- 1Health Research Institute of Navarra (IdiSNA), Pamplona, Spain
- 2Programs in Solid Tumors and Neuroscience, Foundation for the Applied Medical Research, Pamplona, Spain
- 3Department of Pediatrics, Clínica Universidad de Navarra, Pamplona, Spain
Immunotherapy has seen tremendous strides in the last decade, acquiring a prominent position at the forefront of cancer treatment since it has been proven to be efficacious for a wide variety of tumors. Nevertheless, while immunotherapy has changed the paradigm of adult tumor treatment, this progress has not yet been translated to the pediatric solid tumor population. For this reason, alternative curative therapies are urgently needed for the most aggressive pediatric tumors. In recent years, oncolytic virotherapy has consolidated as a feasible strategy for cancer treatment, not only for its tumor-specific effects and safety profile but also for its capacity to trigger an antitumor immune response. This review will summarize the current status of immunovirotherapy to treat cancer, focusing on pediatric solid malignancies. We will revisit previous basic, translational, and clinical research and discuss advances in overcoming the existing barriers and limitations to translate this promising therapeutic as an every-day cancer treatment for the pediatric and young adult populations.
Introduction of Pediatric Cancer
Pediatric cancer includes all malignancies that occur in children and adolescents between birth and 19 years of age, and it is estimated that every year approximately 400,000 cases are diagnosed worldwide (1, 2). Recent advances in cancer research have resulted in a marked increase in the cure rates of both adult and pediatric cancers. However, cancer remains a significant cause of death for children and adolescents (3). Among the factors that explain the improvement in survival rates are the optimization of supportive care, advances in biological and clinical tumor characterization and the development of new risk-adapted therapies are the most remarkable (4, 5).
Unfortunately, this improvement has not always been accompanied by improved quality of life because of side effects and long-term health complications in survivors of childhood cancers as they reach older age (6). These data underscore the necessity to develop safe and efficacious treatments that overcome the current limitations in the field of pediatric cancer.
In recent years, cancer therapy has experienced a remarkable transformation due to the advent of new classes of immunotherapies, including immune checkpoint inhibitors, bispecific T cell engagers (BiTEs) and CAR-T cells. In fact, CAR-T cells have provided a new paradigm for treatment, especially for liquid tumors (7, 8). Another approach that has gained popularity is the use of oncolytic viruses (OVs). These viruses combine their cytotoxic capacity with the ability to trigger an immune response, rendering them interesting therapeutic tools. The notion of viruses as anticancer agents came from anecdotic observations where tumors regressed spontaneously after the patients naturally acquired viral infections (9–11). These reports were mainly from the early 1900s, and it was not until the late 1980s that OVs were evaluated in depth, in part due to the development of research tools such as cell lines and animal models that have facilitated the evaluation of these agents (12). Since then, multiple investigations have been carried out, and the first OV has been approved for clinical use in the USA. The use of Talimogene Laherparepvec in clinical practice for recurrent melanoma represents a before-and-after picture for OVs, indicating the possibility of developing new, functional and perfectly designed tools for tumor treatment (13).
In this review, we discuss the role of OVs as a therapy for pediatric solid malignancies. We review the different types of viruses and their mechanism of action. We recapitulate the basic, translational and clinical research using virotherapy, alone or in combination with other therapies, to treat pediatric solid tumors, and we conclude with our thoughts regarding the potential future and hurdles for the development of this field to achieve its full therapeutic potential.
Oncolytic Viruses
Oncolytic viral therapy is a promising therapeutic method that employs naturally occurring or genetically modified OVs that selectively proliferate in and kill tumor cells while causing no harm to healthy cells (14). OVs can be classified as DNA or RNA viruses on the basis of their genome. Furthermore, they differ in their viral envelope based on host cell membranes and viral glycoproteins. According to these criteria, these viruses can be classified as enveloped DNA OVs (herpesvirus, poxvirus), unenveloped DNA OVs (adenovirus, parvovirus), enveloped RNA OVs (paramyxovirus, rhabdovirus, togavirus, orthomyxovirus), or unenveloped RNA OVs (reovirus, picornavirus) (Figure 1).
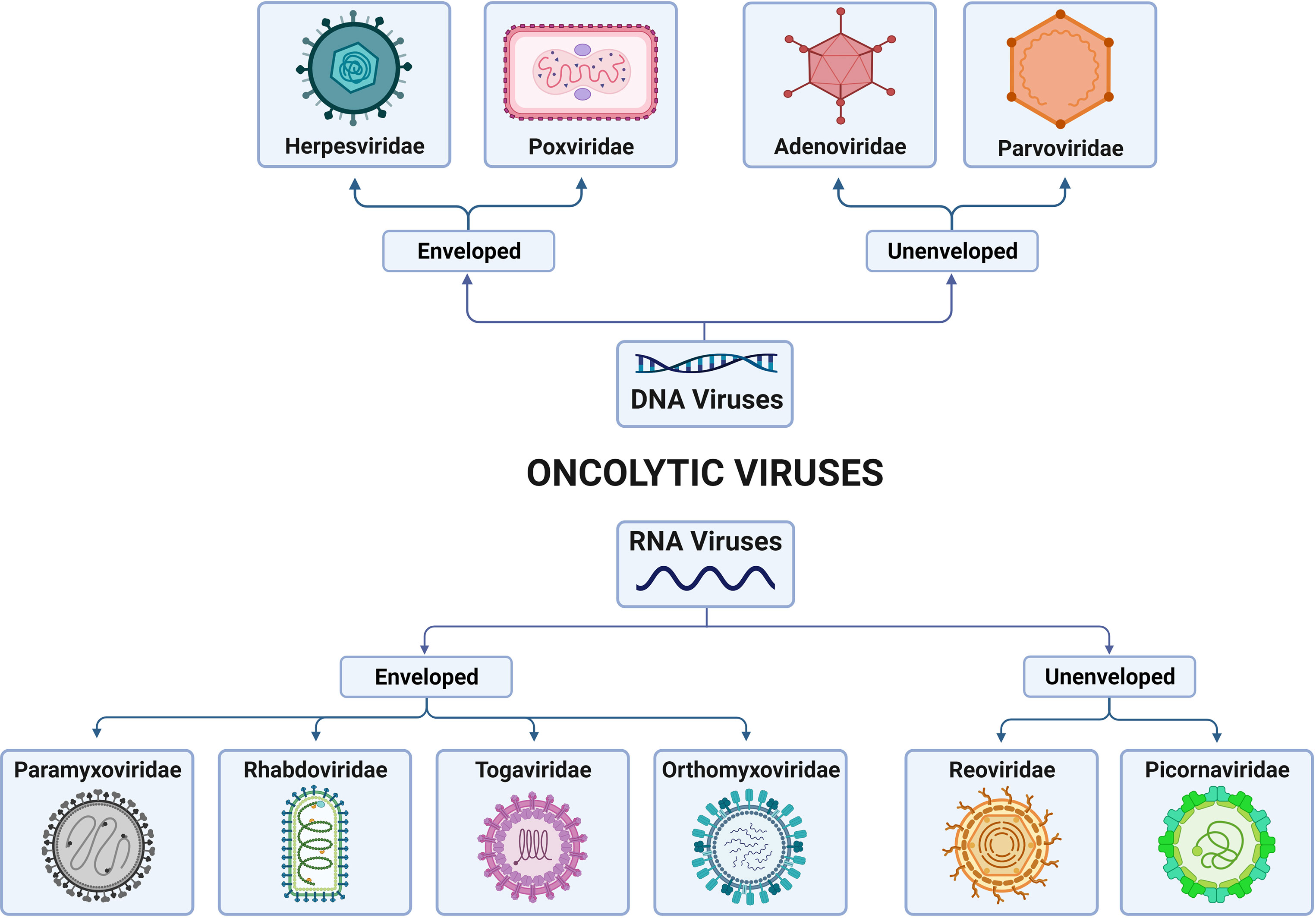
Figure 1 Classification of families of oncolytic viruses (OVs) according to the genome and presence/absence of the viral envelope. OVs can be differentiated into DNA viruses and RNA viruses, depending on their genomic nature. In addition, both types can present, or not present, virus envelopes. Thus, DNA-enveloped viruses include those of the Herpesviridae and Poxviridae families; non-DNA-enveloped viruses include those of the Adenoviridae and Paroviridae families; RNA-enveloped viruses include those of the Paramyxoviridae, Rhabdoviridae, Togaviridae and Orthoyxoviridae families; and non-RNA-enveloped viruses include those of the Reoviridae and Picornaviridae families. Created with BioRender.com.
OVs elicit antitumor responses mainly through two mechanisms: selective killing of tumor cells and stimulation of systemic antitumor immunity (15). Cancer cells provide an ideal setting for the selective replication of various OVs that take advantage of physiological changes in these cells. Several signaling pathways engaged in viral elimination, including interferon, Toll-like receptor (TLR) or Janus kinase-signal transducer and activator of transcription (JAK-STAT) pathways, may be defective or inhibited, enabling OVs to spread in tumor cells. Regarding infectivity, cancer cells may also overexpress different surface receptors, such as CD46, ICAM-1, CD55, CD155 or integrins, allowing OVs to infect cancer cells (16). In addition to directly killing infected tumor cells via several oncolytic mechanisms, OVs have the ability to turn tumors from immunologically ‘cold’ to ‘hot’ by inducing proinflammatory conditions within the tumor microenvironment (TME) (15). Oncolytic cell death and subsequent release of tumor-associated antigens can induce innate and adaptive immune responses, resulting in therapeutic responses in both locally injected tumors and tumor metastases (15, 17, 18) (Figure 2).
