaccination: Descriptive Study. BMJ (2020) 371:m4704. doi: 10.1136/bmj.m4704
10. Krause PR, Fleming TR, Ellenberg SS, Henao-Restrepo AM, Group WHOAHCTE. Maintaining Confidentiality of Emerging Results in COVID-19 Vaccine Trials Is Essential. Lancet (2020) 396(10263):1611–3. doi: 10.1016/S0140-6736(20)32259-5
11. Chen X, Chen Z, Azman AS, Deng X, Sun R, Zhao Z, et al. Serological Evidence of Human Infection With SARS-CoV-2: A Systematic Review and Meta-Analysis. Lancet Glob Health (2021) 9(5):e598–609. doi: 10.1016/S2214-109X(21)00026-7
12. Lou B, Li TD, Zheng SF, Su YY, Li ZY, Liu W, et al. Serology Characteristics of SARS-CoV-2 Infection After Exposure and Post-Symptom Onset. Eur Respir J (2020) 56(2):2000763. doi: 10.1183/13993003.00763-2020
13. Seow J, Graham C, Merrick B, Acors S, Pickering S, Steel KJA, et al. Longitudinal Observation and Decline of Neutralizing Antibody Responses in the Three Months Following SARS-CoV-2 Infection in Humans. Nat Microbiol (2020) 5(12):1598–607. doi: 10.1038/s41564-020-00813-8
14. Fernández-Barat L, López-Aladid R, Torres A. The Value of Serology Testing to Manage SARS-CoV-2 Infections. Eur Respir J (2020) 56(2):2002411. doi: 10.1183/13993003.02411-2020
15. Stringhini S, Wisniak A, Piumatti G, Azman AS, Lauer SA, Baysson H, et al. Seroprevalence of Anti-SARS-CoV-2 IgG Antibodies in Geneva, Switzerland (SEROCoV-POP): A Population-Based Study. Lancet (2020) 396(10247):313–9. doi: 10.1016/S0140-6736(20)31304-0
16. Fu Y, Li Y, Guo E, He L, Liu J, Yang B, et al. Dynamics and Correlation Among Viral Positivity, Seroconversion, and Disease Severity in COVID-19 : A Retrospective Study. Ann Intern Med (2021) 174(4):453–61. doi: 10.7326/M20-3337
17. Li K, Huang B, Wu M, Zhong A, Li L, Cai Y, et al. Dynamic Changes in Anti-SARS-CoV-2 Antibodies During SARS-CoV-2 Infection and Recovery From COVID-19. Nat Commun (2020) 11(1):6044. doi: 10.1038/s41467-020-19943-y
18. Greczmiel U, Oxenius A. The Janus Face of Follicular T Helper Cells in Chronic Viral Infections. Front Immunol (2018) 9:1162. doi: 10.3389/fimmu.2018.01162
19. Kervevan J, Chakrabarti LA. Role of CD4+ T Cells in the Control of Viral Infections: Recent Advances and Open Questions. Int J Mol Sci (2021) 22(2):523. doi: 10.3390/ijms22020523
20. Luo W, Yin Q. B Cell Response to Vaccination. Immunol Invest (2021) 50(7):780–801. doi: 10.1080/08820139.2021.1903033
21. Vella LA, Herati RS, Wherry EJ. CD4(+) T Cell Differentiation in Chronic Viral Infections: The Tfh Perspective. Trends Mol Med (2017) 23(12):1072–87. doi: 10.1016/j.molmed.2017.10.001
22. Xu L, Cao Y, Xie Z, Huang Q, Bai Q, Yang X, et al. The Transcription Factor TCF-1 Initiates the Differentiation of T(FH) Cells During Acute Viral Infection. Nat Immunol (2015) 16(9):991–9. doi: 10.1038/ni.3229
23. Du B, Teng J, Yin R, Tian Y, Jiang T, Du Y, et al. Increased Circulating T Follicular Helper Cells Induced via IL-12/21 in Patients With Acute on Chronic Hepatitis B Liver Failure. Front Immunol (2021) 12:641362. doi: 10.3389/fimmu.2021.641362
24. Chen DY, Wolski D, Aneja J, Matsubara L, Robilotti B, Hauck G, et al. Hepatitis C Virus-Specific CD4+ T Cell Phenotype and Function in Different Infection Outcomes. J Clin Invest (2020) 130(2):768–73. doi: 10.1172/JCI126277
25. Vinuesa CG, Linterman MA, Yu D, MacLennan IC. Follicular Helper T Cells. Annu Rev Immunol (2016) 34:335–68. doi: 10.1146/annurev-immunol-041015-055605
26. Duan YQ, Xia MH, Ren L, Zhang YF, Ao QL, Xu SP, et al. Deficiency of Tfh Cells and Germinal Center in Deceased COVID-19 Patients. Curr Med Sci (2020) 40(4):618–24. doi: 10.1007/s11596-020-2225-x
27. Xu L, Huang Q, Wang H, Hao Y, Bai Q, Hu J, et al. The Kinase Mtorc1 Promotes the Generation and Suppressive Function of Follicular Regulatory T Cells. Immunity (2017) 47(3):538–51.e5. doi: 10.1016/j.immuni.2017.08.011
28. Crotty S. T Follicular Helper Cell Biology: A Decade of Discovery and Diseases. Immunity (2019) 50(5):1132–48. doi: 10.1016/j.immuni.2019.04.011
29. Xiao F, Han M, Rui K, Ai X, Tian J, Zhang W, et al. New Insights Into Follicular Helper T Cell Response and Regulation in Autoimmune Pathogenesis. Cell Mol Immunol (2021) 18(6):1610–2. doi: 10.1038/s41423-021-00688-7
30. Qi H. T Follicular Helper Cells in Space-Time. Nat Rev Immunol (2016) 16(10):612–25. doi: 10.1038/nri.2016.94
31. Song W, Craft J. T Follicular Helper Cell Heterogeneity: Time, Space, and Function. Immunol Rev (2019) 288(1):85–96. doi: 10.1111/imr.12740
32. Deng J, Wei Y, Fonseca VR, Graca L, Yu D. T Follicular Helper Cells and T Follicular Regulatory Cells in Rheumatic Diseases. Nat Rev Rheumatol (2019) 15(8):475–90. doi: 10.1038/s41584-019-0254-2
33. Shao F, Zheng P, Yu D, Zhou Z, Jia L. Follicular Helper T Cells in Type 1 Diabetes. FASEB J (2020) 34(1):30–40. doi: 10.1096/fj.201901637R
