- 1Department of Rheumatology and Clinical Immunology, Amsterdam University Medical Center, VU University Medical Center (VUmc), Amsterdam, Netherlands
- 2Department of Radiology & Nuclear Medicine, Amsterdam University Medical Center, VU, Amsterdam, Netherlands
- 3Department of Chemistry, Purdue University, West Lafayette, IN, United States
- 4Department of Rheumatology and Clinical Immunology, Amsterdam University Medical Center, AMC, Amsterdam, Netherlands
Non-invasive imaging modalities constitute an increasingly important tool in diagnostic and therapy response monitoring of patients with autoimmune diseases, including rheumatoid arthritis (RA). In particular, macrophage imaging with positron emission tomography (PET) using novel radiotracers based on differential expression of plasma membrane proteins and functioning of cellular processes may be suited for this. Over the past decade, selective expression of folate receptor β (FRβ), a glycosylphosphatidylinositol-anchored plasma membrane protein, on myeloid cells has emerged as an attractive target for macrophage imaging by exploiting the high binding affinity of folate-based PET tracers. This work discusses molecular, biochemical and functional properties of FRβ, describes the preclinical development of a folate-PET tracer and the evaluation of this tracer in a translational model of arthritis for diagnostics and therapy-response monitoring, and finally the first clinical application of the folate-PET tracer in RA patients with active disease. Consequently, folate-based PET tracers hold great promise for macrophage imaging in a variety of (chronic) inflammatory (autoimmune) diseases beyond RA.
1 The Role of Folate Receptor Beta For PET Imaging in Arthritis
1.1 Synovial Macrophages as Biomarkers for RA Disease Activity Assessment
Rheumatoid arthritis (RA) is an autoimmune disease of the joints characterized by the infiltration of various immune cells in the synovium amongst which macrophages play an important role (1, 2). Macrophages impact on other immune cells and inflammatory processes via the release of proinflammatory cytokines (e.g. TNFα) and chemokines, which may promote activation of T cells and other immune cells, trigger endothelial cell activation and (pathological) angiogenesis, and induce osteoclast activation (3, 4). Synovial tissue analysis has pointed out that the (activated) synovial macrophage is a key biomarker for disease activity assessment from the early disease onwards and for monitoring of therapeutic efficacy at later stages of the disease (5, 6). The basic synovial joint architecture of the healthy joint is comprised of a double layered structure (synovial lining) which holds tissue resident macrophages, and underneath a vascularized sub-lining layer of connective tissue (7). In the early stages of RA, infiltration of immune cells is observed in combination with activation of resident macrophages present in the synovial lining layer (2). Established RA is marked by progressive macrophage infiltration in the synovium (~10-20 layers) (8). In fact, macrophages represent one of the most prominent cell types present in the synovium during early stage and also established RA (2, 9, 10), being responsive to treatment (6), and thus underscoring their exploitation as a biomarker for the assessment of RA disease through positron emission tomography (PET) imaging.
The importance of macrophages as key player in the pathogenesis of RA has been explored in both preclinical and clinical studies. It has for example been shown in animal models of arthritis that depletion of macrophages significantly decreases the severity of the disease (11, 12). Also, in RA patients, macrophage infiltration in the RA synovium has been found to significantly correlate with disease severity (e.g. with changes in disease activity score (DAS) and composite change index) (5, 6, 13). In addition, recent in depth cellular and molecular analyses of RA synovial tissues revealed that RA patients could be stratified in 3 pathology groups based on the presence of specific immune cell types (14–16). These 3 pathotypes were designated diffuse-myeloid (characterized by predominantly myeloid cell infiltration, notably macrophages), lympho-myeloid (predominantly B-cell infiltration), and pauci-immune (low immune cell infiltration) (14). Remarkably, a higher diffuse-myeloid gene expression profile, thus characterized by macrophage infiltration, was associated with a higher DAS 28-ESR and a larger DAS 28-ESR reduction after treatment with disease modifying anti-rheumatic drugs (DMARDs) (14), again underscoring the importance of the synovial macrophage as biomarker for RA disease activity.
Although it is well recognized that macrophages play an important role in the pathology of RA, they can exert both pro- and anti-inflammatory roles associated with their polarization (17). The synovial cytokine milieu, in particular granulocyte-macrophage colony-stimulating factor (GM-CSF) and macrophage colony-stimulating-factor (M-CSF), constitutes the driving force in skewing macrophages to the M1-type pro-inflammatory phenotype and the M2-type anti-inflammatory macrophage, respectively (9, 18–20). M1 and M2 represent the extremes of macrophage polarization and have been characterized based on differences in their transcriptome, secretome and proteome profiles (19–22). Several (membrane) marker proteins are commonly used to classify M1 (e.g. CD80, TNFα, iNOS) and M2 (e.g. CD163, IL-10, Arginase) macrophage subpopulations, and have been associated to an inflammatory or remission state of RA. For example, macrophage subpopulations were found associated with RA disease remission such as synovial tissue macrophages that are MerTK positive (MerTKpos), lymphatic vessel endothelial hyaluronan receptor 1 positive (LYVE1pos) and have a high expression of Folate Receptor (FR) beta (FRβ-high) (23). In the context of this review, FRβ expression has long been recognized on macrophages that are triggered by inflammatory stimuli and on activated macrophages in inflamed joints of RA patients (24, 25). In ex-vivo M-CSF skewed monocyte-derived macrophages, FRβ is differentially expressed on M2-type macrophages (26–28). However, in inflamed RA synovium, these FRβ-expressing M2-macrophages can produce pro-inflammatory cytokines when exposed to either pro-inflammatory stimuli (i.e. lipo-polysaccharide (LPS) + interferon-γ (IFNγ) (19) or an RA synovial microenvironment with anti-citrullinated protein antibodies or complex IgGs (29, 30). Together, FRβ is a bona fide marker on synovial macrophage subpopulations, even though its exact role in function in either pro- and anti-inflammatory macrophages needs to be defined in greater detail. FRβ expression on (activated) macrophages in RA has initiated research aimed at therapeutic targeting as well disease monitoring with imaging modalities (31, 32), which will be discussed in the next sections.
1.2 Folate Receptors: Function, Structure and Targeting
Human FRs are high affinity binding proteins for folates (folic acid and reduced folate cofactors) which are essential vitamins necessary for single carbon transfer reactions in amino acid biosynthesis (e.g. conversion of homocysteine into methionine) and for de novo purine and thymidylate biosynthesis (33–35). There are four types of FRs: FR-alpha (FRα), FR-beta (FRβ), FR-gamma (FRγ) and FR-delta (FRδ) (Figure 1A). Of those, FRα, FRβ and FRδ are glycosylphosphatidylinositol (GPI) membrane anchored, whereas FRγ is a secreted form (mainly from hematopoietic cells) because of a lack of an efficient signal for GPI modification (36–39). FRα is expressed on normal epithelial cells (e.g. kidney, spleen and lung tissue) (40) and tumor tissues like ovarian, breast (41), pancreatic and lung carcinomas (42, 43). FRβ is selectively expressed on cells of the myeloid lineage (44, 45) and is upregulated on activated macrophages in active RA disease (24, 25) wherein its expression is regulated by PU.1 transcription factor (46). The FRδ gene was originally identified being highly homologous mouse folate binding protein 3 (Folbp3) (47), but does not harbor folate binding capacity (48). FRδ (and splice variants thereof) is expressed on regulatory T cells and has a proposed function in immune regulation (49).