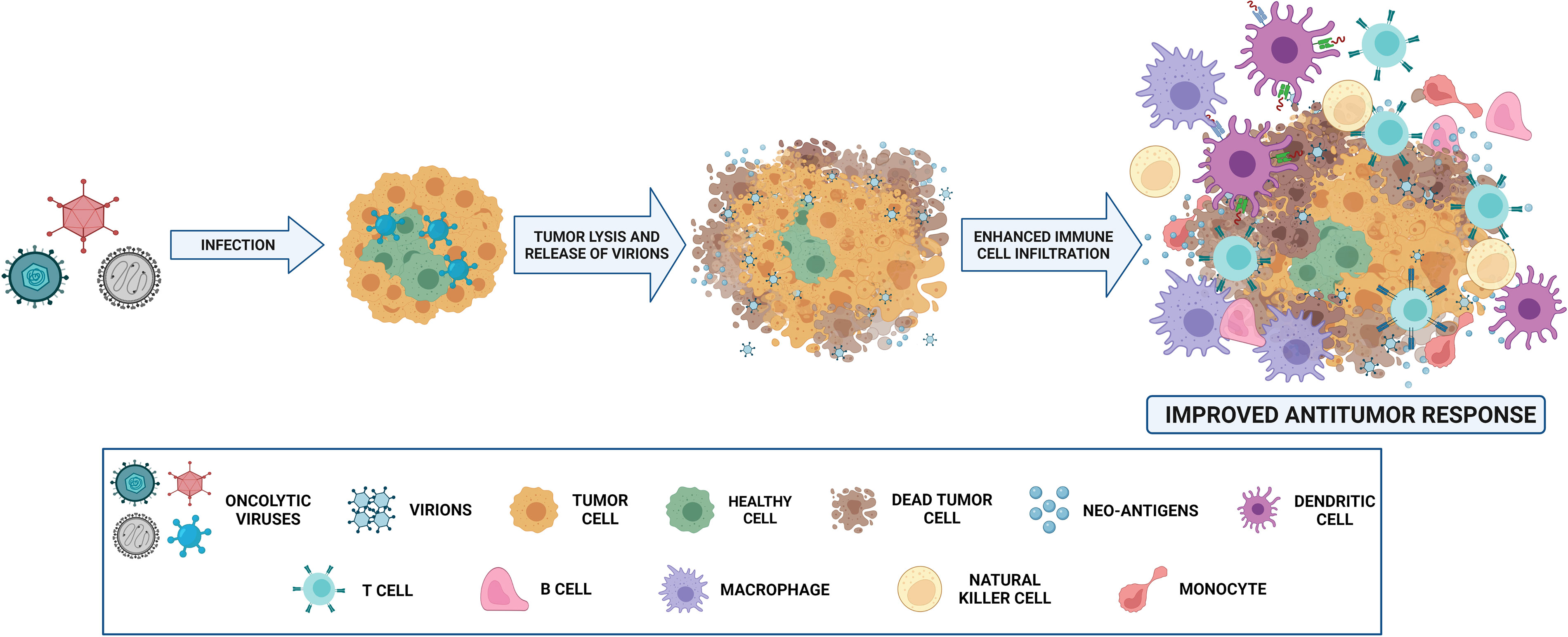
Figure 2 Mechanism of action of oncolytic viruses (OVs). OVs, administered intravenously or intratumorally, infect normal and tumor cells. Nevertheless, they replicate only in malignant cells, and virion release then occurs. The infection and destruction of tumor cells lead to the release of antigens that cause proinflammatory recruitment of different immune cells, such as T and B cells, macrophages, NK cells, monocytes and dendritic cells. Thus, antitumoral effect is mediated not only by oncolytic capability, but also by immune microenvironment reshaping. Created with BioRender.com.
With their multimodal antitumor activity, oncolytic virotherapies are now a key subject of study in cancer treatment research, and many clinical trials are currently being conducted for the utilization of OVs as therapeutic agents for different types of advanced malignancies (19, 20). OVs for different types of malignancies are chosen based on a variety of factors. Some of them may have intrinsic tropism and a preference for selective replication in cancer cells, whereas others can be genetically modified to elicit selective targeting of cancer cells (21, 22). Generally, OVs infect tumor cells via specific receptors on the cell surface. Once inside, the virus particles migrate through the cytoskeleton and start replication, being internalized or not into the nucleus. The viral seizure of the cellular machinery allows the generation of new virions, which then facilitate lysis of the infected cell and spread to infect new cells (23). New advances in oncolytic virotherapies are being achieved with genetic deletions and genetic engineering to improve tumor-selective replication and oncolytic capability while lowering viral pathogenicity for patient safety (21, 22, 24). Additionally, OVs can be designed to express novel therapeutic genes to enhance the antitumor action, generation of immunological responses, and suppression of tumor angiogenesis, along with other mechanisms (21, 25). Reoviruses, parvovirus H-1 and Newcastle disease virus (NDV) are examples of OVs that are naturally inclined to replicate in cancer cells (20). On the other hand, other OVs have been genetically modified for oncoselectivity, including adenoviruses, vaccinia virus (VV), vesicular stomatitis virus (VSV) and herpes simplex virus (HSV) (26, 27).
Preclinical Studies Using Virotherapy in Pediatric Solid Tumors
Adenovirus
Adenoviruses are among the most commonly used OVs in research (28). Among the different pediatric solid tumors, those of the central nervous system (CNS) are the most common malignancies among children aged 0 to 14 years, even more so than leukemias, and are among the most prevalent and deadly within the adolescent group (5–24) and young adults (25-39 years) (29). There are more than 100 types of brain tumors (30–32), each with its own characteristics. However, for the purpose of this review, we will focus on those with poor prognosis.
One adenovirus that has been extensively characterized in pediatric and adult brain tumors is Delta-24-RGD. This adenovirus, serotype 5, was specifically designed to destroy tumor cells (33, 34). It contains two genetic modifications: a 24-base pair deletion in E1A, which restricts virus replication in tumor cells, and the addition of the RGD-4C binding motif, which improves the infectivity, allowing the virus to target tumor cells via avβ3 and avβ5 integrins, which are overexpressed in a wide range of tumors. Delta-24-RGD showed a robust antiglioma effect in preclinical and clinical studies in adult patients with recurrent gliomas (34–36). Moreover, the virus displayed the capacity to trigger immune-mediated responses with an increased number of immune populations inside the tumors (37, 38). Our group has evaluated this virus in the context of high-risk pediatric brain tumors. We observed that treatment with Delta-24-RGD resulted in increased survival in human xenograft and syngeneic mouse models of pediatric high-grade gliomas (pHGGs) and diffuse midline gliomas (DMGs) (39). In immunocompetent mice, virus treatment led to robust recruitment of lymphocyte populations (including CD4+ and CD8+). Delta-24-RGD combined with radiotherapy exerted an improved antitumor effect in this type of tumors (40). Furthermore, we evaluated this virus in models of atypical teratoid/rhabdoid tumors (AT/RTs) and CNS-primitive neuroectodermal tumors (CNS-PNETs), rare pediatric embryonal tumors with a survival time of 6-12 months (41, 42). The adenovirus replicated efficiently in AT/RT and CNS-PNET cell lines, leading to a robust cytotoxic effect. In vivo, Delta-24-RGD expanded the overall survival in several animal models, leading, in some cases, to a long-term survival rate of 70%. The interrogation of the immune response triggered by Delta-24-RGD in humanized immunocompetent mouse models revealed an increase in CD8+ T cell infiltration and a general remodeling of the TME toward a proinflammatory phenotype (43). In this line of thinking, another adenovirus, VCN-01, armed with hyaluronidase, which allows the degradation of the extracellular matrix (44), also significantly extended the overall survival of mice bearing orthotopic PNET (45). Mesenchymal stem cells (MSCs), as OV carriers, have also been studied in treating DMGs. Chastkofsky and colleagues encapsulated the adenovirus CRAd.S.pK7 into MSCs to facilitate its delivery to the brainstem and to avoid potential fast clearance by the immune system (46). Although the virus replicated in vitro, the experiments performed in animal models did not show clinical benefit, and it was necessary to add radiotherapy to extend the overall survival.
Another strategy used to enhance the efficacy of oncolytic adenoviruses has been combination with gene therapy. In this sense, Arnone et al. explored the idea of treating a pediatric high-grade glioma with the OV Delta-24 in combination with a replication incompetent adenovirus encoding a BiTE which targets human hepatocellular carcinoma A2 receptor (EphA2), a protein that is correlated with tumor aggressiveness and poor patient outcome. The authors showed that the combination treatment was more efficient than either treatment alone in improving tumor burden and overall survival (47).
Neuroblastoma is a rare neuroendocrine childhood cancer that arises in any neural crest element from the developing sympathetic nervous system (48). It is the most common extracranial solid tumor in childhood and the most common malignancy diagnosed during the first year of life (49). Although outcomes in these patients have improved in recent decades, this improvement is attributable mainly to better cure rates among patients with low-risk neuroblastoma (49), whereas children bearing the more aggressive form of the disease have shown a modest advance (50). In this context, the adenoviruses OBP-301 and OBP-702, the tumor specificity of which is driven by the hTERT promoter, have been evaluated. The authors showed that treatment with either of these viruses produced an antitumor response in cell lines with high hTERT expression and reduced growth in a subcutaneous neuroblastoma model (51). Other approaches have employed cellular carriers to deliver the virus such as Celyvir; autologous MSCs loaded with Icovir-5 (an adenovirus dependent on an aberrant RB pathway). Mice bearing neuroblastomas and treated intravenously with Celyvir displayed a reduced tumor volume, recruitment of immune cells and a weaker protumoral and stronger inflammatory profile in the TME (52). This strategy is currently being evaluated in the clinic and is discussed below.
Pediatric sarcomas, which account for 10% of solid tumors in children, are a group of mesenchymal tumors originating from bone or soft tissue precursors (53). Although current treatment regimens based on chemotherapy, surgery and radiation have improved the 5-year OSR to 60-70%, patients with metastatic disease or recurrence have a poor prognosis, with a 5-year OSR of 30% or less (54, 55). The lack of efficacy of emergent therapies such as immunotherapy (56) has prompted the emergence of OVs as an alternative solution.