34. Kroenke MA, Eto D, Locci M, Cho M, Davidson T, Haddad EK, et al. Bcl6 and Maf Cooperate to Instruct Human Follicular Helper CD4 T Cell Differentiation. J Immunol (2012) 188(8):3734–44. doi: 10.4049/jimmunol.1103246
35. Yu D, Rao S, Tsai LM, Lee SK, He Y, Sutcliffe EL, et al. The Transcriptional Repressor Bcl-6 Directs T Follicular Helper Cell Lineage Commitment. Immunity (2009) 31(3):457–68. doi: 10.1016/j.immuni.2009.07.002
36. He J, Tsai LM, Leong YA, Hu X, Ma CS, Chevalier N, et al. Circulating Precursor CCR7(lo)PD-1(Hi) CXCR5(+) CD4(+) T Cells Indicate Tfh Cell Activity and Promote Antibody Responses Upon Antigen Reexposure. Immunity (2013) 39(4):770–81. doi: 10.1016/j.immuni.2013.09.007
37. Ueno H, Banchereau J, Vinuesa CG. Pathophysiology of T Follicular Helper Cells in Humans and Mice. Nat Immunol (2015) 16(2):142–52. doi: 10.1038/ni.3054
38. Linterman MA, Beaton L, Yu D, Ramiscal RR, Srivastava M, Hogan JJ, et al. IL-21 Acts Directly on B Cells to Regulate Bcl-6 Expression and Germinal Center Responses. J Exp Med (2010) 207(2):353–63. doi: 10.1084/jem.20091738
39. Akiyama M, Suzuki K, Yoshimoto K, Yasuoka H, Kaneko Y, Takeuchi T. Peripheral TIGIT+ T Follicular Helper Cells That Produce High Levels of Interleukin-21 via OX40 Represent Disease Activity in IgG4-Related Disease. Front Immunol (2021) 12:651357. doi: 10.3389/fimmu.2021.651357
40. Yang J, Geng L, Ma Y, Tang X, Peng H, Tian J, et al. SLAMs Negatively Regulate IL-21 Production in Tfh-Like Cells From Allergic Rhinitis Patients. J Asthma Allergy (2021) 14:361–9. doi: 10.2147/JAA.S291879
41. Pontarini E, Murray-Brown WJ, Croia C, Lucchesi D, Conway J, Rivellese F, et al. Unique Expansion of IL-21+ Tfh and Tph Cells Under Control of ICOS Identifies Sjogren’s Syndrome With Ectopic Germinal Centres and MALT Lymphoma. Ann Rheum Dis (2020) 79(12):1588–99. doi: 10.1136/annrheumdis-2020-217646
42. Olatunde AC, Hale JS, Lamb TJ. Cytokine-Skewed Tfh Cells: Functional Consequences for B Cell Help. Trends Immunol (2021) 42(6):536–50. doi: 10.1016/j.it.2021.04.006
43. Crotty S. T Follicular Helper Cell Differentiation, Function, and Roles in Disease. Immunity (2014) 41(4):529–42. doi: 10.1016/j.immuni.2014.10.004
44. Bauquet AT, Jin H, Paterson AM, Mitsdoerffer M, Ho IC, Sharpe AH, et al. The Costimulatory Molecule ICOS Regulates the Expression of C-Maf and IL-21 in the Development of Follicular T Helper Cells and TH-17 Cells. Nat Immunol (2009) 10(2):167–75. doi: 10.1038/ni.1690
45. Panneton V, Chang J, Witalis M, Li J, Suh WK. Inducible T-Cell Co-Stimulator: Signaling Mechanisms in T Follicular Helper Cells and Beyond. Immunol Rev (2019) 291(1):91–103. doi: 10.1111/imr.12771
46. Aoki N, Kido M, Iwamoto S, Nishiura H, Maruoka R, Tanaka J, et al. Dysregulated Generation of Follicular Helper T Cells in the Spleen Triggers Fatal Autoimmune Hepatitis in Mice. Gastroenterology (2011) 140(4):1322–33 e1-5. doi: 10.1053/j.gastro.2011.01.002
47. Linterman MA, Rigby RJ, Wong RK, Yu D, Brink R, Cannons JL, et al. Follicular Helper T Cells are Required for Systemic Autoimmunity. J Exp Med (2009) 206(3):561–76. doi: 10.1084/jem.20081886
48. Shi J, Hou S, Fang Q, Liu X, Liu X, Qi H. PD-1 Controls Follicular T Helper Cell Positioning and Function. Immunity (2018) 49(2):264–74.e4. doi: 10.1016/j.immuni.2018.06.012
49. Curran CS, Gupta S, Sanz I, Sharon E. PD-1 Immunobiology in Systemic Lupus Erythematosus. J Autoimmun (2019) 97:1–9. doi: 10.1016/j.jaut.2018.10.025
50. Wan Z, Lin Y, Zhao Y, Qi H. TFH Cells in Bystander and Cognate Interactions With B Cells. Immunol Rev (2019) 288(1):28–36. doi: 10.1111/imr.12747
51. Liang K, He J, Wei Y, Zeng Q, Gong D, Qin J, et al. Sustained Low-Dose Interleukin-2 Therapy Alleviates Pathogenic Humoral Immunity via Elevating the Tfr/Tfh Ratio in Lupus. Clin Transl Immunol (2021) 10(6):e1293. doi: 10.1002/cti2.1293
52. Chen W, Yang F, Xu G, Ma J, Lin J. Follicular Helper T Cells and Follicular Regulatory T Cells in the Immunopathology of Primary Sjogren’s Syndrome. J Leukoc Biol (2021) 109(2):437–47. doi: 10.1002/JLB.5MR1020-057RR
53. Fazilleau N, Mark L, McHeyzer-Williams LJ, McHeyzer-Williams MG. Follicular Helper T Cells: Lineage and Location. Immunity (2009) 30(3):324–35. doi: 10.1016/j.immuni.2009.03.003
54. Shekhar S, Yang X. The Darker Side of Follicular Helper T Cells: From Autoimmunity to Immunodeficiency. Cell Mol Immunol (2012) 9(5):380–5. doi: 10.1038/cmi.2012.26
55. Mintz MA, Cyster JG. T Follicular Helper Cells in Germinal Center B Cell Selection and Lymphomagenesis. Immunol Rev (2020) 296(1):48–61. doi: 10.1111/imr.12860
56. Wu H, Deng Y, Zhao M, Zhang J, Zheng M, Chen G, et al. Molecular Control of Follicular Helper T Cell Development and Differentiation. Front Immunol (2018) 9:2470. doi: 10.3389/fimmu.2018.02470
