- 1Department of Rheumatology, The Second Affiliated Hospital of Zhejiang Chinese Medical University, Hangzhou, China
- 2School of Basic Medical Science, Zhejiang Chinese Medical University, Hangzhou, China
The cause of Systemic Lupus Erythematosus (SLE) remains largely unknown, despite the fact that it is well understood that a complex interaction between genes and environment is required for disease development. Microbiota serve as activators and are essential to immune homeostasis. Lactobacillus is thought to be an environmental agent affecting the development of SLE. However, beneficial therapeutic and anti-inflammatory effects of Lactobacillus on SLE were also explored. The discovery of Lactobacillus involvement in SLE will shed light on how SLE develops, as well as finding microbiota-targeted biomarkers and novel therapies. In this review, we attempt to describe the two sides of Lactobacillus in the occurrence, development, treatment and prognosis of SLE. We also discuss the effect of different strains Lactobacillus on immune cells, murine lupus, and patients. Finally, we try to illustrate the potential immunological mechanisms of Lactobacillus on SLE and provide evidence for further microbiota-targeted therapies.
Introduction
Systemic lupus erythematosus (SLE) is an autoimmune, multisystem disorder that leads to tissue damage involving almost every organ (1). The estimated worldwide prevalence of SLE is about 30-50/100,000 (2, 3) and it is larger in developing countries (4). The 10-year survival rate is about 90%, seriously affecting the physical and mental health and quality of life of patients (5). The adaptive immune system is dysregulated in SLE, resulting in a variety of abnormalities including as autoantibody production, activation of auto aggressive T effector cells and B cells, and a reduction in regulatory T cells (Tregs). SLE treatment includes glucocorticoids (GCs), antimalarial medicines, nonsteroidal anti-inflammatory drugs (NSAIDs), immunosuppressive agents, and B cell–targeting biologics and Chinese herbal medicine (6). However, the side effects such as gastrointestinal reaction, metabolic disorders and infections enable the doctors and scientists to seek new therapies. Recently, there were a large number of evidence about the relationship between the gut microbiota especially Lactobacillus spp. and SLE (7–9).
SLE is distinguished by the presence of high-titer serological autoantibodies, such as antibodies that bind to double-stranded DNA (dsDNA), SmRNP, SSA/Ro, and SSB/La (10). Bagavant et al. found that Aggregatibacter actinomycetemcomitans, Porphyromonas gingivalis, Treponema denticola, and the commensal Capnocytophaga ochracea have higher expression in SLE patients with higher anti-dsDNA antibody levels compared to patients without anti-dsDNA. Furthermore, Anti-SmRNP antibodies were shown to be related with increased anti-bacterial antibody titers against all the bacteria. However, anti-SSA/Ro and anti-SSB/La failed to associate with any of the periodontal bacteria (11).
The Lactobacilli, which are used commercially in the production of fermented foods, are an important component of the microbiota of human beings. Recently, Lactobacilli is thought to be an environmental agent affecting the development of SLE (12, 13). It was found that the Lactobacillus were significantly enriched in active SLE patients compared with healthy controls and rheumatoid arthritis (RA) patients in China (14, 15). Moreover, Lactobacillus reuteri exacerbated lupus-related pathogenesis in TLR7-dependent Mice (16). Particularly, women of reproductive age are nine times more likely than males to be diagnosed with SLE (10). Given that there is a link between sex and disease, gaining a better understanding of gender differences in gut microbiota will aid in interpreting therapeutic options. In MRL/lpr mice, Zhang H, et al. found females had significantly higher Lactobacillaceae and Streptococcaceae levels than did male mice. Importantly, retinoic acid improved lupus symptoms in MRL/lpr mice by restoring Lactobacillus spp. gut colonization (17).
There were also some recent studies on the oral microbiota of SLE. A preliminary study of Chinese SLE patients found that family Lactobacillaceae and the genus Lactobacillus were increased in patients with SLE (18). Actinomyces and Lactobacillus relative abundance in oral samples was substantially linked with their relative abundance in fecal samples. The difference in Lactobacillus relative abundance between SLE and healthy controls was significant in oral samples but not in fecal samples (19).
However, the variable effects of Lactobacilli on SLE highlight their dual role as beneficial or harmful depending on the context (20). In newly diagnosed SLE Egyptian patients, Lactobacillus showed a significant decrease (7). Several species of Lactobacilli can confer a range of beneficial effects on the host of SLE. For example, L. delbrueckii and L. rhamnosus have been shown to be effective in the elevation of Tregs and the decrease of inflammatory cytokines and disease severity in SLE‐induced mice (21). Mu et al. found that Lactobacillus spp. supplementation plays an anti-inflammatory role through reducing IL-6 and enhancing IL-10 generation in the intestine (22).
In order to understand the different roles of species of Lactobacilli and how the proper species of Lactobacilli can be targeted as therapy for SLE, we review prior previous findings regarding Lactobacillus in SLE patients and murine lupus models as well as the immune cells relevant to SLE. In addition, we will highlight the possible approaches to investigate the different species of Lactobacilli and to find the new way for microbiome-based precision medicine.
The Potential Immunological Mechanisms of Lactobacillus on SLE
Probiotics, including Lactobacillus species, favorably impact the host organism by enhancing and stabilizing gut flora (23). Lactobacillus might cling to intestinal epithelial cells and produce antibiotic chemicals, inhibiting the growth of dangerous germs. They can boost the nonspecific cellular immune response by activating macrophages, natural killer cells, and releasing numerous cytokines, as mentioned in the immune cells part. Moreover, they can boost the immune system of the gut mucosa by raising the number of IgA+ cells (24).
Lactobacillus adheres to the gastrointestinal mucosa by means of an interaction with toll-like receptors (25), by surface proteins and fatty acids (L. rhamnosus PEN) (26), and by proteinaceous surface layer components (L. plantarum 91) (27) to trigger the host’s immune response. Furthermore, they could upregulate tight-junction (TJ) proteins and MUC2, MUC3 and MUC1 in colonic epithelial cells and elevate butyrate levels, as well as microbiome modulation (28).
Lactobacilli can produce cytoprotective compounds, including short chain fatty acids, (SCFAs). SCFAs which act as local energy carriers for bacteria and gut epithelial cells, have emerged as key mediators in in the relationship between diet, gut microbiota, and human health (29, 30). SFCAs could stimulate the generation of retinoic acid by epithelial cells (31), a vitamin A-derived metabolite that cooperates with TGF-β to enhance Treg differentiation (32) and prevent Th17 cell differentiation (33). Studies have revealed several mechanisms through which retinoic acid treatment may modulate the course of SLE. For example, retinoic acid has been shown to restore the Lactobacilli in MRL/lpr mice and ameliorate the inflammatory symptoms of lupus (34, 35). In addition, retinoic acid suppresses the signaling of transcriptional regulators needed for lupus onset. For example, retinoic acid could inhibit prolyl isomerase Pin1, a regulator of IL-1 receptor-associated kinase 1 (IRAK1) in the TLR7/TLR9 signaling pathway. Moreover, interferon regulatory factor 7 (IRF-7) signaling, which has been linked to lupus progression, was also reported inhibited by retinoic acid (36).
