- Department of Interventional Medicine, Zhuhai People’s Hospital (Zhuhai Hospital affiliated with Jinan University), Zhuhai, China
γδ T cells are one of only three immune cell types that express antigen receptors that undergo somatic recombination, and they contribute to immune responses to infection, cellular transformation, and tissue damage. As a “bridge” between the innate and adaptive immune systems, γδ T cells have been noted to be involved in various immune responses during cancer progression. The purpose of our study was to review current published information on γδ T cells and investigate their functions in different types of malignancy using bibliometric and bioinformatic methods. Our results indicated that studies on γδ T cells and cancer progression increased from 2014, and the number had peaked by 2021. We discovered that there is international cooperation in the performance of studies among 26 countries, where China was identified as the most productive with the highest citations. Using keyword co-occurrence analysis, we found that among all the cancer types investigated, gastric and breast cancers were most closely related to γδ T cells. Furthermore, interleukin (IL)-17 and IL-2 were the most common cytokines linked to γδ T cells and our investigation of their potential involvement in the prognosis of gastric and breast cancers, identified their different roles in various malignancies. Thus, we concluded that γδ T cells might influence the progression of different cancers in diverse ways.
Introduction
T lymphocytes play a critical role in the response and regulation of human immune functions (1). Human T lymphocytes can be divided into αβ (such as CD4 and CD8) and γδ T cells according to the T cell receptor (TCR) structures. In human peripheral blood lymphocytes, αβ T cells are the dominant cells, whereas γδ T cells generally account for only 1% to 5% (2). The functions of these two types of cells and their response mechanisms in the immune response are also different. For example, the recognition of antigens by αβ T but not γδ cells depends on major histocompatibility complex (MHC) molecules (3).
Human γδ T cells have numerous unique biological functions. Based on their distribution and ability to recognize antigens, this population of cells is considered a “bridge” between innate and adaptive immunity (4). According to the structural differences between the γ and δ chains, γδ T cells can be divided into two main subgroups, namely Vδ1 and Vδ2, with distinct functions (5). For example, the Vδ2 cell subset inhibits bacterial infection and tumor progression (6, 7). In human peripheral blood, 50–90% of γδ T cells express Vγ9Vδ2 receptors (8). An increasing number of studies have shown that Vγ9Vδ2 T cells have a very important inhibitory effect on the occurrence and development of tumors by significantly inhibiting the growth of tumor cells (9).
However, a pro-tumor role for interleukin (IL)-17-producing γδ T cells has also been reported in human cancers. Specifically, Vδ1 T cells are the major source of the IL-17 involved in chronic inflammation in colorectal cancer (10). These results indicate that γδ T cells might be a “double-edged sword” in cancer treatment. In this study, we aimed to summarize the current knowledge of γδ T cell research and investigate their functions in different subtypes of malignancy using bibliometrics and bioinformatics methods.
Methods
Scopus Search
Scopus (Elsevier, Amsterdam, The Netherlands) was chosen as the main database for our literature search (11, 12). The following search formulas were used in Scopus: TITLE-ABS-KEY (“γδT cell” and “cancer”) AND PUBYEAR>1993 AND PUBYEAR<2022. To avoid citation duplication, the literature search and extraction were completed on a single day, January 10, 2022 and the result yielded 239 studies.
The titles, abstracts, and keywords of the 239 studies were scanned and filtered manually. Full texts were further examined where necessary. To achieve precise and non-duplicated results, we set the inclusion criteria as (1) a clear correlation between γδT cell and cancer; (2) human, mouse, or cell-based studies; and (3) document type as “article”. Finally, 190 studies were included and summarized in a csv file for the subsequent analysis.
Analysis Using VOSviewer
We uniformed “γδt cell, γδt cells, γδt-cell, γδt-cells, gdT cells, gamma delta T cell, gamma delta T cells, and gamma delta T lymphocyte” to “γδ T cell”. In addition, “natural killer cell, natural killer cells, nkt cell, and nkt cells” were uniformed to “nk cells”. Then, the csv file was uploaded into VOSviewer to conduct co-occurrence analysis of authors, countries, and keywords. The minimum number of occurrences of each keyword was set to five and the total intensity of co-occurrence bonds to other keywords was also derived.
Overall Survival Estimation of γδ T Cell in Different Malignancies
The Kaplan–Meier plotter (13) is an online tool for assessing the correlation between the expression of 30 k genes (mRNA, miRNA, and protein) and survival in 25 k+ samples from 21 tumor types. We conducted a survival analysis of γδT cells with different subtypes of cancer. Based on the median transcription level of each target gene, patients were allocated to the high and low expression groups, and Kaplan–Meier plots were generated accordingly. The hazard ratio (HR) with the 95% confidence interval and log-rank p-values were also calculated. Statistical significance was set at p < 0.05.
Results
Our literature search identified 190 studies on the relationship between γδT cells and cancer conducted from 1993 to 2021. The results indicated that before 2006, there were few studies on γδ T cells and cancer progression annually, whereas the number increased from 2014 and had peaked by 2021 (Figure 1A). There was international cooperation in conducting studies among 26 countries, and China had the most publications as well as the highest citations, followed by Japan and the US (Figure 1B). The top-20 cited publications are listed in Table 1. The paper “Enterococcus hirae and Barnesiella intestinihominis facilitate cyclophosphamide-induced therapeutic immunomodulatory Effects” by Daillère et al. (13) had the highest citations at 312 times. The publication “γδT17 cells promote the accumulation and expansion of myeloid-derived suppressor cells in human colorectal cancer” by Wu et al. (10) had the second highest number of citations at 301 times.
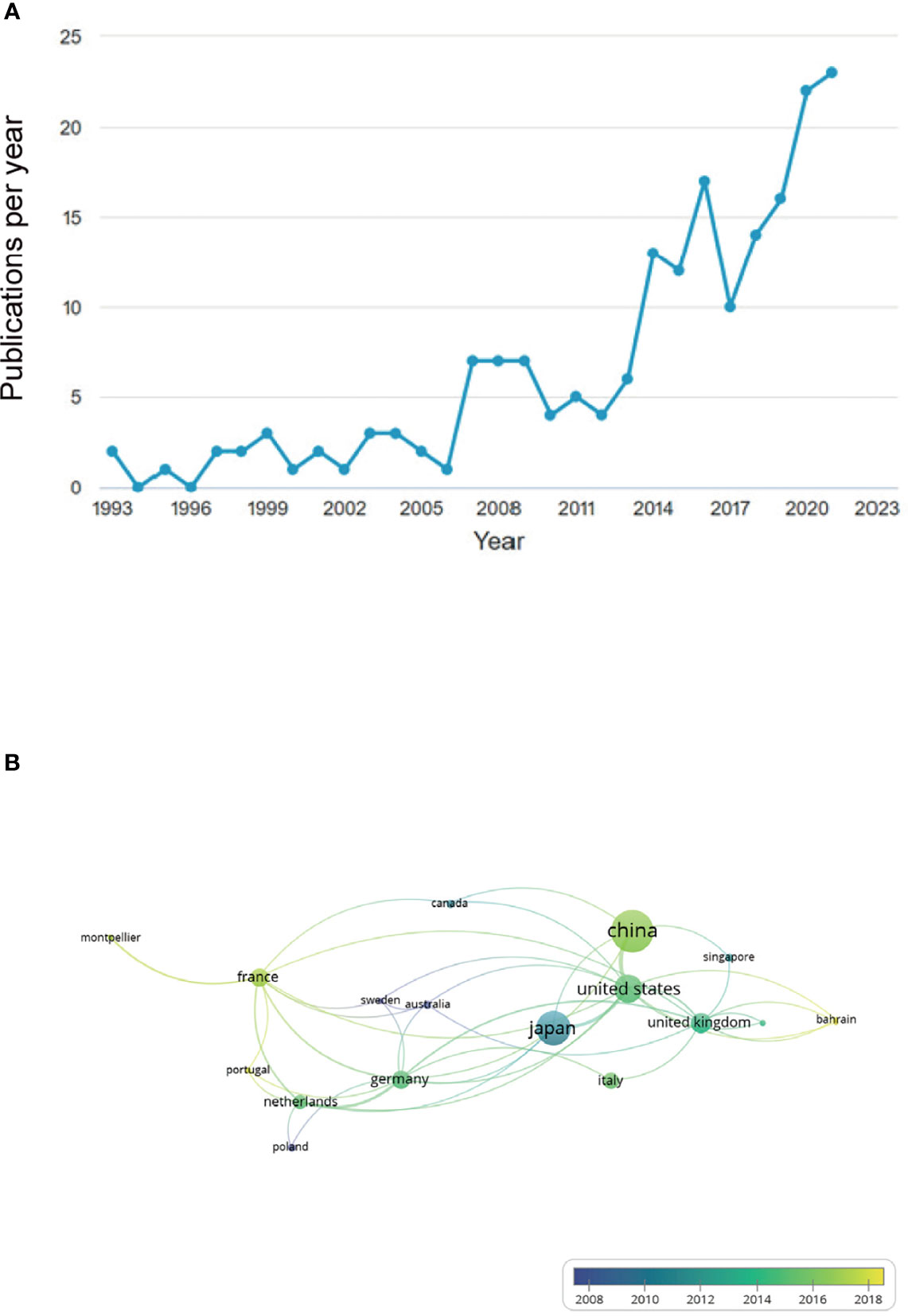
Figure 1 Annual number of publications related to γδ T cell and cancer increased from 2014 to 2021 and international cooperation in related research occurred between different countries. (A) Before 2006, there were few studies on γδ T cell and cancer progression, annually, but number of studies increased from 2014 and peaked by 2021. (B) Numerous national connections were established in this field of study, with China, Japan, and the US emerging as the most productive countries.
