- 1Department of Laboratory Medicine, Shengjing Hospital of China Medical University, Shenyang, China
- 2Department of Laboratory Medicine, Beijing Haidian Hospital, Beijing Haidian Section of Peking University Third Hospital, Beijing, China
Membranous nephropathy (MN) is the main cause of adult nephrotic syndrome (NS). The pathogenesis of MN is complex and involves subepithelial immune complex deposition. Approximately one-third of patients with MN develop end-stage renal disease (ESRD). Timely diagnosis and reasonable intervention are the keys to improving prognosis. In recent years, with the development of high-throughput technologies, such as mass spectrometry (MS), microarray, and sequencing technologies, the discovery of biomarkers for MN has become an important area of research. In this review, we summarize the significant progress in biomarker identification. For example, a variety of podocyte target antigens and their autoantibodies have been reported. Phospholipase A2 receptor (PLA2R) is the most well-established target antigen in MN. PLA2R and its autoantibodies have clinical significance, with both diagnostic and therapeutic value for MN. In addition, a variety of new biomarkers, including proteins, metabolites, noncoding RNAs (ncRNAs), and immune cells, have recently been found. These MN-related biomarkers have great significance in the diagnosis, progression, prognosis, and treatment response of MN.
Introduction
Membranous nephropathy (MN) is the major cause of adult nephrotic syndrome(NS) and the second leading cause of primary glomerulonephritis and end-stage renal disease (ESRD). Approximately 80% of MN cases have no clear secondary causes, referred to as idiopathic membranous nephropathy (IMN) or primary membranous nephropathy (PMN), and approximately 20% of MN cases are secondary to autoimmune diseases, infections, malignancies, drug use, and heavy metal poisoning, referred to as secondary membranous nephropathy (SMN) (1). The pathological features of IMN are thickening of glomerular basement membrane(GBM) (Figure 1A) and antigen-antibody immune complex deposition in the subepithelial area of GBM (Figure 1B) under light microscopy. In addition, the glomerular capillary walls show bright granular staining of IgG deposition by immunofluorescence microscopy (Figure 1C), and electron-dense deposits in the GBM are visible by electron microscopy (Figure 1D).
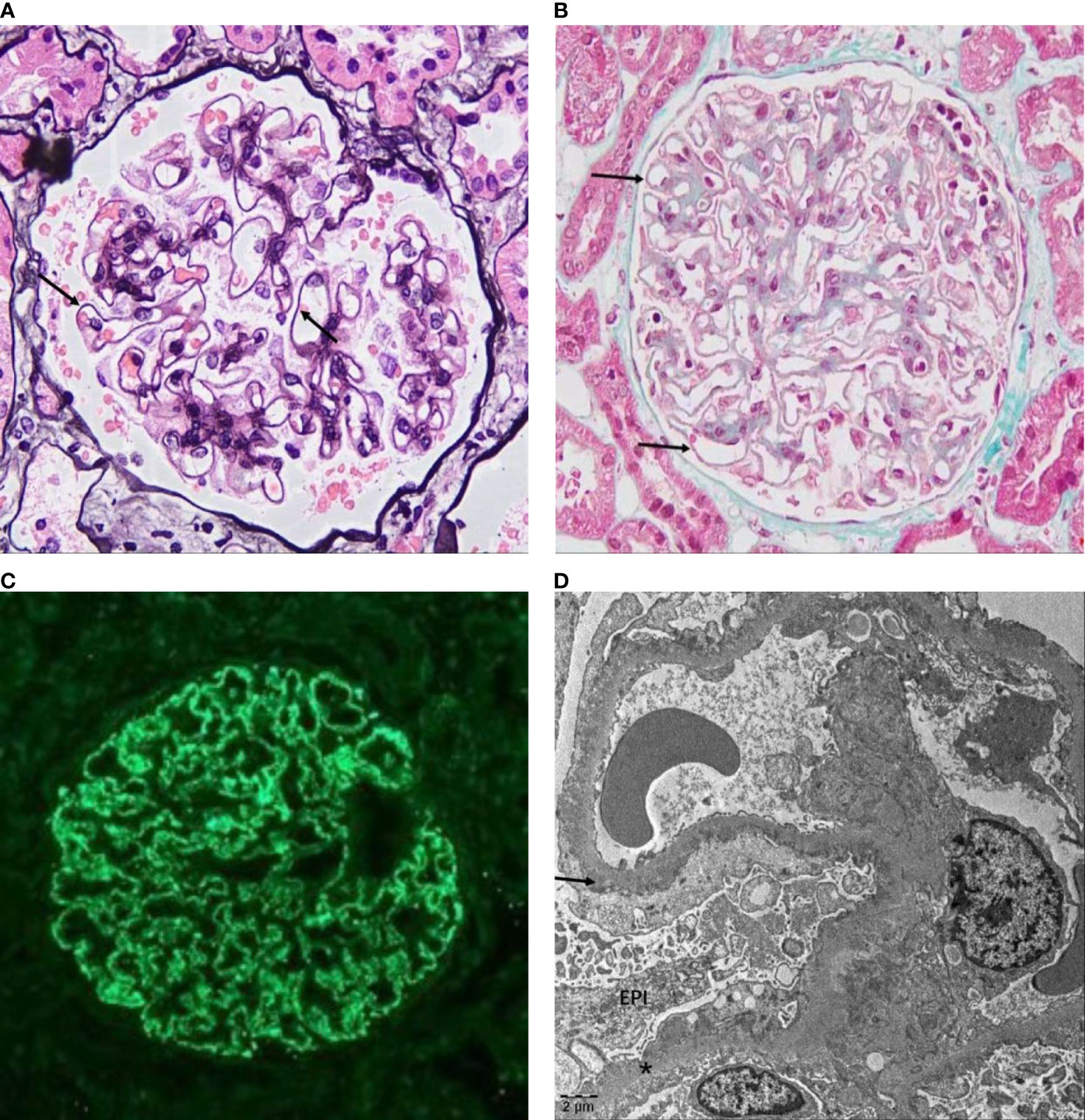
Figure 1 Pathological features of IMN. (A) Glomerulus from a patient with IMN showing the thickened GBM (arrow) under a light microscope stained with PASM (original magnification, 400×) and (B) pathognomonic antigen–antibody immune complex deposition on the epithelial side (arrow) stained with Masson (original magnification, 400×). (C) Immunofluorescence microscopy in IMN showed IgG (+++), fine granular deposition along capillary loops. (D) Electron micrograph of IMN showed that the GBM is irregularly thickened, the foot processes are diffusely fused, and more electron-dense deposits (arrow) can be seen under the epithelium (EPI) and in the basement membrane (*) (images were based on pathological data for renal biopsy cases in the Department of Nephrology of Shengjing Hospital).
The pathogenesis of MN is complex, and the core process is the formation of an immune complex in the GBM. It is currently suggested that in situ immune complexes of circulating autoantibodies and their target antigens on glomerular podocytes are deposited under epithelial cells, resulting in complement activation, thereby destroying the podocyte structure and resulting in proteinuria (2). In addition, the deposition of preformed circulating antigens, such as viral, tumor, and thyroid antigens, under the epithelium that binds to corresponding antibodies to form an in situ immune complex and preformed circulating immune complexes, such as circulating double-stranded DNA (dsDNA)–double-stranded DNA antibody (dsDNA Ab) complexes in patients with systemic lupus erythematosus, under the epithelium may also be involved in the pathogenesis of MN (3–6) (Figure 2).
The clinical manifestations of MN are mainly NS and asymptomatic proteinuria. The disease course is characterized by a “one-third law”, in which approximately one-third of cases resolve spontaneously without treatment, one-third show persistent proteinuria, and one-third progress to ESRD in 5–10 years (7, 8).
At present, the gold standard for MN diagnosis is renal biopsy; however, owing to its invasive nature, its repeated application for clinical diagnosis is limited. Monitoring and the determination of curative effects in MN still largely depend on quantitative indicators of urinary protein and renal function; however, these indexes lack specificity. Therefore, it is of great clinical significance to explore sensitive, specific, and noninvasive biomarkers for the diagnosis and treatment of MN. In recent years, advances in high-throughput technologies have provided a basis for substantial breakthroughs in biomarker identification for MN diagnosis, disease monitoring, the judgement of treatment efficacy, prognostic evaluation, etc. In particular, we describe podocyte target antigens and autoantibody biomarkers, protein and metabolic markers, noncoding RNAs, and immune cell markers, with a focus on the detection technology and clinical value of various marker types.
1 MN-Related Podocyte Target Antigen and Autoantibody Biomarkers
The pathogenesis of MN involves complement-mediated podocyte injury caused by antibodies targeting podocyte antigens. Therefore, MN-related podocyte target antigens and their autoantibodies can provide reliable markers for diagnosis and monitoring.
