- 1Clinical Research Center, Chongqing Public Health Medical Center, Chongqing, China
- 2Infectious Diseases and Immunity in Global Health Program, Research Institute, McGill University Health Centre, Montréal, QC, Canada
- 3Chronic Viral Illness Service, McGill University Health Centre, Montréal, QC, Canada
- 4Canadian HIV Trials Network (CTN), Canadian Institutes of Health Research (CIHR), Vancouver, BC, Canada
- 5Division of Hematology, McGill University Health Centre, Montréal, QC, Canada
The intestinal microbiome is an essential so-called human “organ”, vital for the induction of innate immunity, for metabolizing nutrients, and for maintenance of the structural integrity of the intestinal barrier. HIV infection adversely influences the richness and diversity of the intestinal microbiome, resulting in structural and functional impairment of the intestinal barrier and an increased intestinal permeability. Pathogens and metabolites may thus cross the “leaky” intestinal barrier and enter the systemic circulation, which is a significant factor accounting for the persistent underlying chronic inflammatory state present in people living with HIV (PLWH). Additionally, alcohol use and abuse has been found to be prevalent in PLWH and has been strongly associated with the incidence and progression of HIV/AIDS. Recently, converging evidence has indicated that the mechanism underlying this phenomenon is related to intestinal microbiome and barrier function through numerous pathways. Alcohol acts as a “partner” with HIV in disrupting microbiome ecology, and thus impairing of the intestinal barrier. Optimizing the microbiome and restoring the integrity of the intestinal barrier is likely to be an effective adjunctive therapeutic strategy for PLWH. We herein critically review the interplay among HIV, alcohol, and the gut barrier, thus setting the scene with regards to development of effective strategies to counteract the dysregulated gut microbiome and the reduction of microbial translocation and inflammation in PLWH.
Introduction
Although the widespread use of antiretroviral therapy (ART) has resulted in an increase in the lifespan of people living with HIV (PLWH), HIV/AIDS currently remains a major global public health problem (1). According to the Joint United Nations Programme on HIV/AIDS (UNAIDS) report, 690 000 people succumbed to AIDS-related diseases in 2019 (2). On the other hand, it is estimated that alcohol abuse causes 2.5 million deaths worldwide every year, and the World Health Organization lists alcohol consumption as an important risk factor for disease and disability worldwide (3).
Notably, alcohol use and abuse has been found to be highly prevalent in PLWH (4) and alcohol is strongly associated with the incidence and progression of HIV, including towards AIDS. Indeed, alcohol increases the risk of multiple comorbidities, such as hepatic fibrosis, hepatic cirrhosis, neurocognitive impairment, and AIDS-related dementia (HAD) (5–11). Moreover, alcohol abuse increases the risk of HIV infection by promoting risky behaviors (12). Alcohol abuse has also been associated with failure of ART to achieve virologic inhibition in PLWH (13–15).
Recent evidence highlights the fact that HIV and alcohol act as two “partners” in the disruption of the gut microbiota composition and impairment of the intestinal barrier, which leads to high-levels of microbial translocation and chronic immune activation in PLWH (16–18). The entire gastro-intestinal system hosts a large concentration and diversity of microbes. A population of nearly 100 trillion different microbes inhabits the human gut, comprising bacteria, archaea, fungi, yeasts, and viruses (19). Intestinal microbiota not only plays a vital role in maintaining the normal intestinal tract of individuals, but is also indispensable for the general health (20–22). Intestinal microbial dysbiosis, a disbalance of the gut microbiota composition, has been shown to be positively associated with several chronic conditions, such as obesity, cardiovascular disease, inflammatory bowel disease, cancer, and alcoholic liver disease (23, 24). HIV infection is known to be associated with microbial dysbiosis, intestinal barrier injury, and intestinal leakage (25). The “leaky gut” is now known to be one of the main factor causing the persistent underlying chronic inflammation in PLWH on ART, and is associated with poor recovery of CD4+ T-cell counts and the development and progression of non-AIDS-related conditions (26, 27). Moreover, alcohol use and abuse are known to enhance HIV-induced injury to the gut (16, 28). The present review will focus on the mechanisms whereby HIV and alcohol increase disruption to the gut microbiota and intestinal barrier, causing microbial translocation, and chronic systemic inflammation in PLWH. We will also discuss possible therapeutic strategies for the restoration of the structural and functional integrity of the intestinal barrier.
Alcohol Use Accelerates the Progression of HIV Infection
Alcohol use is prevalent among PLWH around the world (4, 29–34). Due to the different population involved in alcohol use studies, the percentage of alcohol user varies in these studies. A study from Kampala, Uganda, observed that 33% of HIV-infected individuals self-reported any alcohol use, and 18.6% HIV-infected individuals were classified as alcohol abusers in 2012-2013 (33). Another study included 8567 HIV-infected individuals from the United States during 2013-2015, and showed that 41% of those were low alcohol users and 27% were hazardous alcohol users (34). The longitudinal cohort study by Kelso-Chichetto et al., found that among PLWH who were drinking alcohol, female were significantly less frequently found than male, and the percentage of heavy drinkers decreased in HIV-infected women during 10 years of follow-up; in contrast, the percentage of heavy drinkers in HIV-infected men who have sex with men (MSM) increased during follow-up (31). However, no consensus is currently accepted for the burden of alcohol use and abuse in PLWH (35, 36). Marshall et al. performed a longitudinal analysis among HIV-infected MSM, and found that the percentage of hazardous drinkers decreased from 29.0% to 24.2% during the eight-year follow-up (35). Moreover, a study in Porto Alegre, southern Brazil reported that heavy alcohol consumption among PLWH was even lower than the general population (5.6 vs. 10.3%) (36). The authors of the preceding study propose that the lower prevalence of risky alcohol consumption in PLWH may be secondary to their concern related to the perceived harmful consequences of alcohol use in negatively impacting HIV control (36). Importantly, PLWH experienced increased mortality and physiologic injury at lower levels of alcohol use compared with the general population (37).
The prevalence of alcohol use and abuse in PLWH is likely to induce tissue injury and reduce survival. Non-hazardous alcohol consumption once per week or more was reported to decrease survival in PLWH by 1 year, and by 6.4 years for those with daily hazardous consumption (38). In addition, alcohol consumption independently increases the risk for several comorbidities in PLWH, including the risk of dementia (8), cardiovascular disease (39, 40), hepatic cirrhosis (41), and pneumonia (42). A study by Freiberg et al., showed that compared with infrequent and moderate drinking, hazardous drinking and alcohol abuse were associated with a higher prevalence of cardiovascular diseases (39). Moreover, liver injury is known to be a major cause of morbidity and mortality among PLWH (43, 44). Alcohol is a potent trigger of HIV-mediated liver damage, which accelerates hepatic disease progression and eventually results in advanced fibrosis and cirrhosis (7, 45). A probable mechanism for liver inflammation and fibrosis was proposed by Chen et al. (46): alcohol increases intestinal permeability and gut-derived pathogens cross the intestinal barrier to enter into the liver, then hepatic stellate cells, Kupffer cells, and hepatocytes are activated to secrete pro-inflammatory cytokines and chemokines, causing persistent inflammation and injury to the liver (46). Alcohol may also promote HIV-mediated liver injury through increased oxidative stress and mitochondrial disorders, leading to increased virus replication and hepatocyte apoptosis (41, 44, 47–51). Reports have shown that alcohol use can activate microglial cells and astrocytes, promoting neuronal cell death by enhancing oxidative stress and gut microbiome changes, eventually leading to impaired cognition and behavioral deficits, and possibly death (8, 52–55).
Alcohol modulates immune cells and increases systemic inflammation, which has been considered to be one of the main mechanisms for adverse outcomes induced by alcohol use and abuse. In simian immunodeficiency virus (SIV)-infected rhesus macaques, alcohol use has been shown to accelerate the decline of peripheral CD4+ T-cells (56). However, the results of related observational studies in PLWH in Uganda have been conflicting, indicating conversely, that unhealthy alcohol use may not accelerate CD4+ T-cell decline in PLWH (57). Alcohol use is also reported to alter CD8+ T-cell phenotypes in PLWH, and alcohol is positively associated with activated-senescent and terminal effector memory CD45RA+CD8+ T-cells, but not CD4+ T-cells (17). In addition, alcohol use additively or synergistically increases systemic inflammatory factors in PLWH. A study of HIV-infected individuals in Russia showed that alcohol use and abuse independently increased levels of the following biomarkers: soluble CD14 (sCD14), interleukin (IL)-6, and D-dimer (58). Monnig et al. reported that HIV-infected individuals had higher levels of lipopolysaccharide (LPS), LPS-binding protein (LBP), sCD14, and soluble CD163 (sCD163) than uninfected individuals with similar alcohol use (59). Of note, these biomarkers have been associated with increased mortality risk in PLWH (60–62).
Moreover, alcohol use and abuse in PLWH has become an important factor in reducing adherence to ART, leading to poor ART efficacy (63–66), and increasing the possibility of antiretroviral drug resistance (67, 68). An epidemiological study of HIV-infected women on ART by Howard et al., illustrated the relationship between antiretroviral adherence and viral load. Virological failure occurred in 17% of women with adherence rates of greater than or equal to 88%, in 28% of those with 45-87% adherence, in 43% of those with 13-44% adherence, and in 71% of those with less than or equal to 12% adherence (69). Alcohol use was a significant predictor of lower adherence (70, 71), and in an investigation by Braithwaite and colleagues, they observed that regardless of HIV status and temporal and dose-response relationships between alcohol consumption and missed HIV medications, consumption of alcohol was associated with decreased adherence to medications on that day and on the following two days. In particular, among non-binge drinkers (i.e., drinkers who consumed less than five standard drinks per day), 3.5% missed medication doses on drinking days, 3.1% missed medication on post-drinking days, and 2.1% missed medication on non-drinking days (p<0.001 for trend). Among binge drinkers (i.e., drinkers who consumed five or more drinks per day), 11.0% missed doses on drinking days, 7.0% missed medication on post-drinking days, and 4.1% missed medication on non-drinking days (p<0.001 for trend) (72).
Furthermore, alcohol may aggravate the toxicity of ART drugs, which is likely to decrease ART adherence (65). Hepatoxicity is one of most common side effects for ART drugs. In the liver, the main metabolic pathway for the metabolism of alcohol as well as antiretroviral drugs (such as zidovudine, stavudine, and nevirapine) is the cytochrome P450 pathway; thus alcohol use may aggravate the adverse effects of antiretroviral drugs via competitive inhibition of the cytochrome P450 pathway (7, 73). In addition, alcohol may increase the adverse effects of ART drugs on testicular function (74). Moreover, beliefs that mixing alcohol and ART drugs is toxic, and that drug treatments should be interrupted when drinking are common among PLWH, thus also leading to treatment nonadherence (4).
Aside from poor adherence to ART caused by alcohol, increased viral replication induced by alcohol is a further potential reason for ART failure. In HIV-infected peripheral blood lymphocytes (PBLs) pretreated with alcohol, HIV-1 DNA increased 10-fold, and it has been observed that alcohol enhanced the expression of the chemokine receptor 4 (CXCR4) HIV-entry co-receptor (75). Two studies of chronic alcohol consumption in rhesus macaques observed similar results, with the plasma viral load in the alcohol group being much higher than that in the control group (76, 77).
HIV Infection Is Associated With Gut Microbiome Dysbiosis and Related Inflammation
The gut contains a large proportion of lymphoid tissue and lymphocytes of the human body (78, 79), and is one of the earliest targets of, and a reservoir for, HIV infection (80). HIV directly attacks the gut mucosal epithelium, leading to intercellular tight junction disruption and death of enterocytes, increasing gut permeability (26, 81, 82). Primary gut mucosal CD4+ T-cells have higher chemokine receptor 5 (CCR5) co-receptor expression than peripheral blood CD4+ T-cells (83–85). Thus, gut CD4+ T-cells are priority targets for HIV, support higher levels of viral replication (85, 86), and are massively depleted during early HIV infection (87). One study reported that CD4+ T-cell depletion occurs during all stages of HIV disease, and this occurs predominantly in the gastrointestinal tract (GI) (87). Overall, HIV infection leads to intestinal epithelial damage and a reduction in numbers of immune cells.