Our group has evaluated the antisarcoma effect of the RB pathway-based viruses Delta-24-RGD (57) and VCN-01 (58). Both adenoviruses were able to control tumor volume, and specifically for Delta-24-RGD, the use of cisplatin as a combinatorial treatment improved the antitumor virus response, showing that combination therapies are, in fact, a possible solution. In the quest to further improve the efficacy, our group engineered D24-ACT, which is based on the D24-RGD platform and armed with the immune costimulatory molecule 4-1BB ligand (4-1BBL), to improve the antitumor immune response. Local treatment with Delta-24-ACT in mice bearing orthotopic osteosarcoma murine tumors led to a reduction in both the primary tumor and metastases, and a significant increase in CD3+ and CD8+ T cells, among other immune populations, was found when comparing D24-ACT vs. D24-RGD (59). Our results suggest that potentiating the immune response could boost the efficacy in this type of tumor. In another study, a murine version of Celyvir (OAd-MSCs) was tested in combination with granulocyte-colony stimulating factor (G-CSF). The combination significantly reduced tumor growth in vivo, with tumors presenting higher infiltration of some immune cell populations (including CD4+ and CD8+ T cells) and reduced T cell exhaustion (60). OBP-502 has also been evaluated in osteosarcoma preclinical models. This adenovirus reduced the viability of cancer cells and induced immunogenic cell death in vitro, whereas intratumoral injection in combination with an anti-PD-1 antibody in subcutaneous models reduced tumor growth and enhanced tumor-infiltrating CD8+ T cells (61).
Herpes Simplex Virus
HSV is among the largest DNA viruses developed for gene transfer. It is nonintegrative, very potent as a lytic virus, highly replicative and with high cell tropism (62). HSV type I G207, which contains deletions in both copies of the neurovirulence gene γ134.5 and a disabling lacZ insertion within the ICP6 gene (63), has been proven to be safe when injected into the cerebellum (64) and developing mouse brains (65). In preclinical studies, HSV-1 G207 and M002 (encoding human interleukin-12) demonstrated efficacy in pediatric high-grade glioma (66) and medulloblastoma (67). Another oncolytic herpes virus, HSV1716 (Seprehvir), showed efficacy in preclinical studies of high-grade gliomas and DMGs via changes in cytoskeletal dynamics and in molecular pathways related to cell polarity, migration, and movement (68). HSV-1 rRp450, which expresses the rat CYP2B1 enzyme and is able to activate the chemotherapeutic prodrug cyclophosphamide, prolonged overall survival in medulloblastoma and AT/RT, and its efficacy was further enhanced when cyclophosphamide was included in the treatment schedule (69).
In neuroblastoma models, HSV-1 M002 produced cell death in different neuroblastoma cell lines in vitro and reduced, alone and in combination with radiation, the tumor growth of this tumor in vivo (70). Similarly, a nestin-targeted oncolytic HSV also killed neuroblastoma tumor-initiating cells and prevented tumor formation in xenograft-bearing mice (71). FusOn-H2 (type 2 HSV), which specifically targets tumor cells with an aberrant Ras signaling pathway, exhibited efficacy in a syngeneic mouse model not only at the virus injection site but also at distant metastases (18).
In Ewing sarcoma, the second most common bone tumor in children and adolescents and a difficult to treat cancer (72), HSV-1 rRp450 was combined with macrophage reduction drugs. The combined treatment improved the efficacy of each agent alone and led to a reduction in M2-like macrophages in the tumor and spleen (73). HSV has also been tested in Rhabdomyosarcoma, the most common soft tissue sarcoma (74). Similar to other sarcomas, the chance of cure for metastatic and recurrent tumors is incredibly low (< 20%). In this context, HSV-1 M002 exhibited replication and oncolytic activity via apoptosis, reduced tumor growth and acted synergistically with radiation in subcutaneous mouse models (75). This virus has been additionally evaluated in serendipitous murine models of undifferentiated sarcoma, leading to an increase in effector CD4+ and CD8+ T cells, activated monocytes and a decrease in myeloid-derived suppressor cells (76).
Other Oncolytic Viruses
Parvovirus H-1 (H-1PV) is an apathogenic in humans and non-recombinant OV that occurs naturally in rats (77), and long-term survival was observed for adult high-grade gliomas mouse models after intratumoral, intravenous or intranasal virus application (78, 79). H-1PV showed efficacy in in vitro models of pediatric high-grade glioma (80), medulloblastoma (81) and Ewing sarcoma but failed to improve survival in vivo in this tumor (82).
Medulloblastoma and pleomorphic xanthoastrocytoma (a rare condition comprising <1% of all primary brain tumors) have been evaluated with PVSRIPO. PVSRIPO is an attenuated polio: rhinovirus chimera without neurovirulence and had been evaluated previously in adult recurrent glioblastoma patients with promising results (83). The authors observed that PVSRIPO could be used against these two malignancies in vitro (84). Other types of OVs have been used in preclinical models, such as a measles virus in AT/RT models (85) or the picornavirus Seneca Valley virus (SVV-001) in pediatric glioma (86), with an improvement observed in mice bearing tumor cells. Another study described the use of the oncolytic vesicular stomatitis virus VSVΔM51 and oncolytic myxoma virus (87) as treatments in vitro and in vivo with good responses, although the models used were subcutaneous.
A specific Semliki Forest virus (SFV4miRT), an Alphavirus belonging to the Togaviridae family and modified to reduce neurovirulence through insertion of three microRNAs, prolonged survival in neuroblastoma and glioblastoma mouse models with low interferon-α/β secretion (88). Another OV belonging to this family, Sindbis virus (SINV), exhibited efficacy in vitro via apoptosis, reduced tumor growth and extended overall survival in subcutaneous neuroblastoma models (89). An attenuated, nonneurovirulent poliovirus was evaluated and exhibited replication in neuroblastoma cells and significant reduction in tumor growth in subcutaneous tumor-bearing mice (90). Additionally, in another study, MV-CEA (an engineered measles virus) produced neuroblastoma cell death via apoptosis in vitro and extended the overall survival in xenograft models after five doses were injected intratumorally (91). Interestingly, treatment with an OV that expresses a CXCR4 antagonist injected intravenously augmented the efficacy of DC vaccines (92). Another oncolytic Seneca Valley virus (NTX-010) proved effective in a subcutaneous neuroblastoma and Ewing sarcoma models (93). Zika virus has been evaluated as an OV. Mazar and colleagues showed that neuroblastoma cells are widely permissive to Zika infection and require CD24, although the efficacy in cell death has not been proven (94).
Measles OV decreased tumor growth of subcutaneous xenografts and prolonged survival with orthotopic and pulmonary metastatic osteosarcoma tumors (95). Also in osteosarcoma, other viruses such as the previously mentioned parvovirus H-1PV (96), oncolytic vesicular stomatitis virus VSVΔM51 in combination with phosphoinositide 3-kinase inhibitor (97) and myxoma virus treated with immune checkpoint inhibitors (98) successfully demonstrated an antitumor effect. Interestingly, coculture of an Ewing sarcoma cell line with NK cells led to a better oncolytic effect of a measles OV (99).
The reovirus Reolysin exhibited efficacy in the treatment of osteosarcoma, Ewing sarcoma and rhabdomyosarcoma cell lines in vitro and in flank xenografts in vivo, in combination with radiotherapy or the chemotherapeutic cisplatin, injecting three doses every three weeks via tail vein administration (100). Phelps and collaborators developed a recombinant oncolytic myxoma virus engineered with CRIPSR/Cas9 gene editing capability targeting NRAS (Myx : NRAS). While nonmodified myxoma virus slightly improved the overall survival in rhabdomyosarcoma subcutaneous models, the clinical effect was improved greatly by using Myx : NRAS, and long-term survival was achieved (101). VSVΔM51 has also been evaluated in rhabdomyosarcoma and synergized with the Smac mimetic compound LCL161 in vitro and in vivo in a syngeneic murine model (102). In another elegant work, Petrov and colleagues used canine adipose-derived MSCs as carriers of vaccinia viruses as a “Trojan Horse” to circumvent an early immune attack to treat a canine soft tissue sarcoma (103).
Oncolytic Viruses in Clinical Trials
In this section, we recapitulate the latest updates from clinical trials using OVs as therapeutic agents in pediatric solid cancers. All clinical trials using OVs that have been conducted in pediatric populations are included in Table 1.