Furthermore, we also conducted the co-occurrences analysis of keywords with the software VOSviewer, in order to figure out the relationship between γδT cells and other important scientific issues. The minimum number of occurrences of a keyword was set to 5 and 14 were finally identified among all 564 keywords. The hotspots in γδT cell functions are presented in the overlay visualization map scaled by occurrences (Figure 2). Accordingly, γδT cells were shown to be responsible for the immune response and communicated with dendritic cells and NK cells. We also found that among all the types of cancer, gastric and breast cancer were most closely linked to γδ T cells. Furthermore, IL-17 and IL-2 are the most common cytokines linked to γδ T cells.
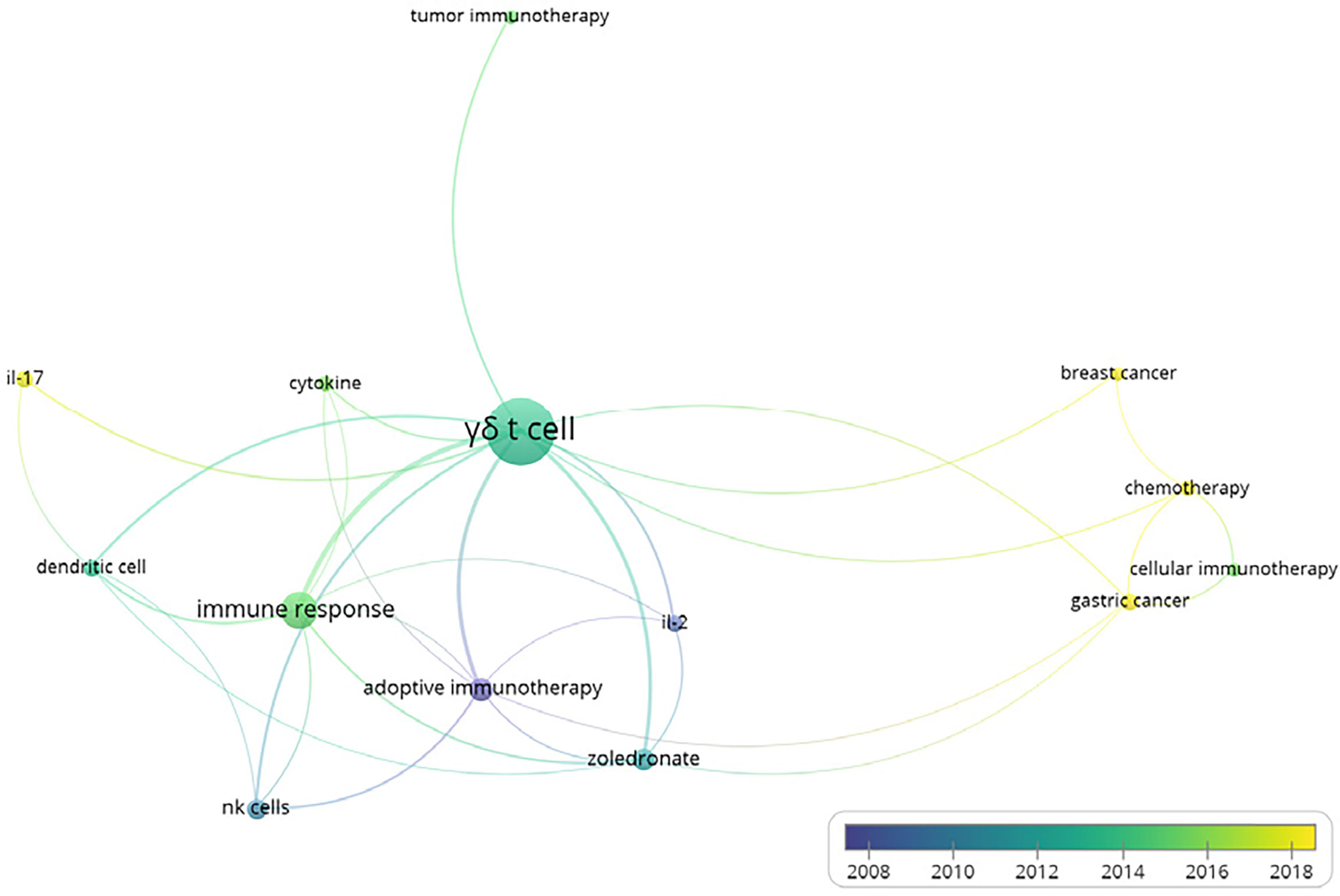
Figure 2 Overlay visualization map of author keywords co-occurrence analysis. Map shows that γδ T cells mediated immune responses. Of all investigated cancers, gastric and breast cancers were most closely linked to γδ T cells. Interleukin (IL)-17 and IL-2 were identified as the most common cytokines linked to γδ T cells.
IL-17 production by certain γδ T cell subsets has been reported to recruit immunosuppressive cells such as myeloid-derived suppressor cells (MDSCs) or small peritoneal macrophages, which can promote angiogenesis, tumor cell growth, and inducible regulatory T (Treg) cell differentiation (7). IL-2 could augment the γδT-17 response in favor of short-lived effectors with limited plasticity, particularly in the presence of IL-1β and IL-23 (33). Taken together, these results led us to conclude that γδ T cells could have a role in cancer development via IL-2 or IL-17. Consequently, we investigated the effects of IL-17 and IL-2 on gastric and breast cancer, and identified their distinct roles in different malignancies.