57. Bettelli E, Campbell DJ. Circulating TFH Cells as a Marker for Early Therapeutic Intervention in T1D. Nat Immunol (2020) 21(10):1141–2. doi: 10.1038/s41590-020-0766-6
58. Choi JY, Ho JH, Pasoto SG, Bunin V, Kim ST, Carrasco S, et al. Circulating Follicular Helper-Like T Cells in Systemic Lupus Erythematosus: Association With Disease Activity. Arthritis Rheumatol (2015) 67(4):988–99. doi: 10.1002/art.39020
59. Huang Q, Xu L, Ye L. T Cell Immune Response Within B-Cell Follicles. Adv Immunol (2019) 144:155–71. doi: 10.1016/bs.ai.2019.08.008
60. Velu V, Mylvaganam GH, Gangadhara S, Hong JJ, Iyer SS, Gumber S, et al. Induction of Th1-Biased T Follicular Helper (Tfh) Cells in Lymphoid Tissues During Chronic Simian Immunodeficiency Virus Infection Defines Functionally Distinct Germinal Center Tfh Cells. J Immunol (2016) 197(5):1832–42. doi: 10.4049/jimmunol.1600143
61. Velu V, Mylvaganam G, Ibegbu C, Amara RR. Tfh1 Cells in Germinal Centers During Chronic HIV/SIV Infection. Front Immunol (2018) 9:1272. doi: 10.3389/fimmu.2018.01272
62. Schmitt N, Bentebibel SE, Ueno H. Phenotype and Functions of Memory Tfh Cells in Human Blood. Trends Immunol (2014) 35(9):436–42. doi: 10.1016/j.it.2014.06.002
63. Yao Y, Chen CL, Yu D, Liu Z. Roles of Follicular Helper and Regulatory T Cells in Allergic Diseases and Allergen Immunotherapy. Allergy (2021) 76(2):456–70. doi: 10.1111/all.14639
64. Nakayamada S, Kanno Y, Takahashi H, Jankovic D, Lu KT, Johnson TA, et al. Early Th1 Cell Differentiation is Marked by a Tfh Cell-Like Transition. Immunity (2011) 35(6):919–31. doi: 10.1016/j.immuni.2011.11.012
65. Liang H, Tang J, Liu Z, Liu Y, Huang Y, Xu Y, et al. ZIKV Infection Induces Robust Th1-Like Tfh Cell and Long-Term Protective Antibody Responses in Immunocompetent Mice. Nat Commun (2019) 10(1):3859. doi: 10.1038/s41467-019-11754-0
66. Fonseca VR, Romao VC, Agua-Doce A, Santos M, Lopez-Presa D, Ferreira AC, et al. The Ratio of Blood T Follicular Regulatory Cells to T Follicular Helper Cells Marks Ectopic Lymphoid Structure Formation While Activated Follicular Helper T Cells Indicate Disease Activity in Primary Sjogren’s Syndrome. Arthritis Rheumatol (2018) 70(5):774–84. doi: 10.1002/art.40424
67. Yang M, Cao P, Zhao Z, Wang Z, Jia C, Guo Y, et al. An Enhanced Expression Level of CXCR3 on Tfh-Like Cells From Lupus Skin Lesions Rather Than Lupus Peripheral Blood. Clin Immunol (2021) 226:108717. doi: 10.1016/j.clim.2021.108717
68. Rao DA, Gurish MF, Marshall JL, Slowikowski K, Fonseka CY, Liu Y, et al. Pathologically Expanded Peripheral T Helper Cell Subset Drives B Cells in Rheumatoid Arthritis. Nature (2017) 542(7639):110–4. doi: 10.1038/nature20810
69. Hutloff A. T Follicular Helper-Like Cells in Inflamed Non-Lymphoid Tissues. Front Immunol (2018) 9:1707. doi: 10.3389/fimmu.2018.01707
70. Yoshitomi H, Ueno H. Shared and Distinct Roles of T Peripheral Helper and T Follicular Helper Cells in Human Diseases. Cell Mol Immunol (2021) 18(3):523–7. doi: 10.1038/s41423-020-00529-z
71. Zhang YN, Song J, Wang H, Wang H, Zeng M, Zhai GT, et al. Nasal IL-4(+)CXCR5(+)CD4(+) T Follicular Helper Cell Counts Correlate With Local IgE Production in Eosinophilic Nasal Polyps. J Allergy Clin Immunol (2016) 137(2):462–73. doi: 10.1016/j.jaci.2015.07.025
72. Gowthaman U, Chen JS, Zhang B, Flynn WF, Lu Y, Song W, et al. Identification of a T Follicular Helper Cell Subset That Drives Anaphylactic IgE. Science (2019) 365(6456):eaaw6433. doi: 10.1126/science.aaw6433
73. Chen JS, Grassmann JDS, Gowthaman U, Olyha SJ, Simoneau T, Berin MC, et al. Flow Cytometric Identification of Tfh13 Cells in Mouse and Human. J Allergy Clin Immunol (2021) 147(2):470–83. doi: 10.1016/j.jaci.2020.04.063
74. Wang W, Lyu SC, Ji X, Gupta S, Manohar M, Dhondalay GKR, et al. Transcriptional Changes in Peanut-Specific CD4+ T Cells Over the Course of Oral Immunotherapy. Clin Immunol (2020) 219:108568. doi: 10.1016/j.clim.2020.108568
75. Chung Y, Tanaka S, Chu F, Nurieva RI, Martinez GJ, Rawal S, et al. Follicular Regulatory T Cells Expressing Foxp3 and Bcl-6 Suppress Germinal Center Reactions. Nat Med (2011) 17(8):983–8. doi: 10.1038/nm.2426
76. Linterman MA, Pierson W, Lee SK, Kallies A, Kawamoto S, Rayner TF, et al. Foxp3+ Follicular Regulatory T Cells Control the Germinal Center Response. Nat Med (2011) 17(8):975–82. doi: 10.1038/nm.2425
77. Aloulou M, Carr EJ, Gador M, Bignon A, Liblau RS, Fazilleau N, et al. Follicular Regulatory T Cells Can be Specific for the Immunizing Antigen and Derive From Naive T Cells. Nat Commun (2016) 7:10579. doi: 10.1038/ncomms10579
78. Sage PT, Sharpe AH. T Follicular Regulatory Cells. Immunol Rev (2016) 271(1):246–59. doi: 10.1111/imr.12411
79. Sage PT, Sharpe AH. T Follicular Regulatory Cells in the Regulation of B Cell Responses. Trends Immunol (2015) 36(7):410–8. doi: 10.1016/j.it.2015.05.005
