- 1Department of Integrated Traditional and Western Nephrology, the First Affiliated Hospital of Zhengzhou University, Research Institute of Nephrology, Zhengzhou University, Henan Province Research Center For Kidney Disease, Key Laboratory of Precision Diagnosis and Treatment for Chronic Kidney Disease in Henan Province, Zhengzhou, China
- 2Engineering Research Center of Agricultural Microbiology Technology, Ministry of Education, Heilongjiang University, Harbin, China
- 3Heilongjiang Provincial Key Laboratory of Ecological Restoration and Resource Utilization for Cold Region, School of Life Sciences, Heilongjiang University, Harbin, China
- 4School of Mechanical and Aerospace Engineering, Nanyang Technological University, Singapore, Singapore
Cancer immunotherapy has emerged as a novel anti-tumor treatment. Despite significant breakthroughs, cancer immunotherapy remains focused on several types of tumors that are sensitive to the immune system. Therefore, effective strategies to expand its indications and improve its efficacy become key factors for the further development of cancer immunotherapy. In recent decades, the anticancer activities of natural products are reported to have this effect on cancer immunotherapy. And the mechanism is largely attributed to the remodeling of the tumor immunosuppressive microenvironment. The compelling data highlight that natural products offer an alternative method option to improve immune function in the tumor microenvironment (TME). Currently, more attention is being paid to the discovery of new potential modulators of tumor immunotherapy from natural products. In this review, we describe current advances in employing natural products and natural small-molecule drugs targeting immune cells to avoid tumor immune escape, which may bring some insight for guiding tumor treatment.
Introduction
The immune system is a sophisticated integrated network consisted of various immune cells, organs, and soluble mediators that developed to protect the biosome against outside assault which threatens the integrity of biosome (1). The immune system’s critical role in host defense is best seen when things go wrong; underactivity leads to severe infections and tumors, while abnormal activity leads to allergy and autoimmune illnesses, especially tumor escape. Immune cells are an important part of the immune system, including lymphocytes, dendritic cells (DCs), monocytes/macrophages, natural killer (NK) cells, and so on (2). It is now clear that in addition to cancer cells, the tumor microenvironment (TME) contains a repertoire of endothelial cells, stromal cells and immune cells (3). In contrast to traditional chemotherapy, immunotherapy focuses on the specific recognition and attack of cancer cells using immune cells inside and outside of TME (4). Thus, immunotherapy approaches are theoretically shown to have higher specificity and lower side effects.
Cancer immunotherapy is defined that regulates immunological response through activating the organism’s immune defense system to suppress and prevent tumor growth (5). Now, a variety of cancer vaccines, immune-checkpoint inhibitors and adoptive immune-cell immunotherapies for anti-cancer treatments are approved by the US Food and Drug Administration (FDA) (6–8). Despite significant breakthroughs, cancer immunotherapy remains focused on several types of tumors that are sensitive to the immune system. The main reason for this limitation is the immunosuppressive microenvironment within the tumor site, which effectively blunts cancer immunotherapy (9). Therefore, effective strategies that can reverse and reshape the complex immunosuppressive microenvironment within tumors are a key factor in expanding the indications for cancer immunotherapy.
Natural products used to treat human diseases can date back over 3000 years and contain several active components found in medicinal plants. Furthermore, mangy natural products were verified and documented in several publications in ancient China. Numerous studies currently have demonstrated that natural products play an important role in the development of new anti-cancer drugs and lead compounds due to their wide range of sources, low cost, structural diversity, diverse biological activities and low adverse effects (10, 11). Several well-known natural products, including polysaccharides (e.g., astragalus polysaccharides, shiitake polysaccharides), alkaloids (e.g., matrine, berberine), saponins (e.g., ginsenoside, total saponin of acanthopanax bark), flavonoids (e.g., baicalin, apigenin), and terpenoids (e.g., artemisinin, paclitaxel) have potential anti-tumor immunomodulatory effects (12) (Table 1). In addition, natural products have achieved great success in effectively expanding the indications and improving the efficacy of various types of cancer immunotherapy, such as immune checkpoint inhibitors, cancer vaccines, and adoptive immune cell transfer therapy (13–15). In this paper, we describe the immunomodulatory effects of natural products, as well as the underlying processes of the immune response activation in TME (16–18).
Immune modulation of natural products to immune cells
In general, the immune cells that have an effect on tumors can be divided into two types: tumor-promoting and tumor-antagonizing immune cells. These two types of cells play various roles at different stages of tumor progression (3, 19). The tumor-antagonizing immune cells mainly consist of NK cells, effector T cells (including effector CD4+ T cells and CD8+ cytotoxic T cells), M1-polarized macrophages and DCs. Except for the tumor-antagonizing immune cells, there are a plenty of tumor-promoting immune cells mainly consisting of regulatory T cells (Tregs) (4) (Figure 1). Natural products can activate specific intrinsic immune cells to kill tumor cells by enhancing antigen presentation or cellular immune processes. They can also inhibit the formation of blood vessels in the TME and the metastasis of tumors by suppressing certain intrinsic immune cells (Figure 2).
