- Department of Immunoregulation, Institute of Medical Science, Tokyo Medical University, Tokyo, Japan
The interleukin-6 (IL-6)/IL-12 family of cytokines plays critical roles in the induction and regulation of innate and adaptive immune responses. Among the various cytokines, only this family has the unique characteristic of being composed of two distinct subunits, α- and β-subunits, which form a heterodimer with subunits that occur in other cytokines as well. Recently, we found a novel intracellular role for one of the α-subunits, Epstein-Barr virus-induced gene 3 (EBI3), in promoting the proper folding of target proteins and augmenting its expression at the protein level by binding to its target protein and a well-characterized lectin chaperone, calnexin, presumably through enhancing chaperone activity. Because calnexin is ubiquitously and constitutively expressed but EBI3 expression is inducible, these results could open an avenue to establish a new paradigm in which EBI3 plays an important role in further increasing the expression of target molecules at the protein level in collaboration with calnexin under inflammatory conditions. This theory well accounts for the heterodimer formation of EBI3 with p28, and probably with p35 and p19 to produce IL-27, IL-35, and IL-39, respectively. In line with this concept, another β-subunit, p40, plays a critical role in the assembly-induced proper folding of p35 and p19 to produce IL-12 and IL-23, respectively. Thus, chaperone-like activities in proper folding and maturation, which allow the secretion of biologically active heterodimeric cytokines, have recently been highlighted. This review summarizes the current understanding of chaperone-like activities of EBI3 to form heterodimers and other associations together with their possible biological implications.
Introduction
The interleukin-6 (IL-6) family of cytokines was originally defined as cytokines that utilize the common signaling receptor subunit β-receptor glycoprotein 130 (gp130) and comprise IL-6, IL-11, oncostatin M, leukemia inhibitory factor, ciliary neurotrophic factors, cardiotrophin 1, and cardiotrophin-like cytokine factor 1 (1). IL-6 has two very unique signaling pathways: IL-6 classic signaling and IL-6 trans-signaling. IL-6 classic signaling is generally through the receptor complex of membrane-bound IL-6Rα and gp130. By contrast, IL-6 trans-signaling occurs under pathological conditions, in which the soluble form of IL-6Rα (sIL-6Rα) is generated and IL-6 bound to it (IL-6/sIL-6Rα) signals through gp130. The IL-6/sIL-6Rα complex is similar to the IL-12 family of cytokines in molecular structure in that it is a heterodimeric glycoprotein consisting of two distinct subunits, the α- and β-subunit; the α-subunit includes IL-12p35, IL-23p19, and IL-27p28, and the β-subunit includes IL-12p40 and Epstein-Barr virus (EBV)-induced gene 3 (EBI3) (2). Therefore, the IL-6 and IL-12 families of cytokines together are called the IL-6/IL-12 family. This suggests that six potential heterodimers can be formed: IL-12 (p35/p40) (3, 4), IL-23 (p19/p40) (5), IL-27 (p28/EBI3) (6), IL-35 (p35/EBI3) (7), and IL-39 (p19/EBI3) (8–10) are bioactive, and p28/p40, which is putatively called IL-Y (Figure 1) (11). The subunits of IL-12, IL-23, and IL-Y are connected by a disulfide bridge with each subunit, whereas the subunits of IL-27 and IL-35 are not. As receptor subunits, IL-12 and IL-23 utilize the receptor complex between IL-12 receptor β1 (IL-12Rβ1) (12) and IL-12Rβ2 (13), and IL-12Rβ1 (5) and IL-23Rα (14), respectively. IL-27 and IL-Y signal via the receptor complexes between gp130 and the IL-27 receptor (WSX-1) (15) and possibly between IL-12Rβ1 and WSX-1, respectively, although the latter complex has not yet been proven (11). For IL-35, four receptor complexes have been described: IL-12Rβ2/gp130, IL-12Rβ1/WSX-1, IL-12Rβ2/IL-12Rβ2, and gp130/gp130 (16). For IL-39, the receptor complex between IL-23Rα and gp130 has been reported (10). Of note, by shuffling the extracellular and intracellular domains of the IL-6/IL-12 cytokine receptors, synthetic IL-35 and IL-39 signaling was demonstrated to be biologically active (17). Interestingly, these analyses further identified additional biologically active synthetic receptor combinations, IL-12Rβ1/gp130 and IL-23Rα/IL-12Rβ2, whose ligands have not yet been identified (17). As downstream signaling molecules, Janus kinase (JAK)/signal transducer and activator of transcription (STAT) play critical roles in the induction of respective cellular responses. IL-12 and IL-23 mainly activate STAT4 (18) and STAT3 (14), respectively, whereas IL-27 and IL-39 mainly activate STAT1 and STAT3, respectively (9, 19, 20). By contrast, IL-35 mainly activates both STAT1 and STAT4 (16).
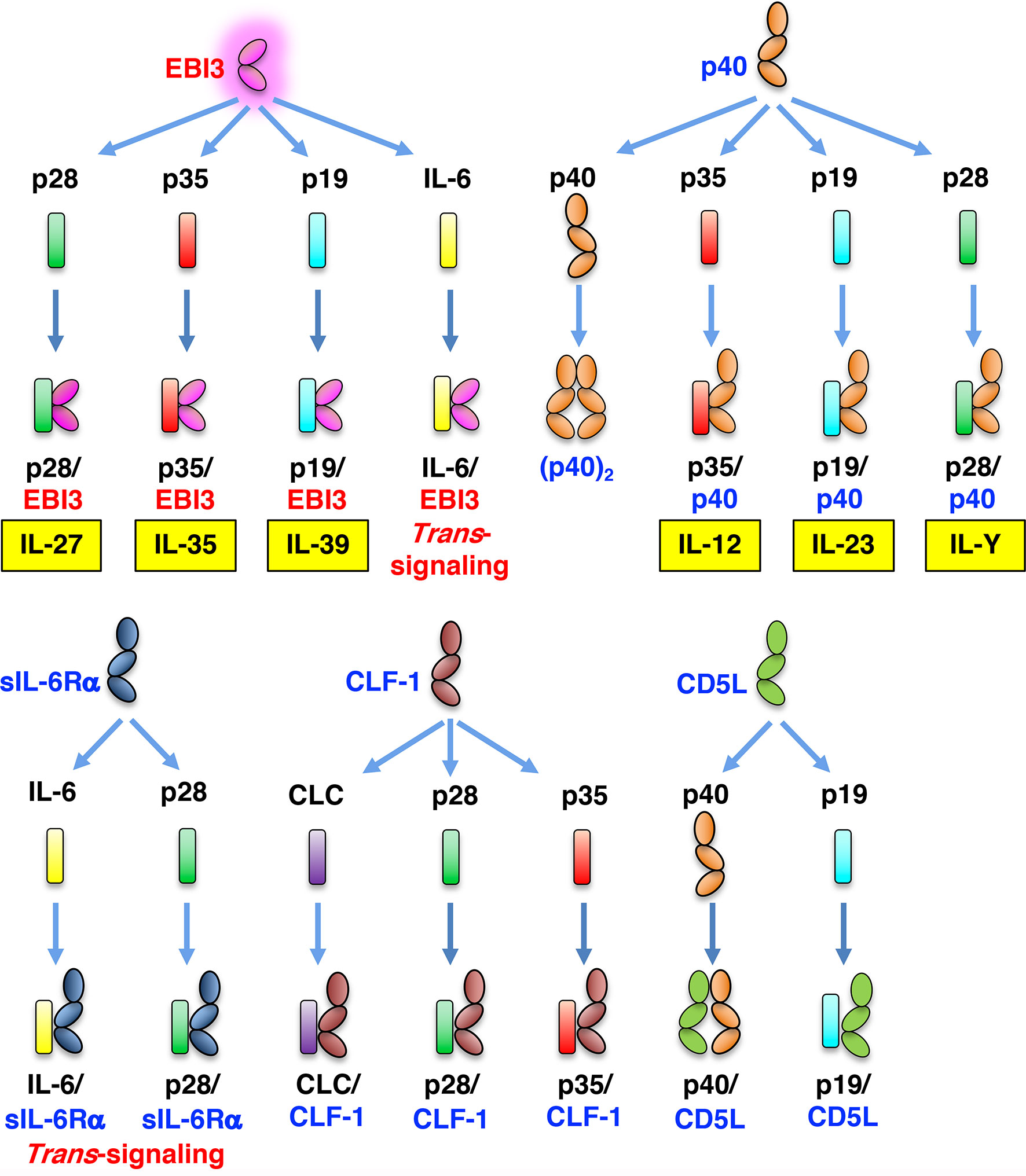
Figure 1 Expanding diversity of the IL-6/IL-12 heterodimeric cytokines and their related heterodimeric molecules. Analogous to the complex of IL-6 and soluble IL-6Rα (IL-6/sIL-6Rα), which induces IL-6 trans-signaling in gp130-expressing cells, various heterodimeric cytokines have been reported and their number is still increasing. CLC, cardiotrophin-like cytokine.