80. Ye Y, Wang M, Huang H. Follicular Regulatory T Cell Biology and its Role in Immune-Mediated Diseases. J Leukoc Biol (2021) 110(2):239–55. doi: 10.1002/JLB.1MR0321-601RR
81. Gonzalez-Figueroa P, Roco JA, Papa I, Nunez Villacis L, Stanley M, Linterman MA, et al. Follicular Regulatory T Cells Produce Neuritin to Regulate B Cells. Cell (2021) 184(7):1775–89.e19. doi: 10.1016/j.cell.2021.02.027
82. Fonseca VR, Ribeiro F, Graca L. T Follicular Regulatory (Tfr) Cells: Dissecting the Complexity of Tfr-Cell Compartments. Immunol Rev (2019) 288(1):112–27. doi: 10.1111/imr.12739
83. Wing JB, Tekguc M, Sakaguchi S. Control of Germinal Center Responses by T-Follicular Regulatory Cells. Front Immunol (2018) 9:1910. doi: 10.3389/fimmu.2018.01910
84. Xie MM, Dent AL. Unexpected Help: Follicular Regulatory T Cells in the Germinal Center. Front Immunol (2018) 9:1536. doi: 10.3389/fimmu.2018.01536
85. Gong Y, Tong J, Wang S. Are Follicular Regulatory T Cells Involved in Autoimmune Diseases? Front Immunol (2017) 8:1790. doi: 10.3389/fimmu.2017.01790
86. Qiu H, Wu H, Chan V, Lau CS, Lu Q. Transcriptional and Epigenetic Regulation of Follicular T-Helper Cells and Their Role in Autoimmunity. Autoimmunity (2017) 50(2):71–81. doi: 10.1080/08916934.2017.1284821
87. Fazilleau N, McHeyzer-Williams LJ, Rosen H, McHeyzer-Williams MG. The Function of Follicular Helper T Cells is Regulated by the Strength of T Cell Antigen Receptor Binding. Nat Immunol (2009) 10(4):375–84. doi: 10.1038/ni.1704
88. Sahoo A, Alekseev A, Tanaka K, Obertas L, Lerman B, Haymaker C, et al. Batf is Important for IL-4 Expression in T Follicular Helper Cells. Nat Commun (2015) 6:7997. doi: 10.1038/ncomms8997
89. Kim SJ, Schatzle S, Ahmed SS, Haap W, Jang SH, Gregersen PK, et al. Increased Cathepsin S in Prdm1(-/-) Dendritic Cells Alters the TFH Cell Repertoire and Contributes to Lupus. Nat Immunol (2017) 18(9):1016–24. doi: 10.1038/ni.3793
90. Goenka R, Barnett LG, Silver JS, O’Neill PJ, Hunter CA, Cancro MP, et al. Cutting Edge: Dendritic Cell-Restricted Antigen Presentation Initiates the Follicular Helper T Cell Program But Cannot Complete Ultimate Effector Differentiation. J Immunol (2011) 187(3):1091–5. doi: 10.4049/jimmunol.1100853
91. Zou X, Sun G, Huo F, Chang L, Yang W. The Role of Dendritic Cells in the Differentiation of T Follicular Helper Cells. J Immunol Res (2018) 2018:7281453. doi: 10.1155/2018/7281453
92. Krishnaswamy JK, Gowthaman U, Zhang B, Mattsson J, Szeponik L, Liu D, et al. Migratory CD11b(+) Conventional Dendritic Cells Induce T Follicular Helper Cell-Dependent Antibody Responses. Sci Immunol (2017) 2(18):eaam9169. doi: 10.1126/sciimmunol.aam9169
93. Ballesteros-Tato A, Randall TD. Priming of T Follicular Helper Cells by Dendritic Cells. Immunol Cell Biol (2014) 92(1):22–7. doi: 10.1038/icb.2013.62
94. Wang Z, Li M, Zhou M, Zhang Y, Yang J, Cao Y, et al. A Novel Rabies Vaccine Expressing CXCL13 Enhances Humoral Immunity by Recruiting Both T Follicular Helper and Germinal Center B Cells. J Virol (2017) 91(3):e01956–16. doi: 10.1128/JVI.01956-16
95. Durand M, Walter T, Pirnay T, Naessens T, Gueguen P, Goudot C, et al. Human Lymphoid Organ Cdc2 and Macrophages Play Complementary Roles in T Follicular Helper Responses. J Exp Med (2019) 216(7):1561–81. doi: 10.1084/jem.20181994
96. Choi YS, Kageyama R, Eto D, Escobar TC, Johnston RJ, Monticelli L, et al. ICOS Receptor Instructs T Follicular Helper Cell Versus Effector Cell Differentiation via Induction of the Transcriptional Repressor Bcl6. Immunity (2011) 34(6):932–46. doi: 10.1016/j.immuni.2011.03.023
97. Ma CS, Suryani S, Avery DT, Chan A, Nanan R, Santner-Nanan B, et al. Early Commitment of Naive Human CD4(+) T Cells to the T Follicular Helper (T(FH)) Cell Lineage is Induced by IL-12. Immunol Cell Biol (2009) 87(8):590–600. doi: 10.1038/icb.2009.64
98. Zhang L, Li W, Cai Y, Liu X, Peng Q, Liang L. Aberrant Expansion of Circulating CD4(+) CXCR5(+) CCR7(lo) PD1(hi) Tfh Precursor Cells in Idiopathic Inflammatory Myopathy. Int J Rheum Dis (2020) 23(3):397–405. doi: 10.1111/1756-185X.13782
99. Pedros C, Altman A, Kong KF. Role of TRAFs in Signaling Pathways Controlling T Follicular Helper Cell Differentiation and T Cell-Dependent Antibody Responses. Front Immunol (2018) 9:2412. doi: 10.3389/fimmu.2018.02412
100. Liu D, Xu H, Shih C, Wan Z, Ma X, Ma W, et al. T-B-Cell Entanglement and ICOSL-Driven Feed-Forward Regulation of Germinal Centre Reaction. Nature (2015) 517(7533):214–8. doi: 10.1038/nature13803
101. Jacquemin C, Schmitt N, Contin-Bordes C, Liu Y, Narayanan P, Seneschal J, et al. OX40 Ligand Contributes to Human Lupus Pathogenesis by Promoting T Follicular Helper Response. Immunity (2015) 42(6):1159–70. doi: 10.1016/j.immuni.2015.05.012