Tryptophan is an important amino acid that some Lactobacilli strains convert into strong immune-modulating compounds that bind the Aryl hydrocarbon receptor (AhR). Tryptophanase-positive bacteria, such as Lactobacillus reuteri, produce various indole metabolites from dietary tryptophan that can stimulate AhR activity (37). AhR is a transcription factor found in the cytosol of a variety of cells. It has been observed that activating AhR regulates the methylation status of FoxP3 and IL-17 gene promoters and ameliorated experimental colitis (38). Recent research found considerable AhR activation in the peripheral blood of active SLE patients (39) (Figure 1).
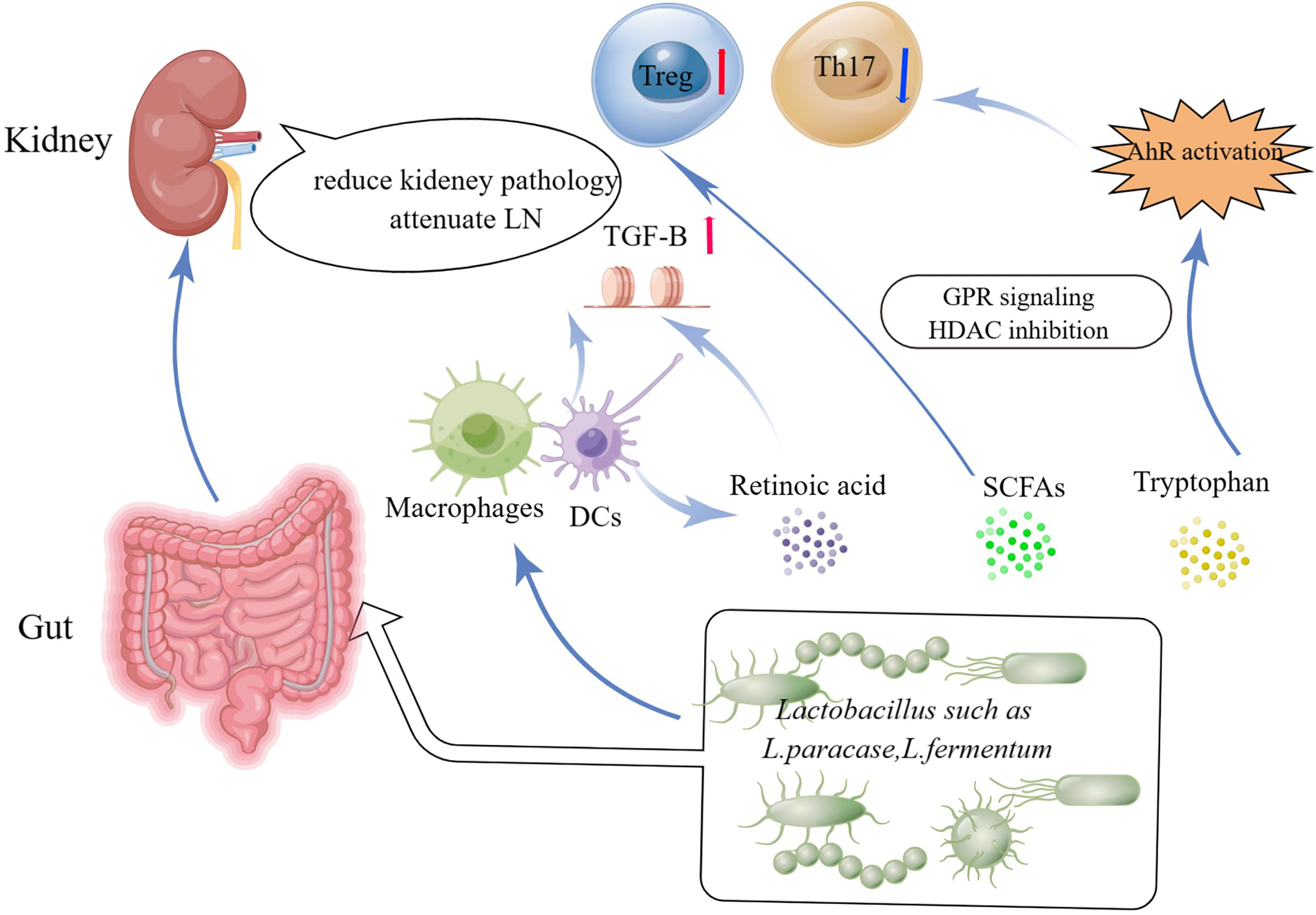
Figure 1 Potential beneficial functions of Lactobacillus and Lactobacillus-derived bioactive metabolites in the treatment of SLE and the communication pathways on Lactobacilli-kidney axis. HDACs, histone deacetylases; GPCRs, ligands for G-protein-coupled receptors; SCFAs, Short-chain fatty acids.
From Gut-Kidney Axis to Lactobacilli-Kidney axis
SLE is a chronic autoimmune disease that affects the kidneys in approximately half of all patients. Lupus nephritis (LN) is a significant risk factor for SLE morbidity and death (40). LN were linked to intestinal wall congestion, metabolic acidosis, frequent antibiotic usage, all of which have an influence on intestinal tight junctions and led to an increase in bacterial metabolic products crossing the intestinal barrier (41, 42). Moreover, the increased gastrointestinal urea output led in gut microbial dysbiosis. Furthermore, urea supplementation in drinking water related to changes in gut flora (43). Mu et al. and his colleagues demonstrated that a mixture of 5 Lactobacillus strains (Lactobacillus oris, Lactobacillus rhamnosus, Lactobacillus reuteri, Lactobacillus johnsonii, and Lactobacillus gasseri) restored the mucosal barrier function and reduced kidney pathology by increasing Treg cells and suppressing pathogenic Th17 cells (22). Future studies about Lactobacillus treatment in SLE patients are warranted to determine and needed (Figure 1).
Lactobacillus Are Altered in SLE Patients
Although there were few randomized controlled trials (RCTs) about the Lactobacillus’s effect on SLE patients, a large amount of cohort clinical studies on the relation between Lactobacillus and SLE were increasing. Newly diagnosed treatment-naive SLE cases (44) and SLE patients that had not received glucocorticoid (GC) therapy (45) possessed a decrease of Lactobacillus in feces in Chinese and Egyptian patients compared with the healthy controls. However, SLE patients in most cohorts both active and remissive, both adults and children all manifested the enrichment of Lactobacillus in both fecal and oral sample in China and Netherlands (14, 15, 18, 19, 46) (Table 1). Notably, Lactobacillus enriched in active SLE patients and lupus erythematosus skin lesions patients (47) as disease severity might play a role in abundance (14). It is hard to illustrate whether the Lactobacillus correlated with disease severity or higher dose of GC treatment. Moreover, SLE patients received GC therapy also have abundance in Lactobacillus (45). To illustrate the problem, more RCTs about the Lactobacillus’s effect on SLE patients should be recommended.
