- 1Division of Rheumatology, Department of Medicine, Solna and Center for Molecular Medicine, Karolinska Institutet, Stockholm, Sweden
- 2Rheumatology, Karolinska University Hospital, Stockholm, Sweden
- 3Dermato-Venereology, Karolinska University Hospital, Stockholm, Sweden
Anti-neutrophil cytoplasmic antibody (ANCA)- associated vasculitis (AAV) is a group of systemic autoimmune diseases characterized by inflammation of small- and medium-sized vessels. The three main types of AAV are granulomatosis with polyangiitis (GPA), microscopic polyangiitis (MPA) and eosinophilic granulomatosis with polyangiitis (EGPA). A growing number of studies focus on natural killer (NK) cells in AAV. NK cells are innate lymphoid cells with important roles in anti-viral and anti-tumor defense, but their roles in the pathogenesis of autoimmunity is less well established. In this review, we will present a summary of what is known about the number, phenotype and function of NK cells in patients with AAV. We review the literature on NK cells in the circulation of AAV patients, studies on tissue resident NK cells and how the treatment affects NK cells.
Highlights
● The phenotype of circulating NK cells is altered in AAV patients and indicates activation.
● NK cells are found in the inflamed organs but in low numbers.
● The possible role of NK cells in the pathogenesis of AAV remains to be determined.
● NK are influenced by, and potentially contribute, to the effect of medications used in AAV.
Introduction
Vasculitis is a group of diseases characterized by inflammation of blood vessels. Anti-neutrophil cytoplasmic antibody (ANCA)-associated vasculitis (AAV) is an autoimmune disease with inflammation affecting small- and medium-sized vessels associated with the presence of ANCA (1, 2). AAV can be further subdivided in three diagnostic entities, granulomatosis with polyangiitis (GPA), microscopic polyangiitis (MPA) and eosinophilic granulomatosis with polyangiitis (EGPA) with the presence of ANCA autoantibodies targeting the myeloperoxidase (MPO) or the neutrophil cytoplasmic antigens proteinase 3 (PR3). AAV are systemic diseases that can affect multiple organs including the upper and/or lower respiratory tracts, kidneys, skin and neural tissue and many patients also have common symptoms with fatigue, fever, and musculoskeletal symptoms. Although the different subgroups of AAV share many clinical characteristics, distinct associations to certain HLA-types being either associated to increased risk of disease or shown to be protective have been found (3–8), suggesting that the diseases may be separate entities.
The role of the immune system in the disease pathogenesis of AAV is also well established. Key events include loss of tolerance of T and B cells, followed by production of ANCA and subsequent activation of neutrophils and the destruction of vessels (9). Other immune cells have also implicated to have a role in the disease pathogenesis, including natural killer (NK) cells (10, 11). In this review, we focus on the current knowledge of NK cells in AAV.
NK Cell Biology and Function
NK cells are innate lymphoid cells that participate in the early anti-viral and anti-tumor defenses by recognizing and eliminating target cells without the need for prior activation (12). In addition to the killing of cancer and infected cells, NK cells can also eliminate otherwise stressed cells including senescent cells, over activated CD4+ T cells and autoreactive CD8+ T cells (13–15), and a growing number of studies suggest possible roles for NK cells also in autoimmunity. NK cells lack antigen specific receptors, but instead NK cell activation is accomplished by input through activating and inhibiting receptors and the net input determines if the release of perforin and granzyme B and target cell lysis will occur (16). Cytotoxicity by NK can also be performed via death receptor-mediated apoptosis (17). NK cells can furthermore eliminate antibody coated cells via engagement of the FcγRIIIA (CD16) receptor, leading to antibody dependent cellular cytotoxicity (ADCC) and cytokine production (11). The activation of NK cells via CD16 does not require any co-stimulation and is also an important link between NK cells and the adaptive immunity (18).
In addition to cytotoxic functions, NK cells can secrete cytokines like IL-10, TGFβ, TNFα or IFNγ and thereby exhibit immunomodulatory functions, which can either prevent or promote inflammatory processes (19). NK cell activation can also be achieved by a wide range of pro-inflammatory cytokines like IL‐2, IL‐12, IL‐15, IL‐18 or signals through toll-like receptors (TLR´s) (20). Phenotypically, NK cells are characterized by surface expression of the adhesion molecule CD56 and absence of CD3. The percentage of NK cells in healthy human peripheral blood is highly variable, approximately 2 – 30% of total lymphocytes (21, 22). Variations in the phenotypic markers used to define NK cells in the different studies complicate comparisons. For example, older studies are limited by the identification of NK cells only with one phenotypic marker, often CD16, and in studies performed with clinical routine staining CD16 and CD56 are sometimes indistinguishable (23). The majority of NK cells in peripheral blood are CD56dim, while CD56bright NK cells are enriched in secondary lymphoid organs, such as lymph node or tonsil, and other tissue including liver (24–26). Classically, CD56dim NK cells are known to be more cytotoxic and stronger activated by cell-cell contact. CD56bright NK cells are rather cytokine producers and more responsive tosoluble factors, including chemokines and cytokines, but both subsets can exert cytotoxicity and release cytokines upon appropriate stimulus (27, 28). Another subset of NK cells, expressing the maturation marker CD57, is characterized by a cytotoxic phenotype but less sensitive in the response to cytokines (29).