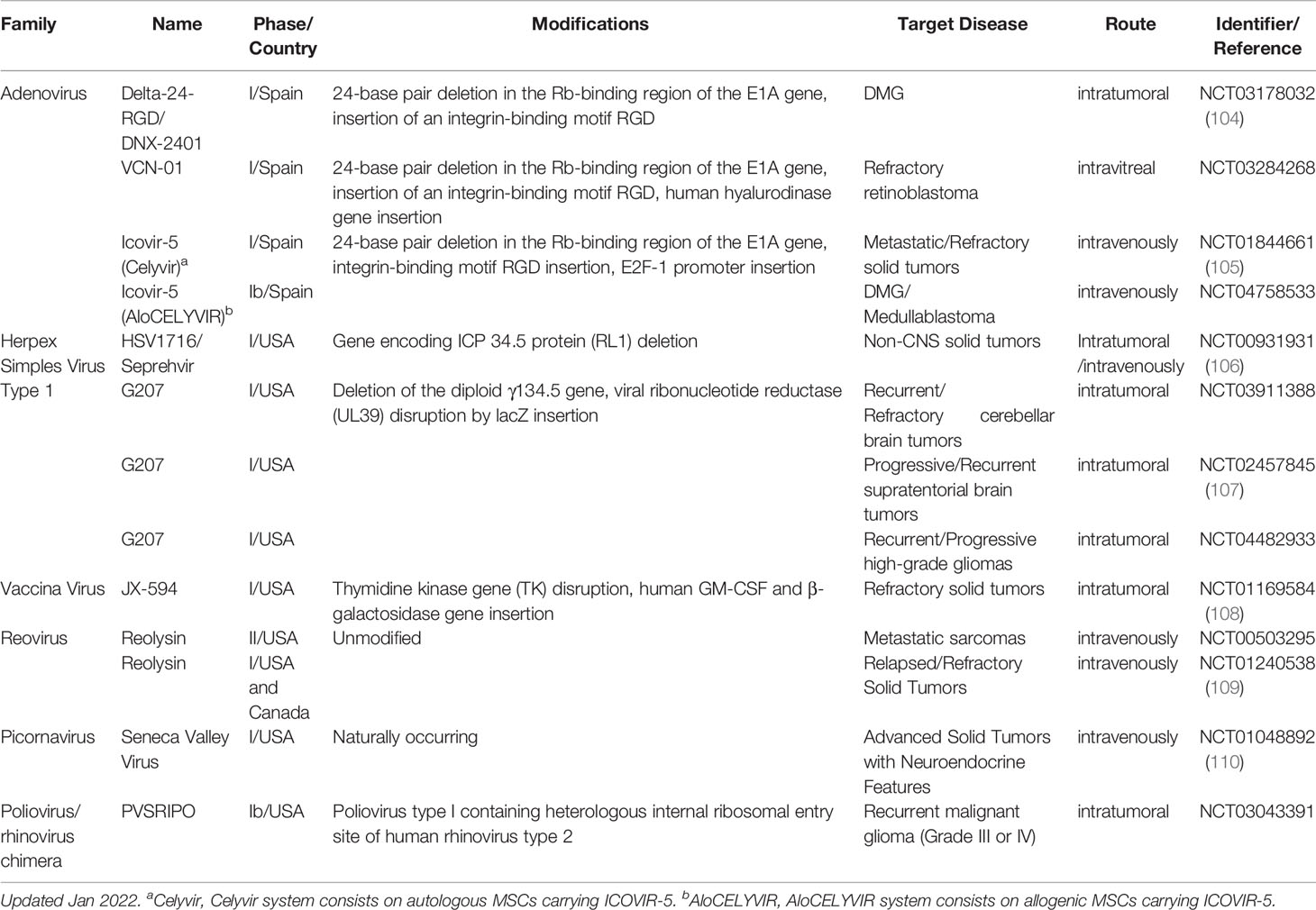
Table 1 Clinical trials, completed or active, using oncolytic viruses as treatment in pediatric solid tumors.
One of the first clinical approaches using an OV in the pediatric population was a case report published in 2006 (111). A 12-year-old boy with anaplastic astrocytoma who was subjected to conventional therapy (surgery, radiation and chemotherapy) and progressed was treated with MTH-68/H (attenuated strain of paramyxovirus NDV) as a compassionate use. MRI scans showed 30% tumor regression two months after viral infusion. However, the patient’s condition began to decline 4 months after the first MTH-68/H treatment due to the growth of new tumor nodules, and additional surgery was required. The patient passed away after 41 months.
Since then, several formal clinical trials utilizing different OVs have been performed, all of them looking into safety and feasibility. For example, Seneca Valley virus (NTX-010) was used in advanced solid tumors with neuroendocrine features in a phase I trial that included adults and children (112). Patients with neuroblastoma, rhabdomyosarcoma, Wilms tumor, carcinoid tumor and adrenocorticocarcinoma were included in this trial, which included two arms: one with just the OV and another with the virus combined with cyclophosphamide. Dose-limiting toxicity was observed in the first arm but not in the second. Adverse events of grade ≤3, such as leukopenia, neutropenia, nausea, or anemia, were described. Unfortunately, no complete or partial responses were observed, and only stable disease was observed in 6 out of 12 patients in the first arm (neuroblastoma n = 4, carcinoid tumor n=1; and rhabdomyosarcoma n=1) and 4 out of 6 in the second arm (neuroblastoma n = 2; and Wilms tumor n = 2).
JX-594 (Pexa-Vec; a vaccinia virus) was evaluated in six patients with metastatic neuroblastoma, hepatocellular carcinoma and Ewing sarcoma. No severe toxicity was associated with virus administration. Regarding efficacy, 4 out of 6 patients presented stable disease and 2 progressive disease, and uninjected lesions progressed in all patients except one, whose lung nodules were stable (108).
The oncolytic reovirus Reolysin was the drug chosen for another phase I clinical trial (109). Twenty-four children with relapsed or refractory extracranial solid tumors were administered Reolysin intravenously for 5 consecutive days every 28 days, alone or in combination with the chemotherapeutic cyclophosphamide. Adverse events of grade ≤4 and even grade 5 thromboembolism in one patient were described. Regarding efficacy, only three patients with stable disease received a second cycle, whereas two patients received a third cycle prior to progressive disease.
In a study published by Melen and collaborators, Celyvir was chosen for compassionate use for 13 patients with advanced refractory neuroblastoma. Children received weekly multidoses as a sole treatment. The only adverse effects found were mild and autolimited viral-related toxicities, and none of the patients experienced grade 3+ toxicities. Regarding clinical outcomes, progression was the most common (n=8), with stable disease (n=1), partial response (n=3) and complete response (n=1). The authors found that the nonresponder patients’ MSCs showed lower levels of expression of adhesion molecules and migration capacities, and a higher number of T cell lymphocytes was found in responder patients (113).
Our group has finished a phase I, dose-escalation clinical trial using DNX-2401 followed by standard radiotherapy in naïve DMGs, in which 12 patients were enrolled (114). Correct infusion of viral particles was checked using gadolinium in all patients (104). The treatment regimen was well tolerated, with asthenia, headache, vomiting, pyrexia and neurological deterioration being the most commonly reported adverse events. The three serious adverse events reported were grade 3 abdominal pain, grade 3 lymphopenia and grade 3 clinical deterioration. Regarding efficacy, tumor reduction was observed in 9 out of 12 patients. The final report of this study is still pending. The preclinical studies mentioned above have allowed the transfer of HSV-1 G207 in a phase I clinical trial to treat pediatric high-grade gliomas, in which supratentorial tumors had, at recurrence, a median life expectancy of only 5.6 months. The injection was performed via catheterization, and dose-limiting toxicities in the 12 patients enrolled were classified as grade 1 and 2 (more common) and 3 (less common) related to HSV-1 G207. The median overall survival was 12.2 months, and 4 patients were still alive 18 months after HSV-1 G207 injection. Importantly, posttreatment tissues from patients showed a substantial increase in CD3+, CD4+ and CD8+ T cells (107).
The oncolytic herpes virus HSV1716, mentioned above in preclinical research, has been evaluated in a preclinical trial for the pediatric population (115). In this study, Streby et al. recruited nine patients with relapsed or refractory extracranial solid tumors (pediatric sarcomas, neuroblastoma and cholangiocarcinoma). No dose-limiting toxicities were observed, and all the adverse events were of grade ≤ 3. However, only two patients exhibited disease stabilization in response to the virus, and months later, the tumors started to progress (106).
Perspective and Concluding Remarks
As depicted along this review, OVs have exhibited potential applications in the treatment of pediatric solid tumors with encouraging results. However, OVs have been regularly evaluated in tumors whose survival has not improved much in recent decades (including pediatric cancers). Despite the great efforts and multitude of clinical trials carried out, these diseases continue to be devastating. This constitutes a double-edged sword for OVs. On the one hand, they offer an alternative for cancer patients for whom the currently available treatments do not lead to sufficient improvement, much less cure, of the pathology. On the other hand, all of these tumors are very aggressive, and there could be no room to obtain tangible improvement, at least with monotherapy. Notably, although OVs have shown marked antitumor effects in preclinical models, their clinical translation has not been so successful. Indeed, most of the clinical trials recapitulated the above recruitment of patients in their last stages of their diseases and therefore with modest results. Nevertheless, in recent years, combinations of OVs with several chemo- and immunotherapy regimens have been proposed, and preclinical research and clinical trials are currently being conducted that show benefit compared to OVs alone (116–119).
Another point in favor of OVs is their low toxicity and extremely safe profile with very few secondary effects. This makes them very attractive, especially in the pediatric population. Viral production is also not a problem, as these viruses are easy and affordable to manufacture.
We consider that the main disadvantage of OVs is the route of administration. In intravascular administration, OVs are recognized and inactivated by humoral components of the innate and adaptative immune system in the blood (120), so this route is frequently dismissed. Therefore, intratumoral delivery is the dominant route, which allows direct targeting of the tumor using simple clinical procedures. However, there are also some difficulties, such as the presence of tissue barriers that might prevent the spread of the virus or the existence of metastases which might compromise the oncolytic efficacy. For that reason, approaches that improve virus delivery are under investigation and will be key to the further development of the field. In that regard, the use of cellular carriers such as MSCs (as explained above) (46, 52, 60, 103, 113), protective coatings and genetic modifications of OVs are other strategies that are considered for delivery optimization (121).
Interpretation of virotherapy responses through imaging within the clinical trial is another cornerstone where numerous efforts are being allocated. It is imperative to understand better what the imaging is telling us and define parameters that allow us to identify responses and other biological parameters intrinsic to the treatment with OVs.
In closing, the establishment of OVs as a therapeutic option for the treatment of tumors with poor prognosis, including pediatric solid malignancies, is encouraging. In recent years, investigations using OVs as therapy have grown exponentially. The FDA approval of Talimogene Laherparepvec has demonstrated that OVs are actually being considered as therapeutic options. Although we still need to overcome some barriers regarding OV application, their feasibility and, on some occasions, efficacy in treatment have been demonstrated. Further efforts will be needed and, given that virotherapy is now in its adolescence, there is great room for optimization. In the short-middle term, we believe that OVs will constitute a feasible therapeutic option to use alone or in combination with other strategies, for patients with pediatric solid tumors.
Author Contributions
DN: Conceptualization, writing, review, editing and figure design. KS: Writing and figure design. MA: Conceptualization, supervision, writing, review and editing. All authors contributed to the article and approved the submitted version.