The Role of Lactobacillus on Immune Cells
Macrophages Especially Kidney Macrophages
In SLE, IgG antibodies induce inflammation and organ damage. Recent research suggests that inhibiting FcR-dependent macrophage activation may be an effective therapy strategy for SLE (48, 49). Inhibiting kidney macrophage glycolysis reduced autoantibody-induced inflammation, which may have therapeutic implications for lupus nephritis(LN) (50). Four Probiotic Lactobacillus strains (Lactobacillus rhamnosus GG, L. rhamnosus KLSD, L. helveticus IMAU70129, and L. casei IMAU60214) induced early proinflammatory cytokines such as IL-8, TNF-α, IL-12p70, and IL-6 and activated human macrophages. Moreover, the NF-κB pp65 and TLR2-dependent signaling were also increased by treatment with the strains (51). In addition, Lactobacillus salivarius strains induced cytokine responses that are Toll-like receptor2 (TLR2) independent but myeloid differentiation primary response88 (MyD88) dependent in both human and mouse macrophages with the upregulation of Tlr1, Tlr2 and Clec4e PRR genes (52). TLR-MyD88 pathway has been proved to be involved in the pathogenesis of SLE (53, 54). Therefore, some species of Lactobacillus may be beneficial for SLE through the pathway of TLR-MyD88 targeting on the kidney macrophages.
Dendritic Cells
SLE is associated with excessive uncontrolled inflammatory immunological responses that result in immune tolerance loss. Dendritic cells (DCs) are critical immune cells that govern immune responses. Renal DCs and macrophages contribute to chronic inflammation in LN. The involvement of these cells in SLE kidneys sparked interest when studies revealed that interstitial CD68+ mononuclear phagocyte infiltration is related with a worse prognosis in patients (55, 56). Several subtypes of these cells (CD103+ DCs, myeloid DCs, inflammatory Ly6Chi macrophages, and resident F4/80hi/Ly6Clo macrophages) have been identified by immunohistochemistry in LN biopsies (57) and by flow cytometry in mouse SLE models (58). In recent research, after treatment with GM-CSF and IL-4, together with L. delbrueckii and L. rhamnosus, the expression of indoleamine 2,3dioxygenase (IDO) and IL-10 increased, but IL-12 dropped. IDO was substantially expressed in mature DCs from SLE patients (59). Moreover, L. delbrueckii and L. rhamnosus could decrease expression of CXCR3, CCR5, CCR4, and CCR3 on the tolerogenic phenotype of DCs in SLE patients (60). Another study showed that Lactobacillus plantarum NCU116 increased the expression levels of Th17, Treg and specific transcription factors RORγt and Foxp3 in CTX-induced immunosuppression mice. In addition, it boosted the number of CD4+T cells and DCs considerably and enhanced the expression of Toll-like receptors (TLRs) (61).
B Cells
SLE is associated with B cell hyperactivity (62). BAFF (the B cell activating factor) over-expression has been commonly detected among patients with SLE and seems to be strongly involved in the pathogenesis of SLE (63). Belimumab (Benlysta®), which is the only biological agent currently approved for the treatment of SLE, has been used worldwide (64).The systemic review provided the evidence for the efficacy and good response of Belimumab. However, the adverse effects could not be reduced compared with the standard care (65). The findings on the effect of Lactobacillus to B cells could shed some light on reducing the gastrointestinal side effects when added into treat SLE. Studies showed that Lactobacillus rhamnosus (LGG) intervention can promote the development and function of B lymphocytes by increasing the expression of CD40, CD80 and MHC-II on B cell surface and activating the secretion of IgA and IgM as well as decreasing the IgG (66). In addition, a randomized trial demonstrated that LGG has an immediate effect in the human gut with upregulating genes related to B cell activation (67). Follicular helper T (Tfh) cells, which participate in the transmission of information in the process of B cell differentiation, assist in the activation of B cells. After administration of Lactobacillus paracasei MCC1849, IgA secretion was elevated and Follicular helper T (Tfh) cells was induced in the BALB/c mice. Moreover, MCC1849 boosted the expression of genes involved in Tfh cell development, such as IL-12p40, IL-10, IL-21, STAT4, and Bcl-6 (68).
T Cells
With age, B220+CD4−CD8− double-negative (DN) T cells increase in peripheral lymphoid organs of MRL/lpr mice. Lactobacillus casei was found to lower B220+ T cells to prevent the lymphadenopathy and prolong the lifespan of MRL/lpr mice (69). Regulatory T cells (Tregs) and Helper T cells (Ths) play a significant role in the control of immune responses and induction of peripheral tolerance. Dysregulation of Tregs and Th1/Th2/Th17 are involved in the pathogenesis of SLE. Many species of Lactobacillus have been reported to reprogram the CD4+ T cells into immunoregulatory T cells as well as regulating the Th cells and regulatory T cells. Marco Colonna and his colleagues showed that the Lactobacillus reuteri provides indole-3-lactic acid, that activate AhR and lead to inducing immunoregulatory T cells -gut intraepithelial CD4+CD8αα+ T cells (70). When pristane-induced lupus mice were administrated L. delbrueckii and L. rhamnosus,the disease severity was ameliorated by upregulating the Treg cells and decreasing anti-dsDNA and IL-6 (21). In addition, Lactobacillus sakei WIKIM30 was reported to ameliorate atopic dermatitis by decreasing the levels of Th2 cytokines (IL-4, IL-5, and IL-13) and enhancing Treg production and increasing the relative abundance of Treg-promoting bacteria (71). Moreover, Lactobacillus rhamnosus GG relieve allergies in mice by regulating the balance of T/B cells and Th1/Th2 and Th17/Treg cytokines (72). When Lactobacillus acidipiscis was orally administrated in a mouse model of experimental autoimmune encephalomyelitis (EAE), the generation of regulatory γδ T cells was induced and Th1 and Th17 cells decreased (73). Lactobacillus has been proved to modulate the Th17 cells in ameliorating many autoimmune and metabolic diseases such as SLE, hypertension, EAE, rheumatoid arthritis and colitis (74–78).
The Role of Lactobacillus on Animal Models of SLE
There was still a controversial issue on whether the changes in the microbiome communities in Lupus are direct drivers of pathogenesis. Interestingly, transplanting fecal samples from mice with a Lupus-like syndrome into germ-free mice induced higher increased levels of anti-dsDNA antibodies and encouraged an inflammatory alteration in recipient mice’s immune systems (79). Early and short-term antibiotics exposure exacerbated lupus severity by diminishing beneficial gut microbiota for lupus including Lactobacillus (80).
Mouse models of SLE can be divided into four groups: spontaneous models, induced models, genetically modified models, and humanized mouse models (81). Spontaneous mice models, which have a good genetic background and genetic stability, mainly include NZB/WF1 model, MRL model, BXSB model, Palmerston North mice, NZB/KN model, NZM model, BXD2 model. Among which, the NZB/WF1 model and MRL model were the most classic ones and commonly used in the research (82).
Lactobacillus abundance varies depending on the SLE animal models (MRL/lpr mice or NZB/WF1 mice). This might imply that interactions between gut microorganisms and the host, rather than the enrichment of specific gut microorganisms, are more important in the development of SLE. The gut microbiota of MRL/lpr lupus-prone mice are enriched in Lachnospiraceae and depleted in Lactobacillaceae (17). In MRL/lpr mice, Lactobacillus might play a preventive role in terms of lupus pathogenesis due to the relative absence before SLE onset. Moreover, Lactobacillus addition reduce proteinuria and autoantibodies and improve the renal condition in MRL/lpr mice (22). However, Lactobacillus play a contradictory role in NZB/WF1 mice. Luo et al. and his colleague investigated that the gut microbiota changed throughout the mouse lifetime in NZB/WF1 mice. The alterations were most noticeable before and after lupus onset. The relative abundance of Lactobacillus increased substantially during lupus development, which was reduced by dexamethasone. The relative abundance of Lactobacillus species was shown to be positively related to poorer renal function and higher-level systemic autoimmunity (83) (Figure 2).