In breast cancer, high expression levels of IL-17 and IL-2 indicated a promising prognosis. The median survival of patients with breast cancer with low and high expression levels of IL-2 was 43 months and 56 months, respectively (HR = 0.86, p = 0.0031, Figure 3A). Furthermore, the median survival of breast cancer patients with low and high expression levels of IL-17 was 216.66 and 228.85 months, respectively (HR = 0.8, p < 0.001, Figure 3B). However, IL-2 and IL-17 played a dinstinct role in gastric cancer prognosis. Our results also showed that the median survival of patients with gastric cancer who had low and high expression levels of IL-2 was 35.4 and 22 months, respectively (HR = 1.58, p < 0.001, Figure 3C). In addition, for patients with gastric cancer with low and high expression levels of IL-17, the median survival was 34.7 and 19.5 months (HR = 1.6, p < 0.001, Figure 3D).
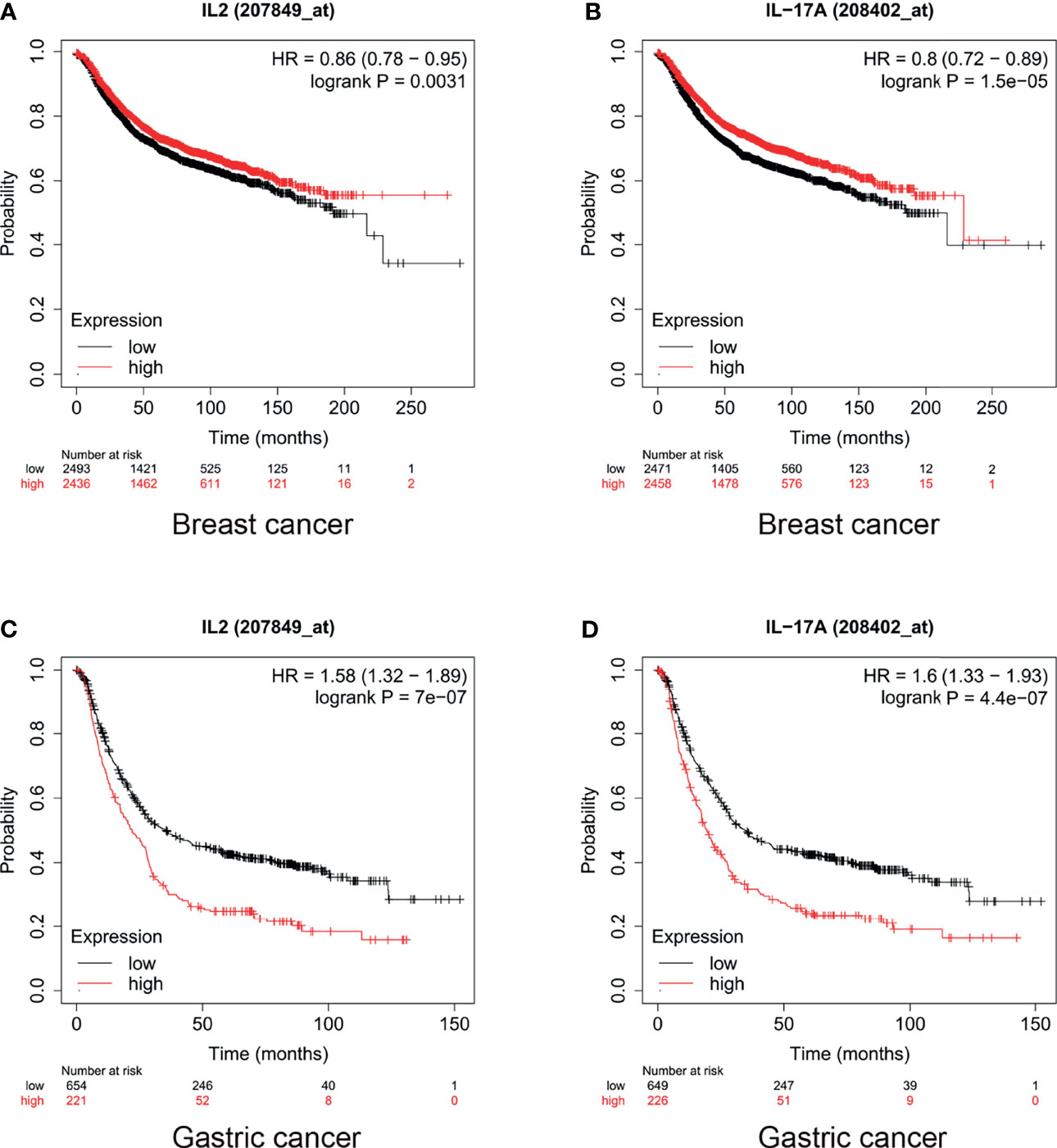
Figure 3 Levels of interleukin (IL)-2 and IL-17 played different roles in breast and gastric cancer prognosis. High IL-17 and IL-2 expression indicated (A, B) promising prognosis in breast cancer and (C, D) poor prognosis in gastric cancer.
Furthermore, we conducted a subgroup analysis of these two types of cancers. For breast cancer, we examined the status of the estrogen receptor (ER), progesterone receptor (PR), and human epidermal growth factor receptor 2 (HER2). As shown in Figure 4, the median survival of ER-negative (ER-) patients with low expression of IL-2 was 18 months, whereas that of patients with high expression was 25 months (HR = 0.79, p = 0.015, Figure 4B). For HER2- patients, the median survival of patients with low expression of IL-2 was 50 months, whereas that of patients with high expression was 61.92 months (HR = 0.86, p = 0.011, Figure 4F). The expression of IL-2 did not influence survival in ER-positive (ER+), HER2+, PR+, or PR- subgroups (Figures 4A, C–E).
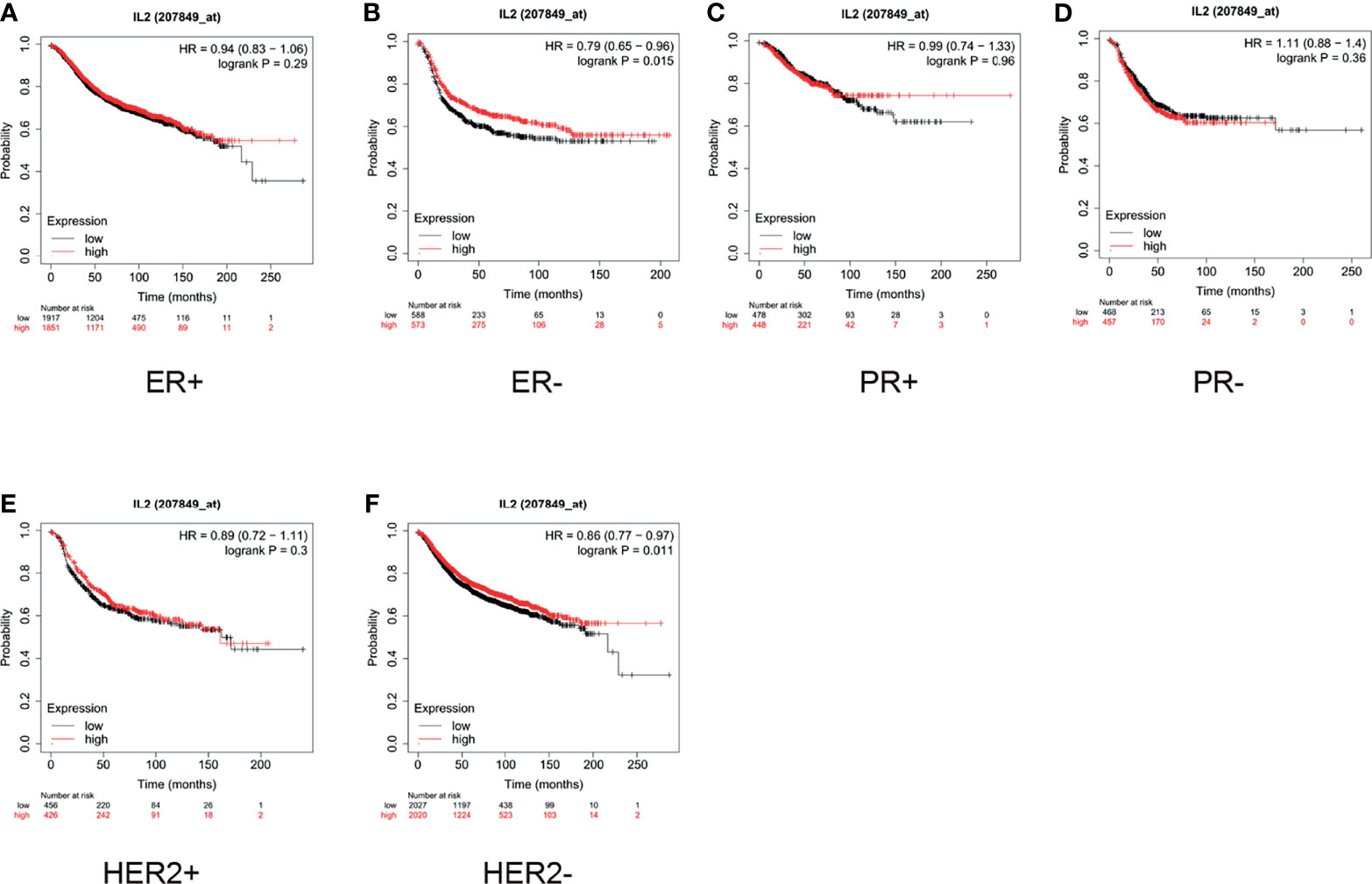
Figure 4 In patients with breast cancer, high interleukin (IL)-2 expression only indicated better prognosis in estrogen receptor-negative (ER-) and human epidermal growth factor receptor 2 negative (HER2-) subgroups. (A) High expression of IL-2 (A) did not correlate with better prognosis in ER+ patients (hazard ratio [HR] = 0.94, p = 0.29) and (B) indicated better prognosis in ER- patients (HR = 0.79, p = 0.015). (C, D). IL-2 expression did not significantly influence survival in progesterone receptor-positive (PR+; HR = 0.99, p = 0.96) or PR- (HR = 1.11, p = 0.36) subgroups. High expression of IL-2 (E) did not correlate with better prognosis in HER2+ patients (HR = 0.89, p = 0.3) and (F) indicated better prognosis in HER2- patients (HR = 0.86, p = 0.011).
As shown in Figure 5, for ER+ patients, high expression of IL-17 was significantly correlated with a longer survival time (HR = 0.85, p = 0.0095, Figure 5A). For ER- patients, the median survival of those with low and high expression of IL-17 was 18 and 28.75 months, respectively (HR = 0.73, p = 0.0009, Figure 5B). The expression of IL-17 did not significantly influence the overall survival, regardless of whether the patients were PR+ or PR- (Figures 5C, D). As demonstrated in Figure 5E, high expression of IL-17 did not correlate with better prognosis in HER2+ patients (HR = 0.89, p = 0.3). For HER2- patients, high expression of IL-17 indicated a better prognosis than low expression did (HR = 0.79, p < 0.001, Figure 5F).
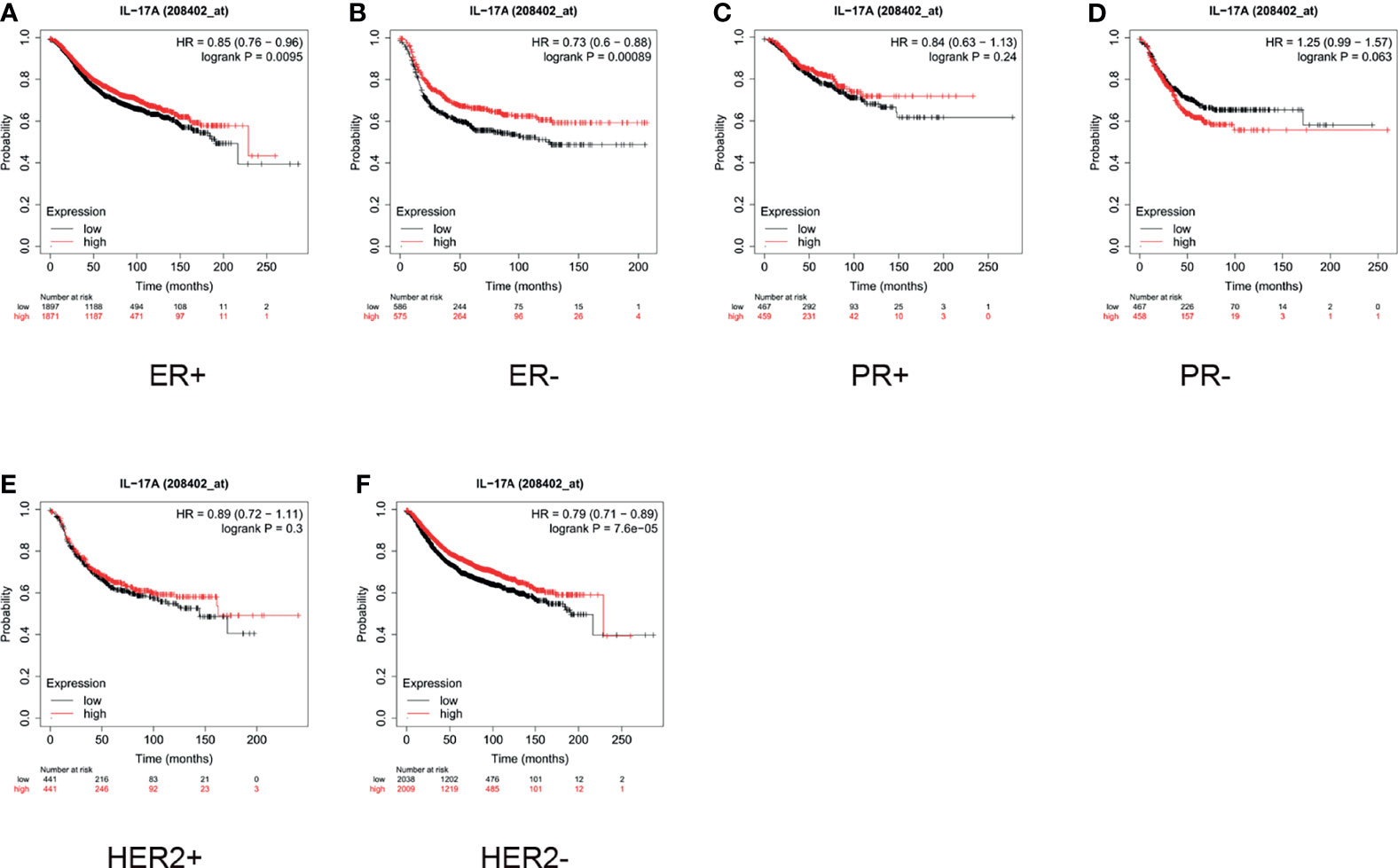
Figure 5 In breast cancer patients, high expression of interleukin (IL)-17 only indicated better prognosis in estrogen receptor-positive (ER+), ER-negative (ER-), and human epidermal growth factor receptor 2 negative (HER2-) subgroups. High expression of IL-17 indicated better prognosis in (A) ER+ (hazard ratio [HR] = 0.85, p < 0.001) and (B) ER- (HR = 0.73, p < 0.001) patients. (C, D) IL-17 expression did not significantly influence survival in progesterone-positive (PR+; HR = 0.84, p = 0.24) or PR- (HR = 1.25, p = 0.063) subgroups. (E) High expression of IL-17 did not correlate with better prognosis in HER2+ patients (HR = 0.89, p = 0.3). (F) High expression of IL-2 indicated better prognosis in HER2- patients (HR = 0.79, p < 0.001).