The Role of NK Cells in Autoimmune Diseases
The exact role for NK cells in autoimmunity is still controversial and data support both pathogenic and protective functions. On one hand, NK cells could directly participate in destroying host tissue, produce high levels of pro-inflammatory cytokines and kill T regulatory cells that normally prevent autoimmunity (30–32). On the other hand, NK cells do not only have cytotoxic functions, but can also secrete inhibitory cytokines, such as IL-10 and TGFβ, thus leading to a down regulation of the immune system and can block autoreactive CD8+ T cells (33). Hence, dysfunctional NK cells can result in an imbalance of the immune response which could both dampen and exacerbate autoimmune disease. NK cells can further participate in cytotoxicity of cells bound to therapeutic antibodies via ADCC in patients with autoimmune disease. There are many reviews focusing on the role of NK cells in autoimmunity (34–40), and some examples from different autoimmune diseases are outlined here. NK cell numbers are reduced in the circulation of many autoimmune diseases, including systemic lupus erythematosus (SLE), rheumatoid arthritis (RA), and Sjögren’s syndrome (41, 42). In patients with SLE, little is known about tissue infiltrating NK cells. Recently however, NK cell transcripts were detected by single cell RNA sequencing of kidney tissue from lupus nephritis patients in which they found two NK cell subsets, one with cytotoxic and one with tissue residency properties (43). In a mouse model for type 1 diabetes (T1D) NK cells with a more active phenotype and function were found in the pancreas (44) and in a unique small study of human pancreas sections from recent onset T1D patients half of the analyzed patients displayed cellular infiltrates dominated by NK cells (45). In addition, NK cells are present in actively demyelinating MS lesions in human patients (46) and in patients with RA, NK cells infiltrating inflamed joints were more prone to IFNγ secretion than NK cells from the circulation, indicating a possible disease-promoting function in the target tissue (31). Moreover, NK cells from the synovial fluids have been shown to secrete TNFα and IFNγ, leading to an activation state of synovial fibroblasts expressing HLA-DR+CD90+, and are considered to be involved in bone destruction (47, 48). Furthermore, a recent study showed an inverse correlation between NK cell numbers in the circulation and disease severity in RA (49). Conclusively, NK cells can exhibit disease-protecting or disease-enhancing properties in autoimmunity and because of the different properties reported on circulating and tissue infiltrating NK cells investigations both in the target organs as well as in the circulation are needed (Figure 1).
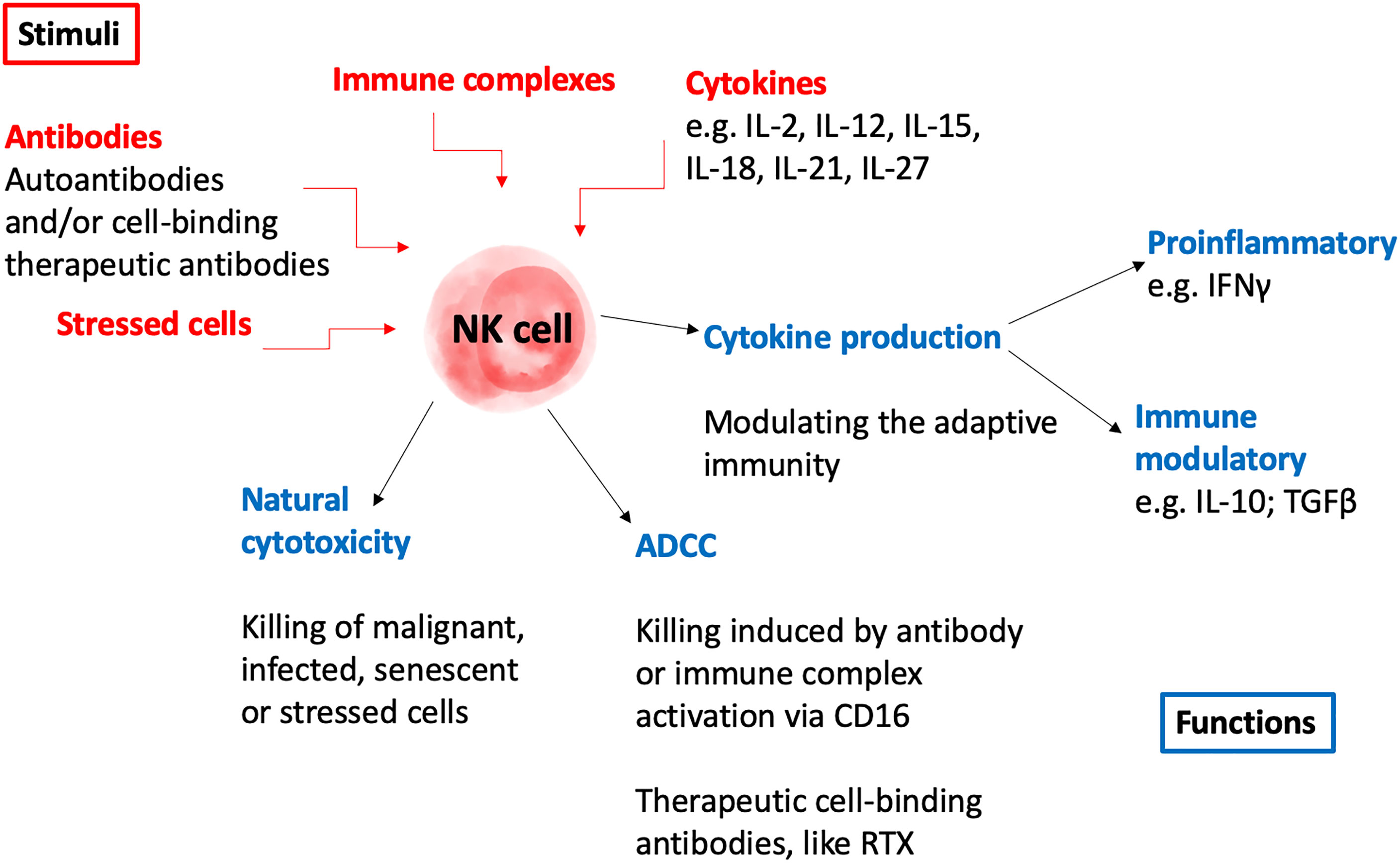
Figure 1 Schematic illustration of potential roles of NK cells in the pathogenesis of autoimmunity. AAV, anti-neutrophil cytoplasmic antibody (ANCA)-associated vasculitis; ADCC, antibody dependent cellular cytoxicity; IFNγ, interferon gamma; IL, interleukin; NK, natural killer; RTX, rituximab; TGFβ, transforming growth factor beta.
NK Cells in AAV
Data on the role of NK cells in AAV are largely lacking. In the present review we included all English written paper identified on PubMed on NK cells in AAV until June 2021, searching for NK cells, innate lymphoid cell 1 and AAV as well as the disease subtypes GPA, MPA and EGPA. We also screened the literature by using the old terms of the diseases, Churg-Strauss syndrome and Wegener’s granulomatosis.
