- 1Department of Immunology, Faculty of Medical Sciences, Tarbiat Modares University, Tehran, Iran
- 2Department of Immunology, School of Medicine, Iran University of Medical Sciences, Tehran, Iran
- 3Department of Immunology, School of Medicine, Shahid Beheshti University of Medical Sciences, Tehran, Iran
Various factors, including viral and bacterial infections, autoimmune responses, diabetes, drugs, alcohol abuse, and fat deposition, can damage liver tissue and impair its function. These factors affect the liver tissue and lead to acute and chronic liver damage, and if left untreated, can eventually lead to cirrhosis, fibrosis, and liver carcinoma. The main treatment for these disorders is liver transplantation. Still, given the few tissue donors, problems with tissue rejection, immunosuppression caused by medications taken while receiving tissue, and the high cost of transplantation, liver transplantation have been limited. Therefore, finding alternative treatments that do not have the mentioned problems is significant. Cell therapy is one of the treatments that has received a lot of attention today. Hepatocytes and mesenchymal stromal/stem cells (MSCs) are used in many patients to treat liver-related diseases. In the meantime, the use of mesenchymal stem cells has been studied more than other cells due to their favourable characteristics and has reduced the need for liver transplantation. These cells increase the regeneration and repair of liver tissue through various mechanisms, including migration to the site of liver injury, differentiation into liver cells, production of extracellular vesicles (EVs), secretion of various growth factors, and regulation of the immune system. Notably, cell therapy is not entirely excellent and has problems such as cell rejection, undesirable differentiation, accumulation in unwanted locations, and potential tumorigenesis. Therefore, the application of MSCs derived EVs, including exosomes, can help treat liver disease and prevent its progression. Exosomes can prevent apoptosis and induce proliferation by transferring different cargos to the target cell. In addition, these vesicles have been shown to transport hepatocyte growth factor (HGF) and can promote the hepatocytes’(one of the most important cells in the liver parenchyma) growths.
1 Introduction
Inflammation is an immunological condition that has an important role in controlling microbial infections and is the basis of many natural physiological events of the body in healthy conditions (1). This physiological process plays a vital role in embryo implantation, tissue repair, fetal growth, etc. (2). These inflammations must be carefully regulated to maintain the homeostasis of various tissues and organs. Failure to control inflammation can lead to multiple injuries to the tissues involved and lead to the loss of part or all of that tissue (3). Hemostatic inflammation is an integral part of liver health, and treatment strategies should focus on reversing pathological inflammation to hemostatic inflammation (4).
The liver is one of the vital organs that has a great ability to regenerate itself due to liver cells’ characteristics (5, 6). But when the amount of damage is so much and exceeds liver cells’ ability to regenerate, the function of this tissue is impaired (7). Acute liver failure (ALF) is more common in adolescents and is associated with high mortality (8). In ALF, the metabolic and immunological function of the liver is impaired. It presents with manifestations such as blood coagulation defects, cardiovascular instability, liver encephalopathy, susceptibility to infection, and progressive multiple organ failure (9, 10). The interval between the onset of symptoms and the onset of hepatic encephalopathy distinguishes different forms of the disease (11). In this way, if the time between them is a few hours, it is called hyperacute liver failure (12), but if this interval occurs more slowly and lasts from a few days to a few weeks, it is called acute or sub-acute damage (13). Fibrosis is an intrinsic response to injury that maintains the integrity of the various organs involved in tissue damage due to extensive necrosis or apoptosis (14). Chronic liver disease occurs during persistent inflammation and is associated with the destruction and regeneration of the liver parenchyma, which eventually leads to fibrosis and cirrhosis. There are many different causes for chronic liver disease, autoimmune diseases, including long-term alcohol abuse, toxins, infections, genetic and metabolic disorders (15). For example, during myocardial infarction, fibrosis and collagen fibres become apparent by the end of the first week, preventing heart rupture by maintaining the heart’s structure (16). Tissue fibrosis is also seen in the kidney after ischemia (17) and in the lung after H5N1 influenza and COVID-19 infection (18–20). Figure 1 summarizes some acute and chronic liver diseases and the main mechanisms involved in these diseases development (Figure 1). It is worth noting that various treatments can prevent the progression of liver fibrosis (21) but do not lead to the reversion of fibrous tissue, and there is no definitive treatment for fibrosis.
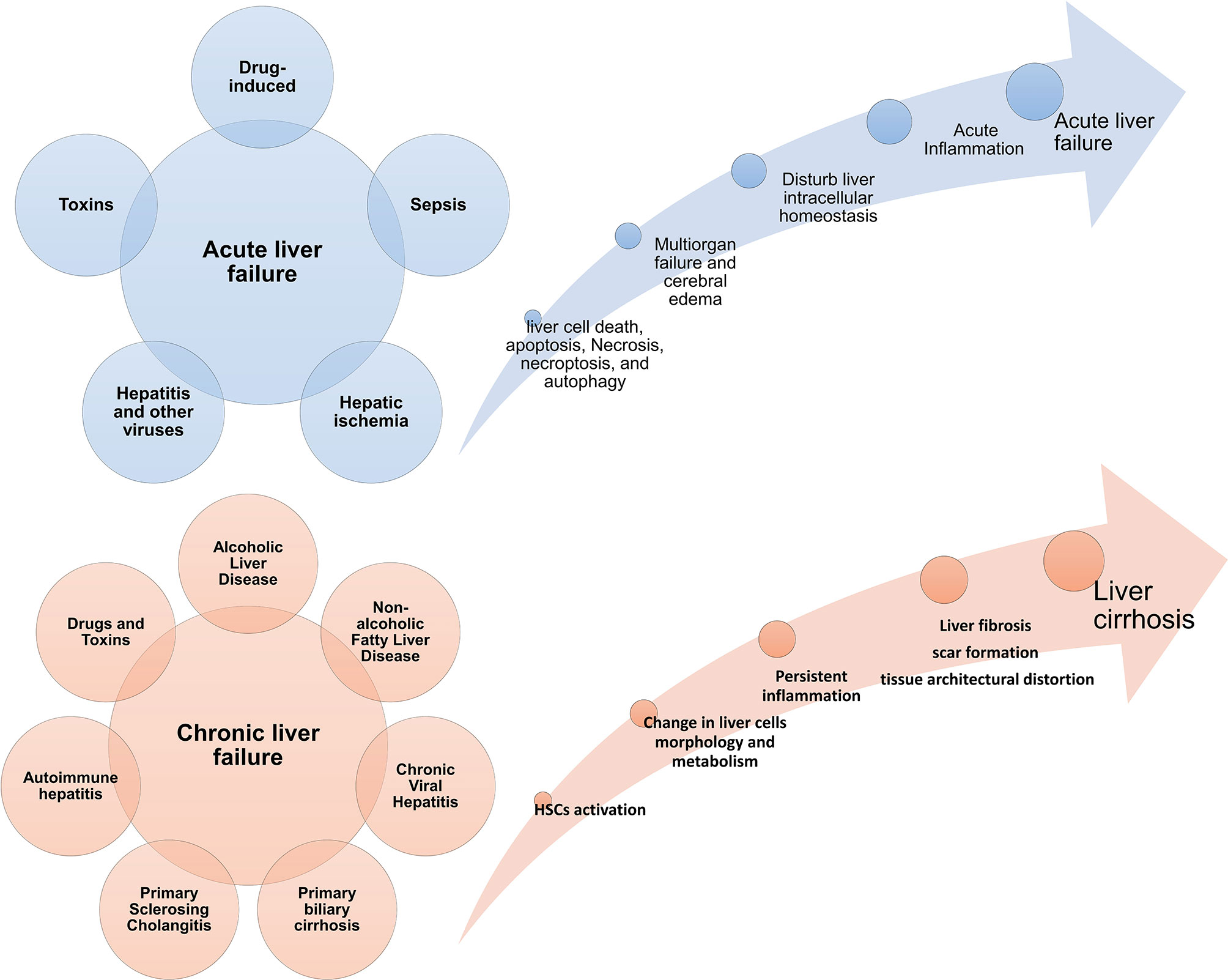
Figure 1 Acute and chronic liver diseases. Diseases Different conditions can cause acute and chronic inflammatory conditions in the liver. If the damages are not controlled in the acute stage, they progress to a chronic state and are associated with fibrosis and cirrhosis of the liver tissue. Failure in acute liver disease treatment can also lead to the onset and progression of liver cancer; HSC, hepatic stellate cell.
There is currently no specific drug for treating liver disease, and many of the current treatments are used only in very acute cases due to their side effects (22). Therefore, there is an urgent need to find appropriate treatment methods to prevent liver disease progression and repair liver tissue. Today cell therapy is one of the fields that has raised many hopes and interests in the field of regenerative medicine. Cells such as mesenchymal stromal/stem cells, hepatocytes, hematopoietic cells, immune system cells and endothelial progenitor cells have been used in various studies to treat liver disease (23).
There are advantages and disadvantages to using each of these cells, which are summarized in Table 1. Among these cells, mesenchymal stem cells have been used extensively in studies due to their desirable properties and have shown promising results. The desirable characteristics of these cells are various isolation sources, easy isolation methods, easy culture methods, expansion and storage, low immunogenicity, self-renewal ability, ability to differentiate into different cell types, immunomodulatory properties, The ability to produce soluble factors such as exosomes and growth factors and the ability to migrate to the site of tissue damage were noted (39, 40). Due to the favourable properties of MSCs-derived extracellular vesicles (EVs), the therapeutic ability of these vesicles has been investigated. This review discusses the effect of inflammation on the progression of liver disease, the role of liver cells and immune cells in the pathogenesis of the liver disease, and studies of MSCs and their EVs (mechanisms).
2 Inflammation Role in Liver Diseases (LDs)
Under physiological conditions, the liver constantly contacts food-derived foreign proteins, drugs, chemicals, toxins, and intestinal microbiota (41). Kupffer cells (KCs) and dendritic cells (DCs), as well as circulating immune cells such as bone marrow-derived macrophages, natural killer (NK) cells, and neutrophils (42), play an important role in the formation of the liver immune microenvironment and hepatic immune responses (43).
Kupffer cells become highly active in the liver during this disease and produce inflammatory cytokines, including IL-1, IL-6, and TNF-α. In addition, due to the activation of other immune system cells that are recruited from the bloodstream to the liver tissue, systemic inflammation develops that can stimulate necrosis and apoptosis in hepatocytes (44, 45). Overall, inflammation, immune cells and liver cells inflammatory responses play an essential role in developing liver-related diseases (46).
Hepatic steatosis, or fatty liver disease (FLD), is the most common liver disease in the United States (47), occurs in response to alcohol, chemotherapy, toxins such as vanilla chloride, and insulin-related metabolic syndrome and can lead to liver tissue damage (48). This damage is associated with inflammation and fibrosis, alters hepatocyte gene expression, and leads to increased TLRs ligands, TGF-β, CXCL10, and IL-1A receptors (49). In hepatitis, activation of the NF-κB signaling pathway in activated hepatocytes has also been observed. In this pathway, the activated cells release several pro-inflammatory cytokines and chemokines such as TNF-α, IL-6, and CCL2, which mediate liver inflammation (50). Inflammation can lead to necrosis and necroptosis of liver cells (especially in hepatocytes) and result in releasing of a group of molecules called alarmin from them. Alarmins such as the high-mobility group B1 (HMGB1), IL-33, ATP, and formyl peptide is released from necrotic cells (51). In fact, ATP and formyl peptide act as absorbers for neutrophils to the site of tissue damage in the liver. Activation of inflammasomes by reactive oxygen species (ROS) from damaged cells and other damage-associated molecular patterns (DAMPs) can also play a role in liver inflammation (52, 53). As a result of liver inflammation, extensive necrosis and apoptosis in hepatocytes eventually lead to loss of liver function (54). Therefore, controlling inflammatory responses is of particular importance and, if left untreated, can lead to fibrosis (55).
Fibrosis that has occurred in chronic liver disease and inflammation has been extensively studied, but its underlying mechanism in acute liver failure is still unclear (56). The progression of fibrosis leads to cirrhosis, hepatocellular carcinoma, liver failure, and portal hypertension (57). Liver fibrosis is caused by the activation of hepatic stellate cells (HSCs) and the extracellular matrix (ECM) deposition (58). When these cells are activated, the amount of vitamin A and adipogenic-related transcription factors decreases and leads to their differentiation into myofibroblasts, which are the main source of ECM production in liver fibrosis (59). In addition, HSCs contribute to the progression of liver fibrosis by producing inflammatory cytokines and chemokines (60). Immune system cells, especially KCs and circulating macrophages, play a significant role in the TGF-β1-mediated activation of the liver HSCs and increase their survival by the NF-κB-dependent manner (61). reactive oxygen species (ROS) production by KCs and macrophages is another fibrosis stimulant in hepatitis that stimulates the production of collagen 1 in HSCs/myofibroblasts (62).
In response to lipopolysaccharide (LPS), HSCs express chemokines such as IP-10, MCP-1, MIP-1α, MIP-1β, MIP-2, RANTES, and adhesive molecules E-selectin, ICAM-1, VCAM-1, and leading to migration of immune system cells into the liver (63). If liver fibrosis is left untreated, Cirrhosis develops. Cirrhosis is the end stage of progressive fibrosis that lacks effective and comprehensive medical treatment (64). Liver cancer is another consequence of fibrosis and liver inflammation.
Studies have identified the importance of inflammation-related signaling pathways and transcription factors, including STAT3 (55) and NF-κB (65), in liver cancer progression by four mechanisms. (1) Increases the expression of epithelial-to-mesenchymal transition (EMT) associated genes, including matrix metalloproteinases (MMPs) 2 and 9 (in breast cancer), E-cadherin, TWIST (in nasopharyngeal cancer), and cathepsins B. (2) Stimulates angiogenesis and increases tumor growth by regulating the expression of vascular endothelial growth factor (VEGF) (66). (3) Increases the expression of Bcl-2 members and cFLIP families and helps neoplastic cells proliferation and inhibit their apoptosis (67). (4) Increase production of inflammatory cytokines such as IL-1α, TNF-α, IL-1β, EGF-R, IL-6, and CCL2 (50, 68).
3 The Role of Liver Cells in Liver Inflammation
There are some major types of cells in the liver that regulate liver different functions, including hepatocytes, hepatic sinusoidal endothelial cells (LSECs), bile epithelial cells (cholangiocytes), HSCs, and KCs (69). Each of these cells performs their specific function, and a defect in their function leads to a defect in the function of the liver (70). In addition, some of these cells play vital roles in regulating the liver microenvironment and participate in inflammation and immune responses (Figure 2).