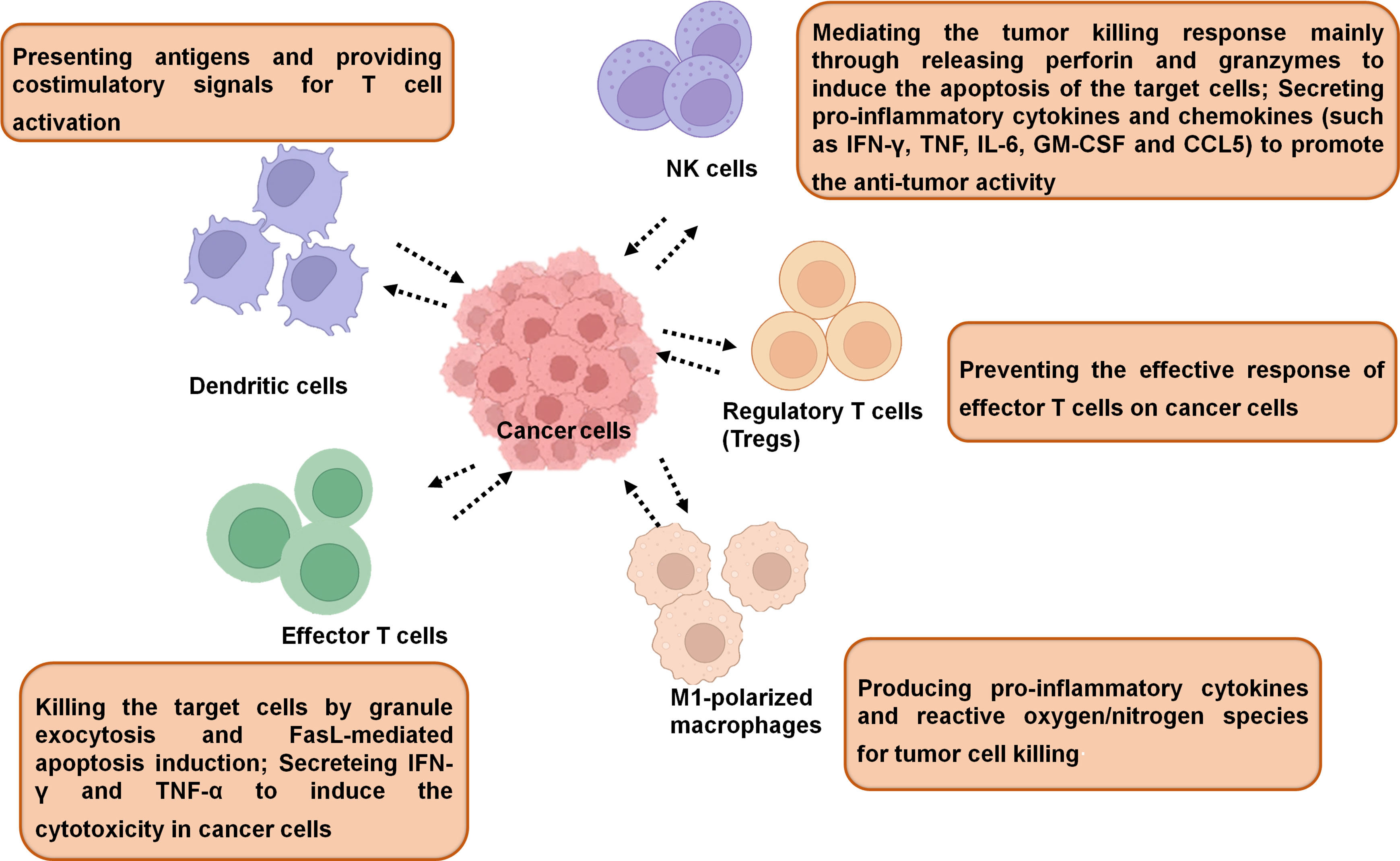
Figure 1 The immune cells in TME. The tumor-associated immune cells can be divided into two types: tumor-antagonizing and tumor-promoting immune cells.
Effects of natural products on monocytes and macrophages
Monocytes/macrophages are derived from bone marrow stem cells, which can enter the bloodstream and be distributed in various organ tissues after developing into monocytes.
In the process of participating in innate immunity, monocytes/macrophages can identify pathogen-associated molecular patterns (PAMP) and play an important role in innate immunity. On the other hand, monocytes/macrophages also can mediate and promote inflammatory responses and implement immunity killing, and antigen presentation (20, 21). Tumor associated macrophages (TAM) are traditionally classified into two kinds, termed as polarization: the immunosuppressive/anabolic M2 phenotype and the classical inflammatory M1 phenotype. M1 macrophages are the main effector cells for host destruction of pathogens and M2 macrophages have anti-inflammatory and angiogenic functions in tumors (22, 23). M1 can produce pro-inflammatory cytokines and reactive oxygen/nitrogen species, which are essential for host defense and tumor cell killing and are therefore considered to be ‘good’ macrophages (24).
Natural products can inhibit the M2-like polarization of TAM and block tumor growth and migration. Emodin attenuated tumor growth by inhibiting IRF4, STAT6, and C/EBPβ signaling and M2-like polarization (25). Astragalus has been demonstrated to have anti-inflammatory and anti-fibrotic properties. Astragalus can inhibit the aggregation and activation of monocytes/macrophages, and reduce the production of TGF-β1 at the peritoneal site (26). Phenylpropanoid is the main active ingredient in ginger, which can directly inhibit cytoplasmic phospholipase 2 (cPLA2) and IL-1β in macrophage expression (27). Inonotus sanghuang, a medicinal plant rich in quercetin, isorhamnetin, quercitrin, rutin and chlorogenic acid, has been demonstrated to decrease inflammation via altering the interaction of macrophages and fat cells. It has been proposed that doing so may improve insulin resistance and the metabolic syndrome (28). Additionally, Garlic water-soluble extract has been shown to elevate intracellular thiol and glutathione concentrations in human primary monocytes. These results suggest that the extract of Garlic can regulate the differentiation of monocytes into macrophages, thereby playing a protective role (29). Macrophages are critical players in the development of nonalcoholic steatohepatitis (NASH) and hepatocarcinoma. Natural products have crucial roles in modulating macrophage activation, recruitment, and polarization, making them promising treatment possibilities for hepatocarcinoma (Figure 3) (30). Angiogenesis in the tumor microenvironment not only nourishes tumor cells and promotes their growth, but is also closely associated with tumor metastasis. Lentinan is a bioactive compound extracted from Lentinus edodes, which promotes the expression of the angiogenesis inhibitory factor IFN-γ, thereby inhibiting tumor angiogenesis (31).
Effects of natural products on dendritic cells
DCs are the most powerful antigen-presenting cells, acting to condition the adaptive immune system to identify foreign antigens and serving as a link between the innate and adaptive immune responses (32). Different subsets of DCs can induce naïve CD4+ T cells to develop into Th1, Th2, Th17 and Treg cells, thereby modulating T cell-mediated immune response types that act as effector cells in the innate immune response (33, 34). DCs can exert their ability to induce, regulate and control T cell responses (35). Infiltration of mature, active DCs into the tumor increases immune activation and recruitment of immune effector cells.