Peripheral NK Cells in AAV
Several publications describe unaltered NK cell numbers and percentages in peripheral blood mononuclear cells (PBMCs) of AAV patients compared to control (Table 1) (52, 54, 58, 59). The literature focusing on NK cell numbers or percentage in AAV is however inconclusive, and some studies instead report a decrease or increase of NK cell numbers or percentage in these patients (23, 53, 55–57), in line with what has been reported for some other autoimmune diseases (60). Also in healthy persons there is however great variations in percentage and absolute numbers of NK cells and high age is generally an important contributing factor that may vary between studies (23). Hypothetically, this incongruence of data might also be explained by differences in the study population but also by the fact that the amount of NK cells in circulation can vary between AAV disease phenotype, different phases of the disease and in relation to treatment. In most studies of peripheral NK cells in AAV the ratio between CD56bright and CD56dim cells was unaltered in AAV compared to controls (52, 54, 55, 59). However, ANCA-negative AAV patients had a lower proportion of CD56dim cells compared to healthy controls whereas the frequency of CD56bright cells and CD56dim cells expressing high levels of CD57 were not influenced by occurrence of ANCA (59). Decreased NK cell numbers, and also specifically CD56dim cells, were further found in the active phase of disease (57) and levels returned to normal in disease remission (56, 57), but these differences were not confirmed in all studies (58). One interesting possibility is that the decrease of NK cells in the circulation in active phase of AAV compared to healthy controls reflects recruitment to the site of inflammation, thus lowering the number in circulation. Since the numbers of circulating NK cells negatively correlate with disease activity in GPA patients (55, 57), one possibility to further explore is if NK cells can act as a marker for disease activity in all AAV patients. The decrease in NK cell numbers during the active phase of disease was also confirmed in an analysis of GPA and MPA patient groups separately, but this study was not able to confirm an increase in remission (56).
Phenotype analyses of NK cells have shown more activated NK cells in AAV compared to controls, as indicated by a higher expression of the early activation marker CD69 (54, 55). The frequency of NK cells expressing the activating killer immunoglobulin-like receptor (KIR) KIR2DS3 was decreased in AAV patients compared to controls (51), but other activating and inhibitory NK cell receptors were unaltered. This included activating NKp46, NKp44, NKp30, NKG2D and DNAM-1 receptors and their co- receptors NTB-A and 2B4 (53–55), as well as activating KIRs, except KIR2D3, and inhibitory CD94/NKG2A and CD161 receptors (50, 54). The recruitment of distinct sets of NK cells to sites of infection, malignant transformation or autoimmune inflammation is guided by stimulation of cytokine and chemokine receptors expressed on the NK cells (61, 62). The analysis of chemokine receptors of NK cells, showed enhanced CCR5 expression in active phase of GPA but unaltered expression of CXCR3 and CXCR4 (50, 57). A higher expression of adhesion molecule ICAM-1 and NKG2C on CD56dim cells was also observed in AAV (57). Besides KIRs, NK cells also carry TLRs to recognize or respond to pathogen-associated molecular patterns such as bacterial or viral structures leading to cytotoxicity and cytokine production. An increased expression of TLRs 2, 4 and 9 on NK cells in AAV was observed, which could be explained by a pro-inflammatory microenvironment in these patients (52). Contradictory to this publication is the expression analysis of TLR2 on different subtypes of NK cells which was lower in AAV, possibly indicating a reduced capacity to get activated, while TLR9 expression on NK cells was unaltered (59). A decreased natural cytotoxicity was furthermore observed in peripheral NK cells from GPA patients in active phase compared to control and killing correlated to the percentage of NK cells (57). However, the production of IFNγ in response to viral infection and systemic inflammation and the degranulation activity of NK cells were unaltered in AAV. This indicates that GPA NK cells are at least partially functional. There is furthermore support that GPA patient NK cells participate in cytotoxicity of cells bound Rituximab (RTX) via ADCC. Certain polymorphisms of FcγRIIa and FcγRIIIa are also associated with the risk for disease relapse in GPA (63), and other with the treatment response to RTX and cyclophosphamide (64).
NK Cells in AAV Tissue
Most studies on NK cells in AAV have focused on findings in peripheral blood, however the local immune response can differ from what is observed in the circulation, making the analysis of NK cells in tissue inflammation important. A complicating factor is the lack of specific markers to identify NK cells and hence the need for more complex immunofluorescence co-staining when assessing tissue samples. There are a few studies where NK cells were detected by their expression of CD56, a marker for NK cells, but also for a subset of T cells (Table 2). Such basic studies detected no or rare presence of CD56+ cells in lung and kidney specimens of patients with GPA and pauci-immune necrotizing glomerulonephritis (55, 59, 66, 69). Using CD16 as NK cell defining marker, CD16+ cells were also identified in nasal tissue samples from few MPA patients with vasculitic neuropathies, however, CD16 is also expressed on neutrophils, monocytes and macrophages making the findings difficult to interpret (65).
A possible direct role for NK cells in local cytotoxicity within the target organ was further suggested by the detection of NK cell activating ligands MICA/B and the NK cell activating cytokine IL-15 in active GPA nasal biopsies (67, 68). The low numbers of NK cells and/or their absence in AAV tissue could lead to the speculation that NK cells might not contribute to local inflammation or to granuloma formation (55, 59). However, in a GPA-like syndrome characterized by chronic granulomatous lesions in the upper respiratory tract and skin vasculitis, a strong infiltration of CD3-CD56+ NK cells into skin was observed (70). This raises the question whether NK cells could be recruited differently to different organs. In line with this possibility, microvascular endothelial cells (MECs) were used to study their response to inflammatory stimuli and transmigration of immune cells into the inflamed tissue. MECs from lung and renal specimens were stimulated with PR3 which lead to a stronger degranulation of NK cells as compared to dermal MEC´s (54). Further, renal MECs seem to be more susceptible to the proinflammatory stimuli IFNγ and TNFα leading to ICAM1, VCAM1 and CCL2 upregulation, which could result in stronger renal recruitment of NK cells. GPA-derived NK cells were also able to directly kill renal MEC´s, indicating a potential role of NK cells in GPA pathogenesis (54). No studies have so far been performed on tissue infiltrating NK cells in EGPA. Further in-depth analysis of NK cells in AAV affected tissue using for example state of the art single cell RNA sequencing or laser capture microdissection of NK cells from tissue followed by RNA or proteomic profiling would be of interest. Single cell analysis of kidney tissue of ANCA-associated glomerulonephritis exists, however, NK cells were not addressed here (71). In summary, studies on NK cells at the site of inflammation are so far few and there is a need to analyze NK cells in tissue of AAV patients to advance the field.