102. Tahiliani V, Hutchinson TE, Abboud G, Croft M, Salek-Ardakani S. OX40 Cooperates With ICOS To Amplify Follicular Th Cell Development and Germinal Center Reactions During Infection. J Immunol (2017) 198(1):218–28. doi: 10.4049/jimmunol.1601356
103. Chen Y, Yu D. TCF-1 at the Tfh and Th1 Divergence. Trends Immunol (2015) 36(12):758–60. doi: 10.1016/j.it.2015.11.001
104. Choi YS, Gullicksrud JA, Xing S, Zeng Z, Shan Q, Li F, et al. LEF-1 and TCF-1 Orchestrate T(FH) Differentiation by Regulating Differentiation Circuits Upstream of the Transcriptional Repressor Bcl6. Nat Immunol (2015) 16(9):980–90. doi: 10.1038/ni.3226
105. Auderset F, Schuster S, Fasnacht N, Coutaz M, Charmoy M, Koch U, et al. Notch Signaling Regulates Follicular Helper T Cell Differentiation. J Immunol (2013) 191(5):2344–50. doi: 10.4049/jimmunol.1300643
106. Dell’Aringa M, Reinhardt RL. Notch Signaling Represents an Important Checkpoint Between Follicular T-Helper and Canonical T-Helper 2 Cell Fate. Mucosal Immunol (2018) 11(4):1079–91. doi: 10.1038/s41385-018-0012-9
107. Stone EL, Pepper M, Katayama CD, Kerdiles YM, Lai CY, Emslie E, et al. ICOS Coreceptor Signaling Inactivates the Transcription Factor FOXO1 to Promote Tfh Cell Differentiation. Immunity (2015) 42(2):239–51. doi: 10.1016/j.immuni.2015.01.017
108. Papillion A, Powell MD, Chisolm DA, Bachus H, Fuller MJ, Weinmann AS, et al. Inhibition of IL-2 Responsiveness by IL-6 is Required for the Generation of GC-TFH Cells. Sci Immunol (2019) 4(39):eaaw7636. doi: 10.1126/sciimmunol.aaw7636
109. Johnston RJ, Choi YS, Diamond JA, Yang JA, Crotty S. STAT5 is a Potent Negative Regulator of TFH Cell Differentiation. J Exp Med (2012) 209(2):243–50. doi: 10.1084/jem.20111174
110. Choi J, Diao H, Faliti CE, Truong J, Rossi M, Belanger S, et al. Bcl-6 is the Nexus Transcription Factor of T Follicular Helper Cells via Repressor-of-Repressor Circuits. Nat Immunol (2020) 21(7):777–89. doi: 10.1038/s41590-020-0706-5
111. Liu X, Nurieva RI, Dong C. Transcriptional Regulation of Follicular T-Helper (Tfh) Cells. Immunol Rev (2013) 252(1):139–45. doi: 10.1111/imr.12040
112. Huang Q, Hu J, Tang J, Xu L, Ye L. Molecular Basis of the Differentiation and Function of Virus Specific Follicular Helper CD4(+) T Cells. Front Immunol (2019) 10:249. doi: 10.3389/fimmu.2019.00249
113. Achour A, Simon Q, Mohr A, Seite JF, Youinou P, Bendaoud B, et al. Human Regulatory B Cells Control the TFH Cell Response. J Allergy Clin Immunol (2017) 140(1):215–22. doi: 10.1016/j.jaci.2016.09.042
114. Lu Y, Jiang R, Freyn AW, Wang J, Strohmeier S, Lederer K, et al. CD4+ Follicular Regulatory T Cells Optimize the Influenza Virus-Specific B Cell Response. J Exp Med (2021) 218(3):e20200547. doi: 10.1084/jem.20200547
115. Deng J, Fan C, Gao X, Zeng Q, Guo R, Wei Y, et al. Signal Transducer and Activator of Transcription 3 Hyperactivation Associates With Follicular Helper T Cell Differentiation and Disease Activity in Rheumatoid Arthritis. Front Immunol (2018) 9:1226. doi: 10.3389/fimmu.2018.01226
116. Ma X, Nakayamada S, Kubo S, Sakata K, Yamagata K, Miyazaki Y, et al. Expansion of T Follicular Helper-T Helper 1 Like Cells Through Epigenetic Regulation by Signal Transducer and Activator of Transcription Factors. Ann Rheum Dis (2018) 77(9):1354–61. doi: 10.1136/annrheumdis-2017-212652
117. Sheikh AA, Groom JR. Transcription Tipping Points for T Follicular Helper Cell and T-Helper 1 Cell Fate Commitment. Cell Mol Immunol (2021) 18(3):528–38. doi: 10.1038/s41423-020-00554-y
118. Wang P, Wang Y, Xie L, Xiao M, Wu J, Xu L, et al. The Transcription Factor T-Bet Is Required for Optimal Type I Follicular Helper T Cell Maintenance During Acute Viral Infection. Front Immunol (2019) 10:606. doi: 10.3389/fimmu.2019.00606
119. Sheikh AA, Cooper L, Feng M, Souza-Fonseca-Guimaraes F, Lafouresse F, Duckworth BC, et al. Context-Dependent Role for T-Bet in T Follicular Helper Differentiation and Germinal Center Function Following Viral Infection. Cell Rep (2019) 28(7):1758–72.e4. doi: 10.1016/j.celrep.2019.07.034
120. Oestreich KJ, Mohn SE, Weinmann AS. Molecular Mechanisms That Control the Expression and Activity of Bcl-6 in TH1 Cells to Regulate Flexibility With a TFH-Like Gene Profile. Nat Immunol (2012) 13(4):405–11. doi: 10.1038/ni.2242
121. Park HJ, Kim DH, Lim SH, Kim WJ, Youn J, Choi YS, et al. Insights Into the Role of Follicular Helper T Cells in Autoimmunity. Immune Netw (2014) 14(1):21–9. doi: 10.4110/in.2014.14.1.21
122. Hercor M, Anciaux M, Denanglaire S, Debuisson D, Leo O, Andris F. Antigen-Presenting Cell-Derived IL-6 Restricts the Expression of GATA3 and IL-4 by Follicular Helper T Cells. J leukocyte Biol (2017) 101(1):5–14. doi: 10.1189/jlb.1HI1115-511R