Natural products can enhance anti-tumor immunity by promoting DCs maturation. Ganoderma lucidum polysaccharides, the main biologically active ingredient of Ganoderma lucidum, efficiently stimulate the activation and maturation of human monocyte-derived DCs. Ganoderma lucidum polysaccharides can also increase the expression of CD80, CD86, CD83, CD40, CD54, and human leukocyte antigen DR on the DC surface (36). Lycium barbarum polysaccharide (LBP), the main active ingredient in lycium barbarum, upregulates CD11c expression and induces DCs maturation through the TLR2/TLR4-mediated NF-κB pathway. LBP-treated DCs are more effective in promoting lymphocyte activation and proliferation, enhancing the immune response (37). Recently, there is increasing evidence that components of natural products can modulate the immune system by targeting DCs, including flavonoids, polysaccharides, phenolic compounds, saponins, and so on (38).
Effects of natural products on natural killer cells
NK cells are also produced from bone marrow stem cells, which are mostly found in peripheral circulation and serve as the body’s first line of defense. At the same time, it can also participate in the cellular immune response and play an important role in tumor diseases in vivo (39). NK cells have a powerful cytolytic activity and play an important role in immune control (40). NK cells mediate the tumor killing response mainly by releasing perforin and granzyme to induce apoptosis in target cells (41). In addition, NK cells secrete chemokines and pro-inflammatory cytokines to promote anti-tumor activity (42). Besides, the NK cells may promote the formation and response of tumor-specific CD4+ and CD8+T cells (43).
The production of IL-2 and IFN- by CD4+ T cells can activate NK cells (44). When activated, NK cells release perforin and granzyme B, which cause apoptosis and necrosis in target cells. Numerous studies have revealed that resveratrol can activate and enhance the killing ability of NK cells. The low concentration of resveratrol can increase the expression of NKG2D and IFN-γ in NK cells (45). The resveratrol treatment group can upregulate its anti-tumor and anti-infective abilities by enhancing the activity of NK cells (46). In animal models, green tea catechin metabolitesincrease NK cell cytotoxicity, while quercetin increases NK cell lytic activity (47, 48). Berries high in flavonoids and pro-anthocyanidins not only prevent the progress of cancer but also play a role in the modulation of NK cell (49). After taking garlic extract for 90 days, NKG2D was found to be up-regulated in NK cells. It proves that garlic extract can improve the activity of NK cells and enhance immunity (50). When vitamin A, B, C, D, and E are applied to NK cells, they have stimulatory properties. Although the precise mechanism remains unknown in the majority of cases, components appear to be promising candidates for NK cell-stimulating drugs in tumors (51).
Effects of natural products on regulatory T cells
Regulatory T (Treg) cells are a subtype of T cells with immunomodulatory functions that are closely related to the pathological processes of a variety of human tumors (52). When the suppressive function of Treg cells is compromised, the activity of helper T cells (Th) and killer T cells can induce autoimmune disorders, such as rheumatoid arthritis, multiple sclerosis, and systemic lupus erythematosus (53, 54). On the contrary, the immunosuppressive function of Treg cells is too strong to cause tumor immune escape, such as tumorigenesis (55, 56). Similar to M2 macrophages, Treg cells can inhibit the release of cytokines from Th1 cells and promote angiogenesis in the tumor microenvironment (57).
Kaempferol enhanced Treg cell immunosuppressive activity by inhibiting the activity of proto-oncogene serine/threonine kinase (PIM1) (58). The compound triptolide isolated from Triptolide obviously inhibited the Th2, Th1, and Th17 cell-mediated inflammatory responses and up-regulated the expression of FOXP3 (59). Evidence has demonstrated that lentinan decreased tumor vascular function in a non-T-cell dependent manner by increasing IFNγ production, and showed anti-tumor effect in LAP0297 lung tumor model (31). Lentinan significantly inhibited anti-inflammatory IL-10 and TGF-β 1, and increased the expression of pro-inflammatory chemokines/cytokines (IFN-γ and TNF-α) and IL-12, and decreased immunosuppressive Treg cells. The downregulation of Treg cells is associated with the over-induction of IFN-γ and TNF-α in non-small cell lung cancer (NSCLC), and the inflammatory state of Lentinoglycan treated NSCLC patients can change from Th2 to Th1 (60).
Effects of natural products on effector T cells
Effector T cells include CD8+ cytotoxic T cells (CTLs) and effector CD4+ T cells. In the “activated” state, CTL can induce target cancer cells killing through granule cytokinesis and Fas ligand (FasL)-mediated apoptosis (61). CD4+ T cells are helper T cells that aid in the activation and regulation of immune cells. CD4+ T cells can directly help CD8+ T cell activation and proliferation (62–65). Furthermore, they can also help to shape CD8+ T cells into memory CTLs (66). When the T cell receptor (TCR) is activated, CD4+ T cells differentiate into Th1 or Th2 cells. The balance of Th1 and Th2 plays vital roles in the progress of cancers. With further study of T cells, researchers discovered a new T cell subpopulation called Th17 cells (67, 68). Th17 cells produce IL-17, IL-22, and chemokine ligands 20 (CCL20) (69, 70).
Ginsenosides are the active ingredients of ginseng. Studies have confirmed that ginsenoside Rg1 has a direct effect on the activity of Th and the development of the Th1/Th2 system (71). In addition, ginsenoside Rg1 can selectively enhance the expression of germline transcription products (GLTs), increase the production of IgA antibodies and promote humoral immunity (72). In a mouse model of melanoma, ginsenoside Rh2 induced a large number of CD4+ and CD8a+T lymphocytes to infiltrate into the tumor tissue, indicating an enhanced immune response and enhanced cytotoxicity of lymphocytes to melanoma cells B16-F10 (73). In mice, polyphenols, such as apigenin and chrysin inhibit ovalbumin immunization-induced serum IgE by downregulating Th2 responses (74). Likewise, tea polyphenols, such as EGCG, diminish Th1 differentiation and the numbers of Th17 and Th9 cells (61), while resveratrol reduces Th17 cell counts (75). Resveratrol dramatically reduced the fraction of CD4+CD25+ cells among CD4+ T cells in both in vitro and in vivo tests, demonstrating a dose-dependent mechanism (76).
Natural products effectively expanding indications of various types cancer immunotherapy
Recent studies have shown that natural products can enhance the therapeutic effect of cancer vaccines and immune checkpoints inhibitors. Next, we focus on how natural products can improve both treatments through multi-cellular and multi-pathway modulation.