Funding
The performed work was supported through a Predoctoral Fellowship from Instituto de Salud Carlos III (FI20/00020 DN), Instituto de Salud Carlos III y Fondos Feder (PI19/01896 MA); Fundación La Caixa (MA); Fundación El sueño de Vicky, Asociación Pablo Ugarte-Fuerza Julen, Fundación ADEY, Fundación ACS, (MA); Department of Defense (DOD) Team Science Award undergrant (CA 160525 MA); ChadTough Defeat DIPG Foundation (MA) and AECC (PRYGN21937ALON MA). This project also received funding from the European Research Council (ERC) under the European Union´s Horizon 2020 Research and Innovation Programme (817884 ViroPedTher to MA).
Conflict of Interest
MA has a research grant from DNAtrix not related to this work.
The remaining authors declare that the research was conducted in the absence of any commercial or financial relationships that could be construed as a potential conflict of interest.
Publisher’s Note
All claims expressed in this article are solely those of the authors and do not necessarily represent those of their affiliated organizations, or those of the publisher, the editors and the reviewers. Any product that may be evaluated in this article, or claim that may be made by its manufacturer, is not guaranteed or endorsed by the publisher.
Acknowledgments
We want to thank the patients’ and their family for their selfless contribution of the advancement of science. We apologize to all the colleagues that have not been cited due to the limited extension.
References
1. Steliarova-Foucher E, Colombet M, Ries LAG, Moreno F, Dolya A, Bray F, et al. International Incidence of Childhood Cancer, 2001–10: A Population-Based Registry Study. Lancet Oncol (2017) 18:719–31. doi: 10.1016/S1470-2045(17)30186-9
2. World Health Organization. CureAll Framework: WHO Global Initiative for Childhood Cancer: Increasing Access, Advancing Quality, Saving Lives. Lyon (France): IARC Press (2021).
3. Steliarova-Foucher E, Stiller C, Kaatsch P, Berrino F, Coebergh JW, Lacour B, et al. Geographical Patterns and Time Trends of Cancer Incidence and Survival Among Children and Adolescents in Europe Since the 1970s (the ACCIS Project): An Epidemiological Study. Lancet (2004) 364:2097–105. doi: 10.1016/S0140-6736(04)17550-8
4. Hudson MM, Link MP, Simone JV. Milestones in the Curability of Pediatric Cancers. J Clin Oncol (2014) 32:2391–7. doi: 10.1200/JCO.2014.55.6571
5. Pritchard-Jones K, Pieters R, Reaman GH, Hjorth L, Downie P, Calaminus G, et al. Sustaining Innovation and Improvement in the Treatment of Childhood Cancer: Lessons From High-Income Countries. Lancet Oncol (2013) 14:e95–e103. doi: 10.1016/S1470-2045(13)70010-X
6. Kattner P, Strobel H, Khoshnevis N, Grunert M, Bartholomae S, Pruss M, et al. Compare and Contrast: Pediatric Cancer Versus Adult Malignancies. Cancer Metastasis Rev (2019) 38:673–82. doi: 10.1007/s10555-019-09836-y
7. June CH, O’Connor RS, Kawalekar OU, Ghassemi S, Milone MC. CAR T Cell Immunotherapy for Human Cancer. Science (2018) 359:1361–5. doi: 10.1126/science.aar6711
8. Larson RC, Maus MV. Recent Advances and Discoveries in the Mechanisms and Functions of CAR T Cells. Nat Rev Cancer (2021) 21:145–61. doi: 10.1038/s41568-020-00323-z
9. Dock G. The Influence of Compliting Disease Upon Leukaemia. Am J Med Sci (1904) 127:563–92. doi: 10.1097/00000441-190412740-00001
10. Pelner L, Fowler GA, Nauts HC. Effects of Concurrent Infections and Their Toxins on the Course of Leukemia. Acta Med Scand Suppl (1958) 338:1–47. doi: 10.1111/j.0954-6820.1958.tb17327.x
11. Bierman HR, Crile DM, Dod KS, Kelly KH, Petrakis NI, White LP, et al. Remissions in Leukemia of Childhood Following Acute Infectious Disease. Staphylococcus and Streptococcus, Varicella, and Feline Panleukopenias. Cancer (1953) 6:591–605. doi: 10.1002/1097-0142(195305)6:3<591::AID-NCR2820060317>3.0.CO;2-M
12. Kelly E, Russell SJ. History of Oncolytic Viruses: Genesis to Genetic Engineering. Mol Ther (2007) 15:651–9. doi: 10.1038/sj.mt.6300108
13. Sheridan C. First Oncolytic Virus Edges Towards Approval in Surprise Vote. Nat Biotechnol (2015) 33:569–70. doi: 10.1038/nbt0615-569
14. Chaurasiya S, Chen NG, Fong Y. Oncolytic Viruses and Immunity. Curr Opin Immunol (2018) 51:83–90. doi: 10.1016/j.coi.2018.03.008
15. Kaufman HL, Kohlhapp FJ, Zloza A. Oncolytic Viruses: A New Class of Immunotherapy Drugs. Nat Rev Drug Discov (2015) 14:642–62. doi: 10.1038/nrd4663
16. Shi T, Song X, Wang Y, Liu F, Wei J. Combining Oncolytic Viruses With Cancer Immunotherapy: Establishing a New Generation of Cancer Treatment. Front Immunol (2020) 11:683. doi: 10.3389/fimmu.2020.00683
17. Raja J, Ludwig JM, Gettinger SN, Schalper KA, Kim HS. Oncolytic Virus Immunotherapy: Future Prospects for Oncology. J Immunother Cancer (2018) 6:1–13. doi: 10.1186/s40425-018-0458-z
18. Li H, Dutuor A, Tao L, Fu X, Zhang X. Virotherapy With a Type 2 Herpes Simplex Virus-Derived Oncolytic Virus Induces Potent Antitumor Immunity Against Neuroblastoma. Clin Cancer Res (2007) 13:316–22. doi: 10.1158/1078-0432.CCR-06-1625
19. Marchini A, Daeffler L, Pozdeev VI, Angelova A, Rommelaere J. Immune Conversion of Tumor Microenvironment by Oncolytic Viruses: The Protoparvovirus H-1PV Case Study. Front Immunol (2019) 10:1848. doi: 10.3389/fimmu.2019.01848
20. Mondal M, Guo J, He P, Zhou D. Recent Advances of Oncolytic Virus in Cancer Therapy. Hum Vaccines Immunother (2020) 16:2389–402. doi: 10.1080/21645515.2020.1723363
21. Macedo N, Miller DM, Haq R, Kaufman HL. Clinical Landscape of Oncolytic Virus Research in 2020. J Immunother Cancer (2020) 8:e001486. doi: 10.1136/jitc-2020-001486
22. Peters C, Rabkin SD. Designing Herpes Viruses as Oncolytics. Mol Ther - Oncol (2015) 2:15010. doi: 10.1038/mto.2015.10
23. Parato KA, Senger D, Forsyth PAJ, Bell JC. Recent Progress in the Battle Between Oncolytic Viruses and Tumours. Nat Rev Cancer (2005) 5:965–76. doi: 10.1038/nrc1750
24. Wang G, Kang X, Chen KS, Jehng T, Jones L, Chen J, et al. An Engineered Oncolytic Virus Expressing PD-L1 Inhibitors Activates Tumor Neoantigen-Specific T Cell Responses. Nat Commun (2020) 11:1–14. doi: 10.1038/s41467-020-15229-5
25. El-Shemi AG, Ashshi AM, Na Y, Li Y, Basalamah M, Al-Allaf FA, et al. Combined Therapy With Oncolytic Adenoviruses Encoding TRAIL and IL-12 Genes Markedly Suppressed Human Hepatocellular Carcinoma Both In Vitro and in an Orthotopic Transplanted Mouse Model. J Exp Clin Cancer Res (2016) 35:1–16. doi: 10.1186/s13046-016-0353-8
26. Chiocca EA, Rabkin SD. Oncolytic Viruses and Their Application to Cancer Immunotherapy. Cancer Immunol Res (2014) 2:295–300. doi: 10.1158/2326-6066.CIR-14-0015
27. Hartley A, Kavishwar G, Salvato I, Marchini A. A Roadmap for the Success of Oncolytic Parvovirus-Based Anticancer Therapies. Annu Rev Virol (2020) 7:537–57. doi: 10.1146/annurev-virology-012220-023606
28. Harrington K, Freeman DJ, Kelly B, Harper J, Soria JC. Optimizing Oncolytic Virotherapy in Cancer Treatment. Nat Rev Drug Discov (2019) 18:689–706. doi: 10.1038/s41573-019-0029-0
29. Ostrom QT, Gittleman H, De Blank PM, Finlay JL, Gurney JG, McKean-Cowdin R, et al. American Brain Tumor Association Adolescent and Young Adult Primary Brain and Central Nervous System Tumors Diagnosed in the United States in 2008-2012. Neuro Oncol (2015) 18:i1–i50. doi: 10.1093/neuonc/nov297
30. World Health Organization Classification of Tumours Editorial Board. World Health Organization Classification of Tumours of the Central Nervous System, 5th Edition. Lyon (France): IARC Press (2022).
31. Louis DN, Perry A, Wesseling P, Brat DJ, Cree IA, Figarella-Branger D, et al. The 2021 WHO Classification of Tumors of the Central Nervous System: A Summary. Neuro Oncol (2021) 23:1231–51. doi: 10.1093/neuonc/noab106
32. Pfister SM, Reyes-Múgica M, Chan JKC, Hasle H, Lazar AJ, Rossi S, et al. A Summary of the Inaugural WHO Classification of Pediatric Tumors: Transitioning From the Optical Into the Molecular Era. Cancer Discov (2022) 12:331–55. doi: 10.1158/2159-8290.cd-21-1094
33. Suzuki K, Fueyo J, Krasnykh V, Reynolds PN, Curiel DT, Alemany R. A Conditionally Replicative Adenovirus With Enhanced Infectivity Shows Improved Oncolytic Potency. Clin Cancer Res (2001) 7:120–6.