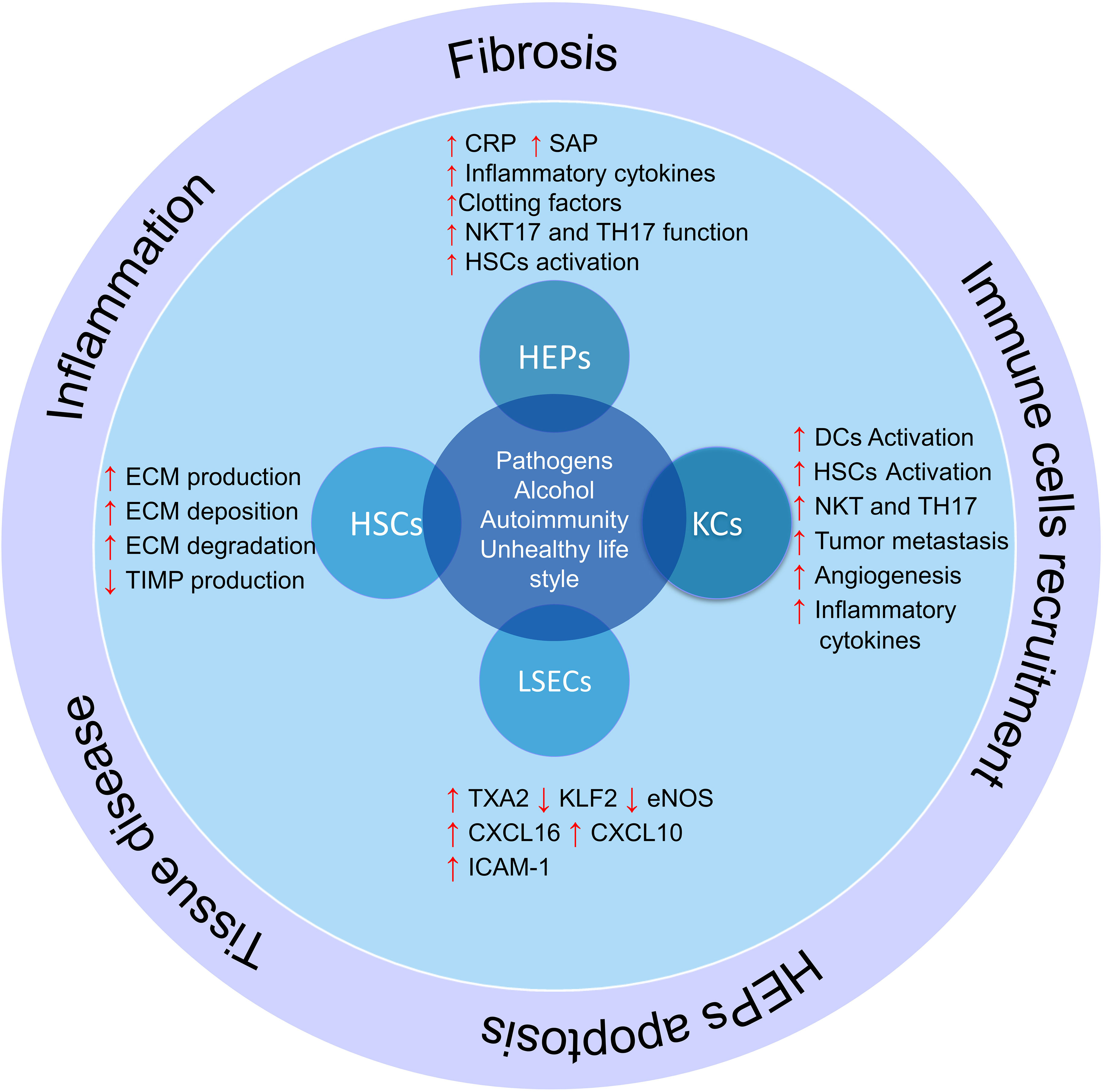
Figure 2 The role of liver cells in inflammation and liver damage. Liver cells help produce and activate immune cells by producing cytokines and inflammatory chemokines. On the other hand, hepatic stellate cells produce fibrosis of the liver tissue and lose their function by producing different components of the extracellular matrix and differentiation into myofibroblasts in inflammatory conditions; HSCs, hepatic stellate cells; KCs, Kupffer cells; LSECs, hepatic sinusoidal endothelial cells; HEPs, Hepatocytes; DCs, Dendritic cells; ECM, extracellular matrix; TXA2, Thromboxane A2; KLF2, Krüppel factor 2 transcription factor; TIMP, Tissue inhibitors of matrix metalloproteinases.
3.1 Hepatocytes
Hepatocytes are the most abundant liver cells, which make up about 90% of its biomass, and are one of the main culprits in the liver’s inflammatory response (71). In pathological conditions, hepatocytes produce different chemokines that trigger the immune system cells and create a complex local reaction at the site of infection (72). Hepatocytes express a large number and variety of pattern recognition receptors and identify different types of molecular patterns with pathogens and damage (PAMP, DAMP) (73). (1) Cell surface receptors such as quasi-tuft receptors such as TLR2,4; (2) Endosomal receptors such as TLR3 and (3) Cytoplasmic receptors, such as stimulators of IFN [STING] genes, members of the nucleotide-binding oligomerization domain (NOD) family, and retinoic acid 1 (RIG-1) induction gene (72). During the acute phase response, circulating levels of several proinflammatory cytokines, including IL-1a, TNF-a, and IL-6, increase (74). The most important cytokine affecting hepatocytes’ function is IL-6 (75) and induces the expression of acute-phase proteins including serum amyloid A, reactive protein C, haptoglobin, α1-antichymotrypsin and fibrinogen (76).
Hepatocytes also interact with innate and acquired immune cells and activate or inhibit their responses by expressing different ligands. These cells express MIC-A, MIC-B, and CD1d (in mice, not humans) and thus interact with NK and NKT cells (76, 77). There is also evidence that these cells interact with T cells to alter their responses and regulate their function (78, 79).
3.2 Kpffer Cells (KCs)
Kupffer cells make up 20% of non-parenchymal cells in the liver and are located around the portal vein (80). Therefore, due to the potential and different functions of these cells, they play an important role in liver immunology, tissue homeostasis, as well as various liver diseases, including liver cancer, ischemia-reperfusion (I/R) injury, liver fibrosis and infectious diseases (81). Actually, different signals in the liver microenvironment, such as PAMPs and DAMPs, affect the function of Kupffer cells. For example, HMGB1 released by hepatocytes can activate KCs in Acetaminophen-induced liver injury (AILI), Non-alcoholic steatohepatitis (NASH), and I/R injury (82, 83).In liver diseases, KCs interact with other liver cells such as hepatocytes, cholangiocytes, LSECs, HSCs, and other immune cells. This interaction can exacerbate tissue damage or help to repair and regenerate the liver tissue depending on the type of cells and signalling pathways (81).
3.3 Hepatic Stellate Cells
HSCs, as another main cell of liver tissue, make up 13% of sinusoidal cells and 5 to 8% of all liver cells that perform various functions (84). Among the physiological roles of these cells is (1) synthesis of ECM, (2) regulation of sinusoidal blood flow, (3) storage of vitamin A and (4) synthesis of metalloproteinases (85). After various damage to liver tissue (cause inappropriate function) and apoptosis of its cells, HSCs loses their fat-rich granules and differentiate into trans-smooth muscle alpha-actin containing myofibroblasts, producing ECM and inflammatory cytokines (86). Table 2 shows the different functions of these cells and their role in liver inflammation in various diseases (Table 2).
3.4 Liver Sinusoidal Endothelial Cells
LSECs are one of the most specialized endothelial cells with high permeability and have the highest capacity for endocytosis that interface with blood cells on the one side and hepatocytes and HSCs on the other (96). Under pathological conditions, the function of LSECs is changed (97). Capillarization is a process that LSECs acquire vasoconstrictive, thrombotic, and proinflammatory properties (98). During I/R injury and hypoxia due to disruption of blood flow to the liver, LSECs become rounded cells due to vacuolation of the nuclei and reduced ATP supply. In addition, stress on blood flow to the liver leads to a decrease in the Krüppel factor 2 transcription factor (KLF2) in LSECs and its target genes, including the endothelial nitric oxide synthase (eNOS) (99). Since the expression of ICAM-1 and Stabilin-1 in LSECs increases during inflammation, the transmigration of immune cells increases to the liver tissue (100, 101). Expression of mentioned adhesion molecules by LSECs leads to platelet adhesion, vascular microthrombi formation, and platelet-activating factor (PAF) production by platelets, which activates neutrophils and increases ROS production and damages liver tissue (97).
In chronic inflammation, these cells lose their function and play a key role in the onset and progression of chronic liver disease through four processes: sinus capillary formation, angiogenesis, angiocrine signals, and vasoconstriction (102). Decreased NO produced by eNOS by decreased KLF2 activity and increased ROS leads to HSC activation, which is associated with the production and deposition of ECM, increased vasoconstrictors including TXA2 and endothelin-1, and the production of proinflammatory cytokines (103–105).
4 Mesenchymal Stem Cells Application in LDs
In vitro studies and co-culture of MSCs with liver tissue-derived cells can increase our understanding of their therapeutic properties and their effectiveness in the treatment of liver disease (106) (Figure 3). In vivo injections of MSCs into animals to treat various liver diseases have shown very promising results (107). These cells exert their therapeutic functions in laboratory models through various mechanisms (108), and in many studies, only some of the therapeutic aspects of these cells have been addressed. Overall these studies show that MSCs exert their therapeutic effect by modulating the immune environment and increasing the regeneration of liver tissue.
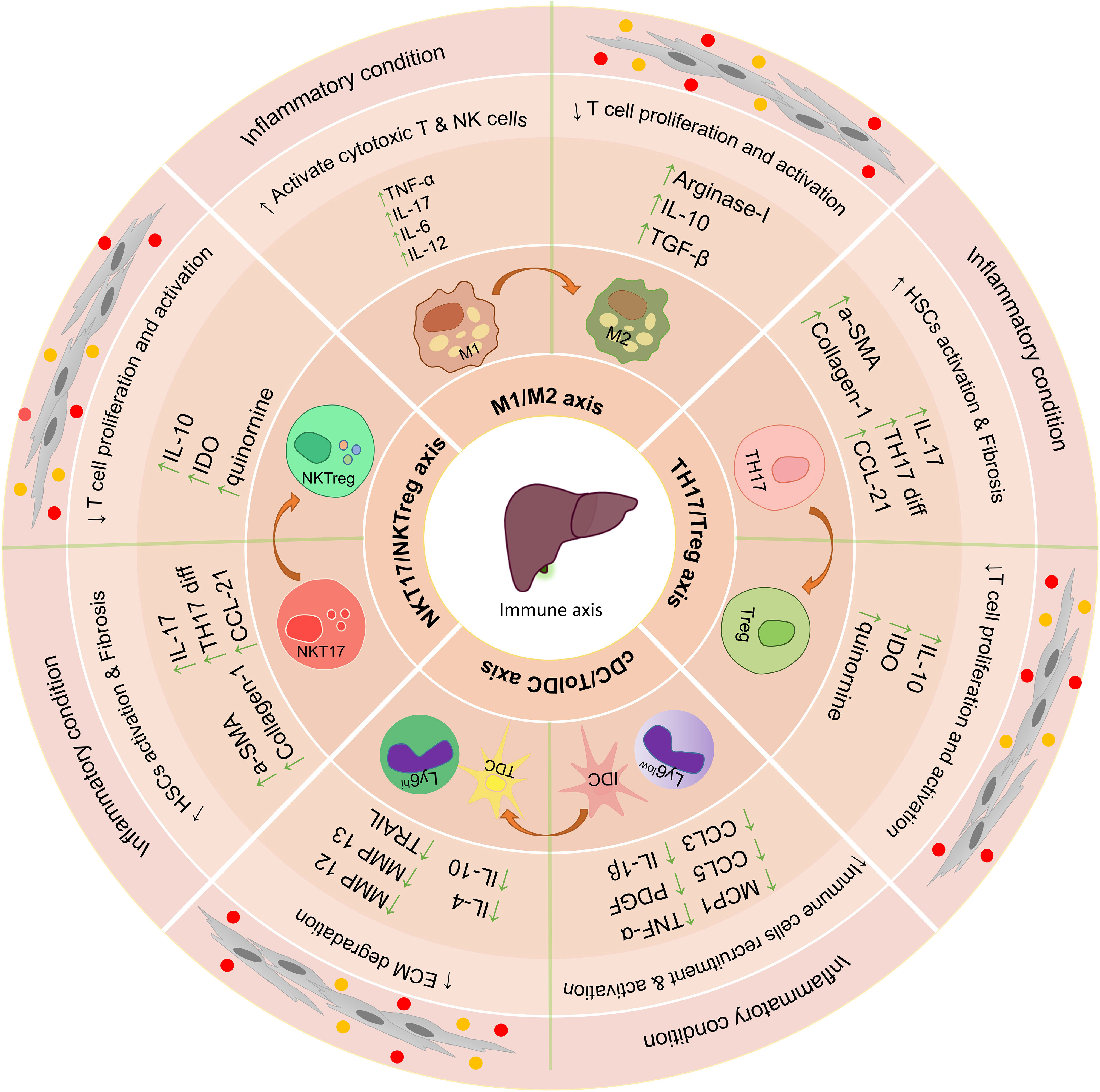
Figure 3 The effect of using mesenchymal stem cells and extracellular vesicles on the important axis of the immune system in the liver. Immunomodulatory properties of mesenchymal stem cells and their extracellular vesicles lead to the suppression of inflammatory responses in the liver’s microenvironment and increase anti-inflammatory responses. Some of the most important axis of the immune system involved in liver disease is TH17/Treg, NKT17/NKTreg, and M1/M2. The use of mesenchymal stem cells and extracellular vesicles leads to suppression of function Th17, NKT17, M1, and IDC and increase the differentiation and function of Treg, NKTreg, M2, and Tol DCs; HSCs, hepatic stellate cells; KCs, Kupffer cells; LSECs, hepatic sinusoidal endothelial cells; HEPs, Hepatocytes; Tol DCs, Tolerogenic dendritic cells; cDC, Classic dendritic cells; ECM, extracellular matrix; MSCs, Mesenchymal stromal/stem cells;, M1 Macrophage type 1; M2, Macrophage type 2; MMPs, Matrix metalloproteinase; EVs, Extracellular vesicles.
4.1 The Role of MSCs in Modulating Immune and Inflammatory Responses
Considering the significant role of immune cells in inflammation-induced liver damage, it is very important to evaluate the therapeutic effects of MSCs on localized hepatic inflammatory responses. MSCs perform their immunomodulatory functions by cell-cell interaction or by producing soluble factors such as cytokines and extracellular vesicles (Figure 4).