Of note, the diversity in molecular structures and functions of the IL-6/IL-12 family of cytokines and their related heterodimeric molecules [e.g., p28/sIL-6Rα (21), p28/cytokine-like factor-1 (CLF-1) (22), p35/CLF-1 (23), IL-6/EBI3 (24), p40/CD5L (25), p19/CD5L (26); Figure 1] are still growing (2). For proper folding and maturation to form such bioactive and secreted heterodimeric cytokines, their chaperone-like activities have been recently highlighted (27–31). In this review, we summarize and discuss recent advances on the possible molecular mechanisms underlying the generation of such diversity via the chaperone-like functions of EBI3 and possibly p40.
Ability of EBI3 to Associate With Other Proteins
EBI3 was originally identified as an inducible cytokine receptor-like molecule homologous to p40 in B lymphocytes after EBV infection (32). EBI3 is a 34 kDa glycoprotein that contains a signal peptide and two fibronectin type III domains but lacks a membrane-anchoring motif; it is secreted and is also present on the plasma membrane and endoplasmic reticulum (ER) (32). Although the original cloning paper (32) demonstrated that EBI3 associates with calnexin (33, 34), p62 (sequestone-1 or SQSTM1) (35, 36), and an uncharacterized protein (called p78), the physiological significance of these interactions remains unknown, with the exception of calnexin as mentioned below (27). Calnexin plays a critical role in assisting a quality control ensuring proper folding of proteins destined for the plasma membrane or secretion through binding to partially folded or misfolded proteins (34). Calnexin contains a lectin site that recognizes substrate proteins and by this interaction calnexin binds to and participates in the folding of most if not all glycoproteins including MHC class I molecules (33). Calnexin recruits enzymes that catalyze disulfide bond formation and isomeriztion to aid in folding. Calnexin binding retains substrate proteins in the ER until they are fully matured or, for terminally misfolded proteins, until they are targeted for degradation. p62 is a multi-domain and multi-functional adaptor protein, representing not only a selective cargo receptor for autophagy thus inhibiting inflammasome activation and inflammation, but also a central signaling hub, linking several important pro- and anti-inflammatory pathways (35, 36). By binding to the Nrf2 inhibitor Keap1 and resultant autophagosomal degradation, p62 activates Nrf2 and attenuates inflammation. Moreover, p62 activates the central kinase mTOR of the mTORC1 sensor complex that controls cell proliferation and differentiation, and also activates NF-κB, an important regulation of inflammation and cancer development. Subsequently, EBI3 was shown to associate with p35 to form a novel heterodimer p35/EBI3 (37), but the functions of this complex remained unknown until 2017, when their immunosuppression functions were clarified and the composite molecule was named IL-35 (7).
EBI3 Is Necessary for the Proper Folding of IL-23Rα via the Chaperone Calnexin
EBI3 expression is constitutively highest in the placenta but is inducible by infection with EBV in B lymphocytes and activation through Toll-like receptors (TLRs) in dendritic cells (DCs) (32). However, we initially found that EBI3 expression is also induced in naive CD4+ T cells by stimulation with plate-bound anti-cluster of differentiation 3 (CD3) and anti-CD28, and we eventually identified a novel role for EBI3 as an intracellular chaperone-like molecule in CD4+ T cells (27). By using a T cell-dependent mouse colitis model in which colitis is induced by adoptive transfer of naive CD4+ T cells into immunodeficient mice, EBI3-deficient naive CD4+ T cells failed to induce colitis with reduced interferon (IFN)-γ production in the intestinal lamina propria lymphocytes. Similarly, IFN-γ production in EBI3-deficient CD4+ T cells differentiated under pathogenic T helper 17 (Th17) polarizing conditions with IL-23 in vitro was also reduced. However, this effect of EBI3 was not mediated by its culture supernatant, which may contain soluble EBI3 and/or EBI3-related heterodimeric cytokines, suggesting that it was attributed to its intracellular function. During differentiation into pathogenic Th17 cells with IL-23, the expression of one of the IL-23 receptor (IL-23R) subunits, IL-23Rα, but not another IL-23R subunit, IL-12Rβ1, in EBI3-deficient CD4+ T cells was selectively decreased at the protein but not mRNA level. Of note, this reduction in IL-23Rα expression was due to synthesis of its misfolded protein and resultant increased proteasomal degradation of IL-23Rα, indicating a chaperone-like role for EBI3 in the ER. Therefore, these results prompted us to consider calnexin, which is a well-known lectin chaperone involved in the proper folding of newly synthesized glycoproteins in the lumen of the ER (38, 39), and whose association with EBI3 in the ER has already been reported (32). As expected, EBI3 augmented IL-23Rα expression via binding to calnexin and IL-23Rα in a peptide-dependent but not glycan-dependent manner (27). In the absence of endogenous calnexin, EBI3 failed to augment IL-23Rα expression. Moreover, EBI3 bound to the extracellular region of IL-23Rα, but its binding to the IL-23Rα variant G149R was reduced and therefore EBI3 failed to increase its variant protein expression. Consistent with these results, the IL-23Rα variant G149R, which is associated with protection against the development of inflammatory bowel disease in humans, was recently demonstrated to have its loss of function due to impaired protein stability and resultant decreased signaling by IL-23 (40). Thus, EBI3 plays an important role in further augmenting the expression of IL-23Rα at the protein level in collaboration with calnexin (Figure 2).