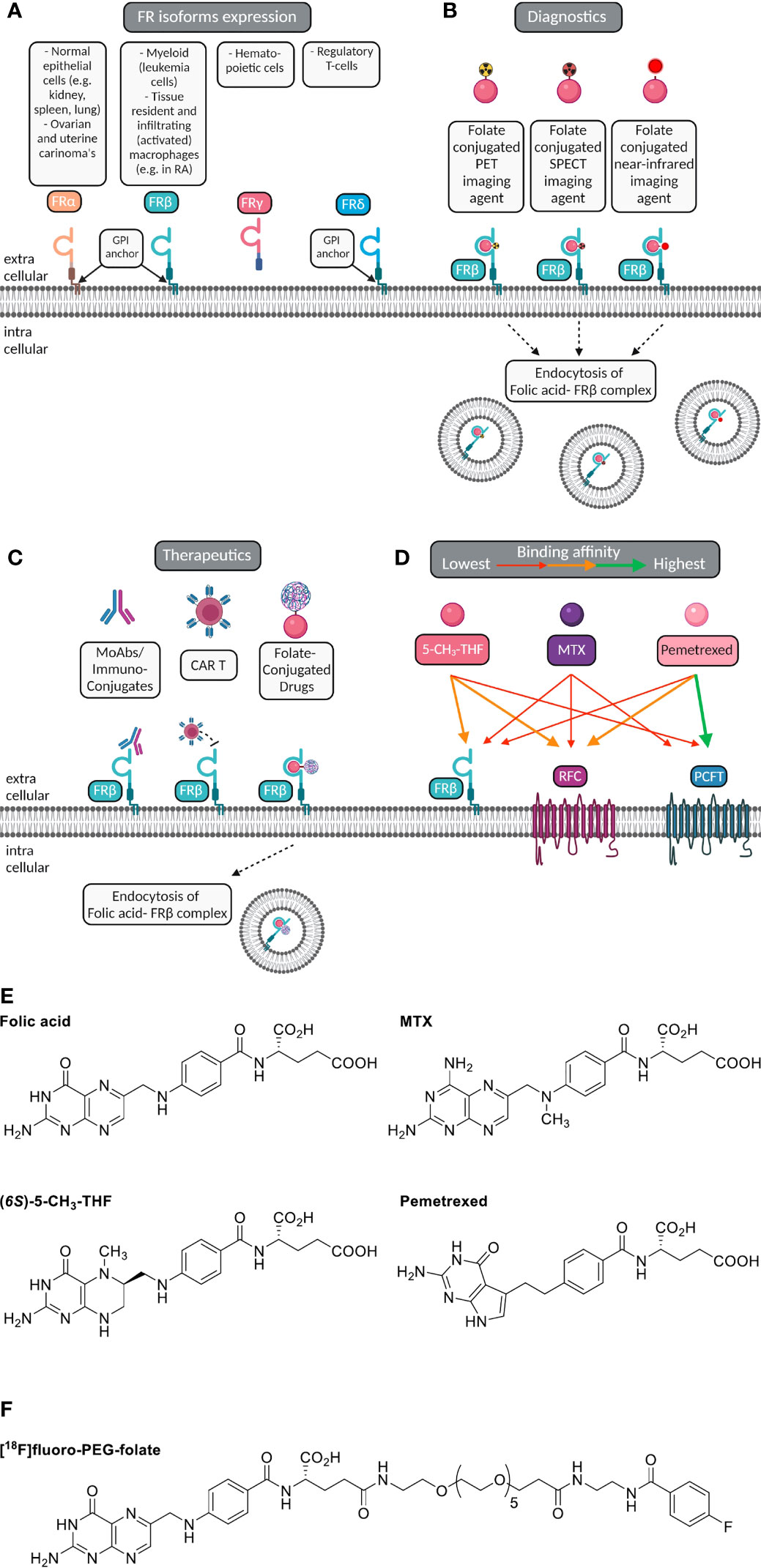
Figure 1 Macrophage FRβ for folate-based imaging and therapeutic targeting. (A) Folate receptor isoforms FRα, FRβ, FRγ and FRδ, their GPI-membrane anchoring (except FRγ), and cell/tissue expression. (B) Selective expression of FRβ (cyanin), a glycosylphosphatidylinositol-anchored plasma membrane protein, on myeloid cells (e.g. macrophages) constitutes a suitable target for imaging of inflammatory disease, including RA. Folate (pink ellipse) is coupled to a radioactive isotope (for PET or SPECT) or near infrared fluorescent dye (for optical imaging). Following high affinity binding to FRβ, these imaging agents may stay membrane bound or potentially internalized via endocytosis. (C) Macrophage FRβ can also be subject to therapeutic targeting to ameliorate inflammation. This can be achieved by (drug-conjugated) monoclonal antibodies, folate-conjugated drugs, CAR-T cells targeted towards FRβ and with small molecule folate antagonists. (D) Folic acid, the primary circulating plasma folate form 5-CH3-THF, and folate antagonist therapeutic drugs MTX or PMX can bind to FRβ or one of the two other folate carriers expressed on macrophages, i.e. RFC/SLC19A1 and PCFT/SLC46A1. The binding affinity of 5-CH3-THF, MTX and PMX varies for FRβ, RFC and PCFT, respectively, as indicated by colored arrows. For example, 5-CH3-THF binding affinity to FRβ > MTX and PMX. MTX transport is facilitated by all three folate carriers, but with slightly higher affinities for RFC and PCFT. PMX displays the highest affinity to PCFT, moderate affinity to RFC and the lowest affinity for FRβ. (E) Chemical structures of folic acid, (6S)-5CH3-THF, methotrexate (MTX) and pemetrexed (PMX) illustrating the shared pterin moiety which is captured in the folic acid binding cleft of FRβ. (F) Chemical structure of [18F]fluoro-PEG-Folate, the folate PET imaging agent for high-affinity binding to FRβ on macrophages and utilization for diagnostics and therapy response monitoring.
Macrophage FRβ is a valid target for folate-based imaging (Figure 1B), along with other FRβ targeting approaches (Figure 1C) by (drug-conjugated) monoclonal antibodies, folate-conjugated drugs, CAR T cells and folate antagonists (25, 31, 34, 50, 51). FRβ contains a binding pocket for folic acid where binding of folic acid is facilitated by a conformational change in two regions of the receptor, in particular the region connecting beta strand 1 and 2 and the region of alpha helix 1 (52). Like folic acid, folate antagonist therapeutic drugs such as methotrexate (MTX, the anchor drug in RA treatment) (53, 54) and pemetrexed (PMX) (55) share a pterin moiety in their structure which can bind in the hydrophobic region of the binding pocket of FRβ (52) (Figure 1D). FRα and FRβ share high affinity binding of folic acid with Kd’s in the low nanomolar range (0.1-1 nM) (36). Structure activity testing disclosed anti-folate structures with FRα and FRβ affinities close to folic acid, 2-3 orders of magnitude lower affinities for MTX than folic acid and intermediate affinities for PMX (25, 56).
In order to elicit therapeutic activity against macrophages, a folate antagonist should compete with FRβ binding of the primary circulating reduced folate in plasma, 5-methyltetrahydrofolate (5-CH3-THF) (25). Also FRβ functions as one of three transport proteins for folates and antifolates, the two others are the reduced folate carrier (RFC, SLC19A1) and proton-coupled folate transporter (PCFT, SLC46A1) (57) (Figure 1E). The expression of these 3 folate transporters does vary between polarized macrophages; in ex vivo skewed monocytes to M1-type macrophages by GM-CSF and M2-type macrophage by M-CSF, RFC gene expression is differentially higher in M1-type macrophages, whereas FRβ and PCFT expression are markedly higher in M2-type macrophages (27, 28). Given that FRα/β also retain a high affinity binding profile for folate conjugates, this allowed the design of folate conjugates which could serve as folate based imaging agents like [18F]fluoro-PEG-folate (Figure 1F) (58–60).
1.3 PET Imaging of Macrophages
Over the past decade, non-invasive molecular imaging techniques that assess RA disease activity using macrophage PET imaging have been developed (31, 58, 61–63). Disease activity assessment of RA is currently performed clinically through calculation of the DAS, which takes into account the number of swollen and tender joints, the erythrocyte sedimentation rate (ESR) and the visual analogue score (VAS) (1). However, the DAS score contains subjective elements and is limited to the sensitivity and specificity of clinical assessment of tenderness and swelling of the joints (64). To make further steps in improvement of diagnostics and monitoring of disease activity, objective tools such as macrophage (whole body) PET imaging may offer new opportunities for early diagnosis and early determination of the treatment outcome. Early diagnosis (even before clinical diagnosis) and effective, personalized treatment may ultimately result in prevention of (progression of) joint damage (1).
Initially, imaging studies were performed for FRα-expressing tumors with folate-SPECT tracer [99Tc]EC20 (48). By serendipity, imaging of a cancer patient who had an arthritic comorbidity showed a positive scan of the inflamed knee joint due to infiltration of FRβ-positive macrophages (65). This was the start of exploration of arthritis imaging by FRβ targeting. The easy accessibility as GPI-linked plasma membrane protein and its myeloid cell specific expression constitutes FRβ a suitable target for macrophage imaging. [99Tc]EC20 proved its suitability in visualizing FRβ-positive macrophages in inflamed joints of arthritic rats (66) and RA patients (67). These findings encouraged the development of folate-based PET tracer which would provide a higher sensitivity and spatial resolution compared to scintigraphy.