Induced models of lupus include pristane induced lupus model, graft versus host disease induced lupus model, common human anti-DNA idiotype (16/6 id) induced lupus model, imiquimod/resiquimod induced lupus model, gut bacteria induced lupus model, anti-autoantibodies induced lupus glomerulonephritis model and environmental exposures induced lupus model. For example, resiquimod, Toll-like receptor 7/8 agonist, induced lupus model which were TLR7/9- dependent mice lupus models (82). Lactobacillus. reuteri increased over time in the feces of mice from both TLR7-dependent mice lupus models (16) as their disease progress and in the SLE patients (12) Moreover, L. reuteri worsens autoimmune manifestations by engaging type I interferon pathways in TLR7-dependent lupus mice. However, Lactobacillus fermentum CECT5716 was reported to prevent hypertension and endothelial dysfunction in lupus model mice induced by imiquimod, the agonist of TLR-7 (84).
On the other hand, the beneficial anti-inflammatory and immunoregulation effect of different strains of Lactobacilli were explored in NZB/W F1 mice (85–89) and MRL/lpr mice (22). Some of the studies focused on the therapeutic effect of Lactobacilli on organ damage such as on cardiac tissue (85, 87), liver (86) and the kidney (22). Moreover, enhancement of Tregs and the decrease of Th17 and Th1 cells were explored as the immunoregulation effect of Lactobacilli in pristane-induced mice, MRL/lpr mice, and NZB/W F1 mice models (21, 22, 88, 90) (Table 2).
Until now, there were few studies on the roles of Lactobacilli on genetically modified models, and humanized mouse models of lupus. In the future, more microbiota research especially about Lactobacilli are needed in these two models, which imitate the cross- interaction and immunological damage more similar to SLE patients.
Perspectives on Lactobacillus Treatment in SLE
Putting FMT Into Clinical Trials on SLE Would Shed Light on Its Beneficial Effects
With respect to the data on Lactobacillus, it is still a controversial issue on whether they would be potential immunoregulators in SLE. Although fecal microbiota transplant (FMT) has been used in several lupus models, TLR-7 dependent lupus model and MRL/lpr mice, and achieved satisfactory results resulting to restore the gut microbiota and ameliorate the lupus severity (80, 91). It is still a long way to put FMT into clinical trials on SLE owing to the ethic and patient’s wishes. Many probiotics researches are supported by commercial probiotic business groups. Lack of sufficient medical evidence and the commercial interests make objective interpretation of the Lactobacillus as probiotics close to impossible. However, recent microbiome findings, as well as the advent of innovative high-throughput sequencing and testing approaches, may allow us to concentrate on patient-oriented therapies.
Lactobacillus-Based Precision Medicine and Lactobacillus-Oriented Components in Food and Herbs May Have Great Potential to SLE Treatment
As glucocorticoids were still the most effective immunosuppressant and widely used for the treatment of SLE, Lactobacillus-based precision medicine would be a better adjuvant therapy to reduce the side effects. Zhixing He and his colleagues had proved that both the FMT of prednisone-treated MRL/lpr mice (92) and bromofuranone, inhibitor of AI-2 quorum sensing (93), could promote efficacy of prednisone and reduce the side effects through increasing the beneficial bacteria including Lactobacillus. Interestingly, orange intake was directly associated with Lactobacillus in non-active (SLEDAI score ≤ 8) Caucasian SLE patients (94). Hence, the low-fat/high-fiber diet and even the useful components Lactobacillus-oriented extracted from the orange or Chinese medicinal herbs along with the FMT would shed light on the treatment of SLE.
Comparative Proteomics or Transcriptomics Are Encouraged to Explore the Two Sides of the Lactobacillus
Different strains of Lactobacillus pose different effects even completely opposite. Interaction between different strains to produce an effect would also be another focusing point. Comparative proteomics or transcriptomics on the exact difference about the structure of the strain may provide new evidence on the treatment of SLE. To our best knowledge, although there were no adverse effects on the RCT trials of Lactobacillus on inflammatory bowel disease (95) and rheumatoid arthritis (96), the adverse effects of Lactobacillus as probiotics must be further investigated utilizing additional antibiotic regimens, probiotic strain combinations, and human microbiome transplants into germ-free mice. Therefore, the potential long-term clinical consequences of Lactobacillus-induced dysbiosis could be assessed.
Conclusions
This review shows that some beneficial strains of Lactobacillus have anti-inflammatory and immunoregulation effects on SLE and may become a better adjuvant therapy to reduce the side effects of the glucocorticoids and immunosuppressant. However, there are still many data on the harmful strains of Lactobacillus that contradicts this tendency. Since Lactobacillus could interact with the occurrence, development and treatment of SLE and different animal models of lupus, the question of whether Lactobacillus will be friend or foe could depend on the strain itself and the circumstances. Finally, we believe that we would witness useful medications and therapies about Lactobacillus on SLE in the future, when large cohort are available, and further studies might need to form national or even international randomized and blinded clinical trials.
Author Contributions
WW contributed to the conception of the study and wrote the first draft of the manuscript. XW and YF revised the manuscript. YF supervised the review. All authors contributed to manuscript revision, read and approved the submitted version.
Funding
This research was supported by National Natural Science Foundation of China (NO.82004238); Natural Science Foundation of Zhejiang Province (NO. LQ20H270007; LBY21H270001) and Zhejiang Medicine and Health Science and Technology Project (NO. 2021KY843).
Conflict of Interest
The authors declare that the research was conducted in the absence of any commercial or financial relationships that could be construed as a potential conflict of interest.
Publisher’s Note
All claims expressed in this article are solely those of the authors and do not necessarily represent those of their affiliated organizations, or those of the publisher, the editors and the reviewers. Any product that may be evaluated in this article, or claim that may be made by its manufacturer, is not guaranteed or endorsed by the publisher.
Acknowledgments
We would like to thank Figdraw (www.figdraw.com) for the image processing and Freescience for language editing.
References
1. Mu Q, Zhang H, Luo XM. SLE: Another Autoimmune Disorder Influenced by Microbes and Diet? Front Immunol (2015) 6:608. doi: 10.3389/fimmu.2015.00608
2. Ingvarsson RF, Bengtsson AA, Jönsen A. Variations in the Epidemiology of Systemic Lupus Erythematosus in Southern Sweden. Lupus (2016) 25:772–80. doi: 10.1177/0961203316635288
3. Tsioni V, Andreoli L, Meini A, Frassi M, Raffetti E, Airò P, et al. The Prevalence and Incidence of Systemic Lupus Erythematosus in Children and Adults: A Population-Based Study in a Mountain Community in Northern Italy. Clin Exp Rheumatol (2015) 33:681–7.