123. Ma X, Nakayamada S. Multi-Source Pathways of T Follicular Helper Cell Differentiation. Front Immunol (2021) 12:621105. doi: 10.3389/fimmu.2021.621105
124. Maehara T, Moriyama M, Nakashima H, Miyake K, Hayashida JN, Tanaka A, et al. Interleukin-21 Contributes to Germinal Centre Formation and Immunoglobulin G4 Production in IgG4-Related Dacryoadenitis and Sialoadenitis, So-Called Mikulicz’s Disease. Ann Rheum Dis (2012) 71(12):2011–9. doi: 10.1136/annrheumdis-2012-201477
125. Wichner K, Stauss D, Kampfrath B, Kruger K, Muller G, Rehm A, et al. Dysregulated Development of IL-17- and IL-21-Expressing Follicular Helper T Cells and Increased Germinal Center Formation in the Absence of RORgammat. FASEB J (2016) 30(2):761–74. doi: 10.1096/fj.15-274001
126. Wen Z, Xu L, Xu W, Xiong S. RORgammat Licenses the Differentiation and Function of a Unique Subset of Tfh Cells in Response to Immunogenic Self-DNA in Systemic Lupus Erythematosus. Arthritis Rheumatol (2021) 73(8):1489–500. doi: 10.1002/art.41687
127. Spinner CA, Lazarevic V. Transcriptional Regulation of Adaptive and Innate Lymphoid Lineage Specification. Immunol Rev (2021) 300(1):65–81. doi: 10.1111/imr.12935
128. Wing JB, Ise W, Kurosaki T, Sakaguchi S. Regulatory T Cells Control Antigen-Specific Expansion of Tfh Cell Number and Humoral Immune Responses via the Coreceptor CTLA-4. Immunity (2014) 41(6):1013–25. doi: 10.1016/j.immuni.2014.12.006
129. Zhang H, Dai D, Hu Q, Yang F, Xue Y, Li F, et al. Bach2 Attenuates IL-2R Signaling to Control Treg Homeostasis and Tfr Development. Cell Rep (2021) 35(6):109096. doi: 10.1016/j.celrep.2021.109096
130. Sayin I, Radtke AJ, Vella LA, Jin W, Wherry EJ, Buggert M, et al. Spatial Distribution and Function of T Follicular Regulatory Cells in Human Lymph Nodes. J Exp Med (2018) 215(6):1531–42. doi: 10.1084/jem.20171940
131. Wing JB, Lim EL, Sakaguchi S. Control of Foreign Ag-Specific Ab Responses by Treg and Tfr. Immunol Rev (2020) 296(1):104–19. doi: 10.1111/imr.12888
132. Carpio VH, Aussenac F, Puebla-Clark L, Wilson KD, Villarino AV, Dent AL, et al. T Helper Plasticity Is Orchestrated by STAT3, Bcl6, and Blimp-1 Balancing Pathology and Protection in Malaria. iScience (2020) 23(7):101310. doi: 10.1016/j.isci.2020.101310
133. Sage PT, Paterson AM, Lovitch SB, Sharpe AH. The Coinhibitory Receptor CTLA-4 Controls B Cell Responses by Modulating T Follicular Helper, T Follicular Regulatory, and T Regulatory Cells. Immunity (2014) 41(6):1026–39. doi: 10.1016/j.immuni.2014.12.005
134. Hao H, Nakayamada S, Yamagata K, Ohkubo N, Iwata S, Inoue Y, et al. Conversion of T Follicular Helper Cells to T Follicular Regulatory Cells by Interleukin-2 Through Transcriptional Regulation in Systemic Lupus Erythematosus. Arthritis Rheumatol (2021) 73(1):132–42. doi: 10.1002/art.41457
135. Wu X, Wang Y, Huang R, Gai Q, Liu H, Shi M, et al. SOSTDC1-Producing Follicular Helper T Cells Promote Regulatory Follicular T Cell Differentiation. Science (2020) 369(6506):984–8. doi: 10.1126/science.aba6652
136. Shen E, Rabe H, Luo L, Wang L, Wang Q, Yin J, et al. Control of Germinal Center Localization and Lineage Stability of Follicular Regulatory T Cells by the Blimp1 Transcription Factor. Cell Rep (2020) 31(4):107575. doi: 10.1016/j.celrep.2020.107575
137. Sage PT, Sharpe AH. The Multifaceted Functions of Follicular Regulatory T Cells. Curr Opin Immunol (2020) 67:68–74. doi: 10.1016/j.coi.2020.10.009
138. Huang Y, Chen Z, Wang H, Ba X, Shen P, Lin W, et al. Follicular Regulatory T Cells: A Novel Target for Immunotherapy? Clin Transl Immunol (2020) 9(2):e1106. doi: 10.1002/cti2.1106
139. Lin X, Wang X, Xiao F, Ma K, Liu L, Wang X, et al. IL-10-Producing Regulatory B Cells Restrain the T Follicular Helper Cell Response in Primary Sjogren’s Syndrome. Cell Mol Immunol (2019) 16(12):921–31. doi: 10.1038/s41423-019-0227-z
140. Wang X, Chan CC, Yang M, Deng J, Poon VK, Leung VH, et al. A Critical Role of IL-17 in Modulating the B-Cell Response During H5N1 Influenza Virus Infection. Cell Mol Immunol (2011) 8(6):462–8. doi: 10.1038/cmi.2011.38
141. Ma CS, Deenick EK. Human T Follicular Helper (Tfh) Cells and Disease. Immunol Cell Biol (2014) 92(1):64–71. doi: 10.1038/icb.2013.55
142. Chen X, Cao G, Wu J, Wang X, Pan Z, Gao J, et al. The Histone Methyltransferase EZH2 Primes the Early Differentiation of Follicular Helper T Cells During Acute Viral Infection. Cell Mol Immunol (2020) 17(3):247–60. doi: 10.1038/s41423-019-0219-z
143. Deng J, Chen Q, Chen Z, Liang K, Gao X, Wang X, et al. The Metabolic Hormone Leptin Promotes the Function of TFH Cells and Supports Vaccine Responses. Nat Commun (2021) 12(1):3073. doi: 10.1038/s41467-021-23220-x
144. Baumjohann D, Fazilleau N. Antigen-Dependent Multistep Differentiation of T Follicular Helper Cells and Its Role in SARS-CoV-2 Infection and Vaccination. Eur J Immunol (2021) 51(6):1325–33. doi: 10.1002/eji.202049148