The original synthesis of folate-based PET tracers relied on chemistry linking folic acid with an spacer moiety (polyethylene glycol) as a precursor molecule to which the PET isotope is coupled (68). This approach was adopted in studies by Gent et al. (58) and Kularatne et al. (59) to synthesize [18F]fluoro-PEG-folate (Figure 1F). Assessment of FR binding affinity for fluoro-PEG-folate in a [3H]folic acid competition assay showed that the unlabeled tracer had a 2-fold lower affinity than for folic acid, but a 2.5-fold higher affinity than for the circulating plasma folate 5CH3-THF (58). After fulfilling this important criterium of high affinity binding and outcompeting binding of circulating plasma 5-CH3-THF, next [18F]fluoro-PEG-folate was examined further for in vivo PET–based monitoring of disease activity and therapy response in a preclinical model of RA (see section 2).
2 Folate Receptor β-Targeted Imaging in the Antigen-Induced Arthritis Model
2.1 The Antigen-Induced Arthritis Model
In the preclinical assessment of FRβ as a macrophage target for PET imaging of arthritis, Chandrupatla et al. established a pre-clinical rat model of arthritis with sustained macrophage infiltration in the joints (69). In this model arthritis is induced through immunization with methylated bovine serum albumin (mBSA) in complete Freund’s adjuvant (CFA) and custom Bordetella pertussis antigen (CBP) following one or repeated intra-articular (i.a.) injections with mBSA in one knee of the rat (leaving the contralateral knee as control), after which synovial inflammation accompanied by an increase in knee thickness occurs over 3 days (Figures 2A, B) (69). This antigen-induced arthritis model was selected for PET imaging of molecular markers in arthritis and therapeutic evaluations due to several reasons; (i) the model is mono-articular, and hence the contra-lateral and other joints can serve as control, (ii) the model is relatively mild and is not accompanied by severe bone destruction or polyarticular involvement, (iii) the model is also reminiscent of human RA featuring macrophage infiltration (71, 72) (Figure 2C) and moderate systemic inflammation manifested by modest macrophage infiltration in the liver and spleen (70), (iv) macrophage infiltration can be sustained by repeated mBSA injections, which is a good condition for therapeutic monitoring (69), and (v) the larger size of the rat allows injecting more radioactivity and the larger knee size of the rat (compared to a mouse) is advantageous given the spatial resolution of most PET scanners. This model therefore allows for studying if [18F]fluoro-PEG-folate is a suitable tracer for objective macrophage imaging in arthritis affected joints and for longitudinally studying the effect of anti-rheumatic drugs on joint macrophage infiltration (58, 62, 70).
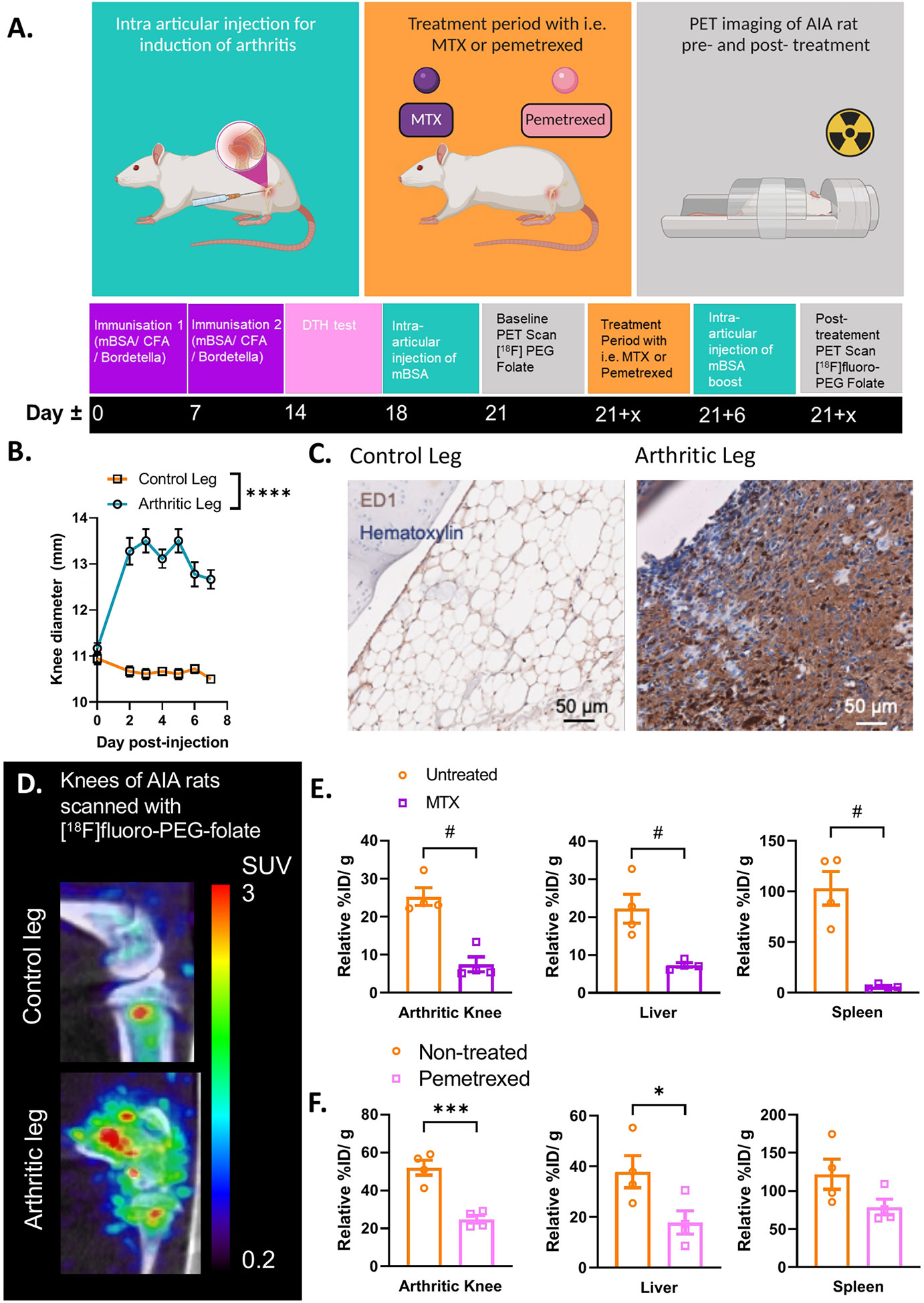
Figure 2 Antigen induced arthritis (AIA) rat model used in preclinical studies for PET imaging with [18F]fluoro-PEG-folate tracer. (A) Schematic representation of the preclinical set up of the experiment: starting at day 0 and day 7 with an immunization of the rat with an emulsion of Complete Freund’s Adjuvant, Custom Bordetella Pertussis and methylated BSA (mBSA). Immunization status is checked at day 14 with a delayed type hypersensitivity (DTH) test through injection of mBSA in the ear. Around day 18, an intra-articular (i.a.) injection is given in one of the knees with mBSA. During the whole duration of the experiment or after i.a. injection, the rat can be treated with FRβ-targeted folate antagonist such as MTX or PMX. A baseline (/pre-treatment) PET scan and post-treatment PET scan with [18F]fluoro-PEG-folate have been used to monitor the effect of antifolate therapy. (B) After 1-3 days post i.a. injection a significant swelling of the knee diameter is seen in the arthritis-affected leg (****p < 0.0001, two-way ANOVA, N = 9 rats/group, paired samples). (C) Representative image of macrophage infiltration in the synovium of the arthritic leg as detected by immunohistochemical (IHC) DAB-staining of rat knee tissue with ED-1 antibody (HM3029, Hycult Biotech) (scale bar = 50μm). (D) Illustrative image of increased [18F]fluoro-PEG-folate uptake in the arthritic knee (lower panel, arthritic leg) vs. the non-arthritic, contra-lateral knee (upper panel, control leg) of an AIA rat. Both images are scaled to the same standard uptake value based on the injected dose (in MBq/ml) of the tracer and the body weight of the animal (in g.). (E) Biodistribution of [18F]fluoro-PEG-folate in the arthritic knee, liver and spleen of non-treated and MTX-treated AIA rats. Data were corrected for blood %ID/g. (F) Biodistribution of [18F]fluoro-PEG-folate in the arthritic knee, liver and spleen of non-treated and PMX-treated AIA rats. Data were corrected for blood %ID/g. Statistics for images E-F were performed in Graph-Pad Prism version 9, #p < 0.01, Mann-Whitney U test for non-parametric divided data, *p < 0.05, ***p < 0.001, unpaired T-TEST for parametric divided data, N = 4 rats/group. All results described in Figure 2 were derived (and reanalyzed were indicated) from own research (58, 62, 69, 70).