Research aimed at the discovery of MN-related podocyte target antigens and their autoantibodies has continued for decades. A variety of human podocyte target antigens and their autoantibodies have been found. In 2002, Debiec et al. found that the autoantigen leading to neonatal MN is a neutral endopeptidase (NEP) in podocytes (9), and its autoantibodies are maternal alloantibodies caused by a hereditary NEP deficiency in the mother, rather than the antibodies produced by the child. It was not until 2009 that the first adult MN podocyte target antigen, M-type phospholipase A2 receptor (PLA2R), was detected in 70% of IMN serum and tissues by liquid chromatography with tandem mass spectrometry (LC-MS/MS) (10). Since the discovery of PLA2R, a variety of MN podocyte target antigens have been discovered by high-throughput MS. In 2014, Thomas et al. (11) identified another IMN podocyte target antigen, thrombospondin type-1 domain-containing 7A (THSD7A), in PLA2R-negative patients with IMN by MS. In 2019, Sethi et al. (12) identified exostatin/exostatin 2 (EXT1/EXT2) as a target antigen of MN in PLA2R-negative MN by laser microdissection and MS (LMD-MS). EXT1/EXT2 is the most common specific target antigen of PLA2R-negative MN and exists in SMN. In 2020, Sethi et al. (13) used LMD-MS to identify neural epidermal growth factor-like 1 (NELL-1) as another target antigen for PLA2R and THSD7A double negative patients. In the same year, LMD-MS was used to identify another target antigen, semaphorin-3b (Sema-3B), in the patients negative for PLA2R, THSD7A, EXT1/EXT2, and NELL-1 (14). In 2021, Caza et al. (15) identified neural cell adhesion molecule 1 (NCAM-1) as a new autoantigen of membranous lupus nephritis (LN) by LMD-MS. In the same year, high-temperature recombinant protein A1 (HTRA1) (16) and protocadherin 7 (PCDH7) (17) were identified as additional target antigens. Although the clinical characteristics of PCDH7 are not clear, it is speculated that it is an IMN target antigen. IMN-related target antigens can be detected in 80% of cases, of which approximately 70%–80% of IMN cases are PLA2R positive, approximately 1% are PLA2R and THSD7A double positive, and another 20%–30% are negative for PLA2R, including THSD7A, NELL-1, Sema3B, and THRA-related IMN (approximately 13%); however, 10%–20% of IMN-related target antigens remain to be discovered (1, 2, 16, 18–20) (Figure 3).
There are different predominant IgG subtypes in different podocyte target antigen-associated MN (Table 1). The majority of IgG subclasses of the earliest discovered autoantigens PLA2R- and THSD7A-associated IMN (approximately 80%–85% IMN) are IgG4, while EXT1/EXT2-related SMN (approximately 30%–40% SMN) is IgG1 (18–20). Therefore, in the majority of cases of MN, the dominant IgG subtypes in IMN and SMN are IgG4 and IgG1, respectively. However, it cannot accurately distinguish IMN from SMN, especially for the new antigens discovered in recent years. For example, the predominant autoantibody subtype in patients with NELL-1 and Sema-3B-related IMN is IgG1 (13, 14), and NEP, NCAM-1, and PCDH7 do not have the single majority IgG subtype (15, 17, 19). In addition, the main patient groups for different podocyte target antigen-related MN are different. Most of the patients with MN were adults, while the patients with NEP-related MN were mainly newborns, and Sema-3B-related MN patients were mainly children (Table 1).
A large number of studies have proven that the above-mentioned MN-related podocyte antigens and autoantibodies can be used as biomarkers of MN and play auxiliary roles in the diagnosis, analyses of treatment responses, and prediction of prognosis. The clinical value of podocyte target antigen-based biomarkers (including autoantigens and their autoantibodies) of MN (Table 1) is described in the following sections.
1.1 PLA2R
Among the MN podocyte target antigen and autoantibody biomarkers found to date, PLA2R and anti- PLA2R antibody have better clinical diagnostic value for IMN than other biomarkers. A meta-analysis based on data from 10 studies involving 1,550 participants showed that in the active phase, the sensitivity and specificity of serum PLA2R antibody levels for the diagnosis of IMN are approximately 74.0% and 95.0%, respectively (21). Many studies have also shown that the sensitivity of anti-PLA2R antibodies for IMN diagnosis is >70%, and the specificity is close to 100% (22–24). Despite the high sensitivity and specificity of serum anti-PLA2R antibody levels for the diagnosis of IMN, there is no consensus on whether this approach can replace renal biopsy. Some scholars believe that renal pathology can provide more information than serum antibodies. In 2021, KDIGO guidelines indicated that patients with nephrotic syndrome (NS) and positive anti-PLA2R antibodies may not need renal biopsy for MN diagnosis. For patients with positive PLA2R antibodies, if there is an unexplained decrease in the estimated glomerular filtration rate (eGFR) or unresponsive to immunosuppressive therapy, renal biopsy is recommended (25).
Many studies have shown that PLA2R is more common in IMN than in SMN (26–31), suggesting that PLA2R has certain clinical value for distinguishing between the two types. However, it has also been detected in SMN (26–31), especially in hepatitis, sarcoidosis, and tumor-related SMN. Larsen et al. (31) detected PLA2R1 in 80 cases of SMN renal tissues by indirect immunofluorescence. PLA2R1 was positive in 64% of cases with hepatitis C (7/11) and in 75% of cases with sarcoidosis (3/4), 25% with tumors (3/12), and 2% with autoimmune diseases (1/46)-related SMN. Therefore, although PLA2R is more common in IMN, it cannot reliably distinguish IMN from SMN.
A number of studies have shown that PLA2R and its autoantibodies are closely related to the severity, treatment efficacy, and prognosis of MN. Levels of serum anti-PLA2R antibodies are related to serum albumin, serum creatinine, eGFR, and proteinuria. Compared with glomerular PLA2R deposition, serum anti-PLA2R antibody levels are more closely correlated with disease activity and renal function (32). Serum anti-PLA2R antibody levels are detectable months to years before proteinuria production (33) and decline or disappear early before immune deposits start to recede (34). In addition, proteinuria levels are higher in patients with anti-PLA2R antibody-positive IMN than in patients with anti-PLA2R antibody-negative IMN (26, 35), and immunosuppressive therapy is more effective against PLA2R antibody-negative IMN than against PLA2R-positive IMN (29). When the anti-PLA2R level is <40 IU/ml, patients are expected to benefit from conservative treatment (36, 37), avoiding the side effects of immunosuppressive agents. Dong et al. (38) found that the remission rate was lower in the serum anti-PLA2R antibody-positive group than in the PLA2R-negative group, with no significant difference between the PLA2R-positive group and the PLA2R-negative group in renal tissues, suggesting that compared with tissue PLA2R, serum anti-PLA2R antibodies are better correlated with the progression and therapeutic response of IMN. Other studies have shown that spontaneous remission rates are higher in patients with MN with low anti-PLA2R titers than in patients with high anti-PLA2R titers (36, 39). In addition, approximately one-third of patients with ESRD undergo renal transplantation, and 30%–50% of these patients have recurrent MN (40, 41). Increased anti-PLA2R antibodies levels in patients with MN before renal transplantation indicate a risk of recurrence after renal transplantation (42–44). Therefore, to evaluate the treatment response and adjust the treatment strategy, KDIGO 2021 glomerular disease management guidelines (25) recommend evaluation at 3 or 6 months after the start of treatment, and the anti-PLA2R antibodies level should be monitored longitudinally.
1.2 THSD7A
At present, there are insufficient data to support the application of anti-THSD7A antibodies as a diagnostic biomarker for MN instead of biopsy (25). Although THSD7A has good specificity for the diagnosis of IMN, its sensitivity is low. A recent meta-analysis of 10 studies involving 4,545 patients showed that the total sensitivity of the anti-THSD7A antibody for the diagnosis of IMN was 4%, the specificity was 99%, the total sensitivity for PLA2R-negative IMN was 8% (6%–10%), and the specificity was 100% (99%–100%), suggesting that anti-THSD7A antibodies have high diagnostic value for PLA2R-negative IMN and can be used as an auxiliary diagnostic method (45).
Similar to the anti-PLA2R antibody, the anti-THSD7A antibody is mostly detected in IMN; however, it cannot completely distinguish IMN from SMN. Maifata et al. (30) detected the serum anti-THSD7A antibody in four patients with IMN (4/47, 8.5%) and in two patients with SMN (2/22, 9.1%). In addition, studies have shown that the anti-THSD7A antibodies levels are associated with the treatment response and disease activity (46). Patients with high anti-THSD7A titer at baseline had poor prognosis (46). Therefore, monitoring the autoantibody titer can contribute to disease monitoring and prediction of curative effects in THSD7A-related MN.