For gastric cancer, we used the Lauren classification to divide the cohort into three subgroups: intestinal, diffuse, and mixed. As shown in Figure 6, the analysis of the expression of IL-2 in different subtypes of gastric cancer showed that low expression of IL-2 indicated a better prognosis in both intestinal and diffuse patients than high expression levels did (Figures 6A, B). However, the difference was not statistically significant in patients with mixed conditions (Figure 6C). Moreover, the median survival of intestinal patients with low expression of IL-17 was 123.8 months, whereas that of patients with high expression was only 23.4 months (HR = 2.02, p < 0.001, Figure 6D), and the difference did not have statistical significance in diffuse and mixed subgroups (Figures 6E, F).
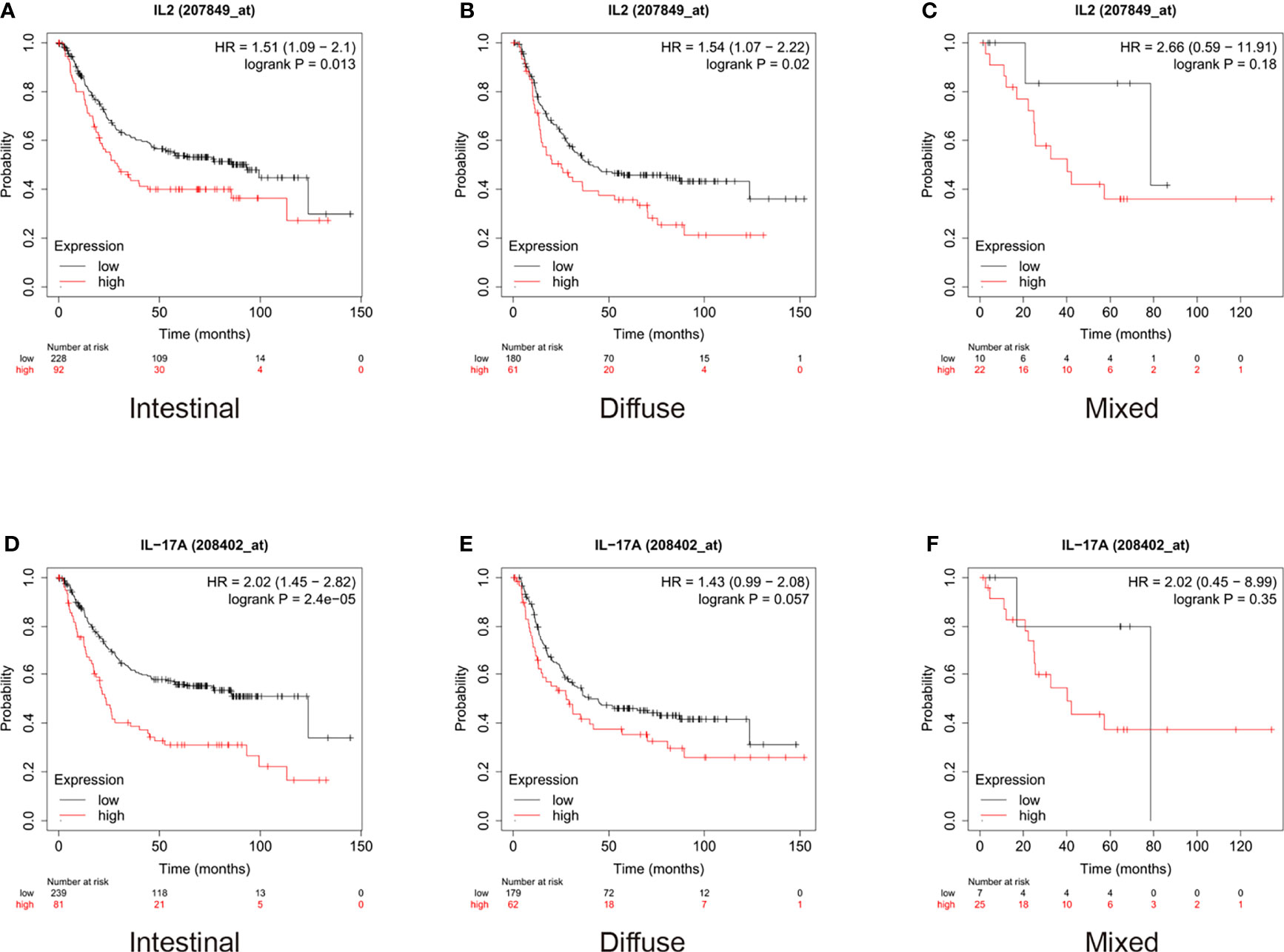
Figure 6 In gastric cancer patients, high expression of interleukin (IL)-2 indicated worse prognosis in patients with both intestinal and diffuse conditions and high expression of IL-17 indicated worse prognosis only in patients with intestinal condition. (A, B) High expression of IL-2 indicated worse prognosis in patients with both intestinal (hazard ratio [HR] = 1.51, p = 0.013) and diffuse (HR = 1.54, p = 0.3) conditions. (C) High expression of IL-2 did not significantly influence survival in mixed patients (HR = 2.66, p = 0.18). (D) High expression of IL-17 indicated worse prognosis in patients with intestinal conditions (HR = 2.02, p < 0.001). (E, F) High expression of IL-17 did not significantly influence survival in patients with diffuse (HR = 1.43, p = 0.057) and mixed (HR = 2.02, p = 0.35) conditions.
Discussion
Our results showed that the number of studies on γδ T cells has increased since 2014, suggesting that most were likely novel. The examination of the top-20 cited publications revealed that numerous studies concentrated on the role of γδ T cells in cancer treatment. In addition, the co-occurrence analysis revealed that γδ T cells were more closely related to breast and gastric cancers than they were to the other investigated malignancies. IL-2 and IL-17 are the two most important cytokines related to γδ T cells; therefore, we investigated their influence on breast and gastric cancers.
The two cytokines, IL-2 and IL-17, which we focused on in this study, play different roles in breast and gastric cancers, where they promote the development of gastric cancer but inhibit the progression of breast cancer. The results of the subgroup analysis further clarified this finding. For intestinal gastric cancer, low expression of IL-2 and IL-17 indicated a promising prognosis, whereas for diffuse and mixed gastric cancers, the expression of these cytokines did not significantly affect survival. The cases of intestinal gastric cancer were deemed to be early stage and, therefore, we speculated that γδ T cells might affect the prognosis of gastric cancer at the disease onset.
In breast cancer, ER, PR, and HER2 status have been verified as important prognostic factors; therefore, based on these different statuses, we also investigated how the expression of IL-2 and IL-17 influenced patient survival. Our results showed that regardless of ER status, high expression of IL-17 indicated a longer survival time, but only a better prognosis in HER2- patients than low expression did. In addition, high expression of IL-2 indicated a better prognosis in patients with ER- or HER2- breast cancer than low expression did. However, regardless of whether PR was positive or negative, the expression of IL-2 and IL-17 did not influence the survival of patients with breast cancer in this subgroup analysis. Based on these results, we hypothesized that HER2 might play an important role in breast cancer treatment via γδ T cells.