2.2 FRβ-Targeted Macrophage PET Imaging in Arthritic Rats
Initially, imaging for FRβ in preclinical arthritis models and RA patients was performed with the SPECT tracer [99mTc]EC20 (66, 67). It has only been up until recently that the [18F]-PEG-folate tracer has been tested pre-clinically in a model of arthritis (i.e. the AIA model). Gent et al. showed that in arthritic rats scanned with [18F]fluoro-PEG-folate had a ~50% increased tracer uptake in the arthritic knee compared to the contralateral uninflamed knee joint (58) (see illustrative image Figure 2D). Gent et al. also showed that the [18F]fluoro-PEG-folate tracer specifically targeted folate receptors since blocking of this receptor with unlabeled glucosamine-folate significantly abolished [18F]fluoro-PEG-folate uptake in the arthritic knees (58).
Treatment of arthritic rats with different dosages of the folate antagonist MTX significantly reduced (~2-4 fold) tracer uptake in the arthritic knee compared to the arthritic knee of untreated rats (62, 70) (Figure 2E). Consistently, histological analysis demonstrated that macrophage infiltration was also ~2-4 fold reduced in the arthritic knee as well as in the liver and spleen following MTX therapy (70). Involvement of liver and spleen point to systemic inflammation in the arthritic rat model, which is suppressed by MTX treatment (70). This is in line with the systemic character of RA in patients (73). Similar results were obtained after treatment of arthritic rats with the second-generation folate antagonist pemetrexed (PMX)/Alimta (74). [18F]fluoro-PEG-folate uptake in the arthritic knee, liver and spleen of PMX treated rats was reduced ~2.2; 3.2 and 1.6-fold, respectively compared to untreated rats (Figure 2F). PMX was originally developed to overcome MTX resistance in cancer chemotherapy (55) by harboring more efficient transport properties via RFC, PCFT and FR than MTX (Figure 1C). PMX has shown anti-arthritic effects by suppressing cytokine production in activated T cells of RA patients (75), experimental arthritis models (74) and polarized macrophages in vitro (28). Together, these preclinical studies demonstrate the suitability and feasibility of macrophage PET with [18F]fluoro-PEG-folate for arthritis disease monitoring and therapy response monitoring thereby supporting clinical studies in RA. Preclinical animal studies also revealed that [18F]fluoro-PEG-folate may provide improved arthritis imaging compared to an established macrophage translocator protein (TSPO) tracer (R)-[11C]PK11195 (58) as a 2.3-fold higher arthritic knee over blood ratios was observed for [18F]fluoro-PEG-folate than for (R)-[11C]PK11195.
3 Clinical Evaluation of Folate PET Imaging in RA Patients
3.1 First Clinical Study With [18F]fluoro-PEG-Folate in RA Patients
Immuno-histochemical and immuno-fluorescent analysis of FRβ expression in RA synovial biopsies showed marked expression of double CD68 and FRβ-positive macrophages in both lining and sublining of RA synovial tissue (representative example shown in Figure 3A) (25), thus encouraging further clinical evaluation of the [18F]fluoro-PEG-folate PET tracer. The first clinical evaluation of the [18F]fluoro-PEG-folate PET tracer for arthritis was performed by Verweij et al. in RA patients (61). This study showed uptake of the [18F]fluoro PEG folate PET tracer in clinically active joints (Figure 3B). Although the absolute tracer uptake of [18F]fluoro-PEG-folate in arthritic joints was ~ 2.5-fold lower than for the previously investigated macrophage tracer (R)-[11C]-PK11195, the target-to-background ratios of [18F]fluoro-PEG-folate PET-CT were significantly higher (3.5 ± 2.2 versus 1.7 ± 0.6; p<0.02; n=6 patients) (61). This was a relevant improvement regarding clinical application of macrophage PET imaging in RA, since PK11195 (first generation TSPO) imaging in RA is limited by relatively high background uptake in bone marrow and peri-articular tissues (e.g. muscle) (76). As a consequence, more subtle arthritis activity can easily be missed, which is particularly relevant for early disease assessment and highly sensitive monitoring of therapeutic efficacy. Both clinically and sub-clinically inflamed joints were imaged by folate PET-CT with lower false positive and false negative findings (as compared to clinical findings) than PK11195 PET-CT (61). This holds promise for [18F]fluoro-PEG-folate PET-CT in terms of potential predictive value in clinical RA diagnosis and development of relapse in established disease, since previous studies with PK11195 PET-CT already demonstrated predictive value for these clinical applications (76, 77), which thus may be further improved using [18F]fluoro-PEG-folate whole body PET-CT. Our preliminary data also point at the potential [18F]fluoro-PEG-folate PET-CT to monitor treatment efficacy of anti-rheumatic drugs including of anti-folates (70). [18F]fluoro-PEG-folate binding affinity towards FRβ outweighs methotrexate by at least 2–3 orders of magnitude (26, 58). If, additionally, a safe time window of 7 days is applied between last methotrexate administration and [18F]fluoro-PEG-folate, no blockade of [18F]fluoro-PEG-folate binding in arthritic joints of RA patients by anti-folates (61).
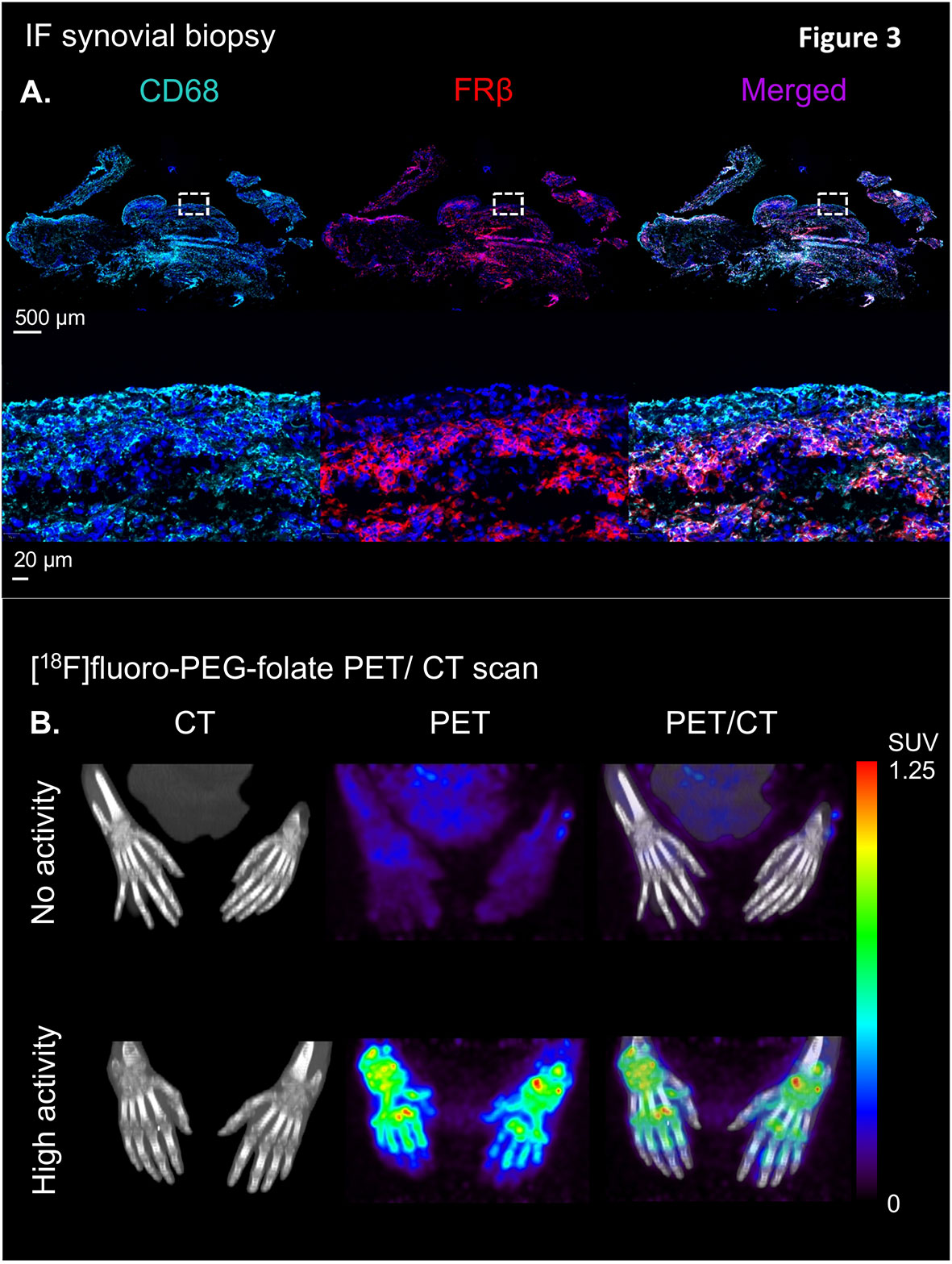
Figure 3 Illustration of the clinical validation of the [18F]fluoro-PEG-folate tracer in patients with rheumatoid arthritis. (A) Upper panel showing an illustrative example of immunofluorescent staining of CD68 (left), FRβ (middle) and CD68 + FRβ merged staining in a synovial knee biopsy of an RA patient with active disease. Lower panel depicts marked expression of CD68- and FRβ-positive macrophages in both synovial lining and sublining (see the zoomed view of the dashed marked box in the upper panel of the RA synovial tissue. (B) Representative example of high specific uptake of [18F]fluoro-PEG-folate in the hand joints of a patient with high RA disease activity vs. marginal uptake in the hand joints of a RA patient with low disease activity in the hands. Both images are scaled to the same standard uptake value based on the injected dose (in MBq/ml) of the tracer and the body weight of the patient (in kg.). All representative images of Figure 3 were derived from own research (25, 61).