1.3 EXT1/EXT2 and NCAM-1
The etiology of SMN is mainly autoimmune diseases, and LN is the main cause of SMN caused by autoimmune diseases. Approximately 30%–40% of SMN are EXT1/EXT2-related MN, and nearly 35% of EXT1/EXT2-related SMN are membranous LN. Therefore, EXT1/EXT2 is considered the main subtype of SMN and can be used as a potential representative biomarker of SMN and LN (12). In addition, a retrospective study has shown that patients with LN who are EXT1/EXT2 positive have fewer chronic renal changes and a lower rate of progression to ESRD than negative patients (47); therefore, EXT1/EXT2 may have predictive value for the prognosis of LN. However, to date, anti-EXT1/EXT2 antibodies have been identified only in renal tissues and not in the peripheral circulation (12), which indicates that they may not be considered to be noninvasive biomarkers for MN diagnosis.
NCAM-1 is another podocyte target antigen in LN. It is found in the tissues of patients with membranous LN, with no positive staining in normal kidney tissues. Studies have shown that approximately 6.6% of NCAM-1 associated renal disease is membranous LN and 2.0% is PMN. Circulating anti-NCAM1 antibodies have been confirmed to exist in a subset of lupus patients (15). Both NCAM-1 and EXT1/EXT2 are considered the first-generation biomarkers of membranous LN (20).
1.4 NELL-1, Sema-3B, PCDH7, and HTRA1
In NELL-1-, Sema-3B-, PCDH7-, and HTRA1-related MN, their respective autoantigens and circulating autoantibodies can be identified, which can be considered as potential biomarkers for MN diagnosis. In addition, NELL-1-, Sema-3B-, PCDH7-, and HTRA1-related MN also have their own clinical characteristics, which are helpful for accurate clinical diagnosis and treatment.
One characteristic of NELL-1 associated MN is that malignant tumors may exist in it. The incidence of NELL-1-related MN malignant tumors ranges from 11.7% to 33% (48). MN may also occur before the detection of malignant tumors. Therefore, MN patients who are NELL-1 serum antibody- positive may need to consider regular physical examinations to exclude the presence of malignant tumors.
Sema-3B-associated MN occurs in children and young people. Sethi et al. showed that among 11 cases of Semaphorin 3B-associated MN, 8 were pediatric cases and 5 cases started at or below the age of 2 (14). In pediatric patients, if renal biopsy is not performed, MN may be misdiagnosed. Therefore, if the Sema-3B antibody is detected in children with NS, MN should be considered.
HTRA1 and PCDH7 are newly discovered MN podocyte target antigens based on MS (16, 17). It has been reported that approximately 1%–2% of the target antigens in IMN may be HTRA1 (16) and 5%–6% of the target antigens in PLA2R-MN may be PCDH7 (17). To date, it is not clear whether or not PCDH7-associated MN belongs to IMN. What the characteristic of PCDH7-related MN has been found is that complement activation is rarely activated or not activated, and some patients have spontaneous remission without immunosuppressive therapy (17); however, further clinical studies are needed to determine whether PCDH7 can be used as a biomarker of spontaneous remission of MN (18).
2 MN-Related Non-Podocyte Autoantigen or Autoantibody Biomarkers and Metabolic Biomarkers
Exploring differentially expressed proteins and metabolites between patients with MN and healthy individuals is an important research goal, providing candidate biomarkers and improving our understanding of the underlying molecular mechanisms. Recently, various proteins and metabolic biomarkers have been detected by proteome and metabolome analysis based on MS technologies (Table 2).
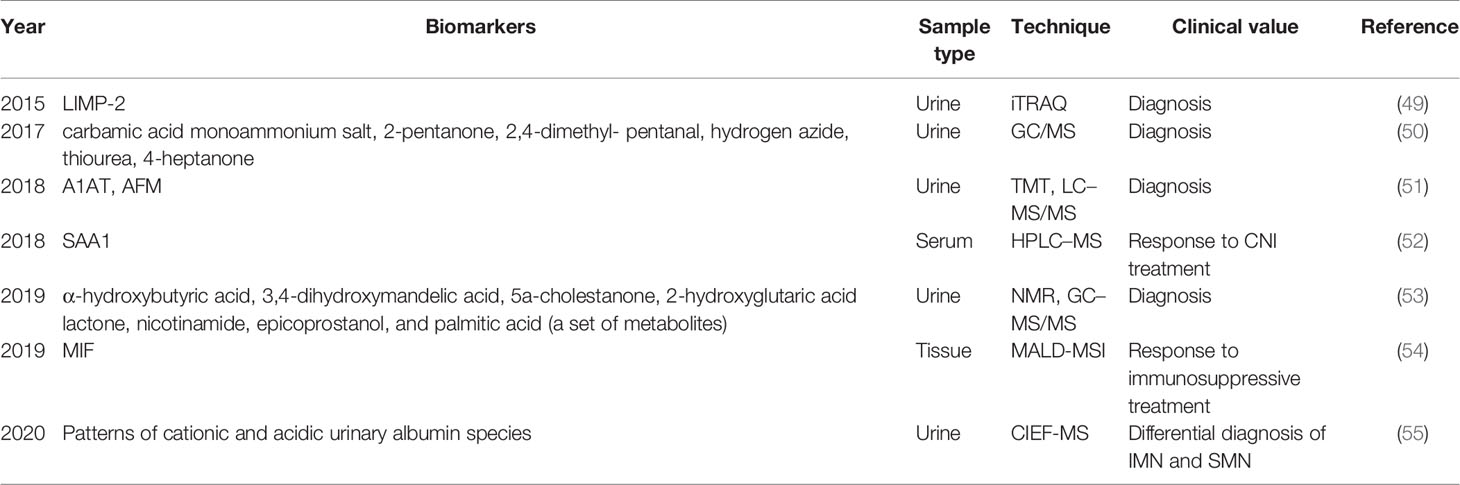
Table 2 Summary of research progress on MN-related non-podocyte target antigen proteins and metabolic biomarkers.
Differences in the levels of various non-podocyte autoantigen or autoantibody proteins and metabolites have recently been reported between MN and healthy controls or patients with other glomerular diseases. These differential proteins and metabolomics are expected to become potential diagnostic biomarkers for MN. Using the iTRAQ (relative and absolute quantitative isobaric labeling) technique, Root et al. (49) found that the level of lysosomal-integrated membrane protein (LIMP-2) in urinary microbubbles obtained from patients with MN was twofold higher than those in idiopathic focal segmental glomerulosclerosis (FSGS) and normal controls. Wang et al. (50) used gas chromatography/mass spectrometry (GC/MS) to analyze the contents of volatile organic compounds in the urine of 63 patients with IMN and 15 normal controls. The results showed that six volatile organic compounds (carbamic acid monoammonium salt, 2-pentanone, 2,4-dimethyl-pentanal, hydrogen azide, thiourea, and 4- heptanone) differed significantly between patients with IMN and normal controls (p < 0.05). Pang et al. (51) performed two technical replicates (TMT1 and TMT2) by using tandem mass tag (TMT) systems combined with nanoscale LC-MS/MS to analyze and compare the urine proteomes of PMN patients and healthy controls. In total, 509 and 411 proteins were identified in TMT1 and TMT2, respectively, and 249 proteins were identified in two technical replicates, of which urine alpha-1-antitrypsin (A1AT) and afamin (AFM) were oversecreted in patients with PMN. In addition, Taherkhani et al. (53) analyzed the urinary metabolomes of 66 patients with MN, 31 healthy controls, and 72 disease controls by nuclear magnetic resonance (NMR) and GC-MS/MS. A set of seven metabolites (a-hydroxybutyric acid, 3,4-dihydroxymandelic acid, 5a-cholestanone, 2-hydroxyglutaric acid lactone, nicotinamide,epicoprostanol, and palmitic acid) was the best diagnostic predictor of MN. The detection sensitivity and specificity of these seven metabolites were 100%.
Tie et al. (55) used a newly developed the capillary isoelectric focusing-mass spectrometry (CIEF-MS) method to compare the types of urinary albumin in patients with IMN and SMN. Cationic and acidic albumin in the urine patterns differed between IMN and SMN, suggesting that the detection of urinary albumin patterns could be used to distinguish between IMN and SMN.
In addition, Yu et al. (52) used nano- high performance liquid chromatography (HPLC)-MS to screen differential proteins between an IMN remission group and non-remission group after treatment with calcineurin inhibitors (CNI). The level of serum amyloid A1 protein (SAA1) in the remission group was significantly higher than that in the non-remission group, suggesting that SAA1 is a candidate marker to predict the efficacy of calcineurin inhibitor treatment in IMN.
A study of two groups of patients with different responses to immunosuppressive therapy (Ponticelli protocol) had kidney biopsies and performed matrix-assisted laser desorption/ionization mass spectrometry imaging (MALDI-MSI), which revealed that the signal at m/z 1,303 showed the greatest discriminatory power, and the corresponding trypsin peptide was identified as a macrophage migration inhibitory factor (MIF). These results suggest that MIF may be a predictor of the response to immunosuppressive therapy in MN (54).
Although these non-podocyte target antigens and autoantibody biomarkers have not been widely validated and little is known about correlations between these biomarkers and clinical manifestations and pathological features, the discovery of these biomarkers provides a basis and new directions for diagnosis, differential diagnosis, monitoring, and treatment of MN.
3 MN-Related ncRNAs
Noncoding RNAs (ncRNAs) are a kind of RNA that do not encode proteins but have biological functions at the RNA level, including ribosomal RNAs, transport RNAs, small nucleolar RNAs, small nucleolar RNAs, long-chain noncoding RNAs (lncRNAs), circular RNAs (circRNAs), microRNAs (miRNAs), and small interfering RNAs. With the development of gene sequencing technology and bioinformatics methods, the biological functions of ncRNAs have become a focus of recent research. Many studies have shown that imbalances in ncRNA expression are related to the occurrence and development of many diseases. Because ncRNAs stably exist in body fluids and can be detected noninvasively, they are a promising new biomarker type for disease prediction. Recent studies have shown that the expression levels of ncRNAs in the urine, blood, and kidneys of patients with MN differ from those in healthy individuals (Table 3). Most studies of MN-related ncRNAs are focused on miRNAs, followed by circRNAs and lncRNAs. The discovery of these MN-related ncRNA markers not only has implications for the clinical diagnosis and treatment of MN but also helps to further reveal the mechanism underlying podocyte injury in the occurrence and development of MN.
3.1 MN-Related miRNAs
MiRNAs are small noncoding RNAs containing approximately 22 nucleotides that are involved in posttranscriptional gene silencing. In recent years, some studies have shown that abnormal miRNAs play a significant role in the pathogenesis of many kidney diseases (69, 70). However, studies of miRNAs in MN are limited at present. In 2014, the first study on the differentially expressed miRNAs (DEMs) in peripheral blood lymphocytes of patients with MN was reported, in which high-throughput sequencing technology was used to analyze the miRNA expression profiles in peripheral blood lymphocytes (PBMCs) of patients with MN and healthy controls, revealing 326 DEMs between the two groups (71). Since then, many studies have used high-throughput technology to detect DEMs between MN patients and healthy controls (63, 72). Although these DEMs may play an important role in the pathogenesis of MN and are expected to become potential biomarkers, further clinical studies are needed to confirm their clinical values in MN. At present, only a few miRNAs have been identified as potential biomarkers of diagnosis or podocyte apoptosis in MN, which are reviewed in the following paragraphs.
3.1.1 miR-217
Li et al. (58) found that compared with levels in healthy controls, the expression level of miR-217 was significantly downregulated in MN, and upregulation of miR-217 expression in vitro could inhibit the expression of the target gene TNFSF11 and protect podocytes. The downregulation of miR-217 expression can lead to increased levels of the target gene TNFSF11 and podocyte apoptosis, suggesting that miR-217 participates in podocyte apoptosis by negatively regulating the expression of the target gene TNFSF11. In addition, the area under the curve (AUC) for miR-217 for MN diagnosis was 0.941 (95% CI, 0.904–0.979), and the sensitivity and specificity were 88.9% and 75.9%, respectively. These results showed that miR-217 is involved in podocyte apoptosis and has potential diagnostic value in MN.
3.1.2 miR-130a-5p
miR-130a-5p expression was found to be significantly decreased in renal biopsy specimens in MN patients, and miR-130a-5p overexpression by miR-130a-5p agomir strongly alleviated renal injury in MN mice. In addition, miR-130a-5p was downregulated and further increased the expression of the target gene PLA2R in the podocyte damage model stimulated by Ang II. All these findings indicated that the decrease in miR-130a-5p expression in MN patients may accelerate podocyte apoptosis by upregulating the expression of PLA2R, thereby promoting IMN development (59).
3.1.3 miR-193
Zhang et al. (60) showed upregulated miR-193a expression and downregulated WT1/PODXL expression in patients with IMN. In addition, four indexes, including high miR-193a expression, low WT1/PODXL expression, high proteinuria (>3.79 g/24 h)/serum creatinine (>174.63 μmol/L), and low GFR (≤68.13 ml/min/1.73 m2), are important biomarkers of poor renal survival in patients with IMN. The combined detection of miR-193a with PODXL and WT1 can be used as a strategy for diagnosing IMN (AUC = 0.994) and predicting renal survival status (AUC = 0.824). Although this study failed to figure out how miR-193a/WT1/PODXL axis functioned underlying the etiology of IMN, another study (61) established a cationic bovine serum albumin (cBSA)-induced rat model of MN and found that inhibiting miR-193a can inhibit renal injury in rats, and podocyte injury leads to tissue cell apoptosis. Furthermore, WT1 is a target gene of miR-193a, and the expression of WT1 increases after the inhibition of miR-193a. Therefore, membrane nephropathy may be improved by inhibiting miR-193a.
3.1.4 miR-195-5P, miR-192-3P, and miR-328-5p
Zhou et al. analyzed the mRNA database GSE73953 (eight MN cases and two healthy controls) and miRNA database GSE64306 (four MN cases and six healthy controls) datasets (62) and found that the interactions among miRNA-195-5P, miRNA-192-3P, and miR-328-5p and their corresponding target genes PPM1A, RAB1A, and BRSK1 in peripheral blood and urine may be involved in MN development and podocyte apoptosis. In addition, PPM1A and BRSK1 may be involved in MN by the MAPK-related signaling pathway, while RAB1A may be associated with MN by involving the p53 signaling pathway. However, further investigation is needed to validate the relationship between these miRNAs and their target genes in in vitro or in vivo experiments.
3.1.5 miR-107, miR-423-5p, Let-7a-5p, etc.
A recent study (63) confirmed 10 miRNAs (miR-107, miR-423-5p, Let-7a-5p, etc.) to be DEMs in renal tissue between MN patients and the control group by quantitative real-time PCR (qRT-PCR). According to Gene Ontology analysis, these 10 miRNAs may regulate pathways of epidermal growth factor receptor (EGFR), platelet activation, transforming growth factor beta receptor (TGFBR), mitotic G2/M transition, Forkhead box O (FoxO), extracellular matrix (ECM) disassembly, and SMAD binding, which are associated with MN pathogenesis, including the cell cycle, proliferation, and apoptosis. In addition, transient silencing of miRNAs (let-7a-5p, let-7c-5p) in A498 cells significantly upregulated IL-6 and MYC which have strong negative correlations with clinical parameters, such as proteinuria and total cholesterol. These data indicated that the 10 miRNAs and their downstream network could be considered as potential diagnostic biomarkers of MN and may be involved in MN pathogenesis.
3.1.6 miR-30, miR-186
Sha et al. (57) showed that miR-186 is significantly downregulated in MN, that its expression is regulated by TLR4, and that miR-186 mimic treatment reversed the effects of apoptosis induced by Angiotensin II in podocytes. Recently, another study predicted that the miR-30 family may participate in the pathogenesis of MN by targeting different genes involved in the TGF-β, Notch1, and p53 signaling pathways based on bioinformatics analysis and a literature review. In addition, miR-30c and miR-186 levels were significantly elevated in PBMC (p = 0.037) and plasma (p = 0.035) samples in MN patients compared with the control group, respectively. Moreover, plasma and PBMC miR-30 levels combined with miR-186 detection can be used as a potential biomarker for NS diagnosis (AUC ≥ 0.72) (66).
3.1.7 miR-106a, miR-19b, and miR-17
Wu et al. (67) showed that compared with levels in the control group, the expression levels of miR-106a, miR-19b, and miR-17 in the IMN group were significantly lower, whereas phosphatase and tensin homolog (PTEN) concentration was increased significantly and negatively correlated with the expression of miR-106a and miR-19b in the IMN group, which suggests that miR-106a, miR-19b, miR-17, and PTEN are involved in the pathogenesis of IMN, although their roles in the pathogenesis of IMN are unclear and need to be further explored. In addition, miR-106a, miR-19b, and miR-17 are potential biomarkers for IMN diagnosis with the AUC values for serum miR-106a, miR-19b, and miR-17 of 0.66 (95% CI, 0.56–0.76), 0.81 (95% CI, 0.73–0.89), and 0.69 (95% CI, 0.59–0.79), respectively.
