- 1Department of Rehabilitation Medicine, Guangzhou Panyu Central Hospital, Guangzhou, China
- 2The First People's Hospital of Changde City, Changde, China
- 3People's Hospital of Ningxiang City, Ningxiang, China
- 4Dental Materials Science, Applied Oral Sciences and Community Dental Care, Faculty of Dentistry, The University of Hong Kong, Hong Kong, Hong Kong SAR, China
- 5Guangzhou University of Chinese Medicine, Guangzhou, China
- 6Joint Shantou International Eye Center of Shantou University and The Chinese University of Hong Kong, Shantou University Medical College, Shantou, China
- 7Hunan University of Chinese Medicine, Changsha, China
- 8Department of Rheumatology and Clinical Immunology, Peking Union Medical College Hospital, Chinese Academy of Medical Sciences & Peking Union Medical College, Beijing, China
- 9Qiqihar Medical University, Qiqihar, China
Objective: To evaluate safety and efficacy of dietary polyphenols in the treatment of rheumatoid arthritis (RA).
Methods: CNKI, Pubmed, Cochrane library, Embase were searched to collect randomized controlled trials (RCTs) of dietary polyphenols in the treatment of RA. The databases were searched from the time of their establishment to November 8nd, 2022. After 2 reviewers independently screened the literature, extracted data, and assessed the risk of bias of the included studies, Meta-analysis was performed using RevMan5.4 software.
Results: A total of 49 records (47 RCTs) were finally included, involving 3852 participants and 15 types of dietary polyphenols (Cinnamon extract, Cranberry extract, Crocus sativus L. extract, Curcumin, Garlic extract, Ginger extract, Hesperidin, Olive oil, Pomegranate extract, Puerarin, Quercetin, Resveratrol, Sesamin, Tea polyphenols, Total glucosides of paeony). Pomegranate extract, Resveratrol, Garlic extract, Puerarin, Hesperidin, Ginger extract, Cinnamon extract, Sesamin only involve in 1 RCT. Cranberry extract, Crocus sativus L. extract, Olive oil, Quercetin, Tea polyphenols involve in 2 RCTs. Total glucosides of paeony and Curcumin involve in more than 3 RCTs. These RCTs showed that these dietary polyphenols could improve disease activity score for 28 joints (DAS28), inflammation levels or oxidative stress levels in RA. The addition of dietary polyphenols did not increase adverse events.
Conclusion: Dietary polyphenols may improve DAS28, reduce C-reactive protein (CRP) and erythrocyte sedimentation rate (ESR), and improve oxidative stress, etc. However, more RCTs are needed to verify or modify the efficacy and safety of dietary polyphenols.
Systematic review registration: https://www.crd.york.ac.uk/prospero/, identifier CRD42022315645.
1 Introduction
Rheumatoid arthritis (RA) is a chronic, systemic, highly disabling autoimmune disease with non-infectious, symmetrical, progressive polyarthritis as the main clinical manifestation. It primarily affects the joints, but should be considered as a syndrome that includes extra-articular manifestations, such as rheumatoid nodules, pulmonary involvement, or vasculitis, as well as systemic comorbidities (1, 2). It is the most common systemic irritant arthritis and is one of the world’s major public health challenges, affecting approximately 1% of the world’s population (3). RA can occur at any age, and its incidence begins to increase significantly at age 25; at age 55, the incidence of rheumatoid arthritis peaks (4, 5). The prevalence of RA varies among different ethnic groups. The incidence of RA is 7 percent among Native Americans and 0.2 to 0.4 percent in some other countries (6). For example, the prevalence of RA in China is 0.2% to 4% (7), the number of patients is as high as 4 million, and the remission rate is roughly only 8.6% (8). Like most other autoimmune diseases, RA is more common in women than in men in a ratio of 2-3:1 (9).
Although RA has a high disability rate and high prevalence, its pathogenesis is not fully understood. At present, the treatment of RA has entered into a comprehensive management strategy, which aims to slow down the progression of the disease, reduce the occurrence of pain and bone destruction, preserve the joint mobility of patients as much as possible, and avoid disability (10, 11). The main treatments for RA include conventional synthetic antirheumatic drugs (DMARDs), glucocorticoids, non-steroidal anti-inflammatory drugs (NSAIDs), targeted synthetic DMARDs, bio-original DMARDs and biosimilar DMARDs (12, 13). Although the above drugs can significantly relieve clinical symptoms, they have a single target, many adverse reactions (such as allergic reactions and blood diseases), poor long-term efficacy, and high cost (6). Meanwhile, in the vast developing countries, due to the huge economic burden, it is currently difficult for most RA patients to receive standardized and effective treatment, which is accompanied by serious physical and mental injuries (11). As an important complementary alternative therapy, dietary supplements are now also an important adjunct option for RA patients. For example, dietary polyphenols have the characteristics of multi-target, multi-component, and multi-mechanism in pharmacology, and have definite clinical efficacy, as well as the advantages of less toxic side effects and no drug resistance (14).
Polyphenols are a diverse class of plant-derived compounds with water-soluble chemical properties (15). They are widely found in herbs, fruits, teas, red berries, coffee, red wine, and dark chocolate worldwide, and are well-known antioxidants and have been proposed as treatments for several inflammatory and metabolic disorders (16–19). Studies have shown that polyphenols can prevent oxidative stress, inhibit inflammation and modulate immunity and other pharmacological effects (20), especially inhibiting inflammation and immunity. These compounds may improve fibroblast-like synoviocytes (FLS) in rats with adjuvant arthritis (AIA) by inducing inhibition of several pro-inflammatory immunochemokines and promoting apoptosis through mitochondrial signaling pathways and endoplasmic reticulum stress (21). Currently, many randomized controlled trials (RCTs) of dietary polyphenols in the treatment of RA have been published. However, the results and treatment measures of these RCTs are different, which cannot provide a basis for clinicians to formulate treatment plans for RA. Therefore, a comprehensive and in-depth summary of these RCTs is urgently needed to complement the therapeutic modalities for the treatment of RA. Hence, this study was the first comprehensive systematic review and meta-analysis of RCTs on the treatment of RA with dietary polyphenols in order to provide high-quality evidence for clinicians.
2 Materials and methods
2.1 Protocol
This systematic review and meta-analysis were conducted strictly in accordance with the protocol registered in PROSPERO (CRD42022315645) and PRISMA-guidelines (see Supplementary Materials) (22).
2.2 Literature search strategy
The databases were searched from the time of their establishment to November 8nd, 2022. The databases include China National Knowledge Infrastructure (CNKI), Web of Science, Sinomed, VIP Database for Chinese Technical Periodicals, Medline Complete, ArXiv, Pubmed, Embase, Wanfang Database on Academic Institutions in China, ClinicalTrials.gov and Cochrane Library were searched from. The search strategy was shown in Table S1.
2.3 Inclusion and exclusion criteria
2.3.1 Participants
Participants were RA patients. The diagnosis of RA conformed to the RA diagnostic criteria in the 2010 Rheumatoid Arthritis Diagnosis and Treatment Guidelines of the Rheumatology Branch of the Chinese Medical Association or the RA diagnostic criteria proposed by the American College of Rheumatology/2017 European Federation of Rheumatology in 1987 or other recognized criteria.
2.3.2 Intervention
The experimental group was treated with dietary polyphenols, and the route of administration, preparation type, etc. were not limited; the treatment could be combined with conventional treatment or control group treatment. Treatment in the control group was conventional therapy or a placebo or other non-dietary polyphenol therapy (RCT researchers claimed to limit the consumption of polyphenol-rich diets in the control group.).
2.3.3 Outcomes
(1) Efficacy indicators: Disease activity score for 28 joints (DAS28), American College of Rheumatology 20% (ACR20), American College of Rheumatology 50% (ACR50), American College of Rheumatology 70% (ACR70). (2) Inflammatory parameters in serum: C-reactive protein (CRP), erythrocyte sedimentation rate (ESR), tumor necrosis factor (TNF-α), interleukin (IL-6), rheumatoid factor (RF). (3) Oxidative stress markers: malondialdehyde (MDA) and total antioxidant capacity (TAC). (4) Adverse events.
2.3.4 Study design
The study design included RCTs with no restrictions on publication time, language, quality and publication status.
2.3.5 Exclusion criteria
(1) non-RCT; (2) review; (3) cohort study; (4) control group also used dietary polyphenol-enriched therapy
2.4 Literature quality evaluation and data extraction
Two reviewers independently searched the database according to the search strategy, and initially excluded studies that did not belong to the treatment of RA with polyphenols after reading the titles and abstracts. They then independently screened literature, assessed quality, and extracted data according to inclusion and exclusion criteria, and any disagreements were resolved by brainstorming with other reviewers. The data in the literature were registered and managed in Excel form, and the extracted data included the name of the investigator, the year of publication of the literature, the general characteristics of the included patients and the number of cases, intervention measures, outcomes, etc (23). The methodological quality of the included studies was assessed according to the Cochrane Collaboration’s Risk of Bias Assessment Tool (24). The main contents of the evaluation are: (1) the application of random allocation method; (2) the implementation of random concealment; (3) the implementation of blinding of research subjects and treatment plans; (4) the implementation of blinding of outcome measurers; (5) Integrity of Outcomes; (6) Selective reporting; (7) Other bias.
2.5 Statistical analysis
Meta-analysis was performed using Review Manager 5.4 provided by the Cochrane Collaboration. Enumeration data were expressed as risk ratio (RR), measurement data were expressed as weighted mean difference (WMD) or standard mean difference (SMD), and each effect size was expressed as 95% confidence interval (CI). The chi-square test was used to analyze the heterogeneity between the results. P>0.1 and I²≤50% was considered low heterogeneity; P<0.1 and I²>50% was considered high heterogeneity (25). Regardless of heterogeneity, due to the different sources of polyphenols, we use the random effects model for all outcome. Outcomes with included RCTs ≥ 4 were selected for subgroup analysis, sensitivity analysis, publication bias assessment, meta-regression analysis and GRADE score. Sensitivity analysis, publication bias assessment, meta-regression were analyzed by STATA 15.0; GRADE score was assessed by the GRADEprofiler software (26).
3 Results
3.1 Literature search results
A total of 53 relevant studies were obtained in the initial examination, and after screening, 49 records were finally included (27–76), and 2 records were excluded for they are not RCTs (77, 78). The literature screening process and results are shown in (Figure 1).
3.2 Description of included trials
Two records (28, 29) came from the same RCT and were therefore recorded as Javadi et al., 2017 (28, 29). Two records (32, 33) came from the same RCT and were therefore recorded as Moosavian et al., 2020 (32, 33). Some RCTs contain multiple groups and are therefore split into groups a and b. The included RCTs involved 15 dietary polyphenols (Cinnamon extract, Cranberry extract, Crocus sativus L. extract, Curcumin, Garlic extract, Ginger extract, Hesperidin, Olive oil, Pomegranate extract, Puerarin, Quercetin, Resveratrol, Sesamin, Tea polyphenols, Total glucosides of paeony) and were from 7 different countries (Iran, Korea, Egypt, China, Japan, Brazil, India). The details of study characteristics are presented in Table 1.
3.3 Risk of bias assessments
The summary and graph of risk of bias ware shown in Figure 2.
3.4 Pomegranate extract for RA
Only Ghavipour et al., 2016 from Iran reported the results of Pomegranate extract in the treatment of RA involving 30 participants in Pomegranate extract group and 25 in control group (27). The control group received cellulose capsules as a placebo. They found that compared with placebo, pomegranate extract reduced DAS28 scores, improved joint swelling and tenderness, decreased ESR levels, and increased gluthation peroxidase (GPx) concentrations (27). This suggests that Pomegranate extract may play a role in the treatment of RA through anti-inflammatory and anti-oxidation. However, its lowering effect on MMP3, CRP and MDA has not yet been found.
3.5 Quercetin for RA
Javadi et al., 2017 (28, 29) from Iran and Bae et al., 2009 (29) from Korea reported the results of quercetin in the treatment of RA involving 120 participants. Because the results of the 2 RCTs could not be combined, only a systematic review was performed. Javadi et al., 2017 found that DAS-28 decreased and serum TNF-α levels were significantly reduced after quercetin intervention compared to placebo (28, 29). However, Bae et al., 2009 showed no significant differences in serum proinflammatory cytokines (TNF-α and IL-1β) and CRP levels after quercetin treatment compared with placebo (30). This suggests that the effect of quercetin in the treatment of RA needs more research.
3.6 Resveratrol for RA
Khojah et al., 2018 from Egypt reported the results of resveratrol in the treatment of RA involving 100 participants (50 in each group) (31). They found that resveratrol treatment reduced swollen and tender joint counts, decreased DAS28, and decreased serum CRP, ESR, hypocarboxylated osteocalcin, matrix metalloproteinase (MMP)3, TNF-α, and IL-6.
3.7 Garlic extract for RA
Moosavian et al., 2020 from Iran reported the results of garlic extract in the treatment of RA involving 62 participants (31 in each group) (32). They found that the Garlic extract intervention improved antioxidant levels (increased TAC, decreased MDA), improved quality of life, and improved inflammation (decreased CRP and TNF-α), decreased joint pain and tenderness, and decreased DAS28 compared with the placebo group.
3.8 Total glucosides of paeony for RA
A total of 23 RCTs reported total glucosides of paeony in the treatment of RA, efficacy indicators, inflammatory parameters and adverse event outcomes were reported, so a meta-analysis was performed.
3.8.1 Efficacy indicators
Efficacy indicators include DAS28, ACR20, ACR50 and ACR70.
1. A total of 9 RCTs reported DAS28. Heterogeneity analysis suggested high heterogeneity among RCTs (I2 = 95%, P<0.00001), and a random-effects model was used. The results showed that compared with the control group, the DAS28 of the total glucosides of paeony was lower [WMD=-0.92 95%CI (-1.52, -0.31), P=0.003] (Figure 3A).
2. Only 2 RCTs reported ACR20. Heterogeneity analysis suggested low heterogeneity among RCTs (I2 = 0%, P=0.53), and a random-effects model was used. The results showed that compared with the control group, the ACR20 of the total glucosides of paeony was lower [RR=1.24 95%CI(1.04, 1.48), P=0.02] (Figure 3B).
3. Only 2 RCTs reported ACR50. Heterogeneity analysis suggested high heterogeneity among RCTs (I2 = 69%, P=0.07), and a random-effects model was used. The results showed that the ACR50 between two groups was of no statistical significance [RR=1.15 95%CI(0.58, 2.27), P=0.69] (Figure 3C).
4. Only 2 RCTs reported ACR70. Heterogeneity analysis suggested low heterogeneity among RCTs (I2 = 0%, P=0.53), and a random-effects model was used. The results showed that compared with the control group, the ACR70 of the total glucosides of paeony was lower [RR=1.61 95%CI(1.09, 2.37), P=0.02] (Figure 3D).
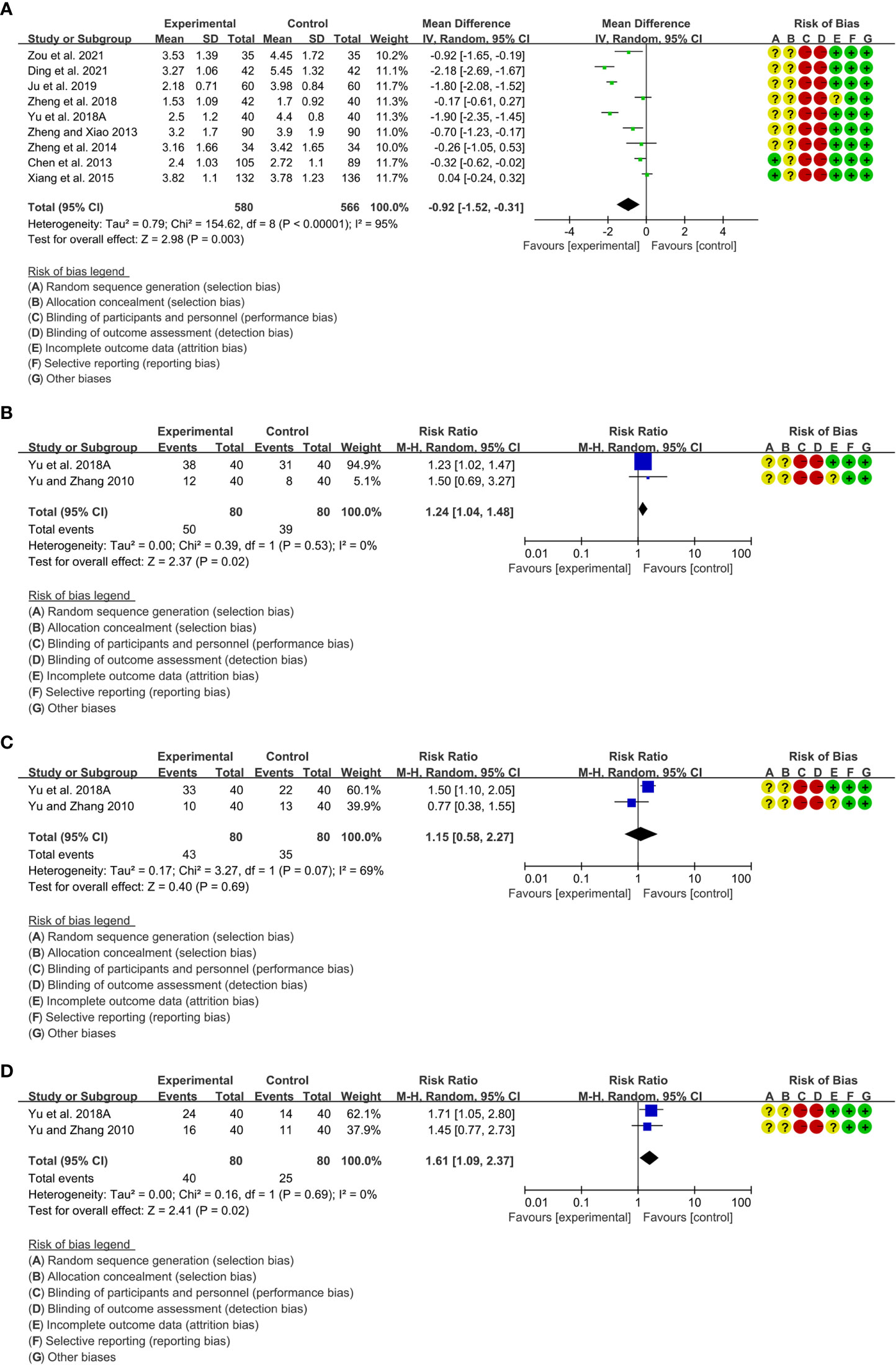
Figure 3 Efficacy indicators of Total glucosides of paeony (A) DAS28; (B) ACR20; (C) ACR50; (D) ACR70.
3.8.2 Inflammatory parameters
Inflammatory parameters include CRP, ESR, RF, IL-6 and TNF-α.
1. A total of 17 RCTs reported CRP. Heterogeneity analysis suggested high heterogeneity among RCTs (I2 = 94%, P<0.00001), and a random-effects model was used. The results showed that compared with the control group, the CRP of the total glucosides of paeony was lower [SMD=-1.32 95%CI (-1.81, -0.83), P<0.00001] (Figure 4A).
2. A total of 16 RCTs reported ESR. Heterogeneity analysis suggested high heterogeneity among RCTs (I2 = 84%, P<0.00001), and a random-effects model was used. The results showed that compared with the control group, the ESR of the total glucosides of paeony was lower [WMD=-6.44 95%CI (-9.24, -3.63), P<0.00001] (Figure 4B).
3. A total of 10 RCTs reported RF. Heterogeneity analysis suggested high heterogeneity among RCTs (I2 = 98%, P<0.00001), and a random-effects model was used. The results showed that compared with the control group, the RF of the total glucosides of paeony was lower [SMD=-2.01 95%CI (-3.01, -1.01), P<0.0001] (Figure 4C).
4. Only 3 RCTs reported IL-6. Heterogeneity analysis suggested low heterogeneity among RCTs (I2 = 99%, P<0.00001), and a random-effects model was used. The results showed that compared with the control group, the IL-6 of the total glucosides of paeony was lower [WMD=-10.64 95%CI(1.08, 2.36), P=0.02] (Figure 4D).
5. Only Yu et al., 2018B reported TNF-α. They found that the TNF-α in total glucosides of paeony group was lower than that in control group (P<0.05).
3.8.3 Adverse events
A total of 15 RCTs reported adverse events. Heterogeneity analysis suggested low heterogeneity among RCTs (I2 = 0%, P=0.98), and a random-effects model was used. The results showed that compared with the control group, the adverse events of the total glucosides of paeony were lower [SMD=0.55 95%CI (0.44, 0.69), P<0.00001] (Figure 5).
3.9 Tea polyphenols for RA
Only 2 RCTs reported tea polyphenols in the treatment of RA, efficacy indicators, inflammatory parameters and adverse event outcomes were reported, so a meta-analysis was performed.
3.9.1 Efficacy indicators
Efficacy indicators include DAS28, ACR20, ACR50 and ACR70.
1. Both 2 RCTs reported DAS28. Heterogeneity analysis suggested low heterogeneity among RCTs (I2 = 98%, P<0.00001), and a random-effects model was used. The results showed that compared with the control group, the DAS28 of the tea polyphenols were lower [WMD=-1.76 95%CI (-2.71, -0.81), P=0.0003] (Figure 6).
2. Only Alghadir et al., 2016 reported ACR20, ACR50 and ACR70 and they found that after the addition of tea polyphenols, the ACR20, ACR50 and ACR70 was higher than control group (58).
3.9.2 Inflammatory parameters
Inflammatory parameters include CRP and ESR.
1. Both 2 RCTs reported CRP. Heterogeneity analysis suggested high heterogeneity among RCTs (I2 = 96%, P<0.00001), and a random-effects model was used. The results showed that compared with the control group, the CRP of the tea polyphenols was lower [WMD=-1.83 95%CI (-3.08, -0.59), P=0.004] (Figure 7).
2. Only Alghadir et al., 2016 reported ESR and they found that after the addition of tea polyphenols, the ESR was higher than control group.
3.9.3 Adverse events
Mirtaheri et al., 2021 showed that no adverse effects of treatment were recorded in the tea polyphenol group and the control group (57). Alghadir et al., 2016 did not report any adverse reactions, possibly because they were not monitored (58).
3.10 Puerarin for RA
Yang et al., 2018 from China reported Puerarin in the treatment of RA, involving 60 participants in experiments group and 59 participants in control group (59). They found that DAS28, ESR, CRP, IL-6 was lower after Puerarin intervention. They also found no significant difference in adverse event rates between the two groups.
3.11 Hesperidin for RA
Kometani et al., 2008 from Japan reported Hesperidin in the treatment of RA, involving 9 participants in experiments group and 10 participants in control group (60). They found that RA symptoms improved (3 out of 9 patients) after the patients drank the Hesperidin-containing beverage, compared with only 1 improvement in 10 patients in the control group.
3.12 Crocus sativus L. extract for RA
Only 2 RCTs reported Crocus sativus L. extract in the treatment of RA, efficacy indicators, inflammatory parameters, oxidative stress markers and adverse event outcomes were reported, so a meta-analysis was performed.
3.12.1 Efficacy indicators
Both 2 RCTs reported DAS28. Heterogeneity analysis suggested high heterogeneity among RCTs (I2 = 78%, P=0.03), and a random-effects model was used. The results showed that the difference of DAS29 between two groups was of no statistical significance [WMD=–0.48 95%CI (-1.31, 0.35), P=0.26] (Figure 8).
3.12.2 Inflammatory parameters
Inflammatory parameters include CRP and ESR.
1. Only Hamidi et al., 2020 reported CRP. They found that the CRP decreased after Crocus sativus L. extract intervention.
2. Both 2 RCTs reported ESR. Heterogeneity analysis suggested high heterogeneity among RCTs (I2 = 62%, P=0.10), and a random-effects model was used. The results showed that the difference of ESR between two groups was of no statistical significance [WMD=-4.01 95%CI (-11. 80, 3.78), P=0.31] (Figure 9).
3.12.3 Oxidative stress markers
Only Hamidi et al., 2020 reported MDA and TAC. They found that both MDA and TAC increased after the intervention, but there was no significant difference compared with the control group (P>0.05) (62).
3.12.4 Adverse events
Adverse events were not reported in either RCTs, probably because they did not monitor for adverse events.
3.13 Ginger extract for RA
Only Aryaeian et al., 2019 reported Ginger extract in the treatment of RA. They found that DAS29 was reduced after the ginger intervention compared to the control group. Regarding inflammatory parameters, they found that peroxidase-activated proliferative receptor (PPAR)-γ gene expression was significantly increased in the ginger group and the control group, but there was no significant difference between the two groups (63).
3.14 Cinnamon extract for RA
Only Shishehbor et al., 2018 reported Cinnamon extract in the treatment of RA. They found that DAS28 decreased, the number of tender and swollen joints decreased, and serum CRP and TNF-α levels were significantly reduced after the cinnamon extract intervention (64). However, no difference in ESR between the two groups was observed.
3.15 Sesamin for RA
Only Helli et al., 2019 reported Sesamin in the treatment of RA. They found that compared with the placebo group, after sesamin intervention, the joint pain was relieved, the number of tender joints was reduced, and the serum hs-CRP, TNF-α and cyclooxysynthase (COX)-2 levels were significantly reduced (65, 66).
3.16 Cranberry extract for RA
Only 2 RCTs reported Cranberry extract in the treatment of RA. Thimóteo et al., 2019 found that after 12 weeks of cranberry extract treatment, DAS28 was decreased, but inflammatory parameters (such as CRP, ESR, RF) were not significantly different compared with the control group (67). Fatel et al., 2021 found that ESR, CRP and DAS28 were both decreased after fish oil + cranberry extract treatment compared with the control group and the treatment group with fish oil alone (68).
3.17 Olive extracts and oil for RA
Only 2 RCTs reported olive oil and 1 RCTs reported olive extracts in the treatment of RA. Hekmatpou et al., 2020 found that after adding olive oil massage, DAS28 decreased, joint pain was relieved, and the number of painful joints and the number of swollen joints decreased (69). Berbert et al., 2005 also found relief of joint pain and decreased duration of morning stiffness, episodes of fatigue, and Ritchie joint index in patients treated with olive oil (70).
Bitler et al., 2007 showed that after treatment with frozen-dried olive vegetation water, RA patients had reduced pain and improved activities of daily living, significantly decreased homocysteine, and was well tolerated by frozen-dried olive vegetation water (71).
3.18 Curcumin for RA
A total of 5 RCTs reported curcumin in the treatment of RA, efficacy indicators, inflammatory parameters and adverse event outcomes were reported, so a meta-analysis was performed.
3.18.1 Efficacy indicators
Four (4) RCTs reported DAS28. Heterogeneity analysis suggested high heterogeneity among RCTs (I2 = 85%, P<0.00001), and a random-effects model was used. The results showed that compared with the control group, the DAS29 in curcumin was lower [WMD=-1.10 95%CI (-1.67, -0.53), P=0.0002] (Figure 10).
3.18.2 Inflammatory parameters
Inflammatory parameters include CRP, ESR and RF.
1. Three (3) RCTs reported CRP. Heterogeneity analysis suggested high heterogeneity among RCTs (I2 = 93%, P<0.00001), and a random-effects model was used. The results showed that compared with the control group, the CRP in curcumin was lower [WMD=-0.35 95%CI (-0.55, -0.15), P=0.0005] (Figure 11A).
2. Five (5) RCTs reported ESR. Heterogeneity analysis suggested high heterogeneity among RCTs (I2 = 99%, P<0.00001), and a random-effects model was used. The results showed that compared with the control group, the ESR in curcumin was lower [WMD=-54.67 95%CI (-88.32, -21.02), P=0.001] (Figure 11B).
3. Two (2) RCTs reported RF. Heterogeneity analysis suggested high heterogeneity among RCTs (I2 = 0%, P=0.97), and a random-effects model was used. The results showed that compared with the control group, the RF in curcumin was lower [WMD=-51.30 95%CI (-60.59, -42.01), P<0.00001] (Figure 11C).
3.18.3 Adverse events
Three (3) RCTs reported adverse events. Heterogeneity analysis suggested high heterogeneity among RCTs (I2 = 0%, P=0.76), and a random-effects model was used. The results showed that the addition of curcumin may not increase the incidence of adverse events [RR=0.31 95%CI (0.06, 1.67), P=0.17] (Figure 12).
3.19 Further analysis for outcomes
3.19.1 Subgroup analysis
The number of RCTs for total glucosides of paeony and curcumin exceeded 4, so a subgroup analysis was performed according to the duration and dosage (Tables 2, 3).
1. Total glucosides of paeony: The difference from the results above is that although the summary results of DAS28 were statistically significant, subgroup analysis showed no statistical difference in DAS28 at each time point. The ESR results also showed lower ESR when receiving ≤12 weeks of treatment, while the effect of receiving 24 weeks of treatment was similar to that of the control group (Because there is only one RCT result at 36 weeks, it is not clear that the treatment effect of 36 weeks is better than that of the control group). In addition, for RF, patients receiving total glucosides of paeony twice a day had lower RF than controls, while patients receiving total glucosides of paeony three times a day had similar RF to controls.
2. Curcumin: The difference from the results above is that after subgroup analysis of doses, there was no statistically significant difference in ESR between the experimental and control groups regardless of doses ≤ 250 mg or 500 mg. And after subgroup analysis of time. At 8 weeks of curcumin treatment, the ESR and CRP of the experimental group were not statistically different from those of the control group, and the statistical difference only appeared at 12 weeks.
3.19.2 Sensitivity analysis
The sensitivity analysis was performed for total glucosides of paeony and curcumin (Figure 13). The sensitivity analysis showed that no matter which RCTs were removed, it had little effect on the overall results, suggesting that the results were stable.
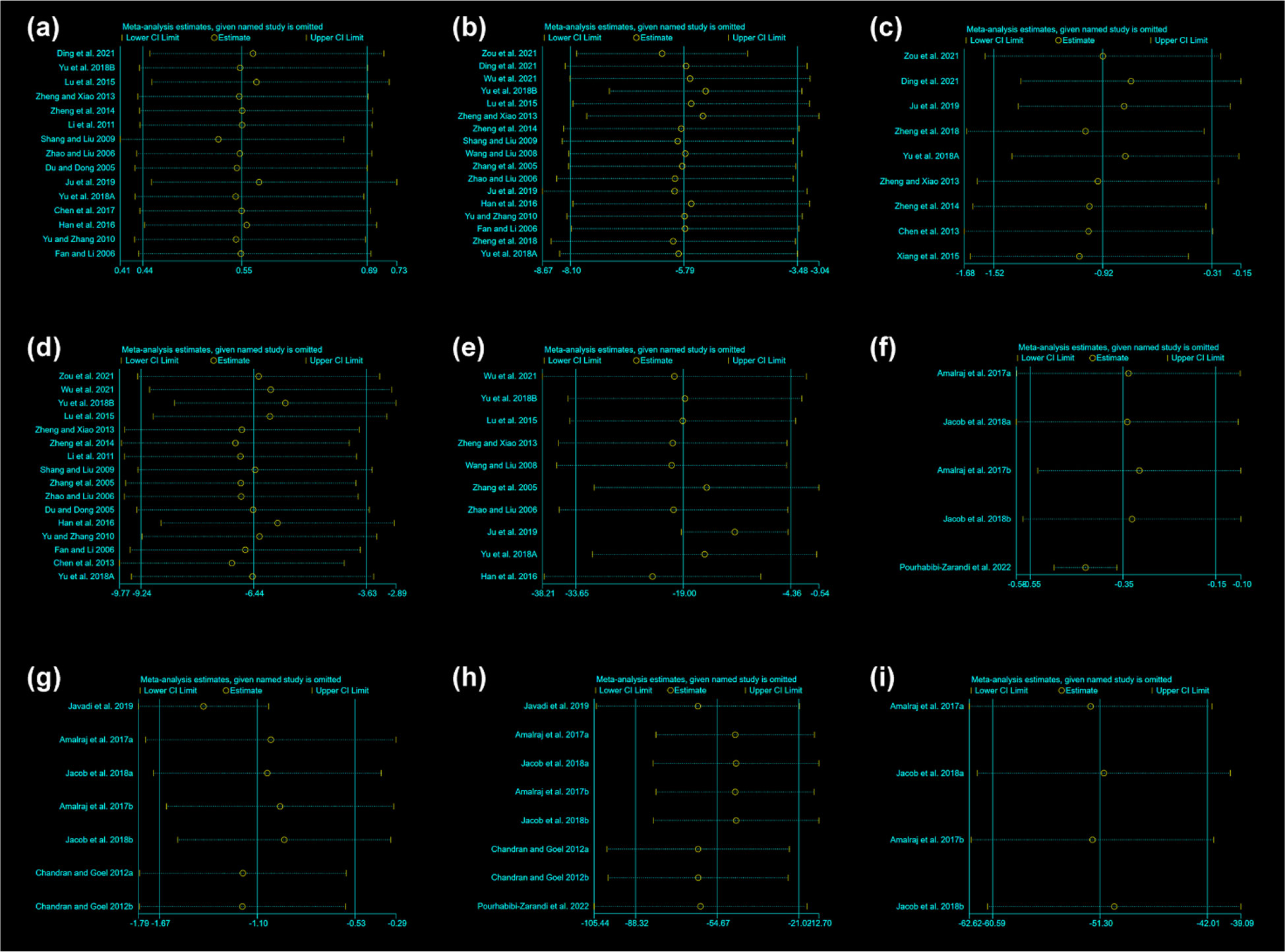
Figure 13 Sensitivity Analysis results (A) adverse events of total glucosides of paeony; (B) CRP of total glucosides of paeony; (C) DAS28 of total glucosides of paeony; (D) ESR of total glucosides of paeony; (E) RF of total glucosides of paeony; (F) CRP of curcumin; (G) DAS28 of curcumin; (H) ESR of curcumin; (I) RF of curcumin.
3.19.3 Publication bias analysis
The publication bias was performed for total glucosides of paeony and curcumin (Figure 14). (1) adverse events of total glucosides of paeony: it may not have publication bias (P=0.802); (2) CRP of total glucosides of paeony: it may have publication bias (P=0.091); (3) DAS28 of total glucosides of paeony: it may not have publication bias (P=0.759); (4) ESR of total glucosides of paeony: it may not have publication bias (P=0.130); (5) RF of total glucosides of paeony: it may have publication bias (P=0.012); (6) CRP of curcumin: it may have publication bias (P=0.007); (7) DAS28 of curcumin: it may not have publication bias (P=0.441); (8) ESR of curcumin: it may have publication bias (P=0.059); (9)RF of curcumin: it may have publication bias (P=0.060) (Figure 14).
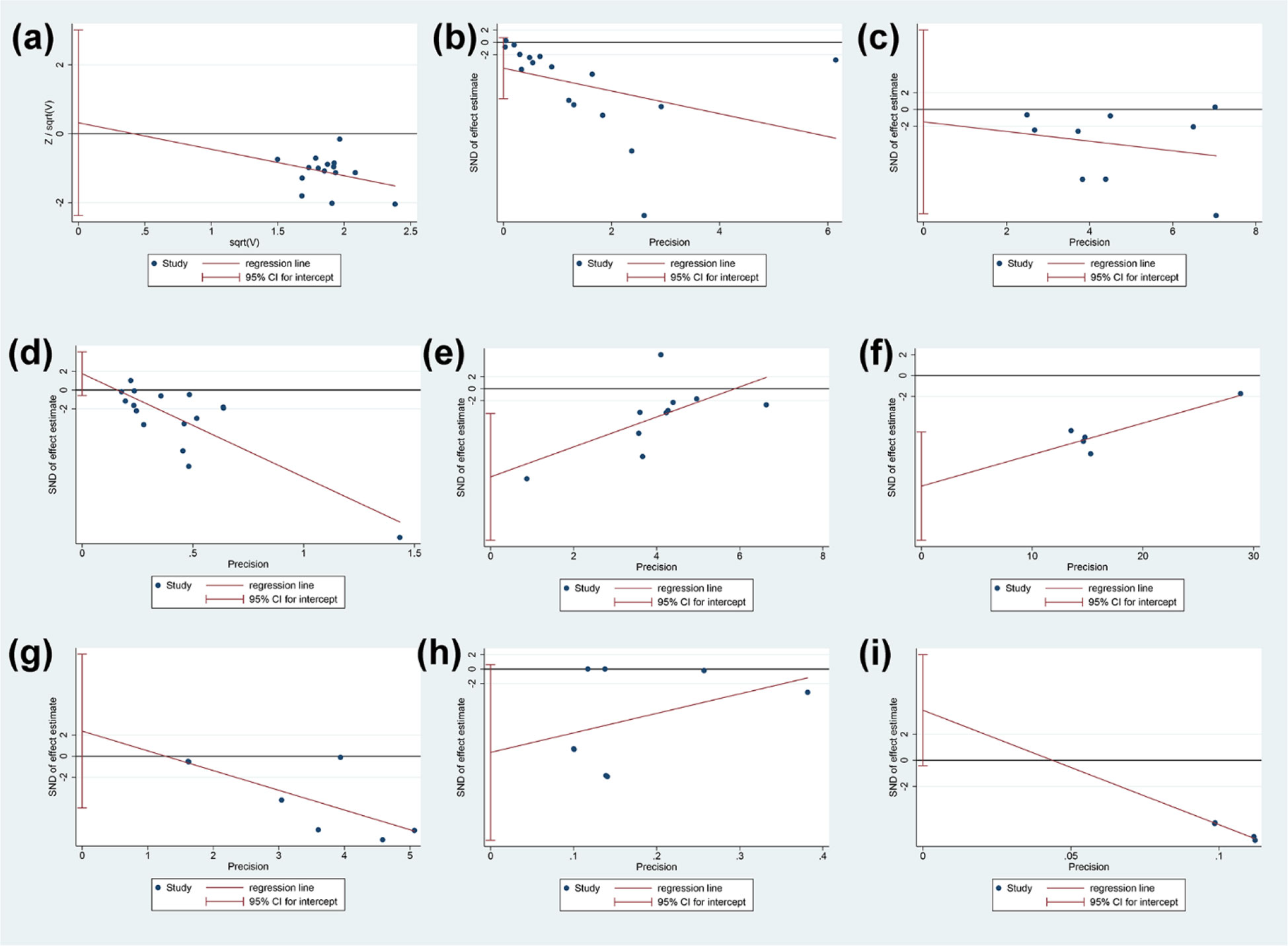
Figure 14 Publication bias results (A) adverse events of total glucosides of paeony; (B) CRP of total glucosides of paeony; (C) DAS28 of total glucosides of paeony; (D) ESR of total glucosides of paeony; (E) RF of total glucosides of paeony; (F) CRP of curcumin; (G) DAS28 of curcumin; (H) ESR of curcumin; (I) RF of curcumin.
3.19.4 Meta-regression results
The results of meta-regression showed that the duration of intervention was not the source of heterogeneity in DAS28 (P=0.065), ESR (P=0.890), CRP (P=0.324), RF (P=0.271) and adverse events (P=0.859) of total glucosides of paeony. The duration of intervention was also not the source of heterogeneity in DAS28 (P=0.228) of curcumin. However, the duration of intervention may be the source of heterogeneity in ESR (P<0.0001) and CRP (P=0.005) of curcumin (Figure 15).
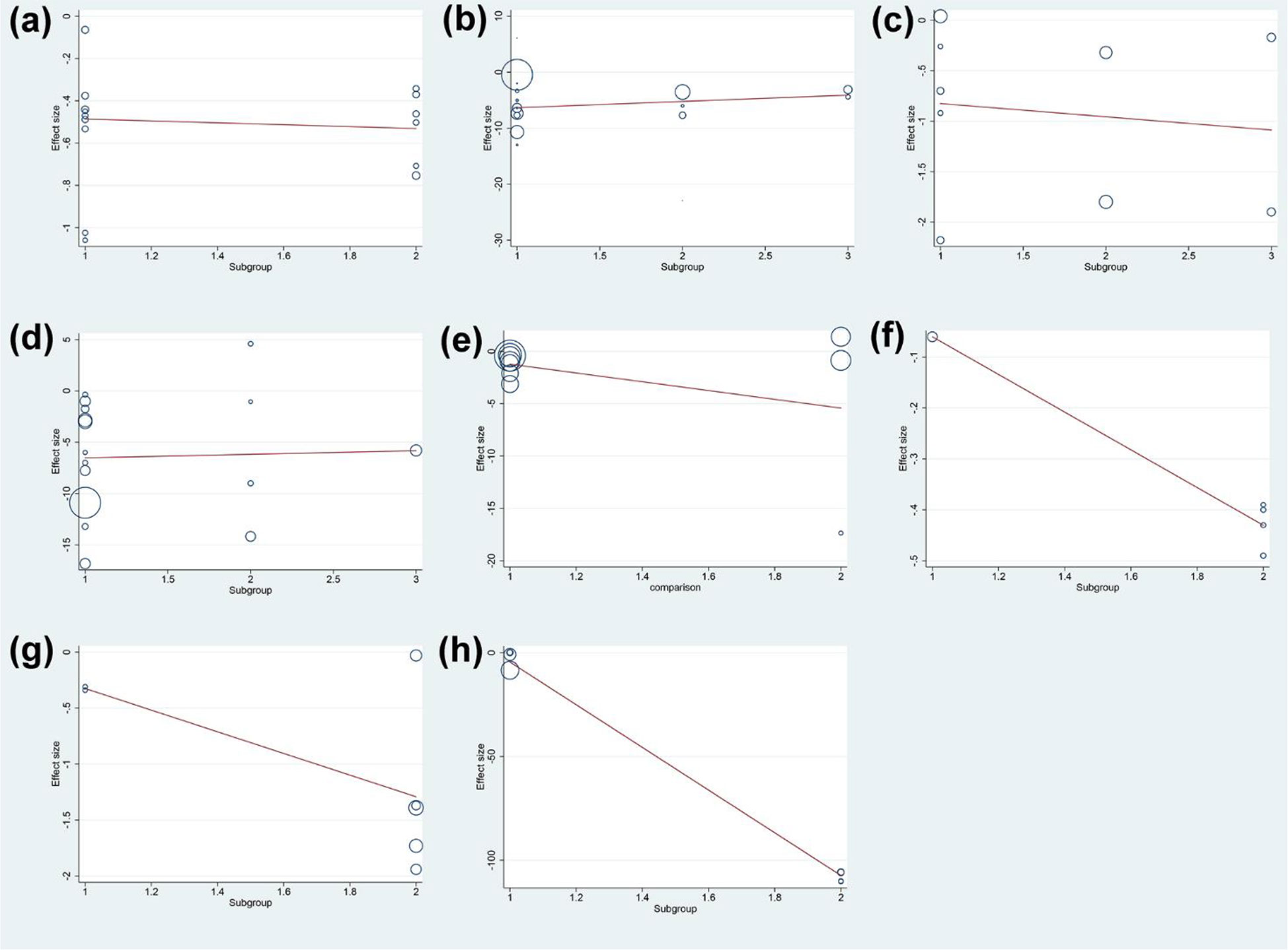
Figure 15 Meta-regression Results of the duration of intervention (A) adverse events of total glucosides of paeony; (B) CRP of total glucosides of paeony; (C) DAS28 of total glucosides of paeony; (D) ESR of total glucosides of paeony; (E) RF of total glucosides of paeony; (F) CRP of curcumin; (G) DAS28 of curcumin; (H) ESR of curcumin.
Meanwhile, the dose was not the source of heterogeneity in DAS28 (P=0.283) and adverse events (P=0.646) of total glucosides of paeony. However, the dose may be the source of heterogeneity in ESR (P=0.021), CRP (P=0.001) and RF (P=0.047) of total glucosides of paeony. In addition, the dose was not the source of heterogeneity in DAS28 (P=0.638), ESR (P=0.553), CRP (P=0.704), RF (P=0.885) (Figure 16).
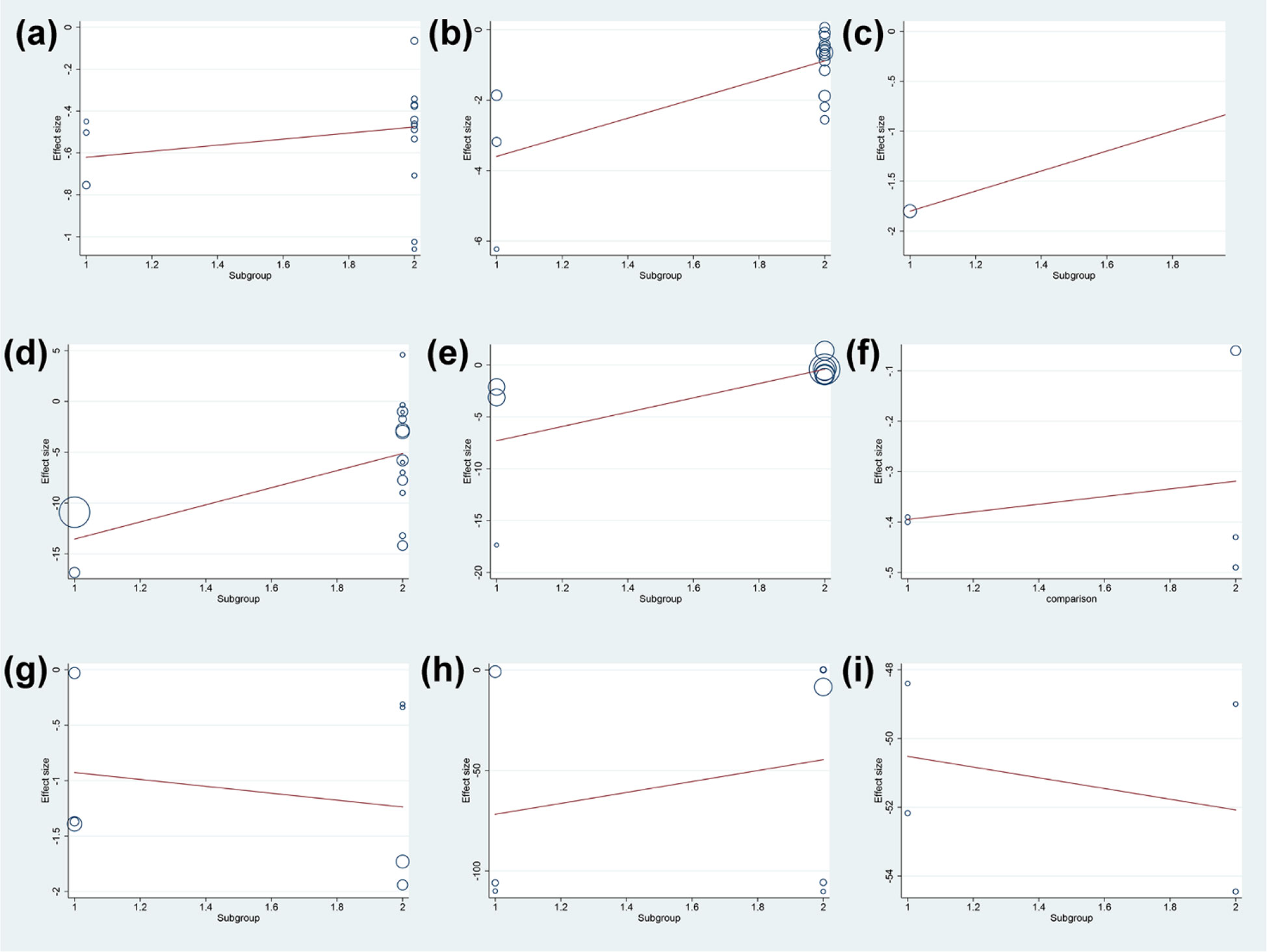
Figure 16 Meta-regression Results of dose (A) adverse events of total glucosides of paeony; (B) CRP of total glucosides of paeony; (C) DAS28 of total glucosides of paeony; (D) ESR of total glucosides of paeony; (E) RF of total glucosides of paeony; (F) CRP of curcumin; (G) DAS28 of curcumin; (H) ESR of curcumin; (I) RF of curcumin.
3.19.5 Evidence quality assessment
The evidence quality of total glucosides of paeony and curcumin was assessed by GRADE pro CDT (Tables 4, 5). The recommended rating for all results is low to very low.
4 Discussion
4.1 Pomegranate extract for RA
Punica granatum L. is rich in vitamins, minerals, organic acids, proteins (79), and also rich in phenolic and flavonoid active ingredients. Pomegranate peel polyphenols mainly include gallic tannin, ellagitannin, ellagic acid, chlorogenic acid, gallic acid, catechin, epicatechin, anthocyanin, ferulic acid and quercetin (80, 81). Studies have shown that pomegranate has been consumed as a medicinal plant for thousands of years and has various properties such as anti-inflammatory, antioxidant, anti-cancer, anti-diabetic, anti-hyperlipidemic, anti-hypertensive and cardiovascular protection (82, 83). In addition, animal (84) and human (85, 86) studies did not report any serious adverse outcomes following pomegranate consumption. Animal studies have shown that ethanolic extract of pomegranate peel can significantly increase paw withdrawal latency and reduce adverse histological changes and arthritis scores (87), and reduce serum RF, MDA, IL-1β and TNFα. Karwasra et al. reported that Pomegranate extract can significantly inhibit hind paw swelling and bone destruction, and reduce complications such as erythema, improve joint inflammation, synovial hyperplasia, inflammatory cell infiltration, periarticular bone resorption, bone erosion and joint space narrowing (88).
In vitro studies indicated that pomegranate seed oil and fermented pomegranate juice extract can inhibit COX and lipoxygenase involved in triggering the inflammatory cascade (89). The main fatty acid punicic acid in pomegranate seed oil inhibits prostaglandin biosynthesis (90) and inhibits neutrophil activation and lipid peroxidation outcomes (91). In addition to the inhibitory effect of pomegranate on eicosanoid production, pomegranate also exerts its anti-inflammatory effect by inhibiting the p38-MAPK pathway and the transcription factor NF-κB (89–91). Extracts of phenolic compounds present in pomegranate peel are potential scavengers of diphenylpicrylhydrazine (DPPH) free radicals. This may be because the phenolic substances in the peel extract have strong proton-donating ability and can agglomerate with hydroxyl groups to stabilize free radicals (92, 93). Morvaridzadeh et al. demonstrated that pomegranate juice caused a non-significant increase in TAC and paraoxonase and a non-significant decrease in MDA concentration in humans (94).
The limitation of this systematic review is that it contains only 1 RCT, and they found that compared with placebo, pomegranate extract reduced DAS28 scores, improved joint swelling and tenderness, decreased ESR levels, and increased GPx concentrations.
4.2 Quercetin for RA
Quercetin is a flavonoid commonly found in fruits and vegetables such as onions, apples, beans and various berries (95). It has anti-inflammatory, anti-angiogenic, anti-cancer, hepatoprotective, cardiovascular, anti-aging, and neuroprotective potentials (96–103). In a large number of preclinical or clinical studies, quercetin has also been shown to have a certain effect on RA (30, 104). Preclinical studies have found that quercetin can regulate Th17/Treg cell balance, reduce Th17 cell-related cytokines (IL-17A, IL-21 and IL-23), and increase Treg cell-related cytokines (IL-10 and TGF-β), and reduce the level of autoantibodies (105–109). Quercetin also reduces pro-inflammatory cytokines [TNF-α, IL-1β, IL-6, IL-8, prostaglandin (PG) E2, COX-2, inducible nitric oxide synthase (iNOS) and prepro-ET-1] by modulating MAPKs (ERK, p38, JNK), NF-κB and Nrf2/HO-1 signaling pathways, and the lncRNA XIST/miR-485/PSMB8 axis (110–116). In terms of bone protection, quercetin can modulate mTOR, ERK, IκB-α and AMPK signaling pathways and inhibit the expression of receptor activator of NF-κB ligand (RANKL) in FLS (30–32). It can also inhibit the activation of MAPKs (ERK, p38, JNK) and NF-κB signaling pathway to inhibit the expression of MMP-1 and MMP-3 in FLS (117–119).
Only two RCTs reported quercetin in the treatment of RA. Javadi et al., 2017 found that DAS-28 decreased and serum TNF-α levels were significantly reduced after quercetin intervention compared to placebo (28, 29). However, Bae et al., 2009 showed a negative result (30). Because meta-analyses could not be combined, the results need to be interpreted with caution.
4.3 Resveratrol for RA
Resveratrol is a natural polyphenolic antioxidant that is abundantly present in a variety of plants, especially red grape skins, and has pharmacological properties, including antioxidants, reactive oxygen species with scavenging, anticancer, and anti-inflammatory effects (120–123). Previous studies have shown that resveratrol has beneficial effects on the occurrence and development of RA (124–126). Resveratrol acts directly on mitochondrial production of ROS. Its ROS scavenging potential may be driven by the dissociative junction of leaked electrons from the respiratory chain (127–129). Several studies have reported that resveratrol can reduce the activity of COX-1, COX-2, and reduce the expression of potent inflammatory parameters such as prostaglandins and leukotrienes (130, 131). In addition, resveratrol reduced TNF-α and IL-1β levels in rats with adjuvant arthritis (132). Cheon et al. demonstrated that resveratrol supplementation significantly reduced inflammation, pannus formation, and cartilage damage in mice with collagen-induced arthritis, and reduced bone destruction (133).
In this systematic review, only 1 RCT reported resveratrol in the treatment of RA. Khojah et al., 2018 found that resveratrol treatment reduced swollen and tender joint counts, decreased DAS28, and decreased serum CRP, ESR, hypocarboxylated osteocalcin, MMP3, TNF-α, and IL-6. More RCTs are needed for further research in the future (31).
4.4 Garlic extract for RA
Garlic is a functional food that has been used worldwide for thousands of years. It is rich in bioactive compounds including allicin, ajoene, s-allyl cysteine, s-methyl cysteine, diallyl disulfide (DADS), diallyl sulfide (DAS), alliin, amino acids, polysaccharides and different polyphenols (134–136). The main phenolic compounds are β-resorcinol, gallic acid, pyrogallol, quercetin, rutin and protocatechuic acid (137). Garlic improves immune system function by stimulating certain cell types, such as macrophages and lymphocytes, and reduces cytokine secretion (138). The anti-inflammatory effects of allicin and DAS have been demonstrated in several studies (139, 140). Garlic-derived compounds can reduce serum TNF-α, IL-6 and CRP levels by inhibiting cell signaling pathways, including COX-2 and inhibit NF-κB activation (141, 142). In addition, scientific studies have reported the analgesic and anti-fatigue effects of garlic (143–145). The antioxidant properties of garlic may help reduce pain in patients with rheumatoid arthritis (146). For example, garlic supplements can relieve pain in patients with knee osteoarthritis (OA) (147–150). Hussain and Salalah (149) showed that 900 mg of garlic (capsules) per day for 8 weeks significantly reduced TNF-α concentrations in patients with knee OA (17). Treatment with garlic (400 mg/kg) reduced writhing caused by PG, a dose that showed similar effects to aspirin, one of the most commonly used analgesics (33, 151, 152).
Only one RCT reported Garlic extract in the treatment of RA. Moosavian et al., 2020 found that Garlic extract intervention improved antioxidant levels (increased TAC, decreased MDA), improved quality of life, and improved inflammation (decreased CRP and TNF-α), decreased joint pain and tenderness, and decreased DAS28 compared with the placebo group (32, 33).
4.5 Total glucosides of paeony for RA
Total glucosides of paeony are extracted from the dried roots of Paeonia lactiflora (153), including paeoniflorin, hydroxypaeoniflorin, paeoniflorin, paeoniflorin, and benzoylpaeoniflorin (154). Total glucosides of paeony have a wide range of anti-inflammatory and immunomodulatory effects, and have been widely used in the treatment of autoimmune diseases, including RA, systemic lupus erythematosus (SLE), psoriasis, allergic contact dermatitis, etc. (155–159). In terms of intervening in RA, total glucosides of paeony regulate the function and activation of immune cells in RA, reduce the production of inflammatory mediators, and restore abnormal signaling pathways (160, 161). Total Paeoniflorin can balance immune cell subsets (such as macrophage-like synoviocytes, fibroblast-like synoviocytes, etc.). Paeoniflorin can regulate signaling pathways (GPCR pathway, MAPKs/NF-κB pathway, PI3K/Akt/mTOR pathway, JAK2/STAT3 pathway, TGFβ/Smads, etc.) in experimental arthritis FLS (160, 162–165).
This meta-analysis showed that total glucosides of paeony may improve the clinical manifestations of RA (decrease DAS28, ACR20 and ACR70) and inhibit inflammatory (reduce CRP, ESR, RF, IL-6 and TNF-α). Meanwhile, the addition of total glucosides of paeony may reduce the incidence of adverse events.
4.6 Tea polyphenols for RA
Tea polyphenols (such as catechins, etc.) are the most abundant in tea (166, 167). Therapeutic benefits of green tea have been seen in neurodegenerative, inflammatory, cardiovascular, and various cancers (168, 169). Catechins have demonstrated their anti-inflammatory effects in many studies related to pathological conditions in which inflammation is a core driver (170). Extensive in vitro studies have shown that catechins have promising applications in the treatment of RA, with differential modulation of cartilage, bone, and synovial fibroblast activity (171). In cartilage, catechins have been found to inhibit IL-1β-induced inducible NOS (iNOS) and COX-2 expression by inhibiting IκBα phosphorylation and proteasomal degradation (172, 173). Catechin also inhibits IL-1β-induced phosphorylation of c-Jun, thereby preventing activating protein 1 (AP-1) from binding to DNA (174). Akhtar and Haqqi found that when IL-1β was inhibited, IL-6, IL-8 and TNF-α were also down-regulated due to the inhibition of NF-κB (175). An early study suggests that prophylactic consumption of green tea may help improve inflammation and reduce cartilage destruction associated with different forms of arthritis (176). In bone biology, catechins are thought to reduce the amount of osteoclast formation by reducing osteoblast differentiation (177). Catechin blocks the receptor activator of RANKL-mediated activation of JNK and NF-κB pathways to inhibit the expression of the transcription factor NFATc1 required for osteoclast differentiation (178). Catechins can regulate B cell activating factors belonging to the TNF family (BAFF)/PI3K/AKT/mTOR pathway to induce apoptosis, also in B lymphocytes (179). In regulating apoptosis, catechin treatment selectively downregulated Mcl-1 (anti-apoptotic protein) expression, thereby increasing the sensitivity of synovial fibroblasts to apoptosis (180). Studies have also shown that catechins can reduce the production of MMP-1, MMP-2 and MMP-3 by RA synovial fibroblasts to prevent further cartilage and bone destruction (181, 182). Therefore, although tea and tea polyphenols can neutralize the inflammatory effects of IL-1β and IL-6, they also effectively utilize TNF-α to play its basic function of regulating the uncontrolled proliferation of activated synovial fibroblasts to improve the functional status of arthritis joints.
This meta-analysis showed that tea polyphenols may improve the clinical manifestations of RA (decrease DAS28, ACR20 and ACR70) and inhibit inflammatory (reduce CRP and ESR). Meanwhile, the addition of total glucosides of paeony may not increase the incidence of adverse events.
4.7 Puerarin for RA
Puerarin exists in the roots of the genus Pueraria (common name Pueraria), which is isolated from Pueraria and other species (183–185). Numerous health benefits have been attributed to puerarin, namely antioxidant (186), anti-inflammatory (187), neuroprotective (188), liver protection (189), anticancer (190), antidiabetic (191), cardioprotective (192) and anti-atherosclerotic effect (193). In terms of bone protection, puerarin is an effective compound that inhibits bone resorption and improves bone structure. It can stimulate osteoblast differentiation and inhibit osteoclastogenesis at the same time (183, 194). In addition, the Ca 2+ content in the culture supernatant decreased after puerarin treatment. Puerarin can stimulate bone formation and regulate bone metabolism by inhibiting bone resorption (195). Multiple studies have found that puerarin attenuates inflammation and oxidation in mice with collagen antibody-induced arthritis through the TLR4/NF-κB signaling pathway (196). Puerarin derivative (4AC) antioxidant and inhibits TNF-α activity via MAPKs/NF-κB signaling pathway in RAW264.7 cells and collagen-induced arthritis rats (197). Puerarin inhibits inflammation and ECM degradation through the Nrf2/HO-1 axis in chondrocytes and reduces pain symptoms in osteoarthritis mice (198).
The anti-atherosclerotic properties of puerarin also include inhibition of lipopolysaccharide or ovalbumin-induced inflammation (199–201), protection of endothelial cells from damage induced by oxidized LDL or Aß40, and reduction of lipid accumulation in vessel walls (199–203). The vasoprotective effect of puerarin inhibits vascular smooth muscle cells (204) and protects against ischemia and reperfusion injury (205–207). This systematic review showed that puerarin may decrease DAS28, ESR, CRP, IL-6.
4.8 Hesperidin for RA
Hesperidin is a flavonoid that is abundant in citrus fruits. Hesperidin has many biological functions, including antioxidant, anti-inflammatory, antiviral, and anticancer activities (208). Ahmad et al. found that after treatment with hesperidin, plasma CML and IgG PTD levels were restored to 93% and 16%, respectively, through the free radical scavenging activity of hesperidin, thereby alleviating RA disease by reducing the concentration of AGEs. Therefore, the use of hesperidin may help reduce the severity of RA disease. Umar et al. found that hesperidin may inhibit collagen-induced arthritis by inhibiting free radical load and reducing neutrophil activation and infiltration (209). Qi et al. (210) found that in complete Freund’s adjuvant-induced arthritis in mice, hesperidin inhibited synovial cell inflammation and macrophage polarization by inhibiting the PI3K/AKT pathway. Liu et al. (211) found that hesperidin derivative 11 inhibited the proliferation of fibroblast-like synoviocytes by activating frizzled-related protein 2 secreted in adjuvant arthritis rats. Li et al. found the therapeutic effect of hesperetin on adjuvant arthritis in rats by inhibiting the JAK2-STAT3 signaling pathway (212). Hesperidin also promotes the anti-inflammatory and analgesic activities of Siegesbeckia pubescens makino by inhibiting COX-2 expression and inflammatory cell infiltration (213). This systematic review showed that hesperidin may improve RA symptoms.
4.9 Crocus sativus L. extract for RA
Crocus sativus L. extract is well known in herbal medicine and has attracted the attention of researchers for its properties, especially its anti-inflammatory and proliferative abilities in bone and cartilage destructive diseases (214, 215). Among these bioactive components, there are four recognized components that may be associated with the therapeutic potential of saffron, including crocin, saffron flavonoids, saffron aldehyde, and saffron (216, 217). Recent studies have revealed other therapeutic and pharmacological activities of saffron (218–225), such as neuroprotection, neurogenetics, antidepressant, antiapoptotic, antioxidant, and anti-inflammatory. One study found that crocin modulates serum levels of enzymatic and non-enzymatic inflammatory cytokines, including MMP-13, MMP-3, MMP-9, HAases, TNF-α, IL-1β, NF-κB, IL -6, COX-2 and PGE2 and ROS media (226). Crocin also increased levels of GSH, SOD, CAT and GST. In addition, inhibition of the exoglycosidase cathepsin-D and tartrate-resistant acid phosphatase in the bone near the joint by crocin protects bone resorption (226). Rasol et al. found that TNF-α and IL-1β levels were decreased and SOD and GR activities were increased after crocin intervention (226). Hu et al. found that paw swelling and ankle diameter were significantly reduced in crocin-treated rats. Histological analysis also showed reduced inflammation in joints and other organs, such as the spleen. In addition, TNF-α and TGF-β1 levels were decreased in synovial tissue (227). In a similar study, Liu et al. found that MMP-1, MMP-3, and MMP-13 protein expression levels were decreased in RA rats after crocin intervention (228). Li et al. showed similar results, suggesting that crocin had a positive effect on RA-induced rats (229). In an in vitro study, Li et al. demonstrated that crocin at 500 µM (5,000 mg/ml) reduced levels of TNF-α, IL-1β, and IL-6 in human FLS. Furthermore, crocin caused lower levels of p-IκBα, p-IκB kinase α/β and p65 expression, demonstrating its effect on the NF-κB pathway (230). Wang et al. showed that crocin inhibits Wnt/β-catenin and Wnt signaling pathways to reduce pain-related cytokines, and glial activation may alleviate RA-induced neuropathic pain in rats (231).
However, this meta-analysis showed that the efficacy of Crocus sativus L. extract may not be significantly different from the control group.
4.10 Ginger extract for RA
Ginger has been cultivated in China and other countries around the world since ancient times as a source of medicinal plants for spice and therapeutic benefits (232). The main components of ginger are 6-shogaol, ginger oil terpene, water fennel, camphor terpene, gingerol, eucalyptus, etc. (232–234). Evidence suggests that consuming ginger may help relieve joint pain associated with RA (235). Kiuchi et al. discovered the potential of ginger to inhibit the synthesis of prostaglandins, which are key to inflammation. Further research found that ginger exhibits anti-inflammatory activity by inhibiting the biosynthesis of prostaglandins and leukotrienes (236). Ribel-Madsen et al. (237) conducted the in vitro anti-inflammatory effect of ginger, and ginger-treated synoviocytes showed similar inhibitory effects as betamethasone by inhibiting the production of cytokines IL-1 and IL-6. Yang et al. observed the analgesic and anti-inflammatory effects of 6-gingerol (238). Ojewole observed potential analgesic and anti-inflammatory activity of ginger, which can be used to reduce pain and inflammation caused by arthritis (239). Srivastava et al. observed the antiarthritic activity of ginger in patients who independently experienced RA, OA and muscle discomfort (240). The beneficial effect of ginger on reducing RA-related pain may be due to the inhibition of prostaglandin and leukotriene biosynthesis (240). van Breemen et al. found that 10-gingerol, 8-shogaol, and 10-shogaol strongly inhibited COX 2, thereby significantly reducing inflammation (241). In addition, ginger can inhibit the biosynthesis of leukotrienes by inhibiting 5-lipoxygenase (242). Nurtjahja-Tjendraputra et al. also demonstrated the inhibitory effect of ginger on COX-1 activity (243). One study found that components of ginger significantly inhibited the release of pro-inflammatory cytokines (IL-12, TNF-α and IL-1 β) and pro-inflammatory chemokines in LPS-induced macrophages (244). 6-Gingerol significantly inhibits Ikβα phosphorylation, NF-κβ nuclear activation and PKC-α translocation, which in turn inhibits Ca mobilization and disruption of mitochondrial membrane potential in LPS-stimulated macrophages, thereby inhibiting inducible nitric oxide synthase and TNF-α express and reduce inflammation (245). This systematic review showed that Ginger extract may decrease DAS28.
4.11 Cinnamon extract for RA
Cinnamon, one of the most commonly used spices in the world and one of the oldest herbal medicines used to treat certain diseases and inflammations, has been used to treat RA in China for nearly 2000 years (246). Cinnamon can modulate immune system function by modulating anti-inflammatory and pro-inflammatory gene expression (247–249). Cinnamaldehyde is the main active component of cinnamon, and its anti-inflammatory effect has been observed in several studies (250–252). Several other flavonoids, including anti-inflammatory hesperidin and quercetin, have also been extracted from cinnamon (253, 254). Scientific evidence suggests that cinnamon extract can be used to modulate the immune system, as well as prevent and treat inflammation (255). The beneficial effects of cinnamon extract and its polyphenols on reducing serum levels of TNF-α, CRP and IL-6, as well as improving clinical symptoms and antioxidant activity have been reported in animal models (256). In vitro experiments also demonstrated the beneficial effects of cinnamon polyphenols in improving immune responses by modulating the expression of pro- and anti-inflammatory cytokine genes (257, 258). In experimental arthritis studies, cinnamaldehyde in cinnamon significantly inhibits joint disease in experimentally arthritic animals. Cinnamaldehyde can not only significantly reduce the IL-6 content of inflammatory mediator TNF-α in peripheral mononuclear cells of RA patients (259), but also inhibit the release of IL-1β and matrix MMP-13 from synovial fibroblasts in arthritis patients (260). Cinnamaldehyde can significantly reduce the levels of TNF-α, IL-6 and IL-1β in the peripheral blood of collagen-induced arthritic rats, and significantly increase the content of the anti-inflammatory factor IL-10, exerting a systemic anti-inflammatory effect (261). Liu et al. found that cinnamaldehyde may affect the production of IL-1β by inhibiting HIF-1α, and may affect the maturation of IL-1β by regulating the formation of NLRP3 inflammasome through the succinate/HIF-1α axis (262).
This systematic review showed that Ginger extract may decrease DAS28, the number of tender and swollen joints, and serum CRP and TNF-α levels.
4.12 Sesamin for RA
Sesame is an important traditional health food that has been used in Asian countries for thousands of years to improve nutritional status and prevent various diseases (263). Sesame seeds contain high amounts of oil and protein, as well as various lignans (such as sesamin) (264, 265). Several studies have shown that sesamin (one of the most abundant lignans in sesame) has various physiological functions, including antioxidant, antihypertensive, anti-obesity and hypolipidemic effects (266–269). The anti-inflammatory properties of sesame compounds have been reported in rat models (270). The results of another study showed that sesamin inhibited lipopolysaccharide-induced inflammation by inhibiting p38 mitogen-activated protein kinase and NF-kB, which are the major pathways regulating cytokine production. Based on this result, sesamin may also prevent cartilage degeneration in other joint diseases such as RA (271, 272).
This systematic review showed that sesamin may improve the joint pain, decrease the number of tender joints, and decrease serum hs-CRP, TNF-α and COX-2 levels.
4.13 Cranberry extract for RA
Cranberry (Vaccinium macrocarpon) juice has strong antioxidant activity, mainly containing polyphenolic compounds such as flavonols (myricetin and quercetin), anthocyanins and procyanidins (273). Clinical studies have shown that cranberry juice has beneficial effects on biomarkers of cardiovascular disease risk (274, 275). Several intervention studies have found that cranberry has beneficial effects on biomarkers of oxidative stress, dyslipidemia, and inflammation in healthy people (276, 277) and in patients with type 2 diabetes (278) and metabolic syndrome (279). Studies have found that quercetin (flavonol), which is abundant in cranberry, can lead to a significant down-regulation of the nuclear factor kappa B (NF-κB) pathway (280). Additionally, resveratrol has been shown to be another abundant polyphenol in cranberries. It can inhibit the expression of inflammatory genes related to cardiovascular disease by activating NF-κB and Janus kinase/signal transducer and transcriptional pathway activator in cultured cells (273). NF-κB regulates the expression of many pro-inflammatory genes, including adhesion molecules, IL-6, and TNF-α. Other components present in cranberries, such as proanthocyanidins, anthocyanins, hydroxycinnamic acid, and acetylsalicylic acid, inhibit NF-κB activation (275).
This systematic review showed that Cranberry extract may decrease DAS28, and serum ESR and CRP.
4.14 Olive extracts and oil for RA
The current study found that olive oil contains high amounts of polyphenolic compounds. Numerous studies have found polyphenolic extracts of olive oil as antioxidants to prevent and treat cardiovascular disease and prevent certain types of cancer, as well as reduce the incidence of coronary heart disease and stroke (281–283). Furthermore, it has been shown to inhibit IL-1β-induced MMP, TNF-α and IL-6 production in the SW982 human synovial fibroblast cell line (284). Polyphenol extracts also down-regulated COX-2 and mPGE-1 induced by IL-1β. In addition, it inhibits MAPK phosphorylation and NF-κB activation (285, 286). This systematic review showed that Olive oil may decrease DAS28, relieve joint pain and decrease the number of painful joints and the number of swollen joints. The study also found that a Mediterranean diet centered on olive oil can reduce the risk of autoimmune diseases such as RA (287, 288), which is strong evidence in the treatment of RA.
4.15 Curcumin for RA
Curcumin, a yellow pigment and active component of turmeric (Curcuma longa), is one of the well-known natural compounds with a wide range of pharmacological activities and potential immunomodulatory properties (289, 290). Curcumin effectively inhibits the production of several pro-inflammatory mediators by mediating multiple inflammatory signaling pathways (such as JAK-STAT signaling pathway/P38 MAPK signaling pathway, NF-κB signaling pathway, etc.), including TNF-α, IL-1β, IL-6, IL-12 and IL-8 (291–294). Curcumin has a high ability to modulate inflammation in RA by suppressing pro-inflammatory immune cell populations and suppressing the production of immune-inflammatory cytokines and chemokines (295, 296). Studies have found that curcumin can significantly block the expression of IL-6 in FLS stimulated by IL-1β in RA patients (297). Curcumin can also reduce the protein expression levels of IL-6, IL-8, MCP-1, MMP-1 and MMP-3 in FLS of RA patients (298). Several studies on animal models of RA also confirmed this finding. Curcumin can reduce the production of pro-inflammatory cytokines, including TNF-α, IL-1β, IL-6 and MCP-1 (299–304). Curcumin has also been found to inhibit the activation, proliferation and differentiation of naive CD4+ T cells into T helper (TH) 1 and TH 17 subtypes. These two cells play a key role in the pathogenesis of RA by producing key pro-inflammatory cytokines including TNF-α, IFN-γ, IL-17, IL-21 and IL-23 and are responsible for joint and bone destruction (305, 306). In addition, curcumin has a strong ability to induce regulatory T cell (Treg) differentiation and inhibit TH1- and TH17-mediated inflammatory responses (306). This meta-analysis showed that curcumin may decrease DAS28, CRP, ESR and RF.
4.16 Mechanisms of other dietary polyphenols with medicinal potential
Dietary polyphenols are currently widely used as alternative therapies, and preclinical and clinical studies have demonstrated their efficacy in the treatment of RA. In addition to the 15 dietary polyphenols (Cinnamon extract, Cranberry extract, Crocus sativus L. extract, Curcumin, Garlic extract, Ginger extract, Hesperidin, Olive oil, Pomegranate extract, Puerarin, Quercetin, Resveratrol, Sesamin, Tea polyphenols, Total glucosides of paeony) in this study, there are many other polyphenols in the world. The reason why this study did not include other polyphenols is that this study is about the systematic review and meta-analysis of RCTs, so these animal experiments were not included. In order to further provide future researchers with reference information for dietary polyphenols in the treatment of RA, we summarized dietary polyphenols with potential medicinal value. Dietary polyphenols derived from dietary fruits, vegetables and natural herbs for anti-rheumatic activity in the treatment of RA include Stilbenes, Phenolic acids, Flavonoids, etc. (307–310). Among them, phenolic acids such as benzoic acid and cinnamic acid (311). Hydroxybenzoic acids (including gallotannins and ellagitannins) also exhibit anti-RA effects (312). Natural flavonoids also exhibit strong anti-RA activity, such as anthocyanins, flavanols (catechins, epigallocatechin gallate), flavonoids (luteolin, apigenin), flavanones (naringenin), flavonols (quercetin and kaempferol), isoflavones (genistein, daidzein and glycitein) (313, 314). Some herbs have also been shown to exhibit prominent anti-RA activity, including Adhatoda vasica Nees (pyrroloquinazoline), Ajuga bracteosa wall (Withaferin-A, Ajugarin-I), Aconitum carmechaeli Debeaux (aconitine, benzoylmethasone), Barleria prionitis (triterpenoids), pine (pinitol) (315). These polyphenols play an anti-RA role mainly by regulating immune-inflammation-related signaling pathways, anti-oxidation-related signaling pathways and triggering synoviocyte/pathogenic immune cell programmed death (apoptosis, pyroptosis, ferroptosis) (316–319). At present, the most studied pathways are immune inflammation-related signaling pathways to reduce bone and joint damage, such as NFKB signaling pathways, TLR signaling pathways, TNF signaling pathways, etc. (320, 321). The antioxidant-related signaling pathways regulated by these polyphenols mainly include SIRT1 signaling pathway, Keap1-Nrf2-ARE pathway, NOX4/ROS signaling pathway, HO-1 signaling pathway and iNOS signaling pathway (320, 322, 323). These dietary polyphenols can also regulate programmed cell death signaling pathways to treat RA, such as MAPK pathway or PI3K/Akt signaling pathway and epigenetics-related regulatory signaling pathways (316, 324, 325).
4.17 Strength and limitations
The strengths of this research are that compared with previous studies, this study included the results of a meta-analysis and included more RCTs (48 RCTs were included in this study and involved 15 dietary polyphenols: Cinnamon extract, Cranberry extract, Crocus sativus L. extract, Curcumin, Garlic extract, Ginger extract, Hesperidin, Olive oil, Pomegranate extract, Puerarin, Quercetin, Resveratrol, Sesamin, Tea polyphenols, Total glucosides of paeony). Sensitivity analysis, publication bias assessment, meta-regression, and quality of evidence ratings were also performed in this meta-analysis.
The limitations of this research are that: (1) There is obvious heterogeneity in outcomes. According to meta-regression, part of the heterogeneity of total glucosides of paeony and curcumin may come from drug dosage and intervention time. The remaining heterogeneity may be due to the selection of the population, the choice of dietary polyphenol preparations for treatment of dietary polyphenols, and information biases during data collection. (2) Although a total of 48 RCTs were included, except for total glucosides of paeony, each type of dietary polyphenol supplements included no more than 10 RCTs, and the number of participants in each RCT was mostly less than 100. (3) The RCT languages included in this study were only Chinese and English, and no RCTs in other languages were found, which may have an impact on the results. (4) The follow-up time of the RCTs ranged from 8 to 48 weeks, and there was no observation for more than 3 years and before 8 weeks, which may affect the generality of the results. (5) The RCTs in this research have a high risk of bias. The authors of some RCTs are funded or employed by drug manufacturers, which may introduce bias.
4.18 Inspiration for the future
Through the systematic review and meta-analysis of this study, it can be found that the research on the efficacy and mechanism of various dietary polyphenols in relieving RA has made significant progress. Among them, curcumin and total glucosides of paeony have improved the treatment of rheumatoid arthritis. Symptoms, inhibition of inflammation and other outcome indicators. In general, dietary polyphenols can be used as an effective anti-inflammatory drug in the adjuvant treatment of RA, and can be used for daily dietary supplementation with relatively good safety.
However, there are still some knowledge gaps in current clinical research and basic research, which need to be addressed in future research. In terms of basic research, most previous studies have cultured a single phenolic compound or a mixture of multiple phenolic compounds with synovial cells, lacking pharmacokinetic studies targeting dietary polyphenols in animal studies. This situation is even more lacking in clinical studies, and few clinical studies have explored the pharmacokinetics of dietary polyphenols in RA patients (such as, absorption and metabolism of polyphenols, and interactions with gut microbiota). For example, in previous studies, most studies using animal or cell culture models focused on the biological activity of polyphenols themselves. Moreover, few studies have focused on the microbial metabolism of polyphenols. In particular, the current study (326–328) showed that the α-diversity and β-diversity of gut microbiota in RA patients were significantly lower than those in healthy people. Among them, Bacteroidetes and Shiga toxoid-producing Escherichia coli were more abundant in RA patients, in contrast, Lactobacillus, Ravivibacterium isoprawizia, Enterobacter and Stinkbacter were less abundant in the RA group. More studies have found that the intestinal flora can affect the development of RA by regulating immune molecules (including immune cells (such as regulatory B cells and CD4 T helper T cells, etc.), immune factors, etc.) (329–332). Therefore, research on the interaction between dietary polyphenols and gut microbiota of RA disease is the main direction of future basic and clinical research. In addition, most dietary polyphenols are degraded by gut flora in the colon due to poor polyphenol absorption. The biological activity of dietary polyphenols may be partially attributed to microbial metabolites. The exact role or biological activity of microbial metabolites should be focused on in future studies.
4.19 Prospects
Dietary polyphenols are a group of biologically active phytochemicals with a wide range of sources, diverse structures, low toxicity, and good biological activity. In terms of curative effect in the treatment of RA, it is mainly attributed to the immuno-inflammation modulating activity of dietary polyphenols, which provides a new option for the comprehensive management strategy of RA, and dietary polyphenols also alleviate the adverse reactions of DMARDs. Since these dietary polyphenols are ubiquitously present in the diet, medication adherence may be better in RA patients. Meanwhile, studies have found that some dietary polyphenols can reduce the side effects of glucocorticoids (such as bone destruction, drug resistance, etc.) (333–338). Therefore, the current preliminary preclinical data on dietary polyphenols indicate that polyphenols have great potential in the treatment of RA, and the dietary types that have been carried out RCTs are mainly Cinnamon extract, Cranberry extract, Crocus sativus L. extract, Curcumin, Garlic extract, Ginger extract, Hesperidin, Olive oil, Pomegranate extract, Puerarin, Quercetin, Resveratrol, Sesamin, Tea polyphenols, Total glucosides of paeony. In addition, there are many dietary polyphenols such as rutin, chlorogenic acid, anthocyanins, luteolin, lignans, etc., which need extensive clinical research in the future to provide more clinical evidence for the future clinical treatment of RA. Dietary polyphenols have multi-target effects on signaling pathways in RA, so it is important to further explore the pharmacological mechanism of these dietary polyphenols, which will also provide the molecular structure core of lead compounds or new drugs for RA drug development. Based on this systematic review and meta-analysis, we look forward to clinicians and patients using dietary polyphenols as adjunctive therapy or in combination with other current DMARDs in a comprehensive management strategy for RA. In addition, since some polyphenols are mainly derived from herbs, it is necessary in clinical practice to study the pharmacokinetic results of the interaction of herbs as a multi-component drug (not only polyphenols) with DMARDs to prevent potential adverse drug reactions. Some polyphenol active substances have been formulated into new delivery systems to improve solubility, permeability and enhance the therapeutic effect in preclinical studies due to problems such as absorption. In the future, it is necessary to explore the efficacy and safety of this new type of administration.
5 Conclusion
Although the number of RCTs on dietary polyphenols is limited, existing evidence shows their potential benefits, mainly increasing DAS28, reducing CRP and ESR, and improving oxidative stress, etc. However, given the small number of patients recruited, the study designs varied widely between RCTs and the characteristics of RA patients varied; it is difficult to immediately extrapolate these results to RA patients in general. More RCTs are needed in the future to determine the efficacy and safety of dietary polyphenols.
Data availability statement
The original contributions presented in the study are included in the article/Supplementary Material. Further inquiries can be directed to the corresponding author.
Author contributions
ZL, WaX, QH and WeX are responsible for the study concept and design. ZL, WX, QH, WeX, HW, HL, HG, YC, MY, XY, LZ, KY, YD, ZH are responsible for the data collection, data analysis and interpretation; ZL, WaX and KY drafted the paper; ZH and WeX supervised the study; all authors participated in the analysis and interpretation of data and approved the final paper.
Conflict of interest
The authors declare that the research was conducted in the absence of any commercial or financial relationships that could be construed as a potential conflict of interest.
Publisher’s note
All claims expressed in this article are solely those of the authors and do not necessarily represent those of their affiliated organizations, or those of the publisher, the editors and the reviewers. Any product that may be evaluated in this article, or claim that may be made by its manufacturer, is not guaranteed or endorsed by the publisher.
Supplementary material
The Supplementary Material for this article can be found online at: https://www.frontiersin.org/articles/10.3389/fimmu.2023.1024120/full#supplementary-material
References
1. Scherer HU, Häupl T, Burmester GR. The etiology of rheumatoid arthritis. J Autoimmun (2020) 110:102400. doi: 10.1016/j.jaut.2019.102400
2. Radu AF, Bungau SG. Management of rheumatoid arthritis: An overview. Cells (2021) 10(11):2857. doi: 10.3390/cells10112857
3. Sparks JA. Rheumatoid arthritis. Ann Intern Med (2019) 170(1):ITC1–ITC16. doi: 10.7326/AITC201901010
4. Cush JJ. Rheumatoid arthritis: Early diagnosis and treatment. Med Clin North Am (2021) 105(2):355–65. doi: 10.1016/j.mcna.2020.10.006
5. Dai Y, Wang W, Yu Y, Hu S. Rheumatoid arthritis-associated interstitial lung disease: an overview of epidemiology, pathogenesis and management. Clin Rheumatol (2021) 40(4):1211–20. doi: 10.1007/s10067-020-05320-z
6. Safiri S, Kolahi AA, Hoy D, Smith E, Bettampadi D, Mansournia MA, et al. Global, regional and national burden of rheumatoid arthritis 1990-2017: a systematic analysis of the global burden of disease study 2017. Ann Rheum Dis (2019) 78(11):1463–71. doi: 10.1136/annrheumdis-2019-215920
7. Zhao J, Guo S, Schrodi SJ, He D. Molecular and cellular heterogeneity in rheumatoid arthritis: Mechanisms and clinical implications. Front Immunol (2021) 12:790122. doi: 10.3389/fimmu.2021.790122
8. Wang GY, Zhang SL, Wang XR, Feng M, Li C, An Y, et al. Remission of rheumatoid arthritis and potential determinants: a national multi-center cross-sectional survey. Clin Rheumatol (2015) 34(2):221–30. doi: 10.1007/s10067-014-2828-3
9. van Vollenhoven RF. Sex differences in rheumatoid arthritis: more than meets the eye. . BMC Med (2009) 7:12. doi: 10.1186/1741-7015-7-12
10. Burmester GR, Pope JE. Novel treatment strategies in rheumatoid arthritis. Lancet (2017) 389(10086):2338–48. doi: 10.1016/S0140-6736(17)31491-5
11. Dong Y, Cao W, Cao J. Treatment of rheumatoid arthritis by phototherapy: advances and perspectives. Nanoscale (2021) 13(35):14591–608. doi: 10.1039/d1nr03623h
12. Kerschbaumer A, Sepriano A, Smolen JS, van der Heijde D, Dougados M, van Vollenhoven R, et al. Efficacy of pharmacological treatment in rheumatoid arthritis: a systematic literature research informing the 2019 update of the EULAR recommendations for management of rheumatoid arthritis. Ann Rheum Dis (2020) 79(6):744–59. doi: 10.1136/annrheumdis-2019-216656
13. Nagy G, Roodenrijs NMT, Welsing PM, Kedves M, Hamar A, van der Goes MC, et al. EULAR definition of difficult-to-treat rheumatoid arthritis. Ann Rheum Dis (2021) 80(1):31–5. doi: 10.1136/annrheumdis-2020-217344
14. Maity S, Wairkar S. Dietary polyphenols for management of rheumatoid arthritis: Pharmacotherapy and novel delivery systems. Phytother Res (2022) 36(6):2324–41. doi: 10.1002/ptr.7444
15. Tresserra-Rimbau A. Dietary polyphenols and human health. Nutrients (2020) 12(9):2893. doi: 10.3390/nu12092893
16. Zeng L, Yang T, Yang K, Yu G, Li J, Xiang W, et al. Efficacy and safety of curcumin and curcuma longa extract in the treatment of arthritis: A systematic review and meta-analysis of randomized controlled trial. Front Immunol (2022) 13:891822. doi: 10.3389/fimmu.2022.891822
17. Cao H, Ou J, Chen L, Zhang Y, Szkudelski T, Delmas D, et al. Dietary polyphenols and type 2 diabetes: Human study and clinical trial. Crit Rev Food Sci Nutr (2019) 59(20):3371–9. doi: 10.1080/10408398.2018.1492900
18. Luca SV, Macovei I, Bujor A, Miron A, Skalicka-Woźniak K, Aprotosoaie AC, et al. Bioactivity of dietary polyphenols: The role of metabolites. Crit Rev Food Sci Nutr (2020) 60(4):626–59. doi: 10.1080/10408398.2018.1546669
19. Yabas M, Orhan C, Er B, Tuzcu M, Durmus AS, Ozercan IH, et al. A next generation formulation of curcumin ameliorates experimentally induced osteoarthritis in rats via regulation of inflammatory mediators. Front Immunol (2021) 12:609629. doi: 10.3389/fimmu.2021.609629
20. Shakoor H, Feehan J, Apostolopoulos V, Platat C, Al Dhaheri AS, Ali HI, et al. Immunomodulatory effects of dietary polyphenols. Nutrients (2021) 13(3):728. doi: 10.3390/nu13030728
21. Lu J, Zheng Y, Yang J, Zhang J, Cao W, Chen X, et al. Resveratrol alleviates inflammatory injury and enhances the apoptosis of fibroblast-like synoviocytes via mitochondrial dysfunction and ER stress in rats with adjuvant arthritis. Mol Med Rep (2019) 20(1):463–72. doi: 10.3892/mmr.2019.1027
22. Page MJ, McKenzie JE, Bossuyt PM, outron I, Hoffmann TC, Mulrow CD, et al. The PRISMA 2020 statement: an updated guideline for reporting systematic reviews. BMJ (2021) 372:89. doi: 10.1186/s13643-021-01626-4
23. Deeks JJ, Higgins JP, Altman DG. Chapter 16: Special topics in statistics. In: Higgins JP, Green S, editors. Cochrane handbook for systematic reviews of interventions. UK: The Cochrane Collaboration (2020).
24. Deeks JJ, Higgins JP, Altman DG. Chapter 8: assessing risk of bias in included studies. In: Higgins JP Green S, editor. Cochrane handbook or systematic reviews of interventions version 6.1.0. UK: The Cochrane Collaboration (2020).
25. Deeks JJ, Higgins JP, Altman DG. Chapter 9: Analyzing data and undertaking meta-analyses. In: Higgins JP, Green S, editors. Cochrane handbook for systematic reviews of interventions. UK: The Cochrane Collaboration (2020).
27. Ghavipour M, Sotoudeh G, Tavakoli E, Mowla K, Hasanzadeh J, Mazloom Z. Pomegranate extract alleviates disease activity and some blood biomarkers of inflammation and oxidative stress in rheumatoid arthritis patients. Eur J Clin Nutr (2017) 71(1):92–6. doi: 10.1038/ejcn.2016.151
28. Javadi F, Ahmadzadeh A, Eghtesadi S, Aryaeian N, Zabihiyeganeh M, Rahimi Foroushani A, et al. The effect of quercetin on inflammatory factors and clinical symptoms in women with rheumatoid arthritis: A double-blind, randomized controlled trial. J Am Coll Nutr (2017) 36(1):9–15. doi: 10.1080/07315724.2016.1140093
29. Javadi F, Eghtesadi S, Ahmadzadeh A, Aryaeian N, Zabihiyeganeh M, Foroushani AR, et al. The effect of quercetin on plasma oxidative status, c-reactive protein and blood pressure in women with rheumatoid arthritis. Int J Prev Med (2014) 5(3):293–301.
30. Bae SC, Jung WJ, Lee EJ, Yu R, Sung MK. Effects of antioxidant supplements intervention on the level of plasma inflammatory molecules and disease severity of rheumatoid arthritis patients. J Am Coll Nutr (2009) 28(1):56–62. doi: 10.1080/07315724.2009.10719762
31. Khojah HM, Ahmed S, Abdel-Rahman MS, Elhakeim EH. Resveratrol as an effective adjuvant therapy in the management of rheumatoid arthritis: a clinical study. Clin Rheumatol (2018) 37(8):2035–42. doi: 10.1007/s10067-018-4080-8
32. Moosavian SP, Paknahad Z, Habibagahi Z. A randomized, double-blind, placebo-controlled clinical trial, evaluating the garlic supplement effects on some serum biomarkers of oxidative stress, and quality of life in women with rheumatoid arthritis. Int J Clin Pract (2020) 74(7):e13498. doi: 10.1111/ijcp.13498
33. Moosavian SP, Paknahad Z, Habibagahi Z, Maracy M. The effects of garlic (Allium sativum) supplementation on inflammatory biomarkers, fatigue, and clinical symptoms in patients with active rheumatoid arthritis: A randomized, double-blind, placebo-controlled trial. Phytother Res (2020) 34(11):2953–62. doi: 10.1002/ptr.6723
34. Yan Z, Xianming W, Lan X, Yapei X, Dongfeng C. Clinical observation of total glucosides of paeonia lactiflora combined with hydroxychloroquine sulfate tablets in the treatment of T2DM complicated with rheumatoid arthritis. Med Theory Pract (2021) 34(23):4101 –4103. doi: 10.19381/j.issn.1001-7585.2021.23.024
35. Ding B, Li Y, Lu S. Observation of curative effect of total glucosides of paeony and tripterygiumwilfordii in the treatment of rheumatoid arthritis and its effects on inflammatory factors and quality of life. China Sci Technol Tradit Chin Med (2021) 28(05):758–760.
36. Wu T, Chen F, Tang Z, Liu L. Effects of total glucosides of paeony on inflammatory indexes in patients with rheumatoid arthritis. Hebei Med (2021) 27(04):669–72.
37. Ju Y, Li R, Guo D, Feng X. Study on the effect of total glucosides of paeony on liver function protection and treatment of rheumatoid arthritis. Modern Chin Doctor (2019) 57(30):34–7.
38. Zheng H, Wang W, Wang Z, Tao X, Yu J, Zhang W, et al. Efficacy evaluation of total glucosides of paeony combined with methotrexate and hydroxychloroquine in maintenance treatment of rheumatoid arthritis. Zhejiang J Integrated Tradit Chin Western Med (2018) 28(11):944–6.
39. Yu H, Peng J, Ye X. Efficacy and safety of leflunomide and total glucosides of paeony combined with methotrexate in the treatment of rheumatoid arthritis. China Med Innovation (2018) 15(27):8–12.
40. Yu W, Xu J, Liang Y, Li M, Sha H. Clinical observation of total glucosides of paeonia lactiflora combined with methotrexate and leflunomide in the treatment of rheumatoid arthritis. Sichuan Med (2018) 39(01):83–5. doi: 10.16252/j.cnki.issn1004-0501-2018.01.023
41. Chen Z, Liu Q, Liu J, Jiang X, Xue Y. Observation on the effect of total glucosides of paeonia lactiflora combined with methotrexate in the treatment of refractory rheumatoid arthritis. Chin J Pract Diagnosis Treat (2017) 31(07):700 –702. doi: 10.13507/j.issn.1674-3474.2017.07.022
42. Han L, Gujanet Khan B, Bayan A, Shi R, Bahar Guli L, Wei R. Leflunomide combined with total glucosides of paeonia lactiflora in the treatment of elderly efficacy of rheumatoid arthritis and its influence on ESR, CRP and RF. Chin J Hosp Pharm (2016) 36(07):568–71. doi: 10.13286/j.cnki.chinhosppharmacyj.2016.07.12
43. Wu T, He R, Liu J, Li Y. Efficacy observation of leflunomide tablets combined with total glucosides of paeonia lactiflora in the treatment of rheumatoid arthritis. Clin Med Pract (2015) 24(05):342–5. doi: 10.16047/j.cnki.cn14-1300/r.2015.05.008
44. Zheng H, Xiao Y. Clinical observation of total glucosides of paeony and methotrexate in the treatment of senile rheumatoid arthritis. China J Basic Med Tradit Chin Med (2013) 19(12):1496–8.
45. Zheng H, Jun H, Yang Y. Effects of total glucosides of paeony on blood lipids in patients with rheumatoid arthritis. Chin J Basic Med Tradit Chin Med (2014) 20(06):832–4.
46. Li X, Chen M, Wang H. 60 cases of rheumatoid arthritis treated with total glycosides of paeony and tripterygiumwilfordii. J Anhui Univ Tradit Chin Med (2011) 30(03):16–8.
47. Yu J, Zhang H. A clinical study on total glucosides of paeony and leflunomide in the treatment of active rheumatoid arthritis. Chin Med Forum (2010) 25(04):9–10.
48. Shang G, Liu J. Efficacy observation of combined use of methotrexate and total glucosides of paeony in the treatment of rheumatoid arthritis. J Clin Rational Drugs (2009) 2(04):1–2.
49. Wang X, Liu W. Effects of total glucosides of paeony and vitamin D_3 on serum IFN-γ and IL-10 in patients with rheumatoid arthritis. China J Tradit Chin Med (2008) 07):15–7.
50. Fan Y, Li G. Study on total glucosides of paeony combined with lower dose of methotrexate in the treatment of senile-onset rheumatoid arthritis. J Modern Integr Med (2006) 11):1440–2.
51. Shi L, Yang D. Clinical observation of total glucosides of paeonia lactiflora combined with methotrexate in the treatment of rheumatoid arthritis. Hubei J Tradit Chin Med (2006) 03):35–6.
52. Zhang L, Zhou Y, Liu W. Clinical observation of total glucosides of paeonia lactiflora combined with tripterygium wilfordiipolyglycosides in the treatment of rheumatoid arthritis with enhanced efficacy and reduced toxicity. Tianjin Tradit Chin Med (2005) 22(03):207–8.
53. Zhao Y, Liu Y. Clinical observation of leflunomide and total glucosides of paeony in the treatment of rheumatoid arthritis. China J Integr Med (2006) 4):355–7.
54. Du J, Dong B. Comparison of the clinical efficacy of methotrexate alone and combined with total glucosides of paeony in the treatment of rheumatoid arthritis. China J Integr Med (2005) 25(6):540–2.
55. Chen Z, Li XP, Li ZJ, Xu L, Li XM. Reduced hepatotoxicity by total glucosides of paeony in combination treatment with leflunomide and methotrexate for patients with active rheumatoid arthritis. Int Immunopharmacol (2013) 15(3):474–7. doi: 10.1016/j.intimp.2013.01.021
56. Xiang N, Li XM, Zhang MJ, Zhao DB, Zhu P, Zuo XX, et al. Total glucosides of paeony can reduce the hepatotoxicity caused by methotrexate and leflunomide combination treatment of active rheumatoid arthritis. Int Immunopharmacol (2015) 28(1):802–7. doi: 10.1016/j.intimp.2015.08.008
57. Mirtaheri E, Khabbazi A, Nazemiyeh H, Ebrahimi AA, Hajalilou M, Shakibay Novin Z, et al. Stachys schtschegleevii tea, matrix metalloproteinase, and disease severity in female rheumatoid arthritis patients: a randomized controlled clinical trial. Clin Rheumatol (2022) 41(4):1033–44. doi: 10.1007/s10067-021-05981-4
58. Alghadir AH, Gabr SA, Al-Eisa ES. Green tea and exercise interventions as nondrug remedies in geriatric patients with rheumatoid arthritis. J Phys Ther Sci (2016) 28(10):2820–9. doi: 10.1589/jpts.28.2820
59. Yang M, Luo Y, Liu T, Zhong X, Yan J, Huang Q, et al. The effect of puerarin on carotid intima-media thickness in patients with active rheumatoid arthritis: ARandomized controlled trial. Clin Ther (2018) 40(10):1752–1764.e1. doi: 10.1016/j.clinthera.2018.08.014
60. Kometani T, Fukuda T, Kakuma T, Kawaguchi K, Tamura W, Kumazawa Y, et al. Effects of alpha-glucosylhesperidin, a bioactive food material, on collagen-induced arthritis in mice and rheumatoid arthritis in humans. Immunopharmacol Immunotoxicol (2008) 30(1):117–34. doi: 10.1080/08923970701812688
61. Sahebari M, Heidari H, Nabavi S, Khodashahi M, Rezaieyazdi Z, Dadgarmoghaddam M, et al. A double-blind placebo-controlled randomized trial of oral saffron in the treatment of rheumatoid arthritis. Avicenna J Phytomed (2021) 11(4):332–42. doi: 10.22038/AJP.2020.17280
62. Hamidi Z, Aryaeian N, Abolghasemi J, Shirani F, Hadidi M, Fallah S, et al. The effect of saffron supplement on clinical outcomes and metabolic profiles in patients with active rheumatoid arthritis: A randomized, double-blind, placebo-controlled clinical trial. Phytother Res (2020) 34(7):1650–8. doi: 10.1002/ptr.6633
63. Aryaeian N, Shahram F, Mahmoudi M, Tavakoli H, Yousefi B, Arablou T, et al. The effect of ginger supplementation on some immunity and inflammation intermediate genes expression in patients with active rheumatoid arthritis. Gene (2019) 698:179–85. doi: 10.1016/j.gene.2019.01.048
64. Shishehbor F, Rezaeyan Safar M, Rajaei E, Haghighizadeh MH. Cinnamon consumption improves clinical symptoms and inflammatory markers in women with rheumatoid arthritis. J Am Coll Nutr (2018), 1–6. doi: 10.1080/07315724.2018.1460733
65. Helli B, Shahi MM, Mowla K, Jalali MT, Haghighian HK. A randomized, triple-blind, placebo-controlled clinical trial, evaluating the sesamin supplement effects on proteolytic enzymes, inflammatory markers, and clinical indices in women with rheumatoid arthritis. Phytother Res (2019) 33(9):2421–8. doi: 10.1002/ptr.6433
66. Helli B, Mowla K, Mohammadshahi M, Jalali MT. Effect of sesamin supplementation on cardiovascular risk factors in women with rheumatoid arthritis. J Am Coll Nutr (2016) 35(4):300–7. doi: 10.1080/07315724.2015.1005198
67. Thimóteo NSB, Iryioda TMV, Alfieri DF, Rego BEF, Scavuzzi BM, Fatel E, et al. Cranberry juice decreases disease activity in women with rheumatoid arthritis. Nutrition (2019) 60:112–7. doi: 10.1016/j.nut.2018.10.010
68. Fatel ECS, Rosa FT, Alfieri DF, Flauzino T, Scavuzzi BM, Lozovoy MAB, et al. Beneficial effects of fish oil and cranberry juice on disease activity and inflammatory biomarkers in people with rheumatoid arthritis. Nutrition (2021) 86:111183. doi: 10.1016/j.nut.2021.111183
69. Hekmatpou D, Mortaji S, Rezaei M, Shaikhi M. The effectiveness of olive oil in controlling morning inflammatory pain of phalanges and knees among women with rheumatoid arthritis: A randomized clinical trial. Rehabil Nurs (2020) 45(2):106–13. doi: 10.1097/rnj.0000000000000162
70. Berbert AA, Kondo CR, Almendra CL, Matsuo T, Dichi I. Supplementation of fish oil and olive oil in patients with rheumatoid arthritis. Nutrition (2005) 21(2):131–6. doi: 10.1016/j.nut.2004.03.023
71. Bitler CM, Matt K, Irving M, Hook G, Crea R. Olive extract supplement decreases pain and improves daily activities in adults with osteoarthritis and decreases plasma homocysteine in those with rheumatoid arthritis. Nutr Res (2007) 27(8):470–7. doi: 10.1016/j.nutres.2007.06.003
72. Amalraj A, Varma K, Jacob J, Divya C, Kunnumakkara AB, Stohs SJ, et al. A novel highly bioavailable curcumin formulation improves symptoms and diagnostic indicators in rheumatoid arthritis patients: A randomized, double-blind, placebo-controlled, two-dose, three-arm, and parallel-group study. J Med Food (2017) 20(10):1022–30. doi: 10.1089/jmf.2017.3930
73. Pourhabibi-Zarandi F, Rafraf M, Zayeni H, Asghari-Jafarabadi M, Ebrahimi AA. Effects of curcumin supplementation on metabolic parameters, inflammatory factors and obesity values in women with rheumatoid arthritis: A randomized, double-blind, placebo-controlled clinical trial. Phytother Res (2022) 36(4):1797–806. doi: 10.1002/ptr.7422
74. Javadi M, Khadem Haghighian H, Goodarzy S, Abbasi M, Nassiri-Asl M. Effect of curcumin nanomicelle on the clinical symptoms of patients with rheumatoid arthritis: A randomized, double-blind, controlled trial. Int J Rheum Dis (2019) 22(10):1857–62. doi: 10.1111/1756-185X.13688
75. Jacob J, Amalraj A, Raj KKJ, Divya C, Kunnumakkara AB, Gopi S. A novel bioavailable hydrogenated curcuminoids formulation (CuroWhite™) improves symptoms and diagnostic indicators in rheumatoid arthritis patients - a randomized, double blind and placebo controlled study. J Tradit Complement Med (2018) 9(4):346–52. doi: 10.1016/j.jtcme.2018.06.001
76. Chandran B, Goel A. A randomized, pilot study to assess the efficacy and safety of curcumin in patients with active rheumatoid arthritis. Phytother Res (2012) 26(11):1719–25. doi: 10.1002/ptr.4639
77. Matsuno H, Nakamura H, Katayama K, Hayashi S, Kano S, Yudoh K, et al. Effects of an oral administration of glucosamine-chondroitin-quercetin glucoside on the synovial fluid properties in patients with osteoarthritis and rheumatoid arthritis. Biosci Biotechnol Biochem (2009) 73(2):288–92. doi: 10.1271/bbb.80418
78. Balbir-Gurman A, Fuhrman B, Braun-Moscovici Y, Markovits D, Aviram M. Consumption of pomegranate decreases serum oxidative stress and reduces disease activity in patients with active rheumatoid arthritis: a pilot study. Isr Med Assoc J (2011) 13(8):474–9.
79. 1Vučić V, Grabež M, Trchounian A, Arsić A. Composition and potential health benefits of pomegranate: A review. Curr Pharm Des (2019) 25(16):1817–27. doi: 10.2174/1381612825666190708183941
80. Danesi F, Ferguson LR. Could pomegranate juice help in the control of inflammatory diseases? Nutrients (2017) 9(9):958. doi: 10.3390/nu9090958
81. Paller CJ, Pantuck A, Carducci MA. A review of pomegranate in prostate cancer. Prostate Cancer Prostatic Dis (2017) 20(3):265–70. doi: 10.1038/pcan.2017.19
82. Wang D, Özen C, Abu-Reidah IM, Chigurupati S, Patra JK, Horbanczuk JO, et al. Vasculoprotective effects of pomegranate (Punica granatum l.). Front Pharmacol (2018) 9:544. doi: 10.3389/fphar.2018.00544
83. Malek Mahdavi A, Javadivala Z. Systematic review of the effects of pomegranate (Punica granatum) on osteoarthritis. Health Promot Perspect (2021) 11(4):411–25. doi: 10.34172/hpp.2021.51
84. Cerda B, Ceron JJ, Tomas-Barberan FA, Espin JC. Repeated oral administration of high doses of the pomegranate ellagitannin punicalagin to rats for 37 days is not toxic. J Agric Food Chem (2003) 51(11):3493–501. doi: 10.1021/jf020842c
85. Heber D, Seeram NP, Wyatt H, Henning SM, Zhang Y, Ogden LG, et al. Safety and antioxidant activity of a pomegranate ellagitannin enriched polyphenol dietary supplement in overweight individuals with increased waist size. J Agric Food Chem (2007) 55(24):10050–4. doi: 10.1021/jf071689v
86. Pirzadeh M, Caporaso N, Rauf A, Shariati MA, Yessimbekov Z, Khan MU, et al. Pomegranate as a source of bioactive constituents: a review on their characterization, properties and applications. Crit Rev Food Sci Nutr (2021) 61(6):982–99. doi: 10.1080/10408398.2020.1749825
87. Wang Y, He T, Li Z, Gai S. Effect of ethanol extract of punica granatum l against freund’s complete adjuvant-induced arthritis in rats. Trop J Pharm Res (2019) 18(3):591–5.
88. Karwasra R, Singh S, Sharma D, Sharma S, Sharma N, Khanna K. Pomegranate supplementation attenuates inflammation, joint dysfunction via inhibition of NF-κB signaling pathway in experimental models of rheumatoid arthritis. J Food Biochem (2019) 43(8):1–12. doi: 10.1111/jfbc.12959
89. Albogami S, Alhazmi A. Potential effects of taif's punica granatum l. extract on peripheral blood mononuclear cells from patients with rheumatoid arthritis via regulation of the NF-κB signaling pathway by the IκBα gene. Biotechnology (2018) 17(3):113–9. doi: 10.3923/biotech.2018.113.119
90. Seong AR, Yoo JY, Choi KC, Lee MH, Lee YH, Lee J, et al. Delphinidin, a specific inhibitor of histone acetyltransferase, suppresses inflammatory signaling via prevention of NF-κB acetylation in fibroblast-like synoviocyte MH7A cells. Biochem Biophys Res Commun (2011) 410(3):581–6. doi: 10.1016/j.bbrc.2011.06.029
91. Rasheed Z, Akhtar N, Anbazhagan AN, Ramamurthy S, Shukla M, Haqqi TM. Polyphenol-rich pomegranate fruit extract (POMx) suppresses PMACI-induced expression of pro-inflammatory cytokines by inhibiting the activation of MAP kinases and NF-κB in human KU812 cells. J Inflammation (Lond) (2009) 6:1–12. doi: 10.1186/1476-9255-6-1
92. Brand-Williams W, Cuvelier M, Berset C. Use of a free radical method to evaluate antioxidant activity. Lebensm-Wiss Technol (1995) 28(1):25–30. doi: 10.1016/S0023-6438(95)80008-5
93. Nair RK, Haridas A, Ezhuthupurakkal DR. Diversity and comparative account on phytochemical and antioxidant properties of two varieties of musa, nendran and kunnan. South Ind J Biol Sci (2016) 2(1):203–6. doi: 10.22205/sijbs/2016/v2/i1/100396
94. Morvaridzadeh M, Sepidarkish M, Daneshzad E, Akbari A, Mobini GR, Heshmati J. The effect of pomegranate on oxidative stress parameters: a systematic review and meta-analysis. Complement Ther Med (2020) 48:102252. doi: 10.1016/j.ctim.2019.102252
95. Andres S, Pevny S, Ziegenhagen R, Bakhiya N, Schäfer B, Hirsch-Ernst KI, et al. Safety aspects of the use of quercetin as a dietary supplement. Mol Nutr Food Res (2018) 62(1):1700447. doi: 10.1002/mnfr.201700447
96. Marunaka Y, Marunaka R, Sun H, Yamamoto T, Kanamura N, Inui T, et al. Actions of quercetin, a polyphenol, on blood pressure. Molecules (2017) 22(2):209. doi: 10.3390/molecules22020209
97. Li Y, Yao J, Han C, Yang J, Chaudhry MT, Wang S, et al. Quercetin, inflammation and immunity. Nutrients (2016) 8(3):167. doi: 10.3390/nu8030167
98. Lupo G, Cambria MT, Olivieri M, Rocco C, Caporarello N, Longo A, et al. Anti-angiogenic effect of quercetin and its 8-methyl pentamethyl ether derivative in human microvascular endothelial cells. J Cell Mol Med (2019) 23(10):6565–77. doi: 10.1111/jcmm.14455
99. Fernandez-Palanca P, Fondevila F, Mendez-Blanco C, Tunon MJ, Gonzalez-Gallego J, Mauriz JL. Antitumor effects of quercetin in hepatocarcinoma in vitro and in vivo models: a systematic review. Nutrients (2019) 11:12. doi: 10.3390/nu11122875
100. Kim SH, Yoo ES, Woo JS, an SH, Lee JH, Jung SH, et al. Antitumor and apoptotic effects of quercetin on human melanoma cells involving JNK/P38 MAPK signaling activation. Eur J Pharmacol (2019) 860:172568. doi: 10.1016/j.ejphar.2019.172568
101. Tavana E, Mollazadeh H, Mohtashami E, Modaresi SMS, Hosseini A, Sabri H, et al. Quercetin: a promising phytochemical for the treatment of glioblastoma multiforme. Biofactors (2019) 46:356–66. doi: 10.1002/biof.1605
102. Afifi NA, Ibrahim MA, Galal MK. Hepatoprotective influence of quercetin and ellagic acid on thioacetamide-induced hepatotoxicity in rats. Can J Physiol Pharmacol (2018) 96(6):624–9. doi: 10.1139/cjpp-2017-0651
103. Shen P, Lin W, Deng X, Ba X, Han L, Chen Z, et al. Potential implications of quercetin in autoimmune diseases. Front Immunol (2021) 12:689044. doi: 10.3389/fimmu.2021.689044
104. Goyal A, Agrawal N. Quercetin: A potential candidate for the treatment of arthritis. Curr Mol Med (2022) 22(4):325–35. doi: 10.2174/1566524021666210315125330
105. Guardia T, Rotelli AE, Juarez AO, Pelzer LE. Anti-inflammatory properties of plant flavonoids. Effects rutin quercetin hesperidin adjuvant Arthritis rat. Farmaco (2001) 56(9):683–7. doi: 10.1016/S0014-827X(01)01111-9
106. Rotelli AE, Guardia T, Juarez AO, de la Rocha NE, Pelzer LE. Comparative study of flavonoids in experimental models of inflammation. Pharmacol Res (2003) 48(6):601–6. doi: 10.1016/S1043-6618(03)00225-1
107. Guazelli CFS, Staurengo-Ferrari L, Zarpelon AC, Pinho-Ribeiro FA, Ruiz-Miyazawa KW, Vicentini FTMC, et al. Quercetin attenuates zymosan-induced arthritis in mice. BioMed Pharmacother (2018) 102:175–84. doi: 10.1016/j.biopha.2018.03.057
108. Yang Y, Zhang X, Xu M, Wu X, Zhao F, Zhao C. Quercetin attenuates collagen-induced arthritis by restoration of Th17/Treg balance and activation of heme oxygenase 1-mediated anti-inflammatory effect. Int Immunopharmacol (2018) 54:153–62. doi: 10.1016/j.intimp.2017.11.013
109. Kawaguchi K, Kaneko M, Miyake R, Takimoto H, Kumazawa Y. Potent inhibitory effects of quercetin on inflammatory responses of collagen-induced arthritis in mice. Endocr Metab Immune Disord Drug Targets (2019) 19(3):308–15. doi: 10.2174/1871530319666190206225034
110. Shen P, Lin W, Ba X, Huang Y, Chen Z, Han L, et al. Quercetin-mediated SIRT1 activation attenuates collagen-induced mice arthritis. J Ethnopharmacol (2021) 279:114213. doi: 10.1016/j.jep.2021.114213
111. Yang Y, Shi GN, Wu X, Xu M, Chen CJ, Zhou Y, et al. Quercetin impedes Th17 cell differentiation to mitigate arthritis involving ppargamma-driven transactivation of SOCS3 and redistribution corepressor SMRT from PPARgamma to STAT3. Mol Nutr Food Res (2022):e2100826. doi: 10.1002/mnfr.202100826
112. Haleagrahara N, Miranda-Hernandez S, Alim MA, Hayes L, Bird G, Ketheesan N. Therapeutic effect of quercetin in collagen-induced arthritis. BioMed Pharmacother (2017) 90:38–46. doi: 10.1016/j.biopha.2017.03.026
113. Mamani-Matsuda M, Kauss T, Al-Kharrat A, Fawaz F, Thiolat D, Moynet D, et al. Therapeutic and preventive properties of quercetin in experimental arthritis correlate with decreased macrophage inflammatory mediators. Biochem Pharmacol (2006) 72(10):1304–10. doi: 10.1016/j.bcp.2006.08.001
114. El-Said KS, Atta A, Mobasher MA, Germoush MO, Mohamed TM, Salem MM. Quercetin mitigates rheumatoid arthritis by inhibiting adenosine deaminase in rats. Mol Med (2022) 28(1):24. doi: 10.1186/s10020-022-00432-5
115. Saccol R, da Silveira KL, Adefegha SA, Manzoni AG, da Silveira LL, Coelho APV, et al. Effect of quercetin on e-NTPDase/E-ADA activities and cytokine secretion of complete freund adjuvant-induced arthritic rats. Cell Biochem Funct (2019) 37(7):474–85. doi: 10.1002/cbf.3413
116. Yuan K, Zhu Q, Lu Q, Jiang H, Zhu M, Li X, et al. Quercetin alleviates rheumatoid arthritis by inhibiting neutrophil inflammatory activities. J Nutr Biochem (2020) 84:108454. doi: 10.1016/j.jnutbio.2020.108454
117. Costa ACF, de Sousa LM, Dos Santos Alves JM, Goes P, Pereira KMA, Alves APNN, et al. Anti-inflammatory and hepatoprotective effects of quercetin in an experimental model of rheumatoid arthritis. Inflammation (2021) 44:2033–43. doi: 10.1007/s10753-021-01479-y
118. Ibrahim SSA, Kandil LS, Ragab GM, El-Sayyad SM. 26b, 20a inversely correlate with GSK-3 beta/NF-kappaB/NLRP-3 pathway to highlight the additive promising effects of atorvastatin and quercetin in experimental induced arthritis. Int Immunopharmacol (2021) 99:108042. doi: 10.1016/j.intimp.2021.108042
119. Gardi C, Bauerova K, Stringa B, Kuncirova V, Slovak L, Ponist S, et al. Quercetin reduced inflammation and increased antioxidant defense in rat adjuvant arthritis. Arch Biochem Biophys (2015) 583:150–7. doi: 10.1016/j.abb.2015.08.008
120. Chedea VS, Vicaş SI, Sticozzi C, Pessina F, Frosini M, Maioli E, et al. Resveratrol: from diet to topical usage. Food Funct (2017) 8(11):3879–3892. doi: 10.1039/C7FO01086A
121. Kim HJ, Chang EJ, Cho SH, Chung SK, Park HD, Choi SW, et al. Antioxidative activity of resveratrol and its derivatives isolated from seeds of paeonia lactiflora. Biosci Biotechnol Biochem (2002) 66(9):1990–3. doi: 10.1271/bbb.66.1990
122. Wei Y, Jia J, Jin X, Tong W, Tian H. Resveratrol ameliorates inflammatory damage and protects against osteoarthritis in a rat model of osteoarthritis. Mol Med Rep (2018) 17(1):1493–1498. doi: 10.3892/mmr.2017.8036
123. Gülçin İ. Antioxidant properties of resveratrol: a structure–activity insight. Innov Food Sci Emerg Technol (2010) 11(1):210–218. doi: 10.1016/j.ifset.2009.07.002
124. Zhang Y, Zhang S, Liu Z, Zhao X, Yuan Y, Sheng L, et al. Resveratrol prevents atrial fibrillation by inhibiting atrial structural and metabolic remodeling in collagen-induced arthritis rats. Naunyn Schmiedebergs Arch Pharmacol (2018) 391(11):1179–1190. doi: 10.1007/s00210-018-1554-9
125. Zhang J, Song X, Cao W, Lu J, Wang X, Wang G, et al. Autophagy and mitochondrial dysfunction in adjuvant-arthritis rats treatment with resveratrol. Sci Rep (2016) 6:32928. doi: 10.1038/srep32928
126. Zhang N, Weber A, Li B, Lyons R, Contag PR, Purchio AF, et al. An inducible nitric oxide synthase-luciferase reporter system for in vivo testing of anti-inflammatory compounds in transgenic mice. J Immunol (2003) 170(12):6307–19. doi: 10.4049/jimmunol.170.12.6307
127. Pshenichnyuk SA, Komolov AS. Dissociative electron attachment to resveratrol as a likely pathway for generation of the H2 antioxidant species inside mitochondria. J Phys Chem Lett (2015) 6(7):1104–1110. doi: 10.1021/acs.jpclett.5b00368
128. Elmali N, Baysal O, Harma A, Esenkaya I, Mizrak B. Effects of resveratrol in inflammatory arthritis. Inflammation (2007) 30(1):1–6. doi: 10.1007/s10753-006-9012-0
129. Nakayama H, Yaguchi T, Yoshiya S, Nishizaki T. Resveratrol induces apoptosis MH7A human rheumatoid arthritis synovial cells in a sirtuin 1-dependent manner. Rheumatol Int (2012) 32(1):151–157. doi: 10.1007/s00296-010-1598-8
130. Kundu JK, Shin YK, Kim SH, Surh Y-J. Resveratrol inhibits phorbol ester-induced expression of COX-2 and activation of NF-κB in mouse skin by blocking IκB kinase activity. Carcinogenesis (2006) 27(7):1465–1474. doi: 10.1093/carcin/bgi349
131. Yar A, Menevse S, Alp E. The effects of resveratrol on cyclooxygenase-1 and-2, nuclear factor kappa beta, matrix metalloproteinase-9, and sirtuin 1 mRNA expression in hearts of streptozotocin-induced diabetic rats. Genet Mol Res (2011) 10(4):2962–75. doi: 10.4238/2011.November.29.7
132. Chen X-Y, Wang Z-C, Li J, Liu X-L, Sun Y-H. Regulation of synoviocyte activity by resveratrol in rats with adjuvant arthritis. Exp Ther Med (2013) 6(1):172–176. doi: 10.3892/etm.2013.1078
133. Cheon Y-H, Kim H-O, Suh Y-S, Hur JH, Lee S. Inhibitory effects for rheumatoid arthritis of dietary supplementation with resveratrol in collagen-induced arthritis. J Rheum Dis (2015) 22(2):93–101. doi: 10.4078/jrd.2015.22.2.93
134. Amagase H. Clarifying the real bioactive constituents of garlic. J Nutr (2006) 136(3 Suppl):716S–25S. doi: 10.1093/jn/136.3.716S
135. Beato VM, Orgaz F, Mansilla F, Montaño A. Changes in phenolic compounds in garlic (Allium sativum l.) owing to the cultivar and location of growth. Plant Foods Hum Nutr (2011) 66(3):218–23. doi: 10.1007/s11130-011-0236-2
136. Moosavian SP, Arab A, Paknahad Z, Moradi S. The effects of garlic supplementation on oxidative stress markers: A systematic review and meta-analysis of randomized controlled trials. Complement Ther Med (2020) 50:102385. doi: 10.1016/j.ctim.2020.102385
137. Nagella P, Thiruvengadam M, Ahmad A, Yoon J-Y, Chung I-M. Composition of polyphenols and antioxidant activity of garlic bulbs collected from different locations of Korea. Asian J Chem (2014) 26(3):897–902. doi: 10.14233/ajchem.2014.16143A
138. Arreola R, Quintero-Fabián S, López-Roa RI, Flores-Gutiérrez EO, Reyes-Grajeda JP, Carrera-Quintanar L, et al. Immunomodulation and anti-inflammatory effects of garlic compounds. J Immunol Res (2015) 2015:401630. doi: 10.1155/2015/401630
139. Fu E, Tsai MC, Chin YT, Tu HP, Fu MM, Chiang CY, et al. The effects of diallyl sulfide upon porphyromonasgingivalis lipopolysaccharide stimulated proinflammatory cytokine expressions and nuclear factor-kappa b activation in human gingival fibroblasts. J Periodontal Res (2015) 50(3):380–8. doi: 10.1111/jre.12217
140. Kim SR, Jung YR, An HJ, Kim DH, Jang EJ, Choi YJ, et al. Anti-wrinkle and anti-inflammatory effects of active garlic components and the inhibition of MMPs via NF-κB signaling. PloS One (2013) 8(9):e73877. doi: 10.1371/journal.pone.0073877
141. Ho CY, Weng CJ, Jhang JJ, Cheng YT, Huang SM, Yen GC. Diallyl sulfide as a potential dietary agent to reduce TNF-α- and histamine-induced proinflammatory responses in A7r5 cells. Mol Nutr Food Res (2014) 58(5):1069–78. doi: 10.1002/mnfr.201300617
142. Williamson EM, Liu X, Izzo AA. Trends in use, pharmacology, and clinical applications of emerging herbal nutraceuticals. Br J Pharmacol (2020) 177(6):1227–40. doi: 10.1111/bph.14943
143. Mahdizadeh S, Khaleghi Ghadiri M, Gorji A. Avicenna's canon of medicine: a review of analgesics and anti-inflammatory substances. Avicenna J Phytomed (2015) 5(3):182–202.
144. Morihara N, Nishihama T, Ushijima M, Ide N, Takeda H, Hayama M. Garlic as an anti-fatigue agent. Mol Nutr Food Res (2007) 51(11):1329–34. doi: 10.1002/mnfr.200700062
145. Rana S, Pal R, Vaiphei K, Sharma SK, Ola R. Garlic in health and disease. Nutr Res Rev (2011) 24(1):60–71. doi: 10.1017/S0954422410000338
146. Hernández-Cruz EY, Silva-Islas CA, Maldonado PD, Pedraza-Chaverri J, Carballo-Villalobos AI. Antinociceptive effect of garlic, garlic preparations and derivative compounds. Eur J Pain (2022) 26(5):947–64. doi: 10.1002/ejp.1935
147. Aalami-Harandi R, Karamali M, Asemi Z. The favorable effects of garlic intake on metabolic profiles, hs-CRP, biomarkers of oxidative stress and pregnancy outcomes in pregnant women at risk for pre-eclampsia: randomized, double-blind, placebo-controlled trial. J Matern Fetal Neonatal Med (2015) 28(17):2020–7. doi: 10.3109/14767058.2014.977248
148. Hosseinzadeh-Attar MJ, Alipoor E, Dehghani S, Salimzadeh A. Increased efficacy of a garlic supplement on knee osteoarthritis symptoms in patients with obesity. J Herbal Med (2020) 24:100392. doi: 10.1016/j.hermed.2020.10
149. Hussein NA, Sharara GM. Poster 9: effect of combined garlic therapy and comprehensive rehabilitation program versus comprehensive rehabilitation program alone on control of clinical manifestations and quality of life of knee osteoarthritis patients. Arch Phys Med Rehabil (2007) 88:E12. doi: 10.1016/j.apmr.2007.06.584
150. Salimzadeh A, Alipoor E, Dehghani S, Yaseri M, Hosseini M, Feinle-Bisset C, et al. The effect of 12-week garlic supplementation on symptom relief in overweight or obese women with knee osteoarthritis. Int J Clin Pract (2018) 72:1–7. doi: 10.1111/ijcp.13208
151. Farjana F, Sakib N, Hasan MM, Das P, Hossain AI, Mohammed R. Antinociceptive activity studies with methanol extract of onion, garlic and ginger in mice. Adv Natural Appl Sci (2014) 8:169–74.
152. Nirjhor SA, Jannat H, Ahmed M, Ghosh D, Mandal I, Kawchur U, et al. Methanol extract of allium sativum l. leaves show antinociceptive activity in acetic acid-induced writhing tests in mice. Adv Natural Appl Sci (2014) 8:203–7.
153. Jiang H, Li J, Wang L, Wang S, Nie X, Chen Y, et al. Total glucosides of paeony: A review of its phytochemistry, role in autoimmune diseases, and mechanisms of action. J Ethnopharmacol (2020) 258:112913. doi: 10.1016/j.jep.2020.112913
154. Zhang L, Yu J, Wang C, Wei W. The effects of total glucosides of paeony (TGP) and paeoniflorin (Pae) on inflammatory-immune responses in rheumatoid arthritis (RA). Funct Plant Biol (2019) 46(2):107–17. doi: 10.1071/FP18080
155. Luo J, Jin DE, Yang GY, Zhang YZ, Wang JM, Kong WP, et al. Total glucosides of paeony for rheumatoid arthritis: A systematic review of randomized controlled trials. Complement Ther Med (2017) 34:46–56. doi: 10.1016/j.ctim.2017.07.010
156. Jin L, Li C, Li Y, Wu B. Clinical efficacy and safety of total glucosides of paeony for primary sjögren's syndrome: A systematic review. Evid Based Complement Alternat Med (2017) 2017:3242301. doi: 10.1155/2017/3242301
157. Huang Y, Wang H, Chen Z, Wang Y, Qin K, Huang Y, et al. Synergistic and hepatoprotective effect of total glucosides of paeony on ankylosing spondylitis: A systematic review and meta-analysis. Front Pharmacol (2019) 10:231. doi: 10.3389/fphar.2019.00231
158. Chen Y, Wang L, Cao Y, Li N. Total glucosides of paeonia lactiflora for safely reducing disease activity in systemic lupus erythematosus: A systematic review and meta-analysis. Front Pharmacol (2022) 13:834947. doi: 10.3389/fphar.2022.834947. 202.
159. Jia Z, He J. Paeoniflorin ameliorates rheumatoid arthritis in rat models through oxidative stress, inflammation and cyclooxygenase 2. Exp Ther Med (2016) 11(2):655–9. doi: 10.3892/etm.2015.2908
160. Zhang LL, Wei W, Wang NP, Wang QT, Chen JY, Chen Y, et al. Paeoniflorin suppresses inflammatory mediator production and regulates G protein-coupled signaling in fibroblast-like synoviocytes of collagen induced arthritic rats. Inflammation Res (2008) 57(8):388–95. doi: 10.1007/s00011-007-7240-x
161. Ge ZD, Wang Z.A. W, B., Shen YX, Zhang AP, Ding CH, Xu S. Immunoregulatory effects of total glucosides of paeony (TGP), paeoniflorin (PF) and TGP removed PF on adjuvant arthritic rats. Chin Pharmacol Bull (1995) 11(2):303–5.
162. Li PP, Liu DD, Liu YJ, Song SS, Wang QT, Chang Y, et al. BAFF/BAFF-r involved in antibodies production of rats with collagen-induced arthritis via PI3K-Akt-mTOR signaling and the regulation of paeoniflorin. J Ethnopharmacol (2012) 141(1):290–300. doi: 10.1016/j.jep.2012.02.034
163. Shao YX, Xu XX, Li YY, Qi XM, Wang K, Wu YG, et al. