- Division of Infectious Diseases, Chongqing Public Health Medical Center, Chongqing, China
Despite the benefits achieved by the widespread availability of modern antiretroviral therapy (ART), HIV RNA integration into the host cell genome is responsible for the creation of latent HIV reservoirs, and represents a significant impediment to completely eliminating HIV infection in a patient via modern ART alone. Several methods to measure HIV reservoir size exist; however, simpler, cheaper, and faster tools are required in the quest for total HIV cure. Over the past few years, measurement of HIV-specific antibodies has evolved into a promising option for measuring HIV reservoir size, as they can be measured via simple, well-known techniques such as the western blot and enzyme-linked immunosorbent assay (ELISA). In this article, we re-visit the dynamic evolution of HIV-1-specific antibodies and the factors that may influence their levels in the circulation of HIV-positive individuals. Then, we describe the currently-known relationship between HIV-1-specific antibodies and HIV reservoir size based on study of data from contemporary literature published during the past 5 years. We conclude by highlighting current trends, and discussing the individual HIV-specific antibody that is likely to be the most reliable antibody for potential future utilization for quantification of HIV reservoir size.
Introduction
Today, the human immunodeficiency virus (HIV) infection remains a major public health burden despite four decades of massive monetary and intellectual investment into HIV research globally (1). Since 2010, the proportion of HIV-1 infected individuals receiving ART has increased. For example, in 2020, 27.4 million of the 37.6 million people living with HIV (PLWH) are reported to be on ART, which is more than triple the number of patients on ART recorded in 2010 (7.8 million). It is estimated that since 2001, the use of modern ART has prevented 16.2 million deaths. The preceding report also indicates that the number of AIDS-related deaths has fallen by 43% between 2010 and 2020 (2). Indeed, modern antiretroviral therapy (ART) efficiently suppresses HIV-1 replication by targeting key mechanisms in its life cycle (3), which in turn (i) reduces HIV viral RNA load to below detectable levels (4, 5), (ii) increases the number of CD4+ T-cells (6, 7), (iii) reduces the incidence of AIDS-related diseases and/or deaths (6, 8), and (iv) effectively prevents the transmission of HIV to uninfected people (9). However, despite the critically important impact of modern ART regimens, ART does not eliminate the virus from infected patients (10).
The insertion of a DNA copy of the HIV viral genome into the host cell chromosome is a critically important step of the life cycle of HIV. HIV subsequently hijacks the host cellular machinery to its advantage through viral protein and RNA production. An infected cell harboring HIV DNA remains infected for the life of that cell. Thus, ART only suppresses viral replication, and the cessation of ART use in the absence of HIV DNA elimination will inevitably be followed by viral rebound (11). HIV DNA integration results in the establishment of latent infection, leading to the creation of a latent HIV reservoir. Defined as quiescent host cells carrying an integrated copy of the viral genome that does not express HIV viral transcripts or proteins, the latent reservoir is the major component of the HIV reservoir; a minor component of the latent reservoir being the HIV active reservoir (12). The greatest challenge to HIV eradication is the persistence of latent HIV provirus in reservoir cells. Several mechanisms, described in past publications (13–15), are responsible for this outcome. In addition, researchers have observed that individuals harboring low HIV reservoir levels are able to control HIV replication in the absence of ART (16, 17). Therefore, the study of tools that are able to accurately measure HIV reservoir size is crucial for the monitoring of remission and/or prognosis of HIV-infected individuals (10).
Currently, several approaches to quantify the HIV reservoir exist, despite four inherent challenges. Firstly, the majority of proviruses persisting in people living with HIV taking ART harbor mutations and/or deletions that render these particular proviruses defective, and unable to replicate. Secondly, not all proviruses are able to produce viable virions after activation. Thirdly, the frequency of latently-infected cells is inherently very low. Finally, a large proportion of the HIV reservoir is present in tissues that cannot be accurately sampled using currently used specimen-collection approaches (18–21). The current methods used to quantify the HIV reservoir can be classified into four major groups, based on specific aspects of the HIV provirus and its functionality. Thus, there are currently assays (i) measuring levels of replication-competent virus or intact HIV genomes [the quantitative viral outgrowth assay (QVOA) (22, 23) and several PCR-based assays (24, 25)], (ii) measuring levels of translationally competent virus [the enhanced digital p24 single-molecule assay (SIMOA) (26–29), for example], (iii) measuring levels of transcriptionally competent virus [the qPCR and droplet digital PCR assays (30, 31)], and (iv) measuring total and integrated levels of HIV DNA (PCR quantification of total, integrated, and episomal HIV DNA) (12). To measure HIV reservoirs, researchers use biopsies from gut-associated lymphoid tissue (GALT) or lymph nodes (LN), or peripheral blood mononuclear cells (PBMCs) extracted either from 10 ml of blood, or via leukapheresis. It must be noted that none of these collected specimens, taken individually, provide a comprehensive picture of reservoir dynamics in patients taking ART. Moreover, commonly used methods regarded as “standard” (12), such as QVOA and its derivatives, are expensive, labor-intensive, require a large numbers of cells (~20-50 million CD4+ T-cells), require biosafety-containment, and have a tendency to underestimate the size of the replication-competent viral reservoir (18, 32). This highlights the urgent need for simpler, less expensive, and time-saving methods to reliably measure HIV reservoir size.
Of late, the estimation of HIV reservoir size using biomarkers, especially those biomarkers emanating from immune responses, has been recommended as a potentially realistic solution to the current difficulties related to accurate estimation of reservoir size. Thus, the utilization of antibodies for HIV-1 DNA profiling is likely to be simpler, less expensive, and results may be obtained more rapidly. Indeed, quantitative detection of HIV-1-specific antibodies is commonly used in-clinic, mainly via western blot (33, 34), enzyme-linked immunosorbent assay (ELISA) (35), laser induced plasma spectroscopy (LIPS) (36, 37), and microsphere-based array assay (38). Moreover, antibodies as biomarkers could possibly prove to be a significant means to overcome the four inherent challenges referred to in the preceding paragraph, which are encountered by existing approaches to quantify the HIV reservoir. Antibody production is not, to our knowledge, influenced by specific aspects of the HIV provirus and its functionality. Therefore, HIV-1-specific antibodies that are able to accurately predict HIV-1 reservoir size could represent an important and simpler option when considering curative HIV strategies (39, 40). Herein, we review the dynamic evolution of HIV-specific antibodies and the factors influencing their production. We also discuss the possibility of using HIV-1-specific antibodies to estimate HIV reservoir size based on investigational observations published within the past five years.
The Evolution of HIV-1-Specific Antibodies
HIV-1-Specific Antibodies Before ART Initiation
An infant born to an HIV-positive mother acquires maternal antibodies via the placenta. The infant is HIV seropositive due to the passive transfer of maternal immunoglobulin (Ig) G antibodies, which occurs during the last trimester of pregnancy (41, 42). Some reports suggest that these IgG antibodies may persist for up to 18 months (43, 44). At 4–6 months of age, infants start producing their own IgG antibodies (against a limited number of HIV antigens) (45), characterized at first by production of anti-glycoprotein (gp) 160, and subsequently followed by anti-gp120 and anti-gp41 (46) (see Figure 1A).
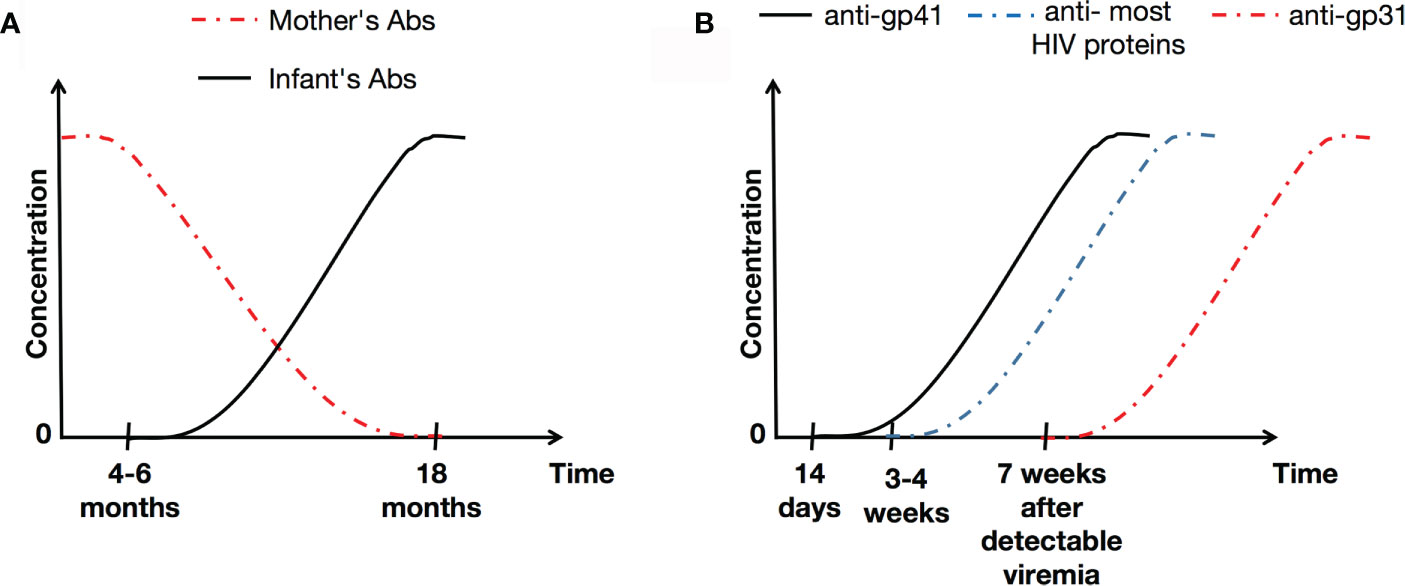
Figure 1 Illustration of HIV-1-specific antibody concentrations over the time in infants (A) and the general population (B). Abs, antibodies.
In adults, it has been reported that the initial specific antibody response to HIV is detected 14 days after infection, and targets gp41 (47). In the absence of appropriate treatment, levels of gp41 remain stable over the time, and correlates with viral load (48). Generally, antibodies to most HIV proteins are detectable within 3-4 weeks of infection, although anti-p31 takes much longer to be produced (at around 7 weeks after detectable viremia) (49) (Figure 1B). It is known that in untreated HIV-positive adults, levels of antibodies to HIV are stable, and correlate positively with viral loads (48).
HIV-1-Specific Antibodies After ART, and Duration on ART
During HIV infection, the administration of ART induces HIV-specific antibody levels to decline (48). Actually, antibodies of all types (with the exception of anti-p17) may be cleared in infants who initiate ART by 3 months of age as a direct consequence of the rapid control of HIV-1 replication (50). It has also been observed that anti-gp41 levels decrease with duration of ART (Pearson r=-026, p<0.0001) (51). In addition, it has been demonstrated (52) that infants on ART with effective viral suppression (<400 RNA copies/mL) have (i) low but stable levels of antibodies against HIV gp41 and gp160, (ii) reduced concentrations of antibodies to p17, p24, and reverse transcriptase (RT), and (iii) low or undetectable concentrations of anti-gp31. Those children on ART between 1 and 5 years old and with viral suppression (<400 RNA copies/mL) have higher levels of the preceding six antibodies than that seen in infants (52). These differences in antibody levels are likely to reflect the timing of ART on the one hand, but also the timing of the generation of HIV-specific-antibodies on the other, as suggested some time ago by Tomaras et al. (49). In other words, timing of HIV infection and persistence of antigen exposure may impact on the quantum of the HIV-1 specific antibody response in HIV-infected individuals (53).
Many past studies have described a corresponding decrease in anti-HIV antibody level with duration on ART, in participants with either primary or chronic infection as well as in perinatally infected children (48, 54–57). It thus appears that early ART initiation may interrupt HIV antigenic stimulation. In other words, ART may have the ability to sustain an HIV-specific antibody response when initiated early (58). Recently, Keating et al. (48), observed that (i) declining antibody levels during ART reflect lower levels of antigen production and/or viral replication and (ii) the higher levels of HIV-1-specific antibodies observed in individuals on suppressive therapy are associated with later initiation of ART, as well as cell-associated DNA and RNA levels. The suppressive activity of ART on the HIV life cycle thus seems to provoke a quasi-null HIV replication rate. However, anti-HIV antibody persistence during this period is probably stimulated by low levels of ongoing viral replication, or the production of translationally competent HIV-1 transcripts (59). To further illustrate this point, Brice et al., have shown that a significant proportion of virologically suppressed HIV-positive children stop producing antibodies, or have progressively lost HIV-specific antibodies, secondary to ART initiation before 2 years of age (56). McManus et al., have also demonstrated that ART limits HIV-specific antibody levels in the plasma of HIV-positive children. Thus, their antibody profiles become similar to those in HIV-uninfected children born to HIV-infected mothers (50). In the light of these details, it would be interesting to study the initial development of HIV-specific antibodies in early-treated patients, to determine precisely in what way the primary adaptative responses are affected, influenced, and restricted by ART. The decreases in specific antibody levels are, logically, likely to reflect a corresponding reduction in circulating antigenic HIV viral particles (56).
The nature of HIV infection in children and in adults is inherently too dissimilar to be able to compare them under identical parameters. Therefore, Figure 2. only presents the evolution of HIV-specific antibodies in adults who initiate and comply with ART treatment without interruption.
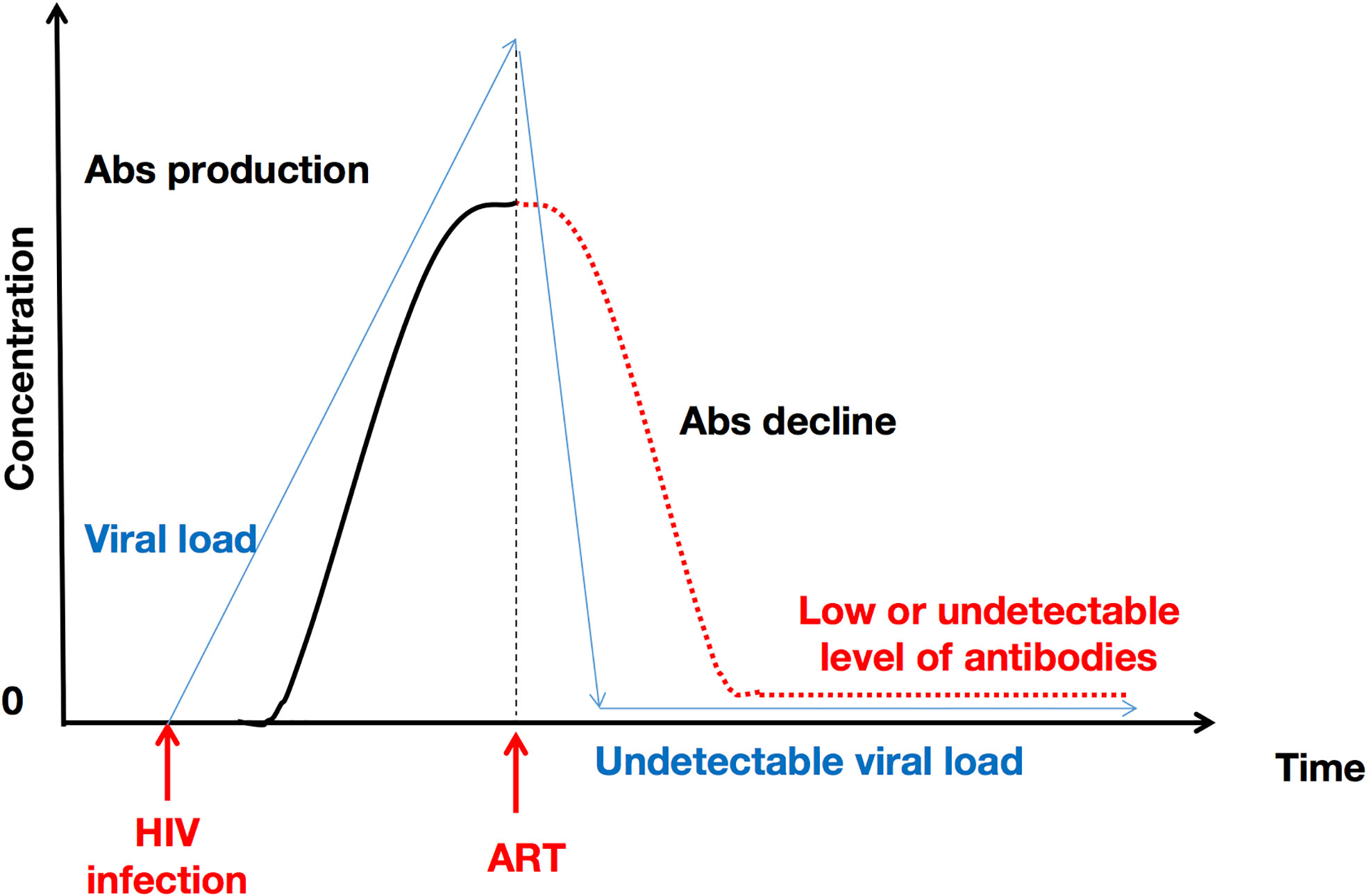
Figure 2 ART initiation and uninterrupted administration over time induces a decline in HIV antibody concentration. This represents the particular case of adults. ART provokes a decrease in viral RNA copies, which in turn explains the subsequent decrease in HIV-1-specific antibody levels. Abs, antibodies.
Non-ART or Viral Load Factors Influencing HIV-1-Specific Antibody Production
Several factors may influence the production of HIV-1-specific antibodies. A comprehensive understanding of HIV antibody trends during HIV infection and the factors that may influence antibody expression is critical if one is to consider using antibody levels as a tool to reflect HIV-1 DNA levels.
Age
In infancy, primary HIV infection is characterized by a high-level plasma viral load which decreases relatively little in the initial phases of the infection (60, 61). In adults, primary HIV infection is characterized by an initial viral load peak, followed rapidly by a 100–1,000-fold decrease in viral RNA copies, to reach a stable ‘set point’ within weeks (62). This age-associated difference noted in HIV viral kinetics may be explained (i) by the larger CD4+ T-cell compartment in infants and children (63–66), and (ii) a relatively less-robust innate immunity and/or a less-effective adaptative immune response in infants and children (66).
Age can influence the ontogeny of HIV-specific antibodies due to the differences observed in individual immune systems. As such, considering the antibodies that are passively transferred from the mother to the infant, researchers have found that baseline age correlates inversely with maternal antibody levels (50). Conversely, it is recognized that infants start producing their own antibodies after approximately 4 months (46). Compared with adults, some scientists suggest that the apparent delay in antibody production in infants, despite high levels of HIV replication, is due to their paucity of CD4+ T-cell-related influence (50). In other words, infant’s and children’s immune systems are characterized by an abundance of naive CD4+ T-cells, coupled with a limited capacity to generate antigen-specific memory cells (67).
Biological Sex
Gender disparities in HIV pathophysiology remain a major area of concern as conflicting reports of more robust immune activation and antiviral responses in HIV-infected women have been published (as reviewed by Scully) (68). At the same time, it is worth noting that when using an identical assay (EIA-RI or recent infection enzyme immunoassay), at least two separate research teams have reported higher antibody levels in ART-treated women, compared with men (51, 54). Some studies have suggested that women are more likely to be categorized as spontaneous controllers of HIV (69, 70). It has been postulated that this gender-dependent HIV profile may be due to the activity of estrogen and estrogen receptor-1. Indeed, Das et al., have observed that estrogen and estrogen receptor-1 inhibit HIV transcription in vitro (71); however, further studies are required to elucidate the determinants of the “spontaneous controller” status of women.
HIV Subtype
The role of HIV subtype in HIV-1-specific antibody production remains to be clarified. It is known that subtype AE is more transcriptionally active and produces a lower degree of latency than subtype B, due, in part, to the GA-binding protein (GABP) site present in the subtype AE long terminal repeat (LTR) (72). Furthermore, researchers have shown that subtype C is less functional and more sensitive to apolipoprotein B mRNA editing enzyme catalytic subunit 3G (APOBEC3G)-mediated viral inhibition, compared to subtype B or subtype AG (73). The subtype C viral genome was observed to be hypermutated with APOBEC3G, with a limited ability of subtype C to infect cells, and thus to replicate. Subtype D has been shown to be more inclined to induce latency than other subtypes in an in vitro model of latency (74). Bachmann et al., found that decay of the latent reservoir was significantly faster in non-B individuals, compared to subtype B-infected individuals (75). Furthermore, it was found that subtype C-infected individuals had lower total levels of HIV-1 DNA than subtype B-infected individuals. In the light of such details, it is legitimate to hypothesize that a less virulent strain (that is more inclined to latency) allows the immune system to develop a stronger humoral antibody response than virulent stains which are highly active with respect to viral replication. This hypothesis is purely speculative, and further targeted investigation could help to determine the antibody profiles in individuals infected by different HIV subtypes. For now, it has only been established that more virulent strains induce a greater depletion of CD4+ T-cells (76) and poorer immune recovery (77).
Co-Infections
Despite being on ART, HIV-infected individuals are known to exhibit an underlying chronic inflammatory state. HIV-1 related-immunodeficiency increases susceptibility to several pathogenic viruses, including cytomegalovirus, the hepatitis viruses, and other viruses, which also contribute to the chronic inflammation (78). Currently, despite some exceptions (79), most studies suggest that HIV reservoir size is shaped in a complex manner by the prevailing inflammatory environment. Simply stated, comorbid infection further enhances existing inflammation, which provokes further immune activation, thus resulting in higher reservoir sizes (19, 80, 81). Similarly, several researchers have found that HIV/simian immunodeficiency virus (SIV) DNA is enriched in cells that express immune activation (82–85). It has also been shown that inflammation of lymphoid tissue is mainly responsible for the immune dysfunction and the reduced immunity to HIV-1. Thus, the question arises as to what the implication of co-infections would be on the dynamic evolution or production of HIV-1-specific antibodies. Perhaps, antibody production in co-infected individuals may be reduced, slower, or dysfunctional. Further investigation into this area of inquiry is warranted.
Allogeneic Stem Cell Transplantation (Allo-HSCT)
Many studies have reported a diminution or clearance of HIV-specific antibodies after allo-HSCT. Indeed, a clinical trial by Salgado et al., including 6 participants with viral suppression resulting from uninterrupted administration of cART, and who survived more than 2 years after allo-HSCT (with CCR5 wild-type cells), reported that all the patients had lost their anti-gp18 antibody, while some displayed decreasing p31 antibody levels (2 participants), or absent anti-p55 and anti-p24 antibodies (2 participants). These observations brought the authors to suppose that a longer interval after allo-HSCT seemed to be associated with greater antibody clearance among patients receiving cART (86). Similarly, Koelsch et al. (87), and Henrich et al. (88), present evidence that implies that allo-HSCT may modify the serological evolution of HIV-positive individuals. In the study by Koelsch et al., four cases were considered in which 3 patients (designated as patients A, B, and C) highlight the substantial changes to the HIV immune response in patients undergoing allo-HSCT. Briefly, the antibody concentrations in patient A were very low at the first measured time point (4 years post-transplant), but increased after the patient experienced viral rebound at the second measurement (31 weeks later). In patient B, antibody concentrations were measured twice, once at 3 years and once at 7.5 months post transplantation. At both time points, antibody concentrations were extremely low. In patient C, a progressive diminution of antibody concentrations was reported between months 6 and 12 following transplantation (87). Henrich et al., have also demonstrated that antibodies levels declined (in two patients, A and B) during the period of virologic suppression post-HSCT (>1000 days). They also noted that an antiretroviral treatment interruption (ATI) was followed by a viral rebound associated with antibody level increase. The well-known cases of the “Berlin” (89) and “London” (90, 91) patients are also worth mentioning. Officially known as functionally cured of HIV infection, both received allogeneic bone marrow transplants from a naturally-mutated CCR5 gene (CCR5 delta 32) donor. Reports suggest that the HIV antibody levels of these patients were readily detectable. Although their HIV antibody levels have continued to decline over time, the levels remained above the cut-off levels for HIV negative individuals (89–91).
Relationship Between HIV-1-Specific Antibodies and HIV-1 DNA
Several published articles suggest that measurement of HIV-specific antibodies may provide a reliable, cost-effective, and highly reproducible tool to estimate HIV reservoir size in HIV-positive individuals on ART. This concept was conceived after researchers observed lower residual blood cell-associated HIV-1 DNA levels (58, 92–96) and low or absent HIV-1-specific antibody levels (66, 97, 98) in the first few months of life in perinatally infected infants, when viral suppression occurs following ART initiation. However, there remains no consensus regarding this point, as some research teams failed to observe any significant association between these two variables (55, 57). Although the individual methods of investigation vary (Table 1), the observations related to this issue are worthy of intellectual contemplation.
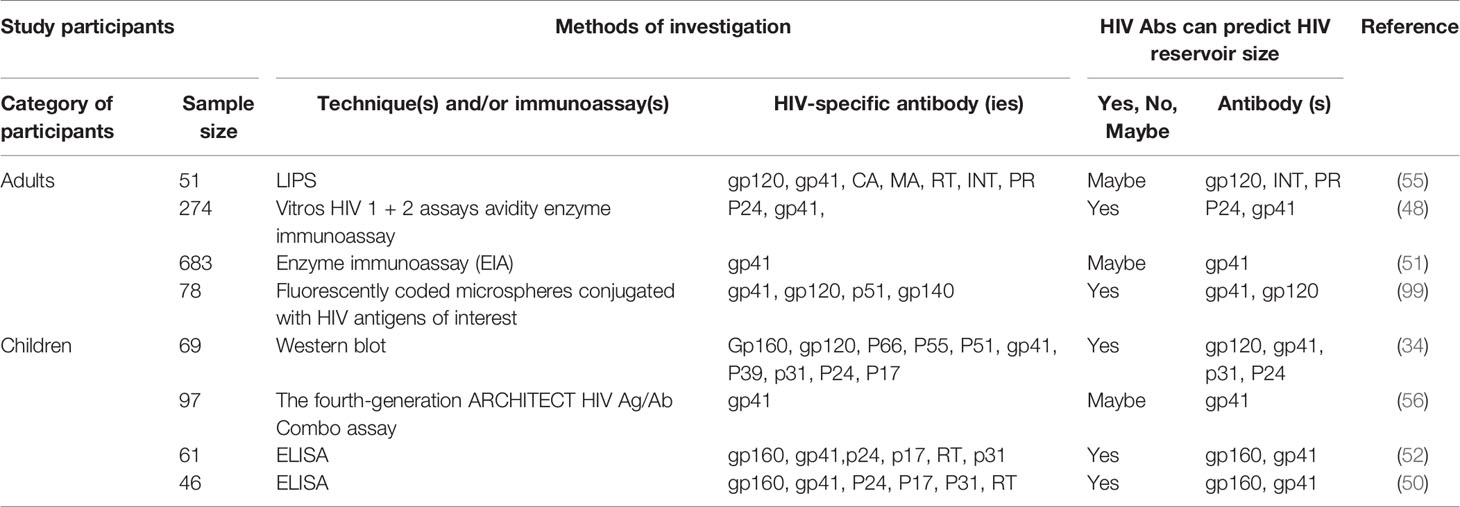
Table 1 Summary of published articles (2016-2021) investigating HIV-1-specific antibody capacity to estimate HIV-1 reservoir size.
In 2016, Lee et al., found (i) a significant positive correlation between anti-HIV integrase and HIV RNA in gut-associated lymphoid tissue and (ii) a negative correlation between anti-HIV matrix and integrated HIV DNA in CD4+ T-cells. Of note, a 0.35-fold decrease in the anti-HIV matrix count was associated with a 2-fold DNA increase. However, the application of more stringent statistical analysis (the Bonferroni-adjustment) has revealed that these associations were not actually statistically significant. Notwithstanding this, Lee and his colleagues have suggested that antibodies against enzymes involved in HIV replication might be of better use than those against HIV structural protein. The level of antibodies against HIV p24, matrix, and gp 41 has been demonstrated to likely reflect the level of HIV replication in an individual (100).
In 2017, Brice et al., observed that the low activity of anti-gp41 was associated with low levels of anti-HIV antibodies and a younger age of ART initiation. The study population comprised of vertically-infected HIV-positive children (median age of 3.3 years, receiving ART for a median 5.4 years) with virological suppression. An interesting finding was that the overall analysis revealed that no correlation existed between anti-gp41 antibodies and HIV DNA. However, children who initiated ART before the age of 2 years had a very low HIV reservoir, in addition to having low antibody levels and activity (56).
In late 2018–early 2019, a French team reported that anti-gp41 levels reflect HIV cellular reservoir size in long-term ART-treated adults (median age 41 years old, treated for a median of 10.7 years, and 77.5% were men). Actually, Delagreverie et al., have demonstrated that a lower titer of anti-gp41 correlates with male gender, longer viral suppression, and lower HIV-1 DNA burden (anti-gp41 and cell associated HIV-1 DNA quantification). Of note, (i) anti-gp41 levels does not correlate with the overall duration since HIV diagnosis, (ii) lower titers of anti-gp41 were observed in participants with non-detectable HIV-1 DNA compared to those with quantifiable HIV-1 DNA, and (iii) anti-gp41 levels were higher in women than in men (51). The results of Delagreverie et al’s., study thus concur with that of Keating et al., from 2017 (in which the study population was recently-infected individuals), despite different approaches in terms of analysis (EIA-RI versus gp41 limiting antigen avidity assay). Indeed, Keating et al., have observed a correlation between anti-gp41 levels and PBMC-associated HIV-1 DNA for up to 6 years of viral suppression (48). Interestingly, Delagreverie et al., and Brice et al., used the EIA-RI approach to estimate anti-gp41 levels; however, lower overall numbers were observed by Brice and his colleagues. This may be explained by the current use of dried serum spot samples and/or the differences in immune response between children and adults, as the study of Brice et al., only included infants. This suggests that antibody titers may be artifactually underestimated if an appropriate sampling method is not used for sample collection.
In 2019, scientists from the University of Massachusetts Medical School have demonstrated that quantitative HIV antibodies correlate with cell-associated DNA levels in children on ART (50). In contrast to the investigation of Brice et al., who did not include children who started ART before 5 months of life, MacManus and her colleagues (50) have included children stratified by age at ART initiation, as early therapy (<3 months of life), and late therapy (3 months to 2 years of age). Furthermore, MacManus et al., have included HIV-negative children born to HIV-positive mothers as controls, and instead of measuring only HIV anti-gp41, they measured antibodies against HIV-1 (i) envelope (gp160, gp41), (ii) gag (capsid, p24, matrix, p17), (iii) reverse transcriptase (p66, p55), and (iv) integrase. Overall, they found that the diminution of the measured HIV-specific antibodies (other than anti-p17) in the early therapy group (and virologic response group) was similar to that of the control group. Most importantly, they found that after one year of age, the levels of antibodies such as anti-gp160 and anti-gp41 were directly associated with circulating HIV DNA levels (R2 = 0.37; p<0.05). Logistic regression has estimated that each unit increase in log anti-gp160 was associated with a 6-fold increase in HIV DNA level. Additionally, they also observed that after one year of age, levels of anti-p31 and anti-p17 are directly associated with plasma HIV RNA levels (R2 = 0.59; p<0.05) (50).
In late 2019–early 2020, McManus, in a related further publication (52), indicated that quantitative anti-gp41 and anti-gp160 levels may serve as rapid and inexpensive tools to screen for low HIV DNA levels in PBMC’s in children with viral suppression. Indeed, two approaches, namely, the receiver operator curve (ROC) approach and the ensemble learning approach, have identified anti-gp41 and anti-gp160 as important predictors of HIV-1 DNA when HIV DNA counts are estimated at less than 10 or less than 100 copies per million PBMC. In 2020, another research team characterized humoral biomarkers of reservoir size in controllers and chronic progressors. Indeed, Das et al., have provided insightful information with regards to whether antibody profiles tracked consistently with estimates of reservoir size across elite controllers, viremic controllers, and untreated chronically infected progressive patients not on ART. After demonstrating that antibodies can indeed be used to discriminate between individuals with higher and lower HIV DNA levels, they have further identified specific antibodies (IgG-gp41, r=0.489, p<0.001 and IgG2-gp41, r=-0.399, p=0.006) which differentiated these controllers by reservoir size. Das et al., further investigated whether humoral biomarkers of reservoir size can estimate the reservoir size in ART-treated subjects, and they observed that IgG-gp41 (r=0.597, p=0.011) and IgG3-gp120 (r=0.135, p=0.029) HIV-specific antibodies correlate with the size of the HIV reservoir in these subjects (99).
In the aforementioned studies, the participants were under ART and had undetectable HIV viral loads, were from the same patient category (either children or adults), and had no co-infection to influence HIV-1-specific antibody production, and to also ensure the comparability of the results. Overall, it appears that these studies favor utilizing anti-gp41 to determine HIV-1 reservoir size. However, only studies conducted in children show a global trend in favor of using HIV-specific antibodies, notably anti-gp41, to monitor HIV reservoir size. In contrast, in studies in adults, approximately half remained skeptical of the use of HIV-specific antibodies to monitor HIV reservoir size. Knowing that the nature and manifestation of HIV infection is vastly different in children compared to adults, it would be wise to wait for additional investigational evidence to make firm conclusions regarding the possibility of using HIV-specific antibodies to monitor HIV DNA in adults. Moreover, ELISA and enzyme immunoassays seem to be the most commonly used methods to investigate the relationship between HIV-1-specific antibodies and HIV-1 DNA in children and adults, respectively. This highlights the presence of additional challenges potentially affecting the relationship between HIV-1-specific antibodies and HIV-1 DNA.
Additional Challenges
Based on contemporary literature, we note that the analysis of the relationships between HIV-1-specific antibodies and HIV-1 DNA may be influenced by the specific methods of investigation. Indeed, it is critical to state which particular method should be used to measure anti-gp41; the specific method used could, otherwise, induce difficulties and/or differences in terms of result interpretation. This is a matter of particular concern in the case of the Berlin patient, for example, where the antibody level measured at four post-transplantation time points with the undiluted HIV-1/2 VITROS assay was relatively stable (~75-80 signal/cut-off ratio) at all four post-transplantation time points considered. However, the same objective using different methods, such as the detuned HIV-1 VITROS assay (from ~0.38-0.39 signal/cut-off ratio to ~0.30 signal/cut-off ratio at the end of the study), or the limiting antigen avidity assays (from ~0.31 normalized optical density to ~0.27 normalized optical density at the end of the study), indicated lower HIV antibody levels, which tended to decrease over the time (89). Similar observations were made by investigators studying the London patient (90). Therefore, further investigation aimed at establishing consensus with respect to the best methods to be used for such tasks, is warranted. Similarly, the best and most appropriate laboratory methods for use in adults and children should be also investigated.
Considering ART-treated patients undergoing HIV curative strategies and in need of HIV DNA estimation via HIV specific antibody quantification, viral rebounds subsequent to ART discontinuation or the emergence of treatment resistant strains should be carefully monitored. The results by Koelsch et al. (87), and Henrich et al. (88), show that viral rebound even after allo-HSCT results in an antibody level increase on the one hand, but may also favor the augmentation of HIV reservoir size on the other. If HIV reservoir size reaches more than 100 copies per million PBMC, the currently understood relationship between HIV-specific antibodies and HIV DNA, as outlined by McManus et al. (52), will no longer be applicable.
Finally, we believe that sample collection and preservation before laboratory studies are conducted, should be particularly taken heed of. As suggested by comparing the study by Brice et al. (56), to the investigation by Delagreverie et al. (51),, dried serum spot samples do not seem appropriate, as they tend to produce relatively lower titers of HIV-specific antibody results. A standardized process for sample collection is, therefore, necessary. In addition, sample size represents an interesting marker of precision. Conclusions based on a large sample are known to provide a much more realistic result compared to those from a limited sample. From the studies cited in this article, the screening of more than five hundred individuals (352 adults and 176 children) seems to favor the notion that gp41 may be a realistic option for HIV-1 DNA quantification in HIV-infected individuals. However, further investigation using larger sample sizes are necessary to generalize results to the large population of people living with HIV globally [officially, 37.6 million in 2020 (2)].
Conclusion
Contemporary literature demonstrates a trend in favor of using HIV-1-specific antibodies to predict HIV-1 reservoir size [5 of 8 papers (62.5%), Table 1], particularly in HIV-positive children [3 of 4 papers (75%), Table 1]. With respect to adults, it is currently not possible to make a similar conclusion, as we failed to find a trend in the data in favor of such an approach. Interestingly, anti-gp41 (one of the first antibodies to be produced in infants and adults) is likely to be the most promising antibody candidate for use as a potential marker for this task. Although HIV-1-specific antibody use seems to be a simpler, less expensive, and less restrictive laboratory tool, their absolute levels are sensitive to several determinants, including viral load, ART use, biological sex, age, HIV subtype, and presence of co-infections. In light of this, we believe that further investigation is warranted in order to provide clearer and definitive recommendations regarding estimation of HIV-reservoir size, particularly in adults.
Author Contributions
SZ and FS wrote the first draft of the manuscript. YC provided critical revision of the manuscript. All authors contributed to the article and approved the submitted version.
Funding
This work was supported by Chongqing Public Health Medical Center Youth Innovation Program (2019QNKYXM04) and the Joint Medical Research Projects of Chongqing Municipal Health Commission and Chongqing Municipal Science and Technology Bureau (2020GDRC004, 2020MSXM097, 2020FYYX066).
Conflict of Interest
The authors declare that the research was conducted in the absence of any commercial or financial relationships that could be construed as a potential conflict of interest.
Publisher’s Note
All claims expressed in this article are solely those of the authors and do not necessarily represent those of their affiliated organizations, or those of the publisher, the editors and the reviewers. Any product that may be evaluated in this article, or claim that may be made by its manufacturer, is not guaranteed or endorsed by the publisher.
References
1. Zaongo SD, Xia H, Ma P. HIV Gene Therapy Strategies and Safety: What do We Know From the Recent Publications? AIDS Rev (2020) 23(3):195–202. doi: 10.24875/AIDSRev.20000008
2. UNAIDS. AIDSinfo (2020). Available at: http://aidsinfo.unaids.org/ (Accessed September 27, 2021).
3. Spach DH. Antiretroviral Medications and Initial Therapy. (2021). Available at: https://www.hiv.uw.edu/go/antiretroviral-therapy/general-information/core-concept/all (Accessed September 27, 2021).
4. Volberding PA, Deeks SG. Antiretroviral Therapy and Management of HIV Infection. Lancet (2010) 376(9734):49–62. doi: 10.1016/S0140-6736(10)60676-9
5. Kalichman SC, Cherry C, Amaral CM, Swetzes C, Eaton L, Macy R, et al. Adherence to Antiretroviral Therapy and HIV Transmission Risks: Implications for Test-and-Treat Approaches to HIV Prevention. AIDS Patient Care STDS (2010) 24(5):271–7. doi: 10.1089/apc.2009.0309
6. Wilson EM, Sereti I. Immune Restoration After Antiretroviral Therapy: The Pitfalls of Hasty or Incomplete Repairs. Immunol Rev (2013) 254(1):343–54. doi: 10.1111/imr.12064
7. Autran B, Carcelain G, Li TS, Blanc C, Mathez D, Tubiana R, et al. Positive Effects of Combined Antiretroviral Therapy on CD4+ T Cell Homeostasis and Function in Advanced HIV Disease. Science (1997) 277(5322):112–6. doi: 10.1126/science.277.5322.112
8. Michaels SH, Clark R, Kissinger P. Declining Morbidity and Mortality Among Patients With Advanced Human Immunodeficiency Virus Infection. N Eng J Med (1998) 339(6):405–6. doi: 10.1056/nejm199808063390612
9. Le Guillou A, Buchbinder S, Scott H, Liu A, Havlir D, Scheer S, et al. Population Impact and Efficiency of Improvements to HIV PrEP Under Conditions of High ART Coverage Among San Francisco Men Who Have Sex With Men. J Acquir Immune Defic Syndr (2021) 88(4):340–47. doi: 10.1097/qai.0000000000002781
10. Mbonye U, Karn J. The Molecular Basis for Human Immunodeficiency Virus Latency. Annu Rev Virol (2017) 4(1):261–85. doi: 10.1146/annurev-virology-101416-041646
11. Ruelas DS, Greene WC. An Integrated Overview of HIV-1 Latency. Cell (2013) 155(3):519–29. doi: 10.1016/j.cell.2013.09.044
12. Abdel-Mohsen M, Richman D, Siliciano RF, Nussenzweig MC, Howell BJ, Martinez-Picado J, et al. Recommendations for Measuring HIV Reservoir Size in Cure-Directed Clinical Trials. Nat Med (2020) 26(9):1339–50. doi: 10.1038/s41591-020-1022-1
13. Taube R, Peterlin M. Lost in Transcription: Molecular Mechanisms That Control HIV Latency. Viruses (2013) 5(3):902–27. doi: 10.3390/v5030902
14. Bosque A, Nilson KA, Macedo AB, Spivak AM, Archin NM, Van Wagoner RM, et al. Benzotriazoles Reactivate Latent HIV-1 Through Inactivation of STAT5 SUMOylation. Cell Rep (2017) 18(5):1324–34. doi: 10.1016/j.celrep.2017.01.022
15. Bosque A, Planelles V. Induction of HIV-1 Latency and Reactivation in Primary Memory CD4+ T Cells. Blood (2009) 113(1):58–65. doi: 10.1182/blood-2008-07-168393
16. Jiang C, Lian X, Gao C, Sun X, Einkauf KB, Chevalier JM, et al. Distinct Viral Reservoirs in Individuals With Spontaneous Control of HIV-1. Nature (2020) 585(7824):261–7. doi: 10.1038/s41586-020-2651-8
17. Noel N, Peña R, David A, Avettand-Fenoel V, Erkizia I, Jimenez E, et al. Long-Term Spontaneous Control of HIV-1 Is Related to Low Frequency of Infected Cells and Inefficient Viral Reactivation. J Virol (2016) 90(13):6148–58. doi: 10.1128/jvi.00419-16
18. Ho YC, Shan L, Hosmane NN, Wang J, Laskey SB, Rosenbloom DI, et al. Replication-Competent Noninduced Proviruses in the Latent Reservoir Increase Barrier to HIV-1 Cure. Cell (2013) 155(3):540–51. doi: 10.1016/j.cell.2013.09.020
19. Banga R, Procopio FA, Noto A, Pollakis G, Cavassini M, Ohmiti K, et al. PD-1(+) and Follicular Helper T Cells Are Responsible for Persistent HIV-1 Transcription in Treated Aviremic Individuals. Nat Med (2016) 22(7):754–61. doi: 10.1038/nm.4113
20. Yukl SA, Gianella S, Sinclair E, Epling L, Li Q, Duan L, et al. Differences in HIV Burden and Immune Activation Within the Gut of HIV-Positive Patients Receiving Suppressive Antiretroviral Therapy. J Infect Dis (2010) 202(10):1553–61. doi: 10.1086/656722
21. Estes JD, Kityo C, Ssali F, Swainson L, Makamdop KN, Del Prete GQ, et al. Defining Total-Body AIDS-Virus Burden With Implications for Curative Strategies. Nat Med (2017) 23(11):1271–6. doi: 10.1038/nm.4411
22. Siliciano JD, Kajdas J, Finzi D, Quinn TC, Chadwick K, Margolick JB, et al. Long-Term Follow-Up Studies Confirm the Stability of the Latent Reservoir for HIV-1 in Resting CD4+ T Cells. Nat Med (2003) 9(6):727–8. doi: 10.1038/nm880
23. Finzi D, Hermankova M, Pierson T, Carruth LM, Buck C, Chaisson RE, et al. Identification of a Reservoir for HIV-1 in Patients on Highly Active Antiretroviral Therapy. Science (1997) 278(5341):1295–300. doi: 10.1126/science.278.5341.1295
24. Bruner KM, Wang Z, Simonetti FR, Bender AM, Kwon KJ, Sengupta S, et al. A Quantitative Approach for Measuring the Reservoir of Latent HIV-1 Proviruses. Nature (2019) 566(7742):120–5. doi: 10.1038/s41586-019-0898-8
25. Gaebler C, Lorenzi JCC, Oliveira TY, Nogueira L, Ramos V, Lu CL, et al. Combination of Quadruplex qPCR and Next-Generation Sequencing for Qualitative and Quantitative Analysis of the HIV-1 Latent Reservoir. J Exp Med (2019) 216(10):2253–64. doi: 10.1084/jem.20190896
26. Wu G, Swanson M, Talla A, Graham D, Strizki J, Gorman D, et al. HDAC Inhibition Induces HIV-1 Protein and Enables Immune-Based Clearance Following Latency Reversal. JCI Insight (2017) 2(16):e92901. doi: 10.1172/jci.insight.92901
27. Passaes CPB, Bruel T, Decalf J, David A, Angin M, Monceaux V, et al. Ultrasensitive HIV-1 P24 Assay Detects Single Infected Cells and Differences in Reservoir Induction by Latency Reversal Agents. J Virol (2017) 91(6):e02296-16. doi: 10.1128/jvi.02296-16
28. Cai Y, Abdel-Mohsen M, Tomescu C, Xue F, Wu G, Howell BJ, et al. BCL6 Inhibitor-Mediated Downregulation of Phosphorylated SAMHD1 and T Cell Activation Are Associated With Decreased HIV Infection and Reactivation. J Virol (2019) 93(2). doi: 10.1128/jvi.01073-18
29. Fidler S, Stöhr W, Pace M, Dorrell L, Lever A, Pett S, et al. Antiretroviral Therapy Alone Versus Antiretroviral Therapy With a Kick and Kill Approach, on Measures of the HIV Reservoir in Participants With Recent HIV Infection (the RIVER Trial): A Phase 2, Randomised Trial. Lancet (2020) 395(10227):888–98. doi: 10.1016/s0140-6736(19)32990-3
30. Procopio FA, Fromentin R, Kulpa DA, Brehm JH, Bebin AG, Strain MC, et al. A Novel Assay to Measure the Magnitude of the Inducible Viral Reservoir in HIV-Infected Individuals. EBioMedicine (2015) 2(8):874–83. doi: 10.1016/j.ebiom.2015.06.019
31. Hong F, Jacobs JL, Aga E, Cillo AR, Fyne E, Koontz DL, et al. Associations Between HIV-1 DNA Copy Number, Proviral Transcriptional Activity, and Plasma Viremia in Individuals Off or on Suppressive Antiretroviral Therapy. Virology (2018) 521:51–7. doi: 10.1016/j.virol.2018.05.018
32. Hosmane NN, Kwon KJ, Bruner KM, Capoferri AA, Beg S, Rosenbloom DI, et al. Proliferation of Latently Infected CD4(+) T Cells Carrying Replication-Competent HIV-1: Potential Role in Latent Reservoir Dynamics. J Exp Med (2017) 214(4):959–72. doi: 10.1084/jem.20170193
33. Jackson JB, Parsons JS, Nichols LS, Knoble N, Kennedy S, Piwowar EM. Detection of Human Immunodeficiency Virus Type 1 (HIV-1) Antibody by Western Blotting and HIV-1 DNA by PCR in Patients With AIDS. J Clin Microbiol (1997) 35(5):1118–21. doi: 10.1128/jcm.35.5.1118-1121.1997
34. Rocca S, Zangari P, Cotugno N, De Rossi A, Ferns B, Petricone D, et al. Human Immunodeficiency Virus (HIV)-Antibody Repertoire Estimates Reservoir Size and Time of Antiretroviral Therapy Initiation in Virally Suppressed Perinatally HIV-Infected Children. J Pediatr Infect Dis Soc (2019) 8(5):433–8. doi: 10.1093/jpids/piy080
35. Mkhize NN, Durgiah R, Ashley V, Archary D, Garrett NJ, Karim QA, et al. Broadly Neutralizing Antibody Specificities Detected in the Genital Tract of HIV-1 Infected Women. AIDS (2016) 30(7):1005–14. doi: 10.1097/QAD.0000000000001038
36. Burbelo PD, Bren KE, Ching KH, Gogineni ES, Kottilil S, Cohen JI, et al. LIPS Arrays for Simultaneous Detection of Antibodies Against Partial and Whole Proteomes of HCV, HIV and EBV. Mol Biosyst (2011) 7(5):1453–62. doi: 10.1039/c0mb00342e
37. Zhang W, Morshed MM, Noyan K, Russom A, Sönnerborg A, Neogi U. Quantitative Humoral Profiling of the HIV-1 Proteome in Elite Controllers and Patients With Very Long-Term Efficient Antiretroviral Therapy. Sci Rep (2017) 7(1):666. doi: 10.1038/s41598-017-00759-8
38. Kong W, Li Y, Cheng S, Yan C, An S, Dong Z, et al. Luminex xMAP Combined With Western Blot Improves HIV Diagnostic Sensitivity. JJ Virol Methods (2016) 227:1–5. doi: 10.1016/j.jviromet.2015.10.007
39. Zaongo SD, Liu Y, Harypursat V, Song F, Xia H, Ma P, et al. P-Selectin Glycoprotein Ligand 1: A Potential HIV-1 Therapeutic Target. Front Immunol (2021) 12:710121. doi: 10.3389/fimmu.2021.710121
40. Zaongo SD, Ma P, Song FZ, Chen YK. Selective Elimination of Host Cells Harboring Replication-Competent Human Immunodeficiency Virus Reservoirs: A Promising Therapeutic Strategy for HIV Cure. Chin Med J (2021). doi: 10.1097/cm9.0000000000001797
41. Niewiesk S. Maternal Antibodies: Clinical Significance, Mechanism of Interference With Immune Responses, and Possible Vaccination Strategies. Front Immunol (2014) 5:446. doi: 10.3389/fimmu.2014.00446
42. Palmeira P, Quinello C, Silveira-Lessa AL, Zago CA, Carneiro-Sampaio M. IgG Placental Transfer in Healthy and Pathological Pregnancies. Clin Dev Immunol (2012) 2012:985646. doi: 10.1155/2012/985646
43. Chantry CJ, Cooper ER, Pelton SI, Zorilla C, Hillyer GV, Diaz C. Seroreversion in Human Immunodeficiency Virus-Exposed But Uninfected Infants. Pediatr Infect Dis J (1995) 14(5):382–7. doi: 10.1097/00006454-199505000-00009
44. Pahwa S. Human Immunodeficiency Virus Infection in Children: Nature of Immunodeficiency, Clinical Spectrum and Management. Pediatr Infect Dis J (1988) 7(5 Suppl):S61–71. doi: 10.1097/00006454-198805001-00013
45. Baroncelli S, Galluzzo CM, Liotta G, Andreotti M, Orlando S, Ciccacci F, et al. Dynamics of Immunoglobulin G Subclasses During the First Two Years of Life in Malawian Infants Born to HIV-Positive Mothers. BMC Pediatr (2020) 20(1):181. doi: 10.1186/s12887-020-02091-z
46. Pollack H, Zhan MX, Ilmet-Moore T, Ajuang-Simbiri K, Krasinski K, Borkowsky W. Ontogeny of Anti-Human Immunodeficiency Virus (HIV) Antibody Production in HIV-1-Infected Infants. Proc Natl Acad Sci USA (1993) 90(6):2340–4. doi: 10.1073/pnas.90.6.2340