IL-2 and IL-17 have opposite roles in the development of breast and gastric cancers and, therefore, we postulated that γδ T cells have distinct functions in different malignancies. Furthermore, we also discovered that even in the same malignancy, γδ T cells might have distinct functions in different forms, implying some underlying mechanism may exist between γδ T cells and cell receptors. The abundant cytokine secretion and non-MHC-restricted antigen recognition capacity of γδ T cells has encouraged the investigation of their application in cancer adoptive immunotherapy (34). Currently, evidence has accumulated from studies in numerous cancers, and the results demonstrate that γδ T cells could be well tolerated in the treatment of cancer (3, 34–36). Studies have also revealed that γδ T cells can exert anticancer activity through various mechanisms, such as eliminating tumor cells via the perforin-granzyme pathway (37), binding to TNF-related apoptosis-inducing ligand (TRAIL) and Fas ligand (FasL) (38), via antibody-dependent cellular cytotoxicity (ADCC) (39), or by secreting interferon (IFN)-γ and tumor necrosis factor (TNF)-α (40, 41). In addition to these direct antitumor effects, specific γδ T cell subsets also exert an indirect antitumor effect, which is complemented by interactions with other immune cells such as B cells, DCs, αβ T cells, and NK cells (42). However, recent studies claim that γδ T cells could stimulate cancer development (43–45) by impairing the antitumor ability of immunocytes or enhancing the function of immunosuppressive cells (10, 46, 47). For instance, γδ T17 cells are a major source of IL-17 in the cancer microenvironment (48), and IL-17 contributes to cancer development by supporting angiogenesis in several malignancies, such as gallbladder cancer, gastric cancer and non-small-cell lung cancer (26, 49, 50).
The limitation of the current study was that we only chose Scopus as our database and did not use databases such as Pubmed or Google Scholar. Besides, as we used the Kaplan Meier plotter as our database to achieve survival data, this database collected information from many independent datasets, which might cause bias in our analysis.
In conclusion, using bibliometric analysis, we identified IL-17 and IL-2 as the most common cytokines linked to γδ T cells. Furthermore, based on our investigation of the role of IL-17 and IL-2 in the prognosis of gastric and breast cancer, we discovered that they play different roles in various malignancies. Moreover, in the same malignancy, the expression levels of certain genes or different variations could impact the function of γδ T cells (Figure 7). Finally, we concluded that γδ T cells might influence the progression of different cancers in diverse ways.
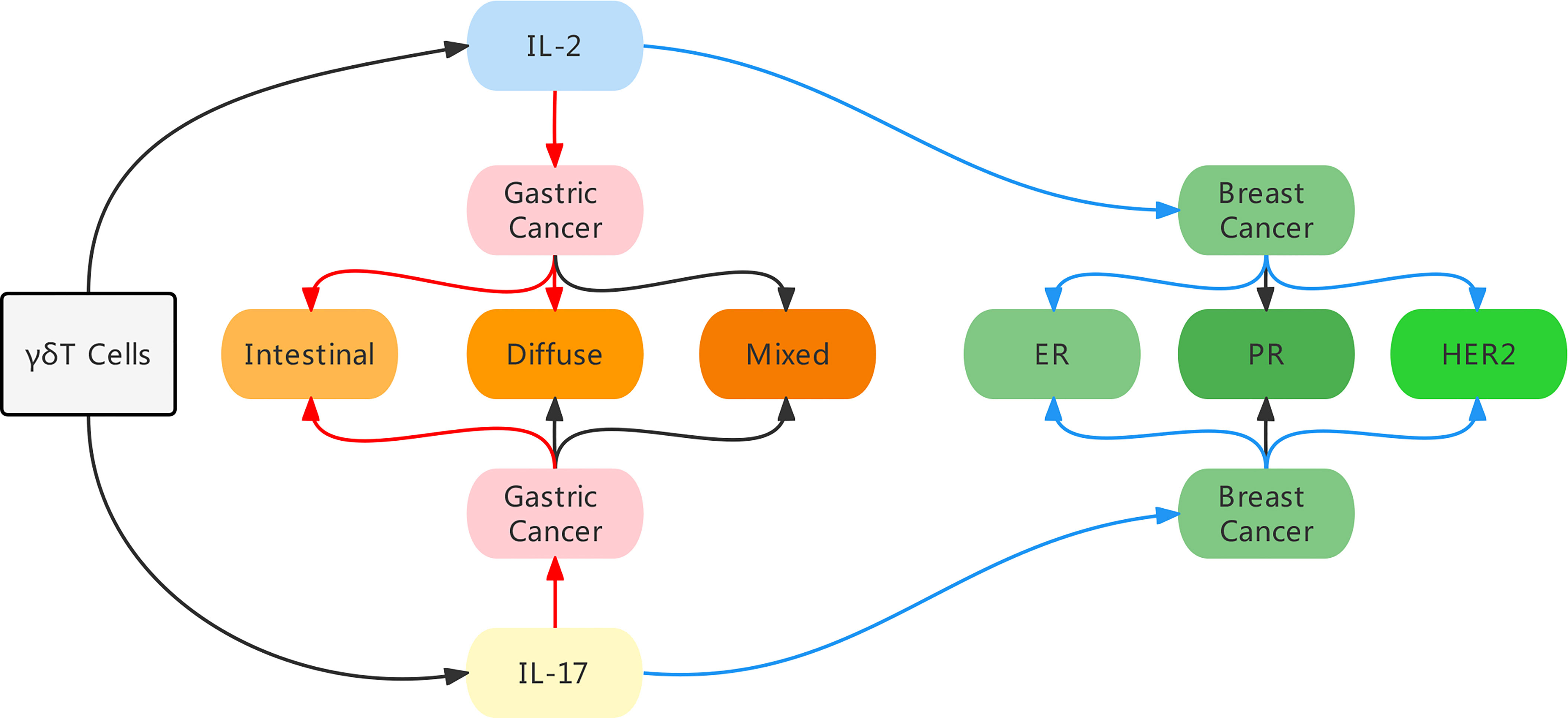
Figure 7 An illustration of the main findings in our study. By bibliometric analysis, we identified IL-17 and IL-2 as the most common cytokines linked to γδ T cells, and these two cytokines influenced the prognosis in different manners in gastric cancer and breast cancer. In breast cancer, high expression of IL-2 and IL-17 indicated a better prognosis, especially in ER negative and HER2 negative patients. While in gastric cancer patients, high level of IL-2 indicated a worse prognosis in intestinal and diffuse conditions, and high level of IL-17 was merely predictive of a poor prognosis in intestinal condition. Red arrow indicates a poor prognosis, blue arrow indicates a good prognosis.
Data Availability Statement
The datasets presented in this study can be found in online repositories. The names of the repository/repositories and accession number(s) can be found in the article/supplementary material.
Author Contributions
BL conducted the data search and paper writing, XH and YW contributed to the bibliometric analysis, J-wH and Y-bZ revised the figures and tables, YL and L-gL designed the study and revised the paper. All authors contributed to the article and approved the submitted version.
Funding
This work is partially supported by National Key Research and Development Program of China (No. 2017YFA0205200) and the National Natural Science Foundation of China (No. 81571785, 81771957, 81801811).
Conflict of Interest
The authors declare that the research was conducted in the absence of any commercial or financial relationships that could be construed as a potential conflict of interest.
Publisher’s Note
All claims expressed in this article are solely those of the authors and do not necessarily represent those of their affiliated organizations, or those of the publisher, the editors and the reviewers. Any product that may be evaluated in this article, or claim that may be made by its manufacturer, is not guaranteed or endorsed by the publisher.