4. Li R, Sun J, Ren LM, Wang HY, Liu WH, Zhang XW, et al. Epidemiology of Eight Common Rheumatic Diseases in China: A Large-Scale Cross-Sectional Survey in Beijing. Rheumatol (Oxford England) (2012) 51:721–9. doi: 10.1093/rheumatology/ker370
5. Durcan L, O'Dwyer T, Petri M. Management Strategies and Future Directions for Systemic Lupus Erythematosus in Adults. Lancet (London England) (2019) 393:2332–43. doi: 10.1016/S0140-6736(19)30237-5
6. Thanou A, Jupe E, Purushothaman M, Niewold TB, Munroe ME. Clinical Disease Activity and Flare in SLE: Current Concepts and Novel Biomarkers. J Autoimmun (2021) 119:102615. doi: 10.1016/j.jaut.2021.102615
7. Hevia A, Milani C, López P, Cuervo A, Arboleya S, Duranti S, et al. Intestinal Dysbiosis Associated With Systemic Lupus Erythematosus. mBio (2014) 5:e01548–01514. doi: 10.1128/mBio.01548-14
8. Chen BD, Jia XM, Xu JY, Zhao LD, Ji JY, Wu BX, et al. An Autoimmunogenic and Proinflammatory Profile Defined by the Gut Microbiota of Patients With Untreated Systemic Lupus Erythematosus. Arthritis Rheumatol (Hoboken N.J.) (2021) 73:232–43. doi: 10.1002/art.41511
9. He Z, Shao T, Li H, Xie Z, Wen C. Alterations of the Gut Microbiome in Chinese Patients With Systemic Lupus Erythematosus. Gut Pathog (2016) 8:64. doi: 10.1186/s13099-016-0146-9
10. Ugarte-Gil MF, González LA, Alarcón GS. Lupus: The New Epidemic. Lupus (2019) 28:1031–50. doi: 10.1177/0961203319860907
11. Bagavant H, Dunkleberger ML, Wolska N, Sroka M, Rasmussen A, Adrianto I, et al. Antibodies to Periodontogenic Bacteria Are Associated With Higher Disease Activity in Lupus Patients. Clin Exp Rheumatol (2019) 37:106–11.
12. Kim JW, Kwok SK, Choe JY, Park SH. Recent Advances in Our Understanding of the Link Between the Intestinal Microbiota and Systemic Lupus Erythematosus. Int J Mol Sci (2019) 20(19):4871. doi: 10.3390/ijms20194871
13. Maeda Y, Takeda K. Host-Microbiota Interactions in Rheumatoid Arthritis. Exp Mol Med (2019) 51:1–6. doi: 10.1038/s12276-019-0283-6
14. Liu F, Ren T, Li X, Zhai Q, Xu X, Zhang N, et al. Distinct Microbiomes of Gut and Saliva in Patients With Systemic Lupus Erythematous and Clinical Associations. Front Immunol (2021) 12:626217. doi: 10.3389/fimmu.2021.626217
15. Li Y, Wang HF, Li X, Li HX, Zhang Q, Zhou HW, et al. Disordered Intestinal Microbes Are Associated With the Activity of Systemic Lupus Erythematosus. Clin Sci (London Engl 1979) (2019) 133:821–38. doi: 10.1042/CS20180841
16. Zegarra-Ruiz DF, El Beidaq A, Iñiguez AJ, Lubrano Di Ricco M, Manfredo Vieira S, Ruff WE, et al. A Diet-Sensitive Commensal Lactobacillus Strain Mediates TLR7-Dependent Systemic Autoimmunity. Cell Host Microbe (2019) 25:113–27.e116. doi: 10.1016/j.chom.2018.11.009
17. Zhang H, Liao X, Sparks JB, Luo XM. Dynamics of Gut Microbiota in Autoimmune Lupus. Appl Environ Microbiol (2014) 80:7551–60. doi: 10.1128/AEM.02676-14
18. Li BZ, Zhou HY, Guo B, Chen WJ, Tao JH, Cao NW, et al. Dysbiosis of Oral Microbiota Is Associated With Systemic Lupus Erythematosus. Arch Oral Biol (2020) 113:104708. doi: 10.1016/j.archoralbio.2020.104708
19. van der Meulen TA, Harmsen HJM, Vila AV, Kurilshikov A, Liefers SC, Zhernakova A, et al. Shared Gut, But Distinct Oral Microbiota Composition in Primary Sjögren's Syndrome and Systemic Lupus Erythematosus. J Autoimmun (2019) 97:77–87. doi: 10.1016/j.jaut.2018.10.009
20. Fine RL, Mubiru DL, Kriegel MA. Friend or Foe? Lactobacillus in the Context of Autoimmune Disease. Adv Immunol (2020) 146:29–56. doi: 10.1016/bs.ai.2020.02.002
21. Khorasani S, Mahmoudi M, Kalantari MR, Lavi Arab F, Esmaeili SA., Mardani F, et al. Amelioration of Regulatory T Cells by Lactobacillus Delbrueckii and Lactobacillus Rhamnosus in Pristane-Induced Lupus Mice Model. J Cell Physiol (2019) 234:9778–86. doi: 10.1002/jcp.27663
22. Mu Q, Zhang H, Liao X, Lin K, Liu H, Edwards MR, et al. Control of Lupus Nephritis by Changes of Gut Microbiota. Microbiome (2017) 5:73. doi: 10.1186/s40168-017-0300-8
23. Reid G. Probiotics: Definition, Scope and Mechanisms of Action. Best Practice & Research. Clin Gastroenterol (2016) 30:17–25. doi: 10.1016/j.bpg.2015.12.001
24. Ashraf R, Shah NP. Immune System Stimulation by Probiotic Microorganisms. Crit Rev Food Sci Nutr (2014) 54:938–56. doi: 10.1080/10408398.2011.619671
25. Rescigno M. The Intestinal Epithelial Barrier in the Control of Homeostasis and Immunity. Trends Immunol (2011) 32:256–64. doi: 10.1016/j.it.2011.04.003
26. Polak-Berecka M, Waśko A, Paduch R, Skrzypek T, Sroka-Bartnicka A. The Effect of Cell Surface Components on Adhesion Ability of Lactobacillus Rhamnosus. Antonie van Leeuwenhoek (2014) 106:751–62. doi: 10.1007/s10482-014-0245-x
27. Yadav AK, Tyagi A, Kumar A, Saklani AC, Grover S, Batish VK. Adhesion of Indigenous Lactobacillus Plantarum to Gut Extracellular Matrix and Its Physicochemical Characterization. Arch Microbiol (2015) 197:155–64. doi: 10.1007/s00203-014-1034-7
28. Ohland CL, Macnaughton WK. Probiotic Bacteria and Intestinal Epithelial Barrier Function. Am J of Physiol Gastrointest Liver Physiol (2010) 298:G807–819. doi: 10.1152/ajpgi.00243.2009
29. Ríos-Covián D, Ruas-Madiedo P, Margolles A, Gueimonde M, de LosReyes-Gavilán CG, Salazar N. Intestinal Short Chain Fatty Acids and Their Link With Diet and Human Health. Front Microbiol (2016) 7:185. doi: 10.3389/fmicb.2016.00185
30. Morrison DJ, Preston T. Formation of Short Chain Fatty Acids by the Gut Microbiota and Their Impact on Human Metabolism. Gut Microbes (2016) 7:189–200. doi: 10.1080/19490976.2015.1134082