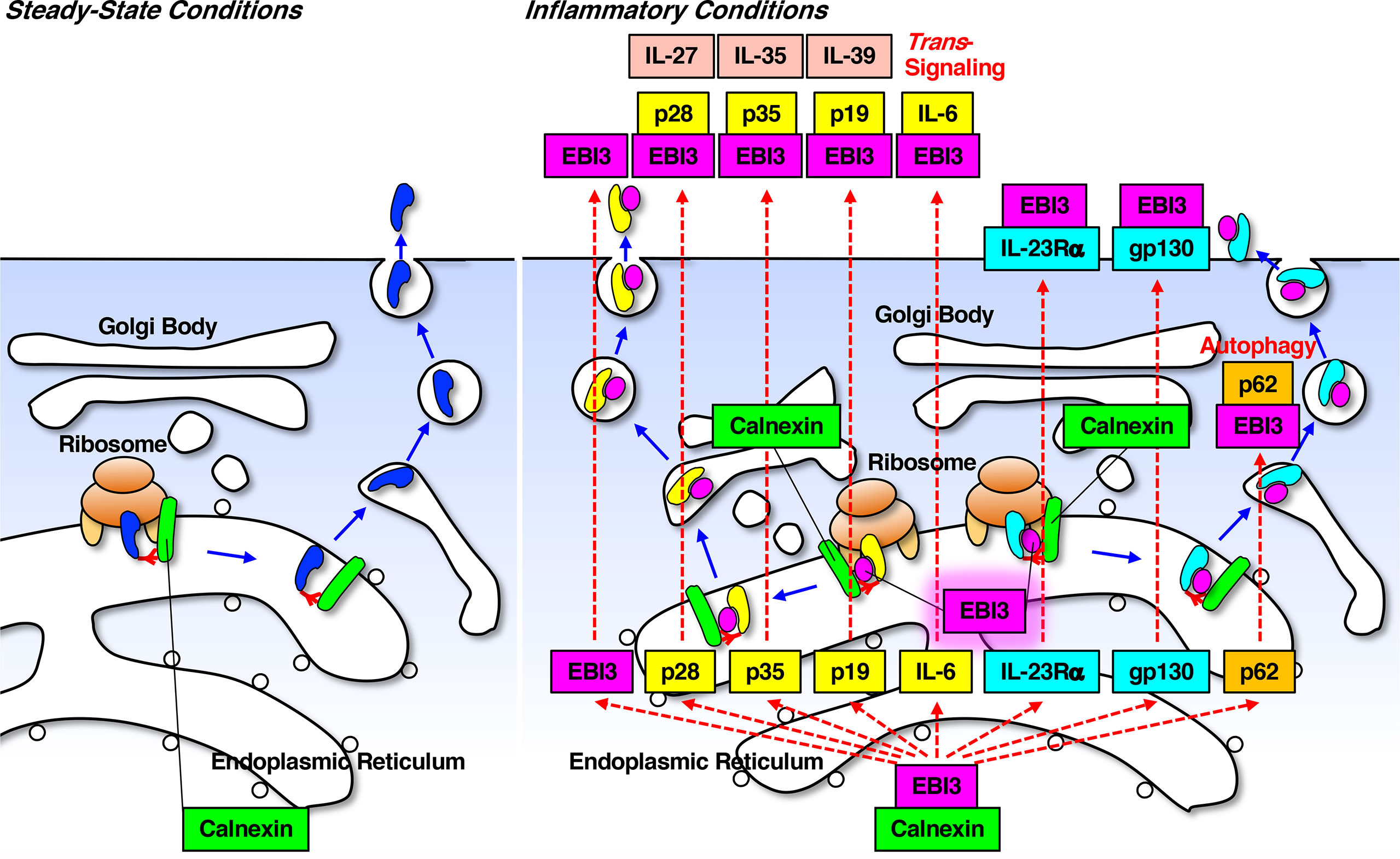
Figure 2 EBI3 plays an important role in promoting the formation of proper protein folding in collaboration with calnexin under inflammatory conditions. Calnexin plays a critical role in assisting a quality control ensuring proper folding of most if not all glycoproteins including MHC class I molecules destined for the plasma membrane or secretion by binding to partially folded or misfolded proteins. p62 is a multi-domain and multi-functional adaptor protein, representing not only a selective cargo receptor for autophagy thus inhibiting inflammasome activation and inflammation, but also a central signaling hub, linking several important pro- and anti-inflammatory pathways including Nrf2, mTOR, NF-κB, and so on. Because calnexin expression is constitutive but EBI3 expression is inducible through activation of TLRs and T-cell receptors, calnexin plays a critical role in the formation of proper protein folding under both steady-state conditions and inflammatory conditions, but under inflammatory conditions, EBI3 plays an additional role to further augment it.
EBI3 Facilitates the Proper Protein Folding of p28 to Form IL-27
Given the chaperone-like activity of EBI3, it is highly conceivable and understandable that EBI3 associates with other molecules such as p28, p35, and p19 to form the heterodimers IL-27, IL-35, and IL-39, respectively (Figure 2). Indeed, another chaperone-like activity of EBI3 was reported for formation of IL-27 (30, 31). In mice, p28, also designated as IL-30, is secreted and exerts immunoregulatory roles (41), while human p28 is not autonomously secreted due to the difference in one amino acid residue cysteine that is necessary for disulfide bond formation (30). Without EBI3 human p28 is retained and becomes degraded by the ER quality control machinery, whereas the presence of EBI3 facilitates the proper protein folding, leading to efficient secretion of human p28 and formation of IL-27 heterodimer. In mice p28 alone is secreted, and the presence of EBI3 further augments the secretion of mouse p28 and formation of IL-27 heterodimer. Although the formation and secretion of IL-27 heterodimer seem efficient, that does not appear to be the case for the IL-35 heterodimer (42, 43) for unknown reasons that might be related to the chaperone-like activity of EBI3. More detailed analyses on the molecular mechanisms underlying how EBI3 selectively binds to them and forms functional heterodimers are necessary.
Chaperone-Like Role for EBI3 in Collaboration With Calnexin Under Inflammatory Conditions
As mentioned above, in addition to the role of EBI3 as a subunit of cytokines, EBI3 has an intracellular chaperone-like role to promote the proper folding of target proteins and increase its expression at the protein level by binding to calnexin and its target protein (27). Although EBI3 is predominantly expressed in placenta among normal tissues (32, 44), its expression is induced under pathological conditions including infectious diseases, inflammatory diseases, autoimmune diseases, cancers, and so on as described below. Stimulation of TLRs with lipopolysaccharide (LPS), poly (I:C), and R848 in DCs, monocytes, macrophages, endothelial cells, keratinocytes, and neutrophils induces EBI3 expression through NF-κB activation (8, 45–48). Moreover, EBI3 expression is induced in B cells after infection with EBV, and it is also induced in malaria-specific CD4+ T cells by activation through T-cell receptors after infection with malaria, to produce IL-27 (32, 49). It was also recently demonstrated that the infection of macrophages with virulent Mycobacterium tuberculosis increases intracellular EBI3 expression via its binding to eukaryotic translation elongation factor 1-α1 (eEF1A1), probably to facilitate infection (50). Because it was reported that eEF1A1 exhibits chaperone-like activity (51), the binding of EBI3 to eEF1A1 reduces Lys48-linked ubiquitination of EBI3, leading to accumulation of EBI3, and consequently inhibits caspase 3-mediated apoptosis in macrophages (50). In addition, there are several reports showing that EBI3 seemingly as a self-standing molecule is involved in the pathogenesis of inflammatory diseases including rheumatoid arthritis (52), inflammatory bowel disease (53), systemic sclerosis (54), cardiac inflammation (55), and several cancers (44, 56–58), whereas whether its chaperone-like role is relevant or not remains unknown. Considering that calnexin expression is constitutive but EBI3 expression is thus inducible, calnexin plays a critical role in the formation of proper protein folding under both steady-state and inflammatory conditions, but under inflammatory conditions, EBI3 plays an additional role to augment it. To date, proper protein folding has been mainly discussed independently of steady-state or inflammatory conditions, and there is no special theory to explain it under inflammatory conditions. Therefore, these findings may open an avenue to establish a new concept in which EBI3 plays an important role in promoting the formation of proper protein folding in collaboration with calnexin under inflammatory conditions (Figure 2). Further identification of other possible target molecules to which EBI3 binds is necessary for confirmation.
Cell Surface Expression of ER Chaperones Including EBI3
Many ER chaperones including calreticulin, heat shock proteins, and isomerases translocate to the cell surface via binding to other cell surface molecules and are also released into the extracellular space upon ER stress induced by stimuli such as chemicals, ultraviolet irradiation, and microorganisms (59). Some chaperones can then act as danger signals to mount protective immune responses via activation of innate and adaptive immune systems. Analogous to these ER chaperones, co-transfection of EBI3 and IL-23Rα expression vectors into a derivative of human embryonic kidney HEK293 cells induces abundant expression of EBI3 on the cell surface, which can be detected by flow cytometry (27). Interestingly, transfection of EBI3 expression vector alone without IL-23Rα significantly induces its cell surface expression, but to a lesser extent, suggesting that EBI3 probably binds to other cell surface molecules that remain to be determined. Further studies are necessary to determine whether the cell surface expression of EBI3 is just a consequence of its chaperone-like activity to increase the expression of target molecules such as IL-23Rα, or whether it has more positive roles such as to function as danger signals, alarmins, find-me signals, eat-me signals, or maybe receptors.
EBI3 Expression Is Highly Associated With Tumor Growth
Whether the expression of EBI3 predicts a good or poor prognosis for cancer patients remains a subject of debate. This is because, in addition to its intracellular functions, the IL-27 heterodimeric cytokine, which uses EBI3 as one of its subunits, has both antitumor and protumor activities (60) and the IL-35 cytokine mainly has protumor activity by inducing immune suppression, and enhancing tumor angiogenesis and myeloid cell accumulation (61–63). Nevertheless, the upregulation of EBI3 expression is highly associated with tumor progression and metastasis in a variety of cancers including breast (58), gastric (64), lung (44), pancreatic (65), cervical (66), and nasopharyngeal (67) cancers. Nishino et al. (43) previously screened genes encoding transmembrane/secretory molecules that were commonly transactivated in lung cancers by gene expression profile analyses of 120 lung cancers. Interestingly, EBI3 was consequently identified as a molecule whose expression was highly correlated with the poor prognosis of patients with non-small cell lung cancer. Inhibition of endogenous EBI3 expression in lung cancer cells by small interfering RNA markedly reduced their growth, while overexpression of exogenous EBI3 in COS-7 monkey kidney cells greatly enhanced their growth (44). Thus, EBI3 seems to be associated with tumor growth and a highly malignant phenotype of lung cancers. Although the mechanisms underlying the growth-promoting effects of EBI3 remain to be clarified, its chaperone-like activity might be relevant. As one possibility, the EBI3-mediated activation of signaling molecules or receptors may be involved as previously reported for the cell surface Y186-Y190 deletion mutant of gp130, which is retained in the ER due to its association with the chaperone calnexin, and induces autonomous cell growth through STAT3 activation in a ligand-independent manner (68). Although the target molecule of EBI3 remains to be identified, the expression of EBI3 is highly associated with tumor growth.
p40-Mediated Assembly-Induced Secretion of IL-12 and IL-23
Another β-subunit, p40, which is overexpressed and secreted alone, is necessary for secretion of p35 after forming the IL-12 heterodimer via a disulfide bond (69). Newly synthesized p35 in the ribosome fails to form its native structure and is misfolded, forming incorrect disulfide bonds (28). However, the co-expression of p40 inhibits the misfolding and allows secretion of biologically active heterodimer IL-12. Interestingly, misfolding also occurs for p19, and a similar mechanism is applied for the synthesis of IL-23 consisting of p19 and p40 (29). Thus, analogous to EBI3 acting like a chaperone, p40 mediates the assembly-induced folding of p35 and p19 and resultant formation in the ER of their heterodimers IL-12 and IL-23, respectively, which seem to be a common principle for secreting them in this heterodimeric cytokine family.
Experimental Evidences for Association of EBI3 With p28, p35, and p19 in Primary Cells
Finally, we refer to the issue whether there is any evidence to prove that EBI3 associates with p28, p35, and p19 in primary cells, to form respective natural heterodimers. This is because EBI3 lacks critical cysteine residue necessary for heterodimerization that is present in p40 to form IL-12 and IL-23 (32, 70), and most of studies so far showed the association of each subunit in the cells transfected with each expression vector having different tags. IL-27 is produced in DCs and macrophages after stimulation with stimuli such as LPS and the association between EBI3 and p28 was detected in the culture supernatant by specific sandwich ELISA (6). IL-35 was identified as an inhibitory cytokine that is produced by regulatory T cells and is necessary for maximal suppressive activity (7). In the supernatant of purified mouse regulatory T cells cultured in the absence of stimuli, the association between EBI3 and p35 was clearly detected by immunoprecipitation with anti-p35 antibody followed by western blotting with anti-EBI3 antibody (7). The association between EBI3 and p19 in primary cells was more clearly demonstrated in the following study (10). The presence of natural IL-39 protein in the culture supernatant of purified mouse primary B cells activated with LPS was detected by immunoprecipitation followed by western blotting using respective antibodies. In addition, sandwich ELISA by using anti-19 and anti-EBI3 antibodies as coating and detection antibodies, respectively, clearly detected and determined the concentration of IL-39 in the supernatant. Overall, these evidences could prove but may not completely yet the association of EBI3 with p28, p35, and p19 in primary cells.
Conclusion and Future Perspectives
Recently, the mutation of another ER chaperone, calreticulin, was demonstrated to be a driver of the development of myeloproliferative neoplasms characterized by the clonal proliferation of hematopoietic stem and progenitor cells (71, 72). This is attributed to constitutive activation of the thrombopoietin receptor, myeloproliferative leukemia protein (MPL), by the mutant calreticulin, resulting in transformation. Surprisingly, the underlying mechanism is so unique that it is currently considered to be due to initial formation of a dimeric or homomultimeric complex of the mutant calreticulin via a novel mutant-specific sequence generated by frameshift mutation, and subsequent interaction with immature asparagine-linked glycan for eventual engagement with immature MPL in the ER (73, 74). The complex formed between mutant calreticulin and MPL is then transported to the cell surface, where it induces constitutive activation of the downstream JAK2/STAT5 signaling pathway in an MPL-dependent manner. Whether such a scenario can also be applied to the EBI3-mediated augmentation of tumor growth as mentioned above is an interesting question but remains unknown.
Currently, chaperone-like functions of cytokine subunits and cytokine-like functions of chaperones together with their physiological significance have garnered great interest. A better understanding of the expanding diversity in molecular structures and functions together with their molecular mechanisms and physiological significance would lead to the identification of new heterodimers and associated molecules and the establishment of novel therapeutic strategies by using them as tools and target molecules.
Author Contributions
All authors contributed to the article and approved the submitted version.
Funding
This study was supported in part by grants from the Ministry of Education, Culture, Sports, Science, and Technology, Japan.
Conflict of Interest
The authors declare that the research was conducted in the absence of any commercial or financial relationships that could be construed as a potential conflict of interest.
Publisher’s Note
All claims expressed in this article are solely those of the authors and do not necessarily represent those of their affiliated organizations, or those of the publisher, the editors and the reviewers. Any product that may be evaluated in this article, or claim that may be made by its manufacturer, is not guaranteed or endorsed by the publisher.
References
1. Rose-John S. Interleukin-6 Family Cytokines. Cold Spring Harb Perspect Biol (2018) 10(2):a028415. doi: 10.1101/cshperspect.a028415
2. Hasegawa H, Mizoguchi I, Chiba Y, Ohashi M, Xu M, Yoshimoto T. Expanding Diversity in Molecular Structures and Functions of the IL-6/IL-12 Heterodimeric Cytokine Family. Front Immunol (2016) 7:479. doi: 10.3389/fimmu.2016.00479
3. Kobayashi M, Fitz L, Ryan M, Hewick RM, Clark SC, Chan S, et al. Identification and Purification of Natural Killer Cell Stimulatory Factor (NKSF), a Cytokine With Multiple Biologic Effects on Human Lymphocytes. J Exp Med (1989) 170(3):827–45. doi: 10.1084/jem.170.3.827
4. Stern AS, Podlaski FJ, Hulmes JD, Pan YC, Quinn PM, Wolitzky AG, et al. Purification to Homogeneity and Partial Characterization of Cytotoxic Lymphocyte Maturation Factor From Human B-Lymphoblastoid Cells. Proc Natl Acad Sci U S A (1990) 87(17):6808–12. doi: 10.1073/pnas.87.17.6808
5. Oppmann B, Lesley R, Blom B, Timans JC, Xu Y, Hunte B, et al. Novel P19 Protein Engages IL-12p40 to Form a Cytokine, IL-23, With Biological Activities Similar as Well as Distinct From IL-12. Immunity (2000) 13(5):715–25. doi: 10.1016/S1074-7613(00)00070-4
6. Pflanz S, Timans JC, Cheung J, Rosales R, Kanzler H, Gilbert J, et al. IL-27, a Heterodimeric Cytokine Composed of EBI3 and P28 Protein, Induces Proliferation of Naive CD4+ T Cells. Immunity (2002) 16(6):779–90. doi: 10.1016/S1074-7613(02)00324-2
7. Collison LW, Workman CJ, Kuo TT, Boyd K, Wang Y, Vignali KM, et al. The Inhibitory Cytokine IL-35 Contributes to Regulatory T-Cell Function. Nature (2007) 450(7169):566–9. doi: 10.1038/nature06306
8. Ramnath D, Tunny K, Hohenhaus DM, Pitts CM, Bergot AS, Hogarth PM, et al. TLR3 Drives IRF6-Dependent IL-23p19 Expression and P19/EBI3 Heterodimer Formation in Keratinocytes. Immunol Cell Biol (2015) 93(9):771–9. doi: 10.1038/icb.2015.77
9. Wang X, Liu X, Zhang Y, Wang Z, Zhu G, Han G, et al. Interleukin (IL)-39 [IL-23p19/Epstein-Barr Virus-Induced 3 (Ebi3)] Induces Differentiation/Expansion of Neutrophils in Lupus-Prone Mice. Clin Exp Immunol (2016) 186(2):144–56. doi: 10.1111/cei.12840
10. Wang X, Wei Y, Xiao H, Liu X, Zhang Y, Han G, et al. A Novel IL-23p19/Ebi3 (IL-39) Cytokine Mediates Inflammation in Lupus-Like Mice. Eur J Immunol (2016) 46(6):1343–50. doi: 10.1002/eji.201546095
11. Wang RX, Yu CR, Mahdi RM, Egwuagu CE. Novel IL27p28/IL12p40 Cytokine Suppressed Experimental Autoimmune Uveitis by Inhibiting Autoreactive Th1/Th17 Cells and Promoting Expansion of Regulatory T Cells. J Biol Chem (2012) 287(43):36012–21. doi: 10.1074/jbc.M112.390625
12. Chua AO, Chizzonite R, Desai BB, Truitt TP, Nunes P, Minetti LJ, et al. Expression Cloning of a Human IL-12 Receptor Component. A New Member of the Cytokine Receptor Superfamily With Strong Homology to Gp130. J Immunol (1994) 153(1):128–36.