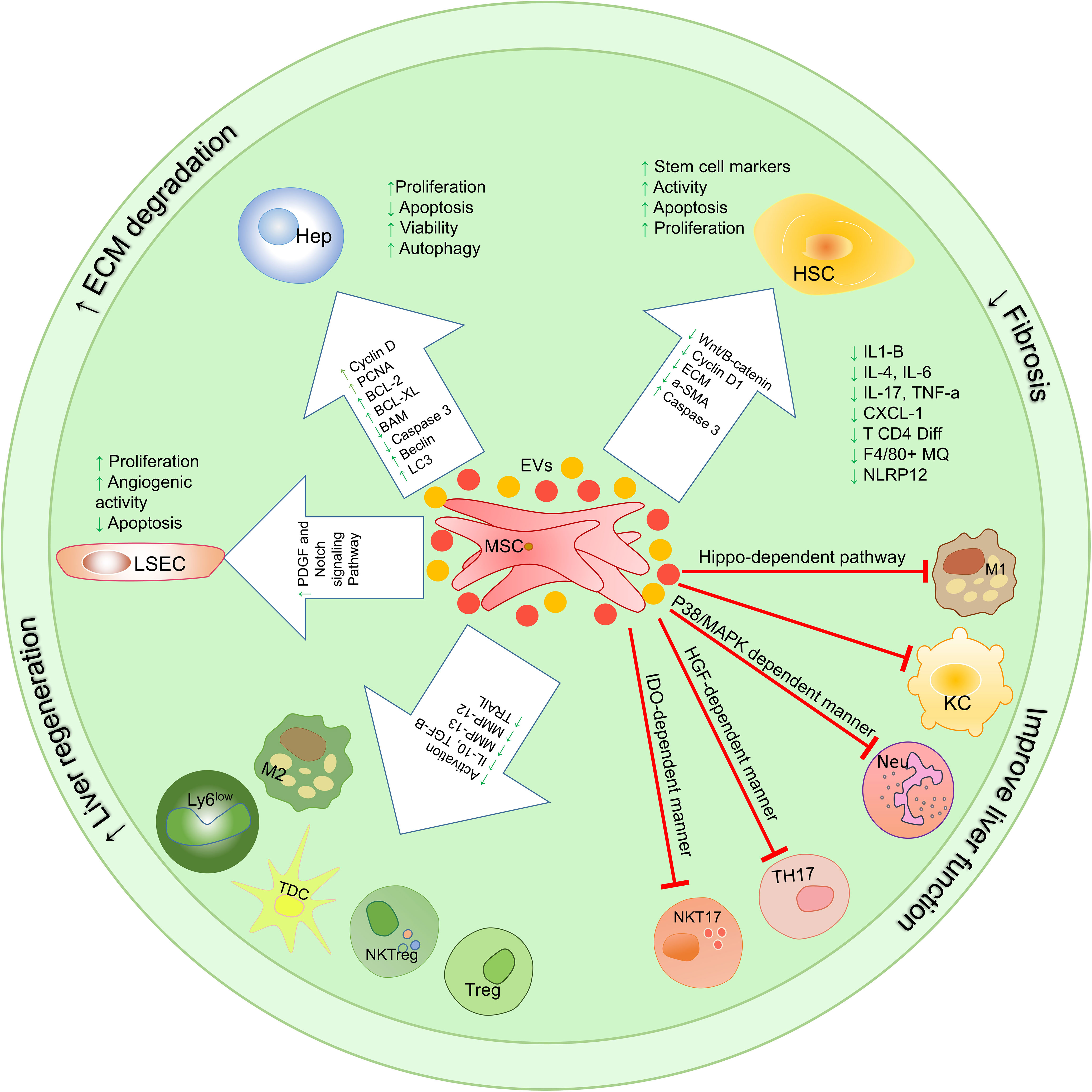
Figure 4 Therapeutic mechanisms involved in using mesenchymal stem cells and their extracellular vesicles on the function of liver cells and immune system cells. MSC-EVs exert their therapeutic functions through 3 mechanisms that have been studied in vitro, pre-clinical, and clinical trials. These mechanisms include (1) proliferation induction/apoptosis suppression, (2) modulation of immune system responses, (3) reduction of fibrosis; HSCs, hepatic stellate cells; KCs, Kupffer cells; LSECs, hepatic sinusoidal endothelial cells; HEPs, Hepatocytes; TolDCs, Tolerogenic dendritic cells; cDC, Classic dendritic cells; ECM, extracellular matrix; MSCs, Mesenchymal stromal/stem cells;, M1 Macrophage type 1; M2, Macrophage type 2; MMPs, Matrix metalloproteinase; EVs, Extracellular vesicles.
4.1.1 Impact on Differentiation and Function of DCs
A study by Yi Zhang et al. (109) Showed that injection of MSCs in the model of liver tissue damage induced by LPS and P. acnes (in mice) stimulates the differentiation of CD11c+ B220- precursors to CD11c+, MHCIIhi, CD80low, CD86low tolerogenic dendritic cells. Further studies showed that MSC-derived prostaglandin E2 (PGE2) binds to the EP4 receptor on the surface of precursors of DCs and plays a key role in their differentiation into regulatory DCs in a phosphoinositide-3-kinase-dependent manner. These DCs produce more TGF-b and IL-10 than conventional DCs and stimulate the differentiation of CD4 + T cells into Treg cells (110). Therefore, these dendritic cells help improve inflammatory damage in the liver by suppressing the activity and differentiation of inflammatory T cells, including Th1 cells (109).
4.1.2 Impact on Macrophages
MSCs play an essential role in modulating macrophages’ M1/M2 axis. It has been shown that the co-culture of bone marrow-derived macrophages with MSCs directs their differentiation through the Hippo-dependent pathway to M2 (111).
As mentioned, monocytes/macrophages play a very important role in liver fibrosis. In mice, Ly6Chi macrophages activate HSCs by producing various cytokines and chemokines, including TGF-β, TNF-α, PDGF, MCP1, IL-1β, CCL5, and CCL3 (62). However, Ly6Clo macrophages suppress the functions of HSCs and stimulate apoptosis in these cells by producing and secreting MMPs 12 and 13 and positive regulation of TNF-induced apoptosis ligand (TRAIL) (112). A study by Yuan-hui Li et al. Showed that transplantation of bone marrow-derived MSCs (BM-MSCs) in C57BL/6 J mice with liver fibrosis reduces serum alanine aminotransferase (ALT) levels and collagen deposition in the liver tissue. A sharp decrease in profibrogenic cytokines including IL-1β, TNF-α, and PDGF derived from profibrotic cells such as Ly6Chi macrophages has also been observed in mice receiving MSCs (113). In fact, transplantation of BM-MSCs has prevented the activation of HSCs through an indirect mechanism by inhibiting Ly6Chi macrophages’ function. These macrophages are the most common cells in the fibrotic liver, which increase in proportion to the healthy liver as the liver fibrosis. It is noteworthy that during the treatment of these mice with BM-MSCs, a phenotypic change occurs in macrophages from the Ly6Chi profibrotic subtype to the regenerative Ly6Clow, IL-4, IL-10 producing subtype. After BM-MSC transplantation in these mice, the ratio of Ly6Chi/Ly6Clow macrophages was reduced by 51% compared to the PBS-treated control group. Therefore, treatment of mouse liver fibrosis model with allogeneic BM-MSCs helps improve liver tissue by suppressing cells involved in liver fibrosis on the one hand and by expanding fibrosis inhibitory cells on the other hand (113).
4.1.3 Neutrophils Migration Suppression
The results of previous studies show that in IRI-related liver injury, innate immune responses play a significant role in inflammatory responses (114). Neutrophils are the most abundant in the bloodstream and reach the site of liver damage as the first leukocytes (115). It has been reported that the severity of the disease and liver damage in I/R and alcoholic liver disease, the amount of neutrophils in the liver tissue is directly related to the severity of the injury (115, 116). However, the induction of liver damage in animals whose neutrophils decreased before induction leads to the limitation of liver damage (117). Therefore, suppressing the harmful responses of these cells can help improve the disease condition (118). The results of a study conducted in 2018 by Shihui Li et al. Show that the use of mesenchymal stem cells in rats with induced liver damage reduces liver damage by lowering neutrophil recruitment and chemotaxis compared to the control group (119).
A chemokine receptor called CXC chemokine receptor 2 (CXCR2) regulates the neutrophil release from bone marrow and its chemotaxis to the inflammation site (120). This study shows that the expression of this receptor on neutrophils in rats with liver damage is significantly reduced after the injection of MSCs (119). In addition, the expression of CXCL2, which is one of the most important chemokines in neutrophil recruitment, decreases in liver ischemic lobes compared to the control group in the experiment. Further analysis of neutrophils isolated from rats with liver damage treated with MSCs showed that p38 MAPK phosphorylation in these neutrophils was the main cause of decreased CXCR2 expression at the cell surface. Interestingly, inhibition of the MAPK p38 phosphorylating enzyme reduces the therapeutic potential of MSCs (119). In addition, since liver macrophages are the main sources of CXCL2-producing and neutrophil recruiting (94), treatment with MSCs by inhibiting NF-κB p65 phosphorylation has been shown to reduce the expression and production of CXCL2 by these cells. Therefore, in general, the use of MSCs reduces the migration of neutrophils to the liver and limits liver damage by reducing the expression of CXCR2 on the surface of neutrophils and reducing the CXCL2 expression and production by liver macrophages. As a result of MSCs therapy, the concentration of liver enzymes in plasma reduces (119). Also, a reduction in hepatocyte apoptosis, improvement in liver function, and a pathological improvement in liver tissue are seen.
4.1.4 Suppression of Activation and Migration of CD4+ T Cells
In general, the use of MSCs to treat liver disease has been shown to reduce the activation and chemotaxis of TCD4+ cells (121). Examination of the expression of different markers of TCD4+ cells isolated from liver injury mice treated with MSCs shows that the expression of CCR7, CXCR3 and CCR5 chemokine receptors is significantly reduced. Also, the production of CXCL9, CCL3, CXCL10, and CCL21 chemokines in the mice’s damaged liver is reduced in the treatment with MSCs. In addition, CD69 and CD44 expression are reduced in MSCs treated mice, thereby suppressing TCD4 + cell activation. Serum concentrations of IFN-γ and TNF-α cytokines in these mice also indicate suppression of CD4 + T cell differentiation into Th1 cells (109, 122).
4.1.5 Impact on the Th17/Treg Axis
Due to the importance of the proven role of IL-17 in liver disease (123), the study of MSCs’ effects on the IL-17 producing cells is of particular significance. As shown in previous studies, the imbalance in Treg/TH17 is associated with many liver diseases, including autoimmune hepatitis, alcoholic liver disease, and chronic hepatitis B (124).
A study by Qi-Hong Chen et al. Shows that the co-culture of MSCs with LPS-stimulated CD4 + T cell populations can induce plasticity in completely differentiated Th17 cells and convert them into functional Treg cells. The use of an anti-HGF antibody to eliminate and inhibit the effects of this factor leads to the inhibition of the modulatory effects of MSCs by regulating the Th17/Treg equilibrium (125).
In a study by Neda Milosavljevic et al., The effect of transplanted MSCs on Th17 cell responses in liver injury was investigated. IL-17 produced by Th17 cells by activating HSCs increases their production of a-SMA, type 1 collagen and TGF-b1. Intravascular injection of BM-MSCs in the calcium tetrachloride(CCL4)-induced fibrotic liver C57BL/6 mice showed a decrease in the number of IL-17 producing Th17 cells and IL-17 serum level, and also an increase in the serum level immune system suppressory factors such as IL-10, IDO and quinornine. Evaluation of liver tissue damage by H&E staining (to evaluate hepatocyte damage and lobular centre congestion with inflammatory cell infiltration) and Sirius red staining (to assess collagen deposition) in hepatic tissue of MSCs treated mice compared with CCL4-induced mice showed a significant reduction in the size of the stained area in dense fibrous tissue (126).
4.1.6 Impact on the NKT17/NKTreg Axis
In addition to Th17 cells, NKT cells also play a role in the production of IL-17 involved in the pathogenesis of the liver disease (127). Intracellular staining of mononuclear cells (MNCs) isolated from damaged liver indicates that most of the IL17-producing cells in liver damage are NKT cells. A study by Neda Milosavljevic et al. showed that intravenous injection of MSCs into liver induced injuries with CCL4 and (a-GalCer) a-galactoceramide in C57BL/6 mice decreases inflammatory NKT cell function. The study results show that serum IL-17 levels are reduced in disease-induced mice treated with MSCs. Further studies show that in mice treated with MSCs compared to disease-induced mice, there was a significant reduction in IL-17-producing NKT CD49b+ CD31+ (NKT17) cells (128).
Interestingly, the application of MSCs to treat liver disease in mice treated with a-GalCer1MSC is associated with a significantly higher number of regulatory NKT FOXP3+ IL10+ (NKTreg) cells (128). Therefore, the results of this study suggest that MSCs help improve liver treatment by modulating the NKT17/NKTreg axis and reducing the harmful inflammatory responses (128). It is noteworthy that the co-culture of hepatic NKT cells in vitro also confirms the results obtained from the In Vivo studies. This function of MSCs is related to IDO and iNOS because of the presence of methyl‐DL‐tryptophan-1 (IDO inhibitor) and L‐NG‐monomethyl arginine citrate (iNOS inhibitor) in the cells and supernatant of injected MSCs imped the protective effect of MSCs on mice. As a result, MSC protects against acute liver damage by reducing the cytotoxicity and capacity of liver NKT17 cells to produce inflammatory cytokines in an IDO-dependent manner (128) (Figure 3).
4.2 The Role of MSCs in Liver Tissue Repair and Regeneration
Mesenchymal stem cells help repair liver tissue through various mechanisms, and there is a very complex relationship to their therapeutic function, which is discussed in more detail below.
4.2.1 Reduction of Fibrosis and Impact on the Function of HSCs
MSCs co-culture with HSCs promotes their apoptosis and reduce the production of ECM components such as collagen from these cells. Reducing the synthesis of ECM components is very important in preventing fibrosis progression and can play an essential role in improving the disease (87, 129). In addition, the indirect culture of MSCs with HSC-related LX2 cell lines has been shown to reduce the proliferation of these cells by producing and secreting inflammatory factors such as IL-6, IL-8, and HGF (130). The results of recent studies show that MSCs suppress the activation of HSCs by producing TNFα-stimulated gene-6 (TSG6) and increase the expression of some stem cell markers in these cells (131). In vitro studies show that HSCs-derived stem cell-like cells can form organoids that help liver tissue regeneration.
4.2.2 Impact on LSECs
Co-culture of MSCs with endothelial progenitor cells to investigate the effects of these cells on hepatic sinusoidal epithelial cells has been shown to increase endothelial precursor cell proliferation and angiogenic capacity by affecting PDGF and Notch-associated receptors and their downstream signaling pathways (132).
Injection of MSCs into concanavalin-A (Con-A) induced liver disease mice improves liver disease by suppressing apoptosis in LSECs and hepatocytes and lowering serum transaminase enzyme levels (133).
4.2.3 Effect on Hepatocytes
The ability of MSC to differentiate into hepatocyte-like cells has been demonstrated in the in vivo and in vitro studies. This ability compensates for hepatocyte reduction through apoptosis during various liver damage. Experimental results show that MSCs can differentiate into hepatocyte-like cells (HLCs) in the presence of specific growth factors such as EGF, OSM, HGF and FGF (134, 135). Also, mimicry of the hepatic fibrosis microenvironment stimulates the differentiation of MSCs into hepatocyte-like cells by using 50 g/l of fibrotic tissue extract of rat liver at a faster rate than growth factors (136).
After differentiation, MSCs exhibit the morphology and function of hepatocytes. Analysis of differentiated cells after human Wharton’s jelly-derived MSCs (hWJ-MSCs) transplantation in rats shows the presence of cells in rat liver with the expression of human hepatocyte markers such as alpha photoprotein (AFP), CK18, CK19 and albumin. These newly differentiated cells have no sign of rat hepatocyte markers, indicating that hWJ-MSCs differentiate into hepatocyte-like cells after migration to the liver (137, 138). Also, the results of a study conducted by Jae Yeon Kim show that injection of MSCs and their supernatant into (D-galactosamine (D-gal) mediated liver damage) rats reduces hepatocytes apoptosis, and a threefold increase occurs in their proliferation. MSCs appear to increase hepatocyte proliferation by activating the IL-6 signaling pathway mediated by rno-miR-21-5p (139).