3.2 Future Perspectives
Given the fact that macrophage PET imaging with FRβ has shown clinical feasibility in RA patients, future challenges will be to use folate PET tracers for detection of disease activity in early stage RA and monitoring/prediction of the therapy response of targeted synthetic or biological DMARDs in joints and other sides affected by systemic inflammation. Furthermore, many other inflammatory diseases with macrophage involvement, e.g. idiopathic pulmonary fibrosis, systemic lupus erythematosus, scleroderma, psoriasis, ulcerative colitis, Crohn’s disease (78, 79), giant cell arteritis (80), cardiovascular diseases (81, 82) and tumor associated macrophages in oncology (43) may benefit from folate PET imaging to detect and monitor disease activity. Recently, also folate PET imaging of lung macrophages in COVID-19 was advocated to identify patients at risk of a severe or even lethal disease (83). Steps forward in the folate-linker chemistry also allow for more rapid synthesis of alternative folate tracers from precursor molecules, e.g. [18F]folate-PEG-NOTA-Al (84), which are promising but warrant further (pre)clinical evaluation. Beyond PET tracers, there is also increasing interest in the development of folate near- infrared/optical imaging agents for FRβ targeting in oncology and inflammatory diseases (85, 86). Altogether, FRβ remains a reputable target for continued research and (pre)clinical testing of imaging and therapeutic agents in a wide range of pathological conditions.
Author Contributions
MS, AE, GJ, and CL: conceptual design, drafting of the article, critical scientific revision and approval of final version. MS: design and scientific translation of the reviewed literature to all figures. MS, FK, CM, PL, GZ, MY, WB, AW, ST, GJ and CL: critical revision and approval of the final version. All authors contributed to the article and approved the submitted version.
Funding
The work of this review was supported in part by grant from the Center for Translationational Molecular Medicine (CTMM TRACER), the Dutch Rheumatism Fund (ReumaNederland, NRF 09-01-404), Cancer Center Amsterdam, and EU-Marie Curie ARCAID program.
Conflict of Interest
The authors declare that the research was conducted in the absence of any commercial or financial relationships that could be construed as a potential conflict of interest.
Publisher’s Note
All claims expressed in this article are solely those of the authors and do not necessarily represent those of their affiliated organizations, or those of the publisher, the editors and the reviewers. Any product that may be evaluated in this article, or claim that may be made by its manufacturer, is not guaranteed or endorsed by the publisher.
Acknowledgments
The authors greatly appreciate the technical assistance of Elise Mantel, Maxime Schreurs, Mariska Verlaan, Yik Kan, Martien Mooijer, Rolf van Kooii and Esther Kooijman at the laboratories of the Amsterdam University Medical Center and Nuclear Medicine Laboratories. Furthermore, we would like to thank Michiel Stork and René Raeven at Intravacc (Bilthoven) for kindly providing CBP for the AIA animal model, and Guus van Dongen, Daniëlle Vugts and Marc Huisman for their advice regarding the (AIA) animal PET experiments. Illustrations were created as original figures specific for this review by using BioRender.com.
References
1. Smolen JS, Aletaha D, Barton A, Burmester GR, Emery P, Firestein GS, et al. Rheumatoid Arthritis. Nat Rev Dis Primers (2018) 4:18001. doi: 10.1038/nrdp.2018.1
2. Boutet MA, Courties G, Nerviani A, Le Goff B, Apparailly F, Pitzalis C, et al. Novel Insights Into Macrophage Diversity in Rheumatoid Arthritis Synovium. Autoimmun Rev (2021) 20(3):102758. doi: 10.1016/j.autrev.2021.102758
3. Brennan FM, McInnes IB. Evidence That Cytokines Play a Role in Rheumatoid Arthritis. J Clin Invest (2008) 118(11):3537–45. doi: 10.1172/JCI36389
4. McInnes IB, Schett G. Cytokines in the Pathogenesis of Rheumatoid Arthritis. Nat Rev Immunol (2007) 7(6):429–42. doi: 10.1038/nri2094
5. Jahangier ZN, Jacobs JW, Kraan MC, Wenting MJ, Smeets TJ, Bijlsma JW, et al. Pretreatment Macrophage Infiltration of the Synovium Predicts the Clinical Effect of Both Radiation Synovectomy and Intra-Articular Glucocorticoids. Ann Rheumatic Dis (2006) 65(10):1286–92. doi: 10.1136/ard.2005.042333
6. Haringman JJ, Gerlag DM, Zwinderman AH, Smeets TJ, Kraan MC, Baeten D, et al. Synovial Tissue Macrophages: A Sensitive Biomarker for Response to Treatment in Patients With Rheumatoid Arthritis. Ann Rheumatic Dis (2005) 64(6):834–8. doi: 10.1136/ard.2004.029751
8. Orr C, Vieira-Sousa E, Boyle DL, Buch MH, Buckley CD, Canete JD, et al. Synovial Tissue Research: A State-of-the-Art Review. Nat Rev Rheumatol (2017) 13(8):463–75. doi: 10.1038/nrrheum.2017.115
9. Hamilton JA, Tak PP. The Dynamics of Macrophage Lineage Populations in Inflammatory and Autoimmune Diseases. Arthritis Rheum (2009) 60(5):1210–21. doi: 10.1002/art.24505
10. Kennedy A, Fearon U, Veale DJ, Godson C. Macrophages in Synovial Inflammation. Front Immunol (2011) 2:52. doi: 10.3389/fimmu.2011.00052
11. Zhang Q, Yuan R, Li C, Wei W, Shen W, Cui Y, et al. Macrophage Depletion With Clodronate-Containing Liposomes Affects the Incidence and Development of Rheumatoid Arthritis. Z Rheumatol (2019) 78(10):996–1003. doi: 10.1007/s00393-018-0563-x
12. Li J, Hsu HC, Yang P, Wu Q, Li H, Edgington LE, et al. Treatment of Arthritis by Macrophage Depletion and Immunomodulation: Testing an Apoptosis-Mediated Therapy in a Humanized Death Receptor Mouse Model. Arthritis Rheum (2012) 64(4):1098–109. doi: 10.1002/art.33423
13. Bresnihan B, Pontifex E, Thurlings RM, Vinkenoog M, El-Gabalawy H, Fearon U, et al. Synovial Tissue Sublining CD68 Expression Is a Biomarker of Therapeutic Response in Rheumatoid Arthritis Clinical Trials: Consistency Across Centers. J Rheumatol (2009) 36(8):1800–2. doi: 10.3899/jrheum.090348
14. Humby F, Lewis M, Ramamoorthi N, Hackney JA, Barnes MR, Bombardieri M, et al. Synovial Cellular and Molecular Signatures Stratify Clinical Response to csDMARD Therapy and Predict Radiographic Progression in Early Rheumatoid Arthritis Patients. Ann Rheumatic Dis (2019) 78(6):761–72. doi: 10.1136/annrheumdis-2018-214539
15. Pitzalis C, Kelly S, Humby F. New Learnings on the Pathophysiology of RA From Synovial Biopsies. Curr Opin Rheumatol (2013) 25(3):334–44. doi: 10.1097/BOR.0b013e32835fd8eb
16. Dennis G Jr, Holweg CT, Kummerfeld SK, Choy DF, Setiadi AF, Hackney JA, et al. Synovial Phenotypes in Rheumatoid Arthritis Correlate With Response to Biologic Therapeutics. Arthritis Res Ther (2014) 16(2):R90. doi: 10.1186/ar4555
17. Ross EA, Devitt A, Johnson JR. Macrophages: The Good, the Bad, and the Gluttony. Front Immunol (2021) 12:708186. doi: 10.3389/fimmu.2021.708186
18. Wang N, Liang H, Zen K. Molecular Mechanisms That Influence the Macrophage M1-M2 Polarization Balance. Front Immunol (2014) 5:614. doi: 10.3389/fimmu.2014.00614
19. Ohradanova-Repic A, Machacek C, Charvet C, Lager F, Le Roux D, Platzer R, et al. Extracellular Purine Metabolism Is the Switchboard of Immunosuppressive Macrophages and a Novel Target to Treat Diseases With Macrophage Imbalances. Front Immunol (2018) 9:852. doi: 10.3389/fimmu.2018.00852
20. Fuentelsaz-Romero S, Cuervo A, Estrada-Capetillo L, Celis R, Garcia-Campos R, Ramirez J, et al. GM-CSF Expression and Macrophage Polarization in Joints of Undifferentiated Arthritis Patients Evolving to Rheumatoid Arthritis or Psoriatic Arthritis. Front Immunol (2020) 11:613975. doi: 10.3389/fimmu.2020.613975
21. Smiljanovic B, Grutzkau A, Sorensen T, Grun JR, Vogl T, Bonin M, et al. Synovial Tissue Transcriptomes of Long-Standing Rheumatoid Arthritis Are Dominated by Activated Macrophages That Reflect Microbial Stimulation. Sci Rep (2020) 10(1):7907. doi: 10.1038/s41598-020-64431-4
22. Navegantes KC, de Souza Gomes R, Pereira PAT, Czaikoski PG, Azevedo CHM, Monteiro MC. Immune Modulation of Some Autoimmune Diseases: The Critical Role of Macrophages and Neutrophils in the Innate and Adaptive Immunity. J Transl Med (2017) 15(1):36. doi: 10.1186/s12967-017-1141-8
23. Alivernini S, MacDonald L, Elmesmari A, Finlay S, Tolusso B, Gigante MR, et al. Distinct Synovial Tissue Macrophage Subsets Regulate Inflammation and Remission in Rheumatoid Arthritis. Nat Med (2020) 26(8):1295–306. doi: 10.1038/s41591-020-0939-8
24. Xia W, Hilgenbrink AR, Matteson EL, Lockwood MB, Cheng JX, Low PS. A Functional Folate Receptor Is Induced During Macrophage Activation and Can Be Used to Target Drugs to Activated Macrophages. Blood (2009) 113(2):438–46. doi: 10.1182/blood-2008-04-150789
25. van der Heijden JW, Oerlemans R, Dijkmans BA, Qi H, van der Laken CJ, Lems WF, et al. Folate Receptor Beta as a Potential Delivery Route for Novel Folate Antagonists to Macrophages in the Synovial Tissue of Rheumatoid Arthritis Patients. Arthritis Rheum (2009) 60(1):12–21. doi: 10.1002/art.24219
26. Puig-Kroger A, Sierra-Filardi E, Dominguez-Soto A, Samaniego R, Corcuera MT, Gomez-Aguado F, et al. Folate Receptor Beta Is Expressed by Tumor-Associated Macrophages and Constitutes a Marker for M2 Anti-Inflammatory/Regulatory Macrophages. Cancer Res (2009) 69(24):9395–403. doi: 10.1158/0008-5472.CAN-09-2050
27. Municio C, Soler Palacios B, Estrada-Capetillo L, Benguria A, Dopazo A, Garcia-Lorenzo E, et al. Methotrexate Selectively Targets Human Proinflammatory Macrophages Through a Thymidylate Synthase/P53 Axis. Ann Rheumatic Dis (2016) 75(12):2157–65. doi: 10.1136/annrheumdis-2015-208736
28. Fuentelsaz-Romero S, Barrio-Alonso C, García Campos R, Torres Torresano M, Muller IB, Triguero-Martínez A, et al. The Macrophage Reprogramming Ability of Antifolates Reveals Soluble CD14 as a Potential Biomarker for Methotrexate Response in Rheumatoid Arthritis. Front Immunol (2021) 12(4580). doi: 10.3389/fimmu.2021.776879
29. Vogelpoel LT, Hansen IS, Rispens T, Muller FJ, van Capel TM, Turina MC, et al. Fc Gamma Receptor-TLR Cross-Talk Elicits Pro-Inflammatory Cytokine Production by Human M2 Macrophages. Nat Commun (2014) 5:5444. doi: 10.1038/ncomms6444
30. Clavel C, Ceccato L, Anquetil F, Serre G, Sebbag M. Among Human Macrophages Polarised to Different Phenotypes, the M-CSF-Oriented Cells Present the Highest Pro-Inflammatory Response to the Rheumatoid Arthritis-Specific Immune Complexes Containing ACPA. Ann Rheumatic Dis (2016) 75(12):2184–91. doi: 10.1136/annrheumdis-2015-208887
31. Chandrupatla D, Molthoff CFM, Lammertsma AA, van der Laken CJ, Jansen G. The Folate Receptor Beta as a Macrophage-Mediated Imaging and Therapeutic Target in Rheumatoid Arthritis. Drug Deliv Transl Res (2019) 9(1):366–78. doi: 10.1007/s13346-018-0589-2
32. Lu YJ, Wheeler LW 2nd, Chu H, Kleindl PJ, Pugh M, You F, et al. Targeting Folate Receptor Beta on Monocytes/Macrophages Renders Rapid Inflammation Resolution Independent of Root Causes. Cell Rep Med (2021) 2(10):100422. doi: 10.1016/j.xcrm.2021.100422
33. Ducker GS, Rabinowitz JD. One-Carbon Metabolism in Health and Disease. Cell Metab (2017) 25(1):27–42. doi: 10.1016/j.cmet.2016.08.009
34. Frigerio B, Bizzoni C, Jansen G, Leamon CP, Peters GJ, Low PS, et al. Folate Receptors and Transporters: Biological Role and Diagnostic/Therapeutic Targets in Cancer and Other Diseases. J Exp Clin Cancer Res (2019) 38(1):125. doi: 10.1186/s13046-019-1123-1
35. Dekhne AS, Hou Z, Gangjee A, Matherly LH. Therapeutic Targeting of Mitochondrial One-Carbon Metabolism in Cancer. Mol Cancer Ther (2020) 19(11):2245–55. doi: 10.1158/1535-7163.MCT-20-0423
36. Elnakat H, Ratnam M. Distribution, Functionality and Gene Regulation of Folate Receptor Isoforms: Implications in Targeted Therapy. Adv Drug Deliv Rev (2004) 56(8):1067–84. doi: 10.1016/j.addr.2004.01.001
37. Wu M, Gunning W, Ratnam M. Expression of Folate Receptor Type Alpha in Relation to Cell Type, Malignancy, and Differentiation in Ovary, Uterus, and Cervix. Cancer Epidemiol Biomarkers Prev (1999) 8(9):775–82.