34. Fueyo J, Alemany R, Gomez-Manzano C, Fuller GN, Khan A, Conrad CA, et al. Preclinical Characterization of the Antiglioma Activity of a Tropism-Enhanced Adenovirus Targeted to the Retinoblastoma Pathway. J Natl Cancer Inst (2003) 95:652–60. doi: 10.1093/jnci/95.9.652
35. Ene CI, Fueyo J, Lang FF. Delta-24 Adenoviral Therapy for Glioblastoma: Evolution From the Bench to Bedside and Future Considerations. Neurosurg Focus (2021) 50:1–6. doi: 10.3171/2020.11.FOCUS20853
36. Alonso MM, Jiang H, Yokoyama T, Xu J, Bekele NB, Lang FF, et al. Delta-24-RGD in Combination With RAD001 Induces Enhanced Anti-Glioma Effect via Autophagic Cell Death. Mol Ther (2008) 16:487–93. doi: 10.1038/sj.mt.6300400
37. Jiang H, Clise-Dwyer K, Ruisaard KE, Fan X, Tian W, Gumin J, et al. Delta-24-RGD Oncolytic Adenovirus Elicits Anti-Glioma Immunity in an Immunocompetent Mouse Model. PloS One (2014) 9:e97407. doi: 10.1371/journal.pone.0097407
38. Lang FF, Conrad C, Gomez-Manzano C, Alfred Yung WK, Sawaya R, Weinberg JS, et al. Phase I Study of DNX-2401 (Delta-24-RGD) Oncolytic Adenovirus: Replication and Immunotherapeutic Effects in Recurrent Malignant Glioma. J Clin Oncol (2018) 36:1419–27. doi: 10.1200/JCO.2017.75.8219
39. Martínez-Vélez N, Garcia-Moure M, Marigil M, González-Huarriz M, Puigdelloses M, Gallego Pérez-Larraya J, et al. The Oncolytic Virus Delta-24-RGD Elicits an Antitumor Effect in Pediatric Glioma and DIPG Mouse Models. Nat Commun (2019) 10:1–10. doi: 10.1038/s41467-019-10043-0
40. Martinez-Velez N, Marigil M, García-Moure M, Gonzalez-Huarriz M, Aristu JJ, Ramos-García LI, et al. Delta-24-RGD Combined With Radiotherapy Exerts a Potent Antitumor Effect in Diffuse Intrinsic Pontine Glioma and Pediatric High Grade Glioma Models. Acta Neuropathol Commun (2019) 7:1–12. doi: 10.1186/s40478-019-0714-6
41. Gajjar A, Bowers DC, Karajannis MA, Leary S, Witt H, Gottardo NG. Pediatric Brain Tumors: Innovative Genomic Information Is Transforming the Diagnostic and Clinical Landscape. J Clin Oncol (2015) 33:2986–98. doi: 10.1200/JCO.2014.59.9217
42. Ostrom QT, Chen Y, De Blank PM, Ondracek A, Farah P, Gittleman H, et al. The Descriptive Epidemiology of Atypical Teratoid/Rhabdoid Tumors in the United States, 2001-2010. Neuro Oncol (2014) 16:1392–9. doi: 10.1093/neuonc/nou090
43. Garcia-Moure M, Gonzalez-Huarriz M, Labiano S, Guruceaga E, Bandres E, Zalacain M, et al. Delta-24-RGD, an Oncolytic Adenovirus, Increases Survival and Promotes Proinflammatory Immune Landscape Remodeling in Models of AT/RT and CNS-PNET. Clin Cancer Res (2021) 27:1807–20. doi: 10.1158/1078-0432.CCR-20-3313
44. Rodríguez-García A, Giménez-Alejandre M, Rojas JJ, Moreno R, Bazan-Peregrino M, Cascalló M, et al. Safety and Efficacy of VCN-01, an Oncolytic Adenovirus Combining Fiber HSG-Binding Domain Replacement With RGD and Hyaluronidase Expression. Clin Cancer Res (2015) 21:1406–18. doi: 10.1158/1078-0432.CCR-14-2213
45. Garcia-Moure M, Martinez-Velez N, Gonzalez-Huarriz M, Marrodán L, Cascallo M, Alemany R, et al. The Oncolytic Adenovirus VCN-01 Promotes Anti-Tumor Effect in Primitive Neuroectodermal Tumor Models. Sci Rep (2019) 9:1–10. doi: 10.1038/s41598-019-51014-1
46. Chastkofsky MI, Pituch KC, Katagi H, Zannikou M, Ilut L, Xiao T, et al. Mesenchymal Stem Cells Successfully Deliver Oncolytic Virotherapy to Diffuse Intrinsic Pontine Glioma. Clin Cancer Res (2021) 27:1766–77. doi: 10.1158/1078-0432.CCR-20-1499
47. Arnone CM, Polito VA, Mastronuzzi A, Carai A, Diomedi FC, Antonucci L, et al. Oncolytic Adenovirus and Gene Therapy With EphA2-BiTE for the Treatment of Pediatric High-Grade Gliomas. J Immunother Cancer (2021) 9:e001930. doi: 10.1136/jitc-2020-001930
48. Matthay KK, Maris JM, Schleiermacher G, Nakagawara A, Mackall CL, Diller L, et al. Neuroblastoma. Nat Rev Dis Prim (2016) 2:16078. doi: 10.1038/nrdp.2016.78
49. Bowen KA, Chung DH. Recent Advances in Neuroblastoma. Curr Opin Pediatr (2009) 21:350–6. doi: 10.1097/MOP.0b013e32832b1240
50. Maris JM, Hogarty MD, Bagatell R, Cohn SL. Neuroblastoma. Lancet (2007) 369:2106–20. doi: 10.1016/S0140-6736(07)60983-0
51. Tanimoto T, Tazawa H, Ieda T, Nouso H, Tani M, Oyama T, et al. Elimination of MYCN-Amplified Neuroblastoma Cells by Telomerase-Targeted Oncolytic Virus via MYCN Suppression. Mol Ther - Oncol (2020) 18:14–23. doi: 10.1016/j.omto.2020.05.015
52. Franco-Luzón L, González-Murillo Á, Alcántara-Sánchez C, García-García L, Tabasi M, Huertas AL, et al. Systemic Oncolytic Adenovirus Delivered in Mesenchymal Carrier Cells Modulate Tumor Infiltrating Immune Cells and Tumor Microenvironment in Mice With Neuroblastoma. Oncotarget (2020) 11:347–61. doi: 10.18632/oncotarget.27401
53. Tejada FNH, Zamudio A, Marques-Piubelli ML, Cuglievan B, Harrison D. Advances in the Management of Pediatric Sarcomas. Curr Oncol Rep (2021) 23:1–9. doi: 10.21037/tau-20-480
54. Hingorani P, Janeway K, Crompton BD, Kadoch C, Mackall CL, Khan J, et al. Current State of Pediatric Sarcoma Biology and Opportunities for Future Discovery: A Report From the Sarcoma Translational Research Workshop. Cancer Genet (2016) 209:182–94. doi: 10.1016/j.cancergen.2016.03.004
55. Collins M, Wilhelm M, Conyers R, Herschtal A, Whelan J, Bielack S, et al. Benefits and Adverse Events in Younger Versus Older Patients Receiving Neoadjuvant Chemotherapy for Osteosarcoma: Findings From a Meta-Analysis. J Clin Oncol (2013) 31:2303–12. doi: 10.1200/JCO.2012.43.8598
56. Roberts SS, Chou AJ, Cheung NKV. Immunotherapy of Childhood Sarcomas. Front Oncol (2015) 5:181. doi: 10.3389/fonc.2015.00181
57. Martinez-Velez N, Xipell E, Jauregui P, Zalacain M, Marrodan L, Zandueta C, et al. The Oncolytic Adenovirus Δ24-RGD in Combination With Cisplatin Exerts a Potent Anti-Osteosarcoma Activity. J Bone Miner Res (2014) 29:2287–96. doi: 10.1002/jbmr.2253
58. Martínez-Vélez N, Xipell E, Vera B, de la Rocha AA, Zalacain M, Marrodan L, et al. The Oncolytic Adenovirus VCN-01 as Therapeutic Approach Against Pediatric Osteosarcoma. Clin Cancer Res (2016) 22:2217–25. doi: 10.1158/1078-0432.CCR-15-1899
59. Martínez-Vélez N, Laspidea V, Zalacain M, Labiano S, Garcia-Moure M, Puigdelloses M, et al. Local Treatment of a Pediatric Osteosarcoma Model With a 4-1BBL Armed Oncolytic Adenovirus Results in an Antitumor Effect and Leads to Immune Memory. Mol Cancer Ther (2021) 21(3):471–80. doi: 10.1158/1535-7163.mct-21-0565
60. Morales-Molina A, Gambera S, Leo A, García-Castro J. Combination Immunotherapy Using G-CSF and Oncolytic Virotherapy Reduces Tumor Growth in Osteosarcoma. J Immunother Cancer (2021) 9:1–13. doi: 10.1136/jitc-2020-001703
61. Mochizuki Y, Tazawa H, Demiya K, Kure M, Kondo H, Komatsubara T, et al. Telomerase-Specific Oncolytic Immunotherapy for Promoting Efficacy of PD-1 Blockade in Osteosarcoma. Cancer Immunol Immunother (2021) 70:1405–17. doi: 10.1007/s00262-020-02774-7
62. Cripe TP, Chen CY, Denton NL, Haworth KB, Hutzen B, Leddon JL, et al. Pediatric Cancer Gone Viral. Part I: Strategies for Utilizing Oncolytic Herpes Simplex Virus-1 in Children. Mol Ther - Oncol (2015) 2:15015. doi: 10.1038/mto.2015.15
63. Rabkin SD, Martuza RL, Manz HJ, Yazaki T. Treatment of Human Malignant Meningiomas by G207, a Replication-Competent Multimutated Herpes Simplex Virus 1. Cancer Res (1995) 55:4752–6.