The Impact of AAV Therapies on NK Cells
AAV patients are treated with glucocorticoids in combination with other immunosuppressing therapies at active flares, then followed by long-term remission maintenance therapy. To induce remission, cyclophosphamide (CYC) or RTX, an anti-CD20 chimeric antibody are commonly used (72–75). Despite intense immunosuppressive treatment, AAV patients have a high relapse rate. Studies on how NK cells are affected by immunosuppressive treatment are scarce and there is no data if they can be used to predict relapses. Recently, low peripheral NK cell numbers and percentages in GPA and EGPA, but not MPA patients, were associated with azathioprine (AZA) treatment in a dose-dependent manner, but with no other investigated immunosuppressive treatments, including mycophenolate mofetil, methotrexate, RTX and CYC (23). The lack of effect of AZA treatment on the NK cells in the MPA group could be explained by the fact that MPA patients included in this study were older than the other AAV groups and age positively correlate with NK cell number. Recently the effect of AZA treatment was analyzed in an SLE cohort and showed that these patients had an increased infection rate which was associated with AZA (76). Also, in patients with inflammatory bowel disease (IBD), AZA treatment was associated with lower NK cell counts in blood and an increased risk of herpes simplex infections, but the possible role of NK cells in that remains to be determined. In a previous study, CYC was also not shown to affect the frequency of circulating NK cells in AAV patients compared to non-CYC treated patients (23, 58). Similarly, RTX treatment or other disease-modifying anti-rheumatic drugs (DMARDs) did not alter the frequencies of NK cells in GPA patients as evaluated during different stages of the disease (55, 77). However, an analysis of the activation maker CD69 on PBMC NK cells from GPA patients prior and directly after RTX infusion showed increased activation. Furthermore, when healthy donor PBMCs were incubated overnight with RTX in vitro, phenotypical and functional changes of NK cells were observed (78). NK cells that were exposed to RTX showed a higher CD107a expression indicating degranulation and an enhanced activation as measured by CD69 expression on CD56dim cells (79). In addition, RTX induced a down regulation of Fc-γ-receptor CD16 which is a sign of NK cell activation and enhanced the expression of the costimulatory receptor CD137 on NK cells (80) and has been implicated in the pathogenesis of autoimmunity (81). Experience from using RTX as cancer treatment suggests that NK cell counts could potentially be used as a prognostic marker for the long-term effect of the treatment. The next generation antibody against CD20, a fully humanized antibody, Obinutzumab (OBZ), showed a higher ADCC and direct B cell killing (82). A study comparing OBZ to RTX showed that OBZ activates NK cells from GPA patients more efficiently and increases the depletion of non-malignant B cells in in vitro setting overnight (79). In conclusion, the effect of treatment on NK cells must be consideration as an important factor when assessing NK cell numbers, phenotype and function in AAV. Conversely, NK cells can potentially also participate in the effects of therapies, including cell-binding antibodies.
Concluding Remarks and Future Perspectives
AAV is a group of complex systemic autoimmune disease involving several target organs. This makes it challenging to firmly conclude about the properties and potential role of NK cells in the disease pathogenesis. NK cells in blood of AAV patients have an altered and activated phenotype, but whether the number and frequency of NK cells are also affected is not conclusively determined. Most published studies suggest that number or percentages of circulating NK cells in AAV patients are unaltered but other studies report an increase or decrease, especially in the active phase of the disease. NK cell numbers and frequency are however difficult to interpret, since there is a vast heterogeneity also in healthy controls. Furthermore, AAV patients’ treatments could potentially impact NK cells in AAV, and evidence for this is particularly strong for AZA (23). A potential role for NK cells in the effect of cell-binding therapeutic antibodies, via ADCC can also be envisioned. NK cells are also able to infiltrate into the target organ, but in low numbers and the biological significance of these cells or their role in the pathogenesis of AAV remains to be explored, by for example single cell analysis. Novel techniques for spatial analysis of protein and RNA expression in tissue sections could further advance the field and increase the knowledge about the immunological landscape in AAV organs, including NK cells.
Author Contributions
SF, AS, and HB wrote the manuscript. IG gave clinical input. All authors participated in discussing and finalizing the manuscript. All authors contributed to the article and approved the submitted version.
Funding
This work was supported by Åke Wiberg Foundation [M19-0665]; Cancerfonden [19 0408 Fk]; Hudfonden [3147]; Magnus Bergsvall Foundation [2019-03538]; Clas Groschinskys Foundation [M19390]; Stockholm county council/Region Stockholm [20190859] and the Swedish Society for Medical Research [S17-0104] to HB, and Karolinska Institutet Foundations to HB [2018-02203, 2018-02839] and SF [2019-01970].
Conflict of Interest
The authors declare that the research was conducted in the absence of any commercial or financial relationships that could be construed as a potential conflict of interest.
Publisher’s Note
All claims expressed in this article are solely those of the authors and do not necessarily represent those of their affiliated organizations, or those of the publisher, the editors and the reviewers. Any product that may be evaluated in this article, or claim that may be made by its manufacturer, is not guaranteed or endorsed by the publisher.
Acknowledgments
HB is supported by the Swedish Society for Medical Research, the Swedish Cancer Society, Region Stockholm, and Clas Groschinsky, Åke Wiberg, Magnus Bergvall, Welander’s, Karolinska Institutet Rheumatology and Karolinska Institutet foundations. We thank Guðný Ella Thorlacius for the illustration of an NK cell.
References
1. Cornec D, Fervenza FC, Specks U. ANCA-Associated Vasculitis — Clinical Utility of Using ANCA Specificity to Classify Patients. Nat Rev Rheumatol (2016) 12(10):570–9. doi: 10.1038/nrrheum.2016.123
2. Kallenberg CGM. Key Advances in the Clinical Approach to ANCA-Associated Vasculitis. Nat Publ Gr (2014) 10:484–93. doi: 10.1038/nrrheum.2014.104
3. Lyons PA, Rayner TF, Trivedi S, Holle JU, Watts RA, Jayne DRW, et al. Genetically Distinct Subsets Within ANCA-Associated Vasculitis. N Engl J Med (2012) 367(3):214–23. doi: 10.1056/NEJMoa1108735
4. Kawasaki A, Hasebe N, Hidaka M, Hirano F, Sada K, Kobayashi S, et al. Protective Role of HLA-DRB1 Ã 13: 02 Against Microscopic Polyangiitis and MPO-ANCA- Positive Vasculitides in a Japanese Population: A Case-Control Study. PloS One (2016) 11(5):e0154393. doi: 10.1371/journal.pone.0154393.