145. Thevarajan I, Nguyen THO, Koutsakos M, Druce J, Caly L, van de Sandt CE, et al. Breadth of Concomitant Immune Responses Prior to Patient Recovery: A Case Report of non-Severe COVID-19. Nat Med (2020) 26(4):453–5. doi: 10.1038/s41591-020-0819-2
146. Schultheiss C, Paschold L, Simnica D, Mohme M, Willscher E, von Wenserski L, et al. Next-Generation Sequencing of T and B Cell Receptor Repertoires From COVID-19 Patients Showed Signatures Associated With Severity of Disease. Immunity (2020) 53(2):442–55.e4. doi: 10.1016/j.immuni.2020.06.024
147. Juno JA, Tan HX, Lee WS, Reynaldi A, Kelly HG, Wragg K, et al. Humoral and Circulating Follicular Helper T Cell Responses in Recovered Patients With COVID-19. Nat Med (2020) 26(9):1428–34. doi: 10.1038/s41591-020-0995-0
148. Bentebibel SE, Lopez S, Obermoser G, Schmitt N, Mueller C, Harrod C, et al. Induction of ICOS+CXCR3+CXCR5+ TH Cells Correlates With Antibody Responses to Influenza Vaccination. Sci Transl Med (2013) 5(176):176ra32. doi: 10.1126/scitranslmed.3005191
149. Huber JE, Ahlfeld J, Scheck MK, Zaucha M, Witter K, Lehmann L, et al. Dynamic Changes in Circulating T Follicular Helper Cell Composition Predict Neutralising Antibody Responses After Yellow Fever Vaccination. Clin Transl Immunol (2020) 9(5):e1129. doi: 10.1002/cti2.1129
150. Gong F, Dai Y, Zheng T, Cheng L, Zhao D, Wang H, et al. Peripheral CD4+ T Cell Subsets and Antibody Response in COVID-19 Convalescent Individuals. J Clin Invest (2020) 130(12):6588–99. doi: 10.1172/JCI141054
151. RydyznskiModerbacher C, Ramirez SI, Dan JM, Grifoni A, Hastie KM, Weiskopf D, et al. Antigen-Specific Adaptive Immunity to SARS-CoV-2 in Acute COVID-19 and Associations With Age and Disease Severity. Cell (2020) 183(4):996–1012.e19. doi: 10.1016/j.cell.2020.09.038
152. Kaneko N, Kuo HH, Boucau J, Farmer JR, Allard-Chamard H, Mahajan VS, et al. Loss of Bcl-6-Expressing T Follicular Helper Cells and Germinal Centers in COVID-19. Cell (2020) 183(1):143–57.e13. doi: 10.1016/j.cell.2020.08.025
153. Neidleman J, Luo X, Frouard J, Xie G, Gill G, Stein ES, et al. SARS-CoV-2-Specific T Cells Exhibit Phenotypic Features of Helper Function, Lack of Terminal Differentiation, and High Proliferation Potential. Cell Rep Med (2020) 1(6):100081. doi: 10.1016/j.xcrm.2020.100081S2666-3791(20)30102-6
154. Mathew D, Giles JR, Baxter AE, Oldridge DA, Greenplate AR, Wu JE, et al. Deep Immune Profiling of COVID-19 Patients Reveals Distinct Immunotypes With Therapeutic Implications. Science (2020) 369(6508):eabc8511. doi: 10.1126/science.abc8511
155. Meckiff BJ, Ramirez-Suastegui C, Fajardo V, Chee SJ, Kusnadi A, Simon H, et al. Imbalance of Regulatory and Cytotoxic SARS-CoV-2-Reactive CD4(+) T Cells in COVID-19. Cell (2020) 183(5):1340–53.e16. doi: 10.1016/j.cell.2020.10.001
156. Yan L, Cai B, Li Y, Wang MJ, An YF, Deng R, et al. Dynamics of NK, CD8 and Tfh Cell Mediated the Production of Cytokines and Antiviral Antibodies in Chinese Patients With Moderate COVID-19. J Cell Mol Med (2020) 24(24):14270–9. doi: 10.1111/jcmm.16044
157. Collora JA, Liu R, Albrecht K, Ho YC. The Single-Cell Landscape of Immunological Responses of CD4+ T Cells in HIV Versus Severe Acute Respiratory Syndrome Coronavirus 2. Curr Opin HIV AIDS (2021) 16(1):36–47. doi: 10.1097/COH.0000000000000655
158. Zhao B, Zhong M, Yang Q, Hong K, Xia J, Li X, et al. Alterations in Phenotypes and Responses of T Cells Within 6 Months of Recovery From COVID-19: A Cohort Study. Virol Sin (2021), 1–10. doi: 10.1007/s12250-021-00348-0
159. Gao L, Zhou J, Yang S, Wang L, Chen X, Yang Y, et al. The Dichotomous and Incomplete Adaptive Immunity in COVID-19 Patients With Different Disease Severity. Signal Transduct Target Ther (2021) 6(1):113. doi: 10.1038/s41392-021-00525-3
160. Koutsakos M, Rowntree LC, Hensen L, Chua BY, van de Sandt CE, Habel JR, et al. Integrated Immune Dynamics Define Correlates of COVID-19 Severity and Antibody Responses. Cell Rep Med (2021) 2(3):100208. doi: 10.1016/j.xcrm.2021.100208
161. Zenarruzabeitia O, Astarloa-Pando G, Terren I, Orrantia A, Perez-Garay R, Seijas-Betolaza I, et al. T Cell Activation, Highly Armed Cytotoxic Cells and a Shift in Monocytes CD300 Receptors Expression Is Characteristic of Patients With Severe COVID-19. Front Immunol (2021) 12:655934. doi: 10.3389/fimmu.2021.655934
162. Adamo S, Chevrier S, Cervia C, Zurbuchen Y, Raeber ME, Yang L, et al. Profound Dysregulation of T Cell Homeostasisand Function in Patients With Severe COVID-19. Allergy (2021) 76(9):2866–81. doi: 10.1111/all.14866
163. Fenoglio D, Dentone C, Parodi A, Di Biagio A, Bozzano F, Vena A, et al. Characterization of T Lymphocytes in Severe COVID-19 Patients. J Med Virol (2021) 93(9):5608–13. doi: 10.1002/jmv.27037