Therefore, in general, it can be said that the use of MSCs induces the repair and regeneration of liver tissue through immune responses modulation, differentiation into HLCs, increased proliferation and decreased apoptosis in hepatocytes, increased apoptosis and reduced function of HSCs and improve the function of LSECs.
5 Clinical Applications of MSCs for the Treatment of Liver Disease
5.1 Cell Source, Injection Method and Injection Dose
According to our search in the National Institutes of Health (NIH), there are currently 61 active clinical trials using MSCs to treat various liver diseases, including Cirrhosis, fibrosis, acute-on-chronic Liver Failure, and hepatitis viruses related liver failure. Table 3 summarizes some of these studies. MSCs in these studies were isolated from various tissues, including umbilical cord (UC), bone marrow (BM) and adipose tissue (AD). According to the results of multiple studies on the function of MSCs derived from different tissues, UC-MSCs are used in clinical applications more than others due to features such as (1) providing significant amounts of MSCs compared to BM (2) non-invasive isolation method (3) higher self-renewability and differentiation capacity of UC-MSCs in comparison with BM-MSCs and (4) lower immunogenicity (140, 141).
Injection methods such as intravenous (portal vein or peripheral), intrahepatic artery and intrasplenic injection are used in the transplantation of MSCs. The intravenous (IV) peripheral injection is more used in studies due to ease of identification and less invasive method. However, animal studies have shown that approximately 60% of IV-injected cells never reach the liver and accumulate in tissues like the lungs and kidneys. Due to the existence of different injection methods, in a study conducted by MEM Amer et al., It was shown that injection of MSCs through the portal vein shows better therapeutic results in patients compared to intrasplenic injection (142). As shown in Table 3, the number of injections doses, the time interval between injections, and the number of injection cells in the studies vary depending on the different groups’ set-up protocols.
5.2 Mechanisms Involved in Liver Disease Treatment After MSCs Transplantation
A phase 1 clinical trial conducted by Kharaziha et al. Showed that the use of BM-MSCs improved patients’ symptoms of uncompensated Cirrhosis. Various scores related to liver healing indicate improvement of the disease without side effects (143). Also, the results of phase 2 of this study, which was performed on patients with alcoholic cirrhosis, showed a reduction in tissue fibrosis and improved liver histology in patients after mesenchymal stem cell transplantation through the hepatic artery (143, 144). Due to the importance of safety in cell therapy studies, a study conducted by Alimoghaddam K et al. has examined this issue in the transplantation of MSCs in various liver diseases, which shows the improvement of liver function in patients without any side effects (145).
A study by Ani Sun et al. Showed that patients receiving routine treatment (RT) in combination with autologous BM-MSCs transplantation had better clinical symptoms such as decreased fatigue and ascites, increased appetite, and abdominal distension than patients receiving only routine treatment. This study also showed that liver function significantly improved in patients receiving combination therapy in terms of MELD (model for end-stage liver disease) and Child-Pugh scores, serum albumin, total bilirubin, aspartate aminotransferase(AST), alanine aminotransferase(ALT), and coagulation function (146).
As mentioned in the in vitro studies and animal models of liver disease, the application of MSCs helps improve liver function with different mechanisms in humans. A study conducted by Lanman Xu in 2014 showed that BM-MSCs improve the symptoms of patients with HBV associated Cirrhosis. The findings in this study showed that the number and ratio of Treg cells in PBMCs isolated from patients increased; however, the number of Th17 cells in patients treated with BM-MSCs decreased. Also, extraction of total RNA and evaluation of the expression of Foxp3 and RORγt transcription factors by Real-time polymerase chain reaction (RT-PCR) showed an increase in Foxp3 expression and a decrease in RORγt expression compared to the control group. Also, examination of patients’ serum in the first week after transplantation showed an increase in TGF-B and a decrease in inflammatory cytokines such as IL1-B, IL-6 and TNF-a. Therefore, in general, transplantation of MSCs by modulating the Treg/Th17 axis and modulating the production of inflammatory cytokines helps improve liver inflammation and patient conditions (147).
The results of a study conducted in 2020 by Federica Casiraghi et al. Show that intravenous injection of MSCs before liver transplantation does not improve the parameters of chance tissue compared to the group that received this treatment. However, a one-year follow-up of patients shows a slight increase in the circulating Treg/memory Treg and tolerant NK subset (CD56bright NK cells) over baseline (not significant) in MSC-treated compared to the control group (148).
As shown in Table 2, the disadvantages of using MSCs, encourage researchers to find safer and more efficient methods. As has been proven in many studies, MSCs perform their therapeutic functions after transplantation in two ways: cell-to-cell communication and secretion of soluble factors (paracrine effect). EVs are one of the main factors in this type (149).
6 MSCs-Derived EVs Application in Liver Disease Treatment
EVs are a significant component of intercellular communication that affect the actions of the target cell by transporting various substances, including proteins, lipids, and nucleic acids, from the producing cell to the target cell (150). These vesicles are divided into microvesicles (MVs), apoptotic bodies and exosomes according to their size, internal contents, biogenesis and different surface markers (151). However, the fourth group of these vesicles, smaller than the rest, has been identified as named exomer (152). Each of these vesicles has different contents, typically containing various proteins (including enzymes, cytokines, growth factors and heat shock proteins), different types of nucleic acids (including DNA, microRNA and lncRNAs) and different types of lipids (153). These molecules change target cell function after transferring by EVs bilipid membrane. As a consequence, and depending on the cell type and its physiological conditions, exosomes can lead to differentiation, increase proliferation and survival, or lead to increased apoptosis and decreased cell activity (154).
Many studies have examined the therapeutic effects of MSC derived EVs (MSC-EVs) in different diseases such as pulmonary fibrosis, osteoporosis, skin diseases, cardiovascular diseases, and various liver-related diseases. The results of new studies show that the application of MSCs-EVs has similar therapeutic effects to MSCs (155–157). In fact, the use of EVs is cell-free cell therapy because it preserves the benefits associated with cell therapy and bypasses its disadvantages. These benefits include: (1) lack of immunogenicity, (2) the ability to store them easily, (3) drug loading and increase their efficiency as a drug delivery system and (4) application as a ready-to-use drug (cryopreservation of EVs) (158).
Given that the results of many in vitro and animal model studies have shown that MSCs can migrate to TME, it is thought to be they can be used as carriers for tumor-targeted therapies (159). However, there is little clinical evidence of MSCs recruitment in hepatocellular carcinoma (160). Also, due to the proliferative and differentiating characteristics of MSCs, considering the possibility of malignant transformation and promotion of tumor progression by these cells, most studies are still in the preclinical stage (159). Therefore, to use the therapeutic properties of these cells, researchers used MSC-EVs that do not have these deficiencies (161). Recent studies show that adipose tissue MSCs derived exosomes (MSC-Ex) can increase the chemical sensitivity of liver cancer cells by affecting the mTOR related signaling pathway and leading to increased expression of chemo-sensitive related genes in cancer cells (162). Further studies have shown that these exosomes perform this function by transferring miR-199a to cancer cells (162). MiR-222-3p in BM-MSC-Ex has also been shown to inhibit cancer cell proliferation and increase their apoptosis (163). Another study showed that miR-302a, carried by UC-MSC-Ex, suppressed cyclin D1 as well as the AKT signaling pathway and thereby suppress tumor progression (164). Also, various anti-tumour drugs can be loaded into exosomes and used as targeted therapy (165). In addition, it has been shown that exosomes can be engineered to migrate to the target site, integrate with cancer cells, and deliver anti-tumor drugs to them (166, 167). However, the application of MSC-Ex is still in its initiation, and further studies are needed for their optimal use.
As mentioned, due to the size of MSCs, when injected intravenously, they accumulate in the lungs while EVs are much smaller and can migrate to the site of injury (168). MSC-EVs exert their therapeutic functions through 3 mechanisms that have been studied in vitro, pre-clinical, and clinical trials. These mechanisms include (1) proliferation induction/apoptosis suppression, (2) modulation of immune system responses, (3) reduction of fibrosis. Table 4 summarizes several new studies on the application of MSC-EVs (Figure 4).
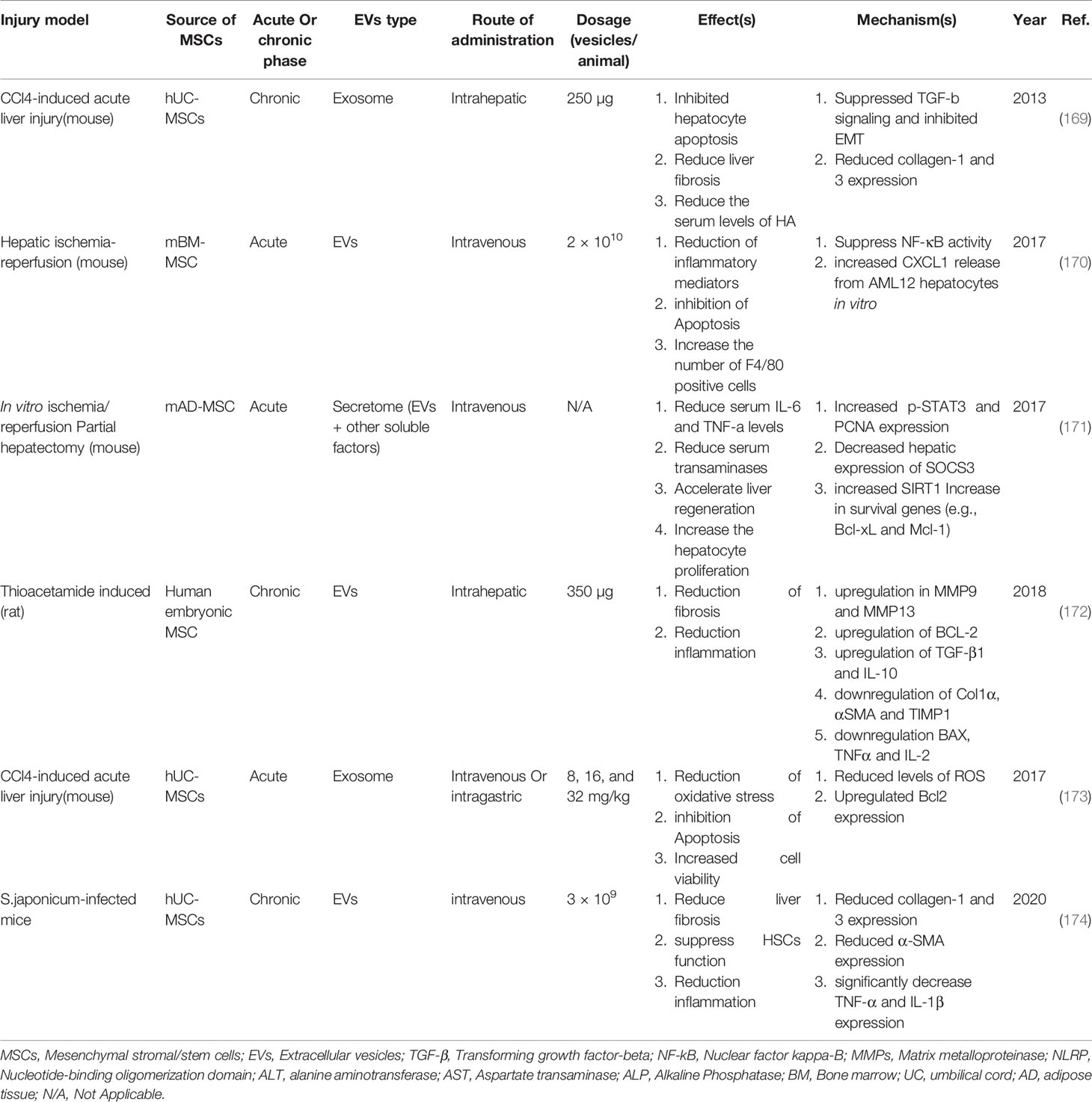
Table 4 Example of studies in the field of MSC-EV application in experimental models of liver injury and their therapeutic mechanisms.
6.1 Stimulation of Proliferation/Suppression of Apoptosis in Hepatocytes
Examination of the effects of MSCs-derived exosomes in vivo shows that these vesicles suppress acetaminophen and H2O2-induced apoptosis in hepatocytes by positively regulating Bcl-xl expression (175). Also, the study shows that MSC-Ex stimulates hepatocytes proliferation in CCL4-induced liver damage in mice. Further studies show that the exosomes positively regulate the priming phase genes, which is subsequently increases the expression of Proliferating cell nuclear antigen (PCNA) and cyclin D1 in the treated group compared to the control group (175).
The results of new studies show that the use of BM-MSCs-Ex reduces apoptosis by increasing autophagy in hepatocytes. A study by Shuxian Zhao et al. Shows that the use of BM-MSCs-Ex reduces D-GaIN/LPS-induced apoptosis in rats hepatocytes. Further studies show that autophagy-related markers such as LC3 and Beclin-1 are increased and have led to autophagosomes formation by hepatocytes. Also, the expression level of apoptosis-related proteins such as Bax and cleaved caspase 3 was decreased, and the expression level of Bcl-2 (anti-apoptotic protein) was increased. Because the use of 3-Methyladenine (3-MA), an autophagy inhibitor, limited the therapeutic effects of BM-MSCs-Ex, this study attributed the main therapeutic mechanism of these vesicles to the regulation of apoptosis in an autophagy-dependent manner (176).
A study conducted by Yinpeng Jin et al. In 2018 shows that the use of AD-MSCs-EVs in the model of acute liver failure in rats increases the survival rate by more than 70% compared to the control group. Liver sequencing of rats treated with AD-MSCs-EVs demonstrated an increase in a non-coding long-stranded RNA (lncRNA) called lncRNA H19 (H19), and when the coding sequence of H19 in AD-MSCs of EVs source was knocked off, survival rates could be 40% higher than the control group. In fact, H19 encoding gene silencing reduces the survival of rats compared to the AD-MSCs-EVs treated group. The results of hepatocytes Co-culture with normal AD-MSCs-EVs and manipulated AD-MSCs-EVs (lacking H19) indicate that this lncRNA increases the proliferation of hepatocytes by the HGF/c-Met signaling pathway as well as reducing apoptosis (177).
6.2 Immunomodulatory Properties
The results of a study by H Haga et al. show that administration of BM-MSC-derived EVs to mice with liver injury induced by Intraperitoneal (IP) injection of TNF-a and D-gal increases the migration of protective macrophages to damaged liver tissue. Another study showed that injections of MSC-EVs into a mouse model of ischemic-reperfusion injury helped reduce inflammatory responses by modulating the expression of NLRP12 and CXCL1. This study also showed that the frequency of F4/80+ cells (expressed in protective macrophages) in the damaged liver increased significantly after MSCs-EVs injection (178).
Con-A-induced liver injury studies in C57/B6 male mice show extensive modulation of the immune system by AD-MSCs-Ex in the treatment group compared to the control group. The analyses showed that the expression of IL-2 (a pro-inflammatory cytokine) was associated with a significant decrease. On the other hand, the expression of cytokine TGF-β and HGF was upregulated. Also, the percentage of Treg cells among non-parenchymal liver cells (NPCs) in the three doses of AD-MSCs-Ex treated group increased significantly. Treg has been reported to be required to induce immune tolerance in this liver injury model (179). This study also compared the therapeutic effects of AD-MSC injection and one-dose injection of AD-MSCs-Ex with 3-dose injection. The present results of the study show a significant difference in the therapeutic application 3-dose injection of AD-MSCs-Ex with other groups. Histological and serum analysis also confirmed a decrease in fibrotic and necrotic areas of the liver and a decrease in ALT levels in the treated groups (177).