References
1. Khan U, Ghazanfar H. T Lymphocytes and Autoimmunity. Int Rev Cell Mol Biol (2018) 341:125–68. doi: 10.1016/bs.ircmb.2018.05.008
2. Morath A, Schamel WW. Alphabeta and Gammadelta T Cell Receptors: Similar But Different. J Leukoc Biol (2020) 107(6):1045–55. doi: 10.1002/JLB.2MR1219-233R
3. Vantourout P, Hayday A. Six-Of-the-Best: Unique Contributions of Gammadelta T Cells to Immunology. Nat Rev Immunol (2013) 13(2):88–100. doi: 10.1038/nri3384
4. Morandi F, Yazdanifar M, Cocco C, Bertaina A, Airoldi I. Engineering the Bridge Between Innate and Adaptive Immunity for Cancer Immunotherapy: Focus on Gammadelta T and NK Cells. Cells (2020) 9(8):1757. doi: 10.3390/cells9081757
5. Shiromizu CM, Jancic CC. Gammadelta T Lymphocytes: An Effector Cell in Autoimmunity and Infection. Front Immunol (2018) 9:2389. doi: 10.3389/fimmu.2018.02389
6. Hoytema van Konijnenburg DP, Reis BS, Pedicord VA, Farache J, Victora GD, Mucida D. Intestinal Epithelial and Intraepithelial T Cell Crosstalk Mediates a Dynamic Response to Infection. Cell (2017) 171(4):783–94.e13. doi: 10.1016/j.cell.2017.08.046
7. Silva-Santos B, Serre K, Norell H. Gammadelta T Cells in Cancer. Nat Rev Immunol (2015) 15(11):683–91. doi: 10.1038/nri3904
8. Silva-Santos B, Mensurado S, Coffelt SB. Gammadelta T Cells: Pleiotropic Immune Effectors With Therapeutic Potential in Cancer. Nat Rev Cancer (2019) 19(7):392–404. doi: 10.1038/s41568-019-0153-5
9. Xu Y, Xiang Z, Alnaggar M, Kouakanou L, Li J, He J, et al. Allogeneic Vgamma9Vdelta2 T-Cell Immunotherapy Exhibits Promising Clinical Safety and Prolongs the Survival of Patients With Late-Stage Lung or Liver Cancer. Cell Mol Immunol (2021) 18(2):427–39. doi: 10.1038/s41423-020-0515-7
10. Wu P, Wu D, Ni C, Ye J, Chen W, Hu G, et al. Gammadeltat17 Cells Promote the Accumulation and Expansion of Myeloid-Derived Suppressor Cells in Human Colorectal Cancer. Immunity (2014) 40(5):785–800. doi: 10.1016/j.immuni.2014.03.013
11. Hassan W, Kamdem JP, Kamal MA, da Rocha JBT. Bibliometric Analysis of Current Drug Metabolism: The Twentieth Anniversary From 2000-2019. Curr Drug Metab (2020) 21(9):685–703. doi: 10.2174/1389200221666200826094233
12. Sweileh WM. Research Trends on Human Trafficking: A Bibliometric Analysis Using Scopus Database. Global Health (2018) 14(1):106. doi: 10.1186/s12992-018-0427-9
13. Lanczky A, Gyorffy B. Web-Based Survival Analysis Tool Tailored for Medical Research (KMplot): Development and Implementation. J Med Internet Res (2021) 23(7):e27633. doi: 10.2196/27633
14. Daillere R, Vetizou M, Waldschmitt N, Yamazaki T, Isnard C, Poirier-Colame V, et al. Enterococcus Hirae and Barnesiella Intestinihominis Facilitate Cyclophosphamide-Induced Therapeutic Immunomodulatory Effects. Immunity (2016) 45(4):931–43. doi: 10.1016/j.immuni.2016.09.009
15. Daley D, Zambirinis CP, Seifert L, Akkad N, Mohan N, Werba G, et al. Gammadelta T Cells Support Pancreatic Oncogenesis by Restraining Alphabeta T Cell Activation. Cell (2016) 166(6):1485–99.e15. doi: 10.1016/j.cell.2016.07.046
16. Sato K, Kimura S, Segawa H, Yokota A, Matsumoto S, Kuroda J, et al. Cytotoxic Effects of Gammadelta T Cells Expanded Ex Vivo by a Third Generation Bisphosphonate for Cancer Immunotherapy. Int J Cancer (2005) 116(1):94–9. doi: 10.1002/ijc.20987
17. Schnurr M, Scholz C, Rothenfusser S, Galambos P, Dauer M, Robe J, et al. Apoptotic Pancreatic Tumor Cells are Superior to Cell Lysates in Promoting Cross-Priming of Cytotoxic T Cells and Activate NK and Gammadelta T Cells. Cancer Res (2002) 62(8):2347–52.
18. Kong Y, Cao W, Xi X, Ma C, Cui L, He W. The NKG2D Ligand ULBP4 Binds to TCRgamma9/delta2 and Induces Cytotoxicity to Tumor Cells Through Both TCRgammadelta and NKG2D. Blood (2009) 114(2):310–7. doi: 10.1182/blood-2008-12-196287
19. Alexander AA, Maniar A, Cummings JS, Hebbeler AM, Schulze DH, Gastman BR, et al. Isopentenyl Pyrophosphate-Activated CD56+ {Gamma}{Delta} T Lymphocytes Display Potent Antitumor Activity Toward Human Squamous Cell Carcinoma. Clin Cancer Res (2008) 14(13):4232–40. doi: 10.1158/1078-0432.CCR-07-4912
20. Sakamoto M, Nakajima J, Murakawa T, Fukami T, Yoshida Y, Murayama T, et al. Adoptive Immunotherapy for Advanced non-Small Cell Lung Cancer Using Zoledronate-Expanded Gammadeltatcells: A Phase I Clinical Study. J Immunother (2011) 34(2):202–11. doi: 10.1097/CJI.0b013e318207ecfb
21. Laurent S, Queirolo P, Boero S, Salvi S, Piccioli P, Boccardo S, et al. The Engagement of CTLA-4 on Primary Melanoma Cell Lines Induces Antibody-Dependent Cellular Cytotoxicity and TNF-Alpha Production. J Transl Med (2013) 11:108. doi: 10.1186/1479-5876-11-108
22. Grose R, Fantl V, Werner S, Chioni AM, Jarosz M, Rudling R, et al. The Role of Fibroblast Growth Factor Receptor 2b in Skin Homeostasis and Cancer Development. EMBO J (2007) 26(5):1268–78. doi: 10.1038/sj.emboj.7601583
23. Capsomidis A, Benthall G, Van Acker HH, Fisher J, Kramer AM, Abeln Z, et al. Chimeric Antigen Receptor-Engineered Human Gamma Delta T Cells: Enhanced Cytotoxicity With Retention of Cross Presentation. Mol Ther (2018) 26(2):354–65. doi: 10.1016/j.ymthe.2017.12.001
24. Okada T, Iiai T, Kawachi Y, Moroda T, Takii Y, Hatakeyama K, et al. Origin of CD57+ T Cells Which Increase at Tumour Sites in Patients With Colorectal Cancer. Clin Exp Immunol (1995) 102(1):159–66. doi: 10.1111/j.1365-2249.1995.tb06650.x
25. Cui J, Wang N, Zhao H, Jin H, Wang G, Niu C, et al. Combination of Radiofrequency Ablation and Sequential Cellular Immunotherapy Improves Progression-Free Survival for Patients With Hepatocellular Carcinoma. Int J Cancer (2014) 134(2):342–51. doi: 10.1002/ijc.28372
26. Patil RS, Shah SU, Shrikhande SV, Goel M, Dikshit RP, Chiplunkar SV. IL17 Producing gammadeltaT Cells Induce Angiogenesis and are Associated With Poor Survival in Gallbladder Cancer Patients. Int J Cancer (2016) 139(4):869–81. doi: 10.1002/ijc.30134
27. Legut M, Cole DK, Sewell AK. The Promise of Gammadelta T Cells and the Gammadelta T Cell Receptor for Cancer Immunotherapy. Cell Mol Immunol (2015) 12(6):656–68. doi: 10.1038/cmi.2015.28
28. Marcu-Malina V, Heijhuurs S, van Buuren M, Hartkamp L, Strand S, Sebestyen Z, et al. Redirecting Alphabeta T Cells Against Cancer Cells by Transfer of a Broadly Tumor-Reactive gammadeltaT-Cell Receptor. Blood (2011) 118(1):50–9. doi: 10.1182/blood-2010-12-325993
29. Ni C, Fang QQ, Chen WZ, Jiang JX, Jiang Z, Ye J, et al. Breast Cancer-Derived Exosomes Transmit lncRNA SNHG16 to Induce CD73+gammadelta1 Treg Cells. Signal Transduct Target Ther (2020) 5(1):41. doi: 10.1038/s41392-020-0129-7
30. Fisher JP, Yan M, Heuijerjans J, Carter L, Abolhassani A, Frosch J, et al. Neuroblastoma Killing Properties of Vdelta2 and Vdelta2-Negative gammadeltaT Cells Following Expansion by Artificial Antigen-Presenting Cells. Clin Cancer Res (2014) 20(22):5720–32. doi: 10.1158/1078-0432.CCR-13-3464
31. Holderness J, Jackiw L, Kimmel E, Kerns H, Radke M, Hedges JF, et al. Select Plant Tannins Induce IL-2Ralpha Up-Regulation and Augment Cell Division in Gammadelta T Cells. J Immunol (2007) 179(10):6468–78. doi: 10.4049/jimmunol.179.10.6468
32. Hu G, Wu P, Cheng P, Zhang Z, Wang Z, Yu X, et al. Tumor-Infiltrating CD39(+)gammadeltaTregs are Novel Immunosuppressive T Cells in Human Colorectal Cancer. Oncoimmunology (2017) 6(2):e1277305. doi: 10.1080/2162402X.2016.1277305
33. Corpuz TM, Vazquez-Lombardi R, Luong JK, Warren J, Stolp J, Christ D, et al. IL-2 Shapes the Survival and Plasticity of IL-17-Producing Gammadelta T Cells. J Immunol (2017) 199(7):2366–76. doi: 10.4049/jimmunol.1700335
34. Dar AA, Patil RS, Chiplunkar SV. Insights Into the Relationship Between Toll Like Receptors and Gamma Delta T Cell Responses. Front Immunol (2014) 5:366. doi: 10.3389/fimmu.2014.00366
35. Yamasaki A, Onishi H, Morisaki T, Katano M. Induction of Cytotoxic T Lymphocytes by CEA Peptide-Pulsed Gammadelta T-Cells Isolated From Patients With Advanced Cancer. Anticancer Res (2011) 31(7):2419–24.
