- 1Department of Immunology, Southmead Hospital, North Bristol National Health Service (NHS) Trust, Bristol, United Kingdom
- 2Severn Infection Sciences and Public Health England National Infection Service South West, Department of Virology, Southmead Hospital, North Bristol NHS Trust, Bristol, United Kingdom
In the era of COVID-19, understanding how our immune system responds to viral infections is more pertinent than ever. Immunodeficiencies with very low or absent B cells offer a valuable model to study the role of humoral immunity against these types of infection. This review looks at the available evidence on viral infections in patients with B cell alymphocytosis, in particular those with X-linked agammaglobulinemia (XLA), Good’s syndrome, post monoclonal-antibody therapy and certain patients with Common Variable Immune Deficiency (CVID). Viral infections are not as infrequent as previously thought in these conditions and individuals with very low circulating B cells seem to be predisposed to an adverse outcome. Particularly in the case of SARS-CoV2 infection, mounting evidence suggests that peripheral B cell alymphocytosis is linked to a poor prognosis.
Introduction
Humoral immunity has been regarded as the pillar of immune defenses against bacterial infections, but was thought to be less essential for viral infections. For the latter, cellular immunity was traditionally considered to be more important, e.g. T and Natural Killer (NK) cells. Data from the world of primary immunodeficiency seems to support this view: patients with Common Variable Immune Deficiency (CVID) generally present with recurrent bacterial infections, while those with Severe Combined Immune Deficiency (SCID) are also predisposed to viral infections (1, 2).
This is however only a simplified paradigm and in reality immune defenses against infection depend on more complex and interlinked processes for example, antibodies are known to be important in neutralizing viral particles and preventing them from entering human tissues (3). They also participate in the elimination of virally infected cells, via the process of opsonization and subsequent phagocytosis (3). IgA is known to play a vital role in antiviral defenses at mucosal sites, e.g. respiratory or gastrointestinal (4).
The importance of humoral immunity in the protection from viral infection is most elegantly exemplified by immunization, e.g. to measles, chicken pox and rubella viruses. Protective immunity by these vaccines relies largely on the development of an adequate humoral immune response to the virus and serology levels are often used as a proxy to assess immunity (5). Viral vaccines that depend solely on T cell-mediated immunity on the other hand are yet to be implemented successfully in clinical practice (6). Another paradigm is the placental transfer of protective IgG antibodies from mother to the fetus, which confers protection from some viral infections during the first months of life (7).
In our attempt to understand the role of the various parts of the immune system in the defense against infections, immunodeficiencies present a valuable model. Immunodeficiencies with absent or very low B cells in particular are very useful to understand the role of these cells in antiviral immunity. The best example is offered by patients with agammaglobulinemia (X-linked or Autosomal Recessive), but patients with Good’s syndrome, those that have undergone B cell-depleting therapy, and certain patients with CVID are also very informative in this respect.
To identify relevant articles in the literature we searched PubMed and Google scholar databases using the following terms: immunodeficiency, immune deficiency, viral disease, viral infection, peripheral B cells, serum B cells, B cell lymphopenia, B cell alymphocytosis, X-linked agammaglobulinemia, XLA, autosomal recessive agammaglobulinemia, Good’s syndrome, common variable immune deficiency, CVID, rituximab, B cell depleting therapy, anti-CD20 monoclonal-antibody therapy, SARS-CoV-2, COVID-19, enteroviruses, JC virus, astrovirus, adenovirus, measles, mumps, rubella, BK virus, West Nile virus, norovirus, HSV, CMV, VZV, HHV7, HHV8, HPV, vaccinia virus, parvovirus B19 and hepatitis virus. Reference lists of retrieved articles were also manually screened for relevant studies.
XLA and AR Agammaglobulinemia
X-linked agammaglobulinemia (XLA) manifests with markedly reduced peripheral B cells, severe hypogammaglobulinaemia and recurrent sinopulmonary infections. The latter are largely bacterial but the role of viruses is recently starting to be recognized (Table 1). Several cases of atypical, unusually severe, recurrent or persistent viral pneumonia have been described in these patients (18, 62, 147, 148). More recently, many cases of persistent SARS-CoV2 pneumonia have been reported, highlighting the importance of B cells in the defense against this virus (132–136).
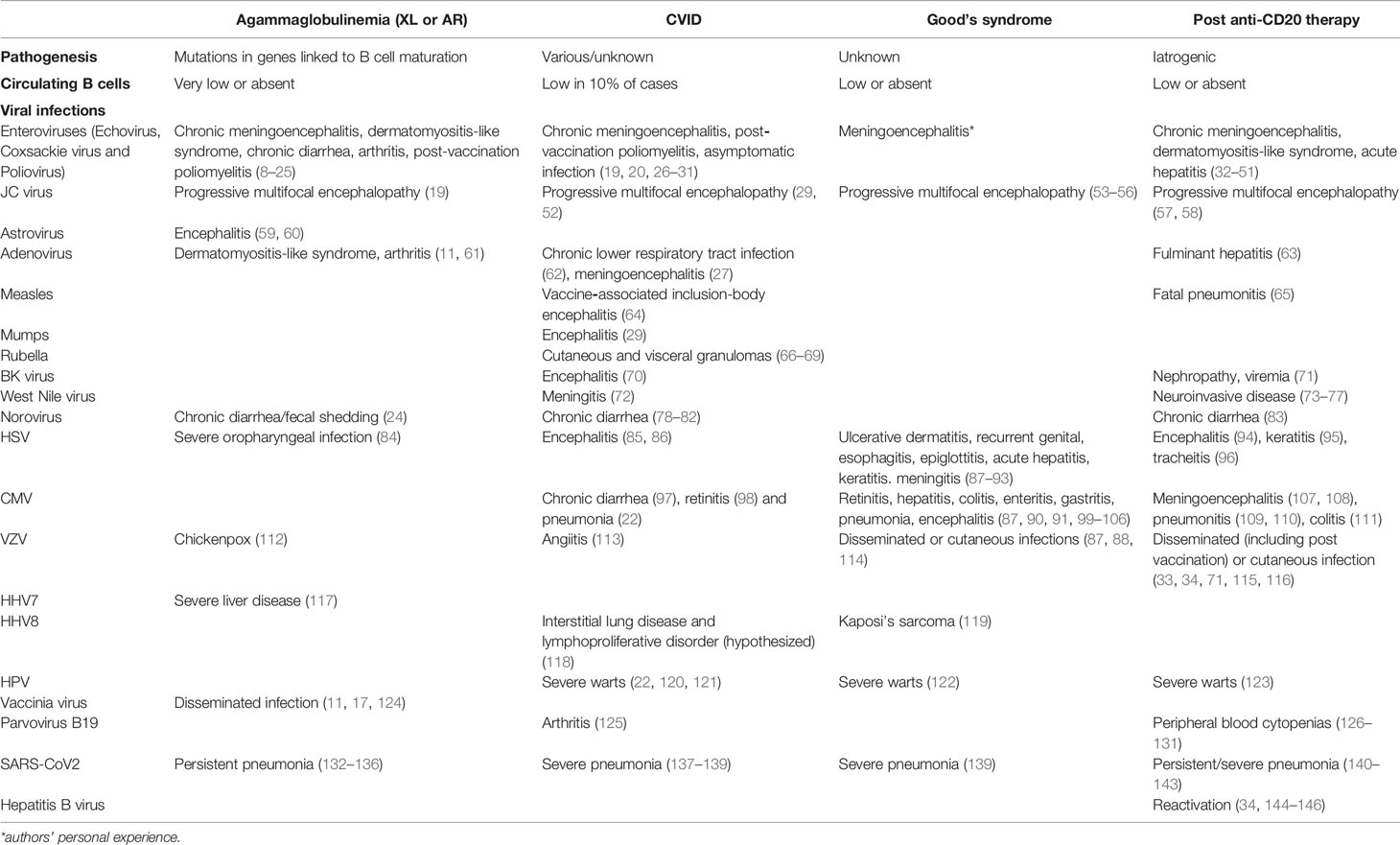
Table 1 Viral infections in patients with agammaglobulinemia, Good’s syndrome, CVID and post-B cell-depleting therapy.
Enteroviral infections are also well documented in these patients; chronic meningoencephalitis being their most serious complication (8–16, 24). Mostly echoviruses, but also coxsackie and polio viruses have been reported (17, 18). Chronic enteroviral infection can also manifest as a dermatomyositis-like syndrome in some patients, with muscle weakness, rash and edema (8, 11). Cases of chronic diarrhea or arthritis from echoviruses have also been reported (11, 18, 61). Enteroviral infections are more common in untreated patients or those that are on suboptimal immunoglobulin replacement, again suggesting an important role for humoral immunity (19, 149).
Before it was recognized that live vaccines pose a significant threat in these patients, several cases of post-vaccine infection were reported, e.g. post-poliomyelitis vaccination (8) or disseminated vaccinia virus infection (11, 17, 124) (). In a recent systematic review, agammaglobulinemic patients exhibited the highest likelihood of developing vaccine-derived poliovirus infection in comparison to other primary immunodeficiency disorders (20). Interestingly, the risk was lowest in patients with SCID, suggesting that cellular immunity is less important for the protection against this virus (20).
Other viruses that have been linked to encephalitis in XLA include astrovirus (59, 60) and JC virus (19). Adenovirus has been linked to the development of a dermatomyositis-like syndrome and arthritis (11, 61). Finally, chronic fecal shedding of norovirus is very common in XLA children, occurring in 40% of cases (150). Interestingly, EBV and herpes-viral infections are only rarely reported in these patients (112, 117, 151).
AR mutations in the genes encoding for IgM heavy chain, Igα (CD79α), λ5, BLNK and LRRC8 cause a similar disease phenotype to XLA. These defects are very uncommon, but enteroviral infections have also been reported in these patients, both post-vaccination and naturally acquired (21–23, 25).