Using the protein chip method by Xiaoli Rong et al. showed that in treatment of D-GaIN/LPS induced damages in rats with AD-MSCs-EVs, the expression of inflammatory cytokines including IL-6, IL-1α, IL-1β, IL-1ra and IL-17 in was significantly lower than in the Phosphate-buffered saline (PBS) treatment group. Also, the level of inflammatory chemokines such as CXCL7, CXCL9, CXCL10, CCL20, CX3CL1, CINC-3, CINC-2α/β, CINC-1, CNTF, and LECAM-1 decreases in the AD-MSCs-Ev treated group compared to the control group PBS treated group. Simultaneous reduction of inflammatory cytokines and chemokines ultimately leads to a reduction in liver tissue necrosis and an increase in the survival rate of rats (180). Evaluation of inflammatory cytokines including TNF-α, IL-1, IL-2, IL-6, IL-8, and IL-10 and in liver tissue using RT-PCR showed a significant reduction in rats treated by hBM-MSCs and hBM-MSCs-Ex. However, the results of this study showed that the expression of IL-6 and IL-1 in the hBM-MSCs-Ex treatment group was significantly lower than in the hBM-MSCs treated group (181).
Also, the study of malondialdehyde (MDA) levels, which is the end product of membrane lipid peroxidation, can be used as a marker for oxidative stress and liver cell damage. Analysis in hBM-MSCs-Ex treated rats (CCL4-induced liver injury) showed a significant reduction in MDA level (181).
6.3 Reduce Hepatic Fibrosis
The results of a study conducted in 2019 (181) show that the administration of hBM-MSCs-Ex to rats with CCL4-induced liver damage effectively reduces liver fibrosis. Visual examination showed that the liver of mice treated with hBM-MSCs-Ex had a smooth, uniform surface and softer tissue than the control group. Sirus red and H&E staining show reduction of fibrous areas and collagen deposition in the treated liver. In addition, comparing the therapeutic effects of hBM-MSCs-Exo and hBM-MSCs indicates the better therapeutic outcome in the application of hBM-MSCs-Ex due to the collagen deposition level and Ishak fibrosis score. Examination of the hydroxyproline levels, unnecessary amino acid and a major component of collagen also shows a decrease in this amino acid in the treated group and indicates a reduction in collagen deposition. In this study, the biochemical analysis showed that the level of AST, ALT, ALP, total bilirubin (TBIL) and gamma-glutamyl transferase (γ-GT) decreases, and serum total protein (TP) levels increases in serum. Examination of Wnt/β-catenin signaling related factors including PPARγ, Wnt10b, Wnt3a, β-catenin, and WISP1 in both in vivo and in vitro conditions indicates the use of hBM- MSCs and hBM-MSCs-Ex (have better therapeutic results) reduce the expression of these factors in HSCs (in vitro) and with rat fibrous liver. Due to the reduction of collagen deposition and fibrotic tissue in the damaged liver, the results of this study suggest using hBM-MSCs-Ex leads to decreased HSCs function and reduced fibrosis by inhibiting the Wnt/β-catenin signaling pathway (181).
7 Conclusions and Future Prospects
Due to the urgent need to treat tissue degenerative diseases, methods that can accelerate tissue repair are of great value. Using MSCs as multipotent stem cells is one of these widely used methods due to its properties. As mentioned, these cells uses different mechanisms such as differentiation into hepatocyte-like cells, reducing apoptosis and increasing hepatocyte proliferation, reducing inflammation, suppressing tissue-damaging immune cells, producing various growth factors, suppressing the function of HSC, and improving the function of LSECs to improve liver diseases. The use of these cells not only prevents further damage to the liver tissue but also accelerates and increases the repair of liver tissue. MSCs migrate to the site of liver injury after intravenous injection due to their chemokine receptors and perform their therapeutic actions there. As mentioned earlier, due to the limitations of cell therapy, MSC-EVs are used today. Many studies have been conducted on the application of MSC-EVs in preclinical studies. The results of these studies indicate the potential ability of these vesicles to improve liver cell function and modulate the immune system (182). Some studies comparing the therapeutic effect of MSCs and MSC-EVs have shown that MSC-EVs have a higher therapeutic potential and better outcomes. Although the therapeutic effects of EVs on liver repair in this study are divided into three distinct mechanisms, these processes are interrelated and complement each other’s functions. In fact, the therapeutic potential of MSC-EVs likely lies in their ability to act simultaneously through multiple signaling pathways. Therefore, using these vesicles does not have cell therapy disadvantages. Due to its benefits, it can perform better therapeutic performance in liver injury animals models.
Although there are numerous clinical trials on the use of MSCs in the treatment of liver disease, to date, no clinical trials have been performed on the use of MSC-EVs despite their benefits. However, it has been used to treat many disorders, including orthopaedic disease (151), neurodegenerative disease (183), myocardial disease (184), renal disease, graft versus host disease (GvHD), pancreatic cancer and type 1 diabetes and has shown promising results (185). Despite the therapeutic potential of MSC-EVs and their beneficial effects in preclinical studies, several issues related to the clinical use of these vesicles remain unresolved. Exosomes as an EV circulate in the blood and transfer their cargos to the target cell through phagocytosis, receptor-mediated endocytosis, fusion, micropinocytosis (186). In the body, the half-life of EVs is estimated at a few minutes and are removed from the bloodstream within a few hours. Therefore, we need multiple injections to treat diseases. Due to this issue, producing EVs in an industrial scale and repeatable method is significant. It is also important to note that despite effective and proven results in animal models, the improvement of these models is usually incomplete. Therefore, more research is needed to investigate the long-term effects of MSC-EVs.
Author Contributions
AH: Conceptualization, Investigation, Writing - original draft, Writing - review and editing, Validation. KM: Writing - review and editing, Validation. SS: Edited final version of the manuscript. SH: Supervision and final approval of the manuscript. All authors contributed to the article and approved the submitted version.
Conflict of Interest
The authors declare that the research was conducted in the absence of any commercial or financial relationships that could be construed as a potential conflict of interest.
Publisher’s Note
All claims expressed in this article are solely those of the authors and do not necessarily represent those of their affiliated organizations, or those of the publisher, the editors and the reviewers. Any product that may be evaluated in this article, or claim that may be made by its manufacturer, is not guaranteed or endorsed by the publisher.
Abbreviations
AD, adipose tissue; AFP, alpha photoprotein; ALF, Acute liver failure; ALP, Alkaline Phosphatase; ALT, alanine aminotransferase; AST, Aspartate transaminase; BM, Bone marrow; CMV, Cytomegalovirus; Con-A, concanavalin-A; DCs, Dendritic cells; ECM, extracellular matrix; EVs, Extracellular vesicles; FLD, Fatty liver disease; HGF, hepatocyte growth factor; HLCs, hepatocyte-like cells; HMGB1, High mobility group box 1; HSCs, hepatic stellate cells; IDO, indolamine 2,3-dioxygenase; IV, intravenous(; KCs, Kupffer cells; KLF2, Krüppel factor 2 transcription factor; LDs, Liver disease; LPS, Lipopolysaccharide; LSECs, hepatic sinusoidal endothelial cells; MMPs, Matrix metalloproteinase; MSCs, Mesenchymal stromal/stem cells; NF-kB, Nuclear factor kappa-B; NK, Natural killer cell; NLRP, Nucleotide-binding oligomerization domain; ROS, Reactive oxygen species; TGF-β, Transforming growth factor-beta; TLR, Toll-like receptor; TSG6, TNFα-stimulated gene-6; Tumor necrosis factor, TNF; TXA2, Thromboxane A2; UC, umbilical cord; WJ, Wharton’s jelly.
References
1. Ertek S, Cicero A. Impact of Physical Activity on Inflammation: Effects on Cardiovascular Disease Risk and Other Inflammatory Conditions. Arch Med Sci: AMS (2012) 8(5):794. doi: 10.5114/aoms.2012.31614
2. Kiecolt-Glaser JK, Gouin J-P, Hantsoo L. Close Relationships, Inflammation, and Health. Neurosci Biobehav Rev (2010) 35(1):33–8. doi: 10.1016/j.neubiorev.2009.09.003
3. Verhamme P, Hoylaerts MF. Hemostasis and Inflammation: Two of a Kind? Thromb J (2009) 7(1):1–3. doi: 10.1186/1477-9560-7-15
4. Starlinger P, Luyendyk JP, Groeneveld DJ eds. Hemostasis and Liver Regeneration. In: Seminars in Thrombosis and Hemostasis. Thieme Medical Publishers.
5. Ang CH, Hsu SH, Guo F, Tan CT, Victor CY, Visvader JE, et al. Lgr5+ Pericentral Hepatocytes Are Self-Maintained in Normal Liver Regeneration and Susceptible to Hepatocarcinogenesis. Proc Natl Acad Sci (2019) 116(39):19530–40. doi: 10.1073/pnas.1908099116
6. Fausto N, Campbell JS, Riehle KJ. Liver Regeneration. J Hepatol (2012) 57(3):692–4. doi: 10.1016/j.jhep.2012.04.016
7. Laleman W, Claria J, van der Merwe S, Moreau R, Trebicka J. Systemic Inflammation and Acute-on-Chronic Liver Failure: Too Much, Not Enough. Can J Gastroenterol Hepatol (2018) 2018:1027152. doi: 10.1155/2018/1027152
8. Stravitz RT, Lee WM. Acute Liver Failure. Lancet (2019) 394(10201):869–81. doi: 10.1016/S0140-6736(19)31894-X
9. Anderson SH, Richardson P, Wendon J, Pagliuca A, Portmann B. Acute Liver Failure as the Initial Manifestation of Acute Leukaemia. Liver (2001) 21(4):287–92. doi: 10.1034/j.1600-0676.2001.021004287.x
10. Shakil AO, Kramer D, Mazariegos GV, Fung JJ, Rakela J. Acute Liver Failure: Clinical Features, Outcomeanalysis, and Applicability of Prognostic Criteria. Liver Transplant (2000) 6(2):163–9. doi: 10.1016/S1527-6465(00)80005-2
11. Jalan R. Acute Liver Failure: Current Management and Future Prospects. J Hepatol (2005) 42(1):S115–S23. doi: 10.1016/j.jhep.2004.11.010
12. Kanjo A, Ocskay K, Gede N, Kiss S, Szakács Z, Párniczky A, et al. Efficacy and Safety of Liver Support Devices in Acute and Hyperacute Liver Failure: A Systematic Review and Network Meta-Analysis. Sci Rep (2021) 11(1):1–10. doi: 10.1038/s41598-021-83292-z
13. Bernal W, Wendon J. Acute Liver Failure. N Engl J Med (2013) 369(26):2525–34. doi: 10.1056/NEJMra1208937
14. Bataller R, Brenner DA. Liver Fibrosis. J Clin Invest (2005) 115(2):209–18. doi: 10.1172/JCI24282
15. Tripodi A, Mannucci PM. The Coagulopathy of Chronic Liver Disease. N Engl J Med (2011) 365(2):147–56. doi: 10.1056/NEJMra1011170
16. Tsukada S, Parsons CJ, Rippe RA. Mechanisms of Liver Fibrosis. Clin Chim Acta (2006) 364(1-2):33–60. doi: 10.1016/j.cca.2005.06.014
17. Skrypnyk NI, Harris RC, de Caestecker MP. Ischemia-Reperfusion Model of Acute Kidney Injury and Post Injury Fibrosis in Mice. J Vis Exp: JoVE (2013) 78:e50495. doi: 10.3791/50495
18. Vasarmidi E, Tsitoura E, Spandidos DA, Tzanakis N, Antoniou KM. Pulmonary Fibrosis in the Aftermath of the COVID-19 Era. Exp Ther Med (2020) 20(3):2557–60. doi: 10.3892/etm.2020.8980
19. Renk H, Regamey N, Hartl D. Influenza A (H1N1) Pdm09 and Cystic Fibrosis Lung Disease: A Systematic Meta-Analysis. PloS One (2014) 9(1):e78583. doi: 10.1371/journal.pone.0078583
20. Sadeghi S, Soudi S, Shafiee A, Hashemi SM. Mesenchymal Stem Cell Therapies for COVID-19: Current Status and Mechanism of Action. Life Sci (2020) 262:118493. doi: 10.1016/j.lfs.2020.118493
21. Khosrojerdi A, Soudi S, Hosseini AZ, Khaligh SG, Hashemi SM. Imipenem Alters Systemic and Liver Inflammatory Responses in CLP-Induced Sepsis Mice in a Dose-Dependent Manner. Int Immunopharmacol (2021) 93:107421. doi: 10.1016/j.intimp.2021.107421
22. Yang X, Meng Y, Han Z, Ye F, Wei L, Zong C. Mesenchymal Stem Cell Therapy for Liver Disease: Full of Chances and Challenges. Cell Biosci (2020) 10(1):1–18. doi: 10.1186/s13578-020-00480-6
23. Lee SM, Lee SD, Wang SZ, Sarkar D, Lee HM, Khan A, et al. Effect of Mesenchymal Stem Cell in Liver Regeneration and Clinical Applications. Hepatoma Res (2021) 7:53. doi: 10.20517/2394-5079.2021.07
24. Kaushal S, Amiel GE, Guleserian KJ, Shapira OM, Perry T, Sutherland FW, et al. Functional Small-Diameter Neovessels Created Using Endothelial Progenitor Cells Expanded Ex Vivo. Nat Med (2001) 7(9):1035–40. doi: 10.1038/nm0901-1035
25. Asahara T, Murohara T, Sullivan A, Silver M, van der Zee R, Li T, et al. Isolation of Putative Progenitor Endothelial Cells for Angiogenesis. Science (1997) 275(5302):964–6. doi: 10.1126/science.275.5302.964
26. Martinez-Agosto JA, Mikkola HK, Hartenstein V, Banerjee U. The Hematopoietic Stem Cell and Its Niche: A Comparative View. Genes Dev (2007) 21(23):3044–60. doi: 10.1101/gad.1602607
27. Panteleev A, Vorob'ev I. Expression of Early Hematopoietic Markers in Cord Blood and Mobilized Blood. Tsitologiia (2012) 54(10):774–82. doi: 10.21037/atm.2020.03.218
28. Lázaro CA, Rhim JA, Yamada Y, Fausto N. Generation of Hepatocytes From Oval Cell Precursors in Culture. Cancer Res (1998) 58(23):5514–22.