31. Brun PJ, Grijalva A, Rausch R, Watson E, Yuen JJ, Das BC, et al. Retinoic Acid Receptor Signaling Is Required to Maintain Glucose-Stimulated Insulin Secretion and β-Cell Mass. Faseb j (2015) 29:671–83. doi: 10.1096/fj.14-256743
32. Wang Y, Zhong YJ, Wang YY, Xing J, Wang ZM. All-Trans Retinoic Acid Prevents the Development of Type 1 Diabetes by Affecting the Levels of Interferon Gamma and Interleukin 4 in Streptozotocin-Induced Murine Diabetes Model. Genet Mol Res (2016) 15:1–9. doi: 10.4238/gmr.15017522
33. Juang JH, Van YH, Kuo CH, Lin MY, Liu YH, Chang HY. Prevention and Reversal of Diabetes by All-Trans Retinoid Acid and Exendin-4 in NOD Mice. Int J Endocrinol (2014) 2014:435481. doi: 10.1155/2014/435481
34. Pérez de Lema G, Lucio-Cazaña FJ, Molina A, Luckow B, Schmid H, de Wit C, et al. Retinoic Acid Treatment Protects MRL/lpr Lupus Mice From the Development of Glomerular Disease. Kidney Int (2004) 66:1018–28. doi: 10.1111/j.1523-1755.2004.00850.x
35. Theus MH, Sparks JB, Liao X, Ren J, Luo XM. All- Trans-Retinoic Acid Augments the Histopathological Outcome of Neuroinflammation and Neurodegeneration in Lupus-Prone MRL/lpr Mice. J Histochem Cytochem (2017) 65:69–81. doi: 10.1369/0022155416679638
36. Wei S, Yoshida N, Finn G, Kozono S, Nechama M, Kyttaris VC, et al. Pin1-Targeted Therapy for Systemic Lupus Erythematosus. Arthritis Rheumatol (Hoboken N.J.) (2016) 68:2503–13. doi: 10.1002/art.39741
37. Zelante T, Iannitti RG, Cunha C, De Luca A, Giovannini G, Pieraccini G, et al. Tryptophan Catabolites From Microbiota Engage Aryl Hydrocarbon Receptor and Balance Mucosal Reactivity via Interleukin-22. Immunity (2013) 39:372–85. doi: 10.1016/j.immuni.2013.08.003
38. Singh NP, Singh UP, Singh B, Price RL, Nagarkatti M, Nagarkatti PS. Activation of Aryl Hydrocarbon Receptor (AhR) Leads to Reciprocal Epigenetic Regulation of FoxP3 and IL-17 Expression and Amelioration of Experimental Colitis. PloS One (2011) 6:e23522. doi: 10.1371/journal.pone.0023522
39. Dorgham K, Amoura Z, Parizot C, Arnaud L, Frances C, Pionneau C, et al. Ultraviolet Light Converts Propranolol, A Nonselective β-Blocker and Potential Lupus-Inducing Drug, Into a Proinflammatory AhR Ligand. Eur J Immunol (2015) 45:3174–87. doi: 10.1002/eji.201445144
40. Almaani S, Meara A, Rovin BH. Update on Lupus Nephritis. Clin J Am Soc Nephrol (2017) 12:825–35. doi: 10.2215/CJN.05780616
41. Sabatino A, Regolisti G, Brusasco I, Cabassi A, Morabito S, Fiaccadori E. Alterations of Intestinal Barrier and Microbiota in Chronic Kidney Disease. Nephrology Dialysis Transplant (2015) 30:924–33. doi: 10.1093/ndt/gfu287
42. Chen YY, Chen DQ, Chen L, Liu JR, Vaziri ND, Guo Y, et al. Microbiome-Metabolome Reveals the Contribution of Gut-Kidney Axis on Kidney Disease. J Trans Med (2019) 17:5. doi: 10.1186/s12967-018-1756-4
43. Chaves LD, McSkimming DI, Bryniarski MA, Honan AM, Abyad S, Thomas SA, et al. Chronic Kidney Disease, Uremic Milieu, and Its Effects on Gut Bacterial Microbiota Dysbiosis. Am J Physiol Renal Physiol (2018) 315:F487–502. doi: 10.1152/ajprenal.00092.2018
44. Gerges MA, Esmaeel NE, Makram WK, Sharaf DM, Gebriel MG. Altered Profile of Fecal Microbiota in Newly Diagnosed Systemic Lupus Erythematosus Egyptian Patients. Int J Microbiol (2021) 2021:9934533. doi: 10.1155/2021/9934533
45. Guo M, Wang H, Xu S, Zhuang Y, An J, Su C, et al. Alteration in Gut Microbiota Is Associated With Dysregulation of Cytokines and Glucocorticoid Therapy in Systemic Lupus Erythematosus. Gut Microbes (2020) 11:1758–73. doi: 10.1080/19490976.2020.1768644
46. Wen M, Liu T, Zhao M, Dang X, Feng S, Ding X, et al. Correlation Analysis Between Gut Microbiota and Metabolites in Children With Systemic Lupus Erythematosus. J Immunol Res (2021) 2021:5579608. doi: 10.1155/2021/5579608
47. Zhao Y, Zhang B, Liu C, Ren G. Method for Accurate Diagnose of Lupus Erythematosus Skin Lesions Based on Microbial rDNA Sequencing. Saudi J Biol Sci (2020) 27:2111–5. doi: 10.1016/j.sjbs.2020.05.035
48. Kaneko Y, Nimmerjahn F, Madaio MP, Ravetch JV. Pathology and Protection in Nephrotoxic Nephritis is Determined by Selective Engagement of Specific Fc Receptors. J Exp Med (2006) 203:789–97. doi: 10.1084/jem.20051900
49. Brownlie RJ, Lawlor KE, Niederer HA, Cutler AJ, Xiang Z, Clatworthy MR, et al. Distinct Cell-Specific Control of Autoimmunity and Infection by FcgammaRIIb. J Exp Med (2008) 205:883–95. doi: 10.1084/jem.20072565
50. Jing C, Castro-Dopico T, Richoz N, Tuong ZK, Ferdinand JR, Lok LSC, et al. Macrophage Metabolic Reprogramming Presents a Therapeutic Target in Lupus Nephritis. Proc Natl Acad Sci USA (2020) 117:15160–71. doi: 10.1073/pnas.2000943117
51. Rocha-Ramírez LM, Pérez-Solano RA, Castañón-Alonso SL, Moreno Guerrero SS, Ramírez Pacheco A, García Garibay M, et al. Probiotic Lactobacillus Strains Stimulate the Inflammatory Response and Activate Human Macrophages. J Immunol Res (2017) 2027:4607491. doi: 10.1155/2017/4607491
52. Udayan S, Buttó LF, Rossini V, Velmurugan J, Martinez-Lopez M, Sancho D, et al. Macrophage Cytokine Responses to Commensal Gram-Positive Lactobacillus Salivarius Strains are TLR2-Independent and Myd88-Dependent. Sci Rep (2021) 11:5896. doi: 10.1038/s41598-021-85347-7
53. Ehlers M, Fukuyama H, McGaha TL, Aderem A, Ravetch JV. TLR9/MyD88 Signaling Is Required for Class Switching to Pathogenic IgG2a and 2b Autoantibodies in SLE. J Exp Med (2006) 203:553–61. doi: 10.1084/jem.20052438