Good’s Syndrome
Patients with Good’s syndrome present with thymoma and immunodeficiency. Hypogammaglobulinemia is most commonly described but very low or even absent B cells are also characteristic (90, 152). CD4 T cell lymphopenia is less frequent and seems to be less clinically relevant: T cell counts do not readily predict the risk of acquiring opportunistic infections in these patients, particularly cytomegalovirus (CMV) (90, 153). In the largest case series to date (n=78) the average level of peripheral CD4 T cells in patients with Good’s syndrome was normal, while B cells were virtually absent (152). The cause of B cell deficiency in these patients is unknown but certain evidence suggests that it is a bone marrow defect, with an underlying arrest in B cell development that may be responsible (153, 154). The clinical picture is similar to that of XLA, but opportunistic infections are more common.
Chronic or recurrent viral infections in particular are seen in up to 40% of patients, usually in the context of normal CD4 T cells (Table 1) (87, 90, 91). CMV infection is common, resulting in retinitis, hepatitis, colitis, enteritis, gastritis, pneumonia or encephalitis (87, 90, 91, 99–106). Other infections include chronic human papilloma virus (HPV; warts) (122), HSV (ulcerative dermatitis, recurrent genital infection, esophagitis, epiglottitis, acute hepatitis, keratitis or meningitis) (88-93), human herpesvirus 8 (HHV8; Kaposi’s sarcoma) (119), JC virus (progressive multifocal encephalopathy) (53–56) and VZV (herpes zoster or disseminated infection) (87, 88, 114). A case of severe SARS-CoV2 infection has also been documented in a patient with Good’s syndrome and absent B cells (139). Finally, a significant proportion of patients that developed yellow fever vaccine-associated viscerotropic disease post vaccination had a history of thymus disease, suggesting possible Good’s syndrome (155).
Although hypogammaglobulinemia is generally thought to be the main driver in this disease, B cell counts correlate better with the risk of infection (153, 156). Moreover, cases with normal immunoglobulins but absent B cells and recurrent infections are reported in the literature (157).Thymectomy can cure the autoimmune features of this disease, but not B cell lymphopenia, suggesting that this may be the primary defect in these patients (158).
CVID
Patients with CVID generally have normal levels of circulating B cells, but in up to 10% of cases B cells can be low (159). Of note, it is patients with absent B cells that tend to have the worse prognosis (91).
Although bacterial sinopulmonary infections are typically documented in CVID, various atypical viral infections have been described and these may be more common than previously thought (Table 1). Kainulainen et al. found that latent or chronic lower respiratory tract viral infections are frequently detected in these patients, usually caused by adenovirus (62). Similar findings were noted in a more recent study of 1303 immunocompromised patients; viral infections were identified in 35% of 2666 bronchoalveolar lavage samples, with rhinovirus-enterovirus, parainfluenza and coronavirus being the most common (160). Severe SARS-CoV2 infection has also been documented in 2 CVID patients with very low peripheral B cells (137, 139). In a recent international study of patients with inborn errors of immunity, CVID patients comprised a large proportion of the total number of deaths from SARS-CoV2 (138).
Although less common in comparison to XLA, chronic meningoencephalitis by enteroviruses and post-vaccination poliomyelitis have also been described in these patients (19, 26, 27, 29). Asymptomatic infection is also common (30, 31). In a recent systematic review, CVID patients exhibited the lowest probability of clearing vaccine-derived poliovirus infection in comparison to other primary immunodeficiency patients (20). Serum B cell counts are unfortunately not generally cited in these cases, with the exception of a recent report of a patient with monogenic CVID and fatal enteroviral encephalitis (28). Peripheral B cells were absent in this patient and interestingly, her primary and secondary lymphoid tissues also lacked B cells (28). Throughout the initial course of her disease she was on adequate immunoglobulin replacement, suggesting that B cell lymphopenia may have been the primary driver of her poor clinical outcome (28).
Other reported viral causes of meningoencephalitis in CVID include BK virus (70), adenovirus (27), mumps (29), West Nile virus (72), and HSV (85, 86). Also JC virus-induced PML has been cited (29, 52). Measles inclusion-body encephalitis caused by the vaccine strain of the virus has been documented in a young boy with features suggestive of CVID (64).
Up to 10% of CVID patients develop an inflammatory bowel disease-like disorder, and viral infections are thought to play a key role in its pathogenesis (97). CMV has been implicated (97) but most commonly chronic norovirus infection is cited (78–82). Interestingly, these patients tend to have very low or absent peripheral B cells (78) and very few plasma cells in their intestine (161). Of note, even non-CVID patients that suffer from chronic norovirus infection tend to have absent B cells, suggesting a vital role for these cells against this virus (78).
Other severe or chronic viral infections that have been documented in CVID include VZV-induced angiitis (113), parvovirus B19-induced arthritis (125), and severe HPV-associated warts (22, 120, 121). Apart from enteritis, CMV infection has also been linked to retinitis (98) and pneumonia (22). Rubella virus vaccine strains are postulated to play a role in the development of the cutaneous and visceral granulomas that are seen in these patients (66–69, 162). Finally, HHV8 is suspected to play a role in the pathogenesis of interstitial lung disease and of lymphoproliferative disorders that are more frequent in these patients (118).
B Cell-Depleting Therapies
Rituximab, an anti-CD20 monoclonal antibody, depletes peripheral B-cells and causes subsequent hypogammaglobulinemia with impaired specific-antibody production (163, 164). Its effects can last for several months, particularly when given in multiple cycles, and in many cases circulating B cells remain low long-term (165–168).
Rituximab has now been used in clinical practice for more than 20 years and its role as an independent risk-factor for the development of viral infections is well documented (34, 71). Cases of viral reactivation are reported in the literature (Table 1), most commonly by hepatitis B virus (34, 144–146), VZV (disseminated or cutaneous infection) (33, 34, 71, 115) and CMV (meningoencephalitis (107, 108), pneumonitis (109, 110), colitis (111)). JC virus-induced PML (57, 58), BK virus-associated nephropathy/viremia (71), severe warts (123), adenovirus-induced fulminant hepatitis (63), and HSV-induced encephalitis (94), keratitis (95) and tracheitis (96) have also been reported. These associations have led professional societies to recommend hepatitis screening before treatment initiation (169, 170) and/or herpes zoster prophylaxis post-treatment (171).
De-novo viral infections are equally common, with the most common examples being parvovirus B19-induced peripheral cytopenias (126–131) and West Nile virus neuroinvasive disease (73–77). More recently, several cases of persistent (140, 141, 143) or fatal (142) SARS- CoV2 infection have been documented in patients that previously had rituximab treatment. Several cases of enteroviral meningoencephalitis are also documented (32–45) suggesting that absent peripheral B cells, irrespective of the cause, is the key driver for this disease. Cases of enterovirus-induced acute liver failure are also cited (46, 47), as well as cases of chronic norovirus diarrhea (83) and fatal measles pneumonitis (65).A case of fatal, disseminated VZV infection following zoster vaccination was recently documented in a patient that previously had rituximab-inclusive chemotherapy (116). Some of the above studies included patients that were receiving additional immunosuppressive agents, making it difficult to delineate the individual contribution of rituximab to the patients’ immune status; but in several others, the role of rituximab as an independent risk factor was established (168, 172-174).
Not surprisingly, other anti-CD20 monoclonal antibodies have a similar risk-profile. Several cases of post-obinutuzumab enteroviral meningoencephalitis have been reported and these patients also sometimes develop a dermatomyositis-like syndrome (48–50). Finally, a patient with echovirus-induced fulminant hepatitis after ocrelizumab has been reported (51).
Discussion
The COVID-19 pandemic has brought viral infections into the spotlight of global scientific interest and understanding how our immune system protects us from these infections is today more pertinent than ever. The immunodeficiency disorders discussed above offer an insight into the role of humoral immunity in antiviral defenses. Contrary to the common belief that patients with humoral immune defects are predisposed to bacterial infections alone, viral infections are frequently reported in these patients, particularly in those with absent peripheral B cells. Viral infections are of course also common in healthy individuals, who may also present with unusually severe or prolonged viral infections. The cases described above however highlight an atypical and opportunistic pattern of infections in patients with absent circulating B cells.
Chronic enteroviral infection is one of the most characteristic examples and strongly suggests that very low B cells are an important predisposing factor for an adverse outcome, irrespective of the cause. IgG replacement therapy helps prevent severe sequelae in most, but not all patients, suggesting that low circulating B cells may represent an independent risk factor. Persistent Norovirus infection is another example that is seen almost exclusively in individuals with low or absent circulating B cells, irrespective of the cause (XLA, CVID and even in the general population). B cells are also absent in intestinal biopsies from these patients, highlighting an important role for local humoral immunity. Mounting evidence also suggests that B cell alymphocytosis is linked to an adverse outcome from SARS CoV2 infection. In certain situations, cellular immunity seems to be even less relevant than humoral, e.g. XLA patients had a higher likelihood to acquire vaccine-derived poliovirus infection in comparison to patients with combined immune deficiencies.
Viral pathogenesis of course also plays an important role in the outcome of any viral infection. Most viruses spread freely in tissues via lytic cellular infection but some can also spread directly, from cell-to-cell. It is the latter types that are more protected by the effects of humoral immunity and lack of B cells would not be expected to make a significant difference to their survival. Indeed, herpes viruses are known to spread via direct cell-to-cell contact in neuronal networks (175) and this may explain why reports of severe or atypical herpes viral infections in adult XLA patients are lacking in the literature. Another example of a virus that is not reported frequently in patients with absent circulating B cells is Epstein Barr virus (EBV), in agreement with the notion that B lymphocytes likely represent the primary reservoir for this virus (151).
The examples discussed above are unlikely to be the only ones. Severe or persistent viral infections are much more likely to be diagnosed and/or be reported in the literature, but mild viral infections are likely to be frequent and may contribute to the development of other infections as well. In comparison to bacterial infections, viral infections tend to be more difficult to diagnose and widely available diagnostic tests are lacking for most of them. Another complicating factor is that serological methods tend to be unreliable in antibody deficient patients, both due to their dependency on native IgG production and due to the frequent external administration of therapeutic IgG. Furthermore, viral infections tend to be of lower clinical interest than bacterial, e.g. in lower respiratory tract infection, and are less often interrogated by clinicians. The small numbers of patients that suffer with congenital B cell alymphocytosis have made it more difficult for this association to be established in the past, but the more prevalent use of rituximab in recent years has helped bring it into focus. The few studies that have specifically looked into the frequency of viral infections in patients with agammaglobulinemia also lend support to this association.