3.2 MN-Related circRNAs
Previous studies have shown that the expression levels of circRNAs in the serum and urine exosomes, peripheral blood, and renal tissues differ between patients with IMN and healthy controls. Jin et al. (64) detected 955 differentially expressed circRNAs (645 upregulated and 310 downregulated) between the peripheral blood of patients with IMN and healthy individuals using circRNA chip technology. Among these, circ_101319 expression was significantly upregulated and effectively predicted IMN (AUC = 0.89). In addition, a coexpression network analysis revealed that circ_101319 may be related to pathways related to IMN via various miRNAs, providing novel insights for targeted gene therapy.
A circRNA sequencing analysis of serum and urine exosomes from patients with IMN and healthy controls revealed 89 significantly differentially expressed circRNAs in the serum exosomes, of which 49 were upregulated and 40 were downregulated in IMN. There were 60 significantly differentially expressed circRNAs in the urine exosomes, of which 54 were upregulated and 6 were downregulated in IMN. These differentially expressed circRNAs may be involved in the pathogenesis of MN (73).
Recently, Sun et al. (68) found that circ_0000524 is significantly upregulated in the renal tissues of MN. Circ_0000524 regulates the expression of CXCL16 by sponging miR-500a-5p. Downregulating circ_0000524 can inhibit angiotensin II-induced podocyte apoptosis via the circ_0000524/miR-500a-5p/CXCL16 pathway, which may play an important role in podocyte injury in MN.
3.3 MN-Related lncRNAs
Huang et al. found that lncRNA XIST is upregulated in renal tubular epithelial cells and glomerular cells of mice with MN, and its expression is positively correlated with the severity of MN. In addition, XIST levels are significantly elevated in the urine of MN mouse model and in patients with glomerulonephritis (including MN), and XIST concentrations in the urine are positively correlated with the severity of MN. Accordingly, urine XIST may be a potential noninvasive biomarker of MN (56). In addition, another recent study demonstrated that the downregulation of XIST could reduce podocyte apoptosis via miRNA-217/TLR4 pathway (65).
4 MN-Associated Immune Cell Biomarkers
MN is an autoimmune disease, and immune cells are involved in the occurrence and development of MN. Studies have shown increases in the CD4+/CD8+ T-cell ratio and Th2/Th1 cell ratio in the peripheral blood of patients with IMN (74). Although the CD4+/CD8+ T-cell ratio and Th2/Th1 cell ratio are not good biomarkers for IMN, their increase shows a relative increase in Th2 cells. Th2 cells, as the main group of CD4+T cells, can stimulate B cells to produce IgG4 by secreting cytokines IL-4 and IL-10 (74). In addition, anti-CD20 monoclonal antibody (rituximab) achieved good results in the clinical treatment of MN (75, 76). All these results indicated that IMN may be dominated by humoral immunity. However, peripheral blood B cells, as the main lymphocytes of humoral immunity, are not a reliable biomarker of disease activity and treatment outcomes. A study measured the lymphocyte subsets of 66 patients with clinically diagnosed IMN disease and 40 healthy controls. Compared with healthy subjects, it was found that the number of B lymphocytes in peripheral blood had no correlation with renal function indexes. In addition, although the amount of B-lymphocyte infiltration in IMN patients was higher, it was unrelated to the amount of B-lymphocyte infiltration in peripheral blood (77).
T cells participate in the activation and proliferation of B cells in the occurrence and development of MN, and B cells proliferate and differentiate into plasma cells for autoantibody production. Autoantibodies and complement activation can cause the infiltration of macrophages derived from peripheral blood monocytes. In recent years, some subsets of T cells and monocytes in the peripheral blood of patients with MN have been identified as candidate biomarkers to evaluate the curative effect and progression of MN. The candidate immune cell biomarkers and their potential clinical values in MN are described as follows:
4.1 Treg Cells
Treg cells are TCRαβ+Foxp3+CD4+ T cells that are endowed with suppressive activity, and the main role of Treg cells is to control the inflammatory and immune response to self-antigens, thereby preventing autoimmune disease; therefore, the lack of Treg cells may occur in many autoimmune conditions (78, 79). Motavalli et al. (80) found that the proportion of Treg cells in the peripheral blood and the expression of Foxp3 are decreased in IMN. However, Treg cells increase significantly in response to rituximab (81, 82). The percentage of Tregs in patients with a clinical response to rituximab was lower at baseline and increased significantly on day 8 of rituximab treatment but remained unchanged in non-responders and in patients receiving only supportive treatment. Therefore, evaluating Tregs may help to predict the early response to rituximab (82).
4.2 ICOS+ Follicular Helper T (Tfh) Cells and PD-1+ Tfh Cells
Tfh cells are an independent CD4+ cell subgroup located in lymphatic follicles and are thought to be critical for the formation and function of germinal center in the generation of memory B cells and long-lived plasma cells. ICOS and PD-1 are the two main extracellular markers expressed by Tfh cells. IL-21, as a key effector of activated Tfh cells, induces B-cell proliferation, mediates the differentiation of activated B cells into plasma cells, and promotes IgM, IgG, and IgA production. Shi et al. (83) found that the ratio of ICOS+/PD-1+ Tfh cells and IL-21+ Tfh cells in the peripheral blood cells of patients with IMN is positively correlated with 24-h urinary protein levels. These results suggest that ICOS+ Tfh cells and PD-1+ Tfh cells are potential biomarkers for evaluating the development of IMN.
4.3 CD14+ CD163+ CD206+ M2-Like Monocytes
Monocytes, as progenitors of dendritic cells or macrophages in tissues, are important regulators of the immune response. Human PBMCs can be differentiated into M2 monocytes, which are characterized by CD14+ CD163+. After activation, the expression levels of CD206 and CD115 increase and anti-inflammatory mediators, such as interleukin IL-10, are secreted. Hou et al. (84) found that the level of serum IL-10 in patients with early IMN is significantly higher than that in healthy controls. In addition, the number of CD14+ CD163+ CD206+ M2-like cells in patients with early IMN was positively correlated with the 24- h urinary albumin levels and serum PLA2R. Accordingly, CD14+ CD163+ CD206+ M2- like monocytes are potential markers for the severity of IMN.
Conclusions and Prospects
MN is one of the main pathological types of NS and the major cause of ESRD. At present, renal biopsy is the gold standard for MN diagnosis. Due to its invasive limitation, exploring new biomarkers in MN has become a research hotspot. In recent years, the discovery of MN biomarkers has advanced substantially since the development of high-throughput MS and gene sequencing technologies. Proteomic, metabolomic, and transcriptomic approaches have been used to evaluate the molecular basis of MN.
A variety of MN podocyte target antigens and their autoantibodies have been discovered, among which the anti-PLA2R antibody provides a good theoretical basis for the diagnosis of clinical IMN. However, of the IMN cases, the percentage of PLA2R-positive MN is approximately 70%–80%, and there are still 10%–20% of IMN-related podocyte target antigens unknown that need to be discovered.
Furthermore, various new biomarker types, such as proteins, metabolic markers, ncRNAs, and immune cells, have recently been shown to have diagnostic, monitoring, and prognostic significance in MN. Although these new markers have not been used in clinical settings and need to be verified, they may guide the development of diagnostic and therapeutic approaches for MN and improve our understanding of the pathogenic mechanism of MN.
Author Contributions
XQ and QL participated in the conceptual design and wrote the manuscript. QL, JL, BX, YZ, MM, and MY wrote the manuscript and created the figures and tables. XQ, QL and JL revised and edited the manuscript. All authors contributed to the article and approved the submitted version.
Funding
This study was supported by the National Key Research and Development Program of China (No. 2018YFE0207300). This work was also supported in part by the “345 Talent Project” of Shengjing Hospital of China Medical University (No. 50A) and Major Special Project of Construction Program of China Medical University in 2018 (No. 112/3110118034).
Conflict of Interest
The authors declare that the research was conducted in the absence of any commercial or financial relationships that could be construed as a potential conflict of interest.
Publisher’s Note
All claims expressed in this article are solely those of the authors and do not necessarily represent those of their affiliated organizations, or those of the publisher, the editors and the reviewers. Any product that may be evaluated in this article, or claim that may be made by its manufacturer, is not guaranteed or endorsed by the publisher.