54. Pacheco GV, Novelo Noh IB, Velasco Cárdenas RM, Angulo Ramírez AV, López Villanueva RF, Quintal Ortiz IG, et al. Expression of TLR-7, MyD88, NF-Kb, and INF-α in B Lymphocytes of Mayan Women With Systemic Lupus Erythematosus in Mexico. Front Immunol (2016) 7:22. doi: 10.3389/fimmu.2016.00022
55. Hill GS, Delahousse M, Nochy D, Rémy P, Mignon F, Méry JP, et al. Predictive Power of the Second Renal Biopsy in Lupus Nephritis: Significance of Macrophages. Kidney Int (2001) 59:304–16. doi: 10.1046/j.1523-1755.2001.00492.x
56. Dias CB, Malafronte P, Lee J, Resende A, Jorge L, Pinheiro CC, et al. Role of Renal Expression of CD68 in the Long-Term Prognosis of Proliferative Lupus Nephritis. J Nephrol (2017) 30:87–94. doi: 10.1007/s40620-015-0252-7
57. Berthier CC, Bethunaickan R, Gonzalez-Rivera T, Nair V, Ramanujam M, Zhang W, et al. Cross-Species Transcriptional Network Analysis Defines Shared Inflammatory Responses in Murine and Human Lupus Nephritis. J Immunol (2012) 189:988–1001. doi: 10.4049/jimmunol.1103031
58. Maria NI, Davidson A. Renal Macrophages and Dendritic Cells in SLE Nephritis. Curr Rheumatol Rep (2017) 19:81. doi: 10.1007/s11926-017-0708-y
59. Esmaeili SA, Mahmoudi M, Rezaieyazdi Z, Sahebari M, Tabasi N, Sahebkar A, et al. Generation of Tolerogenic Dendritic Cells Using Lactobacillus Rhamnosus and Lactobacillus Delbrueckii as Tolerogenic Probiotics. J Cell Biochem (2018) 119:7865–72. doi: 10.1002/jcb.27203
60. Esmaeili SA, Taheri RA, Mahmoudi M, Momtazi-Borojeni AA, Morshedi M, Bahramifar A, et al. Inhibitory Effects of Tolerogenic Probiotics on Migratory Potential of Lupus Patient-Derived DCs. Iranian J basic Med Sci (2021) 24:1509–14. doi: 10.22038/ijbms.2021.58438.12982
61. Xie J, Nie S, Yu Q, Yin J, Xiong T, Gong D, et al. Lactobacillus Plantarum NCU116 Attenuates Cyclophosphamide-Induced Immunosuppression and Regulates Th17/Treg Cell Immune Responses in Mice. J Agric Food Chem (2016) 64:1291–7. doi: 10.1021/acs.jafc.5b06177
62. Domeier PP, Rahman ZSM. Regulation of B Cell Responses in SLE by Three Classes of Interferons. Int J Mol Sci (2021) 22:10464. doi: 10.3390/ijms221910464
63. Möckel T, Basta F, Weinmann-Menke J, Schwarting A. B Cell Activating Factor (BAFF): Structure, Functions, Autoimmunity and Clinical Implications in Systemic Lupus Erythematosus (SLE). Autoimmun Rev (2021) 20:102736. doi: 10.1016/j.autrev.2020.102736
64. Blair HA, Duggan ST. Belimumab: A Review in Systemic Lupus Erythematosus. Drugs (2018) 78:355–66. doi: 10.1007/s40265-018-0872-z
65. Singh JA, Shah NP, Mudano AS. Belimumab for Systemic Lupus Erythematosus. Cochrane Database systematic Rev (2021) 2:Cd010668. doi: 10.1002/14651858.CD010668.pub2
66. Shi CW, Zeng Y, Yang GL, Jiang YL, Yang WT, Chen YQ, et al. Effect of Lactobacillus Rhamnosus on the Development of B Cells in Gut-Associated Lymphoid Tissue of BALB/c Mice. J Cell Mol Med (2020) 24:8883–6. doi: 10.1111/jcmm.15574
67. Bornholdt J, Broholm C, Chen Y, Rago A, Sloth S, Hendel J, et al. Personalized B Cell Response to the Lactobacillus Rhamnosus GG Probiotic in Healthy Human Subjects: A Randomized Trial. Gut Microbes (2020) 12:1–14. doi: 10.1080/19490976.2020.1854639
68. Arai S, Iwabuchi N, Takahashi S, Xiao JZ, Abe F, Hachimura S. Orally Administered Heat-Killed Lactobacillus Paracasei MCC1849 Enhances Antigen-Specific IgA Secretion and Induces Follicular Helper T Cells in Mice. PloS One (2018) 13:e0199018. doi: 10.1371/journal.pone.0199018
69. Mike A, Nagaoka N, Tagami Y, Miyashita M, Shimada S, Uchida K, et al. Prevention of B220+ T Cell Expansion and Prolongation of Lifespan Induced by Lactobacillus Casei in MRL/lpr Mice. Clin Exp Immunol (1999) 117:368–75. doi: 10.1046/j.1365-2249.1999.00951.x
70. Cervantes-Barragan L, Chai JN, Tianero MD, Di Luccia B, Ahern PP, Merriman J, et al. Lactobacillus Reuteri Induces Gut Intraepithelial Cd4(+)Cd8αα(+) T Cells. Sci (New York N.Y.) (2017) 357:806–10. doi: 10.1126/science.aah5825
71. Kwon MS, Lim SK, Jang JY, Lee J, Park HK, Kim N, et al. Lactobacillus Sakei WIKIM30 Ameliorates Atopic Dermatitis-Like Skin Lesions by Inducing Regulatory T Cells and Altering Gut Microbiota Structure in Mice. Front Immunol (2018) 9:1905. doi: 10.3389/fimmu.2018.01905
72. Chen X, Zhao X, Hu Y, Zhang B, Zhang Y, Wang S. Lactobacillus Rhamnosus GG Alleviates β-Conglycinin-Induced Allergy by Regulating the T Cell Receptor Signaling Pathway. Food Funct (2020) 11:10554–67. doi: 10.1039/d0fo02124e
73. Ren S, Zhang X, Guan H, Wu L, Yu M, Hou D, et al. Lactobacillus Acidipiscis Induced Regulatory Gamma Delta T Cells and Attenuated Experimental Autoimmune Encephalomyelitis. Front Immunol (2021) 12:623451. doi: 10.3389/fimmu.2021.623451
74. Kim DS, Park Y, Choi JW, Park SH, Cho ML, Kwok SK. Lactobacillus Acidophilus Supplementation Exerts a Synergistic Effect on Tacrolimus Efficacy by Modulating Th17/Treg Balance in Lupus-Prone Mice via the SIGNR3 Pathway. Front Immunol (2021) 12:696074. doi: 10.3389/fimmu.2021.696074
75. Wilck N, Matus MG, Kearney SM, Olesen SW, Forslund K, Bartolomaeus H, et al. Salt-Responsive Gut Commensal Modulates T(H)17 Axis and Disease. Nature (2017) 551:585–9. doi: 10.1038/nature24628
76. Jia L, Wu R, Han N, Fu J, Luo Z, Guo L, et al. Porphyromonas Gingivalis and Lactobacillus Rhamnosus GG Regulate the Th17/Treg Balance in Colitis via TLR4 and TLR2. Clin Trans Immunol (2020) 9:e1213. doi: 10.1002/cti2.1213
77. Jhun J, Min HK, Ryu J, Lee SY, Ryu JG, Choi JW, et al. Lactobacillus Sakei Suppresses Collagen-Induced Arthritis and Modulates the Differentiation of T Helper 17 Cells and Regulatory B Cells. J Trans Med (2020) 18:317. doi: 10.1186/s12967-020-02477-8