5. Tsuchiya N. Genetics of ANCA-Associated Vasculitis in Japan : A Role for HLA - DRB1 * 09 : 01 Haplotype. Clin Exp Nephrol (2013) 17:628–30. doi: 10.1007/s10157-012-0691-6
6. Xie G, Roshandel D, Sherva R, Monach PA, Lu EY, Kung T, et al. Association of Granulomatosis With Polyangiitis (Wegener ‘ s) With HLA – DPB1 * 04 and SEMA6A Gene Variants Evidence From Genome-Wide Analysis. Arthritis Rheum (2013) 65(9):2457–68. doi: 10.1002/art.38036
7. Wieczorek S, Hellmich B, Gross WL, Epplen JT. Associations of Churg-Strauss Syndrome With the HLA-DRB1 Locus, and Relationship to the Genetics of Antineutrophil Cytoplasmic Antibody-Associated Vasculitides: Comment on the Article by Vaglio Et al. [1]. Arthritis Rheum (2008) 58(1):329–30. doi: 10.1002/art.23209
8. Lyons PA, Peters JE, Alberici F, Liley J, Coulson RMR, Astle W, et al. Genome-Wide Association Study of Eosinophilic Granulomatosis With Polyangiitis Reveals Genomic Loci Stratified by ANCA Status. Nat Commun (2019) 10(1):1–13. doi: 10.1038/s41467-019-12515-9
9. Kronbichler A, Lee KH, Denicol S, Choi D, Lee H, Ahn D, et al. Immunopathogenesis of ANCA-Associated Vasculitis. Int J Mol Sci (2020) 21(19):7319. doi: 10.3390/ijms21197319
10. Vivier E, Tomasello E, Baratin M, Walzer T, Ugolini S. Functions of Natural Killer Cells. Nat Immunol (2008) 9(5):503–10. doi: 10.1038/ni1582
11. Vivier E, Raulet DH, Moretta A, Caligiuri MA, Zitvogel L, Lanier LL, et al. Innate or Adaptive Immunity? The Example of Natural Killer Cells. Science (80-) (2011) 331(6013):44–9. doi: 10.1126/science.1198687
12. Sivori S, Vacca P, Del Zotto G, Munari E, Mingari MC, Moretta L. Human NK Cells: Surface Receptors, Inhibitory Checkpoints, and Translational Applications. Cell Mol Immunol (2019) 16(5):430–41. doi: 10.1038/s41423-019-0206-4
13. Poggi A, Zocchi MR. NK Cell Autoreactivity and Autoimmune Diseases. Front Immunol (2014) 5(FEB):1–15. doi: 10.3389/fimmu.2014.00027
14. Shimoda S, Hisamoto S, Harada K, Iwasaka S, Chong Y, Nakamura M, et al. Natural Killer Cells Regulate T Cell Immune Responses in Primary Biliary Cirrhosis. Hepatol (2015) 62(6):1817–27. doi: 10.1002/hep.28122
15. Antonangeli F, Zingoni A, Soriani A, Santoni A. Senescent Cells: Living or Dying is a Matter of NK Cells. J Leukoc Biol (2019) 105(6):1275–83. doi: 10.1002/JLB.MR0718-299R
16. Abel AM, Yang C, Thakar MS, Malarkannan S. Natural Killer Cells: Development, Maturation, and Clinical Utilization. Front Immunol (2018) 9(AUG):1–23. doi: 10.3389/fimmu.2018.01869
17. Prager I, Watzl C. Mechanisms of Natural Killer Cell-Mediated Cellular Cytotoxicity. J Leukoc Biol (2019) 105(6):1319–29. doi: 10.1002/JLB.MR0718-269R
18. Bryceson YT, March ME, Ljunggren H-G, Long EO. Synergy Among Receptors on Resting NK Cells for the Activation of Natural Cytotoxicity and Cytokine Secretion. Blood (2006) 107(1):159–66. doi: 10.1182/blood-2005-04-1351
19. Strowig T, Brilot F, Münz C. Noncytotoxic Functions of NK Cells: Direct Pathogen Restriction and Assistance to Adaptive Immunity. J Immunol (2008) 180(12):7785–91. doi: 10.4049/jimmunol.180.12.7785
20. Zwirner NW, Domaica CI. Cytokine Regulation of Natural Killer Cell Effector Functions. BioFactors (2010) 36(4):274–88. doi: 10.1002/biof.107
21. Bisset LR, Lung TL, Kaelin M, Ludwig E, Dubs RW. Reference Values for Peripheral Blood Lymphocyte Phenotypes Applicable to the Healthy Adult Population in Switzerland. Eur J Haematol (2004) 72(3):203–12. doi: 10.1046/j.0902-4441.2003.00199.x
22. Pascal V, Schleinitz N, Brunet C, Ravet S, Bonnet E, Lafarge X, et al. Comparative Analysis of NK Cell Subset Distribution in Normal and Lymphoproliferative Disease of Granular Lymphocyte Conditions. Eur J Immunol (2004) 34(10):2930–40. doi: 10.1002/eji.200425146
23. Merkt W, Salzer U, Thiel J, Jandova I, Bergner R, Venhoff AC, et al. Blood CD3-(CD56 or 16)+ Natural Killer Cell Distributions are Heterogeneous in Healthy Adults and Suppressed by Azathioprine in Patients With ANCA-Associated Vasculitides. BMC Immunol (2021) 22(1):1–13. doi: 10.1186/s12865-021-00416-w
24. Cooper MA, Fehniger TA, Caligiuri MA. The Biology of Human Natural Killer-Cell Subsets. Trends Immunol (2001) 22(11):633–40. doi: 10.1016/S1471-4906(01)02060-9
25. Doyle EH, Aloman C, El-Shamy A, Eng F, Rahman A, Klepper AL, et al. A Subset of Liver Resident Natural Killer Cells is Expanded in Hepatitis C-Infected Patients With Better Liver Function. Sci Rep (2021) 11(1):1–13. doi: 10.1038/s41598-020-80819-8
26. Ferlazzo G, Thomas D, Lin S-L, Goodman K, Morandi B, Muller WA, et al. The Abundant NK Cells in Human Secondary Lymphoid Tissues Require Activation to Express Killer Cell Ig-Like Receptors and Become Cytolytic. J Immunol (2004) 172(3):1455–62. doi: 10.4049/jimmunol.172.3.1455