References
1. Couser WG. Primary Membranous Nephropathy. Clin J Am Soc Nephrol (2017) 12(6):983–97. doi: 10.2215/CJN.11761116
2. Ronco P, Debiec H. Pathophysiological Advances in Membranous Nephropathy: Time for a Shift in Patient’s Care. Lancet (2015) 385(9981):1983–92. doi: 10.1016/S0140-6736(15)60731-0
3. Lai KN, Li PK, Lui SF, Au TC, Tam JS, Tong KL, et al. Membranous Nephropathy Related to Hepatitis B Virus in Adults. N Engl J Med (1991) 324(21):1457–63. doi: 10.1056/NEJM199105233242103
4. Beck LH Jr, Membranous Nephropathy and Malignancy. Semin Nephrol (2010) 30(6):635–44. doi: 10.1016/j.semnephrol.2010.09.011
5. Jordan SC, Buckingham B, Sakai R, Olson D. Studies of Immune-Complex Glomerulonephritis Mediated by Human Thyroglobulin. N Engl J Med (1981) 14;304(20):1212–5. doi: 10.1056/NEJM198105143042006
6. Shlomchik MJ, Madaio MP. The Role of Antibodies and B Cells in the Pathogenesis of Lupus Nephritis. Springer Semin Immunopathol (2003) 24(4):363–75. doi: 10.1007/s00281-003-0119-1
7. Schieppati A, Mosconi L, Perna A, Mecca G, Bertani T, Garattini S, et al. Prognosis of Untreated Patients With Idiopathic Membranous Nephropathy. N Engl J Med (1993) 329(2):85–9. doi: 10.1056/NEJM199307083290203
8. Segal PE, Choi MJ. Recent Advances and Prognosis in Idiopathic Membranous Nephropathy. Adv Chronic Kidney Dis (2012) 19(2):114–9. doi: 10.1053/j.ackd.2012.01.007
9. Debiec H, Guigonis V, Mougenot B, Decobert F, Haymann JP, Bensman A, et al. Antenatal Membranous Glomerulonephritis Due to Anti-Neutral Endopeptidase Antibodies. N Engl J Med (2002) 346(26):2053–60. doi: 10.1056/NEJMoa012895
10. Beck LH Jr, Bonegio RG, Lambeau G, Beck DM, Powell DW, Cummins TD, et al. M-Type Phospholipase A2 Receptor as Target Antigen in Idiopathic Membranous Nephropathy. N Engl J Med (2009) 361(1):11–21. doi: 10.1056/NEJMoa0810457
11. Tomas NM, Beck LH Jr, Meyer-Schwesinger C, Seitz-Polski B, Ma H, Zahner G, et al. Thrombospondin Type-1 Domain-Containing 7A in Idiopathic Membranous Nephropathy. N Engl J Med (2014) 371(24):2277–87. doi: 10.1056/NEJMoa1409354
12. Sethi S, Madden BJ, Debiec H, Charlesworth MC, Gross L, Ravindran A, et al. Exostosin 1/Exostosin 2-Associated Membranous Nephropathy. J Am Soc Nephrol (2019) 30(6):1123–36. doi: 10.1681/ASN.2018080852
13. Sethi S, Debiec H, Madden B, Charlesworth MC, Morelle J, Gross L, et al. Neural Epidermal Growth Factor-Like 1 Protein (NELL-1) Associated Membranous Nephropathy. Kidney Int (2020) 97(1):163–74. doi: 10.1016/j.kint.2019.09.014
14. Sethi S, Debiec H, Madden B, Vivarelli M, Charlesworth MC, Ravindran A, et al. Semaphorin 3B-Associated Membranous Nephropathy is a Distinct Type of Disease Predominantly Present in Pediatric Patients. Kidney Int (2020) 98(5):1253–64. doi: 10.1016/j.kint.2020.05.030
15. Caza TN, Hassen SI, Kuperman M, Sharma SG, Dvanajscak Z, Arthur J, et al. Neural Cell Adhesion Molecule 1 is a Novel Autoantigen in Membranous Lupus Nephritis. Kidney Int (2021) 100(1):171–81. doi: 10.1016/j.kint.2020.09.016
16. Al-Rabadi LF, Caza T, Trivin-Avillach C, Rodan AR, Andeen N, Hayashi N, et al. Serine Protease HTRA1 as a Novel Target Antigen in Primary Membranous Nephropathy. J Am Soc Nephrol (2021) 32(7):1666–81. doi: 10.1681/ASN.2020101395
17. Sethi S, Madden B, Debiec H, Morelle J, Charlesworth MC, Gross L, et al. Protocadherin 7-Associated Membranous Nephropathy. J Am Soc Nephrol (2021) 32(5):1249–61. doi: 10.1681/ASN.2020081165
18. Sethi S. New ‘Antigens’ in Membranous Nephropathy. J Am Soc Nephrol (2021) 32(2):268–78. doi: 10.1681/ASN.2020071082
19. Ronco P, Debiec H. Molecular Pathogenesis of Membranous Nephropathy. Annu Rev Pathol (2020) 15:287–313. doi: 10.1146/annurev-pathol-020117-043811
20. Ronco P, Plaisier E, Debiec H. Advances in Membranous Nephropathy. J Clin Med (2021) 10(4):607. doi: 10.3390/jcm10040607
21. Hu SL, Wang D, Gou WJ, Lei QF, Ma TA, Cheng JZ. Diagnostic Value of Phospholipase A2 Receptor in Idiopathic Membranous Nephropathy: A Systematic Review and Meta-Analysis. J Nephrol (2014) 27(2):111–6. doi: 10.1007/s40620-014-0042-7
22. Tampoia M, Migliucci F, Villani C, Abbracciavento L, Rossini M, Fumarulo R, et al. Definition of a New Cut-Off for the Anti-Phospholipase A2 Receptor (PLA2R) Autoantibody Immunoassay in Patients Affected by Idiopathic Membranous Nephropathy. J Nephrol (2018) 31(6):899–905. doi: 10.1007/s40620-018-0533-z
23. Dahnrich C, Saschenbrecker S, Gunnarsson I, Schlumberger W, Ronco P, Debiec H. Development of a Standardized Chemiluminescence Immunoassay for the Detection of Autoantibodies Against Human M-Type Phospholipase A2 Receptor in Primary Membranous Nephropathy. Kidney Int Rep (2020) 5(2):182–8. doi: 10.1016/j.ekir.2019.11.008
24. Li C, Li P, Guo W, Chen L, Li J, Wang R, et al. The Optimal Anti-Phospholipase A2 Receptor Cutoff for the Diagnosis of Idiopathic Membranous Nephropathy: A Single-Center Retrospective Study. Korean J Intern Med (2022) 37(1):154–166. doi: 10.3904/kjim.2020.366
25. Kidney Disease: Improving Global Outcomes Glomerular Diseases Work Group. KDIGO 2021 Clinical Practice Guideline for the Management of Glomerular Diseases. Kidney Int (2021) 100(4S):S1–276. doi: 10.1016/j.kint.2021.05.021
26. Li X, Wei D, Zhou Z, Wang B, Xu Y, Pan J, et al. Anti-PLA2R Antibodies in Chinese Patients With Membranous Nephropathy. Med Sci Monitor (2016) 22:1630–6. doi: 10.12659/msm.896090
27. Liu H, Luo W, Gong S, Ding X. Detection and Clinical Significance of Glomerular M-Type Phospholipase A2 Receptor in Patients With Idiopathic Membranous Nephropathy. Intern Med J (2016) 46(11):1318–22. doi: 10.1111/imj.13233
28. Radice A, Pieruzzi F, Trezzi B, Ghiggeri G, Napodano P, D’Amico M, et al. Diagnostic Specificity of Autoantibodies to M-Type Phospholipase A2 Receptor (PLA2R) in Differentiating Idiopathic Membranous Nephropathy (IMN) From Secondary Forms and Other Glomerular Diseases. J Nephrol (2018) 31(2):271–8. doi: 10.1007/s40620-017-0451-5
29. Tian C, Li L, Liu T, Qu X, Qiu Y. Circulating Antibodies Against M-Type Phospholipase A2 Receptor and Thrombospondin Type-1 Domain-Containing 7A in Chinese Patients With Membranous Nephropathy. Int Urol Nephrol (2019) 51(8):1371–7. doi: 10.1007/s11255-019-02146-w
30. Maifata SM, Hod R, Zakaria F, Ghani FA. Role of Serum and Urine Biomarkers (PLA2R and THSD7A) in Diagnosis, Monitoring and Prognostication of Primary Membranous Glomerulonephritis. Biomolecules (2020) 10(2):319. doi: 10.3390/biom10020319
31. Larsen CP, Messias NC, Silva FG, Messias E, Walker PD. Determination of Primary Versus Secondary Membranous Glomerulopathy Utilizing Phospholipase A2 Receptor Staining in Renal Biopsies. Mod Pathol (2013) 26(5):709–15. doi: 10.1038/modpathol.2012.207
32. Pang L, Zhang AM, Li HX, Du JL, Jiao LL, Duan N, et al. Serum Anti-PLA2R Antibody and Glomerular PLA2R Deposition in Chinese Patients With Membranous Nephropathy: A Cross-Sectional Study. Med (Baltimore) (2017) 96(24):e7218. doi: 10.1097/MD.0000000000007218
33. Burbelo PD, Joshi M, Chaturvedi A, Little DJ, Thurlow JS, Waldman M, et al. Detection of PLA2R Autoantibodies Before the Diagnosis of Membranous Nephropathy. J Am Soc Nephrol (2020) 31(1):208–17. doi: 10.1681/ASN.2019050538
34. Francis JM, Beck LH Jr, Salant DJ. Membranous Nephropathy: A Journey From Bench to Bedside. Am J Kidney Dis (2016) 68(1):138–47. doi: 10.1053/j.ajkd.2016.01.030
35. Qin HZ, Zhang MC, Le WB, Ren Q, Chen DC, Zeng CH, et al. Combined Assessment of Phospholipase A2 Receptor Autoantibodies and Glomerular Deposits in Membranous Nephropathy. J Am Soc Nephrol (2016) 27(10):3195–203. doi: 10.1681/ASN.2015080953
36. Rodas LM, Matas-Garcia A, Barros X, Blasco M, Vinas O, Llobell A, et al. Antiphospholipase 2 Receptor Antibody Levels to Predict Complete Spontaneous Remission in Primary Membranous Nephropathy. Clin Kidney J (2019) 12(1):36–41. doi: 10.1093/ckj/sfy005
37. Diaz M, Agraz I, Soler MJ. Anti-Phospholipase A2 Receptor Antibody and Spontaneous Remission in Membranous Nephropathy. Clin Kidney J (2019) 12(1):33–5. doi: 10.1093/ckj/sfy079
38. Dong D, Fan TT, Wang YY, Zhang L, Song L, Zhang L. Relationship Between Renal Tissues Phospholipase A2 Receptor and its Serum Antibody and Clinical Condition and Prognosis of Idiopathic Membranous Nephropathy: A Meta-Analysis. BMC Nephrol (2019) 20(1):444. doi: 10.1186/s12882-019-1638-x
39. Jullien P, Seitz Polski B, Maillard N, Thibaudin D, Laurent B, Ollier E, et al. Anti-Phospholipase A2 Receptor Antibody Levels at Diagnosis Predicts Spontaneous Remission of Idiopathic Membranous Nephropathy. Clin Kidney J (2017) 10(2):209–14. doi: 10.1093/ckj/sfw121
40. El-Zoghby ZM, Grande JP, Fraile MG, Norby SM, Fervenza FC, Cosio FG. Recurrent Idiopathic Membranous Nephropathy: Early Diagnosis by Protocol Biopsies and Treatment With Anti-CD20 Monoclonal Antibodies. Am J Transplant (2009) 9(12):2800–7. doi: 10.1111/j.1600-6143.2009.02851.x
41. Moroni G, Gallelli B, Quaglini S, Leoni A, Banfi G, Passerini P, et al. Long-Term Outcome of Renal Transplantation in Patients With Idiopathic Membranous Glomerulonephritis (MN). Nephrol Dial Transplant (2010) 25(10):3408–15. doi: 10.1093/ndt/gfq223
42. Stahl R, Hoxha E, Fechner K. PLA2R Autoantibodies and Recurrent Membranous Nephropathy After Transplantation. N Engl J Med (2010) 363(5):496–8. doi: 10.1056/NEJMc1003066
43. Quintana LF, Blasco M, Seras M, Perez NS, Lopez-Hoyos M, Villarroel P, et al. Antiphospholipase A2 Receptor Antibody Levels Predict the Risk of Posttransplantation Recurrence of Membranous Nephropathy. Transplantation (2015) 99(8):1709–14. doi: 10.1097/TP.0000000000000630
44. Gupta G, Fattah H, Ayalon R, Kidd J, Gehr T, Quintana LF, et al. Pre-Transplant Phospholipase A2 Receptor Autoantibody Concentration is Associated With Clinically Significant Recurrence of Membranous Nephropathy Post-Kidney Transplantation. Clin Transplant (2016) 30(4):461–9. doi: 10.1111/ctr.12711
45. Liu Y, Zheng S, Ma C, Lian Y, Zheng X, Guan P, et al. Meta-Analysis of the Diagnostic Efficiency of THSD7A-AB for the Diagnosis of Idiopathic Membranous Nephropathy. Glob Chall (2020) 4(11):1900099. doi: 10.1002/gch2.201900099
46. Zaghrini C, Seitz-Polski B, Justino J, Dolla G, Payre C, Jourde-Chiche N, et al. Novel ELISA for Thrombospondin Type 1 Domain-Containing 7A Autoantibodies in Membranous Nephropathy. Kidney Int (2019) 95(3):666–79. doi: 10.1016/j.kint.2018.10.024
47. Ravindran A, Casal Moura M, Fervenza FC, Nasr SH, Alexander MP, Fidler ME, et al. In Patients With Membranous Lupus Nephritis, Exostosin-Positivity and Exostosin-Negativity Represent Two Different Phenotypes. J Am Soc Nephrol (2021) 32(3):695–706. doi: 10.1681/ASN.2020081181
48. Caza TN, Hassen SI, Dvanajscak Z, Kuperman M, Edmondson R, Herzog C, et al. NELL1 is a Target Antigen in Malignancy-Associated Membranous Nephropathy. Kidney Int (2021) 99(4):967–76. doi: 10.1016/j.kint.2020.07.039
49. Rood IM, Merchant ML, Wilkey DW, Zhang T, Zabrouskov V, van der Vlag J, et al. Increased Expression of Lysosome Membrane Protein 2 in Glomeruli of Patients With Idiopathic Membranous Nephropathy. Proteomics (2015) 15(21):3722–30. doi: 10.1002/pmic.201500127
50. Wang M, Xie R, Jia X, Liu R. Urinary Volatile Organic Compounds as Potential Biomarkers in Idiopathic Membranous Nephropathy. Med Princ Pract (2017) 26(4):375–80. doi: 10.1159/000478782
51. Pang L, Li Q, Li Y, Liu Y, Duan N, Li H. Urine Proteomics of Primary Membranous Nephropathy Using Nanoscale Liquid Chromatography Tandem Mass Spectrometry Analysis. Clin Proteomics (2018) 15:5. doi: 10.1186/s12014-018-9183-3
52. Yu X, Cai J, Jiao X, Zhang S, Liu H, Ding X. Response Predictors to Calcineurin Inhibitors in Patients With Primary Membranous Nephropathy. Am J Nephrol (2018) 47(4):266–74. doi: 10.1159/000488728
53. Taherkhani A, Nafar M, Arefi-Oskouie A, Broumandnia N, Parvin M, Mahmoudieh L, et al. Metabolomic Analysis of Membranous Glomerulonephritis: Identification of a Diagnostic Panel and Pathogenic Pathways. Arch Med Res (2019) 50(4):159–69. doi: 10.1016/j.arcmed.2019.08.004
54. L’Imperio V, Smith A, Ajello E, Piga I, Stella M, Denti V, et al. MALDI-MSI Pilot Study Highlights Glomerular Deposits of Macrophage Migration Inhibitory Factor as a Possible Indicator of Response to Therapy in Membranous Nephropathy. Proteomics Clin Appl (2019) 13(3):e1800019. doi: 10.1002/prca.201800019
55. Tie C, Liu L, Feng T, Sa R, Xia Q, Liang H, et al. Differential Analysis of Urinary Albumin for Membranous Nephropathy Patients by Online Capillary Isoelectric Focusing - Mass Spectrometry. J Proteomics (2020) 216:103676. doi: 10.1016/j.jprot.2020.103676
56. Huang YS, Hsieh HY, Shih HM, Sytwu HK, Wu CC. Urinary Xist is a Potential Biomarker for Membranous Nephropathy. Biochem Biophys Res Commun (2014) 452(3):415–21. doi: 10.1016/j.bbrc.2014.08.077
57. Sha WG, Shen L, Zhou L, Xu DY, Lu GY. Down-Regulation of miR-186 Contributes to Podocytes Apoptosis in Membranous Nephropathy. BioMed Pharmacother (2015) 75:179–84. doi: 10.1016/j.biopha.2015.07.021
58. Li J, Liu B, Xue H, Zhou QQ, Peng L. miR-217 Is a Useful Diagnostic Biomarker and Regulates Human Podocyte Cells Apoptosis via Targeting TNFSF11 in Membranous Nephropathy. BioMed Res Int (2017) 2017:2168767. doi: 10.1155/2017/2168767
59. Liu D, Liu F, Wang X, Qiao Y, Pan S, Yang Y, et al. MiR-130a-5p Prevents Angiotensin II-Induced Podocyte Apoptosis by Modulating M-Type Phospholipase A2 Receptor. Cell Cycle (2018) 17(21-22):2484–95. doi: 10.1080/15384101.2018.1542901
60. Zhang W, Ren Y, Li J. Application of miR-193a/WT1/PODXL Axis to Estimate Risk and Prognosis of Idiopathic Membranous Nephropathy. Ren Fail (2019) 41(1):704–17. doi: 10.1080/0886022X.2019.1642210