78. Berer K, Mues M, Koutrolos M, Rasbi ZA, Boziki M, Johner C, et al. Commensal Microbiota and Myelin Autoantigen Cooperate to Trigger Autoimmune Demyelination. Nature (2011) 479:538–41. doi: 10.1038/nature10554
79. Ma Y, Xu X, Li M, Cai J, Wei Q, Niu H. Gut Microbiota Promote the Inflammatory Response in the Pathogenesis of Systemic Lupus Erythematosus. Mol Med (2019) 25:35. doi: 10.1186/s10020-019-0102-5
80. Zhang Y, Liu Q, Yu Y, Wang M, Wen C, He Z. Early and Short-Term Interventions in the Gut Microbiota Affects Lupus Severity, Progression, and Treatment in MRL/lpr Mice. Front Microbiol (2020) 11:628. doi: 10.3389/fmicb.2020.00628
81. Perry D, Sang A, Yin Y, Zheng YY, Morel L. Murine Models of Systemic Lupus Erythematosus. J Biomedicine Biotechnol (2011) 2021:271694. doi: 10.1155/2011/271694
82. Halkom A, Wu H, Lu Q. Contribution of Mouse Models in Our Understanding of Lupus. Int Rev Immunol (2020) 39:174–87. doi: 10.1080/08830185.2020.1742712
83. Luo XM, Edwards MR, Mu Q, Yu Y, Vieson MD, Reilly CM, et al. Gut Microbiota in Human Systemic Lupus Erythematosus and a Mouse Model of Lupus. Appl Environ Microbiol (2018) 84:e02288–17. doi: 10.1128/AEM.02288-17
84. de la Visitación N, Robles-Vera I, Moleón-Moya J, Sánchez M, Jiménez R, Gómez-Guzmán M, et al. Probiotics Prevent Hypertension in a Murine Model of Systemic Lupus Erythematosus Induced by Toll-Like Receptor 7 Activation. Nutrients (2021) 13:2669. doi: 10.3390/nu13082669
85. Hu WS, Rajendran P, Tzang BS, Yeh YL, Shen CY, Chen RJ, et al. Lactobacillus Paracasei GMNL-32 Exerts a Therapeutic Effect on Cardiac Abnormalities in NZB/W F1 Mice. PloS One (2017) 12:e0185098. doi: 10.1371/journal.pone.0185098
86. Hsu TC, Huang CY, Liu CH, Hsu KC, Chen YH, Tzang BS. Lactobacillus Paracasei GMNL-32, Lactobacillus Reuteri GMNL-89 and L. Reuteri GMNL-263 Ameliorate Hepatic Injuries in Lupus-Prone Mice. Br J Nutr (2017) 117:1066–74. doi: 10.1017/S0007114517001039
87. Toral M, Robles-Vera I, Romero M, de laVisitación N, Sánchez M, O'Valle F, et al. Lactobacillus Fermentum CECT5716: A Novel Alternative for the Prevention of Vascular Disorders in a Mouse Model of Systemic Lupus Erythematosus. FASEB J (2019) 33:10005–18. doi: 10.1096/fj.201900545RR
88. Tzang BS, Liu CH, Hsu KC, Chen YH, Huang CY, Hsu TC. Effects of Oral Lactobacillus Administration on Antioxidant Activities and CD4+CD25+forkhead Box P3 (FoxP3)+ T Cells in NZB/W F1 Mice. Br J Nutr 2017 (2017) 118:333–42. doi: 10.1017/S0007114517002112
89. Yeh YL, Lu MC, Tsai BC, Tzang BS, Cheng SM, Zhang X, et al. Heat-Killed Lactobacillus Reuteri GMNL-263 Inhibits Systemic Lupus Erythematosus-Induced Cardiomyopathy in NZB/W F1 Mice. Probiotics Antimicrobial Proteins (2021) 13:51–9. doi: 10.1007/s12602-020-09668-1
90. Mardani F, Mahmoudi M, Esmaeili SA, Khorasani S, Tabasi N, Rastin M. In Vivo Study: Th1-Th17 Reduction in Pristane-Induced Systemic Lupus Erythematosus Mice After Treatment With Tolerogenic Lactobacillus Probiotics. J Cell Physiol (2018) 234:642–9. doi: 10.1002/jcp.26819
91. de la Visitación N, Robles-Vera I, Moleón J, González-Correa C, Aguilera-Sánchez N, Toral M, et al. Gut Microbiota Has a Crucial Role in the Development of Hypertension and Vascular Dysfunction in Toll-Like Receptor 7-Driven Lupus Autoimmunity. Antioxidants (Basel Switzerland) (2021) 10:1426. doi: 10.3390/antiox10091426
92. Wang M, Zhu Z, Lin X, Li H, Wen C, Bao J, et al. Gut Microbiota Mediated the Therapeutic Efficacies and the Side Effects of Prednisone in the Treatment of MRL/lpr Mice. Arthritis Res Ther (2021) 23:240. doi: 10.1186/s13075-021-02620-w
93. He Z, Kong X, Shao T, Zhang Y, Wen C. Alterations of the Gut Microbiota Associated With Promoting Efficacy of Prednisone by Bromofuranone in MRL/lpr Mice. Front Microbiol (2019) 10:978. doi: 10.3389/fmicb.2019.00978
94. Cuervo A, Hevia A, López P, Suárez A, Sánchez B, Margolles A, et al. Association of Polyphenols From Oranges and Apples With Specific Intestinal Microorganisms in Systemic Lupus Erythematosus Patients. Nutrients (2015) 7:1301–17. doi: 10.3390/nu7021301
95. Yılmaz İ, Dolar ME, Özpınar H. Effect of Administering Kefir on the Changes in Fecal Microbiota and Symptoms of Inflammatory Bowel Disease: A Randomized Controlled Trial. Turkish J Gastroenterol (2019) 30:242–53. doi: 10.5152/tjg.2018.18227
Keywords: Lactobacillus, systemic lupus erythematosus (SLE), immunoregulators, pathogenesis, microbiota
Citation: Wang W, Fan Y and Wang X (2022) Lactobacillus: Friend or Foe for Systemic Lupus Erythematosus? Front. Immunol. 13:883747. doi: 10.3389/fimmu.2022.883747
Received: 25 February 2022; Accepted: 26 April 2022;
Published: 23 May 2022.
Edited by:
Lidan Zhao, Peking Union Medical College Hospital (CAMS), ChinaReviewed by:
Gislane Lelis Vilela de Oliveira, São Paulo State University, BrazilGuobing Chen, Jinan University, China
Copyright © 2022 Wang, Fan and Wang. This is an open-access article distributed under the terms of the Creative Commons Attribution License (CC BY). The use, distribution or reproduction in other forums is permitted, provided the original author(s) and the copyright owner(s) are credited and that the original publication in this journal is cited, in accordance with accepted academic practice. No use, distribution or reproduction is permitted which does not comply with these terms.
*Correspondence: Weijie Wang, amFjazE5ODcxNjhAMTYzLmNvbQ==; Xinchang Wang, b3NzYW5pQDEyNi5jb20=