Effects of natural products on the immune checkpoints inhibitors
Immune checkpoint molecules can regulate the immune state by activating or inhibiting immune signaling pathways. Overexpression of some of these molecules in TME leads to T cell dysfunction and ultimately promotes immune escape and tumor survival. Some immune checkpoint antibodies have been used in clinical anti-tumor therapy (77).
The PD-1/PD-L1 pathway, which promotes T cell functional failure, apoptosis and anergy, has stood out among immunological checkpoints due to the outstanding treatment outcomes in many studies (78). However, the presence of an immunosuppressive microenvironment in tumors limits the use of anti-PD-1/PD-L1 antibodies. Natural products have been reported to be key screening targets for PD-1/PD-L1 small molecule inhibitors and reversal of immunosuppression. Besides the ability to regulate the expression of PD-1 and PD-L1, the combination of natural products with anti-PD-1/PD-L1 antibodies has also shown excellent therapeutic efficacy.
The triterpenoid saponin isolated from Anemone flaccida inhibits the growth of hepatocellular carcinoma cells by downregulating the STAT3 signaling pathway to block the activation of PD-1 and PD-L1 (79). Ganoderma lucidum polysaccharide combined with paclitaxel (PTX) preserves the exhausted state of tumor-infiltrating lymphocytes (TILs) by downregulating PD-1 expression (80). Resveratrol can downregulate PD-L1 expression by activating HDAC3/p300/NF-κB signaling pathway in colorectal and breast cancer cells (81). Liu et al. find that combination of andrographolide isolated from Andrographis paniculata and anti-PD-1 antibody is more effective than monotherapy in the treatment of CT26 colon cancer (82). Diosgenin isolated from Acacia concinna in combination with anti-PD-1 antibody can effectively promote necrosis and apoptosis of melanoma cells. Furthermore, their findings suggest that the mechanism of diosgenin sensitivity to anti-PD-1 antibodies mainly contributes to the regulatory function of gut microbiota (83). Therefore, natural products have their unique advantages in immune checkpoints therapy.
Effects of natural products on the cancer vaccines
Cancer vaccines, including cancer treatment vaccines and cancer prevention vaccines, are one of cancer-specific active immunotherapies. The effectiveness of cancer vaccines depends on the optimal combination of adjuvant, antigen, vaccination route and vector. Natural products can improve the immune-stimulate effect of cancer vaccines as adjuvants. QS-21 isolated from Quillaja Saponaria Molina can promote the antigen presentation process and remodel the immunosuppression by regulating Th1 cytokines. Meanwhile a series of Phase I-III clinical trials (leukemia, carcinoma,prostate, ovary, or lung) have investigated the effect of QS-21 as an immune adjuvant in cancer vaccines designed (84). Curcumin has the potential to improve the therapeutic outcome of Bacillus Calmette-Guerin and significantly enhance the efficacy of TRP2 peptide vaccine against melanoma. In addition, curcumin has been reported to inhibit IDO expression by blocking the JAK-STAT1 signaling pathway and to sensitize melanoma FAPαc vaccine by this mechanism (85).
In addition to the effective activation of CD8+ cytotoxic T lymphocytes, cancer vaccines must also face a challenge: poor immunogenicity. It is reported that natural products can enhance tumor immunogenicity by inducing immunogenic cell death (ICD) effects. The pathway of ICD-induced tumour cell death relies on damage-associated molecular patterns (DAMPs) such as heat shock proteins (HSPs), high mobility group box 1 (HMGB1) and calreticulin (CRT), making tumour cells a “therapeutic vaccine” that can induce anti-tumour immunity (86). Capsaicin has been reported to trigger ICD effects in primary effusion lymphoma (PEL) cells by inducing exposure to DAMPs (87). Ginsenoside Rg3 isolated from ginseng can induce ICD and enhance interferon g (IFN-g) secretion to inhibit tumor growth (88). oreover, shikonin can improve the expressions of MHC II and CD86, and enhance tumor-immunogenicity of tumor vaccines via ICD (89).
Discussion
Given the multi-pharmacological activity and chemical diversity, natural products have been described as a non-substitutable source of clinical therapeutics for human tumors. Natural products can mediate multiple immune responses and reduce the tumor escape. They enhance the interaction of immune cells while decreasing the expression of pro-inflammatory cytokines. Previous studies have shown that natural products can exert anticancer activity through immune regulation in vivo and in vitro. Unlike traditional chemoimmunotherapy in tumor, natural products display many advantages, such as wide sources, less toxic and side effects, as well as diverse immunomodulatory activities, suggesting that natural products have an attractive prospect in the research of novel tumor immunotherapy. The combination of natural products and chemotherapeutic drugs may exert stronger therapeutic effect than chemotherapeutic drugs alone in tumors, which has synergistic and reducing toxic and side effects.
Although natural products have made encouraging appear promising and progress in various studies as modulators of tumor immunotherapy. For natural products to be better used in cancer immunotherapy, a number of issues still need to be addressed. First of all, natural products will face the problem of individual patient differences, TME differences, tumor heterogeneity. Secondly, a deeper and more comprehensive exploration of the signalling pathways of the immune system relevant to tumor immunotherapy is needed to help select more effective natural products. Thirdly, most natural products have a wide range of pharmacological effects, but their targets and molecular mechanisms relevant to tumour immunity have not been fully elucidated.
Above all, the emerging role of natural products in tumor immunotherapy still has greater potential and deserves attention. Future research can screen natural products for targeted antitumor immune drugs using advanced technologies, such as metabolomics, single-cell sequencing, novel drug delivery technologies, and computer-aided design techniques.
Author contributions
X-QJ conceptualized the ideas. X-QJ, NL, W-JF, Z-HL, and J-LH performed the literature search, drafted the original manuscript, and drew the figures. Z-SL revised the manuscript. All the authors approved the final version of the manuscript.
Funding
This work was financially supported by General Program of the National Natural Science Foundation of China Joint project (NO. U21A20348), National Natural Science Foundation of China General Project (No.81970633), Heilongjiang Provincial Higher Education Institutions Basic Research Business Expenses (2021KYYWF0046).
Conflict of interest
The authors declare that the research was conducted in the absence of any commercial or financial relationships that could be construed as a potential conflict of interest.
Publisher’s note
All claims expressed in this article are solely those of the authors and do not necessarily represent those of their affiliated organizations, or those of the publisher, the editors and the reviewers. Any product that may be evaluated in this article, or claim that may be made by its manufacturer, is not guaranteed or endorsed by the publisher.
References
1. Venter C, Eyerich S, Sarin T, Klatt KC. Nutrition and the immune system: A complicated tango. Nutrients (2020) 12(3):818. doi: 10.3390/nu12030818
2. Artis D, Spits H. The biology of innate lymphoid cells. Nature (2015) 517(7534):293–301. doi: 10.1038/nature14189
3. Hanahan D, Weinberg RA. Hallmarks of cancer: the next generation. Cell (2011) 144(5):646–74. doi: 10.1016/j.cell.2011.02.013
4. Yost KE, Satpathy AT, Wells DK, Qi Y, Wang C, Kageyama R, et al. Clonal replacement of tumor-specific T cells following PD-1 blockade. Nat Med (2019) 25(8):1251–9. doi: 10.1038/s41591-019-0522-3
5. Finck A, Gill SI, June CH. Cancer immunotherapy comes of age and looks for maturity. Nat Commun (2020) 11(1):3325. doi: 10.1038/s41467-020-17140-5
6. Hargadon KM, Johnson CE, Williams CJ. Immune checkpoint blockade therapy for cancer: An overview of FDA-approved immune checkpoint inhibitors. Int Immunopharmacol (2018) 62:29–39. doi: 10.1016/j.intimp.2018.06.001
7. O’Leary MC, Lu X, Huang Y, Lin X, Mahmood I, Przepiorka D, et al. FDA Approval summary: Tisagenlecleucel for treatment of patients with relapsed or refractory b-cell precursor acute lymphoblastic leukemia. Clin Cancer Res (2019) 25(4):1142–6. doi: 10.1158/1078-0432.CCR-18-2035
8. Bouchkouj N, Kasamon YL, de Claro RA, George B, Lin X, Lee S, et al. FDA Approval summary: Axicabtagene ciloleucel for relapsed or refractory Large b-cell lymphoma. Clin Cancer Res (2019) 25(6):1702–8. doi: 10.1158/1078-0432.CCR-18-2743
9. Garner H, de Visser KE. Immune crosstalk in cancer progression and metastatic spread: a complex conversation. Nat Rev Immunol (2020) 20(8):483–97. doi: 10.1038/s41577-019-0271-z
10. Farzaei MH, Rahimi R, Abdollahi M. The role of dietary polyphenols in the management of inflammatory bowel disease. Curr Pharm Biotechnol (2015) 16(3):196–210. doi: 10.2174/1389201016666150118131704
11. Andreicut AD, Parvu AE, Mot AC, Parvu M, Fischer FE, Catoi AF, et al. Phytochemical analysis of anti-inflammatory and antioxidant effects of mahonia aquifolium flower and fruit extracts. Oxid Med Cell Longev (2018) 2018:2879793. doi: 10.1155/2018/2879793
12. Liao K, Li J, Wang Z. Dihydroartemisinin inhibits cell proliferation via AKT/GSK3beta/cyclinD1 pathway and induces apoptosis in A549 lung cancer cells. Int J Clin Exp Pathol (2014) 7(12):8684–91.
13. Wang Y, Zhang Q, Chen Y, Liang CL, Liu H, Qiu F, et al. Antitumor effects of immunity-enhancing traditional Chinese medicine. BioMed Pharmacother (2020) 121:109570. doi: 10.1016/j.biopha.2019.109570
14. He J, Yin P, Xu K. Effect and molecular mechanisms of traditional Chinese medicine on tumor targeting tumor-associated macrophages. Drug Des Devel Ther (2020) 14:907–19. doi: 10.2147/DDDT.S223646
15. Sudhakaran M, Sardesai S, Doseff AI. Flavonoids: New frontier for immuno-regulation and breast cancer control. Antioxidants (Basel) (2019) 8(4):103. doi: 10.3390/antiox8040103
16. Ferreira SS, Passos CP, Madureira P, Vilanova M, Coimbra MA. Structure-function relationships of immunostimulatory polysaccharides: A review. Carbohydr Polym (2015) 132:378–96. doi: 10.1016/j.carbpol.2015.05.079
17. Gandhi GR, Neta M, Sathiyabama RG, Quintans J, de Oliveira ESA, Araujo A, et al. Flavonoids as Th1/Th2 cytokines immunomodulators: A systematic review of studies on animal models. Phytomedicine (2018) 44:74–84. doi: 10.1016/j.phymed.2018.03.057
18. Boland JW, Foulds GA, Ahmedzai SH, Pockley AG. A preliminary evaluation of the effects of opioids on innate and adaptive human in vitro immune function. BMJ Support Palliat Care (2014) 4(4):357–67. doi: 10.1136/bmjspcare-2013-000573
19. Lei X, Lei Y, Li JK, Du WX, Li RG, Yang J, et al. Immune cells within the tumor microenvironment: Biological functions and roles in cancer immunotherapy. Cancer Lett (2020) 470:126–33. doi: 10.1016/j.canlet.2019.11.009
20. Wynn TA, Chawla A, Pollard JW. Macrophage biology in development, homeostasis and disease. Nature (2013) 496(7446):445–55. doi: 10.1038/nature12034
21. Nikitina E, Larionova I, Choinzonov E, Kzhyshkowska J. Monocytes and macrophages as viral targets and reservoirs. Int J Mol Sci (2018) 19(9):2821. doi: 10.3390/ijms19092821
22. Sica A, Mantovani A. Macrophage plasticity and polarization: in vivo veritas. J Clin Invest (2012) 122(3):787–95. doi: 10.1172/JCI59643
23. Locati M, Curtale G, Mantovani A. Diversity, mechanisms, and significance of macrophage plasticity. Annu Rev Pathol (2020) 15:123–47. doi: 10.1146/annurev-pathmechdis-012418-012718
24. Aras S, Zaidi MR. TAMeless traitors: macrophages in cancer progression and metastasis. Br J Cancer (2017) 117(11):1583–91. doi: 10.1038/bjc.2017.356
25. Iwanowycz S, Wang J, Hodge J, Wang Y, Yu F, Fan D. Emodin inhibits breast cancer growth by blocking the tumor-promoting feedforward loop between cancer cells and macrophages. Mol Cancer Ther (2016) 15(8):1931–42. doi: 10.1158/1535-7163.MCT-15-0987
26. Li Z, Zhang L, He W, Zhu C, Yang J, Sheng M. Astragalus membranaceus inhibits peritoneal fibrosis via monocyte chemoattractant protein (MCP)-1 and the transforming growth factor-beta1 (TGF-beta1) pathway in rats submitted to peritoneal dialysis. Int J Mol Sci (2014) 15(7):12959–71. doi: 10.3390/ijms150712959
27. Nievergelt A, Marazzi J, Schoop R, Altmann KH, Gertsch J. Ginger phenylpropanoids inhibit IL-1beta and prostanoid secretion and disrupt arachidonate-phospholipid remodeling by targeting phospholipases A2. J Immunol (2011) 187(8):4140–50. doi: 10.4049/jimmunol.1100880
28. Zhang M, Xie Y, Su X, Liu K, Zhang Y, Pang W, et al. Corrigendum: Inonotus sanghuang polyphenols attenuate inflammatory response Via modulating the crosstalk between macrophages and adipocytes. Front Immunol (2021) 12:633354. doi: 10.3389/fimmu.2021.633354
29. Morihara N, Ide N, Weiss N. Aged garlic extract inhibits CD36 expression in human macrophages via modulation of the PPARgamma pathway. Phytother Res (2010) 24(4):602–8. doi: 10.1002/ptr.3008
30. Li CL, Zhou WJ, Ji G, Zhang L. Natural products that target macrophages in treating non-alcoholic steatohepatitis. World J Gastroenterol (2020) 26(18):2155–65. doi: 10.3748/wjg.v26.i18.2155
31. Deng S, Zhang G, Kuai J, Fan P, Wang X, Zhou P, et al. Lentinan inhibits tumor angiogenesis via interferon gamma and in a T cell independent manner. J Exp Clin Cancer Res (2018) 37(1):260. doi: 10.1186/s13046-018-0932-y
32. Buckwalter MR, Albert ML. Orchestration of the immune response by dendritic cells. Curr Biol (2009) 19(9):R355–61. doi: 10.1016/j.cub.2009.03.012
33. Peron G, de Lima TL, Camargo DRL, Thome R, Cardoso VL. Modulation of dendritic cell by pathogen antigens: Where do we stand? Immunol Lett (2018) 196:91–102. doi: 10.1016/j.imlet.2018.02.001
34. Shortman K, Liu YJ. Mouse and human dendritic cell subtypes. Nat Rev Immunol (2002) 2(3):151–61. doi: 10.1038/nri746
35. Schulke S. Induction of interleukin-10 producing dendritic cells as a tool to suppress allergen-specific T helper 2 responses. Front Immunol (2018) 9:455. doi: 10.3389/fimmu.2018.00455
36. Lin YL, Liang YC, Lee SS, Chiang BL. Polysaccharide purified from ganoderma lucidum induced activation and maturation of human monocyte-derived dendritic cells by the NF-kappaB and p38 mitogen-activated protein kinase pathways. J Leukoc Biol (2005) 78(2):533–43. doi: 10.1189/jlb.0804481
37. Zhu J, Zhang Y, Shen Y, Zhou H, Yu X. Lycium barbarum polysaccharides induce toll-like receptor 2- and 4-mediated phenotypic and functional maturation of murine dendritic cells via activation of NF-kappaB. Mol Med Rep (2013) 8(4):1216–20. doi: 10.3892/mmr.2013.1608
38. Li J, Li J, Zhang F. The immunoregulatory effects of Chinese herbal medicine on the maturation and function of dendritic cells. J Ethnopharmacol (2015) 171:184–95. doi: 10.1016/j.jep.2015.05.050
39. Mathew A. Defining the role of NK cells during dengue virus infection. Immunology (2018) 154(4):557–62. doi: 10.1111/imm.12928
40. Ruggeri L, Capanni M, Urbani E, Perruccio K, Shlomchik WD, Tosti A, et al. Effectiveness of donor natural killer cell alloreactivity in mismatched hematopoietic transplants. Science (2002) 295(5562):2097–100. doi: 10.1126/science.1068440
41. Voskoboinik I, Smyth MJ, Trapani JA. Perforin-mediated target-cell death and immune homeostasis. Nat Rev Immunol (2006) 6(12):940–52. doi: 10.1038/nri1983
42. Guillerey C, Huntington ND, Smyth MJ. Targeting natural killer cells in cancer immunotherapy. Nat Immunol (2016) 17(9):1025–36. doi: 10.1038/ni.3518
43. Pahl J, Cerwenka A. Tricking the balance: NK cells in anti-cancer immunity. Immunobiology (2017) 222(1):11–20. doi: 10.1016/j.imbio.2015.07.012
44. Hu JY, Zhang J, Cui JL, Liang XY, Lu R, Du GF, et al. Increasing CCL5/CCR5 on CD4+ T cells in peripheral blood of oral lichen planus. Cytokine (2013) 62(1):141–5. doi: 10.1016/j.cyto.2013.01.020
45. Leischner C, Burkard M, Pfeiffer MM, Lauer UM, Busch C, Venturelli S. Nutritional immunology: function of natural killer cells and their modulation by resveratrol for cancer prevention and treatment. Nutr J (2016) 15(1):47. doi: 10.1186/s12937-016-0167-8
46. Lu CC, Lai HC, Hsieh SC, Chen JK. Resveratrol ameliorates serratia marcescens-induced acute pneumonia in rats. J Leukoc Biol (2008) 83(4):1028–37. doi: 10.1189/jlb.0907647
47. Exon JH, Magnuson BA, South EH, Hendrix K. Dietary quercetin, immune functions and colonic carcinogenesis in rats. Immunopharmacol Immunotoxicol (1998) 20(1):173–90. doi: 10.3109/08923979809034816
48. Kim YH, Won YS, Yang X, Kumazoe M, Yamashita S, Hara A, et al. Green tea catechin metabolites exert immunoregulatory effects on CD4(+) T cell and natural killer cell activities. J Agric Food Chem (2016) 64(18):3591–7. doi: 10.1021/acs.jafc.6b01115
49. McAnulty LS, Nieman DC, Dumke CL, Shooter LA, Henson DA, Utter AC, et al. Effect of blueberry ingestion on natural killer cell counts, oxidative stress, and inflammation prior to and after 2.5 h of running. Appl Physiol Nutr Metab (2011) 36(6):976–84. doi: 10.1139/h11-120
50. Nantz MP, Rowe CA, Muller CE, Creasy RA, Stanilka JM, Percival SS. Supplementation with aged garlic extract improves both NK and gammadelta-T cell function and reduces the severity of cold and flu symptoms: a randomized, double-blind, placebo-controlled nutrition intervention. Clin Nutr (2012) 31(3):337–44. doi: 10.1016/j.clnu.2011.11.019
51. Grudzien M, Rapak A. Effect of natural compounds on NK cell activation. J Immunol Res (2018) 2018:4868417. doi: 10.1155/2018/4868417
52. Guo J, Zhou X. Regulatory T cells turn pathogenic. Cell Mol Immunol (2015) 12(5):525–32. doi: 10.1038/cmi.2015.12
53. Buckner JH. Mechanisms of impaired regulation by CD4(+)CD25(+)FOXP3(+) regulatory T cells in human autoimmune diseases. Nat Rev Immunol (2010) 10(12):849–59. doi: 10.1038/nri2889
54. Miyara M, Ito Y, Sakaguchi S. TREG-cell therapies for autoimmune rheumatic diseases. Nat Rev Rheumatol (2014) 10(9):543–51. doi: 10.1038/nrrheum.2014.105
55. Tanaka A, Sakaguchi S. Regulatory T cells in cancer immunotherapy. Cell Res (2017) 27(1):109–18. doi: 10.1038/cr.2016.151
56. Shuai Z, Leung MW, He X, Zhang W, Yang G, Leung PS, et al. Adaptive immunity in the liver. Cell Mol Immunol (2016) 13(3):354–68. doi: 10.1038/cmi.2016.4
57. Facciabene A, Peng X, Hagemann IS, Balint K, Barchetti A, Wang LP, et al. Tumour hypoxia promotes tolerance and angiogenesis via CCL28 and t(reg) cells. Nature (2011) 475(7355):226–30. doi: 10.1038/nature10169
58. Lin F, Luo X, Tsun A, Li Z, Li D, Li B. Kaempferol enhances the suppressive function of treg cells by inhibiting FOXP3 phosphorylation. Int Immunopharmacol (2015) 28(2):859–65. doi: 10.1016/j.intimp.2015.03.044
59. Li Y, Yu C, Zhu WM, Xie Y, Qi X, Li N, et al. Triptolide ameliorates IL-10-deficient mice colitis by mechanisms involving suppression of IL-6/STAT3 signaling pathway and down-regulation of IL-17. Mol Immunol (2010) 47(15):2467–74. doi: 10.1016/j.molimm.2010.06.007
60. Wang XE, Wang YH, Zhou Q, Peng M, Zhang J, Chen M, et al. Immunomodulatory effect of lentinan on aberrant T subsets and cytokines profile in non-small cell lung cancer patients. Pathol Oncol Res (2020) 26(1):499–505. doi: 10.1007/s12253-018-0545-y
61. Tanaka H, Yoshizawa H, Yamaguchi Y, Ito K, Kagamu H, Suzuki E, et al. Successful adoptive immunotherapy of murine poorly immunogenic tumor with specific effector cells generated from gene-modified tumor-primed lymph node cells. J Immunol (1999) 162(6):3574–82.
62. Bourgeois C, Rocha B, Tanchot C. A role for CD40 expression on CD8+ T cells in the generation of CD8+ T cell memory. Science (2002) 297(5589):2060–3. doi: 10.1126/science.1072615
63. Mackey MF, Barth RJ, Noelle RJ. The role of CD40/CD154 interactions in the priming, differentiation, and effector function of helper and cytotoxic T cells. J Leukoc Biol (1998) 63(4):418–28. doi: 10.1002/jlb.63.4.418
64. Mackey MF, Gunn JR, Ting PP, Kikutani H, Dranoff G, Noelle RJ, et al. Protective immunity induced by tumor vaccines requires interaction between CD40 and its ligand, CD154. Cancer Res (1997) 57(13):2569–74.
65. Cheng LE, Ohlen C, Nelson BH, Greenberg PD. Enhanced signaling through the IL-2 receptor in CD8+ T cells regulated by antigen recognition results in preferential proliferation and expansion of responding CD8+ T cells rather than promotion of cell death. Proc Natl Acad Sci USA (2002) 99(5):3001–6. doi: 10.1073/pnas.052676899
66. Borst J, Ahrends T, Babala N, Melief C, Kastenmuller W. CD4(+) T cell help in cancer immunology and immunotherapy. Nat Rev Immunol (2018) 18(10):635–47. doi: 10.1038/s41577-018-0044-0
67. Park H, Li Z, Yang XO, Chang SH, Nurieva R, Wang YH, et al. A distinct lineage of CD4 T cells regulates tissue inflammation by producing interleukin 17. Nat Immunol (2005) 6(11):1133–41. doi: 10.1038/ni1261
68. Harrington LE, Hatton RD, Mangan PR, Turner H, Murphy TL, Murphy KM, et al. Interleukin 17-producing CD4+ effector T cells develop via a lineage distinct from the T helper type 1 and 2 lineages. Nat Immunol (2005) 6(11):1123–32. doi: 10.1038/ni1254
69. Louten J, Boniface K, de Waal MR. Development and function of TH17 cells in health and disease. J Allergy Clin Immunol (2009) 123(5):1004–11. doi: 10.1016/j.jaci.2009.04.003
70. Zheng Y, Danilenko DM, Valdez P, Kasman I, Eastham-Anderson J, Wu J, et al. Interleukin-22, a T(H)17 cytokine, mediates IL-23-induced dermal inflammation and acanthosis. Nature (2007) 445(7128):648–51. doi: 10.1038/nature05505
71. Lee EJ, Ko E, Lee J, Rho S, Ko S, Shin MK, et al. Ginsenoside Rg1 enhances CD4(+) T-cell activities and modulates Th1/Th2 differentiation. Int Immunopharmacol (2004) 4(2):235–44. doi: 10.1016/j.intimp.2003.12.007
72. Park HY, Lee SH, Lee KS, Yoon HK, Yoo YC, Lee J, et al. Ginsenoside Rg1 and 20(S)-Rg3 induce IgA production by mouse b cells. Immune Netw (2015) 15(6):331–6. doi: 10.4110/in.2015.15.6.331
73. Wang M, Yan SJ, Zhang HT, Li N, Liu T, Zhang YL, et al. Ginsenoside Rh2 enhances the antitumor immunological response of a melanoma mice model. Oncol Lett (2017) 13(2):681–5. doi: 10.3892/ol.2016.5490
74. Yano S, Umeda D, Yamashita T, Ninomiya Y, Sumida M, Fujimura Y, et al. Dietary flavones suppresses IgE and Th2 cytokines in OVA-immunized BALB/c mice. Eur J Nutr (2007) 46(5):257–63. doi: 10.1007/s00394-007-0658-7
75. Xuzhu G, Komai-Koma M, Leung BP, Howe HS, McSharry C, McInnes IB, et al. Resveratrol modulates murine collagen-induced arthritis by inhibiting Th17 and b-cell function. Ann Rheum Dis (2012) 71(1):129–35. doi: 10.1136/ard.2011.149831
76. Bahrami A, Fereidouni M, Pirro M, Bianconi V, Sahebkar A. Modulation of regulatory T cells by natural products in cancer. Cancer Lett (2019) 459:72–85. doi: 10.1016/j.canlet.2019.06.001
77. Bagchi S, Yuan R, Engleman EG. Immune checkpoint inhibitors for the treatment of cancer: Clinical impact and mechanisms of response and resistance. Annu Rev Pathol (2021) 16:223–49. doi: 10.1146/annurev-pathol-042020-042741
78. Ishida Y, Agata Y, Shibahara K, Honjo T. Induced expression of PD-1, a novel member of the immunoglobulin gene superfamily, upon programmed cell death. EMBO J (1992) 11(11):3887–95. doi: 10.1002/j.1460-2075.1992.tb05481.x
79. Han L, Yao S, Cao S, Mo G, Li J, Cao Y, et al. Triterpenoid saponins from anemone flaccida suppress tumor cell proliferation by regulating MAPK, PD1/PDL1, and STAT3 signaling pathways and altering cancer metabolism. Onco Targets Ther (2019) 12:10917–30. doi: 10.2147/OTT.S212666
80. Chen Y, Wang Y, Xu L, Zhu W, Xu C, Xu M, et al. Influence of total glucosides of paeony on PD-1/PD-L1 expression in primary sjogren’s syndrome. Int J Rheum Dis (2019) 22(2):200–6. doi: 10.1111/1756-185X.13391
81. Lucas J, Hsieh TC, Halicka HD, Darzynkiewicz Z, Wu JM. Upregulation of PDL1 expression by resveratrol and piceatannol in breast and colorectal cancer cells occurs via HDAC3/p300mediated NFkappaB signaling. Int J Oncol (2018) 53(4):1469–80. doi: 10.3892/ijo.2018.4512
82. Liu W, Fan T, Li M, Zhang G, Guo W, Yang X, et al. Andrographolide potentiates PD-1 blockade immunotherapy by inhibiting COX2-mediated PGE2 release. Int Immunopharmacol (2020) 81:106206. doi: 10.1016/j.intimp.2020.106206
83. Kukhetpitakwong R, Hahnvajanawong C, Homchampa P, Leelavatcharamas V, Satra J, Khunkitti W. Immunological adjuvant activities of saponin extracts from the pods of acacia concinna. Int Immunopharmacol (2006) 6(11):1729–35. doi: 10.1016/j.intimp.2006.07.008
84. Gilewski T, Adluri S, Ragupathi G, Zhang S, Yao TJ, Panageas K, et al. Vaccination of high-risk breast cancer patients with mucin-1 (MUC1) keyhole limpet hemocyanin conjugate plus QS-21. Clin Cancer Res (2000) 6(5):1693–701.
85. Tharakan ST, Inamoto T, Sung B, Aggarwal BB, Kamat AM. Curcumin potentiates the antitumor effects of gemcitabine in an orthotopic model of human bladder cancer through suppression of proliferative and angiogenic biomarkers. Biochem Pharmacol (2010) 79(2):218–28. doi: 10.1016/j.bcp.2009.08.007
86. Kroemer G, Galluzzi L, Kepp O, Zitvogel L. Immunogenic cell death in cancer therapy. Annu Rev Immunol (2013) 31:51–72. doi: 10.1146/annurev-immunol-032712-100008
87. Granato M, Gilardini MM, Filardi M, Faggioni A, Cirone M. Capsaicin triggers immunogenic PEL cell death, stimulates DCs and reverts PEL-induced immune suppression. Oncotarget (2015) 6(30):29543–54. doi: 10.18632/oncotarget.4911
88. Son KJ, Choi KR, Lee SJ, Lee H. Immunogenic cell death induced by ginsenoside Rg3: Significance in dendritic cell-based anti-tumor immunotherapy. Immune Netw (2016) 16(1):75–84. doi: 10.4110/in.2016.16.1.75
Keywords: tumor immunotherapy, immune cells, natural products, active chemicals, tumor microenvironment
Citation: Huo J-L, Fu W-J, Liu Z-H, Lu N, Jia X-Q and Liu Z-S (2022) Research advance of natural products in tumor immunotherapy. Front. Immunol. 13:972345. doi: 10.3389/fimmu.2022.972345
Received: 18 June 2022; Accepted: 18 August 2022;
Published: 02 September 2022.
Edited by:
Xuyao Zhang, Fudan University, ChinaCopyright © 2022 Huo, Fu, Liu, Lu, Jia and Liu. This is an open-access article distributed under the terms of the Creative Commons Attribution License (CC BY). The use, distribution or reproduction in other forums is permitted, provided the original author(s) and the copyright owner(s) are credited and that the original publication in this journal is cited, in accordance with accepted academic practice. No use, distribution or reproduction is permitted which does not comply with these terms.
*Correspondence: Nan Lu, bmFuLmx1QG50dS5lZHUuc2c=; Xiang-Qian Jia, MjAyMTEwOEBobGp1LmVkdS5jbg==; Zhang-Suo Liu, emhhbmdzdW9saXVAenp1LmVkdS5jbg==