27. Wagner JA, Rosario M, Romee R, Berrien-Elliott MM, Schneider SE, Leong JW, et al. CD56bright NK Cells Exhibit Potent Antitumor Responses Following IL-15 Priming. J Clin Invest (2017) 127(11):4042–58. doi: 10.1172/JCI90387
28. De Maria A, Bozzano F, Cantoni C, Moretta L. Revisiting Human Natural Killer Cell Subset Function Revealed Cytolytic CD56dimCD16+ NK Cells as Rapid Producers of Abundant IFN-γ on Activation. Proc Natl Acad Sci USA (2011) 108(2):728–32. doi: 10.1073/pnas.1012356108
29. Lopez-Vergès S, Milush JM, Pandey S, York VA, Arakawa-Hoyt J, Pircher H, et al. CD57 Defines a Functionally Distinct Population of Mature NK Cells in the Human CD56dimCD16+ NK-Cell Subset. Blood (2010) 116(19):3865–74. doi: 10.1182/blood-2010-04-282301
30. Cruz-Muñoz ME, Valenzuela-Vázquez L, Sánchez-Herrera J, Santa-Olalla Tapia J. From the “Missing Self” Hypothesis to Adaptive NK Cells: Insights of NK Cell-Mediated Effector Functions in Immune Surveillance. J Leukoc Biol (2019) 105(5):955–71. doi: 10.1002/JLB.MR0618-224RR
31. Dalbeth N, Callan MFC. A Subset of Natural Killer Cells Is Greatly Expanded Within Inflamed Joints. Arthritis Rheum (2002) 46(7):1763–72. doi: 10.1002/art.10410
32. Poggi A, Zocchi MR. Human Natural Killer Lymphocytes Through the Engagement of Natural Cytotoxicity Receptors and NKG2D can Trigger Self-Aggression. Autoimmun Rev (2007) 6(5):295–9. doi: 10.1016/j.autrev.2006.07.003
33. Lang PA, Crome SQ, Xu HC, Lang KS, Chapatte L, Deenick EK, et al. NK Cells Regulate CD8+ T Cell Mediated Autoimmunity. Front Cell Infect Microbiol (2020) 10(February):1–10. doi: 10.3389/fcimb.2020.00036
34. Gross CC, Schulte-Mecklenbeck A, Wiendl H, Marcenaro E, de Rosbo NK, Uccelli A, et al. Regulatory Functions of Natural Killer Cells in Multiple Sclerosis. Front Immunol (2016) 7(DEC). doi: 10.3389/fimmu.2016.00606
35. Mandal A, Viswanathan C. Natural Killer Cells: In Health and Disease. Hematol Oncol Stem Cell Ther (2015) 8(2):47–55. doi: 10.1016/j.hemonc.2014.11.006
36. Marca VL, Gianchecchi E, Fierabracci A. Type 1 Diabetes and its Multi-Factorial Pathogenesis: The Putative Role of NK Cells. Int J Mol Sci (2018) 19(3):1–16. doi: 10.3390/ijms19030794
37. Popko K, Górska E. The Role of Natural Killer Cells in Pathogenesis of Autoimmune Diseases. Cent Eur J Immunol (2015) 40(4):470–6. doi: 10.5114/ceji.2015.56971
38. Spada R, Rojas JM, Barber DF. Recent Findings on the Role of Natural Killer Cells in the Pathogenesis of Systemic Lupus Erythematosus. J Leukoc Biol (2015) 98(4):479–87. doi: 10.1189/jlb.4RU0315-081RR
39. Zheng M, Sun H, Tian Z. Natural Killer Cells in Liver Diseases. Front Med (2018) 12(3):269–79. doi: 10.1007/s11684-018-0621-4
40. Zitti B, Bryceson YT. Natural Killer Cells in Inflammation and Autoimmunity. Cytokine Growth Factor Rev (2018) 42(August):37–46. doi: 10.1016/j.cytogfr.2018.08.001
41. Li W-X, Pan H-F, Hu J-L, Wang C-Z, Zhang N, Li J, et al. Assay of T- and NK-Cell Subsets and the Expression of NKG2A and NKG2D in Patients With New-Onset Systemic Lupus Erythematosus. Clin Rheumatol (2010) 29(3):315–23. doi: 10.1007/s10067-009-1322-9
42. Izumi Y, Ida H, Huang M, Iwanaga N, Tanaka F, Aratake K, et al. Characterization of Peripheral Natural Killer Cells in Primary Sjögren’s Syndrome: Impaired NK Cell Activity and Low NK Cell Number. J Lab Clin Med (2006) 147(5):242–9. doi: 10.1016/j.lab.2006.01.001
43. Arazi A, Rao DA, Berthier CC, Davidson A, Liu Y, Hoover PJ, et al. The Immune Cell Landscape in Kidneys of Patients With Lupus Nephritis. Nat Immunol (2019) 20(7):902–14. doi: 10.1038/s41590-019-0398-x
44. Brauner H, Elemans M, Lemos S, Broberger C, Holmberg D, Flodström-Tullberg M, et al. Distinct Phenotype and Function of NK Cells in the Pancreas of Nonobese Diabetic Mice. J Immunol (2010) 184(5):2272–80. doi: 10.4049/jimmunol.0804358
45. Dotta F, Censini S, Van Halteren AGS, Marselli L, Masini M, Dionisi S, et al. Coxsackie B4 Virus Infection of β Cells and Natural Killer Cell Insulitis in Recent-Onset Type 1 Diabetic Patients. Proc Natl Acad Sci USA (2007) 104(12):5115–20. doi: 10.1073/pnas.0700442104
46. Gross CC, Schulte-Mecklenbeck A, Rünzi A, Kuhlmann T, Posevitz-Fejfár A, Schwab N, et al. Impaired NK-Mediated Regulation of T-Cell Activity in Multiple Sclerosis is Reconstituted by IL-2 Receptor Modulation. Proc Natl Acad Sci USA (2016) 113(21):E2973–82. doi: 10.1073/pnas.1524924113
47. Yamin R, Berhani O, Peleg H, Aamar S, Stein N, Gamliel M, et al. High Percentages and Activity of Synovial Fluid NK Cells Present in Patients With Advanced Stage Active Rheumatoid Arthritis. Sci Rep (2019) 9(1):1–12. doi: 10.1038/s41598-018-37448-z