38. Shen F, Wu M, Ross JF, Miller D, Ratnam M. Folate Receptor Type Gamma Is Primarily a Secretory Protein Due to Lack of an Efficient Signal for Glycosylphosphatidylinositol Modification: Protein Characterization and Cell Type Specificity. Biochemistry (1995) 34(16):5660–5. doi: 10.1021/bi00016a042
39. Holm J, Hansen SI. Characterization of Soluble Folate Receptors (Folate Binding Proteins) in Humans. Biological Roles and Clinical Potentials in Infection and Malignancy. Biochim Biophys Acta Proteins Proteom (2020) 1868(10):140466. doi: 10.1016/j.bbapap.2020.140466
40. Parker N, Turk MJ, Westrick E, Lewis JD, Low PS, Leamon CP. Folate Receptor Expression in Carcinomas and Normal Tissues Determined by a Quantitative Radioligand Binding Assay. Anal Biochem (2005) 338(2):284–93. doi: 10.1016/j.ab.2004.12.026
41. Crozier JA, Necela BM, Thompson EA, Geiger X, Moreno-Aspitia A, McCullough AE, et al. Increased Expression of Folate Receptor-α (FRA) in Triple-Negative Breast Cancer: A Potential Therapeutic Target. J Clin Oncol (2013) 31(15_suppl):1037. doi: 10.1200/jco.2013.31.15_suppl.1037
42. Toffoli G, Cernigoi C, Russo A, Gallo A, Bagnoli M, Boiocchi M. Overexpression of Folate Binding Protein in Ovarian Cancers. Int J Cancer (1997) 74(2):193–8. doi: 10.1002/(SICI)1097-0215(19970422)74:2<193::AID-IJC10>3.0.CO;2-F
43. Shen J, Hu Y, Putt KS, Singhal S, Han H, Visscher DW, et al. Assessment of Folate Receptor Alpha and Beta Expression in Selection of Lung and Pancreatic Cancer Patients for Receptor Targeted Therapies. Oncotarget (2018) 9(4):4485–95. doi: 10.18632/oncotarget.23321
44. Ross JF, Wang H, Behm FG, Mathew P, Wu M, Booth R, et al. Folate Receptor Type Beta Is a Neutrophilic Lineage Marker and Is Differentially Expressed in Myeloid Leukemia. Cancer (1999) 85(2):348–57. doi: 10.1002/(SICI)1097-0142(19990115)85:2<348::AID-CNCR12>3.0.CO;2-4
45. Shen J, Hilgenbrink AR, Xia W, Feng Y, Dimitrov DS, Lockwood MB, et al. Folate Receptor-Beta Constitutes a Marker for Human Proinflammatory Monocytes. J Leukocyte Biol (2014) 96(4):563–70. doi: 10.1189/jlb.2AB0713-372R
46. Samaniego R, Dominguez-Soto A, Ratnam M, Matsuyama T, Sanchez-Mateos P, Corbi AL, et al. Folate Receptor Beta (FRbeta) Expression in Tissue-Resident and Tumor-Associated Macrophages Associates With and Depends on the Expression of PU.1. Cells (2020) 9(6):1445. doi: 10.3390/cells9061445
47. Spiegelstein O, Eudy JD, Finnell RH. Identification of Two Putative Novel Folate Receptor Genes in Humans and Mouse. Gene (2000) 258(1-2):117–25. doi: 10.1016/S0378-1119(00)00418-2
48. Low PS, Kularatne SA. Folate-Targeted Therapeutic and Imaging Agents for Cancer. Curr Opin Chem Biol (2009) 13(3):256–62. doi: 10.1016/j.cbpa.2009.03.022
49. Tian Y, Wu G, Xing JC, Tang J, Zhang Y, Huang ZM, et al. A Novel Splice Variant of Folate Receptor 4 Predominantly Expressed in Regulatory T Cells. BMC Immunol (2012) 13:30. doi: 10.1186/1471-2172-13-30
50. Rodriguez-Garcia A, Lynn RC, Poussin M, Eiva MA, Shaw LC, O’Connor RS, et al. CAR-T Cell-Mediated Depletion of Immunosuppressive Tumor-Associated Macrophages Promotes Endogenous Antitumor Immunity and Augments Adoptive Immunotherapy. Nat Commun (2021) 12(1):877. doi: 10.1038/s41467-021-20893-2
51. Roy AG, Robinson JM, Sharma P, Rodriguez-Garcia A, Poussin MA, Nickerson-Nutter C, et al. Folate Receptor Beta as a Direct and Indirect Target for Antibody-Based Cancer Immunotherapy. Int J Mol Sci (2021) 22(11):5572. doi: 10.3390/ijms22115572
52. Wibowo AS, Singh M, Reeder KM, Carter JJ, Kovach AR, Meng W, et al. Structures of Human Folate Receptors Reveal Biological Trafficking States and Diversity in Folate and Antifolate Recognition. Proc Natl Acad Sci USA (2013) 110(38):15180–8. doi: 10.1073/pnas.1308827110
53. Brown PM, Pratt AG, Isaacs JD. Mechanism of Action of Methotrexate in Rheumatoid Arthritis, and the Search for Biomarkers. Nat Rev Rheumatol (2016) 12(12):731–42. doi: 10.1038/nrrheum.2016.175
54. Nakashima-Matsushita N, Homma T, Yu S, Matsuda T, Sunahara N, Nakamura T, et al. Selective Expression of Folate Receptor Beta and Its Possible Role in Methotrexate Transport in Synovial Macrophages From Patients With Rheumatoid Arthritis. Arthritis Rheum (1999) 42(8):1609–16. doi: 10.1002/1529-0131(199908)42:8<1609::AID-ANR7>3.0.CO;2-L
55. Shih C, Chen VJ, Gossett LS, Gates SB, MacKellar WC, Habeck LL, et al. LY231514, A Pyrrolo[2,3-D]Pyrimidine-Based Antifolate That Inhibits Multiple Folate-Requiring Enzymes. Cancer Res (1997) 57(6):1116–23.
56. Westerhof GR, Schornagel JH, Kathmann I, Jackman AL, Rosowsky A, Forsch RA, et al. Carrier- and Receptor-Mediated Transport of Folate Antagonists Targeting Folate-Dependent Enzymes: Correlates of Molecular-Structure and Biological Activity. Mol Pharmacol (1995) 48(3):459–71.
57. Zhao R, Matherly LH, Goldman ID. Membrane Transporters and Folate Homeostasis: Intestinal Absorption and Transport Into Systemic Compartments and Tissues. Expert Rev Mol Med (2009) 11:e4. doi: 10.1017/S1462399409000969
58. Gent YY, Weijers K, Molthoff CF, Windhorst AD, Huisman MC, Smith DE, et al. Evaluation of the Novel Folate Receptor Ligand [18F]Fluoro-PEG-Folate for Macrophage Targeting in a Rat Model of Arthritis. Arthritis Res Ther (2013) 15(2):R37. doi: 10.1186/ar4191
59. Kularatne SA, Belanger MJ, Meng X, Connolly BM, Vanko A, Suresch DL, et al. Comparative Analysis of Folate Derived PET Imaging Agents With [(18)F]-2-Fluoro-2-Deoxy-D-Glucose Using a Rodent Inflammatory Paw Model. Mol Pharm (2013) 10(8):3103–11. doi: 10.1021/mp4001684
60. Samaniego R, Palacios BS, Domiguez-Soto A, Vidal C, Salas A, Matsuyama T, et al. Macrophage Uptake and Accumulation of Folates Are Polarization-Dependent In Vitro and In Vivo and Are Regulated by Activin A. J Leukocyte Biol (2014) 95(5):797–808. doi: 10.1189/jlb.0613345
61. Verweij NJF, Yaqub M, Bruijnen STG, Pieplenbosch S, Ter Wee MM, Jansen G, et al. First in Man Study of [(18)F]fluoro-PEG-Folate PET: A Novel Macrophage Imaging Technique to Visualize Rheumatoid Arthritis. Sci Rep (2020) 10(1):1047. doi: 10.1038/s41598-020-57841-x
62. Chandrupatla D, Jansen G, Vos R, Verlaan M, Chen Q, Low PS, et al. In-Vivo Monitoring of Anti-Folate Therapy in Arthritic Rats Using [(18)F]fluoro-PEG-Folate and Positron Emission Tomography. Arthritis Res Ther (2017) 19(1):114. doi: 10.1186/s13075-017-1325-x
63. van der Krogt JMA, van Binsbergen WH, van der Laken CJ, Tas SW. Novel Positron Emission Tomography Tracers for Imaging of Rheumatoid Arthritis. Autoimmun Rev (2021) 20(3):102764. doi: 10.1016/j.autrev.2021.102764
64. van Gestel AM, Prevoo ML, van ‘t Hof MA, van Rijswijk MH, van de Putte LB, van Riel PL. Development and Validation of the European League Against Rheum Response Criteria for Rheumatoid Arthritis. Comparison With the Preliminary American College of Rheumatology and the World Health Organization/International League Against Rheumatism Criteria. Arthritis Rheumatism (1996) 39(1):34–40. doi: 10.1002/art.1780390105
65. Paulos CM, Turk MJ, Breur GJ, Low PS. Folate Receptor-Mediated Targeting of Therapeutic and Imaging Agents to Activated Macrophages in Rheumatoid Arthritis. Adv Drug Deliv Rev (2004) 56(8):1205–17. doi: 10.1016/j.addr.2004.01.012
66. Turk MJ, Breur GJ, Widmer WR, Paulos CM, Xu LC, Grote LA, et al. Folate-Targeted Imaging of Activated Macrophages in Rats With Adjuvant-Induced Arthritis. Arthritis Rheum (2002) 46(7):1947–55. doi: 10.1002/art.10405
67. Matteson EL, Lowe VJ, Prendergast FG, Crowson CS, Moder KG, Morgenstern DE, et al. Assessment of Disease Activity in Rheumatoid Arthritis Using a Novel Folate Targeted Radiopharmaceutical Folatescan. Clin Exp Rheumatol (2009) 27(2):253–9.