164. Spoerl S, Kremer AN, Aigner M, Eisenhauer N, Koch P, Meretuk L, et al. Upregulation of CCR4 in Activated CD8(+) T Cells Indicates Enhanced Lung Homing in Patients With Severe Acute SARS-CoV-2 Infection. Eur J Immunol (2021) 51(6):1436–48. doi: 10.1002/eji.202049135
165. Shaan Lakshmanappa Y, Elizaldi SR, Roh JW, Schmidt BA, Carroll TD, Weaver KD, et al. SARS-CoV-2 Induces Robust Germinal Center CD4 T Follicular Helper Cell Responses in Rhesus Macaques. Nat Commun (2021) 12(1):541. doi: 10.1038/s41467-020-20642-x
166. Sadarangani M, Marchant A, Kollmann TR. Immunological Mechanisms of Vaccine-Induced Protection Against COVID-19 in Humans. Nat Rev Immunol (2021) 21(8):475–84. doi: 10.1038/s41577-021-00578-z
167. Corbett KS, Flynn B, Foulds KE, Francica JR, Boyoglu-Barnum S, Werner AP, et al. Evaluation of the mRNA-1273 Vaccine Against SARS-CoV-2 in Nonhuman Primates. N Engl J Med (2020) 383(16):1544–55. doi: 10.1056/NEJMoa2024671
168. Lederer K, Castaño D, Gómez Atria D, Oguin TH 3rd, Wang S, Manzoni TB, et al. SARS-CoV-2 mRNA Vaccines Foster Potent Antigen-Specific Germinal Center Responses Associated With Neutralizing Antibody Generation. Immunity (2020) 53(6):1281–95.e5. doi: 10.1016/j.immuni.2020.11.009
169. Tauzin A, Nayrac M, Benlarbi M, Gong SY, Gasser R, Beaudoin-Bussières G, et al. A Single Dose of the SARS-CoV-2 Vaccine BNT162b2 Elicits Fc-Mediated Antibody Effector Functions and T Cell Responses. Cell Host Microbe (2021) 29(7):1137–50.e6. doi: 10.1016/j.chom.2021.06.001
170. Tian JH, Patel N, Haupt R, Zhou H, Weston S, Hammond H, et al. SARS-CoV-2 Spike Glycoprotein Vaccine Candidate NVX-CoV2373 Immunogenicity in Baboons and Protection in Mice. Nat Commun (2021) 12(1):372. doi: 10.1038/s41467-020-20653-8
171. Tan HX, Juno JA, Lee WS, Barber-Axthelm I, Kelly HG, Wragg KM, et al. Immunogenicity of Prime-Boost Protein Subunit Vaccine Strategies Against SARS-CoV-2 in Mice and Macaques. Nat Commun (2021) 12(1):1403. doi: 10.1038/s41467-021-21665-8
172. Wu Y, Huang X, Yuan L, Wang S, Zhang Y, Xiong H, et al. A Recombinant Spike Protein Subunit Vaccine Confers Protective Immunity Against SARS-CoV-2 Infection and Transmission in Hamsters. Sci Transl Med (2021) 13(606):eabg1143. doi: 10.1126/scitranslmed.abg1143
173. Xia S, Zhang Y, Wang Y, Wang H, Yang Y, Gao GF, et al. Safety and Immunogenicity of an Inactivated SARS-CoV-2 Vaccine, BBIBP-CorV: A Randomised, Double-Blind, Placebo-Controlled, Phase 1/2 Trial. Lancet Infect Dis (2021) 21(1):39–51. doi: 10.1016/S1473-3099(20)30831-8
174. Zhang Y, Zeng G, Pan H, Li C, Hu Y, Chu K, et al. Safety, Tolerability, and Immunogenicity of an Inactivated SARS-CoV-2 Vaccine in Healthy Adults Aged 18-59 Years: A Randomised, Double-Blind, Placebo-Controlled, Phase 1/2 Clinical Trial. Lancet Infect Dis (2021) 21(2):181–92. doi: 10.1016/S1473-3099(20)30843-4
175. Wu Z, Hu Y, Xu M, Chen Z, Yang W, Jiang Z, et al. Safety, Tolerability, and Immunogenicity of an Inactivated SARS-CoV-2 Vaccine (CoronaVac) in Healthy Adults Aged 60 Years and Older: A Randomised, Double-Blind, Placebo-Controlled, Phase 1/2 Clinical Trial. Lancet Infect Dis (2021) 21(6):803–12. doi: 10.1016/S1473-3099(20)30987-7
176. Ella R, Reddy S, Jogdand H, Sarangi V, Ganneru B, Prasad S, et al. Safety and Immunogenicity of an Inactivated SARS-CoV-2 Vaccine, BBV152: Interim Results From a Double-Blind, Randomised, Multicentre, Phase 2 Trial, and 3-Month Follow-Up of a Double-Blind, Randomised Phase 1 Trial. Lancet Infect Dis (2021) 21(7):950–61. doi: 10.1016/S1473-3099(21)00070-0
Keywords: COVID-19, SARS-CoV-2, Tfh cells, B cells, neutralizing antibody
Citation: Cui D, Tang Y, Jiang Q, Jiang D, Zhang Y, Lv Y, Xu D, Wu J, Xie J, Wen C and Lu L (2021) Follicular Helper T Cells in the Immunopathogenesis of SARS-CoV-2 Infection. Front. Immunol. 12:731100. doi: 10.3389/fimmu.2021.731100
Received: 26 June 2021; Accepted: 01 September 2021;
Published: 16 September 2021.
Edited by:
Mariana Gisela Bego, McGill University, CanadaReviewed by:
Narayanaiah Cheedarla, Emory University, United StatesGuangyong Peng, Saint Louis University, United States
Copyright © 2021 Cui, Tang, Jiang, Jiang, Zhang, Lv, Xu, Wu, Xie, Wen and Lu. This is an open-access article distributed under the terms of the Creative Commons Attribution License (CC BY). The use, distribution or reproduction in other forums is permitted, provided the original author(s) and the copyright owner(s) are credited and that the original publication in this journal is cited, in accordance with accepted academic practice. No use, distribution or reproduction is permitted which does not comply with these terms.
*Correspondence: Dawei Cui, daweicui@zju.edu.cn; Liwei Lu, liweilu@hku.hk; Chengping Wen, wengcp@163.com; Jue Xie, zyyyxj2011@zju.edu.cn