13. Presky DH, Yang H, Minetti LJ, Chua AO, Nabavi N, Wu CY, et al. A Functional Interleukin 12 Receptor Complex is Composed of Two Beta-Type Cytokine Receptor Subunits. Proc Natl Acad Sci U S A (1996) 93(24):14002–7. doi: 10.1073/pnas.93.24.14002
14. Parham C, Chirica M, Timans J, Vaisberg E, Travis M, Cheung J, et al. A Receptor for the Heterodimeric Cytokine IL-23 is Composed of IL-12Rbeta1 and a Novel Cytokine Receptor Subunit, IL-23R. J Immunol (2002) 168(11):5699–708. doi: 10.4049/jimmunol.168.11.5699
15. Pflanz S, Hibbert L, Mattson J, Rosales R, Vaisberg E, Bazan JF, et al. WSX-1 and Glycoprotein 130 Constitute a Signal-Transducing Receptor for IL-27. J Immunol (2004) 172(4):2225–31. doi: 10.4049/jimmunol.172.4.2225
16. Collison LW, Delgoffe GM, Guy CS, Vignali KM, Chaturvedi V, Fairweather D, et al. The Composition and Signaling of the IL-35 Receptor are Unconventional. Nat Immunol (2012) 13(3):290–9. doi: 10.1038/ni.2227
17. Floss DM, Schonberg M, Franke M, Horstmeier FC, Engelowski E, Schneider A, et al. IL-6/IL-12 Cytokine Receptor Shuffling of Extra- and Intracellular Domains Reveals Canonical STAT Activation via Synthetic IL-35 and IL-39 Signaling. Sci Rep (2017) 7(1):15172. doi: 10.1038/s41598-017-15173-3
18. Bacon CM, Petricoin EF 3rd, Ortaldo JR, Rees RC, Larner AC, Johnston JA, et al. Interleukin 12 Induces Tyrosine Phosphorylation and Activation of STAT4 in Human Lymphocytes. Proc Natl Acad Sci U S A (1995) 92(16):7307–11. doi: 10.1073/pnas.92.16.7307
19. Lucas S, Ghilardi N, Li J, de Sauvage FJ. IL-27 Regulates IL-12 Responsiveness of Naive CD4+ T Cells Through Stat1-Dependent and -Independent Mechanisms. Proc Natl Acad Sci U S A (2003) 100(25):15047–52. doi: 10.1073/pnas.2536517100
20. Kamiya S, Owaki T, Morishima N, Fukai F, Mizuguchi J, Yoshimoto T. An Indispensable Role for STAT1 in IL-27-Induced T-Bet Expression But Not Proliferation of Naive CD4+ T Cells. J Immunol (2004) 173:3871–7. doi: 10.4049/jimmunol.173.6.3871
21. Garbers C, Spudy B, Aparicio-Siegmund S, Waetzig GH, Sommer J, Holscher C, et al. An Interleukin -6 Receptor-Dependent Molecular Switch Mediates Signal Transduction of the IL-27 Cytokine Subunit P28 (IL-30) via a Gp130 Receptor Homodimer. J Biol Chem (2013) 288(6):4346–54. doi: 10.1074/jbc.M112.432955
22. Crabe S, Guay-Giroux A, Tormo AJ, Duluc D, Lissilaa R, Guilhot F, et al. The IL-27 P28 Subunit Binds Cytokine-Like Factor 1 to Form a Cytokine Regulating NK and T Cell Activities Requiring IL-6R for Signaling. J Immunol (2009) 183(12):7692–702. doi: 10.4049/jimmunol.0901464
23. Detry S, Skladanowska K, Vuylsteke M, Savvides SN, Bloch Y. Revisiting the Combinatorial Potential of Cytokine Subunits in the IL-12 Family. Biochem Pharmacol (2019) 165:240–8. doi: 10.1016/j.bcp.2019.03.026
24. Chehboun S, Labrecque-Carbonneau J, Pasquin S, Meliani Y, Meddah B, Ferlin W, et al. Epstein-Barr Virus-Induced Gene 3 (EBI3) can Mediate IL-6 Trans-Signaling. J Biol Chem (2017) 292(16):6644–56. doi: 10.1074/jbc.M116.762021
25. Abdi K, Singh NJ, Spooner E, Kessler BM, Radaev S, Lantz L, et al. Free IL-12p40 Monomer is a Polyfunctional Adaptor for Generating Novel IL-12-Like Heterodimers Extracellularly. J Immunol (2014) 192(12):6028–36. doi: 10.4049/jimmunol.1400159
26. Hasegawa H, Mizoguchi I, Orii N, Inoue S, Katahira Y, Yoneto T, et al. IL-23p19 and CD5 Antigen-Like Form a Possible Novel Heterodimeric Cytokine and Contribute to Experimental Autoimmune Encephalomyelitis Development. Sci Rep (2021) 11(1):5266. doi: 10.1038/s41598-021-84624-9
27. Mizoguchi I, Ohashi M, Hasegawa H, Chiba Y, Orii N, Inoue S, et al. EBV-Induced Gene 3 Augments IL-23Ralpha Protein Expression Through a Chaperone Calnexin. J Clin Invest (2020) 130(11):6124–40. doi: 10.1172/JCI122732
28. Reitberger S, Haimerl P, Aschenbrenner I, Esser-von Bieren J, Feige MJ. Assembly-Induced Folding Regulates Interleukin 12 Biogenesis and Secretion. J Biol Chem (2017) 292(19):8073–81. doi: 10.1074/jbc.M117.782284
29. Meier S, Bohnacker S, Klose CJ, Lopez A, Choe CA, Schmid PWN, et al. The Molecular Basis of Chaperone-Mediated Interleukin 23 Assembly Control. Nat Commun (2019) 10(1):4121. doi: 10.1038/s41467-019-12006-x
30. Muller SI, Friedl A, Aschenbrenner I, Esser-von Bieren J, Zacharias M, Devergne O, et al. A Folding Switch Regulates Interleukin 27 Biogenesis and Secretion of its Alpha-Subunit as a Cytokine. Proc Natl Acad Sci U S A (2019) 116(5):1585–90. doi: 10.1073/pnas.1816698116
31. Muller SI, Aschenbrenner I, Zacharias M, Feige MJ. An Interspecies Analysis Reveals Molecular Construction Principles of Interleukin 27. J Mol Biol (2019) 431(12):2383–93. doi: 10.1016/j.jmb.2019.04.032
32. Devergne O, Hummel M, Koeppen H, Le Beau MM, Nathanson EC, Kieff E, et al. A Novel Interleukin-12 P40-Related Protein Induced by Latent Epstein-Barr Virus Infection in B Lymphocytes. J Virol (1996) 70(2):1143–53. doi: 10.1128/JVI.70.2.1143-1153.1996
33. Jackson MR, Cohen-Doyle MF, Peterson PA, Williams DB. Regulation of MHC Class I Transport by the Molecular Chaperone, Calnexin (P88, IP90). Science (1994) 263(5145):384–7. doi: 10.1126/science.8278813