29. Zaret KS, Grompe M. Generation and Regeneration of Cells of the Liver and Pancreas. Science (2008) 322(5907):1490–4. doi: 10.1126/science.1161431
30. Weber A, Touboul T, Mainot S, Branger J, Mahieu-Caputo D. Human Foetal Hepatocytes: Isolation, Characterization, and Transplantation. Hepatocytes: Springer; (2010) p:41–55. doi: 10.1007/978-1-60761-688-7_2
31. Forbes SJ, Gupta S, Dhawan A. Cell Therapy for Liver Disease: From Liver Transplantation to Cell Factory. J Hepatol (2015) 62(1):S157–S69. doi: 10.1016/j.jhep.2015.02.040
32. Zhou H, Liu H, Ezzelarab M, Schmelzer E, Wang Y, Gerlach J, et al. Experimental Hepatocyte Xenotransplantation—A Comprehensive Review of the Literature. Xenotransplantation (2015) 22(4):239–48. doi: 10.1111/xen.12170
33. Mizukoshi E, Kaneko S. Immune Cell Therapy for Hepatocellular Carcinoma. J Hematol Oncol (2019) 12(1):1–11. doi: 10.1186/s13045-019-0742-5
34. Medvedev S, Shevchenko A, Zakian S. Induced Pluripotent Stem Cells: Problems and Advantages When Applying Them in Regenerative Medicine. Acta Naturae (англоязычная версия) (2010) 2(2):18–28.
35. Yu Y, Fisher JE, Lillegard JB, Rodysill B, Amiot B, Nyberg SL. Cell Therapies for Liver Diseases. Liver Transplant (2012) 18(1):9–21. doi: 10.1002/lt.22467
36. Satija NK, Singh VK, Verma YK, Gupta P, Sharma S, Afrin F, et al. Mesenchymal Stem Cell-Based Therapy: A New Paradigm in Regenerative Medicine. J Cell Mol Med (2009) 13(11-12):4385–402. doi: 10.1111/j.1582-4934.2009.00857.x
37. Huang L, Zhang C, Gu J, Wu W, Shen Z, Zhou X, et al. A Randomized, Placebo-Controlled Trial of Human Umbilical Cord Blood Mesenchymal Stem Cell Infusion for Children With Cerebral Palsy. Cell Transplant (2018) 27(2):325–34. doi: 10.1177/0963689717729379
38. Hu C, Zhao L, Zhang L, Bao Q, Li L. Mesenchymal Stem Cell-Based Cell-Free Strategies: Safe and Effective Treatments for Liver Injury. Stem Cell Res Ther (2020) 11(1):1–12. doi: 10.1186/s13287-020-01895-1
39. Kang SH, Kim MY, Eom YW, Baik SK. Mesenchymal Stem Cells for the Treatment of Liver Disease: Present and Perspectives. Gut Liver (2020) 14(3):306. doi: 10.5009/gnl18412
40. Bernardo ME, Cometa AM, Pagliara D, Vinti L, Rossi F, Cristantielli R, et al. Ex Vivo Expansion of Mesenchymal Stromal Cells. Best Pract Res Clin Haematol (2011) 24(1):73–81. doi: 10.1016/j.beha.2010.11.002
42. Wu Z, Han M, Chen T, Yan W, Ning Q. Acute Liver Failure: Mechanisms of Immune-Mediated Liver Injury. Liver Int (2010) 30(6):782–94. doi: 10.1111/j.1478-3231.2010.02262.x
44. Bernal W, Auzinger G, Dhawan A, Wendon J. Acute Liver Failure. Lancet (2010) 376(9736):190–201. doi: 10.1016/S0140-6736(10)60274-7
45. Rutherford A, Chung RT eds. Acute Liver Failure: Mechanisms of Hepatocyte Injury and Regeneration. In: Seminars in Liver Disease. Thieme Medical Publishers.
46. Ramadori G, Moriconi F, Malik I, Dudas J. Physiology and Pathophysiology of Liver Inflammation, Damage and Repair. J Physiol Pharmacol (2008) 59(Suppl 1):107–17.
47. Lazo M, Hernaez R, Bonekamp S, Kamel IR, Brancati FL, Guallar E, et al. Non-Alcoholic Fatty Liver Disease and Mortality Among US Adults: Prospective Cohort Study. BMJ (2011) 343:d6891. doi: 10.1136/bmj.d6891
48. Marjot T, Moolla A, Cobbold JF, Hodson L, Tomlinson JW. Nonalcoholic Fatty Liver Disease in Adults: Current Concepts in Etiology, Outcomes, and Management. Endocr Rev (2020) 41(1):66–117. doi: 10.1210/endrev/bnz009
49. Asrih M, Jornayvaz FR. Inflammation as a Potential Link Between Nonalcoholic Fatty Liver Disease and Insulin Resistance. J Endocrinol (2013) 218(3):R25–36. doi: 10.1530/JOE-13-0201
50. Lin R, Wu D, Wu F-J, Meng Y, Zhang J-H, Wang X-G, et al. Non-Alcoholic Fatty Liver Disease Induced by Perinatal Exposure to Bisphenol a Is Associated With Activated mTOR and TLR4/NF-κb Signaling Pathways in Offspring Rats. Front Endocrinol (2019) 10:620. doi: 10.3389/fendo.2019.00620
51. Arshad MI, Piquet-Pellorce C, Samson M. IL-33 and HMGB 1 Alarmins: Sensors of Cellular Death and Their Involvement in Liver Pathology. Liver Int (2012) 32(8):1200–10. doi: 10.1111/j.1478-3231.2012.02802.x
52. McDonald B, Pittman K, Menezes GB, Hirota SA, Slaba I, Waterhouse CC, et al. Intravascular Danger Signals Guide Neutrophils to Sites of Sterile Inflammation. Science (2010) 330(6002):362–6. doi: 10.1126/science.1195491
53. Mihm S. Danger-Associated Molecular Patterns (DAMPs): Molecular Triggers for Sterile Inflammation in the Liver. Int J Mol Sci (2018) 19(10):3104. doi: 10.3390/ijms19103104
54. Nguyen-Lefebvre AT, Horuzsko A. Kupffer Cell Metabolism and Function. J Enzymol Metab (2015) 1(1):101.
55. Kisseleva T, Brenner D. Molecular and Cellular Mechanisms of Liver Fibrosis and Its Regression. Nat Rev Gastroenterol Hepatol (2021) 18(3):151–66. doi: 10.1038/s41575-020-00372-7
56. He Y, Jin L, Wang J, Yan Z, Chen T, Zhao Y. Mechanisms of Fibrosis in Acute Liver Failure. Liver Int (2015) 35(7):1877–85. doi: 10.1111/liv.12731
57. Poynard T, Mathurin P, Lai C-L, Guyader D, Poupon R, Tainturier M-H, et al. A Comparison of Fibrosis Progression in Chronic Liver Diseases. J Hepatol (2003) 38(3):257–65. doi: 10.1016/S0168-8278(02)00413-0
58. Ramos-Tovar E, Muriel P. Molecular Mechanisms That Link Oxidative Stress, Inflammation, and Fibrosis in the Liver. Antioxidants (2020) 9(12):1279. doi: 10.3390/antiox9121279
59. Lemoinne S, Cadoret A, El Mourabit H, Thabut D, Housset C. Origins and Functions of Liver Myofibroblasts. Biochim Biophys Acta (BBA)-Mol Basis Dis (2013) 1832(7):948–54. doi: 10.1016/j.bbadis.2013.02.019
60. Lu D-H, Guo X-Y, Qin S-Y, Luo W, Huang X-L, Chen M, et al. Interleukin-22 Ameliorates Liver Fibrogenesis by Attenuating Hepatic Stellate Cell Activation and Downregulating the Levels of Inflammatory Cytokines. World J Gastroenterol: WJG (2015) 21(5):1531. doi: 10.3748/wjg.v21.i5.1531
61. Gandhi CR. Hepatic Stellate Cell Activation and Pro-Fibrogenic Signals. J Hepatol (2017) 67(5):1104–5. doi: 10.1016/j.jhep.2017.06.001
62. Tsuchida T, Friedman SL. Mechanisms of Hepatic Stellate Cell Activation. Nat Rev Gastroenterol Hepatol (2017) 14(7):397–411. doi: 10.1038/nrgastro.2017.38
63. Shi H, Dong L, Dang X, Liu Y, Jiang J, Wang Y, et al. Effect of Chlorogenic Acid on LPS-Induced Proinflammatory Signaling in Hepatic Stellate Cells. Inflamm Res (2013) 62(6):581–7. doi: 10.1007/s00011-013-0610-7
64. Pinzani M, Rosselli M, Zuckermann M. Liver Cirrhosis. Best Pract Res Clin Gastroenterol (2011) 25(2):281–90. doi: 10.1016/j.bpg.2011.02.009
65. He G, Karin M. NF-κb and STAT3–key Players in Liver Inflammation and Cancer. Cell Res (2011) 21(1):159–68. doi: 10.1038/cr.2010.183
66. Wu W-Y, Li J, Wu Z-S, Zhang C-L, Meng X-L. STAT3 Activation in Monocytes Accelerates Liver Cancer Progression. BMC Cancer (2011) 11(1):1–10. doi: 10.1186/1471-2407-11-506
67. Finkin S, Pikarsky E. NF-κb in Liver Cancer: The Plot Thickens. NF-kB Health Dis (2010) 349:185–96. doi: 10.1007/82_2010_104
68. Bishayee A. The Inflammation and Liver Cancer. Inflamm Cancer (2014) 816:401–35. doi: 10.1007/978-3-0348-0837-8_16
69. Ölander M, Wiśniewski JR, Artursson P. Cell-Type-Resolved Proteomic Analysis of the Human Liver. Liver Int (2020) 40(7):1770–80. doi: 10.1111/liv.14452
70. Knolle P, Löhr H, Treichel U, Dienes H, Lohse A, Schlaack J, et al. Parenchymal and Nonparenchymal Liver Cells and Their Interaction in the Local Immune Response. Z fur Gastroenterol (1995) 33(10):613–20.
71. Kimura T, Flynn CT, Whitton JL. Hepatocytes Engulf and Rapidly Silence Coxsackievirus, Protecting the Host Against Systemic Viral Pathology. Am Assoc Immnol (2020) 3:1–10. doi: 10.1038/s42003-020-01303-7
72. Zhou Z, Xu M-J, Gao B. Hepatocytes: A Key Cell Type for Innate Immunity. Cell Mol Immunol (2016) 13(3):301–15. doi: 10.1038/cmi.2015.97
73. Kubes P, Mehal WZ. Sterile Inflammation in the Liver. Gastroenterology (2012) 143(5):1158–72. doi: 10.1053/j.gastro.2012.09.008
74. Kushner I, Mackiewicz A. The Acute Phase Response: An Overview. Acute Phase Proteins (2020), 3–19. doi: 10.1201/9781003068587-2
75. Fazel Modares N, Polz R, Haghighi F, Lamertz L, Behnke K, Zhuang Y, et al. IL-6 Trans-Signaling Controls Liver Regeneration After Partial Hepatectomy. Hepatology (2019) 70(6):2075–91. doi: 10.1002/hep.30774
76. Crispe IN. Hepatocytes as Immunological Agents. J Immunol (2016) 196(1):17–21. doi: 10.4049/jimmunol.1501668
77. Kenna T, Golden-Mason L, Norris S, Hegarty JE, O'Farrelly C, Doherty DG. Distinct Subpopulations of γδ T Cells Are Present in Normal and Tumor-Bearing Human Liver. Clin Immunol (2004) 113(1):56–63. doi: 10.1016/j.clim.2004.05.003
78. Benseler V, Warren A, Vo M, Holz LE, Tay SS, Le Couteur DG, et al. Hepatocyte Entry Leads to Degradation of Autoreactive CD8 T Cells. Proc Natl Acad Sci (2011) 108(40):16735–40. doi: 10.1073/pnas.1112251108
79. Ebrahimkhani MR, Mohar I, Crispe IN. Cross-Presentation of Antigen by Diverse Subsets of Murine Liver Cells. Hepatology (2011) 54(4):1379–87. doi: 10.1002/hep.24508
80. Duarte N, Coelho IC, Patarrão RS, Almeida JI, Penha-Gonçalves C, Macedo MP. How Inflammation Impinges on NAFLD: A Role for Kupffer Cells. BioMed Res Int (2015) 2015:984578. doi: 10.1155/2015/984578
81. Ma Y-Y, Yang M-Q, He Z-G, Wei Q, Li J-Y. The Biological Function of Kupffer Cells in Liver Disease. Biol Myelomonocytic Cells (2017) 2. doi: 10.5772/67673
82. Li W, Deng M, Loughran PA, Yang M, Lin M, Yang C, et al. LPS Induces Active HMGB1 Release From Hepatocytes Into Exosomes Through the Coordinated Activities of TLR4 and Caspase-11/GSDMD Signaling. Front Immunol (2020) 11:229. doi: 10.3389/fimmu.2020.00229
83. Gaskell H, Ge X, Nieto N. High-Mobility Group Box-1 and Liver Disease. Hepatol Commun (2018) 2(9):1005–20. doi: 10.1002/hep4.1223
84. Reynaert H, Thompson M, Thomas T, Geerts A. Hepatic Stellate Cells: Role in Microcirculation and Pathophysiology of Portal Hypertension. Gut (2002) 50(4):571–81. doi: 10.1136/gut.50.4.571
85. Fujita T, Narumiya S. Roles of Hepatic Stellate Cells in Liver Inflammation: A New Perspective. Inflamm Regen (2016) 36(1):1–6. doi: 10.1186/s41232-016-0005-6
86. Fujita T, Soontrapa K, Ito Y, Iwaisako K, Moniaga CS, Asagiri M, et al. Hepatic Stellate Cells Relay Inflammation Signaling From Sinusoids to Parenchyma in Mouse Models of Immune-Mediated Hepatitis. Hepatology (2016) 63(4):1325–39. doi: 10.1002/hep.28112
87. Higashi T, Friedman SL, Hoshida Y. Hepatic Stellate Cells as Key Target in Liver Fibrosis. Adv Drug Deliv Rev (2017) 121:27–42. doi: 10.1016/j.addr.2017.05.007
88. Peralta C, Jiménez-Castro MB, Gracia-Sancho J. Hepatic Ischemia and Reperfusion Injury: Effects on the Liver Sinusoidal Milieu. J Hepatol (2013) 59(5):1094–106. doi: 10.1016/j.jhep.2013.06.017
89. Reifart J, Rentsch M, Mende K, Coletti R, Sobocan M, Thasler WE, et al. Modulating CD4+ T Cell Migration in the Postischemic Liver: Hepatic Stellate Cells as New Therapeutic Target? Transplantation (2015) 99(1):41–7. doi: 10.1097/TP.0000000000000461
90. Feng M, Wang Q, Wang H, Wang M, Guan W, Lu L. Adoptive Transfer of Hepatic Stellate Cells Ameliorates Liver Ischemia Reperfusion Injury Through Enriching Regulatory T Cells. Int Immunopharmacol (2014) 19(2):267–74. doi: 10.1016/j.intimp.2014.01.006
91. Heymann F, Hamesch K, Weiskirchen R, Tacke F. The Concanavalin A Model of Acute Hepatitis in Mice. Lab Anim (2015) 49(1_suppl):12–20. doi: 10.1177/0023677215572841
92. Mizuhara H, O'Neill E, Seki N, Ogawa T, Kusunoki C, Otsuka K, et al. T Cell Activation-Associated Hepatic Injury: Mediation by Tumor Necrosis Factors and Protection by Interleukin 6. J Exp Med (1994) 179(5):1529–37. doi: 10.1084/jem.179.5.1529
93. Stark K, Eckart A, Haidari S, Tirniceriu A, Lorenz M, von Brühl M-L, et al. Capillary and Arteriolar Pericytes Attract Innate Leukocytes Exiting Through Venules And'instruct'them With Pattern-Recognition and Motility Programs. Nat Immunol (2013) 14(1):41–51. doi: 10.1038/ni.2477
94. Qin C-C, Liu Y-N, Hu Y, Yang Y, Chen Z. Macrophage Inflammatory Protein-2 as Mediator of Inflammation in Acute Liver Injury. World J Gastroenterol (2017) 23(17):3043. doi: 10.3748/wjg.v23.i17.3043
95. Lim HK, Jeffrey GP, Ramm GA, Soekmadji C. Pathogenesis of Viral Hepatitis-Induced Chronic Liver Disease: Role of Extracellular Vesicles. Front Cell Infect Microbiol (2020) 10. doi: 10.3389/fcimb.2020.587628
96. Marrone G, Shah VH, Gracia-Sancho J. Sinusoidal Communication in Liver Fibrosis and Regeneration. J Hepatol (2016) 65(3):608–17. doi: 10.1016/j.jhep.2016.04.018
97. Gracia-Sancho J, Caparrós E, Fernández-Iglesias A, Francés R. Role of Liver Sinusoidal Endothelial Cells in Liver Diseases. Nat Rev Gastroenterol Hepatol (2021) 18(6):411–31. doi: 10.1038/s41575-020-00411-3
98. Xie G, Wang X, Wang L, Wang L, Atkinson RD, Kanel GC, et al. Role of Differentiation of Liver Sinusoidal Endothelial Cells in Progression and Regression of Hepatic Fibrosis in Rats. Gastroenterology (2012) 142(4):918–27. e6. doi: 10.1053/j.gastro.2011.12.017
99. Lisman T, Luyendyk JP eds. Platelets as Modulators of Liver Diseases. In: Seminars in Thrombosis and Hemostasis. Thieme Medical Publishers.