64. Bernstock JD, Vicario N, Li R, Nan L, Totsch SK, Schlappi C, et al. Safety and Efficacy of Oncolytic HSV-1 G207 Inoculated Into the Cerebellum of Mice. Cancer Gene Ther (2020) 27:246–55. doi: 10.1038/s41417-019-0091-0
65. Radbill AE, Reddy AT, Markert JM, Wyss JM, Pike MM, Akella NS, et al. Effects of G207, a Conditionally Replication-Competent Oncolytic Herpes Simplex Virus, on the Developing Mammalian Brain. J Neurovirol (2007) 13:118–29. doi: 10.1080/13550280601187177
66. Friedman GK, Bernstock JD, Chen D, Nan L, Moore BP, Kelly VM, et al. Enhanced Sensitivity of Patient-Derived Pediatric High-Grade Brain Tumor Xenografts to Oncolytic HSV-1 Virotherapy Correlates With Nectin-1 Expression. Sci Rep (2018) 8:1–10. doi: 10.1038/s41598-018-32353-x
67. Friedman GK, Moore BP, Nan L, Kelly VM, Etminan T, Langford CP, et al. Pediatric Medulloblastoma Xenografts Including Molecular Subgroup 3 and CD133+ and CD15+ Cells are Sensitive to Killing by Oncolytic Herpes Simplex Viruses. Neuro Oncol (2016) 18:227–35. doi: 10.1093/neuonc/nov123
68. Cockle JV, Brüning-Richardson A, Scott KJ, Thompson J, Kottke T, Morrison E, et al. Oncolytic Herpes Simplex Virus Inhibits Pediatric Brain Tumor Migration and Invasion. Mol Ther - Oncol (2017) 5:75–86. doi: 10.1016/j.omto.2017.04.002
69. Studebaker AW, Hutzen BJ, Pierson CR, Haworth KB, Cripe TP, Jackson EM, et al. Oncolytic Herpes Virus Rrp450 Shows Efficacy in Orthotopic Xenograft Group 3/4 Medulloblastomas and Atypical Teratoid/Rhabdoid Tumors. Mol Ther - Oncol (2017) 6:22–30. doi: 10.1016/j.omto.2017.05.005
70. Gillory LA, Megison ML, Stewart JE, Mroczek-Musulman E, Nabers HC, Waters AM, et al. Preclinical Evaluation of Engineered Oncolytic Herpes Simplex Virus for the Treatment of Neuroblastoma. PloS One (2013) 8:1–19. doi: 10.1371/journal.pone.0077753
71. Mahller YY, Williams JP, Baird WH, Mitton B, Grossheim J, Saeki Y, et al. Neuroblastoma Cell Lines Contain Pluripotent Tumor Initiating Cells That Are Susceptible to a Targeted Oncolytic Virus. PloS One (2009) 4:e4235. doi: 10.1371/journal.pone.0004235
72. Balamuth NJ, Womer RB. Ewing’s Sarcoma. Lancet Oncol (2010) 11:184–92. doi: 10.1016/S1470-2045(09)70286-4
73. Denton NL, Chen CY, Hutzen B, Currier MA, Scott T, Nartker B, et al. Myelolytic Treatments Enhance Oncolytic Herpes Virotherapy in Models of Ewing Sarcoma by Modulating the Immune Microenvironment. Mol Ther - Oncol (2018) 11:62–74. doi: 10.1016/j.omto.2018.10.001
74. Skapek SX, Ferrari A, Gupta AA, Lupo PJ, Butler E, Shipley J, et al. Rhabdomyosarcoma. Nat Rev Dis Prim (2019) 5:14–6. doi: 10.1038/s41572-018-0051-2
75. Waters AM, Stafman LL, Garner EF, Mruthyunjayappa S, Stewart JE, Friedman GK, et al. Effect of Repeat Dosing of Engineered Oncolytic Herpes Simplex Virus on Preclinical Models of Rhabdomyosarcoma. Transl Oncol (2016) 9:419–30. doi: 10.1016/j.tranon.2016.07.008
76. Ring EK, Li R, Moore BP, Nan L, Kelly VM, Han X, et al. Newly Characterized Murine Undifferentiated Sarcoma Models Sensitive to Virotherapy With Oncolytic HSV-1 M002. Mol Ther - Oncol (2017) 7:27–36. doi: 10.1016/j.omto.2017.09.003
77. Rommelaere J, Geletneky K, Angelova AL, Daeffler L, Dinsart C, Kiprianova I, et al. Oncolytic Parvoviruses as Cancer Therapeutics. Cytokine Growth Factor Rev (2010) 21:185–95. doi: 10.1016/j.cytogfr.2010.02.011
78. Geletneky K, Kiprianova I, Ayache A, Koch R, Herrero M, Deleu L, et al. Regression of Advanced Rat and Human Gliomas by Local or Systemic Treatment With Oncolytic Parvovirus H-1 in Rat Models. Neuro Oncol (2010) 12:804–14. doi: 10.1093/neuonc/noq023
79. Kiprianova I, Thomas N, Ayache A, Fischer M, Leuchs B, Klein M, et al. Regression of Glioma in Rat Models by Intranasal Application of Parvovirus H-1. Clin Cancer Res (2011) 17:5333–42. doi: 10.1158/1078-0432.CCR-10-3124
80. Josupeit R, Bender S, Kern S, Leuchs B, Hielscher T, Herold-Mende C, et al. Pediatric and Adult High-Grade Glioma Stem Cell Culture Models Are Permissive to Lytic Infection With Parvovirus H-1. Viruses (2016) 8:138. doi: 10.3390/v8050138
81. Lacroix J, Schlund F, Leuchs B, Adolph K, Sturm D, Bender S, et al. Oncolytic Effects of Parvovirus H-1 in Medulloblastoma Are Associated With Repression of Master Regulators of Early Neurogenesis. Int J Cancer (2014) 134:703–16. doi: 10.1002/ijc.28386
82. Lacroix J, Kis Z, Josupeit R, Schlund F, Stroh-Dege A, Frank-Stöhr M, et al. Preclinical Testing of an Oncolytic Parvovirus in Ewing Sarcoma: Protoparvovirus H-1 Induces Apoptosis and Lytic InfectionIn Vitro But Fails to Improve Survival In Vivo. Viruses (2018) 10:1–15. doi: 10.3390/v10060302
83. Desjardins A, Gromeier M, Herndon JE, Beaubier N, Bolognesi DP, Friedman AH, et al. Recurrent Glioblastoma Treated With Recombinant Poliovirus. N Engl J Med (2018) 379:150–61. doi: 10.1056/nejmoa1716435
84. Thompson EM, Brown M, Dobrikova E, Ramaswamy V, Taylor MD, McLendon R, et al. Poliovirus Receptor (CD155) Expression in Pediatric Brain Tumors Mediates Oncolysis of Medulloblastoma and Pleomorphic Xanthoastrocytoma. J Neuropathol Exp Neurol (2018) 77:696–702. doi: 10.1093/jnen/nly045
85. Studebaker AW, Hutzen B, Pierson CR, Shaffer TA, Raffel C, Jackson EM. Oncolytic Measles Virus Efficacy in Murine Xenograft Models of Atypical Teratoid Rhabdoid Tumors. Neuro Oncol (2015) 17:1568–77. doi: 10.1093/neuonc/nov058
86. Liu Z, Zhao X, Mao H, Baxter PA, Huang Y, Yu L, et al. Intravenous Injection of Oncolytic Picornavirus SVV-001 Prolongs Animal Survival in a Panel of Primary Tumor-Based Orthotopic Xenograft Mouse Models of Pediatric Glioma. Neuro Oncol (2013) 15:1173–85. doi: 10.1093/neuonc/not065
87. Wu Y, Lun X, Zhou H, Wang L, Sun B, Bell JC, et al. Oncolytic Efficacy of Recombinant Vesicular Stomatitis Virus and Myxoma Virus in Experimental Models of Rhabdoid Tumors. Clin Cancer Res (2008) 14:1218–27. doi: 10.1158/1078-0432.CCR-07-1330
88. Ramachandran M, Yu D, Dyczynski M, Baskaran S, Zhang L, Lulla A, et al. Safe and Effective Treatment of Experimental Neuroblastoma and Glioblastoma Using Systemically Delivered Triple Microrna-Detargeted Oncolytic Semliki Forest Virus. Clin Cancer Res (2017) 23:1519–30. doi: 10.1158/1078-0432.CCR-16-0925
89. Takenouchi A, Saito K, Saito E, Saito T, Hishiki T, Matsunaga T, et al. Oncolytic Viral Therapy for Neuroblastoma Cells With Sindbis Virus AR339 Strain. Pediatr Surg Int (2015) 31:1151–9. doi: 10.1007/s00383-015-3784-y
90. Toyoda H, Yin J, Mueller S, Wimmer E, Cello J. Oncolytic Treatment and Cure of Neuroblastoma by a Novel Attenuated Poliovirus in a Novel Poliovirus-Susceptible Animal Model. Cancer Res (2007) 67:2857–64. doi: 10.1158/0008-5472.CAN-06-3713
91. Zhang SC, Cai WS, Zhang Y, Jiang KL, Zhang KR, Wang WL. Engineered Measles Virus Edmonston Strain Used as a Novel Oncolytic Viral System Against Human Neuroblastoma Through a CD46 and Nectin 4-Independent Pathway. Cancer Lett (2012) 325:227–37. doi: 10.1016/j.canlet.2012.07.008
92. Komorowski M, Tisonczyk J, Kolakowska A, Drozdz R, Kozbor D. Modulation of the Tumor Microenvironment by CXCR4 Antagonist-Armed Viral Oncotherapy Enhances the Antitumor Efficacy of Dendritic Cell Vaccines Against Neuroblastoma in Syngeneic Mice. Viruses (2018) 10:1–16. doi: 10.3390/v10090455
93. Morton CL, Houghton PJ, Kolb EA, Gorlick R, Reynolds CP, Kang MH, et al. Initial Testing of the Replication Competent Seneca Valley Virus (NTX-010) by the Pediatric Preclinical Testing Program. Pediatr Blood Cancer (2010) 55:295–303. doi: 10.1002/pbc.22535
94. Mazar J, Li Y, Rosado A, Phelan P, Kedarinath K, Parks GD, et al. Zika Virus as an Oncolytic Treatment of Human Neuroblastoma Cells Requires CD24. PloS One (2018) 13:1–21. doi: 10.1371/journal.pone.0200358