There are several reasons why B cell alymphocytosis may confer a particular predisposition to viral infections. Peripheral B cells are generally a good marker of the overall content of tissue B cells and when absent this commonly translates into very poor antibody responses to infection. In most other humoral immunodeficiencies, e.g. in CVID patients with normal B cells, antibody responses may be sufficient enough to confer protection from viral infections. Patients with absent circulating B cells are very likely to have absent IgA and IgM antibodies, the latter having important roles in antiviral immunity; e.g. IgA in mucosal tissues and IgM as the first isotype responder to infection (176). Finally, B cells may also play an important role themselves, due to their ability to act as antigen-presenting cells and thus stimulate cellular immunity.
Of course, patients with defective humoral responses are less prone to serious viral infections than those with defective cellular immunity but that does not necessarily reflect a lack of importance of B cells in antiviral immunity. It rather highlights the importance of T helper cells in priming humoral immunity, via the generation of memory B cells and long-lived plasma cells. Humoral immunity is effective in neutralizing viral particles and thus preventing their spread through tissues but once an infection is established, cellular immunity is more important via its role in eliminating virally infected cells. In line with this notion, hyperimmune globulin, immunoglobulin preparations that are high in antibodies against a specific disease, are available for prophylactic use of several types of viral infections and can even prevent progression when used early on in the course of an infection (177–179). However, they tend to be less effective when it comes to treating an established viral infection (180, 181).
Understanding the importance of humoral immunity, and serum B cells in particular, in the defense against viral infections has important clinical implications. Firstly, circulating B cells may be used as a screening tool to risk-stratify patients that are infected by viruses. This may be particularly important for SARS-CoV2 infection, as is underlined by multitude of the recently published case reports. Secondly, physicians need to be vigilant with the use of B cell depleting therapies, particularly in those patients that have a weakened immune system. The need to monitor B cell counts in these patients has also been recommended in the past. Thirdly, strategies may need to be adapted to protect patients with very low serum B cells, e.g. through the use of infection control measures, antiviral prophylaxis and with prompt investigation and treatment of infectious episodes.
Clinicians caring for patients that harbor humoral immune defects will also need to be vigilant for viral infections and include them in their differential. Live viral vaccines need to be avoided in these patients. Previous cases of post-vaccination poliomyelitis have raised awareness and live OPV is now contraindicated in the above immunodeficiencies. Guidance on the use of other live vaccines is however less clear, e.g. the Green Book, a UK government publication, recommends that live vaccines should not be administered to individuals who are on or have received immunosuppressive biological therapy in the past 12 months (182). However, antibody deficiencies are not considered to be a contraindication (182). The Infectious Diseases Society of America on the other hand proposes that live vaccines should be avoided equally in patients with major antibody deficiencies (e.g. XLA or CVID) and in those on biologic immunosuppressive therapy (183). Similarly, the Immune Deficiency Foundation advises that antibody deficient patients with absent B cells should avoid live viral vaccines (184). The above literature adds support to this view and measuring circulating B cells may even offer an easy way to discriminate between CVID patients that can and those that should not receive these vaccines.
Overall, the evidence summarized above suggests that atypical and opportunistic viral infections are not uncommon in patients with low or absent peripheral B cells and offers us an insight into the importance of humoral immunity in the defense against viral infections. Physicians should be vigilant for these types of infections in patients with low B cells and follow appropriate prophylactic measures.
Data Availability Statement
The original contributions presented in the study are included in the article/supplementary material. Further inquiries can be directed to the corresponding author.
Author Contributions
AG: conceptualized, wrote first draft and edited manuscript. MD: edited manuscript. SJ: edited manuscript. MG: edited manuscript. All authors contributed to the article and approved the submitted version.
Conflict of Interest
The authors declare that the research was conducted in the absence of any commercial or financial relationships that could be construed as a potential conflict of interest.
Publisher’s Note
All claims expressed in this article are solely those of the authors and do not necessarily represent those of their affiliated organizations, or those of the publisher, the editors and the reviewers. Any product that may be evaluated in this article, or claim that may be made by its manufacturer, is not guaranteed or endorsed by the publisher.
References
1. Tam JS, Routes JM. Common Variable Immunodeficiency. Am J Rhinol Allergy (2013) 27:260–5. doi: 10.2500/ajra.2013.27.3899
2. Gaspar HB, Qasim W, Davies EG, Rao K, Amrolia PJ, Veys P. How I Treat Severe Combined Immunodeficiency. Blood (2013) 122:3749–58. doi: 10.1182/blood-2013-02-380105
3. Forthal DN. Functions of Antibodies. Microbiol Spectr (2014) 2:1–17. doi: 10.1128/9781555817411.ch2
4. Lamm ME. The IgA Mucosal Immune System. Am J Kidney Dis (1988) 12:384–7. doi: 10.1016/S0272-6386(88)80030-1
5. Neurath AR. Immune Response to Viruses: Antibody-Mediated Immunity. Encycl Virol (2008) 56–70. doi: 10.1016/B978-012374410-4.00591-4
6. Panagioti E, Klenerman P, Lee LN, van der Burg SH, Arens R. Features of Effective T Cell-Inducing Vaccines Against Chronic Viral Infections. Front Immunol (2018) 9:276. doi: 10.3389/fimmu.2018.00276
7. Clements T, Rice TF, Vamvakas G, Barnett S, Barnes M, Donaldson B, et al. Update on Transplacental Transfer of IgG Subclasses: Impact of Maternal and Fetal Factors. Front Immunol (2020) 11:1920. doi: 10.3389/fimmu.2020.01920
8. Lougaris V, Soresina A, Baronio M, Montin D, Martino S, Signa S, et al. Long-Term Follow-Up of 168 Patients With X-Linked Agammaglobulinemia Reveals Increased Morbidity and Mortality. J Allergy Clin Immunol (2020) 146:429–37. doi: 10.1016/j.jaci.2020.03.001
9. McKinney REJ, Katz SL, Wilfert CM. Chronic Enteroviral Meningoencephalitis in Agammaglobulinemic Patients. Rev Infect Dis (1987) 9:334–56. doi: 10.1093/clinids/9.2.334
10. Wilfert CM, Buckley RH, Mohanakumar T, Griffith JF, Katz SL, Whisnant JK, et al. Persistent and Fatal Central-Nervous-System ECHOvirus Infections in Patients With Agammaglobulinemia. N Engl J Med (1977) 296:1485–9. doi: 10.1056/NEJM197706302962601
11. Lederman HM. Winkelstein JA. X-Linked Agammaglobulinemia: An Analysis of 96 Patients. Med (Baltimore) (1985) 64:145–56. doi: 10.1097/00005792-198505000-00001
12. Bearden D, Collett M, Quan PL, Costa-Carvalho BT, Sullivan KE. Enteroviruses in X-Linked Agammaglobulinemia: Update on Epidemiology and Therapy. J Allergy Clin Immunol Pract (2016) 4:1059–65. doi: 10.1016/j.jaip.2015.12.015
13. Gofshteyn J, Cárdenas AM, Bearden D. Treatment of Chronic Enterovirus Encephalitis With Fluoxetine in a Patient With X-Linked Agammaglobulinemia. Pediatr Neurol (2016) 64:94–8. doi: 10.1016/j.pediatrneurol.2016.06.014
14. Quartier P, Foray S, Casanova JL, Hau-Rainsard I, Blanche S, Fischer A. Enteroviral Meningoencephalitis in X-Linked Agammaglobulinemia: Intensive Immunoglobulin Therapy and Sequential Viral Detection in Cerebrospinal Fluid by Polymerase Chain Reaction. Pediatr Infect Dis J (2000) 19:1106–8. doi: 10.1097/00006454-200011000-00020
15. Misbah SA, Spickett GP, Ryba PC, Hockaday JM, Kroll JS, Sherwood C, et al. Chronic Enteroviral Meningoencephalitis in Agammaglobulinemia: Case Report and Literature Review. J Clin Immunol (1992) 12:266–70. doi: 10.1007/BF00918150
16. Kasahara Y, Imamura M, Shin C, Shimizu H, Utsumi J, Hosokai R, et al. Fatal Progressive Meningoencephalitis Diagnosed in Two Members of a Family With X-Linked Agammaglobulinemia. Front Pediatr (2020) 8:579. doi: 10.3389/fped.2020.00579
17. Sanna PP, Burton DR. Role of Antibodies in Controlling Viral Disease: Lessons From Experiments of Nature and Gene Knockouts. J Virol (2000) 74:9813–7. doi: 10.1128/jvi.74.21.9813-9817.2000
18. Winkelstein JA, Marino MC, Lederman HM, Jones SM, Sullivan K, Burks AW, et al. Ochs HD. X-Linked Agammaglobulinemia: Report on a United States Registry of 201 Patients. Med (Baltimore) (2006) 85:193–202. doi: 10.1097/01.md.0000229482.27398.ad
19. Rudge P, Webster AD, Revesz T, Warner T, Espanol T, Cunningham-Rundles C, et al. Encephalomyelitis in Primary Hypogammaglobulinaemia. Brain (1996) 119(Pt 1):1–15. doi: 10.1093/brain/119.1.1
20. Shaghaghi M, Soleyman-jahi S, Abolhassani H, Yazdani R, Azizi G, Rezaei N, et al. New Insights Into Physiopathology of Immunodeficiency-Associated Vaccine-Derived Poliovirus Infection; Systematic Review of Over 5 Decades of Data. Vaccine (2018) 36:1711–9. doi: 10.1016/j.vaccine.2018.02.059
21. Lopez Granados E, Porpiglia AS, Hogan MB, Matamoros N, Krasovec S, Pignata C, et al. Clinical and Molecular Analysis of Patients With Defects in Micro Heavy Chain Gene. J Clin Invest (2002) 110:1029–35. doi: 10.1172/JCI15658
22. Al-Herz W, Essa S. Spectrum of Viral Infections Among Primary Immunodeficient Children: Report From a National Registry. Front Immunol (2019) 10:1231. doi: 10.3389/fimmu.2019.01231
23. NaserEddin A, Shamriz O, Keller B, Alzyoud RM, Unger S, Fisch P, et al. Enteroviral Infection in a Patient With BLNK Adaptor Protein Deficiency. J Clin Immunol (2015) 35:356–60. doi: 10.1007/s10875-015-0164-2
24. El-Sayed ZA, Abramova I, Aldave JC, Al-Herz W, Bezrodnik L, Boukari R, et al. X-Linked Agammaglobulinemia (XLA): Phenotype, Diagnosis, and Therapeutic Challenges Around the World. World Allergy Organ J (2019) 12:100018. doi: 10.1016/j.waojou.2019.100018
25. Yel L, Minegishi Y, Coustan-Smith E, Buckley RH, Trübel H, Pachman LM, et al. Mutations in the Mu Heavy-Chain Gene in Patients With Agammaglobulinemia. N Engl J Med (1996) 335:1486–93. doi: 10.1056/NEJM199611143352003
26. Radanović I, Rkman D, Zekan P, Kutleša M, Baršić B. Chronic Meningoencephalitis Caused by Echo Virus 6 in a Patient With Common Variable Immunodeficiency: Successful Treatment With Pleconaril. Wien Klin Wochenschr (2018) 130:70–2. doi: 10.1007/s00508-017-1289-5
27. Lau YL, Levinsky RJ, Morgan G, Strobel S. Dual Meningoencephalitis With Echovirus Type 11 and Adenovirus in Combined (Common Variable) Immunodeficiency. Pediatr Infect Dis J (1988) 7:873–6. doi: 10.1097/00006454-198807120-00008
28. Slade CA, McLean C, Scerri T, Giang TB, Megaloudis S, Strathmore A, et al. Fatal Enteroviral Encephalitis in a Patient With Common Variable Immunodeficiency Harbouring a Novel Mutation in NFKB2. J Clin Immunol (2019) 39:324–35. doi: 10.1007/s10875-019-00602-x
29. Nguyen JT-U, Green A, Wilson MR, DeRisi JL, Gundling K. Neurologic Complications of Common Variable Immunodeficiency. J Clin Immunol (2016) 36:793–800. doi: 10.1007/s10875-016-0336-8
30. Driss N, Ben-Mustapha I, Mellouli F, Ben Yahia A, Touzi H, Bejaoui M, et al. High Susceptibility for Enterovirus Infection and Virus Excretion Features in Tunisian Patients With Primary Immunodeficiencies. Clin Vaccine Immunol (2012) 19:1684–9. doi: 10.1128/CVI.00293-12
31. Li L, Ivanova O, Driss N, Tiongco-Recto M, da Silva R, Shahmahmoodi S, et al. Poliovirus Excretion Among Persons With Primary Immune Deficiency Disorders: Summary Of a Seven-Country Study Series. J Infect Dis (2014) 210(Suppl):S368–72. doi: 10.1093/infdis/jiu065
32. Grisariu S, Vaxman I, Gatt M, Elias S, Avni B, Arad A, et al. Enteroviral Infection in Patients Treated With Rituximab for non-Hodgkin Lymphoma: A Case Series and Review of the Literature. Hematol Oncol (2017) 35:591–8. doi: 10.1002/hon.2365
33. Makatsori M, Kiani-Alikhan S, Manson AL, Verma N, Leandro M, Gurugama NP, et al. Hypogammaglobulinaemia After Rituximab Treatment-Incidence and Outcomes. QJM (2014) 107:821–8. doi: 10.1093/qjmed/hcu094
34. Aksoy S, Harputluoglu H, Kilickap S, Dede DS, Dizdar O, Altundag K, et al. Rituximab-Related Viral Infections in Lymphoma Patients. Leuk Lymphoma (2007) 48:1307–12. doi: 10.1080/10428190701411441
35. Schilthuizen C, Berenschot HWA, Levin M-D. Enteroviral Encephalitis in a Patient With a Marginal Zone Lymphomatreated With Rituximab. Neth J Med (2010) 68:221–3.
36. Palacios T, Bartelt L, Scheld W, Lopes MB, Kelting SM, Holland S, et al. Fatal Coxsackie Meningoencephalitis in a Patient With B-Cell Lymphopenia and Hypogammaglobulinemia Following Rituximab Therapy. Ann allergy Asthma Immunol (2015) 115:148–50. doi: 10.1016/j.anai.2015.05.007
37. Tellez R, Lastinger AM, Hogg JP. Chronic Enteroviral Meningoencephalitis in a Patient on Rituximab for the Treatment Of Psoriatic Arthritis: A Case Report and Brief Literature Review. IDCases (2019) 17:e00558. doi: 10.1016/j.idcr.2019.e00558
38. Garzo-Caldas N, Ruiz-Sainz E, Vila-Bedmar S, Llamas-Velasco S, Hernández-Lain A, Ruiz-Morales J, et al. Enteroviral T-Cell Encephalitis Related to Immunosuppressive Therapy Including Rituximab. Neurology (2017) 89:408–9. doi: 10.1212/WNL.0000000000004148
39. Quartier P, Tournilhac O, Archimbaud C, Lazaro L, Chaleteix C, Millet P, et al. Enteroviral Meningoencephalitis After Anti-CD20 (Rituximab) Treatment. Clin Infect Dis (2003) 36:e47–9. doi: 10.1086/345746
40. Padate BP, Keidan J. Enteroviral Meningoencephalitis in a Patient With non-Hodgkin’s Lymphoma Treated Previously With Rituximab. Clin Lab Haematol (2006) 28:69–71. doi: 10.1111/j.1365-2257.2006.00751.x
41. Ahmed R, Buckland M, Davies L, Halmagyi GM, Rogers SL, Oberste S, et al. Enterovirus 71 Meningoencephalitis Complicating Rituximab Therapy. J Neurol Sci (2011) 305:149–51. doi: 10.1016/j.jns.2011.03.009
42. Kiani-Alikhan S, Skoulidis F, Barroso A, Nuovo G, Ushiro-Lumb I, Breuer J, et al. Enterovirus Infection of Neuronal Cells Post-Rituximab. Br J Haematol (2009) 146:333–5. doi: 10.1111/j.1365-2141.2009.07748.x
43. Kassab S, Saghi T, Boyer A, Lafon M-E, Gruson D, Lina B, et al. Fatal Case of Enterovirus 71 Infection and Rituximab Therapy, France, 2012. Emerg Infect Dis (2013) 19:1345–7. doi: 10.3201/eid1908.130202
44. Shaheen N, Mussai F. Enteroviral Encephalitis in a Child With CNS Relapse of Burkitt Leukemia Treated With Rituximab. J Pediatr Hematol Oncol (2019) 41:e27–9. doi: 10.1097/MPH.0000000000001077
45. Sham L, Bitnun A, Branson H, Hazrati L-N, Dell SD, Yeung RSM, et al. Treatment of Rituximab-Associated Chronic CNS Enterovirus Using IVIg and Fluoxetine. Neurology (2019) 92:916–8. doi: 10.1212/WNL.0000000000007468
46. Bajema KL, Simonson PD, Greninger AL, Çoruh B, Pottinger PS, Bhattacharya R, et al. Acute Liver Failure Due to Echovirus 9 Associated With Persistent B-Cell Depletion From Rituximab. Open Forum Infect Dis (2017) 4:ofx174. doi: 10.1093/ofid/ofx174
47. Morgan C, Thomson SJ, Legg J. Narat S. A Case of Fulminant Hepatitis Due to Echovirus 9 in a Patient on Maintenance Rituximab Therapy for Follicular Lymphoma. Case Rep Hematol (2015) 2015:454890. doi: 10.1155/2015/454890
48. Dendle C, Gilbertson M, Korman TM, Golder V, Morand E, Opat S. Disseminated Enteroviral Infection Associated With Obinutuzumab. Emerg Infect Dis (2015) 21:1661–3. doi: 10.3201/eid2109.150104
49. Heger J-M, Eichenauer DA, Kasper P, Böll B, Shimabukuro-Vornhagen A, Kochanek M. Fatal Disseminated Enterovirus Infection in a Patient With Follicular Lymphoma Undergoing Obinutuzumab Maintenance Therapy. Eur J Haematol (2019) 103:268–71. doi: 10.1111/ejh.13278
50. Eyckmans T, Wollants E, Janssens A, Schoemans H, Lagrou K, Wauters J, et al. Coxsackievirus A16 Encephalitis During Obinutuzumab Therapy, Belgium, 2013. Emerg Infect Dis (2014) 20:913–5. doi: 10.3201/eid2005.131766
51. Nicolini LA, Canepa P, Caligiuri P, Mikulska M, Novi G, Viscoli C, et al. Fulminant Hepatitis Associated With Echovirus 25 During Treatment With Ocrelizumab for Multiple Sclerosis. JAMA Neurol (2019) 76:866–7. doi: 10.1001/jamaneurol.2019.0522
52. Narula S, LaRosa DF, Kamoun M, Dalmau J, Levinson AI. Progressive Multifocal Leukoencephalopathy in a Patient With Common Variable Immunodeficiency and Abnormal CD8+ T-Cell Subset Distribution. Ann allergy Asthma Immunol Off Publ Am Coll Allergy Asthma Immunol (2007) 98:483–9. doi: 10.1016/S1081-1206(10)60764-8
53. Squintani G, Ferrari S, Bazzoli E, Eleopra R, La Monaca C, Cagliari E, et al. Progressive Multifocal Leukoencephalopathy in a Patient With Good’s Syndrome. Int J Infect Dis IJID Off Publ Int Soc Infect Dis (2010) 14:e444–7. doi: 10.1016/j.ijid.2009.06.005
54. Sveinsson O, Matell H, Herrman L. Progressive Multifocal Leukoencephalopathy in a Patient With Good’s Syndrome. BMJ Case Rep (2013) 2013. doi: 10.1136/bcr-2013-009763
55. Ueno T, Sato N, Kon T, Haga R, Nunomura J-I, Nakamichi K, et al. Progressive Multifocal Leukoencephalopathy Associated With Thymoma With Immunodeficiency: A Case Report and Literature Review. BMC Neurol (2018) 18:37. doi: 10.1186/s12883-018-1041-4
56. Capobianco M, Pulizzi A, Bertolotto A. Progressive Multifocal Leukoencephalopathy in Good’s Syndrome. Int J Infect Dis (2010) 14(Suppl 3):e367–8. doi: 10.1016/j.ijid.2010.02.2260
57. Tuccori M, Focosi D, Blandizzi C, Pelosini M, Montagnani S, Maggi F, et al. Inclusion of Rituximab in Treatment Protocols for non-Hodgkin’s Lymphomas and Risk for Progressive Multifocal Leukoencephalopathy. Oncologist (2010) 15:1214–9. doi: 10.1634/theoncologist.2010-0098
58. Carson KR, Evens AM, Richey EA, Habermann TM, Focosi D, Seymour JF, et al. Progressive Multifocal Leukoencephalopathy After Rituximab Therapy in HIV-Negative Patients: A Report of 57 Cases From the Research on Adverse Drug Events and Reports Project. Blood (2009) 113:4834–40. doi: 10.1182/blood-2008-10-186999
59. Quan PL, Wagner TA, Briese T, Torgerson TR, Hornig M, Tashmukhamedova A, et al. Astrovirus Encephalitis in Boy With X-Linked Agammaglobulinemia. Emerg Infect Dis (2010) 16:918–25. doi: 10.3201/eid1606.091536
60. Frémond M-L, Pérot P, Muth E, Cros G, Dumarest M, Mahlaoui N, et al. Next-Generation Sequencing for Diagnosis and Tailored Therapy: A Case Report of Astrovirus-Associated Progressive Encephalitis. J Pediatr Infect Dis Soc (2015) 4:e53–7. doi: 10.1093/jpids/piv040
61. Machado P, Santos A, Faria E, Silva J, Malcata A, Chieira C. Arthritis and X-Linked Agammaglobulinemia. Acta Reumatol Port (2008) 33:464–7.
62. Kainulainen L, Vuorinen T, Rantakokko-Jalava K, Osterback R, Ruuskanen O. Recurrent and Persistent Respiratory Tract Viral Infections in Patients With Primary Hypogammaglobulinemia. J Allergy Clin Immunol (2010) 126:120–6. doi: 10.1016/j.jaci.2010.04.016
63. Iyer A, Mathur R, Deepak BV, Sinard J. Fatal Adenoviral Hepatitis After Rituximab Therapy. Arch Pathol Lab Med (2006) 130:1557–60. doi: 10.1043/1543-2165(2006)130[1557:FAHART]2.0.CO;2
64. Bitnun A, Shannon P, Durward A, Rota PA, Bellini WJ, Graham C, et al. Measles Inclusion-Body Encephalitis Caused by the Vaccine Strain of Measles Virus. Clin Infect Dis an Off Publ Infect Dis Soc Am (1999) 29:855–61. doi: 10.1086/520449
65. Jent P, Trippel M, Frey M, Pöllinger A, Berezowska S, Langer R, et al. Fatal Measles Virus Infection After Rituximab-Containing Chemotherapy in a Previously Vaccinated Patient. Open Forum Infect Dis (2018) 5:ofy244–4. doi: 10.1093/ofid/ofy244
66. Bodemer C, Sauvage V, Mahlaoui N, Cheval J, Couderc T, Leclerc-Mercier S, et al. Live Rubella Virus Vaccine Long-Term Persistence as an Antigenic Trigger of Cutaneous Granulomas in Patients With Primary Immunodeficiency. Clin Microbiol Infect Off Publ Eur Soc Clin Microbiol Infect Dis (2014) 20:O656–63. doi: 10.1111/1469-0691.12573
67. Perelygina L, Chen M-H, Suppiah S, Adebayo A, Abernathy E, Dorsey M, et al. Infectious Vaccine-Derived Rubella Viruses Emerge, Persist, and Evolve in Cutaneous Granulomas of Children With Primary Immunodeficiencies. PloS Pathog (2019) 15:e1008080. doi: 10.1371/journal.ppat.1008080
68. Murguia-Favela L, Hiebert J, Haber RM. “Noninfectious” Cutaneous Granulomas in Primary Immunodeficiency Patients and Association With Rubella Virus Vaccine Strain. J Cutan Med Surg (2019) 23:341–2. doi: 10.1177/1203475419825780
69. Perelygina L, Icenogle J, Sullivan KE. Rubella Virus-Associated Chronic Inflammation in Primary Immunodeficiency Diseases. Curr Opin Allergy Clin Immunol (2020) 20:574–81. doi: 10.1097/ACI.0000000000000694
70. Bakri FG, Bahou YG, Al-Sammarrai FA, Hadidy A, Gharaibeh A, Zaid GK, et al. Fatal Encephalitis Due to BK Virus in a Patient With Common Variable Immunodeficiency: A Case Report. J Clin Virol Off Publ Pan Am Soc Clin Virol (2013) 57:363–9. doi: 10.1016/j.jcv.2013.04.016
71. Patel SJ, Devos JM, Knight RJ, Dawson KL, Suki WN, Gonzalez JM, et al. Effects of Rituximab on the Development of Viral and Fungal Infections in Renal Transplant Recipients. ISRN Transplant (2013) 2013:819025. doi: 10.5402/2013/819025
72. Alonto AM, Aronoff DM, Malani PN. West Nile Virus Meningitis in Patient With Common Variable Immunodeficiency. Emerg Infect Dis (2003) 9:1353–4. doi: 10.3201/eid0910.030195
73. Owens M, Choe L, Rivera J, Avila D. West Nile Virus Neuroinvasive Disease Associated With Rituximab Therapy (P4.9-022). Neurology (2019) 92:P4.9–022. doi: 10.1007/s13365-020-00854-z
74. Morjaria S, Arguello E, Taur Y, Sepkowitz K, Hatzoglou V, Nemade A, et al. West Nile Virus Central Nervous System Infection in Patients Treated With Rituximab: Implications for Diagnosis and Prognosis, With a Review of Literature. Open Forum Infect Dis (2015) 2:ofv136–6. doi: 10.1093/ofid/ofv136
75. Honig A, Karussis D. Delayed-Onset Flaccid Paralysis Related to West Nile Virus Reactivation Following Treatment With Rituximab: A Case Report. BMC Res Notes (2014) 7:852. doi: 10.1186/1756-0500-7-852
76. Mawhorter SD, Sierk A, Staugaitis SM, Avery RK, Sobecks R, Prayson RA, et al. Fatal West Nile Virus Infection After Rituximab/Fludarabine–Induced Remission for Non-Hodgkin’s Lymphoma. Clin Lymphoma Myeloma (2005) 6:248–50. doi: 10.3816/CLM.2005.n.053
77. Goates C, Tsuha S, Working S, Carey J, Spivak ES. Seronegative West Nile Virus Infection in a Patient Treated With Rituximab for Rheumatoid Arthritis. Am J Med (2017) 130:e257–8. doi: 10.1016/j.amjmed.2017.01.014
78. Rolfes MC, Sriaroon P, Dávila Saldaña BJ, Dvorak CC, Chapdelaine H, Ferdman RM, et al. Chronic Norovirus Infection in Primary Immune Deficiency Disorders: An International Case Series. Diagn Microbiol Infect Dis (2019) 93:69–73. doi: 10.1016/j.diagmicrobio.2018.08.002
79. Heath Jeffery R, Fadia M, Morey A. Chronic Norovirus Infection in Common Variable Immune Deficiency (CVID)- A Case Report and Literature Review. Pathology (2020) 52:S139. doi: 10.1016/j.pathol.2020.01.019
80. Woodward JM, Gkrania-Klotsas E, Cordero-Ng AY, Aravinthan A, Bandoh BN, Liu H, et al. The Role of Chronic Norovirus Infection in the Enteropathy Associated With Common Variable Immunodeficiency. Am J Gastroenterol (2015) 110:320–7. doi: 10.1038/ajg.2014.432
81. Woodward J, Ng A, Aravinthan A, Bandoh B, Liu H, Davies S, et al. PTU-161 Chronic Norovirus Infection as a Cause of Common Variable Immunodeficiency-Associated Enteropathy. Gut (2012) 61:A251 LP–A251. doi: 10.1136/gutjnl-2012-302514c.161
82. Woodward J, Gkrania-Klotsas E, Kumararatne D. Chronic Norovirus Infection and Common Variable Immunodeficiency. Clin Exp Immunol (2017) 188:363–70. doi: 10.1111/cei.12884
83. Knoll BM, Lindesmith LC, Yount BL, Baric RS, Marty FM. Resolution of Diarrhea in an Immunocompromised Patient With Chronic Norovirus Gastroenteritis Correlates With Constitution of Specific Antibody Blockade Titer. Infection (2016) 44:551–4. doi: 10.1007/s15010-016-0875-1
84. Endo LM, Giannobile JV, Dobbs AK, Foote JB, Szymanska E, Warnock DG, et al. Membranous Glomerulopathy in an Adult Patient With X-Linked Agammaglobulinemia Receiving Intravenous Gammaglobulin. J Investig Allergol Clin Immunol (2011) 21:405–9.
85. Borish L, Ayars AG, Kirkpatrick CH. Common Variable Immunodeficiency Presenting as Herpes Simplex Virus Encephalitis. J Allergy Clin Immunol (2011) 127:541–3. doi: 10.1016/j.jaci.2010.11.004
86. Ansari M, Jha S. Herpes Simplex Encephalitis in a Patient Having Common Variable Immuno-Deficiency. Ann Trop Med Public Heal (2010) 3:30–2. doi: 10.4103/1755-6783.76183
87. Tarr PE, Sneller MC, Mechanic LJ, Economides A, Eger CM, Strober W, et al. Infections in Patients With Immunodeficiency With Thymoma (Good Syndrome). Report of 5 Cases and Review of the Literature. Med (Baltimore) (2001) 80:123–33. doi: 10.1097/00005792-200103000-00005
88. Miyakis S, Pefanis A, Passam FH, Christodulakis GR, Roussou PA, Mountokalakis TD. Thymoma With Immunodeficiency (Good’s Syndrome): Review of the Literature Apropos Three Cases. Scand J Infect Dis (2006) 38:314–20. doi: 10.1080/00365540500372663
89. Beck S, Slater D, Harrington CI. Fatal Chronic Cutaneous Herpes Simplex Associated With Thymoma and Hypogammaglobulinaemia. Br J Dermatol (1981) 105:471–4. doi: 10.1111/j.1365-2133.1981.tb00782.x
90. Jansen A, van Deuren M, Miller J, Litzman J, de Gracia J, Sáenz-Cuesta M, et al. Prognosis of Good Syndrome: Mortality and Morbidity of Thymoma Associated Immunodeficiency in Perspective. Clin Immunol (2016) 171:12–7. doi: 10.1016/j.clim.2016.07.025
91. Malphettes M, Gérard L, Galicier L, Boutboul D, Asli B, Szalat R, et al. Good Syndrome: An Adult-Onset Immunodeficiency Remarkable for its High Incidence of Invasive Infections and Autoimmune Complications. Clin Infect Dis an Off Publ Infect Dis Soc Am (2015) 61:e13–9. doi: 10.1093/cid/civ269
92. Méan M, Schaller MD, Asner S, Meylan P, Pagani J-L, Tarr P, et al. Thymoma, Immunodeficiency, and Herpes Simplex Virus Infections. Med Mal Infect (2009) 39:344–7. doi: 10.1016/j.medmal.2009.01.008
93. Matta L, Ramírez-Velasco MC, Zea-Vera AF. Herpes Simplex Virus Type 2 Meningitis as a Manifestation of Good’s Syndrome. J Neurovirol (2020) 26:429–32. doi: 10.1007/s13365-019-00819-x
94. Vaddiparti A, Manwani B, Hosley C, Tremblay M. HSV Encephalitis Associated With Off-Label Rituximab Use in a Case of Relapsing Multiple Sclerosis. (P5.390). Neurology (2017) 88:P5.390.
95. Bernauer W, Schuler S, Borradori L. Rituximab and Bilateral HSV Epithelial Keratitis in a Patient With Mucous Membrane Pemphigoid. J Ophthalmic Inflammation Infect (2018) 8:12. doi: 10.1186/s12348-018-0153-8
96. Thong L, Plant BJ, McCarthy J, Murphy DM. Herpetic Tracheitis in Association With Rituximab Therapy. Respirol Case Rep (2016) 4:e00158–8. doi: 10.1002/rcr2.158
97. Raeiszadeh M, Kopycinski J, Paston SJ, Diss T, Lowdell M, Hardy GAD, et al. The T Cell Response to Persistent Herpes Virus Infections in Common Variable Immunodeficiency. Clin Exp Immunol (2006) 146:234–42. doi: 10.1111/j.1365-2249.2006.03209.x
98. Aghamohammadi A, Abolhassani H, Hirbod-Mobarakeh A, Ghassemi F, Shahinpour S, Behniafard N, et al. The Uncommon Combination of Common Variable Immunodeficiency, Macrophage Activation Syndrome, and Cytomegalovirus Retinitis. Viral Immunol (2012) 25:161–5. doi: 10.1089/vim.2011.0060
99. Sun X, Shi J, Wang M, Xu K, Xiao Y. Good’s Syndrome Patients Hospitalized for Infections: A Single-Center Retrospective Study. Med (Baltimore) (2015) 94:e2090. doi: 10.1097/MD.0000000000002090
100. Assi AC, Lightman S. Cytomegalovirus Retinitis in Patients With Good Syndrome. Arch Ophthalmol (2002) 120:510–2.
101. Downes KM, Tarasewicz D, Weisberg LJ, Cunningham ETJ. Good Syndrome and Other Causes of Cytomegalovirus Retinitis in HIV-Negative Patients-Case Report and Comprehensive Review of the Literature. J Ophthalmic Inflammation Infect (2016) 6:3. doi: 10.1186/s12348-016-0070-7
102. Mateo-Montoya A, Stanescu D, Wolff B, Sahel J-A, Bonnel S. Cytomegalovirus Retinitis Associated With Good’s Syndrome. Eur J Ophthalmol (2010) 20:479–80. doi: 10.1177/112067211002000238
103. Sen HN, Robinson MR, Fischer SH. CMV Retinitis in a Patient With Good Syndrome. Ocul Immunol Inflammation (2005) 13:475–8. doi: 10.1080/09273940590950963
104. Isobe S, Sano A, Otsuka H, Azuma Y, Koezuka S, Makino T, et al. Good Syndrome With Cytomegalovirus Hepatitis: Successful Resection of Thymoma: A Case Report. J Cardiothorac Surg (2020) 15:141. doi: 10.1186/s13019-020-01187-y
105. Cucchiara BL, Forman MS, McGarvey ML, Kasner SE, King D. Fatal Subacute Cytomegalovirus Encephalitis Associated With Hypogammaglobulinemia and Thymoma. Mayo Clin Proc (2003) 78:223–7. doi: 10.4065/78.2.223
106. Ueno S, Sekimoto-Tsuboi S, Ishiguro Y, Koinuma T, Eguchi H, Machida Y, et al. Good’s Syndrome With Opportunistic Infection of the Central Nervous System: A Case Report. BMC Neurol (2015) 15:150. doi: 10.1186/s12883-015-0406-1
107. Le Clech L, Ianotto J-C, Quintin-Roue I, Tempescul A. Severe CMV Complication Following Maintenance Therapy With Rituximab. BMJ Case Rep (2013) 2013. doi: 10.1136/bcr-2012-006672
108. Siakantaris MP, Argyropoulos KV, Ioannou S, Papadopoulou V, Tzeletas G, Tsonis J, et al. Cytomegalovirus Meningoencephalitis After Rituximab Treatment for Primary Central Nervous System Lymphoma. Neurologist (2015) 19:35–7. doi: 10.1097/NRL.0b013e318287fde0
109. Hilal T. Fatal Cytomegalovirus Disease After Combination Therapy With Corticosteroids and Rituximab for Granulomatosis With Polyangiitis. Case Rep Rheumatol (2015) 2015:538137. doi: 10.1155/2015/538137
110. Suzan F, Ammor M, Ribrag V. Fatal Reactivation of Cytomegalovirus Infection After Use of Rituximab for a Post-Transplantation Lymphoproliferative Disorder. N Engl J Med (2001) 345:1000. doi: 10.1056/NEJM200109273451315
111. Vallet H, Houitte R, Azria A, Mariette X. Cytomegalovirus Colitis and Hypo-IgG After Rituximab Therapy for Rheumatoid Arthritis. J Rheumatol (2011) 38:965 LP – 966. doi: 10.3899/jrheum.100818
112. Soden JS, Narkewicz MR, Haas JE, Sokol RJ. Hepatic Veno-Occlusive Disease and Human Herpes Virus 7 Infection in Primary Agammaglobulinemia. J Pediatr (2009) 154:299–302. doi: 10.1016/j.jpeds.2008.08.048
113. Daugherty WP, Clarke MJ, Cloft HJ, Lanzino GL. Going Viral: Fusiform Vertebrobasilar and Internal Carotid Aneurysms With Varicella Angiitis and Common Variable Immunodeficiency. J Neurosurg Pediatr (2009) 4:528–31. doi: 10.3171/2009.7.PEDS09107
114. Watts RG, Kelly DR. Fatal Varicella Infection in a Child Associated With Thymoma and Immunodeficiency (Good’s Syndrome). Med Pediatr Oncol (1990) 18:246–51. doi: 10.1002/mpo.2950180318
115. Bermúdez A, Marco F, Conde E, Mazo E, Recio M, Zubizarreta A. Fatal Visceral Varicella-Zoster Infection Following Rituximab and Chemotherapy Treatment in a Patient With Follicular Lymphoma. Haematologica (2000) 85:894–5.
116. Costa E, Buxton J, Brown J, Templeton KE, Breuer J, Johannessen I. Fatal Disseminated Varicella Zoster Infection Following Zoster Vaccination in an Immunocompromised Patient. BMJ Case Rep (2016) 2016:bcr2015212688. doi: 10.1136/bcr-2015-212688
117. Nobre FA, Gonzalez IG da S, de Moraes-Pinto MI, Costa-Carvalho BT. Protective Levels of Varicella-Zoster Antibody did Not Effectively Prevent Chickenpox in an X-Linked Agammaglobulinemia Patient. Rev Inst Med Trop Sao Paulo (2015) 57:455–7. doi: 10.1590/s0036-46652015000500017
118. Wheat WH, Cool CD, Morimoto Y, Rai PR, Kirkpatrick CH, Lindenbaum BA, et al. Possible Role of Human Herpesvirus 8 in the Lymphoproliferative Disorders in Common Variable Immunodeficiency. J Exp Med (2005) 202:479–84. doi: 10.1084/jem.20050381
119. Sawai T, Tuchikawa K. Kaposi’s Sarcoma Developed in a Patient With a Thymoma in the Setting of Excess Numbers of CD8-Positive Cells in the Peripheral Blood. Arch Pathol Lab Med (1990) 114:611–3.
120. Lynn J, Knight AK, Kamoun M. Levinson AI. A 55-Year-Old Man With Hypogammaglobulinemia, Lymphopenia, and Unrelenting Cutaneous Warts. J Allergy Clin Immunol (2004) 114:409–14. doi: 10.1016/j.jaci.2004.02.033
121. Lin JH, Wang KY, Kraft S, Roberts RL. Resolution of Warts in Association With Subcutaneous Immunoglobulin in Immune Deficiency. Pediatr Dermatol (2009) 26:155–8. doi: 10.1111/j.1525-1470.2009.00874.x
122. Leiding JW, Holland SM. Warts and All: Human Papillomavirus in Primary Immunodeficiencies. J Allergy Clin Immunol (2012) 130:1030–48. doi: 10.1016/j.jaci.2012.07.049
123. Monastirli A, Matsouka P, Pasmatzi E, Melachrinou M, Georgiou S, Solomou E, et al. Complete Remission of Recalcitrant Viral Warts Under Oral Isotretinoin in a Patient With Low-Grade B-Cell Lymphoma. Acta Derm Venereol (2005) 85:358–60. doi: 10.1080/00015550510027090
124. Olding-Stenkvist E, Nordbring F, Larsson E, Lindblom B, Wigzell H. Fatal Progressive Vaccinia in Two Immunodeficient Infants. Scand J Infect Dis Suppl (1980) Suppl 24:63–7.
125. Gutiérrez L, Albarrán F, Moruno H, Cuende E. Parvovirus B19 Chronic Monoarthritis in a Patient With Common Variable Immunodeficiency. Reumatol Clínica (2014) 11:58-9. doi: 10.1016/j.reuma.2014.07.001
126. Sharma VR, Fleming DR, Slone SP. Pure Red Cell Aplasia Due to Parvovirus B19 in a Patient Treated With Rituximab. Blood (2000) 96:1184–6. doi: 10.1182/blood.v96.3.1184.015k54_1184_1186
127. Isobe Y, Sugimoto K, Shiraki Y, Nishitani M, Koike K, Oshimi K. Successful High-Titer Immunoglobulin Therapy for Persistent Parvovirus B19 Infection in a Lymphoma Patient Treated With Rituximab-Combined Chemotherapy. Am J Hematol (2004) 77:370–3. doi: 10.1002/ajh.20200
128. Kim SY, Lee HJ, Park E, Ahn YH, Ha I-S, Cheong H, et al. Severe Anemia Due to Parvovirus Infection Following Treatment With Rituximab in a Pediatric Kidney Transplant Recipient : Anemia After Treatment of Rituximab in Kidney Recipient Patient. Child Kidney Dis (2015) 19:176–9. doi: 10.3339/chikd.2015.19.2.176
129. Song KW, Mollee P, Patterson B, Brien W, Crump M. Pure Red Cell Aplasia Due to Parvovirus Following Treatment With CHOP and Rituximab for B-Cell Lymphoma*. Br J Haematol (2002) 119:125–7. doi: 10.1046/j.1365-2141.2002.03778.x
130. Hartmann JT, Meisinger I, Krober SM, Weisel K, Klingel K, Kanz L. Progressive Bicytopenia Due to Persistent Parvovirus B19 Infection After Immunochemotherapy With Fludarabine/Cyclophosphamide and Rituximab for Relapsed B Cell Lymphoma. Haematologica (2006) 91:ECR49–9. doi: 10.3324/%x
131. Klepfish A, Rachmilevitch E, Schattner A. Parvovirus B19 Reactivation Presenting as Neutropenia After Rituximab Treatment. Eur J Intern Med (2006) 17:505–7. doi: 10.1016/j.ejim.2006.05.002
132. Jin H, Reed JC, Liu STH, Ho H-E, Lopes JP, Ramsey NB, et al. Three Patients With X-Linked Agammaglobulinemia Hospitalized for COVID-19 Improved With Convalescent Plasma. J Allergy Clin Immunol Pract (2020) 8:3594–3596.e3. doi: 10.1016/j.jaip.2020.08.059
133. Buckland MS, Galloway JB, Fhogartaigh CN, Meredith L, Provine NM, Bloor S, et al. Treatment of COVID-19 With Remdesivir in the Absence of Humoral Immunity: A Case Report. Nat Commun (2020) 11:6385. doi: 10.1038/s41467-020-19761-2
134. Mira E, Yarce OA, Ortega C, Fernández S, Pascual NM, Gómez C, et al. Rapid Recovery of a SARS-CoV-2-Infected X-Linked Agammaglobulinemia Patient After Infusion of COVID-19 Convalescent Plasma. J Allergy Clin Immunol Pract (2020) 8:2793–5. doi: 10.1016/j.jaip.2020.06.046
135. Iaboni A, Wong N, Betschel SD. A Patient With X-Linked Agammaglobulinemia and COVID-19 Infection Treated With Remdesivir and Convalescent Plasma. J Clin Immunol (2021) 41:923–5. doi: 10.1007/s10875-021-00983-y
136. Guetl K, Moazedi-Fuerst F, Rosskopf K, Brodmann M, Krause R, Eller P, et al. SARS-CoV-2 Positive Virus Culture 7 Weeks After Onset of COVID-19 in an Immunocompromised Patient Suffering From X Chromosome-Linked Agammaglobulinemia. J Infect (2021) 82:414–51. doi: 10.1016/j.jinf.2020.10.025
137. Abraham RS, Marshall JM, Kuehn HS, Rueda CM, Gibbs A, Guider W, et al. Severe SARS-CoV-2 Disease in the Context of a NF-κb2 Loss-of-Function Pathogenic Variant. J Allergy Clin Immunol (2021) 147:532–44.e1. doi: 10.1016/j.jaci.2020.09.020
138. Meyts I, Bucciol G, Quinti I, Neven B, Fischer A, Seoane E, et al. Coronavirus Disease 2019 in Patients With Inborn Errors of Immunity: An International Study. J Allergy Clin Immunol (2021) 47(2):520–31. doi: 10.1016/j.jaci.2020.09.010
139. London J, Boutboul D, Lacombe K, Pirenne F, Heym B, Zeller V, et al. Severe COVID-19 in Patients With B Cell Alymphocytosis and Response to Convalescent Plasma Therapy. J Clin Immunol (2021) 41:356–61. doi: 10.1007/s10875-020-00904-5
140. Choi B, Choudhary MC, Regan J, Sparks JA, Padera RF, Qiu X, et al. Persistence and Evolution of SARS-CoV-2 in an Immunocompromised Host. N Engl J Med (2020) 383:2291–3. doi: 10.1056/NEJMc2031364
141. Martinot M, Jary A, Fafi-Kremer S, Leducq V, Delagreverie H, Garnier M, et al. Remdesivir Failure With SARS-CoV-2 RNA-Dependent RNA-Polymerase Mutation in a B-Cell Immunodeficient Patient With Protracted Covid-19. Clin Infect Dis (2020). doi: 10.1093/cid/ciaa1474
142. Tepasse P-R, Hafezi W, Lutz M, Kühn J, Wilms C, Wiewrodt R, et al. Persisting SARS-CoV-2 Viraemia After Rituximab Therapy: Two Cases With Fatal Outcome and a Review of the Literature. Br J Haematol (2020) 190:185–8. doi: 10.1111/bjh.16896
143. Hueso T, Pouderoux C, Péré H, Beaumont A-L, Raillon L-A, Ader F, et al. Convalescent Plasma Therapy for B-Cell–Depleted Patients With Protracted COVID-19. Blood (2020) 136:2290–5. doi: 10.1182/blood.2020008423
144. Castelli R, Ferraris L, Pantaleo G, Lambertenghi Deliliers G, Cicardi M. High Rate of Hepatitis B Viral Breakthrough in Elderly non-Hodgkin Lymphomas Patients Treated With Rituximab Based Chemotherapy. Dig Liver Dis (2016) 48:1394–7. doi: 10.1016/j.dld.2016.08.113
145. Tsutsumi Y, Yamamoto Y, Shimono J, Ohhigashi H, Teshima T. Hepatitis B Virus Reactivation With Rituximab-Containing Regimen. World J Hepatol (2013) 5:612–20. doi: 10.4254/wjh.v5.i11.612
146. Khan ZH, Ilyas K, Ghazanfar H, Khan HH, Hussain Q, Hammad S, et al. Fatal Fulminant Hepatitis From Rituximab-Induced Hepatitis B Reactivation in a Patient With Follicular Lymphoma: A Case Report and a Brief Review of Literature. Cureus (2018) 10:e2257. doi: 10.7759/cureus.2257
147. Siegal FP, Dikman SH, Arayata RB, Bottone EJ. Fatal Disseminated Adenovirus 11 Pneumonia in an Agammaglobulinemic Patient. Am J Med (1981) 71:1062–7. doi: 10.1016/0002-9343(81)90343-0
148. Arroyo-Martinez YM, Saindon M. Raina JS. X-Linked Agammaglobulinemia Presenting With Multiviral Pneumonia. Cureus (2020) 12:e7884–4. doi: 10.7759/cureus.7884
149. Liese JG, Wintergerst U, Tympner KD, Belohradsky BH. High- vs Low-Dose Immunoglobulin Therapy in the Long-Term Treatment of X-Linked Agammaglobulinemia. Am J Dis Child (1992) 146:335–9. doi: 10.1001/archpedi.1992.02160150075025
150. Frange P, Touzot F, Debré M, Héritier S, Leruez-Ville M, Cros G, et al. Prevalence and Clinical Impact of Norovirus Fecal Shedding in Children With Inherited Immune Deficiencies. J Infect Dis (2012) 206:1269–74. doi: 10.1093/infdis/jis498
151. Faulkner GC, Burrows SR, Khanna R, Moss DJ, Bird AG. Crawford DH. X-Linked Agammaglobulinemia Patients are Not Infected With Epstein-Barr Virus: Implications for the Biology of the Virus. J Virol (1999) 73:1555–64. doi: 10.1128/JVI.73.2.1555-1564.1999
152. Zaman M, Huissoon A, Buckland M, Patel S, Alachkar H, Edgar JD, et al. Clinical and Laboratory Features of Seventy-Eight UK Patients With Good’s Syndrome (Thymoma and Hypogammaglobulinaemia). Clin Exp Immunol (2019) 195:132–8. doi: 10.1111/cei.13216
153. Kelleher P, Misbah SA. What is Good’s Syndrome? Immunological Abnormalities in Patients With Thymoma. J Clin Pathol (2003) 56:12 LP – 16. doi: 10.1136/jcp.56.1.12
154. Hayward AR, Paolucci P, Webster AD, Kohler P. Pre-B Cell Suppression by Thymoma Patient Lymphocytes. Clin Exp Immunol (1982) 48:437–42.
155. Barwick Eidex R. History of Thymoma and Yellow Fever Vaccination. Lancet (London England) (2004) 364:936. doi: 10.1016/S0140-6736(04)17017-7
156. Montella L, Masci AM, Merkabaoui G, Perna F, Vitiello L, Racioppi L. Palmieri G. B-Cell Lymphopenia and Hypogammaglobulinemia in Thymoma Patients. Ann Hematol (2003) 82:343–7. doi: 10.1007/s00277-003-0635-z
157. Federico P, Imbimbo M, Buonerba C, Damiano V, Marciano R, Serpico D, et al. Is Hypogammaglobulinemia a Constant Feature in Good’s Syndrome? Int J Immunopathol Pharmacol (2010) 23:1275–9. doi: 10.1177/039463201002300434
158. Kaufman AJ, Palatt J, Sivak M, Raimondi P, Lee D-S, Wolf A, et al. Thymectomy for Myasthenia Gravis: Complete Stable Remission and Associated Prognostic Factors in Over 1000 Cases. Semin Thorac Cardiovasc Surg (2016) 28:561–8. doi: 10.1053/j.semtcvs.2016.04.002
159. Ahn S, Cunningham-Rundles C. Role of B Cells in Common Variable Immune Deficiency. Expert Rev Clin Immunol (2009) 5:557–64. doi: 10.1586/eci.09.43
160. Jahn K, Schumann D, Tamm M, Hirsch H, Halter J, Junker L, et al. Respiratory Viral Infection in Immunocompromised Patients. Eur Respir J (2018) 52:PA4694. doi: 10.1183/13993003.congress-2018.PA4694
161. Agarwal S, Smereka P, Harpaz N, Cunningham-Rundles C, Mayer L. Characterization of Immunologic Defects in Patients With Common Variable Immunodeficiency (CVID) With Intestinal Disease. Inflammation Bowel Dis (2011) 17:251–9. doi: 10.1002/ibd.21376
162. Neven B, Pérot P, Bruneau J, Pasquet M, Ramirez M, Diana J-S, et al. Cutaneous and Visceral Chronic Granulomatous Disease Triggered by a Rubella Virus Vaccine Strain in Children With Primary Immunodeficiencies. Clin Infect Dis (2017) 64:83–6. doi: 10.1093/cid/ciw675
163. Cooper N, Arnold DM. The Effect of Rituximab on Humoral and Cell Mediated Immunity and Infection in the Treatment of Autoimmune Diseases. Br J Haematol (2010) 149:3–13. doi: 10.1111/j.1365-2141.2010.08076.x
164. Kado R, Sanders G, McCune WJ. Diagnostic and Therapeutic Considerations in Patients With Hypogammaglobulinemia After Rituximab Therapy. Curr Opin Rheumatol (2017) 29:228–33. doi: 10.1097/BOR.0000000000000377
165. Shortt J, Spencer A. Adjuvant Rituximab Causes Prolonged Hypogammaglobulinaemia Following Autologous Stem Cell Transplant for non-Hodgkin’s Lymphoma. Bone Marrow Transplant (2006) 38:433–6. doi: 10.1038/sj.bmt.1705463
166. Thiel J, Rizzi M, Engesser M, Dufner A-K, Troilo A, Lorenzetti R, et al. B Cell Repopulation Kinetics After Rituximab Treatment in ANCA-Associated Vasculitides Compared to Rheumatoid Arthritis, and Connective Tissue Diseases: A Longitudinal Observational Study on 120 Patients. Arthritis Res Ther (2017) 19:101. doi: 10.1186/s13075-017-1306-0
167. Roberts DM, Jones RB, Smith RM, Alberici F, Kumaratne DS, Burns S, et al. Rituximab-Associated Hypogammaglobulinemia: Incidence, Predictors and Outcomes in Patients With Multi-System Autoimmune Disease. J Autoimmun (2015) 57:60–5. doi: 10.1016/j.jaut.2014.11.009
168. Gkrania-Klotsas E, Kumararatne DS. Serious Infectious Complications After Rituximab Therapy in Patients With Autoimmunity: Is This the Final Word? Clin Infect Dis (2021) 72:738–42. doi: 10.1093/cid/ciaa131
169. Buch MH, Smolen JS, Betteridge N, Breedveld FC, Burmester G, Dörner T, et al. Updated Consensus Statement on the Use of Rituximab in Patients With Rheumatoid Arthritis. Ann Rheum Dis (2011) 70:909 LP – 920. doi: 10.1136/ard.2010.144998
170. Mikulska M, Lanini S, Gudiol C, Drgona L, Ippolito G, Fernández-Ruiz M, et al. ESCMID Study Group for Infections in Compromised Hosts (ESGICH) Consensus Document on the Safety of Targeted and Biological Therapies: An Infectious Diseases Perspective (Agents Targeting Lymphoid Cells Surface Antigens [I]: CD19, CD20 and CD52). Clin Microbiol Infect Off Publ Eur Soc Clin Microbiol Infect Dis (2018) 24(Suppl 2):S71–82. doi: 10.1016/j.cmi.2018.02.003
171. Raso S, Napolitano M, Carlisi M, Mancuso S, Santoro M, Siragusa S. Rituximab for Immune Thrombocytopenia: A Retrospective Analysis on the Role of Prophylaxis Against Pneumocystis Jirovecii and Herpes Zoster Virus. Blood (2018) 132:4992. doi: 10.1182/blood-2018-99-118585
172. Kamar N, Milioto O, Puissant-Lubrano B, Esposito L, Pierre MC, Mohamed AO, et al. Incidence and Predictive Factors for Infectious Disease After Rituximab Therapy in Kidney-Transplant Patients. Am J Transplant (2010) 10:89–98. doi: 10.1111/j.1600-6143.2009.02785.x
173. Kelesidis T, Daikos G, Boumpas D, Tsiodras S. Does Rituximab Increase the Incidence of Infectious Complications? A Narrative Review. Int J Infect Dis (2011) 15:e2–e16. doi: 10.1016/j.ijid.2010.03.025
174. Kimby E. Tolerability and Safety of Rituximab (MabThera). Cancer Treat Rev (2005) 31:456–73. doi: 10.1016/j.ctrv.2005.05.007
175. Criscuolo E, Castelli M, Diotti RA, Amato V, Burioni R, Clementi M, et al. Cell-To-Cell Spread Blocking Activity Is Extremely Limited in the Sera of Herpes Simplex Virus 1 (HSV-1)- and HSV-2-Infected Subjects. J Virol (2019) 93:e00070–19. doi: 10.1128/JVI.00070-19
176. Gong S, Ruprecht RM. Immunoglobulin M: An Ancient Antiviral Weapon – Rediscovered. Front Immunol (2020) 11:1943. doi: 10.3389/fimmu.2020.01943
177. Libster R, Pérez Marc G, Wappner D, Coviello S, Bianchi A, Braem V, et al. Early High-Titer Plasma Therapy to Prevent Severe Covid-19 in Older Adults. N Engl J Med (2021) 384:610–8. doi: 10.1056/NEJMoa2033700
178. Levin MJ, Duchon JM, Swamy GK, Gershon AA. Varicella Zoster Immune Globulin (VARIZIG) Administration Up to 10 Days After Varicella Exposure in Pregnant Women, Immunocompromised Participants, and Infants: Varicella Outcomes and Safety Results From a Large, Open-Label, Expanded-Access Program. PloS One (2019) 14:e0217749. doi: 10.1371/journal.pone.0217749
179. Salazar G, Zhang N, Fu T-M, An Z. Antibody Therapies for the Prevention and Treatment of Viral Infections. NPJ Vaccines (2017) 2:19. doi: 10.1038/s41541-017-0019-3
180. Davey RT Jr, Fernández-Cruz E, Markowitz N, Pett S, Babiker AG, Wentworth D, et al. Anti-Influenza Hyperimmune Intravenous Immunoglobulin for Adults With Influenza A or B Infection (FLU-IVIG): A Double-Blind, Randomised, Placebo-Controlled Trial. Lancet Respir Med (2019) 7:951–63. doi: 10.1016/S2213-2600(19)30253-X
181. Janiaud P, Axfors C, Schmitt AM, Gloy V, Ebrahimi F, Hepprich M, et al. Association of Convalescent Plasma Treatment With Clinical Outcomes in Patients With COVID-19: A Systematic Review and Meta-Analysis. JAMA (2021) 325:1185–95. doi: 10.1001/jama.2021.2747
182. Salisbury D, Ramsay M, Noakes K.Immunisation Against Infectious Disease, Chapter 6. In: Contraindications and Special Considerations (Green Book). London: Department of Health (2017).
183. Rubin LG, Levin MJ, Ljungman P, Davies EG, Avery R, Tomblyn M, et al. IDSA Clinical Practice Guideline for Vaccination of the Immunocompromised Host. Clin Infect Dis (2014) 58:e44–100. doi: 10.1093/cid/cit684
Keywords: B cells, alymphocytosis, viral infections, immunodeficiency, immune deficiency, humoral immunity, immune responses
Citation: Grammatikos A, Donati M, Johnston SL and Gompels MM (2021) Peripheral B Cell Deficiency and Predisposition to Viral Infections: The Paradigm of Immune Deficiencies. Front. Immunol. 12:731643. doi: 10.3389/fimmu.2021.731643
Received: 27 June 2021; Accepted: 09 August 2021;
Published: 30 August 2021.
Edited by:
Linda F. Van Dyk, University of Colorado Denver, United StatesReviewed by:
Koen Bartholomeeusen, Institute of Tropical Medicine Antwerp, BelgiumMaria George Ioannou, University Hospital of Larissa, Greece
Copyright © 2021 Grammatikos, Donati, Johnston and Gompels. This is an open-access article distributed under the terms of the Creative Commons Attribution License (CC BY). The use, distribution or reproduction in other forums is permitted, provided the original author(s) and the copyright owner(s) are credited and that the original publication in this journal is cited, in accordance with accepted academic practice. No use, distribution or reproduction is permitted which does not comply with these terms.
*Correspondence: Alexandros Grammatikos, YWxleGFuZHJvcy5ncmFtbWF0aWtvc0BuYnQubmhzLnVr