100. Benedicto A, Herrero A, Romayor I, Marquez J, Smedsrød B, Olaso E, et al. Liver Sinusoidal Endothelial Cell ICAM-1 Mediated Tumor/Endothelial Crosstalk Drives the Development of Liver Metastasis by Initiating Inflammatory and Angiogenic Responses. Sci Rep (2019) 9(1):1–12. doi: 10.1038/s41598-019-49473-7
101. Géraud C, Mogler C, Runge A, Evdokimov K, Lu S, Schledzewski K, et al. Endothelial Transdifferentiation in Hepatocellular Carcinoma: Loss of Stabilin-2 Expression in Peri-Tumourous Liver Correlates With Increased Survival. Liver Int (2013) 33(9):1428–40. doi: 10.1111/liv.12262
102. Cogger VC, Arias IM, Warren A, McMahon AC, Kiss DL, Avery VM, et al. The Response of Fenestrations, Actin, and Caveolin-1 to Vascular Endothelial Growth Factor in SK Hep1 Cells. Am J Physiol Gastrointest Liver Physiol (2008) 295(1):G137–45. doi: 10.1152/ajpgi.00069.2008
103. Gracia-Sancho J, Laviña B, Rodríguez-Vilarrupla A, García-Calderó H, Bosch J, García-Pagán JC. Enhanced Vasoconstrictor Prostanoid Production by Sinusoidal Endothelial Cells Increases Portal Perfusion Pressure in Cirrhotic Rat Livers. J Hepatol (2007) 47(2):220–7. doi: 10.1016/j.jhep.2007.03.014
104. Rosado E, Rodríguez-Vilarrupla A, Gracia-Sancho J, Monclús M, Bosch J, García-Pagán JC. Interaction Between NO and COX Pathways Modulating Hepatic Endothelial Cells From Control and Cirrhotic Rats. J Cell Mol Med (2012) 16(10):2461–70. doi: 10.1111/j.1582-4934.2012.01563.x
105. Marrone G, Russo L, Rosado E, Hide D, García-Cardeña G, García-Pagán JC, et al. The Transcription Factor KLF2 Mediates Hepatic Endothelial Protection and Paracrine Endothelial–Stellate Cell Deactivation Induced by Statins. J Hepatol (2013) 58(1):98–103. doi: 10.1016/j.jhep.2012.08.026
106. Forbes SJ, Newsome PN. New Horizons for Stem Cell Therapy in Liver Disease. J Hepatol (2012) 56(2):496–9. doi: 10.1016/j.jhep.2011.06.022
107. Zhang S, Yang Y, Fan L, Zhang F, Li L. The Clinical Application of Mesenchymal Stem Cells in Liver Disease: The Current Situation and Potential Future. Ann Trans Med (2020) 8(8):565. doi: 10.21037/atm.2020.03.218
108. Taghavi-Farahabadi M, Mahmoudi M, Soudi S, Hashemi SM. Hypothesis for the Management and Treatment of the COVID-19-Induced Acute Respiratory Distress Syndrome and Lung Injury Using Mesenchymal Stem Cell-Derived Exosomes. Med Hypotheses (2020) 144:109865. doi: 10.1016/j.mehy.2020.109865
109. Zhang Y, Cai W, Huang Q, Gu Y, Shi Y, Huang J, et al. Mesenchymal Stem Cells Alleviate Bacteria-Induced Liver Injury in Mice by Inducing Regulatory Dendritic Cells. Hepatology (2014) 59(2):671–82. doi: 10.1002/hep.26670
110. Zhang Y, Yoneyama H, Wang Y, Ishikawa S, Hashimoto S-i, Gao J-L, et al. Mobilization of Dendritic Cell Precursors Into the Circulation by Administration of MIP-1α in Mice. J Natl Cancer Inst (2004) 96(3):201–9. doi: 10.1093/jnci/djh024
111. Vasandan AB, Jahnavi S, Shashank C, Prasad P, Kumar A, Prasanna SJ. Human Mesenchymal Stem Cells Program Macrophage Plasticity by Altering Their Metabolic Status via a PGE 2-Dependent Mechanism. Sci Rep (2016) 6(1):1–17. doi: 10.1038/srep38308
112. Taimr P, Higuchi H, Kocova E, Rippe RA, Friedman S, Gores GJ. Activated Stellate Cells Express the TRAIL Receptor-2/Death Receptor-5 and Undergo TRAIL-Mediated Apoptosis. Hepatology (2003) 37(1):87–95. doi: 10.1053/jhep.2003.50002
113. Li Y-H, Shen S, Shao T, Jin M-T, Fan D-D, Lin A-F, et al. Mesenchymal Stem Cells Attenuate Liver Fibrosis by Targeting Ly6Chi/lo Macrophages Through Activating the Cytokine-Paracrine and Apoptotic Pathways. Cell Death Discov (2021) 7(1):1–16. doi: 10.1038/s41420-021-00584-z
114. Saidi RF, Kenari SKH. Liver Ischemia/Reperfusion Injury: An Overview. J Invest Surg (2014) 27(6):366–79. doi: 10.3109/08941939.2014.932473
115. Cannistrà M, Ruggiero M, Zullo A, Gallelli G, Serafini S, Maria M, et al. Hepatic Ischemia Reperfusion Injury: A Systematic Review of Literature and the Role of Current Drugs and Biomarkers. Int J Surg (2016) 33:S57–70. doi: 10.1016/j.ijsu.2016.05.050
116. Shi X-L, Gu J-Y, Han B, Xu H-Y, Fang L, Ding Y-T. Magnetically Labeled Mesenchymal Stem Cells After Autologous Transplantation Into Acutely Injured Liver. World J Gastroenterol: WJG (2010) 16(29):3674. doi: 10.3748/wjg.v16.i29.3674
117. Maher JJ, Scott MK, Saito JM, Burton MC. Adenovirus-Mediated Expression of Cytokine-Induced Neutrophil Chemoattractant in Rat Liver Induces a Neutrophilic Hepatitis. Hepatology (1997) 25(3):624–30. doi: 10.1002/hep.510250322
118. Hewett JA, Schultze AE, VanCise S, Roth R. Neutrophil Depletion Protects Against Liver Injury From Bacterial Endotoxin. Lab Invest J Tech Methods Pathol (1992) 66(3):347–61.
119. Li S, Zheng X, Li H, Zheng J, Chen X, Liu W, et al. Mesenchymal Stem Cells Ameliorate Hepatic Ischemia/Reperfusion Injury via Inhibition of Neutrophil Recruitment. J Immunol Res (2018) 2018:7283703. doi: 10.1155/2018/7283703
120. Eash KJ, Greenbaum AM, Gopalan PK, Link DC. CXCR2 and CXCR4 Antagonistically Regulate Neutrophil Trafficking From Murine Bone Marrow. J Clin Invest (2010) 120(7):2423–31. doi: 10.1172/JCI41649
121. Yi T, Song SU. Immunomodulatory Properties of Mesenchymal Stem Cells and Their Therapeutic Applications. Arch Pharm Res (2012) 35(2):213–21. doi: 10.1007/s12272-012-0202-z
122. Wang H, Zhang H, Huang B, Miao G, Yan X, Gao G, et al. Mesenchymal Stem Cells Reverse High−Fat Diet−Induced Non−Alcoholic Fatty Liver Disease Through Suppression of CD4+ T Lymphocytes in Mice. Mol Med Rep (2018) 17(3):3769–74. doi: 10.3892/mmr.2017.8326
123. Lafdil F, Miller AM, Ki SH, Gao B. Th17 Cells and Their Associated Cytokines in Liver Diseases. Cell Mol Immunol (2010) 7(4):250–4. doi: 10.1038/cmi.2010.5
124. Jin Z, You J, Wang H. The Role of the Balance Between Th17 and Treg in Liver Disease. Zhonghua Gan Zang Bing Za Zhi= Zhonghua Ganzangbing Zazhi= Chin J Hepatol (2017) 25(8):637–40. doi: 10.3760/cma.j.issn.1007-3418.2017.08.017
125. Chen Q-H, Wu F, Liu L, H-b C, Zheng R-Q, Wang H-L, et al. Mesenchymal Stem Cells Regulate the Th17/Treg Cell Balance Partly Through Hepatocyte Growth Factor In Vitro. Stem Cell Res Ther (2020) 11(1):1–11. doi: 10.1186/s13287-020-01612-y
126. Milosavljevic N, Gazdic M, Simovic Markovic B, Arsenijevic A, Nurkovic J, Dolicanin Z, et al. Mesenchymal Stem Cells Attenuate Liver Fibrosis by Suppressing Th17 Cells–an Experimental Study. Transplant Int (2018) 31(1):102–15. doi: 10.1111/tri.13023
127. Yan S, Wang L, Liu N, Wang Y, Chu Y. Critical Role of Interleukin-17/Interleukin-17 Receptor Axis in Mediating Con A-Induced Hepatitis. Immunol Cell Biol (2012) 90(4):421–8. doi: 10.1038/icb.2011.59
128. Milosavljevic N, Gazdic M, Simovic Markovic B, Arsenijevic A, Nurkovic J, Dolicanin Z, et al. Mesenchymal Stem Cells Attenuate Acute Liver Injury by Altering Ratio Between Interleukin 17 Producing and Regulatory Natural Killer T Cells. Liver Transplant (2017) 23(8):1040–50. doi: 10.1002/lt.24784
129. Mazhari S, Gitiara A, Baghaei K, Hatami B, Rad RE, Asadirad A, et al. Therapeutic Potential of Bone Marrow-Derived Mesenchymal Stem Cells and Imatinib in a Rat Model of Liver Fibrosis. Eur J Pharmacol (2020) 882:173263. doi: 10.1016/j.ejphar.2020.173263
130. Chen L, Zhang C, Chen L, Wang X, Xiang B, Wu X, et al. Human Menstrual Blood-Derived Stem Cells Ameliorate Liver Fibrosis in Mice by Targeting Hepatic Stellate Cells via Paracrine Mediators. Stem Cells Trans Med (2017) 6(1):272–84. doi: 10.5966/sctm.2015-0265
131. Wang S, Kim J, Lee C, Oh D, Han J, Kim T-J, et al. Tumor Necrosis Factor-Inducible Gene 6 Reprograms Hepatic Stellate Cells Into Stem-Like Cells, Which Ameliorates Liver Damage in Mouse. Biomaterials (2019) 219:119375. doi: 10.1016/j.biomaterials.2019.119375
132. Liang T, Zhu L, Gao W, Gong M, Ren J, Yao H, et al. Coculture of Endothelial Progenitor Cells and Mesenchymal Stem Cells Enhanced Their Proliferation and Angiogenesis Through PDGF and Notch Signaling. FEBS Open Bio (2017) 7(11):1722–36. doi: 10.1002/2211-5463.12317
133. Hu C, Li L. The Immunoregulation of Mesenchymal Stem Cells Plays a Critical Role in Improving the Prognosis of Liver Transplantation. J Trans Med (2019) 17(1):1–12. doi: 10.1186/s12967-019-02167-0
134. Pournasr B, Mohamadnejad M, Bagheri M, Aghdami N, Shahsavani M, Malekzadeh R, et al. In Vitro Differentiation of Human Bone Marrow Mesenchymal Stem Cells Into Hepatocyte-Like Cells. Arch Iranian Med (2011) 14(4):0–.
135. Yin L, Zhu Y, Yang J, Ni Y, Zhou Z, Chen Y, et al. Adipose Tissue-Derived Mesenchymal Stem Cells Differentiated Into Hepatocyte-Like Cells In Vivo and In Vitro. Mol Med Rep (2015) 11(3):1722–32. doi: 10.3892/mmr.2014.2935
136. Yin F, Wang W-Y, Jiang W-H. Human Umbilical Cord Mesenchymal Stem Cells Ameliorate Liver Fibrosis In Vitro and In Vivo: From Biological Characteristics to Therapeutic Mechanisms. World J Stem Cells (2019) 11(8):548. doi: 10.4252/wjsc.v11.i8.548
137. Khodabandeh Z, Vojdani Z, Talaei-Khozani T, Jaberipour M, Hosseini A, Bahmanpour S. Comparison of the Expression of Hepatic Genes by Human Wharton’s Jelly Mesenchymal Stem Cells Cultured in 2D and 3D Collagen Culture Systems. Iranian J Med Sci (2016) 41(1):28.
138. Anzalone R, Iacono ML, Corrao S, Magno F, Loria T, Cappello F, et al. New Emerging Potentials for Human Wharton’s Jelly Mesenchymal Stem Cells: Immunological Features and Hepatocyte-Like Differentiative Capacity. Stem Cells Dev (2010) 19(4):423–38. doi: 10.1089/scd.2009.0299
139. Kim JY, Jun JH, Park SY, Yang SW, Bae SH, Kim GJ. Dynamic Regulation of miRNA Expression by Functionally Enhanced Placental Mesenchymal Stem Cells Promotes Hepatic Regeneration in a Rat Model With Bile Duct Ligation. Int J Mol Sci (2019) 20(21):5299. doi: 10.3390/ijms20215299
140. Lu L-L, Liu Y-J, Yang S-G, Zhao Q-J, Wang X, Gong W, et al. Isolation and Characterization of Human Umbilical Cord Mesenchymal Stem Cells With Hematopoiesis-Supportive Function and Other Potentials. Haematologica (2006) 91(8):1017–26.