Recently, a number of studies have indicated that HIV infection is associated with intestinal microbial dysbiosis (Figure 1). HIV infection significantly affects gut microbial richness and diversity (26, 81, 88–90), especially in immune discordant patients (91). Lozupone et al. reported that HIV infection may reduce abundance of the symbiotic bacterium Bacteroides fragilis by depleting gut CD4+ T-cells (92). Vujkovic-Cvijin et al. reported that compared with HIV-negative individuals, the gut microbial communities of HIV+ viremic untreated individuals is mainly altered by an increased abundance of Proteobacteria and Bacteroidaceae; however, effective ART fails to completely reverse these changes (93). In addition, several studies have shown that enrichment in the bacterial genus Prevotella and a depletion of Bacteroides occurs in untreated PLWH (92, 94, 95). Compared with HIV+ viremic progressors, the genera Succinivibrio, Sutterella, Rhizobium, Delftia, Anaerofilum, and Oscillospira were more abundant in elite controllers, whereas the genera Blautia and Anaerostipes were depleted (96). Rocafort et al., reported that HIV infection reduces the abundance of Akkermansia, Anaerovibrio, Bifidobacterium, and Clostridium (97). Besides microbial compositions, HIV infection also causes changes to microbial and cellular metabolites. HIV infection and microbial translocation have been linked to increased catabolism of tryptophan into kynurenine. Indoleamine 2,3-dioxygenase 1 (IDO-1) is the main inducible and rate-limiting enzyme for the catabolism of tryptophan through the kynurenine pathway (93, 98), and is up-regulated by interferons (IFNs) and by agonists of Toll-like receptors (TLRs) (99). In PLWH, IDO-1 activity is increased, and this is thought to be related to plasma levels of LPS and (1, 3)-β-D-Glucan (BDG), Treg cell frequency, microbial translocation, immune activation, and HIV reservoir size (93, 100–103). Moreover, the study by Vujkovic-Cvijin et al. showed that HIV infection-related intestinal microbiota participate in tryptophan metabolism, compared with the intestinal microbiota of HIV-negative individuals. HIV-positive viremic, untreated individuals had an enrichment of more genetic homologs to tryptophan catabolism enzymes of the kynurenine pathway in their intestinal microbiota, and that, perhaps, contributes to immunoactive tryptophan catabolism during HIV disease (93). Trimethylamine-N-oxide (TMAO) is an intestinal microbiota-dependent metabolite of phosphatidylcholine (104), and a strong relationship between TMAO and increased risk for atherosclerosis and cardiovascular disease has been reported (105, 106). Shan et al. reported that plasma TMAO levels positively correlate with serum biomarkers of monocyte activation and inflammation, and is associated with progression of carotid atherosclerosis in PLWH (107). Butyrate, one of the most abundant short-chain fatty acids (SCFA) in the intestinal tract, provides the primary energy source for epithelial colonic cells, promotes epithelial barrier integrity, prevents microbial translocation, and further reduces inflammation (108–110). Compared with HIV-negative individuals, a number of the bacterial genera associated with producing butyrate (e.g., Roseburia, Coprococcus, Faecalibacterium prausnitzii, and Eubacterium rectale) are less frequent in HIV-positive individuals (110–112). Moreover, a low abundance of butyrate-producing bacteria in the colon is reported to be associated with microbial translocation and immune activation in PLWH (110). Furthermore, evidence has shown that gut damage and dysbiosis induce higher levels of microbial translocation. One study by Raffatellu et al., observed that after eight hours, SIV-infected macaques had significantly higher levels of Salmonella typhimurium in the mesenteric lymph nodes than SIV-negative macaques, subsequent to injection of S. typhimurium into the gut lumen (113). Estes et al. using quantitative image analysis, revealed that damaged intestinal epithelium was associated with microbial translocation in SIV-infected macaques (81).
Gut microbial translocation resulting from dysbiosis and gut damage plays a prominent role in maintaining a persistent underlying chronic inflammatory state in PLWH, and compliant, long-term ART does not entirely reverse damage to the intestinal tract barrier (81, 90, 114–117). Thus the gut fails to successfully repair in PLWH receiving ART (90, 114, 115). Measurement of specific plasma biomarkers is a convenient way to assess the level of gut damage and microbial translocation, as endoscopy remains difficult (118–121). LPS is a component of the cell wall of Gram-negative bacteria, and is well-known to stimulate innate and adaptive immunity in vivo (90), Marchetti et al., analyzed 1488 biomarker measures from 379 HIV-infected individuals, and observed that LPS was an effective biomarker associated with accelerated disease progression independently of age, HIV RNA loads, and CD4+ T-cell counts (122). Moreover, compared with immunological responders, higher LPS levels were detected in immunological non-responders (INRs), and the higher LPS levels in INRs were associated with impairment of CD4+ T-cell reconstitution by sustaining T-cell hyperactivation (123). BDG is a component of the cell wall of fungi, and identification of plasma BDG is currently used for the clinical diagnosis of invasive fungal infections (124). Morris et al. reported that high serum levels of BDG are associated with a decrease of CD4+ T-cell counts, a higher viral load, and activation of CD8+ T-cells in PLWH (125, 126). Intestinal fatty acid binding protein (I-FABP), expressed in enterocytes, is released upon cell death, and enters into the systemic circulation (127). HIV infection increases plasma levels of I-FABP in PLWH (128, 129), but sustained effective ART has not been shown to completely reverse these levels in plasma (130). Regenerating islet-derived protein 3-α (REG3α) is an antimicrobial peptide secreted by Paneth cells into the gut lumen, and translocates into the blood when the integrity of the intestinal epithelium is compromised (131). REG3α levels are higher in PLWH, and are associated with lower CD4+ T-cell counts and CD4/CD8 ratios, which positively correlate with HIV disease progression (131). Thus, increased microbial translocation in HIV-infected individuals is likely to contribute to persisting inflammation and disease progression in PLWH.
Alcohol Use Causes Disruption of the Intestinal Barrier
The function of the intestinal barrier is to regulate the absorption of water and key nutrients from the gut lumen into the bloodstream, and to prevent pro-inflammatory microbial products from entering into the portal and systemic circulation (132). Intestinal barrier disruption, also referred to as “intestinal leakiness”, results in increasing intestinal permeability, thus permitting the passage of pathogens and microbial products into the bloodstream (133–135). As shown in Figure 1, many studies have indicated that alcohol use disrupts the intestinal barrier and increases intestinal permeability (136–138). Leclercq et al., measured intestinal permeability using an oral stable, non-degradable radioactive chromium-51 probe in the body, called 51Cr-EDTA, and by examining the resulting radioactivity in urine. Their results showed that compared with non-alcohol-user subjects, intestinal permeability was largely increased in alcohol-dependent subjects (139). Tang et al. observed comparable results, showing that chronic alcohol consumption increased intestinal permeability in mice (138).
Several mechanisms have been reported to be associated with the alcohol-induced intestinal disruption. Alcohol and its metabolites damage enterocytes and villi tips directly, and weaken cell membranes by the generation of reactive oxygen species (ROS) released during alcohol metabolism, thus allowing material such as LPS, alcohol, and microbial products to pass directly through the epithelial cells (133, 140, 141). Also, alcohol disrupts intestinal epithelial cellular integrity by inducing enterocytic apoptosis (142) and an intestinal stem cell decrease in frequency (143). Additionally, alcohol reduces expression of intestinal tight junction and adherent junction proteins in enterocytes, which causes disruption of intercellular junctions (142, 144, 145). Ren et al. reported that the down-regulated expression of tight junction proteins in alcohol treated Caco-2 cells activated the tumor necrosis factor alpha (TNF-α) and nuclear factor kappa-B (NF-κB) signaling pathways (146). Moreover, alcohol can cause overexpression of microRNA (miRNA), such as miR-155, miR-122, and miR-212 in the intestine, which may also affect the gut barrier by regulating genes associated with intestinal mucosal cell integrity (147–149).
Studies have also observed that alcohol directly modulates intestinal innate and adaptive immune responses, resulting in modulation on clearance of pathogens and gut-derived inflammation. Alcohol inhibits the intestine’s immune response for clearing S. typhimurium in the gut (150). An early study by Lopez et al. showed the effect of chronic alcohol exposure on intestinal Peyer’s patches (PPs), sites where naive immune cells differentiate into a variety of mature immune cell subsets (151). Compared with a non-exposed mouse model, a significant decrease in the total number of cells was observed in the PPs of mice exposed to alcohol for 5 weeks, and a highly significant decrease was observed in mice exposed to alcohol for 19 weeks (151). Similarly, a study in mice showed that chronic alcohol consumption decreased the proportion of T-cells, induced alterations in dendritic cells and macrophages in the intestine, and decreased the levels of IgA in small intestinal fecal contents (152). Furthermore, alcohol also induces the downregulation of the host antimicrobial peptides regenerating islet-derived protein 3-ß (REG3ß) and regenerating islet-derived protein 3-γ (REG3γ), which resulted in bacterial overgrowth (153). The preceding studies are important in demonstrating that alcohol use adversely affects mucosal immune mechanisms.
Alcohol consumption also causes bacterial overgrowth and dysbiosis in animals and humans (154–157), and alcohol-related microbial products have been reported to contribute to increased intestinal permeability and peripheral immune activation (158). Alcohol-treated mice had both aerobic and anaerobic bacteria more frequently present in the proximal small intestine, compared with control mice fed with an isocaloric liquid diet. Also, frequency of Bacteroidetes and Verrucomicrobia bacteria were up-regulated in alcohol receiving mice. REG3ß and REG3γ genes were down-regulated in alcohol-fed mice (157). In humans, small intestine bacterial overgrowth is closely associated with the severity of alcoholic cirrhosis (159), and is a risk factor for hepatic encephalopathy (160). Chen et al. reported that the abundance of Prevotellaceae, Enterobacteriaceae, Veillonellaceae, and Streptococcaceae was significantly increased in alcoholic cirrhotic patients, compared with control subjects (161). Engen et al. summarized the changes in gut microbial communities in alcoholics and cirrhotic patients, where microbial communities Gammaproteobacteria and Bacilli firmicutes were enriched. In contrast, firmicutes of the class Clostridia were depleted in alcoholics but were not significantly altered in the cirrhotic group (162). Moreover, alcohol consumption has been shown to be associated changes in levels of microbial metabolites, including SCFA, long-chain fatty acids (LCFA), bile acids and tryptophan (163–166). Several investigations have indicated that alcohol decreased SCFA and LCFA levels in a murine model of chronic alcohol consumption, and supplementation of SCFA and LCFA reduces alcohol-induced liver injury (163, 164, 167). Hendrikx et al., reported that alcohol interfered with tryptophan catabolism, and decreased indole-3-acetic acid, resulting in a decreased expression of IL-22 in the intestine and reduced the expression of antimicrobial peptide REG3γ (165). Xie et al. reported that chronic alcohol consumption led to increased expression of genes involved in bile acid biosynthesis and efflux transport in the liver, and observed that taurine-conjugated bile acids were significantly decreased, and unconjugated and glycine-conjugated bile acids increased in the GI of alcohol-treated rats (166). Interestingly, antibiotics have been found to abrogate intestinal bacterial overgrowth and alleviate subsequent liver damage in rodents (168). Several studies have found that probiotics promote growth of beneficial bacteria, alleviate alcoholic liver injury in rats (169), and improve alcohol-associated liver dysfunction in cirrhotic patients (170, 171).
The Combined Effects of HIV and Alcohol on the Intestinal Barrier
Current evidence indicates that HIV and alcohol could have a deleterious synergistic effect in the gut. In a murine model, HIV transgenic rats are more susceptible to alcohol-induced gut leakiness, hepatic steatosis and inflammation than the corresponding wild-type rats (172, 173). Samuelson et al. observed that alcohol-associated intestinal dysbiosis mediated the susceptibility to pneumococcal pneumonia in a humanized mouse HIV model (174). In concordance with the animal model, Webel et al. found that alcohol consumption was associated with a range of markers of gut permeability, microbial translocation, immune activation, and inflammation in ART-treated PLWH (16). Maffei et al. reported that alcohol use is associated with a dysfunctional CD8+ T-Cell phenotype, intestinal leakiness, and dysbiosis among PLWH (17). As far as can currently be ascertained, the potential interactive mechanisms between HIV and alcohol in the GI tract has not yet been well elucidated. However, in view of the known individual effects of HIV and alcohol, we speculate that they (HIV and alcohol) may together exhibit additive or synergistic interactions causing disruption to microbial ecology and impairment of the intestinal barrier through several pathways.
The changes relating to dysbiosis in gut microbiota composition observed in past studies with respect to the effects of alcohol and HIV have not always been found to be consistent, as different studies have involved different populations and these studies were conducted in varying disease contexts. Most studies have shown that both alcohol and HIV can indeed induce the dysbiosis involved with decreased frequency of “beneficial” microorganisms and enrichment of “harmful” pathogens. Specifically, the beneficial bacteria Bifidobacteria, Lactobacillus, and Akkermansia muciniphila were decreased, while Candida albicans was increased in PLWH and in individuals using alcohol (97, 123, 175–178).
Moreover, dysregulation of the gut microbiota metabolism induced by alcohol and HIV may also play a vital role in the disruption of microbial ecology and impairment of the intestinal barrier. In PLWH, there is a lower abundance of butyrate-producing bacteria and butyric acid levels in feces (179). It was also been observed that butyric acid was significantly reduced in colonic and rectal contents in a rat model of chronic alcohol consumption (163). Moreover, in PLWH, the dysbiosis was associated with increased catabolism of tryptophan into kynurenine and resulting in intestinal barrier destruction (93, 180). It has also been reported that alcohol perturbed tryptophan catabolism, decreased indole-3-acetic acid, resulting in a decreased expression of IL-22 in the intestine and a reduction of the expression of the antimicrobial peptide REG3γ (165).