68. Srinivasarao M, Galliford CV, Low PS. Principles in the Design of Ligand-Targeted Cancer Therapeutics and Imaging Agents. Nat Rev Drug Discov (2015) 14(3):203–19. doi: 10.1038/nrd4519
69. Chandrupatla DM, Weijers K, Gent YY, de Greeuw I, Lammertsma AA, Jansen G, et al. Sustained Macrophage Infiltration Upon Multiple Intra-Articular Injections: An Improved Rat Model of Rheumatoid Arthritis for PET Guided Therapy Evaluation. BioMed Res Int (2015) 2015:509295. doi: 10.1155/2015/509295
70. Chandrupatla D, Jansen G, Mantel E, Low PS, Matsuyama T, Musters RP, et al. Imaging and Methotrexate Response Monitoring of Systemic Inflammation in Arthritic Rats Employing the Macrophage PET Tracer [(18)F]Fluoro-PEG-Folate. Contrast Media Mol Imaging (2018) 2018:8092781. doi: 10.1155/2018/8092781
71. Dijkstra CD, Dopp EA, Vogels IM, Van Noorden CJ. Macrophages and Dendritic Cells in Antigen-Induced Arthritis. An Immunohistochemical Study Using Cryostat Sections of the Whole Knee Joint of Rat. Scand J Immunol (1987) 26(5):513–23. doi: 10.1111/j.1365-3083.1987.tb02285.x
72. Richards PJ, Williams AS, Goodfellow RM, Williams BD. Liposomal Clodronate Eliminates Synovial Macrophages, Reduces Inflammation and Ameliorates Joint Destruction in Antigen-Induced Arthritis. Rheumatology (1999) 38(9):818–25. doi: 10.1093/rheumatology/38.9.818
73. Figus FA, Piga M, Azzolin I, McConnell R, Iagnocco A. Rheumatoid Arthritis: Extra-Articular Manifestations and Comorbidities. Autoimmun Rev (2021) 20(4):102776. doi: 10.1016/j.autrev.2021.102776
74. Karatas A, Koca SS, Ozgen M, Dagli AF, Erman F, Sahin N, et al. Pemetrexed Ameliorates Experimental Arthritis in Rats. Inflammation (2015) 38(1):9–15. doi: 10.1007/s10753-014-0002-3
75. van der Heijden JW, Assaraf YG, Gerards AH, Oerlemans R, Lems WF, Scheper RJ, et al. Methotrexate Analogues Display Enhanced Inhibition of TNF-Alpha Production in Whole Blood From RA Patients. Scandinavian J Rheumatol (2014) 43(1):9–16. doi: 10.3109/03009742.2013.797490
76. Gent YY, Ter Wee MM, Voskuyl AE, den Uyl D, Ahmadi N, Dowling C, et al. Subclinical Synovitis Detected by Macrophage PET, But Not MRI, Is Related to Short-Term Flare of Clinical Disease Activity in Early RA Patients: An Exploratory Study. Arthritis Res Ther (2015) 17:266. doi: 10.1186/s13075-015-0770-7
77. Gent YY, Voskuyl AE, Kloet RW, van Schaardenburg D, Hoekstra OS, Dijkmans BA, et al. Macrophage Positron Emission Tomography Imaging as a Biomarker for Preclinical Rheumatoid Arthritis: Findings of a Prospective Pilot Study. Arthritis Rheum (2012) 64(1):62–6. doi: 10.1002/art.30655
78. Hu Y, Wang B, Shen J, Low SA, Putt KS, Niessen HWM, et al. Depletion of Activated Macrophages With a Folate Receptor-Beta-Specific Antibody Improves Symptoms in Mouse Models of Rheumatoid Arthritis. Arthritis Res Ther (2019) 21(1):143. doi: 10.1186/s13075-019-1912-0
79. Schniering J, Benesova M, Brunner M, Haller S, Cohrs S, Frauenfelder T, et al. (18)F-AzaFol for Detection of Folate Receptor-Beta Positive Macrophages in Experimental Interstitial Lung Disease-A Proof-Of-Concept Study. Front Immunol (2019) 10:2724. doi: 10.3389/fimmu.2019.02724
80. Esen I, Jiemy WF, van Sleen Y, van der Geest KSM, Sandovici M, Heeringa P, et al. Functionally Heterogenous Macrophage Subsets in the Pathogenesis of Giant Cell Arteritis: Novel Targets for Disease Monitoring and Treatment. J Clin Med (2021) 10(21):4958. doi: 10.3390/jcm10214958
81. Jahandideh A, Uotila S, Stahle M, Virta J, Li XG, Kyto V, et al. Folate Receptor Beta-Targeted PET Imaging of Macrophages in Autoimmune Myocarditis. J Nucl Med (2020) 61(11):1643–9. doi: 10.2967/jnumed.119.241356
82. Jager NA, Westra J, van Dam GM, Teteloshvili N, Tio RA, Breek JC, et al. Targeted Folate Receptor Beta Fluorescence Imaging as a Measure of Inflammation to Estimate Vulnerability Within Human Atherosclerotic Carotid Plaque. J Nucl Med (2012) 53(8):1222–9. doi: 10.2967/jnumed.111.099671
83. Muller C, Schibli R, Maurer B. Can Nuclear Imaging of Activated Macrophages With Folic Acid-Based Radiotracers Serve as a Prognostic Means to Identify COVID-19 Patients at Risk? Pharmaceut (Basel) (2020) 13(9):238. doi: 10.3390/ph13090238
84. Chen Q, Meng X, McQuade P, Rubins D, Lin SA, Zeng Z, et al. Synthesis and Preclinical Evaluation of Folate-NOTA-Al(18)F for PET Imaging of Folate-Receptor-Positive Tumors. Mol Pharm (2016) 13(5):1520–7. doi: 10.1021/acs.molpharmaceut.5b00989
85. Kelderhouse LE, Mahalingam S, Low PS. Predicting Response to Therapy for Autoimmune and Inflammatory Diseases Using a Folate Receptor-Targeted Near-Infrared Fluorescent Imaging Agent. Mol Imaging Biol (2016) 18(2):201–8. doi: 10.1007/s11307-015-0876-y
86. Boogerd LSF, Hoogstins CES, Gaarenstroom KN, de Kroon CD, Beltman JJ, Bosse T, et al. Folate Receptor-Alpha Targeted Near-Infrared Fluorescence Imaging in High-Risk Endometrial Cancer Patients: A Tissue Microarray and Clinical Feasibility Study. Oncotarget (2018) 9(1):791–801. doi: 10.18632/oncotarget.23155
Keywords: PET imaging, folate receptor beta, rheumatoid arthritis, macrophage, antigen-induced arthritis
Citation: Steinz MM, Ezdoglian A, Khodadust F, Molthoff CFM, Srinivasarao M, Low PS, Zwezerijnen GJC, Yaqub M, Beaino W, Windhorst AD, Tas SW, Jansen G and van der Laken CJ (2022) Folate Receptor Beta for Macrophage Imaging in Rheumatoid Arthritis. Front. Immunol. 13:819163. doi: 10.3389/fimmu.2022.819163
Received: 20 November 2021; Accepted: 11 January 2022;
Published: 02 February 2022.
Edited by:
Marco Erreni, Humanitas Research Hospital, ItalyReviewed by:
Venkatesh Chelvam, Indian Institute of Technology Indore, IndiaFengying Sun, Jilin University, China
Hannes Stockinger, Medical University of Vienna, Austria
Copyright © 2022 Steinz, Ezdoglian, Khodadust, Molthoff, Srinivasarao, Low, Zwezerijnen, Yaqub, Beaino, Windhorst, Tas, Jansen and van der Laken. This is an open-access article distributed under the terms of the Creative Commons Attribution License (CC BY). The use, distribution or reproduction in other forums is permitted, provided the original author(s) and the copyright owner(s) are credited and that the original publication in this journal is cited, in accordance with accepted academic practice. No use, distribution or reproduction is permitted which does not comply with these terms.
*Correspondence: Conny J. van der Laken, ai52YW5kZXJsYWtlbkBhbXN0ZXJkYW11bWMubmw=