141. Deuse T, Stubbendorff M, Tang-Quan K, Phillips N, Kay MA, Eiermann T, et al. Immunogenicity and Immunomodulatory Properties of Umbilical Cord Lining Mesenchymal Stem Cells. Cell Transplant (2011) 20(5):655–67. doi: 10.3727/096368910X536473
142. Amer M-EM, El-Sayed SZ, Abou El-Kheir W, Gabr H, Gomaa AA, El-Noomani N, et al. Clinical and Laboratory Evaluation of Patients With End-Stage Liver Cell Failure Injected With Bone Marrow-Derived Hepatocyte-Like Cells. Eur J Gastroenterol Hepatol (2011) 23(10):936–41. doi: 10.1097/MEG.0b013e3283488b00
143. Kharaziha P, Hellström PM, Noorinayer B, Farzaneh F, Aghajani K, Jafari F, et al. Improvement of Liver Function in Liver Cirrhosis Patients After Autologous Mesenchymal Stem Cell Injection: A Phase I–II Clinical Trial. Eur J Gastroenterol Hepatol (2009) 21(10):1199–205. doi: 10.1097/MEG.0b013e32832a1f6c
144. Suk KT, Yoon JH, Kim MY, Kim CW, Kim JK, Park H, et al. Transplantation With Autologous Bone Marrow-Derived Mesenchymal Stem Cells for Alcoholic Cirrhosis: Phase 2 Trial. Hepatology (2016) 64(6):2185–97. doi: 10.1002/hep.28693
145. Mohamadnejad M, Bashtar M, Ardeshir G, Malekzadeh R. 106: Phase 1 Trial of Autologous Bone Marrow Mesenchymal Stem Cell Transplantation in Patients With Decompensated. Arch Iranian Med (2007) 10(4):459–66.
146. Sun A, Gao W, Xiao T. Autologous Bone Marrow Stem Cell Transplantation via the Hepatic Artery for the Treatment of Hepatitis B Virus-Related Cirrhosis: A PRISMA-Compliant Meta-Analysis Based on the Chinese Population. Stem Cell Res Ther (2020) 11(1):1–17. doi: 10.1186/s13287-020-01627-5
147. Xu L, Gong Y, Wang B, Shi K, Hou Y, Wang L, et al. Randomized Trial of Autologous Bone Marrow Mesenchymal Stem Cells Transplantation for Hepatitis B Virus Cirrhosis: Regulation of T Reg/T H17 Cells. J Gastroenterol Hepatol (2014) 29(8):1620–8. doi: 10.1111/jgh.12653
148. Casiraghi F, Perico N, Podestà MA, Todeschini M, Zambelli M, Colledan M, et al. Third-Party Bone Marrow–Derived Mesenchymal Stromal Cell Infusion Before Liver Transplantation: A Randomized Controlled Trial. Am J Transplant (2021) 21(8):2795–809. doi: 10.1111/ajt.16468
149. Fan X-L, Zhang Y, Li X, Fu Q-L. Mechanisms Underlying the Protective Effects of Mesenchymal Stem Cell-Based Therapy. Cell Mol Life Sci (2020) 77(14):2771–94. doi: 10.1007/s00018-020-03454-6
150. Zarà M, Guidetti GF, Camera M, Canobbio I, Amadio P, Torti M, et al. Biology and Role of Extracellular Vesicles (EVs) in the Pathogenesis of Thrombosis. Int J Mol Sci (2019) 20(11):2840. doi: 10.3390/ijms20112840
151. Malekpour K, Hazrati A, Zahar M, Markov A, Zekiy AO, Navashenaq JG, et al. The Potential Use of Mesenchymal Stem Cells and Their Derived Exosomes for Orthopedic Diseases Treatment. Stem Cell Rev Rep (2021), 1–19. doi: 10.1007/s12015-021-10185-z
152. Anand S, Samuel M, Mathivanan S. Exomeres: A New Member of Extracellular Vesicles Family. Subcell Biochem (2021) 97:89–97. doi: 10.1007/978-3-030-67171-6_5
153. Pegtel DM, Gould SJ. Exosomes. Annu Rev Biochem (2019) 88:487–514. doi: 10.1146/annurev-biochem-013118-111902
154. Jabbari N, Akbariazar E, Feqhhi M, Rahbarghazi R, Rezaie J. Breast Cancer-Derived Exosomes: Tumor Progression and Therapeutic Agents. J Cell Physiol (2020) 235(10):6345–56. doi: 10.1002/jcp.29668
155. Lai P, Weng J, Guo L, Chen X, Du X. Novel Insights Into MSC-EVs Therapy for Immune Diseases. biomark Res (2019) 7(1):1–10. doi: 10.1186/s40364-019-0156-0
156. Alcaraz MJ, Compañ A, Guillén MI. Extracellular Vesicles From Mesenchymal Stem Cells as Novel Treatments for Musculoskeletal Diseases. Cells (2020) 9(1):98. doi: 10.3390/cells9010098
157. Khoei SG, Dermani FK, Malih S, Fayazi N, Sheykhhasan M. The Use of Mesenchymal Stem Cells and Their Derived Extracellular Vesicles in Cardiovascular Disease Treatment. Curr Stem Cell Res Ther (2020) 15(7):623–38. doi: 10.2174/1574888X15666200501235201
158. Rostom DM, Attia N, Khalifa HM, Abou Nazel MW, El Sabaawy EA. The Therapeutic Potential of Extracellular Vesicles Versus Mesenchymal Stem Cells in Liver Damage. Tissue Eng Regen Med (2020) 17:537–52. doi: 10.1007/s13770-020-00267-3
159. Yin Z, Jiang K, Li R, Dong C, Wang L. Multipotent Mesenchymal Stromal Cells Play Critical Roles in Hepatocellular Carcinoma Initiation, Progression and Therapy. Mol Cancer (2018) 17(1):1–17. doi: 10.1186/s12943-018-0926-6
160. Shojaei S, Hashemi SM, Ghanbarian H, Salehi M, Mohammadi-Yeganeh S. Effect of Mesenchymal Stem Cells-Derived Exosomes on Tumor Microenvironment: Tumor Progression Versus Tumor Suppression. J Cell Physiol (2019) 234(4):3394–409. doi: 10.1002/jcp.27326
161. Zhang F, Guo J, Zhang Z, Qian Y, Wang G, Duan M, et al. Mesenchymal Stem Cell-Derived Exosome: A Tumor Regulator and Carrier for Targeted Tumor Therapy. Cancer Lett (2022) 526:29–40. doi: 10.1016/j.canlet.2021.11.015
162. Lou G, Chen L, Xia C, Wang W, Qi J, Li A, et al. MiR-199a-Modified Exosomes From Adipose Tissue-Derived Mesenchymal Stem Cells Improve Hepatocellular Carcinoma Chemosensitivity Through mTOR Pathway. J Exp Clin Cancer Res (2020) 39(1):1–9. doi: 10.1186/s13046-019-1512-5
163. Zhang F, Lu Y, Wang M, Zhu J, Li J, Zhang P, et al. Exosomes Derived From Human Bone Marrow Mesenchymal Stem Cells Transfer miR-222-3p to Suppress Acute Myeloid Leukemia Cell Proliferation by Targeting IRF2/INPP4B. Mol Cell Probes (2020) 51:101513. doi: 10.1016/j.mcp.2020.101513
164. Li X, Wang K, Ai H. Human Umbilical Cord Mesenchymal Stem Cell-Derived Extracellular Vesicles Inhibit Endometrial Cancer Cell Proliferation and Migration Through Delivery of Exogenous miR-302a. Stem Cells Int (2019) 2019:8108576. doi: 10.1155/2019/8108576
165. Bagheri E, Abnous K, Farzad SA, Taghdisi SM, Ramezani M, Alibolandi M. Targeted Doxorubicin-Loaded Mesenchymal Stem Cells-Derived Exosomes as a Versatile Platform for Fighting Against Colorectal Cancer. Life Sci (2020) 261:118369. doi: 10.1016/j.lfs.2020.118369
166. Nitzsche F, Müller C, Lukomska B, Jolkkonen J, Deten A, Boltze J. Concise Review: MSC Adhesion Cascade—Insights Into Homing and Transendothelial Migration. Stem Cells (2017) 35(6):1446–60. doi: 10.1002/stem.2614
167. Wu Q, Zhou L, Lv D, Zhu X, Tang H. Exosome-Mediated Communication in the Tumor Microenvironment Contributes to Hepatocellular Carcinoma Development and Progression. J Hematol Oncol (2019) 12(1):1–11. doi: 10.1186/s13045-019-0739-0
168. Borrelli DA, Yankson K, Shukla N, Vilanilam G, Ticer T, Wolfram J. Extracellular Vesicle Therapeutics for Liver Disease. J Control Release (2018) 273:86–98. doi: 10.1016/j.jconrel.2018.01.022
169. Li T, Yan Y, Wang B, Qian H, Zhang X, Shen L, et al. Exosomes Derived From Human Umbilical Cord Mesenchymal Stem Cells Alleviate Liver Fibrosis. Stem Cells Dev (2013) 22(6):845–54. doi: 10.1089/scd.2012.0395
170. Haga H, Yan IK, Borrelli DA, Matsuda A, Parasramka M, Shukla N, et al. Extracellular Vesicles From Bone Marrow–Derived Mesenchymal Stem Cells Protect Against Murine Hepatic Ischemia/Reperfusion Injury. Liver Transplant (2017) 23(6):791–803. doi: 10.1002/lt.24770
171. Lee SC, Kim K-H, Kim O-H, Lee SK, Hong H-E, Won SS, et al. Determination of Optimized Oxygen Partial Pressure to Maximize the Liver Regenerative Potential of the Secretome Obtained From Adipose-Derived Stem Cells. Stem Cell Res Ther (2017) 8(1):1–12. doi: 10.1186/s13287-017-0635-x
172. Mardpour S, Hassani SN, Mardpour S, Sayahpour F, Vosough M, Ai J, et al. Extracellular Vesicles Derived From Human Embryonic Stem Cell-MSCs Ameliorate Cirrhosis in Thioacetamide-Induced Chronic Liver Injury. J Cell Physiol (2018) 233(12):9330–44. doi: 10.1002/jcp.26413
173. Yan Y, Jiang W, Tan Y, Zou S, Zhang H, Mao F, et al. hucMSC Exosome-Derived GPX1 is Required for the Recovery of Hepatic Oxidant Injury. Mol Ther (2017) 25(2):465–79. doi: 10.1016/j.ymthe.2016.11.019
174. Dong L, Pu Y, Chen X, Qi X, Zhang L, Xu L, et al. hUCMSC-Extracellular Vesicles Downregulated Hepatic Stellate Cell Activation and Reduced Liver Injury in S. Japonicum-Infected Mice. Stem Cell Res Ther (2020) 11(1):1–11.
175. Tan CY, Lai RC, Wong W, Dan YY, Lim S-K, Ho HK. Mesenchymal Stem Cell-Derived Exosomes Promote Hepatic Regeneration in Drug-Induced Liver Injury Models. Stem Cell Res Ther (2014) 5(3):1–14. doi: 10.1186/scrt465
176. Zhao S, Liu Y, Pu Z. Bone Marrow Mesenchymal Stem Cell-Derived Exosomes Attenuate D-GaIN/LPS-Induced Hepatocyte Apoptosis by Activating Autophagy In Vitro. Drug Design Dev Ther (2019) 13:2887. doi: 10.2147/DDDT.S220190
177. Tamura R, Uemoto S, Tabata Y. Immunosuppressive Effect of Mesenchymal Stem Cell-Derived Exosomes on a Concanavalin A-Induced Liver Injury Model. Inflamm Regen (2016) 36(1):1–11. doi: 10.1186/s41232-016-0030-5
178. Haga H, Yan IK, Takahashi K, Matsuda A, Patel T. Extracellular Vesicles From Bone Marrow-Derived Mesenchymal Stem Cells Improve Survival From Lethal Hepatic Failure in Mice. Stem Cells Trans Med (2017) 6(4):1262–72. doi: 10.1002/sctm.16-0226
179. You Q, Cheng L, Kedl RM, Ju C. Mechanism of T Cell Tolerance Induction by Murine Hepatic Kupffer Cells. Hepatology (2008) 48(3):978–90. doi: 10.1002/hep.22395
180. Jin Y, Wang J, Li H, Gao S, Shi R, Yang D, et al. Extracellular Vesicles Secreted by Human Adipose-Derived Stem Cells (hASCs) Improve Survival Rate of Rats With Acute Liver Failure by Releasing lncRNA H19. EBioMedicine (2018) 34:231–42. doi: 10.1016/j.ebiom.2018.07.015
181. Rong X, Liu J, Yao X, Jiang T, Wang Y, Xie F. Human Bone Marrow Mesenchymal Stem Cells-Derived Exosomes Alleviate Liver Fibrosis Through the Wnt/β-Catenin Pathway. Stem Cell Res Ther (2019) 10(1):1–11. doi: 10.1186/s13287-019-1204-2
182. Heidari N, Abbasi-Kenarsari H, Namaki S, Baghaei K, Zali MR, Ghaffari Khaligh S, et al. Adipose-Derived Mesenchymal Stem Cell-Secreted Exosome Alleviates Dextran Sulfate Sodium-Induced Acute Colitis by Treg Cell Induction and Inflammatory Cytokine Reduction. J Cell Physiol (2021) 236(8):5906–20. doi: 10.1002/jcp.30275
183. Chen Y-A, Lu C-H, Ke C-C, Liu R-S. Mesenchymal Stem Cell-Derived Extracellular Vesicle-Based Therapy for Alzheimer’s Disease: Progress and Opportunity. Membranes (2021) 11(10):796. doi: 10.3390/membranes11100796
184. Liu C, Bayado N, He D, Li J, Chen H, Li L, et al. Therapeutic Applications of Extracellular Vesicles for Myocardial Repair. Front Cardiovasc Med (1802) 2021:8. doi: 10.3389/fcvm.2021.758050
185. Maumus M, Rozier P, Boulestreau J, Jorgensen C, Noël D. Mesenchymal Stem Cell-Derived Extracellular Vesicles: Opportunities and Challenges for Clinical Translation. Front Bioeng Biotechnol (2020) 8:997. doi: 10.3389/fbioe.2020.00997
Keywords: MSCs, exosomes, liver disease, immunomodulation, inflammation, regeneration
Citation: Hazrati A, Malekpour K, Soudi S and Hashemi SM (2022) Mesenchymal Stromal/Stem Cells and Their Extracellular Vesicles Application in Acute and Chronic Inflammatory Liver Diseases: Emphasizing on the Anti-Fibrotic and Immunomodulatory Mechanisms. Front. Immunol. 13:865888. doi: 10.3389/fimmu.2022.865888
Received: 30 January 2022; Accepted: 15 March 2022;
Published: 07 April 2022.
Edited by:
Yu-Chen Fan, Shandong University, ChinaReviewed by:
Ze-Hua Zhao, Shandong University, ChinaBarbara Mara Klinkhammer, University Hospital RWTH Aachen, Germany
Copyright © 2022 Hazrati, Malekpour, Soudi and Hashemi. This is an open-access article distributed under the terms of the Creative Commons Attribution License (CC BY). The use, distribution or reproduction in other forums is permitted, provided the original author(s) and the copyright owner(s) are credited and that the original publication in this journal is cited, in accordance with accepted academic practice. No use, distribution or reproduction is permitted which does not comply with these terms.
*Correspondence: Seyed Mahmoud Hashemi, c21taGFzaGVtaUBzYm11LmFjLmly