48. Zhao S, Grieshaber-Bouyer R, Rao DA, Kolb P, Chen H, Andreeva I, et al. JAK Inhibition Prevents the Induction of Pro-Inflammatory HLA-DR + CD90 + RA Synovial Fibroblasts by Ifnγ. Arthritis Rheumatol (2021) 0–2. doi: 10.1002/art.41958
49. Lin SJ, Hsu CY, Kuo ML, Lee PT, Hsiao HS, Chen JY. Phenotypic and Functional Characterization of Natural Killer Cells in Rheumatoid Arthritis-Regulation With Interleukin-15. Sci Rep (2020) 10(1):4–11. doi: 10.1038/s41598-020-62654-z
50. Kottilil S, Shin K, Planta M, McLaughlin M, Hallahan CW, Ghany M, et al. Expression of Chemokine and Inhibitory Receptors on Natural Killer Cells: Effect of Immune Activation and HIV Viremia. J Infect Dis (2004) 189(7):1193–8. doi: 10.1086/382090
51. Miyashita R, Tsuchiya N, Yabe T, Kobayashi S, Hashimoto H, Ozaki S, et al. Association of Killer Cell Immunoglobulin-Like Receptor Genotypes With Microscopic Polyangiitis. Arthritis Rheum (2006) 54(3):992–7. doi: 10.1002/art.21653
52. Tadema H, Abdulahad WH, Stegeman CA, Kallenberg CGM, Heeringa P. Increased Expression of Toll-Like Receptors by Monocytes and Natural Killer Cells in Anca-Associated Vasculitis. PloS One (2011) 6(9):24315. doi: 10.1371/journal.pone.0024315
53. Puxeddu I, Bongiorni F, Chimenti D, Bombardieri S, Moretta A, Bottino C, et al. Cell Surface Expression of Activating Receptors and Co-Receptors on Peripheral Blood NK Cells in Systemic Autoimmune Diseases. Scand J Rheumatol (2012) 41(4):298–304. doi: 10.3109/03009742.2011.648657
54. Tognarelli S, Gayet J, Lambert M, Dupuy S, Karras A, Cohen P, et al. Tissue-Specific Microvascular Endothelial Cells Show Distinct Capacity To Activate NK Cells: Implications for the Pathophysiology of Granulomatosis With Polyangiitis. J Immunol (2014) 192(7):3399–408. doi: 10.4049/jimmunol.1301508
55. Merkt W, Sturm P, Lasitschka F, Tretter T, Watzl C, Saure D, et al. Peripheral Blood Natural Killer Cell Percentages in Granulomatosis With Polyangiitis Correlate With Disease Inactivity and Stage. Arthritis Res Ther (2015) 17(1):1–11. doi: 10.1186/s13075-015-0851-7
56. Braudeau C, Amouriaux K, Néel A, Herbreteau G, Salabert N, Rimbert M, et al. Persistent Deficiency of Circulating Mucosal-Associated Invariant T (MAIT) Cells in ANCA-Associated Vasculitis. J Autoimmun (2016) 70:73–9. doi: 10.1016/j.jaut.2016.03.015
57. Merkt W, Claus M, Blank N, Hundemer M, Cerwenka A, Lorenz HM, et al. Active But Not Inactive Granulomatosis With Polyangiitis is Associated With Decreased and Phenotypically and Functionally Altered CD56dim Natural Killer Cells. Arthritis Res Ther (2016) 18(1):1–12. doi: 10.1186/s13075-016-1098-7
58. Fazekas B, Moreno-Olivera A, Kelly Y, O’Hara P, Murray S, Kennedy A, et al. Alterations in Circulating Lymphoid Cell Populations in Systemic Small Vessel Vasculitis are non-Specific Manifestations of Renal Injury. Clin Exp Immunol (2018) 191(2):180–8. doi: 10.1111/cei.13058
59. Scrivo R, Peruzzi G, Gattamelata A, Gross CC, Carletti R, Di Gioia C, et al. Multicolor Flow Cytometric Analysis of TLR2 and TLR9 Expression and Function in NK Cells From Patients With ANCA-Associated Vasculitis. Cytom Part B Clin Cytom (2018) 94(3):412–22. doi: 10.1002/cyto.b.21586
60. Kucuksezer UC, Cetin EA, Esen F, Tahrali I, Akdeniz N. The Role of Natural Killer Cells in Autoimmune Diseases. Front Immunol (2021) 12:622306. doi: 10.3389/fimmu.2021.622306
61. Berahovich RD, Lai NL, Wei Z, Lanier LL, Schall TJ. Evidence for NK Cell Subsets Based on Chemokine Receptor Expression. J Immunol (2006) 177(11):7833–40. doi: 10.4049/jimmunol.177.11.7833
62. Bernardini G, Antonangeli F, Bonanni V, Santoni A. Dysregulation of Chemokine/Chemokine Receptor Axes and NK Cell Tissue Localization During Diseases. Front Immunol (2016) 7(OCT):1–9. doi: 10.3389/fimmu.2016.00402
63. Dijstelbloem HM, Scheepers RHM, Oost WW, Stegeman CA, van der Pol WL, Sluiter WJ, et al. Fcγ Receptor Polymorphisms in Wegener’s Granulomatosis: Risk Factors for Disease Relapse. Arthritis Rheum (1999) 42(9):1823–7. doi: 10.1002/1529-0131(199909)42:9<1823::AID-ANR5>3.0.CO;2-X
64. Cartin-Ceba R, Indrakanti D, Specks U, Stone JH, Hoffman GS, Kallenberg CGM, et al. The Pharmacogenomic Association of Fcγ Receptors and Cytochrome P450 Enzymes With Response to Rituximab or Cyclophosphamide Treatment in Antineutrophil Cytoplasmic Antibody–Associated Vasculitis. Arthritis Rheumatol (2017) 69(1):169–75. doi: 10.1002/art.39822
65. Engelhardt A, Lörler H, Neundörfer B. Immunohistochemical Findings in Vasculitic Neuropathies. Acta Neurol Scand (1993) 87(4):318–21. doi: 10.1111/j.1600-0404.1993.tb05515.x
66. Coulomb-L’Hermine A, Capron F, Zou W, Piard F, Galateau F, Laurent P, et al. Expression of the Chemokine RANTES in Pulmonary Wegener’s Granulomatosis. Hum Pathol (2001) 32(3):320–6. doi: 10.1053/hupa.2001.22757