36. Bonneville M, Chen ZW, Dechanet-Merville J, Eberl M, Fournie JJ, Jameson JM, et al. Chicago 2014–30 Years of Gammadelta T Cells. Cell Immunol (2015) 296(1):3–9. doi: 10.1016/j.cellimm.2014.11.001
37. Niu C, Jin H, Li M, Xu J, Xu D, Hu J, et al. In Vitro Analysis of the Proliferative Capacity and Cytotoxic Effects of Ex Vivo Induced Natural Killer Cells, Cytokine-Induced Killer Cells, and Gamma-Delta T Cells. BMC Immunol (2015) 16:61. doi: 10.1186/s12865-015-0124-x
38. Todaro M, D'Asaro M, Caccamo N, Iovino F, Francipane MG, Meraviglia S, et al. Efficient Killing of Human Colon Cancer Stem Cells by Gammadelta T Lymphocytes. J Immunol (2009) 182(11):7287–96. doi: 10.4049/jimmunol.0804288
39. Couzi L, Pitard V, Sicard X, Garrigue I, Hawchar O, Merville P, et al. Antibody-Dependent Anti-Cytomegalovirus Activity of Human Gammadelta T Cells Expressing CD16 (FcgammaRIIIa). Blood (2012) 119(6):1418–27. doi: 10.1182/blood-2011-06-363655
40. Gao Y, Yang W, Pan M, Scully E, Girardi M, Augenlicht LH, et al. Gamma Delta T Cells Provide an Early Source of Interferon Gamma in Tumor Immunity. J Exp Med (2003) 198(3):433–42. doi: 10.1084/jem.20030584
41. Ramstead AG, Jutila MA. Complex Role of Gammadelta T-Cell-Derived Cytokines and Growth Factors in Cancer. J Interferon Cytokine Res (2012) 32(12):563–9. doi: 10.1089/jir.2012.0073
42. Zhao Y, Niu C, Cui J. Gamma-Delta (Gammadelta) T Cells: Friend or Foe in Cancer Development? J Transl Med (2018) 16(1):3. doi: 10.1186/s12967-017-1378-2
43. Coffelt SB, Kersten K, Doornebal CW, Weiden J, Vrijland K, Hau CS, et al. IL-17-Producing Gammadelta T Cells and Neutrophils Conspire to Promote Breast Cancer Metastasis. Nature (2015) 522(7556):345–8. doi: 10.1038/nature14282
44. Jin C, Lagoudas GK, Zhao C, Bullman S, Bhutkar A, Hu B, et al. Commensal Microbiota Promote Lung Cancer Development via Gammadelta T Cells. Cell (2019) 176(5):998–1013.e16. doi: 10.1016/j.cell.2018.12.040
45. McGinley AM, Sutton CE, Edwards SC, Leane CM, DeCourcey J, Teijeiro A, et al. Interleukin-17a Serves a Priming Role in Autoimmunity by Recruiting IL-1beta-Producing Myeloid Cells That Promote Pathogenic T Cells. Immunity (2020) 52(2):342–56 e6. doi: 10.1016/j.immuni.2020.01.002
46. Li J, Liu J, Mao X, Tang Q, Lu H. IL-7 Receptor Blockade Inhibits IL-17-Producing Gammadelta Cells and Suppresses Melanoma Development. Inflammation (2014) 37(5):1444–52. doi: 10.1007/s10753-014-9869-2
47. Kong X, Sun R, Chen Y, Wei H, Tian Z. gammadeltaT Cells Drive Myeloid-Derived Suppressor Cell-Mediated CD8+ T Cell Exhaustion in Hepatitis B Virus-Induced Immunotolerance. J Immunol (2014) 193(4):1645–53. doi: 10.4049/jimmunol.1303432
48. Silva-Santos B. Promoting Angiogenesis Within the Tumor Microenvironment: The Secret Life of Murine Lymphoid IL-17-Producing Gammadelta T Cells. Eur J Immunol (2010) 40(7):1873–6. doi: 10.1002/eji.201040707
49. Wu X, Yang T, Liu X, Guo JN, Xie T, Ding Y, et al. IL-17 Promotes Tumor Angiogenesis Through Stat3 Pathway Mediated Upregulation of VEGF in Gastric Cancer. Tumour Biol (2016) 37(4):5493–501. doi: 10.1007/s13277-015-4372-4
Keywords: bibliometric analysis, γδ T cells, cancer prognosis, immune regulators, IL-17, IL-2
Citation: Liu B, He X, Wang Y, Huang J-w, Zheng Y-b, Li Y and Lu L-g (2022) Bibliometric Analysis of γδ T Cells as Immune Regulators in Cancer Prognosis. Front. Immunol. 13:874640. doi: 10.3389/fimmu.2022.874640
Received: 12 February 2022; Accepted: 15 March 2022;
Published: 14 April 2022.
Edited by:
Xiaoli Wu, Tianjin University, ChinaReviewed by:
Christoph W. Michalski, Ulm University Medical Center, GermanyMasayuki Tanaka, Keio University, Japan
Copyright © 2022 Liu, He, Wang, Huang, Zheng, Li and Lu. This is an open-access article distributed under the terms of the Creative Commons Attribution License (CC BY). The use, distribution or reproduction in other forums is permitted, provided the original author(s) and the copyright owner(s) are credited and that the original publication in this journal is cited, in accordance with accepted academic practice. No use, distribution or reproduction is permitted which does not comply with these terms.
*Correspondence: Yong Li, bG9ycnk1MTYwQDE2My5jb20=; Li-gong Lu, bHVfbGlnb25nQDE2My5jb20=