34. Braakman I, Hebert DN. Protein Folding in the Endoplasmic Reticulum. Cold Spring Harb Perspect Biol (2013) 5(5):a013201. doi: 10.1101/cshperspect.a013201
35. Sanchez-Martin P, Komatsu M. P62/SQSTM1 - Steering the Cell Through Health and Disease. J Cell Sci (2018) 131(21):jcs222836. doi: 10.1242/jcs.222836
36. Hennig P, Fenini G, Di Filippo M, Karakaya T, Beer HD. The Pathways Underlying the Multiple Roles of P62 in Inflammation and Cancer. Biomedicines (2021) 9(7):707. doi: 10.3390/biomedicines9070707
37. Devergne O, Birkenbach M, Kieff E. Epstein-Barr Virus-Induced Gene 3 and the P35 Subunit of Interleukin 12 Form a Novel Heterodimeric Hematopoietin. Proc Natl Acad Sci U S A (1997) 94(22):12041–6. doi: 10.1073/pnas.94.22.12041
38. Helenius A, Aebi M. Roles of N-Linked Glycans in the Endoplasmic Reticulum. Annu Rev Biochem (2004) 73:1019–49. doi: 10.1146/annurev.biochem.73.011303.073752
39. Parodi AJ. Protein Glucosylation and its Role in Protein Folding. Annu Rev Biochem (2000) 69:69–93. doi: 10.1146/annurev.biochem.69.1.69
40. Sivanesan D, Beauchamp C, Quinou C, Lee J, Lesage S, Chemtob S, et al. IL23R (Interleukin 23 Receptor) Variants Protective Against Inflammatory Bowel Diseases (IBD) Display Loss of Function Due to Impaired Protein Stability and Intracellular Trafficking. J Biol Chem (2016) 291(16):8673–85. doi: 10.1074/jbc.M116.715870
41. Min B, Kim D, Feige MJ. IL-30(Dagger) (IL-27A): A Familiar Stranger in Immunity, Inflammation, and Cancer. Exp Mol Med (2021) 53(5):823–34. doi: 10.1038/s12276-021-00630-x
42. Wang RX, Yu CR, Dambuza IM, Mahdi RM, Dolinska MB, Sergeev YV, et al. Interleukin-35 Induces Regulatory B Cells That Suppress Autoimmune Disease. Nat Med (2014) 20(6):633–41. doi: 10.1038/nm.3554
43. Aparicio-Siegmund S, Moll JM, Lokau J, Grusdat M, Schroder J, Plohn S, et al. Recombinant P35 From Bacteria can Form Interleukin (IL-)12, But Not IL-35. PloS One (2014) 9(9):e107990. doi: 10.1371/journal.pone.0107990
44. Nishino R, Takano A, Oshita H, Ishikawa N, Akiyama H, Ito H, et al. Identification of Epstein-Barr Virus-Induced Gene 3 as a Novel Serum and Tissue Biomarker and a Therapeutic Target for Lung Cancer. Clin Cancer Res (2011) 17(19):6272–86. doi: 10.1158/1078-0432.CCR-11-0060
45. Wirtz S, Becker C, Fantini MC, Nieuwenhuis EE, Tubbe I, Galle PR, et al. EBV-Induced Gene 3 Transcription is Induced by TLR Signaling in Primary Dendritic Cells via NF-Kappa B Activation. J Immunol (2005) 174(5):2814–24. doi: 10.4049/jimmunol.174.5.2814
46. Heidemann J, Ruther C, Kebschull M, Domschke W, Bruwer M, Koch S, et al. Expression of IL-12-Related Molecules in Human Intestinal Microvascular Endothelial Cells is Regulated by TLR3. Am J Physiol Gastrointest Liver Physiol (2007) 293(6):G1315–24. doi: 10.1152/ajpgi.00142.2007
47. Scholz GM, Heath JE, Walsh KA, Reynolds EC. MEK-ERK Signaling Diametrically Controls the Stimulation of IL-23p19 and EBI3 Expression in Epithelial Cells by IL-36gamma. Immunol Cell Biol (2018) 96(6):646–55. doi: 10.1111/imcb.12029
48. Cassatella MA, Gardiman E, Arruda-Silva F, Bianchetto-Aguilera F, Gasperini S, Bugatti M, et al. Human Neutrophils Activated by TLR8 Agonists, With or Without IFNgamma, Synthesize and Release EBI3, But Not IL-12, IL-27, IL-35, or IL-39. J Leukoc Biol (2020) 108(5):1515–26. doi: 10.1002/JLB.3MA0520-054R
49. Kimura D, Miyakoda M, Kimura K, Honma K, Hara H, Yoshida H, et al. Interleukin-27-Producing CD4(+) T Cells Regulate Protective Immunity During Malaria Parasite Infection. Immunity (2016) 44(3):672–82. doi: 10.1016/j.immuni.2016.02.011
50. Deng JH, Chen HY, Huang C, Yan JM, Yin Z, Zhang XL, et al. Accumulation of EBI3 Induced by Virulent Mycobacterium Tuberculosis Inhibits Apoptosis in Murine Macrophages. Pathog Dis (2019) 77(1):ftz007. doi: 10.1093/femspd/ftz007
51. Abbas W, Kumar A, Herbein G. The eEF1A Proteins: At the Crossroads of Oncogenesis, Apoptosis, and Viral Infections. Front Oncol (2015) 5:75. doi: 10.3389/fonc.2015.00075
52. Iranshahi N, Assar S, Amiri SM, Zafari P, Fekri A, Taghadosi M. Decreased Gene Expression of Epstein-Barr Virus-Induced Gene 3 (EBI-3) may Contribute to the Pathogenesis of Rheumatoid Arthritis. Immunol Invest (2019) 48(4):367–77. doi: 10.1080/08820139.2018.1549066
53. Wetzel A, Scholtka B, Gerecke C, Kleuser B. Epigenetic Histone Modulation Contributes to Improvements in Inflammatory Bowel Disease via EBI3. Cell Mol Life Sci (2020) 77(23):5017–30. doi: 10.1007/s00018-020-03451-9
54. Kudo H, Wang Z, Jinnin M, Nakayama W, Inoue K, Honda N, et al. EBI3 Downregulation Contributes to Type I Collagen Overexpression in Scleroderma Skin. J Immunol (2015) 195(8):3565–73. doi: 10.4049/jimmunol.1402362
55. Medina TS, Oliveira GG, Silva MC, David BA, Silva GK, Fonseca DM, et al. Ebi3 Prevents Trypanosoma Cruzi-Induced Myocarditis by Dampening IFN-Gamma-Driven Inflammation. Front Immunol (2017) 8:1213. doi: 10.3389/fimmu.2017.01213
56. Gonin J, Larousserie F, Bastard C, Picquenot JM, Couturier J, Radford-Weiss I, et al. Epstein-Barr Virus-Induced Gene 3 (EBI3): A Novel Diagnosis Marker in Burkitt Lymphoma and Diffuse Large B-Cell Lymphoma. PloS One (2011) 6(9):e24617. doi: 10.1371/journal.pone.0024617PONE-D-11-01586