47. Tomaras GD, Yates NL, Liu P, Qin L, Fouda GG, Chavez LL, et al. Initial B-Cell Responses to Transmitted Human Immunodeficiency Virus Type 1: Virion-Binding Immunoglobulin M (IgM) and IgG Antibodies Followed by Plasma Anti-Gp41 Antibodies With Ineffective Control of Initial Viremia. J Virol (2008) 82(24):12449–63. doi: 10.1128/jvi.01708-08
48. Keating SM, Pilcher CD, Jain V, Lebedeva M, Hampton D, Abdel-Mohsen M, et al. HIV Antibody Level as a Marker of HIV Persistence and Low-Level Viral Replication. J Infect Dis (2017) 216(1):72–81. doi: 10.1093/infdis/jix225
49. Tomaras GD, Haynes BF. HIV-1-Specific Antibody Responses During Acute and Chronic HIV-1 Infection. Curr Opin HIV AIDS (2009) 4(5):373–9. doi: 10.1097/COH.0b013e32832f00c0
50. McManus M, Henderson J, Gautam A, Brody R, Weiss ER, Persaud D, et al. Quantitative Human Immunodeficiency Virus (HIV)-1 Antibodies Correlate With Plasma HIV-1 RNA and Cell-Associated DNA Levels in Children on Antiretroviral Therapy. Clin Infect Dis (2019) 68(10):1725–32. doi: 10.1093/cid/ciy753
51. Delagreverie HM, Grude M, Lambert-Niclot S, Nere ML, Jadand C, Leport C, et al. Anti-Gp41 Antibody Levels Reflect HIV Viral Suppression and Cellular Reservoir in Long-Term Antiretroviral-Treated Trial Participants. J Antimicrob Chemother (2019) 74(5):1389–94. doi: 10.1093/jac/dkz004
52. McManus M, Karalius B, Patel K, Persaud D, Luzuriaga K. Quantitative HIV-1-Specific Antibodies as Predictors of Peripheral Blood Cell-Associated HIV-1 DNA Concentrations. AIDS (2020) 34(8):1117–26. doi: 10.1097/qad.0000000000002525
53. Overbaugh J, Morris L. The Antibody Response Against HIV-1. Cold Spring Harb Perspect Med (2012) 2(1):a007039. doi: 10.1101/cshperspect.a007039
54. Chaillon A, Le Vu S, Brunet S, Gras G, Bastides F, Bernard L, et al. Decreased Specificity of an Assay for Recent Infection in HIV-1-Infected Patients on Highly Active Antiretroviral Treatment: Implications for Incidence Estimates. Clin Vaccine Immunol (2012) 19(8):1248–53. doi: 10.1128/cvi.00120-12
55. Lee SA, Bacchetti P, Chomont N, Fromentin R, Lewin SR, O’Doherty U, et al. Anti-HIV Antibody Responses and the HIV Reservoir Size During Antiretroviral Therapy. PloS One (2016) 11(8):e0160192. doi: 10.1371/journal.pone.0160192
56. Brice J, Sylla M, Sayon S, Telly F, Bocar-Fofana D, Murphy R, et al. Qualitative and Quantitative HIV Antibodies and Viral Reservoir Size Characterization in Vertically Infected Children With Virological Suppression. J Antimicrob Chemother (2017) 72(4):1147–51. doi: 10.1093/jac/dkw537
57. Burbelo PD, Bayat A, Rhodes CS, Hoh R, Martin JN, Fromentin R, et al. HIV Antibody Characterization as a Method to Quantify Reservoir Size During Curative Interventions. J Infect Dis (2014) 209(10):1613–7. doi: 10.1093/infdis/jit667
58. Ananworanich J, Puthanakit T, Suntarattiwong P, Chokephaibulkit K, Kerr SJ, Fromentin R, et al. Reduced Markers of HIV Persistence and Restricted HIV-Specific Immune Responses After Early Antiretroviral Therapy in Children. AIDS (2014) 28(7):1015–20. doi: 10.1097/qad.0000000000000178
59. Imamichi H, Dewar RL, Adelsberger JW, Rehm CA, O’Doherty U, Paxinos EE, et al. Defective HIV-1 Proviruses Produce Novel Protein-Coding RNA Species in HIV-Infected Patients on Combination Antiretroviral Therapy. Proc Natl Acad Sci USA (2016) 113(31):8783–8. doi: 10.1073/pnas.1609057113
60. Bitnun A, Ransy DG, Brophy J, Kakkar F, Hawkes M, Samson L, et al. Clinical Correlates of Human Immunodeficiency Virus-1 (HIV-1) DNA and Inducible HIV-1 RNA Reservoirs in Peripheral Blood in Children With Perinatally Acquired HIV-1 Infection With Sustained Virologic Suppression for at Least 5 Years. Clin Infect Dis (2020) 70(5):859–66. doi: 10.1093/cid/ciz251
61. Garcia-Broncano P, Maddali S, Einkauf KB, Jiang C, Gao C, Chevalier J, et al. Early Antiretroviral Therapy in Neonates With HIV-1 Infection Restricts Viral Reservoir Size and Induces a Distinct Innate Immune Profile. Sci Transl Med (2019) 11(520):eaax7350. doi: 10.1126/scitranslmed.aax7350
62. Martinez DR, Permar SR, Fouda GG. Contrasting Adult and Infant Immune Responses to HIV Infection and Vaccination. Clin Vaccine Immunol (2016) 23(2):84–94. doi: 10.1128/cvi.00565-15
63. Krogstad P, Uittenbogaart CH, Dickover R, Bryson YJ, Plaeger S, Garfinkel A. Primary HIV Infection of Infants: The Effects of Somatic Growth on Lymphocyte and Virus Dynamics. Clin Immunol (1999) 92(1):25–33. doi: 10.1006/clim.1999.4728
64. Comans-Bitter WM, de Groot R, van den Beemd R, Neijens HJ, Hop WC, Groeneveld K, et al. Immunophenotyping of Blood Lymphocytes in Childhood. Reference Values for Lymphocyte Subpopulations. J Pediatr (1997) 130(3):388–93. doi: 10.1016/s0022-3476(97)70200-2
65. Erkeller-Yuksel FM, Deneys V, Yuksel B, Hannet I, Hulstaert F, Hamilton C, et al. Age-Related Changes in Human Blood Lymphocyte Subpopulations. J Pediatr (1992) 120(2 Pt 1):216–22. doi: 10.1016/s0022-3476(05)80430-5
66. Luzuriaga K, McManus M, Catalina M, Mayack S, Sharkey M, Stevenson M, et al. Early Therapy of Vertical Human Immunodeficiency Virus Type 1 (HIV-1) Infection: Control of Viral Replication and Absence of Persistent HIV-1-Specific Immune Responses. J Virol (2000) 74(15):6984–91. doi: 10.1128/jvi.74.15.6984-6991.2000
67. Cohn LB, Chomont N, Deeks SG. The Biology of the HIV-1 Latent Reservoir and Implications for Cure Strategies. Cell Host Microbe (2020) 27(4):519–30. doi: 10.1016/j.chom.2020.03.014
68. Scully EP. Sex Differences in HIV Infection. Curr HIV/AIDS Rep (2018) 15(2):136–46. doi: 10.1007/s11904-018-0383-2
69. Crowell TA, Gebo KA, Blankson JN, Korthuis PT, Yehia BR, Rutstein RM, et al. Hospitalization Rates and Reasons Among HIV Elite Controllers and Persons With Medically Controlled HIV Infection. J Infect Dis (2015) 211(11):1692–702. doi: 10.1093/infdis/jiu809
70. Madec Y, Boufassa F, Porter K, Meyer L. Spontaneous Control of Viral Load and CD4 Cell Count Progression Among HIV-1 Seroconverters. AIDS (2005) 19(17):2001–7. doi: 10.1097/01.aids.0000194134.28135.cd
71. Das B, Dobrowolski C, Luttge B, Valadkhan S, Chomont N, Johnston R, et al. Estrogen Receptor-1 Is a Key Regulator of HIV-1 Latency That Imparts Gender-Specific Restrictions on the Latent Reservoir. Proc Natl Acad Sci USA (2018) 115(33):E7795–804. doi: 10.1073/pnas.1803468115
72. Sarabia I, Bosque A. HIV-1 Latency and Latency Reversal: Does Subtype Matter? Viruses (2019) 11(12):1104. doi: 10.3390/v11121104
73. Lisovsky I, Schader SM, Sloan RD, Oliveira M, Coutsinos D, Bernard NF, et al. HIV-1 Subtype Variability in Vif Derived From Molecular Clones Affects APOBEC3G-Mediated Host Restriction. Intervirology (2013) 56(4):258–64. doi: 10.1159/000348513
74. Dahabieh MS, Ooms M, Simon V, Sadowski I. A Doubly Fluorescent HIV-1 Reporter Shows That the Majority of Integrated HIV-1 Is Latent Shortly After Infection. J Virol (2013) 87(8):4716–27. doi: 10.1128/jvi.03478-12
75. Bachmann N, von Siebenthal C, Vongrad V, Turk T, Neumann K, Beerenwinkel N, et al. Determinants of HIV-1 Reservoir Size and Long-Term Dynamics During Suppressive ART. Nat Commun (2019) 10(1):3193. doi: 10.1038/s41467-019-10884-9
76. Li X, Xue Y, Cheng H, Lin Y, Zhou L, Ning Z, et al. HIV-1 Genetic Diversity and Its Impact on Baseline Cd4+T Cells and Viral Loads Among Recently Infected Men Who Have Sex With Men in Shanghai, China. PloS One (2015) 10(6):e0129559. doi: 10.1371/journal.pone.0129559
77. Ge Z, Feng Y, Li K, Lv B, Zaongo SD, Sun J, et al. CRF01_AE and CRF01_AE Cluster 4 Are Associated With Poor Immune Recovery in Chinese Patients Under Combination Antiretroviral Therapy. Clin Infect Dis (2021) 72(10):1799–809. doi: 10.1093/cid/ciaa380
78. Hunt PW, Martin JN, Sinclair E, Epling L, Teague J, Jacobson MA, et al. Valganciclovir Reduces T Cell Activation in HIV-Infected Individuals With Incomplete CD4+ T Cell Recovery on Antiretroviral Therapy. J Infect Dis (2011) 203(10):1474–83. doi: 10.1093/infdis/jir060
79. Gandhi RT, McMahon DK, Bosch RJ, Lalama CM, Cyktor JC, Macatangay BJ, et al. Levels of HIV-1 Persistence on Antiretroviral Therapy Are Not Associated With Markers of Inflammation or Activation. PloS Pathog (2017) 13(4):e1006285. doi: 10.1371/journal.ppat.1006285
80. Chomont N, El-Far M, Ancuta P, Trautmann L, Procopio FA, Yassine-Diab B, et al. HIV Reservoir Size and Persistence Are Driven by T Cell Survival and Homeostatic Proliferation. Nat Med (2009) 15(8):893–900. doi: 10.1038/nm.1972
81. Fromentin R, DaFonseca S, Costiniuk CT, El-Far M, Procopio FA, Hecht FM, et al. PD-1 Blockade Potentiates HIV Latency Reversal Ex Vivo in CD4(+) T Cells From ART-Suppressed Individuals. Nat Commun (2019) 10(1):814. doi: 10.1038/s41467-019-08798-7
82. Lee E, Bacchetti P, Milush J, Shao W, Boritz E, Douek D, et al. Memory CD4 + T-Cells Expressing HLA-DR Contribute to HIV Persistence During Prolonged Antiretroviral Therapy. Front Microbiol (2019) 10:2214. doi: 10.3389/fmicb.2019.02214
83. McGary CS, Deleage C, Harper J, Micci L, Ribeiro SP, Paganini S, et al. CTLA-4(+)PD-1(-) Memory CD4(+) T Cells Critically Contribute to Viral Persistence in Antiretroviral Therapy-Suppressed, SIV-Infected Rhesus Macaques. Immunity (2017) 47(4):776–88.e5. doi: 10.1016/j.immuni.2017.09.018
84. Pardons M, Baxter AE, Massanella M, Pagliuzza A, Fromentin R, Dufour C, et al. Single-Cell Characterization and Quantification of Translation-Competent Viral Reservoirs in Treated and Untreated HIV Infection. PloS Pathog (2019) 15(2):e1007619. doi: 10.1371/journal.ppat.1007619
85. Thornhill JP, Pace M, Martin GE, Hoare J, Peake S, Herrera C, et al. CD32 Expressing Doublets in HIV-Infected Gut-Associated Lymphoid Tissue Are Associated With a T Follicular Helper Cell Phenotype. Mucosal Immunol (2019) 12(5):1212–9. doi: 10.1038/s41385-019-0180-2
86. Salgado M, Kwon M, Gálvez C, Badiola J, Nijhuis M, Bandera A, et al. Mechanisms That Contribute to a Profound Reduction of the HIV-1 Reservoir After Allogeneic Stem Cell Transplant. Ann Intern Med (2018) 169(10):674–83. doi: 10.7326/m18-0759
87. Koelsch KK, Rasmussen TA, Hey-Nguyen WJ, Pearson C, Xu Y, Bailey M, et al. Impact of Allogeneic Hematopoietic Stem Cell Transplantation on the HIV Reservoir and Immune Response in 3 HIV-Infected Individuals. J Acquir Immune Defic Syndr (2017) 75(3):328–37. doi: 10.1097/qai.0000000000001381
88. Henrich TJ, Hanhauser E, Marty FM, Sirignano MN, Keating S, Lee TH, et al. Antiretroviral-Free HIV-1 Remission and Viral Rebound After Allogeneic Stem Cell Transplantation: Report of 2 Cases. Ann Intern Med (2014) 161(5):319–27. doi: 10.7326/m14-1027
89. Yukl SA, Boritz E, Busch M, Bentsen C, Chun TW, Douek D, et al. Challenges in Detecting HIV Persistence During Potentially Curative Interventions: A Study of the Berlin Patient. PloS Pathog (2013) 9(5):e1003347. doi: 10.1371/journal.ppat.1003347
90. Gupta RK, Abdul-Jawad S, McCoy LE, Mok HP, Peppa D, Salgado M, et al. HIV-1 Remission Following CCR5Δ32/Δ32 Haematopoietic Stem-Cell Transplantation. Nature (2019) 568(7751):244–8. doi: 10.1038/s41586-019-1027-4
91. Gupta RK, Peppa D, Hill AL, Gálvez C, Salgado M, Pace M, et al. Evidence for HIV-1 Cure After CCR5Δ32/Δ32 Allogeneic Haemopoietic Stem-Cell Transplantation 30 Months Post Analytical Treatment Interruption: A Case Report. Lancet HIV (2020) 7(5):e340–7. doi: 10.1016/s2352-3018(20)30069-2
92. Martínez-Bonet M, Puertas MC, Fortuny C, Ouchi D, Mellado MJ, Rojo P, et al. Establishment and Replenishment of the Viral Reservoir in Perinatally HIV-1-Infected Children Initiating Very Early Antiretroviral Therapy. Clin Infect Dis (2015) 61(7):1169–78. doi: 10.1093/cid/civ456
93. McManus M, Mick E, Hudson R, Mofenson LM, Sullivan JL, Somasundaran M, et al. Early Combination Antiretroviral Therapy Limits Exposure to HIV-1 Replication and Cell-Associated HIV-1 DNA Levels in Infants. PloS One (2016) 11(4):e0154391. doi: 10.1371/journal.pone.0154391
94. Uprety P, Patel K, Karalius B, Ziemniak C, Chen YH, Brummel SS, et al. Human Immunodeficiency Virus Type 1 DNA Decay Dynamics With Early, Long-Term Virologic Control of Perinatal Infection. Clin Infect Dis (2017) 64(11):1471–8. doi: 10.1093/cid/cix192
95. Kuhn L, Paximadis M, Da Costa Dias B, Loubser S, Strehlau R, Patel F, et al. Age at Antiretroviral Therapy Initiation and Cell-Associated HIV-1 DNA Levels in HIV-1-Infected Children. PloS One (2018) 13(4):e0195514. doi: 10.1371/journal.pone.0195514
96. Tagarro A, Chan M, Zangari P, Ferns B, Foster C, De Rossi A, et al. Early and Highly Suppressive Antiretroviral Therapy Are Main Factors Associated With Low Viral Reservoir in European Perinatally HIV-Infected Children. J Acquir Immune Defic Syndr (2018) 79(2):269–76. doi: 10.1097/qai.0000000000001789
97. Kuhn L, Schramm DB, Shiau S, Strehlau R, Pinillos F, Technau K, et al. Young Age at Start of Antiretroviral Therapy and Negative HIV Antibody Results in HIV-Infected Children When Suppressed. AIDS (2015) 29(9):1053–60. doi: 10.1097/qad.0000000000000677
98. Payne H, Mkhize N, Otwombe K, Lewis J, Panchia R, Callard R, et al. Reactivity of Routine HIV Antibody Tests in Children Who Initiated Antiretroviral Therapy in Early Infancy as Part of the Children With HIV Early Antiretroviral Therapy (CHER) Trial: A Retrospective Analysis. Lancet Infect Dis (2015) 15(7):803–9. doi: 10.1016/s1473-3099(15)00087-0
99. Das J, Devadhasan A, Linde C, Broge T, Sassic J, Mangano M, et al. Mining for Humoral Correlates of HIV Control and Latent Reservoir Size. PloS Pathog (2020) 16(10):e1008868. doi: 10.1371/journal.ppat.1008868
Keywords: HIV-1-specific antibody, marker, HIV-1 DNA, HIV reservoir, Level
Citation: Zaongo SD, Sun F and Chen Y (2021) Are HIV-1-Specific Antibody Levels Potentially Useful Laboratory Markers to Estimate HIV Reservoir Size? A Review. Front. Immunol. 12:786341. doi: 10.3389/fimmu.2021.786341
Received: 30 September 2021; Accepted: 28 October 2021;
Published: 11 November 2021.
Edited by:
Alberto Bosque, George Washington University, United StatesReviewed by:
Maria Salgado, IrsiCaixa, SpainRebecca M. Lynch, George Washington University, United States
Copyright © 2021 Zaongo, Sun and Chen. This is an open-access article distributed under the terms of the Creative Commons Attribution License (CC BY). The use, distribution or reproduction in other forums is permitted, provided the original author(s) and the copyright owner(s) are credited and that the original publication in this journal is cited, in accordance with accepted academic practice. No use, distribution or reproduction is permitted which does not comply with these terms.
*Correspondence: Yaokai Chen, eWFva2FpY2hlbkBob3RtYWlsLmNvbQ==
†These authors have contributed equally to this work