61. Li J, Chen Y, Shen L, Deng Y. Improvement of Membranous Nephropathy by Inhibition of miR-193a to Affect Podocytosis via Targeting WT1. J Cell Biochem (2019) 120(3):3438–46. doi: 10.1002/jcb.27616
62. Zhou G, Zhang X, Wang W, Zhang W, Wang H, Xin G. Both Peripheral Blood and Urinary miR-195-5p, miR-192-3p, miR-328-5p and Their Target Genes PPM1A, RAB1A and BRSK1 May Be Potential Biomarkers for Membranous Nephropathy. Med Sci Monit (2019) 25:1903–16. doi: 10.12659/MSM.913057
63. Barbagallo C, Passanisi R, Mirabella F, Cirnigliaro M, Costanzo A, Lauretta G, et al. Upregulated microRNAs in Membranous Glomerulonephropathy are Associated With Significant Downregulation of IL6 and MYC mRNAs. J Cell Physiol (2019) 234(8):12625–36. doi: 10.1002/jcp.27851
64. Jin X, Deng B, Ye K, Ye D, Huang Y, Chen X, et al. Comprehensive Expression Profiles and Bioinformatics Analysis Reveal Special Circular RNA Expression and Potential Predictability in the Peripheral Blood of Humans With Idiopathic Membranous Nephropathy. Mol Med Rep (2019) 20(5):4125–39. doi: 10.3892/mmr.2019.10671
65. Jin LW, Pan M, Ye HY, Zheng Y, Chen Y, Huang WW, et al. Down-Regulation of the Long Non-Coding RNA XIST Ameliorates Podocyte Apoptosis in Membranous Nephropathy via the miR-217-TLR4 Pathway. Exp Physiol (2019) 104(2):220–30. doi: 10.1113/EP087190
66. Hejazian SM, Ardalan M, Shoja MM, Samadi N, Zununi Vahed S. Expression Levels of miR-30c and miR-186 in Adult Patients With Membranous Glomerulonephritis and Focal Segmental Glomerulosclerosis. Int J Nephrol Renovasc Dis (2020) 13:193–201. doi: 10.2147/IJNRD.S258624
67. Wu L, Zhang X, Luo L, Li X, Liu Y, Qin X. Altered Expression of Serum miR-106a, miR-19b, miR-17, and PTEN in Patients With Idiopathic Membranous Nephropathy. J Clin Lab Anal (2021) 35(4):e23737. doi: 10.1002/jcla.23737
68. Sun Z, Xu Q, Ma Y, Yang S, Shi J. Circ_0000524/miR-500a-5p/CXCL16 Axis Promotes Podocyte Apoptosis in Membranous Nephropathy. Eur J Clin Invest (2021) 51(3):e13414. doi: 10.1111/eci.13414
69. Neal CS, Michael MZ, Pimlott LK, Yong TY, Li JY, Gleadle JM. Circulating microRNA Expression is Reduced in Chronic Kidney Disease. Nephrol Dial Transplant (2011) 26(11):3794–802. doi: 10.1093/ndt/gfr485
70. Lorenzen JM, Thum T. Circulating and Urinary microRNAs in Kidney Disease. Clin J Am Soc Nephrol (2012) 7(9):1528–33. doi: 10.2215/CJN.01170212
71. Chen W, Lin X, Huang J, Tan K, Chen Y, Peng W, et al. Integrated Profiling of microRNA Expression in Membranous Nephropathy Using High-Throughput Sequencing Technology. Int J Mol Med (2014) 33(1):25–34. doi: 10.3892/ijmm.2013.1554
72. Zhang J, Zhu Y, Cai R, Jin J, He Q. Differential Expression of Urinary Exosomal Small RNAs in Idiopathic Membranous Nephropathy. BioMed Res Int (2020) 2020:3170927. doi: 10.1155/2020/3170927
73. Ma H, Xu Y, Zhang R, Guo B, Zhang S, Zhang X. Differential Expression Study of Circular RNAs in Exosomes From Serum and Urine in Patients With Idiopathic Membranous Nephropathy. Arch Med Sci (2019) 15(3):738–53. doi: 10.5114/aoms.2019.84690
74. Kuroki A, Iyoda M, Shibata T, Sugisaki T. Th2 Cytokines Increase and Stimulate B Cells to Produce IgG4 in Idiopathic Membranous Nephropathy. Kidney Int (2005) 68(1):302–10. doi: 10.1111/j.1523-1755.2005.00415.x
75. Sirimongkolrat T, Premasathian N, Vongwiwatana A, Limsrichamrern S, Cheunsuchon B, Vasuvattakul S. Anti-CD20 Monoclonal Antibody (Rituximab) for the Treatment of Membranous Nephropathy After Living-Unrelated Kidney Transplantation: A Case Report. Transplant Proc (2008) 40(7):2440–1. doi: 10.1016/j.transproceed.2008.07.074
76. Ruggenenti P, Cravedi P, Chianca A, Perna A, Ruggiero B, Gaspari F, et al. Rituximab in Idiopathic Membranous Nephropathy. J Am Soc Nephrol (2012) 23(8):1416–25. doi: 10.1681/ASN.2012020181
77. Wang B, Zuo K, Wu Y, Huang Q, Qin WS, Zeng CH, et al. Correlation Between B Lymphocyte Abnormality and Disease Activity in Patients With Idiopathic Membranous Nephropathy. J Int Med Res (2011) 39(1):86–95. doi: 10.1177/147323001103900111
78. Suarez-Fueyo A, Bradley SJ, Klatzmann D, Tsokos GC. T Cells and Autoimmune Kidney Disease. Nat Rev Nephrol (2017) 13(6):329–43. doi: 10.1038/nrneph.2017.34
79. Grant CR, Liberal R, Mieli-Vergani G, Vergani D, Longhi MS. Regulatory T-Cells in Autoimmune Diseases: Challenges, Controversies and–Yet–Unanswered Questions. Autoimmun Rev (2015) 14(2):105–16. doi: 10.1016/j.autrev.2014.10.012
80. Motavalli R, Etemadi J, Soltani-Zangbar MS, Ardalan MR, Kahroba H, Roshangar L, et al. Altered Th17/Treg Ratio as a Possible Mechanism in Pathogenesis of Idiopathic Membranous Nephropathy. Cytokine (2021) 141:155452. doi: 10.1016/j.cyto.2021.155452
81. Roccatello D, Sciascia S, Di Simone D, Solfietti L, Naretto C, Fenoglio R, et al. New Insights Into Immune Mechanisms Underlying Response to Rituximab in Patients With Membranous Nephropathy: A Prospective Study and a Review of the Literature. Autoimmun Rev (2016) 15(6):529–38. doi: 10.1016/j.autrev.2016.02.014
82. Rosenzwajg M, Languille E, Debiec H, Hygino J, Dahan K, Simon T, et al. B- and T-Cell Subpopulations in Patients With Severe Idiopathic Membranous Nephropathy may Predict an Early Response to Rituximab. Kidney Int (2017) 92(1):227–37. doi: 10.1016/j.kint.2017.01.012
83. Shi X, Qu Z, Zhang L, Zhang N, Liu Y, Li M, et al. Increased Ratio of ICOS(+)/PD-1(+) Follicular Helper T Cells Positively Correlates With the Development of Human Idiopathic Membranous Nephropathy. Clin Exp Pharmacol Physiol (2016) 43(4):410–6. doi: 10.1111/1440-1681.12555
Keywords: membranous nephropathy, biomarker, phospholipase A2 receptor, proteome, metabolome, noncoding RNA, immune cell
Citation: Liu Q, Liu J, Lin B, Zhang Y, Ma M, Yang M and Qin X (2022) Novel Biomarkers in Membranous Nephropathy. Front. Immunol. 13:845767. doi: 10.3389/fimmu.2022.845767
Received: 30 December 2021; Accepted: 25 February 2022;
Published: 22 April 2022.
Edited by:
Kenneth Michael Pollard, The Scripps Research Institute, United StatesReviewed by:
Anastasios E Germenis, University of Thessaly, GreeceFlorencia Rosetti, Instituto Nacional de Ciencias Médicas y Nutrición Salvador Zubirán (INCMNSZ), Mexico
Copyright © 2022 Liu, Liu, Lin, Zhang, Ma, Yang and Qin. This is an open-access article distributed under the terms of the Creative Commons Attribution License (CC BY). The use, distribution or reproduction in other forums is permitted, provided the original author(s) and the copyright owner(s) are credited and that the original publication in this journal is cited, in accordance with accepted academic practice. No use, distribution or reproduction is permitted which does not comply with these terms.
*Correspondence: Xiaosong Qin, cWlueHNAc2otaG9zcGl0YWwub3Jn