57. Liang Y, Chen Q, Du W, Chen C, Li F, Yang J, et al. Epstein-Barr Virus-Induced Gene 3 (EBI3) Blocking Leads to Induce Antitumor Cytotoxic T Lymphocyte Response and Suppress Tumor Growth in Colorectal Cancer by Bidirectional Reciprocal-Regulation STAT3 Signaling Pathway. Mediators Inflamm (2016) 2016:3214105. doi: 10.1155/2016/3214105
58. Jiang J, Liu X. Upregulated EBI3 Correlates With Poor Outcome and Tumor Progression in Breast Cancer. Oncol Res Treat (2018) 41(3):111–5. doi: 10.1159/000484935
59. Wiersma VR, Michalak M, Abdullah TM, Bremer E, Eggleton P. Mechanisms of Translocation of ER Chaperones to the Cell Surface and Immunomodulatory Roles in Cancer and Autoimmunity. Front Oncol (2015) 5:7. doi: 10.3389/fonc.2015.00007
60. Yoshimoto T, Chiba Y, Furusawa J, Xu M, Tsunoda R, Higuchi K, et al. Potential Clinical Application of Interleukin-27 as an Antitumor Agent. Cancer Sci (2015) 106(9):1103–10. doi: 10.1111/cas.12731
61. Collison LW, Chaturvedi V, Henderson AL, Giacomin PR, Guy C, Bankoti J, et al. IL-35-Mediated Induction of a Potent Regulatory T Cell Population. Nat Immunol (2010) 11(12):1093–101. doi: 10.1038/ni.1952
62. Wang Z, Liu JQ, Liu Z, Shen R, Zhang G, Xu J, et al. Tumor-Derived IL-35 Promotes Tumor Growth by Enhancing Myeloid Cell Accumulation and Angiogenesis. J Immunol (2013) 190(5):2415–23. doi: 10.4049/jimmunol.1202535
63. Turnis ME, Sawant DV, Szymczak-Workman AL, Andrews LP, Delgoffe GM, Yano H, et al. Interleukin-35 Limits Anti-Tumor Immunity. Immunity (2016) 44(2):316–29. doi: 10.1016/j.immuni.2016.01.013
64. Fan YG, Zhai JM, Wang W, Feng B, Yao GL, An YH, et al. IL-35 Over-Expression is Associated With Genesis of Gastric Cancer. Asian Pac J Cancer Prev (2015) 16(7):2845–9. doi: 10.7314/apjcp.2015.16.7.2845
65. Jin P, Ren H, Sun W, Xin W, Zhang H, Hao J. Circulating IL-35 in Pancreatic Ductal Adenocarcinoma Patients. Hum Immunol (2014) 75(1):29–33. doi: 10.1016/j.humimm.2013.09.018
66. Hou YM, Dong J, Liu MY, Yu S. Expression of Epstein-Barr Virus-Induced Gene 3 in Cervical Cancer: Association With Clinicopathological Parameters and Prognosis. Oncol Lett (2016) 11(1):330–4. doi: 10.3892/ol.2015.3849
67. Zhang Y, Sun H, Wu H, Tan Q, Xiang K. Interleukin 35 is an Independent Prognostic Factor and a Therapeutic Target for Nasopharyngeal Carcinoma. Contemp Oncol (Pozn) (2015) 19(2):120–4. doi: 10.5114/wo.2014.44754
68. Schmidt-Arras D, Muller M, Stevanovic M, Horn S, Schutt A, Bergmann J, et al. Oncogenic Deletion Mutants of Gp130 Signal From Intracellular Compartments. J Cell Sci (2014) 127(Pt 2):341–53. doi: 10.1242/jcs.130294
69. Jalah R, Rosati M, Ganneru B, Pilkington GR, Valentin A, Kulkarni V, et al. The P40 Subunit of Interleukin (IL)-12 Promotes Stabilization and Export of the P35 Subunit: Implications for Improved IL-12 Cytokine Production. J Biol Chem (2013) 288(9):6763–76. doi: 10.1074/jbc.M112.436675
70. Jones LL, Chaturvedi V, Uyttenhove C, Van Snick J, Vignali DA. Distinct Subunit Pairing Criteria Within the Heterodimeric IL-12 Cytokine Family. Mol Immunol (2012) 51(2):234–44. doi: 10.1016/j.molimm.2012.03.025
71. Nangalia J, Massie CE, Baxter EJ, Nice FL, Gundem G, Wedge DC, et al. Somatic CALR Mutations in Myeloproliferative Neoplasms With Nonmutated JAK2. N Engl J Med (2013) 369(25):2391–405. doi: 10.1056/NEJMoa1312542
72. Klampfl T, Gisslinger H, Harutyunyan AS, Nivarthi H, Rumi E, Milosevic JD, et al. Somatic Mutations of Calreticulin in Myeloproliferative Neoplasms. N Engl J Med (2013) 369(25):2379–90. doi: 10.1056/NEJMoa1311347
73. How J, Hobbs GS, Mullally A. Mutant Calreticulin in Myeloproliferative Neoplasms. Blood (2019) 134(25):2242–8. doi: 10.1182/blood.2019000622
Keywords: EBI3, calnexin, chaperone, heterodimer, tumor growth
Citation: Watanabe A, Mizoguchi I, Hasegawa H, Katahira Y, Inoue S, Sakamoto E, Furusaka Y, Sekine A, Miyakawa S, Murakami F, Xu M, Yoneto T and Yoshimoto T (2021) A Chaperone-Like Role for EBI3 in Collaboration With Calnexin Under Inflammatory Conditions. Front. Immunol. 12:757669. doi: 10.3389/fimmu.2021.757669
Received: 12 August 2021; Accepted: 02 September 2021;
Published: 17 September 2021.
Edited by:
Tomozumi Imamichi, Frederick National Laboratory for Cancer Research (NIH), United StatesReviewed by:
Mahdi Taghadosi, Kermanshah University of Medical Sciences, IranKaveh Abdi, National Institute of Allergy and Infectious Diseases, National Institutes of Health (NIH), United States
Copyright © 2021 Watanabe, Mizoguchi, Hasegawa, Katahira, Inoue, Sakamoto, Furusaka, Sekine, Miyakawa, Murakami, Xu, Yoneto and Yoshimoto. This is an open-access article distributed under the terms of the Creative Commons Attribution License (CC BY). The use, distribution or reproduction in other forums is permitted, provided the original author(s) and the copyright owner(s) are credited and that the original publication in this journal is cited, in accordance with accepted academic practice. No use, distribution or reproduction is permitted which does not comply with these terms.
*Correspondence: Takayuki Yoshimoto, eW9zaGltb3RAdG9reW8tbWVkLmFjLmpw