95. Domingo-Musibay E, Allen C, Kurokawa C, Hardcastle JJ, Aderca I, Msaouel P, et al. Measles Edmonston Vaccine Strain Derivatives Have Potent Oncolytic Activity Against Osteosarcoma. Cancer Gene Ther (2014) 21:483–90. doi: 10.1038/cgt.2014.54
96. Geiss C, Kis Z, Leuchs B, Frank-Stöhr M, Schlehofer JR, Rommelaere J, et al. Preclinical Testing of an Oncolytic Parvovirus: Standard Protoparvovirus H-1PV Efficiently Induces Osteosarcoma Cell Lysis In Vitro. Viruses (2017) 9:301. doi: 10.3390/v9100301
97. Jiang J, Wang W, Xiang W, Jiang L, Zhou Q. The Phosphoinositide 3-Kinase Inhibitor ZSTK474 Increases the Susceptibility of Osteosarcoma Cells to Oncolytic Vesicular Stomatitis Virus VSVΔ51 via Aggravating Endoplasmic Reticulum Stress. Bioengineered (2021) 12:11847–57. doi: 10.1080/21655979.2021.1999372
98. Christie JD, Appel N, Canter H, Achi JG, Elliott NM, de Matos AL, et al. Systemic Delivery of TNF-Armed Myxoma Virus Plus Immune Checkpoint Inhibitor Eliminates Lung Metastatic Mouse Osteosarcoma. Mol Ther - Oncol (2021) 22:539–54. doi: 10.1016/j.omto.2021.07.014
99. Klose C, Berchtold S, Schmidt M, Beil J, Smirnow I, Venturelli S, et al. Biological Treatment of Pediatric Sarcomas by Combined Virotherapy and NK Cell Therapy. BMC Cancer (2019) 19:1–15. doi: 10.1186/s12885-019-6387-5
100. Hingorani P, Zhang W, Lin J, Liu L, Guha C, Kolb EA. Systemic Administration of Reovirus (Reolysin) Inhibits Growth of Human Sarcoma Xenografts. Cancer (2011) 117:1764–74. doi: 10.1002/cncr.25741
101. Phelps MP, Yang H, Patel S, Rahman MM, McFadden G, Chen E. Oncolytic Virus-Mediated RAS Targeting in Rhabdomyosarcoma. Mol Ther - Oncol (2018) 11:52–61. doi: 10.1016/j.omto.2018.09.001
102. Dobson CC, Naing T, Beug ST, Faye MD, Chabot J, St-Jean M, et al. Oncolytic Virus Synergizes With Smac Mimetic Compounds to Induce Rhabdomyosarcoma Cell Death in a Syngeneic Murine Model. Oncotarget (2017) 8:3495–508. doi: 10.18632/oncotarget.13849
103. Petrov I, Gentschev I, Vyalkova A, Elashry MI, Klymiuk MC, Arnhold S, et al. Canine Adipose-Derived Mesenchymal Stem Cells (Cadmscs) as a “Trojan Horse” in Vaccinia Virus Mediated Oncolytic Therapy Against Canine Soft Tissue Sarcomas. Viruses (2020) 12:750. doi: 10.3390/v12070750
104. Tejada S, Díez-Valle R, Domínguez PD, Patiño-García A, González-Huarriz M, Fueyo J, et al. DNX-2401, an Oncolytic Virus, for the Treatment of Newly Diagnosed Diffuse Intrinsic Pontine Gliomas: A Case Report. Front Oncol (2018) 8:61. doi: 10.3389/fonc.2018.00061
105. Ruano D, López-Martín JA, Moreno L, Lassaletta Á, Bautista F, Andión M, et al. First-In-Human, First-In-Child Trial of Autologous MSCs Carrying the Oncolytic Virus Icovir-5 in Patients With Advanced Tumors. Mol Ther (2020) 28:1033–42. doi: 10.1016/j.ymthe.2020.01.019
106. Streby KA, Geller JI, Currier MA, Warren PS, Racadio JM, Towbin AJ, et al. Intratumoral Injection of HSV1716, an Oncolytic Herpes Virus, is Safe and Shows Evidence of Immune Response and Viral Replication in Young Cancer Patients. Clin Cancer Res (2017) 23:3566–74. doi: 10.1158/1078-0432.CCR-16-2900
107. Friedman GK, Johnston JM, Bag AK, Bernstock JD, Li R, Aban I, et al. Oncolytic HSV-1 G207 Immunovirotherapy for Pediatric High-Grade Gliomas. N Engl J Med (2021) 384:1613–22. doi: 10.1056/nejmoa2024947
108. Cripe TP, Ngo MC, Geller JI, Louis CU, Currier MA, Racadio JM, et al. Phase 1 Study of Intratumoral Pexa-Vec (JX-594), an Oncolytic and Immunotherapeutic Vaccinia Virus, in Pediatric Cancer Patients. Mol Ther (2015) 23:602–8. doi: 10.1038/mt.2014.243
109. Kolb EA, Sampson V, Stabley D, Walter A, Sol-Church K, Cripe T, et al. A Phase I Trial and Viral Clearance Study of Reovirus (Reolysin) in Children With Relapsed or Refractory Extracranial Solid Tumors: A Children’s Oncology Group Phase I Consortium Report. Pediatr Blood Cancer (2015) 62:751–8. doi: 10.1002/pbc.25464
110. Rudin CM, Poirier JT, Senzer NN, Stephenson J, Loesch D, Burroughs KD, et al. Phase I Clinical Study of Seneca Valley Virus (SVV-001), a Replication-Competent Picornavirus, in Advanced Solid Tumors With Neuroendocrine Features. Clin Cancer Res (2011) 17:888–95. doi: 10.1158/1078-0432.CCR-10-1706
111. Wagner S, Csatary CM, Gosztonyi G, Koch HC, Hartmann C, Peters O, et al. Combined Treatment of Pediatric High-Grade Glioma With the Oncolytic Viral Strain MTH-68/H and Oral Valproic Acid. Apmis (2006) 114:731–43. doi: 10.1111/j.1600-0463.2006.apm_516.x
112. Burke MJ, Ahern C, Weigel BJ, Poirier JT, Rudin CM, Chen Y, et al. Phase I Trial of Seneca Valley Virus (NTX-010) in Children With Relapsed/Refractory Solid Tumors: A Report of the Children’s Oncology Group. Pediatr Blood Cancer (2015) 62:743–50. doi: 10.1002/pbc.25269
113. Melen GJ, Franco-Luzón L, Ruano D, González-Murillo Á, Alfranca A, Casco F, et al. Influence of Carrier Cells on the Clinical Outcome of Children With Neuroblastoma Treated With High Dose of Oncolytic Adenovirus Delivered in Mesenchymal Stem Cells. Cancer Lett (2016) 371:161–70. doi: 10.1016/j.canlet.2015.11.036
114. Tejada S, Alonso M, Patiño A, Fueyo J, Gomez-Manzano C, Diez-Valle R. Phase I Trial of DNX-2401 for Diffuse Intrinsic Pontine Glioma Newly Diagnosed in Pediatric Patients. Clin Neurosurg (2018) 83:1050–6. doi: 10.1093/neuros/nyx507
115. Streby KA, Currier MA, Triplet M, Ott K, Dishman DJ, Vaughan MR, et al. First-In-Human Intravenous Seprehvir in Young Cancer Patients: A Phase 1 Clinical Trial. Mol Ther (2019) 27:1930–8. doi: 10.1016/j.ymthe.2019.08.020
116. Hwang JK, Hong J, Yun CO. Oncolytic Viruses and Immune Checkpoint Inhibitors: Preclinical Developments to Clinical Trials. Int J Mol Sci (2020) 21:1–26. doi: 10.3390/ijms21228627
117. Ajina A, Maher J. Prospects for Combined Use of Oncolytic Viruses and CAR T-Cells. J Immunother Cancer (2017) 5:1–27. doi: 10.1186/s40425-017-0294-6
118. Bommareddy PK, Shettigar M, Kaufman HL. Integrating Oncolytic Viruses in Combination Cancer Immunotherapy. Nat Rev Immunol (2018) 18:498–513. doi: 10.1038/s41577-018-0014-6
119. Ottolino-Perry K, Diallo JS, Lichty BD, Bell JC, Andrea McCart J. Intelligent Design: Combination Therapy With Oncolytic Viruses. Mol Ther (2010) 18:251–63. doi: 10.1038/mt.2009.283
120. Ochsenbein AF, Fehr T, Lutz C, Suter M, Brombacher F, Hengartner H, et al. Control of Early Viral and Bacterial Distribution and Disease by Natural Antibodies. Sci (80- ) (1999) 286:2156–9. doi: 10.1126/science.286.5447.2156
Keywords: oncolytic viruses, pediatric solid tumors, pediatric brain tumors, sarcomas, neuroblastoma, clinical trials
Citation: de la Nava D, Selvi KM and Alonso MM (2022) Immunovirotherapy for Pediatric Solid Tumors: A Promising Treatment That is Becoming a Reality. Front. Immunol. 13:866892. doi: 10.3389/fimmu.2022.866892
Received: 31 January 2022; Accepted: 23 March 2022;
Published: 13 April 2022.
Edited by:
Orazio Vittorio, University of New South Wales, AustraliaReviewed by:
Paul G. Schlegel, University Children’s Hospital Würzburg, GermanyVincenzo Cerullo, University of Helsinki, Finland
Copyright © 2022 de la Nava, Selvi and Alonso. This is an open-access article distributed under the terms of the Creative Commons Attribution License (CC BY). The use, distribution or reproduction in other forums is permitted, provided the original author(s) and the copyright owner(s) are credited and that the original publication in this journal is cited, in accordance with accepted academic practice. No use, distribution or reproduction is permitted which does not comply with these terms.
*Correspondence: Marta M. Alonso, mmalonso@unav.es