Other factors, including apoptosis and oxidative stress of intestinal epithelial cells, and intestinal tight junction and adherent junction protein dysfunction may contribute to their synergistic effects. Indeed, both alcohol and HIV could promote apoptosis of intestinal epithelial cells (142, 181), increase oxidative stress of cells (133, 182) and decrease the expression of intestinal tight junction and adherent junction proteins (26, 142, 143). Overall, these factors are likely to work together to promote gut permeability, enhance microbial translocation, and increase gut and systemic inflammatory responses, further contributing to an increased risk of non-AIDS comorbidities in PLWH. However, the questions as to precisely in what manner alcohol and HIV interact with each other on the disruption of the gut homeostasis, and precisely which factors play the most critical roles in negatively impacting on the intestinal barrier when alcohol and HIV are simultaneously present in the gut, remain to be answered. Future further investigations are warranted to discover coherent answers to these important questions.
Therapeutic Strategies to Improve the Intestinal Barrier
In view of the significant structural and functional changes caused by HIV infection combined with alcohol use to the intestinal epithelial barrier, attempts at optimization of the microbiota and restoration of intestinal barrier function may be an effective adjunctive treatment option in PLWH. Numerous microbiota-based therapies have been studied in the condition of HIV infection and alcohol consumption (Table 1). Aside from these strategies, multiple clinical trials using various interventions are ongoing in individuals with conditions (Table 2).
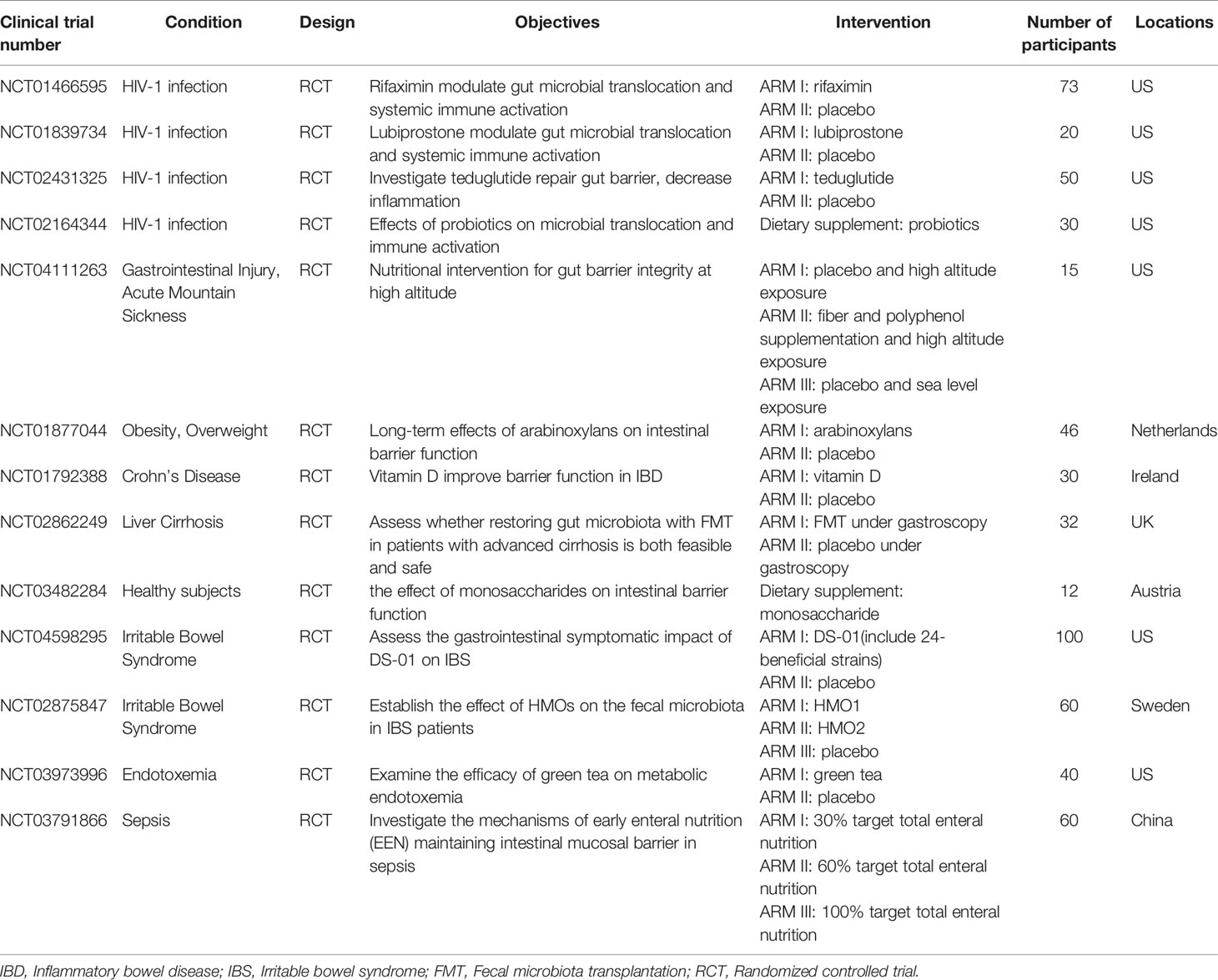
Table 2 Ongoing clinical trials on reduction of microbial translocation and restoration of the intestinal barrier.
Probiotics and prebiotics have been considered to be an effective adjunctive treatment strategy in PLWH. A study by d’Ettorre et al., showed that when oral probiotics (with an abundance of Streptococcus salivarius and Bifidobacteria) were administered together with ART to PLWH, subjects showed a decrease in CD4+ T-cell activation, and lower levels of sCD14, lipopolysaccharide binding protein (LBP), and C-reactive protein (CRP) compared with the control group (194). Serrano-Villar et al. reported that use of oral prebiotics (including short-chain galacto-oligosaccharides, long-chain fructo-oligosaccharides, and glutamine) in PLWH may reduce levels of IL-6, CRP, D-dimer, and T-cell activation, and increase thymic output. In addition, it has been observed in PLWH that prebiotics increased the abundance of Faecalibacterium and Lachnospira, which strongly correlated with a significant increase in butyrate production and a decrease of inflammatory biomarkers sCD14 and CRP (195).
Akkermansia muciniphila, an anaerobic symbiotic bacterium, was reported to increase the thickness of mucus, improve enterocyte monolayer integrity, and counteract inflammation (196–199). It has been reported that the abundance of A. muciniphila is significantly decreased in inflammatory bowel disease (IBD), and supplementation of A. muciniphila in the gut improved colitis by decreasing colon-infiltrating macrophages and cytotoxic T-lymphocytes (CTLs) (200). Moreover, HIV infection and alcohol consumption induced A. muciniphila depletion in the intestine (97, 201). Thus, increasing the abundance of A. muciniphila in the intestine seems to be an effective therapeutic strategy to restore the integrity of the intestinal barrier. An alternative strategy to increase the abundance of A. muciniphila in the gut is by consumption of concord grapes, cranberries, and the camu camu fruit (202). The commonly-used therapeutic drugs metformin and vancomycin have also been reported to increase the abundance of A. muciniphila in the intestinal tract (202, 203).
Fecal microbiota transplantation (FMT) refers to transplantation of fecal microbes from a healthy donor to the GI of a recipient, and is currently being utilized to enhance gut microbial diversity. A large body of evidence has shown that FMT is a highly effective treatment modality against Clostridium difficile infection, and the levels of pro-inflammatory cytokines (TNF-α, IL-1β, IL-6, IL-8 and IL-12) significantly decreased after FMT (204–207). In addition, FMT was reported to restore graft-vs.-host disease (GVHD)-induced intestinal dysbiosis, as reported by Spindelboeck et al., in three severe acute GVHD patients. The restoration of a significantly more diverse microbiome was observed after one to six FMTs delivered via colonoscopy (206). In PLWH and animal models, FMT may be a viable method to restore the intestinal barrier. One study by Hensley-McBain et al., demonstrated that increased peripheral CD4+ T helper (Th)-17 and -22 frequencies and decreased gut CD4+ T-cell activation occurs after transplantation of healthy (SIV-negative) rhesus macaque fecal matter to SIV-infected rhesus macaques (185). A pilot study by Vujkovic-Cvijin et al., showed one-time FMT was well-tolerated in ART-treated PLWH and could lead to partial microbial engraftment including an increase of Faecalibacterium (208), which has exhibited anti-inflammatory effects in cellular and animal models (209). In addition, Serrano-Villar et al. reported that repeated oral FMT capsules caused long-lasting effects in the recipients’ microbiome, specifically in several members of the Lachnospiraceae family. A significant amelioration of the gut damage biomarker I-FABP was also observed in the FMT group (188).
Other strategies to restore intestinal function exist. For example, there may be a role for IL-22-secreting T-cell populations in limiting microbial translocation and systemic inflammation (25). Supplementation of IL-22 may be an effective treatment, and local IL-22 gene delivery improves intestinal inflammation by enhanced signal transducer and activator of transcription 3 (STAT3) activation within colonic epithelial cells in the murine model of ulcerative colitis (210). Studies by Hendrikx et al. observed that feeding mice engineered bacteria that produce IL-22 increased the expression of small intestinal Reg3γ and reduced microbial translocation (165). Furthermore, vitamin A and vitamin D are also known to play a role in maintaining intestinal function. Vitamin A and vitamin D regulate the tight junction protein expression of intestinal tight junction protein 1 (ZO-1), Occludin, and Claudin. In addition, the maturation of group 3 innate lymphoid cells (ILC3) that produce IL-22 and Treg cells that produce IL-10 also requires vitamin A and vitamin D. Interestingly, alcohol consumption was reported to reduce vitamin A and vitamin D circulating levels (211, 212). Supplementation of vitamin A and/or vitamin D may be a potential therapeutic strategy to restore a structurally and functionally intact intestinal barrier (213). The combination of IL-21 and probiotic therapy increases Th17 cell counts and decreases the marker for microbial translocation in ART-treated, SIV-infected rhesus macaques (214). Recombinant human IL-7 increases both circulating and gut-residing naïve and memory CD4+ T-cells, and decreases plasma levels of sCD14 and D-dimer in HIV-infected individuals (215, 216). Finally, Mallarino-Haeger et al. reported that the usage of dipyridamole, a blood vessel dilator, in ART-treated PLWH can significantly increase extracellular adenosine levels, minorly reduce plasma I-FABP levels, and affect regulation of gut mucosal immunity (217).
Conclusion
In summary, in this review we have endeavored to highlight the associations between HIV infection, alcohol use and abuse and gastrointestinal injury in PLWH. Alcohol use and abuse leads to the failure of ART and reduces the survival time of HIV-infected individuals. The intestine is an immunologically indispensable organ, which is structurally and functionally impaired by alcohol use in PLWH. Among HIV-infected individuals, alcohol use further increases intestinal permeability, negatively affects the richness and diversity of the intestinal microbiota, and promotes microbial translocation, chronic immune activation, and chronic inflammation. The resultant underlying state of chronic inflammation increases the risk of development of further comorbidities and disease progression. Several studies have shown that changes in diet and enhancements of the diversity of intestinal microbiota may help reduce intestinal immune activation and subsequent chronic inflammation. Further investigation is warranted in order to study and elucidate the roles of intestinal bacteria and fungi in host immune defense mechanisms, and to explore new potential therapeutic strategies for the effective enhancement of host intestinal immune function, including in the context of alcohol use in PLWH or other conditions.
Author Contributions
JY and JO wrote the first draft of the manuscript. SI, XZ, and VJ provided critical revision of the manuscript. J-PR and YC conceived and designed the manuscript. All authors read and approved the final manuscript.
Funding
This work was supported by the Joint Medical Research Project (2020GDRC010) of Chongqing Science & Technology Bureau and Chongqing Health Commission, Chinese Federation of Public Health foundation (GWLM202024) and Chongqing Talent Cultivation Program (cstc2021ycjh-bgzxm0275).
Conflict of Interest
The authors declare that the research was conducted in the absence of any commercial or financial relationships that could be construed as a potential conflict of interest.
Publisher’s Note
All claims expressed in this article are solely those of the authors and do not necessarily represent those of their affiliated organizations, or those of the publisher, the editors and the reviewers. Any product that may be evaluated in this article, or claim that may be made by its manufacturer, is not guaranteed or endorsed by the publisher.
References
1. Hooshyar D, Hanson DL, Wolfe M, Selik RM, Buskin SE, McNaghten AD. Trends in Perimortal Conditions and Mortality Rates Among HIV-Infected Patients. Aids (2007) 21:2093–100. doi: 10.1097/QAD.0b013e3282e9a664
2. The Joint United Nations Programme on HIV/AIDS. Joint United Nations Programme on HIV/AIDS. UNAIDS DATA 2020. Geneva, Switzerland: UNAIDS (2020).
3. World Health Organisation. Global Status Report on Alcohol and Health 2014. Geneva, Switzerland: World Health Organisation (2014).
4. Kalichman SC, Amaral CM, White D, Swetsze C, Pope H, Kalichman MO, et al. Prevalence and Clinical Implications of Interactive Toxicity Beliefs Regarding Mixing Alcohol and Antiretroviral Therapies Among People Living With HIV/AIDS. AIDS Patient Care STDs (2009) 23:449–54. doi: 10.1089/apc.2008.0184
5. Petry NM. Alcohol Use in HIV Patients: What We Don't Know may Hurt Us. Int J STD AIDS (1999) 10:561–70. doi: 10.1258/0956462991914654
6. Wu ES, Metzger DS, Lynch KG, Douglas SD. Association Between Alcohol Use and HIV Viral Load. J Acquir Immune Defic Syndr (2011) 56:e129–30. doi: 10.1097/QAI.0b013e31820dc1c8
7. Barve S, Kapoor R, Moghe A, Ramirez JA, Eaton JW, Gobejishvili L, et al. Focus on the Liver: Alcohol Use, Highly Active Antiretroviral Therapy, and Liver Disease in HIV-Infected Patients. Alcohol Res Health J Natl Inst Alcohol Abuse Alcoholism (2010) 33:229–36.
8. Persidsky Y, Ho W, Ramirez SH, Potula R, Abood ME, Unterwald E, et al. HIV-1 Infection and Alcohol Abuse: Neurocognitive Impairment, Mechanisms of Neurodegeneration and Therapeutic Interventions. Brain Behav Immun (2011) 25(Suppl 1):S61–70. doi: 10.1016/j.bbi.2011.03.001
9. Heaton RK, Clifford DB, Franklin DR Jr., Woods SP, Ake C, Vaida F, et al. HIV-Associated Neurocognitive Disorders Persist in the Era of Potent Antiretroviral Therapy: CHARTER Study. Neurology (2010) 75:2087–96. doi: 10.1212/WNL.0b013e318200d727
10. Saylor D, Dickens AM, Sacktor N, Haughey N, Slusher B, Pletnikov M, et al. HIV-Associated Neurocognitive Disorder - Pathogenesis and Prospects for Treatment. Nat Rev Neurology (2016) 12:309. doi: 10.1038/nrneurol.2016.53
11. Conigliaro J, Justice AC, Gordon AJ, Bryant K, Alcohol V, Behavior Change Research G. Role of Alcohol in Determining Human Immunodeficiency Virus (HIV)-Relevant Outcomes: A Conceptual Model to Guide the Implementation of Evidence-Based Interventions Into Practice. Med Care (2006) 44:S1–6. doi: 10.1097/01.mlr.0000223659.36369.cf
12. Shuper PA, Joharchi N, Monti PM, Loutfy M, Rehm J. Acute Alcohol Consumption Directly Increases HIV Transmission Risk: A Randomized Controlled Experiment. J Acquir Immune Defic Syndr (2017) 76:493–500. doi: 10.1097/QAI.0000000000001549
13. Azar MM, Springer SA, Meyer JP, Altice FL. A Systematic Review of the Impact of Alcohol Use Disorders on HIV Treatment Outcomes, Adherence to Antiretroviral Therapy and Health Care Utilization. Drug Alcohol Depend (2010) 112:178–93. doi: 10.1016/j.drugalcdep.2010.06.014
14. Chander G, Lau B, Moore RD. Hazardous Alcohol Use: A Risk Factor for Non-Adherence and Lack of Suppression in HIV Infection. J Acquir Immune Defic Syndr (2006) 43:411–7. doi: 10.1097/01.qai.0000243121.44659.a4
15. Palepu A, Tyndall MW, Li K, Yip B, O'Shaughnessy MV, Schechter MT, et al. Alcohol Use and Incarceration Adversely Affect HIV-1 RNA Suppression Among Injection Drug Users Starting Antiretroviral Therapy. J Urban Health Bull New York Acad Med (2003) 80:667–75. doi: 10.1093/jurban/jtg073
16. Webel AR, Sattar A, Funderburg NT, Kinley B, Longenecker CT, Labbato D, et al. Alcohol and Dietary Factors Associate With Gut Integrity and Inflammation in HIV-Infected Adults. HIV Med (2017) 18:402–11. doi: 10.1111/hiv.12442
17. Maffei VJ, Siggins RW, Luo M, Brashear MM, Mercante DE, Taylor CM, et al. Alcohol Use Is Associated With Intestinal Dysbiosis and Dysfunctional CD8+ T-Cell Phenotypes in Persons With Human Immunodeficiency Virus. J Infect Dis (2021) 223:1029–39. doi: 10.1093/infdis/jiaa461
18. Monnig MA, Cohen R. HIV Infection, HCV Coinfection, and Alcohol Use: Associations With Microbial Translocation and Immune Activation. Alcohol Clin Exp Res (2019) 43:1126–34. doi: 10.1111/acer.14032
19. Backhed F, Ley RE, Sonnenburg JL, Peterson DA, Gordon JI. Host-Bacterial Mutualism in the Human Intestine. Science (2005) 307:1915–20. doi: 10.1126/science.1104816
20. Lynch SV, Pedersen O. The Human Intestinal Microbiome in Health and Disease. N Engl J Med (2016) 375:2369–79. doi: 10.1056/NEJMra1600266
21. Ghaisas S, Maher J, Kanthasamy A. Gut Microbiome in Health and Disease: Linking the Microbiome-Gut-Brain Axis and Environmental Factors in the Pathogenesis of Systemic and Neurodegenerative Diseases. Pharmacol Ther (2016) 158:52–62. doi: 10.1016/j.pharmthera.2015.11.012
22. Jandhyala SM, Talukdar R, Subramanyam C, Vuyyuru H, Sasikala M, Nageshwar Reddy D. Role of the Normal Gut Microbiota. World J Gastroenterol (2015) 21:8787–803. doi: 10.3748/wjg.v21.i29.8787
23. Shreiner AB, Kao JY, Young VB. The Gut Microbiome in Health and in Disease. Curr Opin Gastroenterol (2015) 31:69–75. doi: 10.1097/MOG.0000000000000139
24. Frank DN, St Amand AL, Feldman RA, Boedeker EC, Harpaz N, Pace NR. Molecular-Phylogenetic Characterization of Microbial Community Imbalances in Human Inflammatory Bowel Diseases. Proc Natl Acad Sci USA (2007) 104:13780–5. doi: 10.1073/pnas.0706625104
25. Tincati C, Douek DC, Marchetti G. Gut Barrier Structure, Mucosal Immunity and Intestinal Microbiota in the Pathogenesis and Treatment of HIV Infection. AIDS Res Ther (2016) 13:19. doi: 10.1186/s12981-016-0103-1
26. Sandler NG, Douek DC. Microbial Translocation in HIV Infection: Causes, Consequences and Treatment Opportunities. Nat Rev Microbiol (2012) 10:655–66. doi: 10.1038/nrmicro2848
27. Vujkovic-Cvijin I, Sortino O, Verheij E, Sklar J, Wit FW, Kootstra NA, et al. HIV-Associated Gut Dysbiosis Is Independent of Sexual Practice and Correlates With Noncommunicable Diseases. Nat Commun (2020) 11:2448. doi: 10.1038/s41467-020-16222-8
28. Monnig MA, Kahler CW, Cioe PA, Tucker L, Monti PM, Mayer KH, et al. Alcohol Use Predicts Elevation in Inflammatory Marker Soluble CD14 in Men Living With HIV. AIDS Care (2016) 28:1434–40. doi: 10.1080/09540121.2016.1189497
29. Frazier EL, Esser MB. Alcohol Use Among HIV-Positive Women of Childbearing Age, United States, 2013-2014. AIDS Care (2021) 33:1024–36. doi: 10.1080/09540121.2020.1808161
30. Rudolph JE, Cole SR, Edwards JK, Moore R, O'Cleirigh C, Mathews WC, et al. At-Risk Alcohol Use Among HIV-Positive Patients and the Completion of Patient-Reported Outcomes. AIDS Behavior (2018) 22:1313–22. doi: 10.1007/s10461-017-1824-4
31. Kelso-Chichetto NE, Plankey M, Abraham AG, Ennis N, Chen X, Bolan R, et al. Association Between Alcohol Consumption Trajectories and Clinical Profiles Among Women and Men Living With HIV. Am J Drug Alcohol Abuse (2018) 44:85–94. doi: 10.1080/00952990.2017.1335317
32. Marshall BDL, Tate JP, McGinnis KA, Bryant KJ, Cook RL, Edelman EJ, et al. Long-Term Alcohol Use Patterns and HIV Disease Severity. Aids (2017) 31:1313–21. doi: 10.1097/qad.0000000000001473
33. Wandera B, Tumwesigye NM, Nankabirwa JI, Kambugu AD, Parkes-Ratanshi R, Mafigiri DK, et al. Alcohol Consumption Among HIV-Infected Persons in a Large Urban HIV Clinic in Kampala Uganda: A Constellation of Harmful Behaviors. PloS One (2015) 10:e0126236. doi: 10.1371/journal.pone.0126236
34. Crane HM, McCaul ME, Chander G, Hutton H, Nance RM, Delaney JAC, et al. Prevalence and Factors Associated With Hazardous Alcohol Use Among Persons Living With HIV Across the US in the Current Era of Antiretroviral Treatment. AIDS Behavior (2017) 21:1914–25. doi: 10.1007/s10461-017-1740-7
35. Marshall BD, Operario D, Bryant KJ, Cook RL, Edelman EJ, Gaither JR, et al. Drinking Trajectories Among HIV-Infected Men Who Have Sex With Men: A Cohort Study of United States Veterans. Drug Alcohol Depend (2015) 148:69–76. doi: 10.1016/j.drugalcdep.2014.12.023
36. Ikeda ML, Barcellos NT, Alencastro PR, Wolff FH, Moreira LB, Gus M, et al. Alcohol Drinking Pattern: A Comparison Between HIV-Infected Patients and Individuals From the General Population. PloS One (2016) 11:e0158535. doi: 10.1371/journal.pone.0158535
37. Justice AC, McGinnis KA, Tate JP, Braithwaite RS, Bryant KJ, Cook RL, et al. Risk of Mortality and Physiologic Injury Evident With Lower Alcohol Exposure Among HIV Infected Compared With Uninfected Men. Drug Alcohol Depend (2016) 161:95–103. doi: 10.1016/j.drugalcdep.2016.01.017
38. Braithwaite RS, Conigliaro J, Roberts MS, Shechter S, Schaefer A, McGinnis K, et al. Estimating the Impact of Alcohol Consumption on Survival for HIV+ Individuals. AIDS Care (2007) 19:459–66. doi: 10.1080/09540120601095734
39. Freiberg MS, McGinnis KA, Kraemer K, Samet JH, Conigliaro J, Curtis Ellison R, et al. The Association Between Alcohol Consumption and Prevalent Cardiovascular Diseases Among HIV-Infected and HIV-Uninfected Men. J Acquir Immune Defic Syndr (2010) 53:247–53. doi: 10.1097/QAI.0b013e3181c6c4b7
40. Minzer S, Losno RA, Casas R. The Effect of Alcohol on Cardiovascular Risk Factors: Is There New Information? Nutrients (2020) 12:912. doi: 10.3390/nu12040912
41. Debes JD, Bohjanen PR, Boonstra A. Mechanisms of Accelerated Liver Fibrosis Progression During HIV Infection. J Clin Trans hepatol (2016) 4:328–35. doi: 10.14218/JCTH.2016.00034
42. Jolley SE, Alkhafaf Q, Hough C, Welsh DA. Presence of an Alcohol Use Disorder Is Associated With Greater Pneumonia Severity in Hospitalized HIV-Infected Patients. Lung (2016) 194:755–62. doi: 10.1007/s00408-016-9920-1
43. Sherman KE, Rockstroh J, Thomas D. Human Immunodeficiency Virus and Liver Disease: An Update. Hepatology (2015) 62:1871–82. doi: 10.1002/hep.28150
44. Ganesan M, Poluektova LY, Kharbanda KK, Osna NA. Liver as a Target of Human Immunodeficiency Virus Infection. World J Gastroenterol (2018) 24:4728–37. doi: 10.3748/wjg.v24.i42.4728
45. Chaudhry AA, Sulkowski MS, Chander G, Moore RD. Hazardous Drinking Is Associated With an Elevated Aspartate Aminotransferase to Platelet Ratio Index in an Urban HIV-Infected Clinical Cohort. HIV Med (2009) 10:133–42. doi: 10.1111/j.1468-1293.2008.00662.x
46. Chen D, Le TH, Shahidipour H, Read SA, Ahlenstiel G. The Role of Gut-Derived Microbial Antigens on Liver Fibrosis Initiation and Progression. Cells (2019) 8:1324. doi: 10.3390/cells8111324
47. Molina PE, Gardner JD, Souza-Smith FM, Whitaker AM. Alcohol Abuse: Critical Pathophysiological Processes and Contribution to Disease Burden. Physiology (2014) 29:203–15. doi: 10.1152/physiol.00055.2013
48. Molina PE, Simon L, Amedee AM, Welsh DA, Ferguson TF. Impact of Alcohol on HIV Disease Pathogenesis, Comorbidities and Aging: Integrating Preclinical and Clinical Findings. Alcohol Alcohol (2018) 53:439–47. doi: 10.1093/alcalc/agy016
49. Ganesan M, New-Aaron M, Dagur RS, Makarov E, Wang W, Kharbanda KK, et al. Alcohol Metabolism Potentiates HIV-Induced Hepatotoxicity: Contribution to End-Stage Liver Disease. Biomolecules (2019) 9:851. doi: 10.3390/biom9120851
50. Hoek JB, Cahill A, Pastorino JG. Alcohol and Mitochondria: A Dysfunctional Relationship. Gastroenterology (2002) 122:2049–63. doi: 10.1053/gast.2002.33613
51. Vivithanaporn P, Heo G, Gamble J, Krentz HB, Hoke A, Gill MJ, et al. Neurologic Disease Burden in Treated HIV/AIDS Predicts Survival: A Population-Based Study. Neurology (2010) 75:1150–8. doi: 10.1212/WNL.0b013e3181f4d5bb
52. Essa MM, Song BJ, Chang SL. Binge Alcohol and HIV: Leaky Gut and Neurodegeneration Through the Gut-Brain Axis. Int J Nutr Pharmacol Neurol Dis (2019) 9:1–3.
53. Zhao J, Lopez AL, Erichsen D, Herek S, Cotter RL, Curthoys NP, et al. Mitochondrial Glutaminase Enhances Extracellular Glutamate Production in HIV-1-Infected Macrophages: Linkage to HIV-1 Associated Dementia. J Neurochem (2004) 88:169–80. doi: 10.1046/j.1471-4159.2003.02146.x
54. Valles SL, Blanco AM, Pascual M, Guerri C. Chronic Ethanol Treatment Enhances Inflammatory Mediators and Cell Death in the Brain and in Astrocytes. Brain Pathol (2004) 14:365–71. doi: 10.1111/j.1750-3639.2004.tb00079.x
55. Navia BA, Rostasy K. The AIDS Dementia Complex: Clinical and Basic Neuroscience With Implications for Novel Molecular Therapies. Neurotoxicity Res (2005) 8:3–24. doi: 10.1007/BF03033817
56. Poonia B, Nelson S, Bagby GJ, Zhang P, Quniton L, Veazey RS. Chronic Alcohol Consumption Results in Higher Simian Immunodeficiency Virus Replication in Mucosally Inoculated Rhesus Macaques. AIDS Res Hum Retroviruses (2006) 22:589–94. doi: 10.1089/aid.2006.22.589
57. Hahn JA, Cheng DM, Emenyonu NI, Lloyd-Travaglini C, Fatch R, Shade SB, et al. Alcohol Use and HIV Disease Progression in an Antiretroviral Naive Cohort. J Acquir Immune Defic Syndr (2018) 77:492–501. doi: 10.1097/QAI.0000000000001624
58. So-Armah KA, Cheng DM, Freiberg MS, Gnatienko N, Patts G, Ma Y, et al. Association Between Alcohol Use and Inflammatory Biomarkers Over Time Among Younger Adults With HIV-The Russia ARCH Observational Study. PloS One (2019) 14:e0219710. doi: 10.1371/journal.pone.0219710
59. Monnig MA, Cohen R, Ramratnam B, McAdams M, Tashima K, Monti PM. HIV Infection, HCV Coinfection, and Alcohol Use: Associations With Microbial Translocation and Immune Activation. Alcoholism Clin Exp Res (2019) 43:1126–34. doi: 10.1111/acer.14032
60. Kuller LH, Tracy R, Belloso W, De Wit S, Drummond F, Lane HC, et al. Inflammatory and Coagulation Biomarkers and Mortality in Patients With HIV Infection. PloS Med (2008) 5:e203. doi: 10.1371/journal.pmed.0050203
61. Sandler NG, Wand H, Roque A, Law M, Nason MC, Nixon DE, et al. Plasma Levels of Soluble CD14 Independently Predict Mortality in HIV Infection. J Infect Dis (2011) 203:780–90. doi: 10.1093/infdis/jiq118
62. Fuster D, Cheng DM, Quinn EK, Armah KA, Saitz R, Freiberg MS, et al. Inflammatory Cytokines and Mortality in a Cohort of HIV-Infected Adults With Alcohol Problems. Aids (2014) 28:1059–64. doi: 10.1097/QAD.0000000000000184
63. Molina PE, Bagby GJ, Nelson S. Biomedical Consequences of Alcohol Use Disorders in the HIV-Infected Host. Curr HIV Res (2014) 12:265–75. doi: 10.2174/1570162x12666140721121849
64. Bryant KJ, Nelson S, Braithwaite RS, Roach D. Integrating HIV/AIDS and Alcohol Research. Alcohol Res Health J Natl Inst Alcohol Abuse Alcoholism (2010) 33:167–78.
65. Braithwaite RS, Bryant KJ. Influence of Alcohol Consumption on Adherence to and Toxicity of Antiretroviral Therapy and Survival. Alcohol Res Health J Natl Inst Alcohol Abuse Alcoholism (2010) 33:280–7.
66. Farska I. Retraction and Firmness of the Fibrin Clot. Physiologia Bohemoslovaca (1966) 15:79–87.
67. Braithwaite RS, Shechter S, Roberts MS, Schaefer A, Bangsberg DR, Harrigan PR, et al. Explaining Variability in the Relationship Between Antiretroviral Adherence and HIV Mutation Accumulation. J Antimicrob Chemother (2006) 58:1036–43. doi: 10.1093/jac/dkl386
68. Gardner EM, Burman WJ, Steiner JF, Anderson PL, Bangsberg DR. Antiretroviral Medication Adherence and the Development of Class-Specific Antiretroviral Resistance. Aids (2009) 23:1035–46. doi: 10.1097/QAD.0b013e32832ba8ec
69. Howard AA, Arnsten JH, Lo Y, Vlahov D, Rich JD, Schuman P, et al. A Prospective Study of Adherence and Viral Load in a Large Multi-Center Cohort of HIV-Infected Women. Aids (2002) 16:2175–82. doi: 10.1097/00002030-200211080-00010
70. Samet JH, Horton NJ, Meli S, Freedberg KA, Palepu A. Alcohol Consumption and Antiretroviral Adherence Among HIV-Infected Persons With Alcohol Problems. Alcoholism Clin Exp Res (2004) 28:572–7. doi: 10.1097/01.alc.0000122103.74491.78
71. Cook RL, Sereika SM, Hunt SC, Woodward WC, Erlen JA, Conigliaro J. Problem Drinking and Medication Adherence Among Persons With HIV Infection. J Gen Intern Med (2001) 16:83–8. doi: 10.1111/j.1525-1497.2001.00122.x
72. Braithwaite RS, McGinnis KA, Conigliaro J, Maisto SA, Crystal S, Day N, et al. A Temporal and Dose-Response Association Between Alcohol Consumption and Medication Adherence Among Veterans in Care. Alcoholism Clin Exp Res (2005) 29:1190–7. doi: 10.1097/01.alc.0000171937.87731.28
73. Kumar S, Jin M, Ande A, Sinha N, Silverstein PS, Kumar A. Alcohol Consumption Effect on Antiretroviral Therapy and HIV-1 Pathogenesis: Role of Cytochrome P450 Isozymes. Expert Opin Drug Metab Toxicol (2012) 8:1363–75. doi: 10.1517/17425255.2012.714366
74. Ogedengbe OO, Naidu ECS, Azu OO. Antiretroviral Therapy and Alcohol Interactions: X-Raying Testicular and Seminal Parameters Under the HAART Era. Eur J Drug Metab Pharmacokinetics (2018) 43:121–35. doi: 10.1007/s13318-017-0438-6
75. Liu X, Zha J, Nishitani J, Chen H, Zack JA. HIV-1 Infection in Peripheral Blood Lymphocytes (PBLs) Exposed to Alcohol. Virology (2003) 307:37–44. doi: 10.1016/s0042-6822(02)00031-4
76. Kumar R, Perez-Casanova AE, Tirado G, Noel RJ, Torres C, Rodriguez I, et al. Increased Viral Replication in Simian Immunodeficiency Virus/Simian-HIV-Infected Macaques With Self-Administering Model of Chronic Alcohol Consumption. J Acquir Immune Defic Syndr (2005) 39:386–90. doi: 10.1097/01.qai.0000164517.01293.84
77. Poonia B, Nelson S, Bagby GJ, Zhang P, Quniton L, Veazey RS. Chronic Alcohol Consumption Results in Higher Simian Immunodeficiency Virus Replication in Mucosally Inoculated Rhesus Macaques. AIDS Res Hum Retroviruses (2005) 21:863–8. doi: 10.1089/aid.2005.21.863
78. Cerf-Bensussan N, Guy-Grand D. Intestinal Intraepithelial Lymphocytes. Gastroenterol Clin N Am (1991) 20:549–76.
79. Mowat AM, Viney JL. The Anatomical Basis of Intestinal Immunity. Immunol Rev (1997) 156:145–66. doi: 10.1111/j.1600-065x.1997.tb00966.x
80. Wong JK, Yukl SA. Tissue Reservoirs of HIV. Curr Opin HIV AIDS (2016) 11:362–70. doi: 10.1097/COH.0000000000000293
81. Estes JD, Harris LD, Klatt NR, Tabb B, Pittaluga S, Paiardini M, et al. Damaged Intestinal Epithelial Integrity Linked to Microbial Translocation in Pathogenic Simian Immunodeficiency Virus Infections. PloS Pathog (2010) 6:e1001052. doi: 10.1371/journal.ppat.1001052
82. Clayton F, Kapetanovic S, Kotler DP. Enteric Microtubule Depolymerization in HIV Infection: A Possible Cause of HIV-Associated Enteropathy. Aids (2001) 15:123–4. doi: 10.1097/00002030-200101050-00019
83. Lapenta C, Boirivant M, Marini M, Santini SM, Logozzi M, Viora M, et al. Human Intestinal Lamina Propria Lymphocytes Are Naturally Permissive to HIV-1 Infection. Eur J Immunol (1999) 29:1202–8. doi: 10.1002/(SICI)1521-4141(199904)29:04<1202::AID-IMMU1202>3.0.CO;2-O
84. Mehandru S, Tenner-Racz K, Racz P, Markowitz M. The Gastrointestinal Tract Is Critical to the Pathogenesis of Acute HIV-1 Infection. J Allergy Clin Immunol (2005) 116:419–22. doi: 10.1016/j.jaci.2005.05.040
85. Anton PA, Elliott J, Poles MA, McGowan IM, Matud J, Hultin LE, et al. Enhanced Levels of Functional HIV-1 Co-Receptors on Human Mucosal T Cells Demonstrated Using Intestinal Biopsy Tissue. AIDS (2000) 14:1761–5.
86. Poles MA, Elliott J, Taing P, Anton PA, Chen IS. A Preponderance of CCR5(+) CXCR4(+) Mononuclear Cells Enhances Gastrointestinal Mucosal Susceptibility to Human Immunodeficiency Virus Type 1 Infection. J Virol (2001) 75:8390–9. doi: 10.1128/jvi.75.18.8390-8399.2001
87. Brenchley JM, Schacker TW, Ruff LE, Price DA, Taylor JH, Beilman GJ, et al. CD4+ T Cell Depletion During All Stages of HIV Disease Occurs Predominantly in the Gastrointestinal Tract. J Exp Med (2004) 200:749–59. doi: 10.1084/jem.20040874
88. Chege D, Sheth PM, Kain T, Kim CJ, Kovacs C, Loutfy M, et al. Sigmoid Th17 Populations, the HIV Latent Reservoir, and Microbial Translocation in Men on Long-Term Antiretroviral Therapy. Aids (2011) 25:741–9. doi: 10.1097/QAD.0b013e328344cefb
89. Marchetti G, Tincati C, Silvestri G. Microbial Translocation in the Pathogenesis of HIV Infection and AIDS. Clin Microbiol Rev (2013) 26:2–18. doi: 10.1128/CMR.00050-12
90. Brenchley JM, Price DA, Schacker TW, Asher TE, Silvestri G, Rao S, et al. Microbial Translocation Is a Cause of Systemic Immune Activation in Chronic HIV Infection. Nat Med (2006) 12:1365–71. doi: 10.1038/nm1511
91. Noguera-Julian M, Rocafort M, Guillen Y, Rivera J, Casadella M, Nowak P, et al. Gut Microbiota Linked to Sexual Preference and HIV Infection. EBioMedicine (2016) 5:135–46. doi: 10.1016/j.ebiom.2016.01.032
92. Lozupone CA, Rhodes ME, Neff CP, Fontenot AP, Campbell TB, Palmer BE. HIV-Induced Alteration in Gut Microbiota: Driving Factors, Consequences, and Effects of Antiretroviral Therapy. Gut Microbes (2014) 5:562–70. doi: 10.4161/gmic.32132
93. Vujkovic-Cvijin I, Dunham RM, Iwai S, Maher MC, Albright RG, Broadhurst MJ, et al. Dysbiosis of the Gut Microbiota Is Associated With HIV Disease Progression and Tryptophan Catabolism. Sci Transl Med (2013) 5:193ra91. doi: 10.1126/scitranslmed.3006438
94. Lozupone CA, Li M, Campbell TB, Flores SC, Linderman D, Gebert MJ, et al. Alterations in the Gut Microbiota Associated With HIV-1 Infection. Cell Host Microbe (2013) 14:329–39. doi: 10.1016/j.chom.2013.08.006
95. Pinto-Cardoso S, Lozupone C, Briceno O, Alva-Hernandez S, Tellez N, Adriana A, et al. Fecal Bacterial Communities in Treated HIV Infected Individuals on Two Antiretroviral Regimens. Sci Rep (2017) 7:43741. doi: 10.1038/srep43741
96. Vesterbacka J, Rivera J, Noyan K, Parera M, Neogi U, Calle M, et al. Richer Gut Microbiota With Distinct Metabolic Profile in HIV Infected Elite Controllers. Sci Rep (2017) 7:6269. doi: 10.1038/s41598-017-06675-1
97. Rocafort M, Noguera-Julian M, Rivera J, Pastor L, Guillen Y, Langhorst J, et al. Evolution of the Gut Microbiome Following Acute HIV-1 Infection. Microbiome (2019) 7:73. doi: 10.1186/s40168-019-0687-5
98. Gao J, Xu K, Liu H, Liu G, Bai M, Peng C, et al. Impact of the Gut Microbiota on Intestinal Immunity Mediated by Tryptophan Metabolism. Front Cell Infect Microbiol (2018) 8:13. doi: 10.3389/fcimb.2018.00013
99. Mellor AL, Munn DH. IDO Expression by Dendritic Cells: Tolerance and Tryptophan Catabolism. Nat Rev Immunol (2004) 4:762–74. doi: 10.1038/nri1457
100. Mehraj V, Ramendra R, Isnard S, Dupuy FP, Ponte R, Chen J, et al. Circulating (1–>3)-Beta-D-Glucan Is Associated With Immune Activation During Human Immunodeficiency Virus Infection. Clin Infect Dis (2020) 70:232–41. doi: 10.1093/cid/ciz212
101. Hubert A, Subra C, Jenabian MA, Tremblay Labrecque PF, Tremblay C, Laffont B, et al. Elevated Abundance, Size, and MicroRNA Content of Plasma Extracellular Vesicles in Viremic HIV-1+ Patients: Correlations With Known Markers of Disease Progression. J Acquir Immune Defic Syndr (2015) 70:219–27. doi: 10.1097/QAI.0000000000000756
102. Chen J, Xun J, Yang J, Ji Y, Liu L, Qi T, et al. Plasma Indoleamine 2,3-Dioxygenase Activity Is Associated With the Size of the Human Immunodeficiency Virus Reservoir in Patients Receiving Antiretroviral Therapy. Clin Infect Dis an Off Publ Infect Dis Soc America (2019) 68:1274–81. doi: 10.1093/cid/ciy676
103. Jenabian MA, Patel M, Kema I, Kanagaratham C, Radzioch D, Thebault P, et al. Distinct Tryptophan Catabolism and Th17/Treg Balance in HIV Progressors and Elite Controllers. PloS One (2013) 8:e78146. doi: 10.1371/journal.pone.0078146
104. Tang WH, Wang Z, Levison BS, Koeth RA, Britt EB, Fu X, et al. Intestinal Microbial Metabolism of Phosphatidylcholine and Cardiovascular Risk. New Engl J Med (2013) 368:1575–84. doi: 10.1056/NEJMoa1109400
105. Brown JM, Hazen SL. The Gut Microbial Endocrine Organ: Bacterially Derived Signals Driving Cardiometabolic Diseases. Annu Rev Med (2015) 66:343–59. doi: 10.1146/annurev-med-060513-093205
106. Romano KA, Vivas EI, Amador-Noguez D, Rey FE. Intestinal Microbiota Composition Modulates Choline Bioavailability From Diet and Accumulation of the Proatherogenic Metabolite Trimethylamine-N-Oxide. mBio (2015) 6:e02481. doi: 10.1128/mBio.02481-14
107. Shan Z, Clish CB, Hua S, Scott JM, Hanna DB, Burk RD, et al. Gut Microbial-Related Choline Metabolite Trimethylamine-N-Oxide Is Associated With Progression of Carotid Artery Atherosclerosis in HIV Infection. J Infect diseases (2018) 218:1474–9. doi: 10.1093/infdis/jiy356
108. Hague A, Butt AJ, Paraskeva C. The Role of Butyrate in Human Colonic Epithelial Cells: An Energy Source or Inducer of Differentiation and Apoptosis? Proc Nutr Society (1996) 55:937–43. doi: 10.1079/pns19960090
109. Kelly CJ, Zheng L, Campbell EL, Saeedi B, Scholz CC, Bayless AJ, et al. Crosstalk Between Microbiota-Derived Short-Chain Fatty Acids and Intestinal Epithelial HIF Augments Tissue Barrier Function. Cell Host Microbe (2015) 17:662–71. doi: 10.1016/j.chom.2015.03.005
110. Dillon SM, Kibbie J, Lee EJ, Guo K, Santiago ML, Austin GL, et al. Low Abundance of Colonic Butyrate-Producing Bacteria in HIV Infection Is Associated With Microbial Translocation and Immune Activation. AIDS (2017) 31:511–21. doi: 10.1097/QAD.0000000000001366
111. Wang Z, Qi Q. Gut Microbial Metabolites Associated With HIV Infection. Future Virol (2019) 14:335–47. doi: 10.2217/fvl-2019-0002
112. Louis P, Flint HJ. Diversity, Metabolism and Microbial Ecology of Butyrate-Producing Bacteria From the Human Large Intestine. FEMS Microbiol Lett (2009) 294:1–8. doi: 10.1111/j.1574-6968.2009.01514.x
113. Raffatellu M, Santos RL, Verhoeven DE, George MD, Wilson RP, Winter SE, et al. Simian Immunodeficiency Virus-Induced Mucosal Interleukin-17 Deficiency Promotes Salmonella Dissemination From the Gut. Nat Med (2008) 14:421–8. doi: 10.1038/nm1743
114. Deeks SG, Tracy R, Douek DC. Systemic Effects of Inflammation on Health During Chronic HIV Infection. Immunity (2013) 39:633–45. doi: 10.1016/j.immuni.2013.10.001
115. Haynes BF. Gut Microbes Out of Control in HIV Infection. Nat Med (2006) 12:1351–2. doi: 10.1038/nm1206-1351
116. Jiang W, Lederman MM, Hunt P, Sieg SF, Haley K, Rodriguez B, et al. Plasma Levels of Bacterial DNA Correlate With Immune Activation and the Magnitude of Immune Restoration in Persons With Antiretroviral-Treated HIV Infection. J Infect Dis (2009) 199:1177–85. doi: 10.1086/597476
117. Ishizaka A, Koga M, Mizutani T, Parbie PK, Prawisuda D, Yusa N, et al. Unique Gut Microbiome in HIV Patients on Antiretroviral Therapy (ART) Suggests Association With Chronic Inflammation. Microbiol Spectr (2021) 9:e0070821. doi: 10.1128/Spectrum.00708-21
118. Mehraj V, Ghali P, Ramendra R, Costiniuk C, Lebouché B, Ponte R, et al. The Evaluation of Risk-Benefit Ratio for Gut Tissue Sampling in HIV Cure Research. J Virus Erad (2017) 3:212–7.
119. George V, Harrison L, Roach M, Li XD, Tierney C, Fischl MA, et al. Associations of Plasma Cytokine and Microbial Translocation Biomarkers With Immune Reconstitution Inflammatory Syndrome. J Infect Dis (2017) 216:1159–63. doi: 10.1093/infdis/jix460
120. Cassol E, Misra V, Holman A, Kamat A, Morgello S, Gabuzda D. Plasma Metabolomics Identifies Lipid Abnormalities Linked to Markers of Inflammation, Microbial Translocation, and Hepatic Function in HIV Patients Receiving Protease Inhibitors. BMC Infect Dis (2013) 13:203. doi: 10.1186/1471-2334-13-203
121. Steele AK, Lee EJ, Vestal B, Hecht D, Dong Z, Rapaport E, et al. Contribution of Intestinal Barrier Damage, Microbial Translocation and HIV-1 Infection Status to an Inflammaging Signature. PloS One (2014) 9:e97171. doi: 10.1371/journal.pone.0097171
122. Marchetti G, Cozzi-Lepri A, Merlini E, Bellistri GM, Castagna A, Galli M, et al. Microbial Translocation Predicts Disease Progression of HIV-Infected Antiretroviral-Naive Patients With High CD4+ Cell Count. AIDS (2011) 25:1385–94. doi: 10.1097/QAD.0b013e3283471d10
123. Marchetti G, Bellistri GM, Borghi E, Tincati C, Ferramosca S, La Francesca M, et al. Microbial Translocation Is Associated With Sustained Failure in CD4+ T-Cell Reconstitution in HIV-Infected Patients on Long-Term Highly Active Antiretroviral Therapy. AIDS (Lond Engl) (2008) 22:2035–8. doi: 10.1097/QAD.0b013e3283112d29
124. Farhour Z, Mehraj V, Chen J, Ramendra R, Lu H, Routy JP. Use of (1–>3)-Beta-D-Glucan for Diagnosis and Management of Invasive Mycoses in HIV-Infected Patients. Mycoses (2018) 61:718–22. doi: 10.1111/myc.12797
125. Morris A, Hillenbrand M, Finkelman M, George MP, Singh V, Kessinger C, et al. Serum (1–>3)-Beta-D-Glucan Levels in HIV-Infected Individuals Are Associated With Immunosuppression, Inflammation, and Cardiopulmonary Function. J Acquir Immune Defic Syndr (2012) 61:462–8. doi: 10.1097/QAI.0b013e318271799b
126. Isnard S, Lin J, Bu S, Fombuena B, Royston L, Routy JP. Gut Leakage of Fungal-Related Products: Turning Up the Heat for HIV Infection. Front Immunol (2021) 12:656414. doi: 10.3389/fimmu.2021.656414
127. Cheru LT, Park EA, Saylor CF, Burdo TH, Fitch KV, Looby S, et al. I-FABP Is Higher in People With Chronic HIV Than Elite Controllers, Related to Sugar and Fatty Acid Intake and Inversely Related to Body Fat in People With HIV. Open Forum Infect Dis (2018) 5:ofy288. doi: 10.1093/ofid/ofy288
128. Shmagel NG, Shmagel KV, Korolevskaya LB, Saidakova EV, Chereshnev VA. [Systemic Inflammation and Compromised Intestinal Barrier During Successful Treatment of Hiv Infection]. Klinicheskaia meditsina (2016) 94:47–51.
129. El Kamari V, Sattar A, McComsey GA. Brief Report: Gut Structural Damage: An Ongoing Process in Chronically Untreated HIV Infection. J Acquir Immune Defic Syndr (2019) 80:242–5. doi: 10.1097/QAI.0000000000001910
130. Sereti I, Krebs SJ, Phanuphak N, Fletcher JL, Slike B, Pinyakorn S, et al. Persistent, Albeit Reduced, Chronic Inflammation in Persons Starting Antiretroviral Therapy in Acute HIV Infection. Clin Infect Dis an Off Publ Infect Dis Soc America (2017) 64:124–31. doi: 10.1093/cid/ciw683
131. Isnard S, Ramendra R, Dupuy FP, Lin J, Fombuena B, Kokinov N, et al. Plasma Levels of C-Type Lectin REG3alpha and Gut Damage in People With Human Immunodeficiency Virus. J Infect Dis (2020) 221:110–21. doi: 10.1093/infdis/jiz423
132. Turner JR. Intestinal Mucosal Barrier Function in Health and Disease. Nat Rev Immunol (2009) 9:799–809. doi: 10.1038/nri2653
133. Bishehsari F, Magno E, Swanson G, Desai V, Voigt RM, Forsyth CB, et al. Alcohol and Gut-Derived Inflammation. Alcohol Res Curr Rev (2017) 38:163–71.
134. Meroni M, Longo M, Dongiovanni P. Alcohol or Gut Microbiota: Who Is the Guilty? Int J Mol Sci (2019) 20:4568. doi: 10.3390/ijms20184568
135. Schoeler M, Caesar R. Dietary Lipids, Gut Microbiota and Lipid Metabolism. Rev Endocrine Metab Disord (2019) 20:461–72. doi: 10.1007/s11154-019-09512-0
136. Leclercq S, Matamoros S, Cani PD, Neyrinck AM, Jamar F, Starkel P, et al. Intestinal Permeability, Gut-Bacterial Dysbiosis, and Behavioral Markers of Alcohol-Dependence Severity. Proc Natl Acad Sci USA (2014) 111:E4485–93. doi: 10.1073/pnas.1415174111
137. Bode C, Bode JC. Effect of Alcohol Consumption on the Gut. Best Pract Res Clin Gastroenterol (2003) 17:575–92. doi: 10.1016/s1521-6918(03)00034-9
138. Tang Y, Zhang L, Forsyth CB, Shaikh M, Song S, Keshavarzian A. The Role of miR-212 and iNOS in Alcohol-Induced Intestinal Barrier Dysfunction and Steatohepatitis. Alcoholism Clin Exp Res (2015) 39:1632–41. doi: 10.1111/acer.12813
139. Leclercq S, Cani PD, Neyrinck AM, Starkel P, Jamar F, Mikolajczak M, et al. Role of Intestinal Permeability and Inflammation in the Biological and Behavioral Control of Alcohol-Dependent Subjects. Brain Behav Immun (2012) 26:911–8. doi: 10.1016/j.bbi.2012.04.001
140. Rocco A, Compare D, Angrisani D, Sanduzzi Zamparelli M, Nardone G. Alcoholic Disease: Liver and Beyond. World J Gastroenterol (2014) 20:14652–9. doi: 10.3748/wjg.v20.i40.14652
141. Abdelmegeed MA, Banerjee A, Jang S, Yoo SH, Yun JW, Gonzalez FJ, et al. CYP2E1 Potentiates Binge Alcohol-Induced Gut Leakiness, Steatohepatitis, and Apoptosis. Free Radical Biol Med (2013) 65:1238–45. doi: 10.1016/j.freeradbiomed.2013.09.009
142. Cho YE, Yu LR, Abdelmegeed MA, Yoo SH, Song BJ. Apoptosis of Enterocytes and Nitration of Junctional Complex Proteins Promote Alcohol-Induced Gut Leakiness and Liver Injury. J Hepatol (2018) 69:142–53. doi: 10.1016/j.jhep.2018.02.005
143. Lu R, Voigt RM, Zhang Y, Kato I, Xia Y, Forsyth CB, et al. Alcohol Injury Damages Intestinal Stem Cells. Alcoholism Clin Exp Res (2017) 41:727–34. doi: 10.1111/acer.13351
144. Chopyk DM, Kumar P, Raeman R, Liu Y, Smith T, Anania FA. Dysregulation of Junctional Adhesion Molecule-A Contributes to Ethanol-Induced Barrier Disruption in Intestinal Epithelial Cell Monolayers. Physiol Rep (2017) 5:e13541. doi: 10.14814/phy2.13541
145. Wang Y, Tong J, Chang B, Wang B, Zhang D, Wang B. Effects of Alcohol on Intestinal Epithelial Barrier Permeability and Expression of Tight Junction-Associated Proteins. Mol Med Rep (2014) 9:2352–6. doi: 10.3892/mmr.2014.2126
146. Ren L, Jia A, Ren M, Lu G, Feng Y, He S. [Histone Deacetylase 3 Inhibitor Alleviates Alcohol-Induced Disruption of Intestinal Epithelial Barrier via Inhibiting Nuclear Factor Kappab]. Xi bao yu fen zi mian yi xue za zhi = Chin J Cell Mol Immunol (2019) 35:800–5.
147. Lippai D, Bala S, Catalano D, Kodys K, Szabo G. Micro-RNA-155 Deficiency Prevents Alcohol-Induced Serum Endotoxin Increase and Small Bowel Inflammation in Mice. Alcoholism Clin Exp Res (2014) 38:2217–24. doi: 10.1111/acer.12483
148. Meroni M, Longo M, Rametta R, Dongiovanni P. Genetic and Epigenetic Modifiers of Alcoholic Liver Disease. Int J Mol Sci (2018) 19:3857. doi: 10.3390/ijms19123857
149. Tang Y, Forsyth CB, Keshavarzian A. The Role of miRNAs in Alcohol-Induced Endotoxemia, Dysfunction of Mucosal Immunity, and Gut Leakiness. Alcoholism Clin Exp Res (2014) 38:2331–4. doi: 10.1111/acer.12551
150. Sibley D, Jerrells TR. Alcohol Consumption by C57BL/6 Mice Is Associated With Depletion of Lymphoid Cells From the Gut-Associated Lymphoid Tissues and Altered Resistance to Oral Infections With Salmonella Typhimurium. J Infect Dis (2000) 182:482–9. doi: 10.1086/315728
151. Lopez MC, Watzl B, Colombo LL, Watson RR. Alterations in Mouse Peyer's Patch Lymphocyte Phenotype After Ethanol Consumption. Alcohol (1997) 14:107–10. doi: 10.1016/s0741-8329(96)00104-8
152. Lopez MC. Chronic Alcohol Consumption Regulates the Expression of Poly Immunoglobulin Receptor (Pigr) and Secretory IgA in the Gut. Toxicol Appl Pharmacol (2017) 333:84–91. doi: 10.1016/j.taap.2017.08.013
153. Wang L, Fouts DE, Starkel P, Hartmann P, Chen P, Llorente C, et al. Intestinal REG3 Lectins Protect Against Alcoholic Steatohepatitis by Reducing Mucosa-Associated Microbiota and Preventing Bacterial Translocation. Cell Host Microbe (2016) 19:227–39. doi: 10.1016/j.chom.2016.01.003
154. Canesso MCC, Lacerda NL, Ferreira CM, Gonçalves JL, Almeida D, Gamba C, et al. Comparing the Effects of Acute Alcohol Consumption in Germ-Free and Conventional Mice: The Role of the Gut Microbiota. BMC Microbiol (2014) 14:240. doi: 10.1186/s12866-014-0240-4.
155. Mutlu EA, Gillevet PM, Rangwala H, Sikaroodi M, Naqvi A, Engen PA, et al. Colonic Microbiome Is Altered in Alcoholism. Am J Physiol Gastrointest Liver Physiol (2012) 302:G966–78. doi: 10.1152/ajpgi.00380.2011
156. Bode JC, Bode C, Heidelbach R, Durr HK, Martini GA. Jejunal Microflora in Patients With Chronic Alcohol Abuse. Hepato-Gastroenterology (1984) 31:30–4.
157. Yan AW, Fouts DE, Brandl J, Starkel P, Torralba M, Schott E, et al. Enteric Dysbiosis Associated With a Mouse Model of Alcoholic Liver Disease. Hepatology (2011) 53:96–105. doi: 10.1002/hep.24018
158. Samuelson DR, Gu M, Shellito JE, Molina PE, Taylor CM, Luo M, et al. Intestinal Microbial Products From Alcohol-Fed Mice Contribute to Intestinal Permeability and Peripheral Immune Activation. Alcoholism Clin Exp Res (2019) 43:2122–33. doi: 10.1111/acer.14176
159. Casafont Morencos F, de las Heras Castano G, Martin Ramos L, Lopez Arias MJ, Ledesma F, Pons Romero F. Small Bowel Bacterial Overgrowth in Patients With Alcoholic Cirrhosis. Digestive Dis Sci (1996) 41:552–6. doi: 10.1007/BF02282340
160. Lunia MK, Sharma BC, Sharma P, Sachdeva S, Srivastava S. Probiotics Prevent Hepatic Encephalopathy in Patients With Cirrhosis: A Randomized Controlled Trial. Clin Gastroenterol Hepatol Off Clin Pract J Am Gastroenterological Assoc (2014) 12:1003–8.e1. doi: 10.1016/j.cgh.2013.11.006
161. Chen Y, Yang F, Lu H, Wang B, Chen Y, Lei D, et al. Characterization of Fecal Microbial Communities in Patients With Liver Cirrhosis. Hepatology (2011) 54:562–72. doi: 10.1002/hep.24423
162. Engen PA, Green SJ, Voigt RM, Forsyth CB, Keshavarzian A. The Gastrointestinal Microbiome: Alcohol Effects on the Composition of Intestinal Microbiota. Alcohol Res Curr Rev (2015) 37:223–36.
163. Xie G, Zhong W, Zheng X, Li Q, Qiu Y, Li H, et al. Chronic Ethanol Consumption Alters Mammalian Gastrointestinal Content Metabolites. J Proteome Res (2013) 12:3297–306. doi: 10.1021/pr400362z
164. Chen P, Torralba M, Tan J, Embree M, Zengler K, Stärkel P, et al. Supplementation of Saturated Long-Chain Fatty Acids Maintains Intestinal Eubiosis and Reduces Ethanol-Induced Liver Injury in Mice. Gastroenterology (2015) 148:203–14.e16. doi: 10.1053/j.gastro.2014.09.014
165. Hendrikx T, Duan Y, Wang Y, Oh JH, Alexander LM, Huang W, et al. Bacteria Engineered to Produce IL-22 in Intestine Induce Expression of REG3G to Reduce Ethanol-Induced Liver Disease in Mice. Gut (2019) 68:1504–15. doi: 10.1136/gutjnl-2018-317232
166. Xie G, Zhong W, Li H, Li Q, Qiu Y, Zheng X, et al. Alteration of Bile Acid Metabolism in the Rat Induced by Chronic Ethanol Consumption. FASEB J Off Publ Fed Am Societies Exp Biol (2013) 27:3583–93. doi: 10.1096/fj.13-231860
167. Wang Z, Zhang X, Zhu L, Yang X, He F, Wang T, et al. Inulin Alleviates Inflammation of Alcoholic Liver Disease via SCFAs-Inducing Suppression of M1 and Facilitation of M2 Macrophages in Mice. Int Immunopharmacol (2020) 78:106062. doi: 10.1016/j.intimp.2019.106062
168. Adachi Y, Moore LE, Bradford BU, Gao W, Thurman RG. Antibiotics Prevent Liver Injury in Rats Following Long-Term Exposure to Ethanol. Gastroenterology (1995) 108:218–24. doi: 10.1016/0016-5085(95)90027-6
169. Forsyth CB, Farhadi A, Jakate SM, Tang Y, Shaikh M, Keshavarzian A. Lactobacillus GG Treatment Ameliorates Alcohol-Induced Intestinal Oxidative Stress, Gut Leakiness, and Liver Injury in a Rat Model of Alcoholic Steatohepatitis. Alcohol (2009) 43:163–72. doi: 10.1016/j.alcohol.2008.12.009
170. Liu Q, Duan ZP, Ha DK, Bengmark S, Kurtovic J, Riordan SM. Synbiotic Modulation of Gut Flora: Effect on Minimal Hepatic Encephalopathy in Patients With Cirrhosis. Hepatology (2004) 39:1441–9. doi: 10.1002/hep.20194
171. Bruellman R, Llorente C. A Perspective Of Intestinal Immune-Microbiome Interactions In Alcohol-Associated Liver Disease. Int J Biol Sci (2021) 17:307–27. doi: 10.7150/ijbs.53589
172. Banerjee A, Abdelmegeed MA, Jang S, Song BJ. Increased Sensitivity to Binge Alcohol-Induced Gut Leakiness and Inflammatory Liver Disease in HIV Transgenic Rats. PloS One (2015) 10:e0140498. doi: 10.1371/journal.pone.0140498
173. Liu C, Zhu P, Fujino M, Zhu S, Ito H, Takahashi K, et al. 5-ALA/SFC Attenuated Binge Alcohol-Induced Gut Leakiness and Inflammatory Liver Disease in HIV Transgenic Rats. Alcohol Clin Exp Res (2019) 43:1651–61. doi: 10.1111/acer.14117
174. Samuelson DR, Siggins RW, Ruan S, Amedee AM, Sun J, Zhu QK, et al. Alcohol Consumption Increases Susceptibility to Pneumococcal Pneumonia in a Humanized Murine HIV Model Mediated by Intestinal Dysbiosis. Alcohol (Fayetteville NY) (2019) 80:33–43. doi: 10.1016/j.alcohol.2018.08.012
175. Caslin B, Mohler K, Thiagarajan S, Melamed E. Alcohol as Friend or Foe in Autoimmune Diseases: A Role for Gut Microbiome? Gut Microbes (2021) 13:1916278. doi: 10.1080/19490976.2021.1916278
176. Meroni M, Longo M. Alcohol or Gut Microbiota: Who Is the Guilty? Int J Mol Sci (2019) 20:4568. doi: 10.3390/ijms20184568
177. Smirnova E, Puri P, Muthiah MD, Daitya K, Brown R, Chalasani N, et al. Fecal Microbiome Distinguishes Alcohol Consumption From Alcoholic Hepatitis But Does Not Discriminate Disease Severity. Hepatology (2020) 72:271–86. doi: 10.1002/hep.31178
178. Yang AM, Inamine T, Hochrath K, Chen P, Wang L, Llorente C, et al. Intestinal Fungi Contribute to Development of Alcoholic Liver Disease. J Clin Invest (2017) 127:2829–41. doi: 10.1172/jci90562
179. González-Hernández LA, Ruiz-Briseño MDR, Sánchez-Reyes K, Alvarez-Zavala M, Vega-Magaña N, López-Iñiguez A, et al. Alterations in Bacterial Communities, SCFA and Biomarkers in an Elderly HIV-Positive and HIV-Negative Population in Western Mexico.BMC Infect Dis (2019) 19:234. doi: 10.1186/s12879-019-3867-9
180. Favre D, Mold J, Hunt PW, Kanwar B, Loke P, Seu L, et al. Tryptophan Catabolism by Indoleamine 2,3-Dioxygenase 1 Alters the Balance of TH17 to Regulatory T Cells in HIV Disease. Sci Transl Med (2010) 2:32ra6. doi: 10.1126/scitranslmed.3000632
181. Li Q, Estes JD, Duan L, Jessurun J, Pambuccian S, Forster C, et al. Simian Immunodeficiency Virus-Induced Intestinal Cell Apoptosis Is the Underlying Mechanism of the Regenerative Enteropathy of Early Infection. J Infect Dis (2008) 197:420–9. doi: 10.1086/525046
182. Wang XH, Song TZ, Zheng HY, Li YH, Zheng YT. Jejunal Epithelial Barrier Disruption Triggered by Reactive Oxygen Species in Early SIV Infected Rhesus Macaques. Free Radical Biol Med (2021) 177:143–55. doi: 10.1016/j.freeradbiomed.2021.10.026
183. Gori A, Rizzardini G, Van't Land B, Amor KB, van Schaik J, Torti C, et al. Specific Prebiotics Modulate Gut Microbiota and Immune Activation in HAART-Naive HIV-Infected Adults: Results of the "COPA" Pilot Randomized Trial. Mucosal Immunol (2011) 4:554–63. doi: 10.1038/mi.2011.15
184. Stiksrud B, Nowak P, Nwosu FC, Kvale D, Thalme A, Sonnerborg A, et al. Reduced Levels of D-Dimer and Changes in Gut Microbiota Composition After Probiotic Intervention in HIV-Infected Individuals on Stable ART. J Acquir Immune Defic Syndr (2015) 70:329–37. doi: 10.1097/qai.0000000000000784
185. Hensley-McBain T, Zevin AS, Manuzak J, Smith E, Gile J, Miller C, et al. Effects of Fecal Microbial Transplantation on Microbiome and Immunity in Simian Immunodeficiency Virus-Infected Macaques. J Virol (2016) 90:4981–9. doi: 10.1128/jvi.00099-16
186. Vujkovic-Cvijin I, Rutishauser RL, Pao M, Hunt PW, Lynch SV, McCune JM, et al. Limited Engraftment of Donor Microbiome via One-Time Fecal Microbial Transplantation in Treated HIV-Infected Individuals. Gut Microbes (2017) 8:440–50. doi: 10.1080/19490976.2017.1334034
187. Sainz T, Gosalbes MJ, Talavera-Rodríguez A. Effect of a Nutritional Intervention on the Intestinal Microbiota of Vertically HIV-Infected Children: The Pediabiota Study. Nutrients (2020) 12:2112. doi: 10.3390/nu12072112
188. Serrano-Villar S, Talavera-Rodriguez A, Gosalbes MJ, Madrid N, Perez-Molina JA, Elliott RJ, et al. Fecal Microbiota Transplantation in HIV: A Pilot Placebo-Controlled Study. Nat Commun (2021) 12:1139. doi: 10.1038/s41467-021-21472-1
189. Kirpich IA, Solovieva NV, Leikhter SN, Shidakova NA, Lebedeva OV, Sidorov PI, et al. Probiotics Restore Bowel Flora and Improve Liver Enzymes in Human Alcohol-Induced Liver Injury: A Pilot Study. Alcohol (2008) 42:675–82. doi: 10.1016/j.alcohol.2008.08.006
190. Stadlbauer V, Mookerjee RP, Hodges S, Wright GA, Davies NA, Jalan R. Effect of Probiotic Treatment on Deranged Neutrophil Function and Cytokine Responses in Patients With Compensated Alcoholic Cirrhosis. J Hepatol (2008) 48:945–51. doi: 10.1016/j.jhep.2008.02.015
191. Philips CA, Phadke N, Ganesan K, Ranade S, Augustine P. Corticosteroids, Nutrition, Pentoxifylline, or Fecal Microbiota Transplantation for Severe Alcoholic Hepatitis. Indian J Gastroenterol Off J Indian Soc Gastroenterology (2018) 37:215–25. doi: 10.1007/s12664-018-0859-4
192. Macnaughtan J, Figorilli F, García-López E, Lu H, Jones H, Sawhney R, et al. A Double-Blind, Randomized Placebo-Controlled Trial of Probiotic Lactobacillus Casei Shirota in Stable Cirrhotic Patients. Nutrients (2020) 12:1651. doi: 10.3390/nu12061651
193. Wang T, Wang Z, Yang Z, Cui X, Yan L, Xu Z, et al. Effect of the Fermentation Broth of the Mixture of Pueraria Lobata, Lonicera Japonica, and Crataegus Pinnatifida by Lactobacillus Rhamnosus 217-1 on Liver Health and Intestinal Flora in Mice With Alcoholic Liver Disease Induced by Liquor. Front Microbiol (2021) 12:722171. doi: 10.3389/fmicb.2021.722171
194. d'Ettorre G, Ceccarelli G, Giustini N, Serafino S, Calantone N, De Girolamo G, et al. Probiotics Reduce Inflammation in Antiretroviral Treated, HIV-Infected Individuals: Results of the "Probio-HIV" Clinical Trial. PloS One (2015) 10:e0137200. doi: 10.1371/journal.pone.0137200
195. Serrano-Villar S, Vazquez-Castellanos JF, Vallejo A, Latorre A, Sainz T, Ferrando-Martinez S, et al. The Effects of Prebiotics on Microbial Dysbiosis, Butyrate Production and Immunity in HIV-Infected Subjects. Mucosal Immunol (2017) 10:1279–93. doi: 10.1038/mi.2016.122
196. van der Lugt B, van Beek AA, Aalvink S, Meijer B, Sovran B, Vermeij WP, et al. Akkermansia Muciniphila Ameliorates the Age-Related Decline in Colonic Mucus Thickness and Attenuates Immune Activation in Accelerated Aging Ercc1 (-/Δ7) Mice. Immun Ageing I A (2019) 16:6. doi: 10.1186/s12979-019-0145-z
197. Reunanen J, Kainulainen V, Huuskonen L, Ottman N, Belzer C, Huhtinen H, et al. Akkermansia Muciniphila Adheres to Enterocytes and Strengthens the Integrity of the Epithelial Cell Layer. Appl Environ Microbiol (2015) 81:3655–62. doi: 10.1128/AEM.04050-14
198. Ottman N, Reunanen J, Meijerink M, Pietilä TE, Kainulainen V, Klievink J, et al. Pili-Like Proteins of Akkermansia Muciniphila Modulate Host Immune Responses and Gut Barrier Function. PloS One (2017) 12:e0173004. doi: 10.1371/journal.pone.0173004
199. Huck O, Mulhall H, Rubin G, Kizelnik Z, Iyer R, Perpich JD, et al. Akkermansia Muciniphila Reduces Porphyromonas Gingivalis-Induced Inflammation and Periodontal Bone Destruction. J Clin Periodontol (2020) 47:202–12. doi: 10.1111/jcpe.13214
200. Wang L, Tang L, Feng Y, Zhao S, Han M, Zhang C, et al. A Purified Membrane Protein From Akkermansia Muciniphila or the Pasteurised Bacterium Blunts Colitis Associated Tumourigenesis by Modulation of CD8(+) T Cells in Mice. Gut (2020) 69:1988–97. doi: 10.1136/gutjnl-2019-320105
201. Grander C, Adolph TE, Wieser V, Lowe P, Wrzosek L, Gyongyosi B, et al. Recovery of Ethanol-Induced Akkermansia Muciniphila Depletion Ameliorates Alcoholic Liver Disease. Gut (2018) 67:891–901. doi: 10.1136/gutjnl-2016-313432
202. Ouyang J, Lin J, Isnard S, Fombuena B, Peng X, Marette A, et al. The Bacterium Akkermansia Muciniphila: A Sentinel for Gut Permeability and Its Relevance to HIV-Related Inflammation. Front Immunol (2020) 11:645. doi: 10.3389/fimmu.2020.00645
203. Isnard S, Lin J, Fombuena B, Ouyang J, Varin TV, Richard C, et al. Repurposing Metformin in Nondiabetic People With HIV: Influence on Weight and Gut Microbiota. Open Forum Infect Dis (2020) 7:ofaa338. doi: 10.1093/ofid/ofaa338
204. Song Y, Garg S, Girotra M, Maddox C, von Rosenvinge EC, Dutta A, et al. Microbiota Dynamics in Patients Treated With Fecal Microbiota Transplantation for Recurrent Clostridium Difficile Infection. PloS One (2013) 8:e81330. doi: 10.1371/journal.pone.0081330
205. Konturek PC, Koziel J, Dieterich W, Haziri D, Wirtz S, Glowczyk I, et al. Successful Therapy of Clostridium Difficile Infection With Fecal Microbiota Transplantation. J Physiol Pharmacol an Off J Polish Physiol Society (2016) 67:859–66.
206. Cammarota G, Ianiro G, Tilg H, Rajilic-Stojanovic M, Kump P, Satokari R, et al. European Consensus Conference on Faecal Microbiota Transplantation in Clinical Practice. Gut (2017) 66:569–80. doi: 10.1136/gutjnl-2016-313017
207. van Nood E, Vrieze A, Nieuwdorp M, Fuentes S, Zoetendal EG, de Vos WM, et al. Duodenal Infusion of Donor Feces for Recurrent Clostridium Difficile. N Engl J Med (2013) 368:407–15. doi: 10.1056/NEJMoa1205037
208. Paquin-Proulx D, Ching C, Vujkovic-Cvijin I, Fadrosh D, Loh L, Huang Y, et al. Bacteroides Are Associated With GALT iNKT Cell Function and Reduction of Microbial Translocation in HIV-1 Infection. Mucosal Immunol (2017) 10:69–78. doi: 10.1038/mi.2016.34
209. Sokol H, Pigneur B, Watterlot L, Lakhdari O, Bermúdez-Humarán LG, Gratadoux JJ, et al. Faecalibacterium Prausnitzii Is an Anti-Inflammatory Commensal Bacterium Identified by Gut Microbiota Analysis of Crohn Disease Patients. Proc Natl Acad Sci USA (2008) 105:16731–6. doi: 10.1073/pnas.0804812105
210. Sugimoto K, Ogawa A, Mizoguchi E, Shimomura Y, Andoh A, Bhan AK, et al. IL-22 Ameliorates Intestinal Inflammation in a Mouse Model of Ulcerative Colitis. J Clin Invest (2008) 118:534–44. doi: 10.1172/JCI33194
211. Naude CE, Carey PD, Laubscher R, Fein G, Senekal M. Vitamin D and Calcium Status in South African Adolescents With Alcohol Use Disorders. Nutrients (2012) 4:1076–94. doi: 10.3390/nu4081076
212. Lieber CS. Relationships Between Nutrition, Alcohol Use, and Liver Disease. Alcohol Res Health J Natl Inst Alcohol Abuse Alcoholism (2003) 27:220–31.
213. Cantorna MT, Snyder L, Arora J. Vitamin A and Vitamin D Regulate the Microbial Complexity, Barrier Function, and the Mucosal Immune Responses to Ensure Intestinal Homeostasis. Crit Rev Biochem Mol Biol (2019) 54:184–92. doi: 10.1080/10409238.2019.1611734
214. Ortiz AM, Klase ZA, DiNapoli SR, Vujkovic-Cvijin I, Carmack K, Perkins MR, et al. IL-21 and Probiotic Therapy Improve Th17 Frequencies, Microbial Translocation, and Microbiome in ARV-Treated, SIV-Infected Macaques. Mucosal Immunol (2016) 9:458–67. doi: 10.1038/mi.2015.75
215. Sereti I, Estes JD, Thompson WL, Morcock DR, Fischl MA, Croughs T, et al. Decreases in Colonic and Systemic Inflammation in Chronic HIV Infection After IL-7 Administration. PloS Pathog (2014) 10:e1003890. doi: 10.1371/journal.ppat.1003890
216. Nunnari G, Pomerantz RJ. IL-7 as a Potential Therapy for HIV-1-Infected Individuals. Expert Opin Biol Ther (2005) 5:1421–6. doi: 10.1517/14712598.5.11.1421
Keywords: HIV, alcohol, gut, microbial translocation, chronic inflammation
Citation: Yan J, Ouyang J, Isnard S, Zhou X, Harypursat V, Routy J-P and Chen Y (2021) Alcohol Use and Abuse Conspires With HIV Infection to Aggravate Intestinal Dysbiosis and Increase Microbial Translocation in People Living With HIV: A Review. Front. Immunol. 12:741658. doi: 10.3389/fimmu.2021.741658
Received: 16 July 2021; Accepted: 30 November 2021;
Published: 17 December 2021.
Edited by:
Mats Bemark, University of Gothenburg, SwedenReviewed by:
Natalie Chichetto, University of Florida, United StatesDerrick Richard Samuelson, University of Nebraska Medical Center, United States
Copyright © 2021 Yan, Ouyang, Isnard, Zhou, Harypursat, Routy and Chen. This is an open-access article distributed under the terms of the Creative Commons Attribution License (CC BY). The use, distribution or reproduction in other forums is permitted, provided the original author(s) and the copyright owner(s) are credited and that the original publication in this journal is cited, in accordance with accepted academic practice. No use, distribution or reproduction is permitted which does not comply with these terms.
*Correspondence: Jean-Pierre Routy, jean-pierre.routy@mcgill.ca; Yaokai Chen, yaokaichen@hotmail.com
†These authors have contributed equally to this work