67. Capraru D, Müller A, Csernok E, Gross WL, Holl-Ulrich K, Northfield J, et al. Expansion of Circulating NKG2D+ Effector Memory T-Cells and Expression of NKG2D-Ligand MIC in Granulomaous Lesions in Wegener’s Granulomatosis. Clin Immunol (2008) 127(2):144–50. doi: 10.1016/j.clim.2007.12.004
68. De Menthon M, Lambert M, Guiard E, Tognarelli S, Bienvenu B, Karras A, et al. Excessive Interleukin-15 Transpresentation Endows NKG2D+CD4+ T Cells With Innate-Like Capacity to Lyse Vascular Endothelium in Granulomatosis With Polyangiitis (Wegener’s). Arthritis Rheum (2011) 63(7):2116–26. doi: 10.1002/art.30355
69. Zhao L, David MZ, Hyjek E, Chang A, Meehan SM. M2 Macrophage Infiltrates in the Early Stages of ANCA-Associated Pauci-Immune Necrotizing GN. Clin J Am Soc Nephrol (2015) 10(1):54–63. doi: 10.2215/CJN.03230314
70. Moins-Teisserenc H, Gadola SD, Cella M, Dunbar PR, Exley A, Blake N, et al. Association of a Syndrome Resembling Wegener’s Granulomatosis With Low Surface Expression of HLA Class-I Molecules. Lancet (1999) 354(9190):1598–603. doi: 10.1016/S0140-6736(99)04206-3
71. Krebs CF, Reimers D, Zhao Y, Paust H-J, Bartsch P, Nuñez S, et al. Pathogen-Induced Tissue-Resident Memory TH17 (TRM17) Cells Amplify Autoimmune Kidney Disease. Sci Immunol (2020) 5(50):eaba4163. doi: 10.1126/sciimmunol.aba4163
72. Geetha D, Jefferson JA. ANCA-Associated Vasculitis: Core Curriculum 2020. Am J Kidney Dis (2020) 75(1):124–37. doi: 10.1053/j.ajkd.2019.04.031
73. Wallace ZS, Miloslavsky EM. Management of ANCA Associated Vasculitis. BMJ (2020) 368:m421. doi: 10.1136/bmj.m421
74. van Dam LS, Dirikgil E, Bredewold EW, Ray A, Bakker JA, van Kooten C, et al. Proteinase-3-Anti-Neutrophil Cytoplasmic Antibodies (PR3-ANCAs) Predict Relapses in ANCA–Associated Vasculitis Patients After Rituximab. Nephrol Dial Transplant (2020) 36(8):1408–17. doi: 10.1093/ndt/gfaa066
75. Jones RB, Cohen Tervaert JW, Hauser T, Luqmani R, Morgan MD, Peh CA, et al. Rituximab Versus Cyclophosphamide in ANCA-Associated Renal Vasculitis. N Engl J Med (2010) 363(3):211–20. doi: 10.1056/NEJMoa0909169
76. Simard JF, Rossides M, Gunnarsson I, Svenungsson E, Arkema EV. Infection Hospitalisation in Systemic Lupus in Sweden. Lupus Sci Med (2021) 8(1):1–8. doi: 10.1136/lupus-2021-000510
77. Chocova Z, Hruskova Z, Mareckova H, Svobodova B, Duskova D, Bednarova V, et al. Rituximab Use in Patients With ANCA-Associated Vasculitis: Clinical Efficacy and Impact on Immunological Parameters. Clin Rheumatol (2015) 34(1):107–15. doi: 10.1007/s10067-014-2816-7
78. Merkt W, Lorenz HM, Watzl C. Rituximab Induces Phenotypical and Functional Changes of NK Cells in a Non-Malignant Experimental Setting. Arthritis Res Ther (2016) 18(1):1–11. doi: 10.1186/s13075-016-1101-3
79. Urlaub D, Zhao S, Blank N, Bergner R, Claus M, Tretter T, et al. Activation of Natural Killer Cells by Rituximab in Granulomatosis With Polyangiitis. Arthritis Res Ther (2019) 21(1):1–11. doi: 10.1186/s13075-019-2054-0
80. Melero I, Johnston JV, Shufford WW, Mittler RS, Chen L. NK1.1 Cells Express 4-1BB (CDw137) Costimulatory Molecule and Are Required for Tumor Immunity Elicited by Anti-4-1BB Monoclonal Antibodies. Cell Immunol (1998) 190(2):167–72. doi: 10.1006/cimm.1998.1396
81. Zhang Q, Vignali DAA. Co-Stimulatory and Co-Inhibitory Pathways in Autoimmunity. Immun (2016) 44(5):1034–51. doi: 10.1016/j.immuni.2016.04.017
Keywords: natural killer cells, anti-neutrophil cytoplasmic antibody, ANCA - associated vasculitis, microscopic polyangiitis (MPA), granulomatosis with polyangiitis (GPA), eosinophilic granulomatosis with polyangiitis (EGPA)
Citation: Fuchs S, Scheffschick A, Gunnarsson I and Brauner H (2022) Natural Killer Cells in Anti-Neutrophil Cytoplasmic Antibody-Associated Vasculitis - A Review of the Literature. Front. Immunol. 12:796640. doi: 10.3389/fimmu.2021.796640
Received: 17 October 2021; Accepted: 20 December 2021;
Published: 14 January 2022.
Edited by:
A. Richard Kitching, Monash University, AustraliaReviewed by:
Wolfgang Merkt, Heidelberg University Hospital, GermanyCopyright © 2022 Fuchs, Scheffschick, Gunnarsson and Brauner. This is an open-access article distributed under the terms of the Creative Commons Attribution License (CC BY). The use, distribution or reproduction in other forums is permitted, provided the original author(s) and the copyright owner(s) are credited and that the original publication in this journal is cited, in accordance with accepted academic practice. No use, distribution or reproduction is permitted which does not comply with these terms.
*Correspondence: Hanna Brauner, hanna.brauner@ki.se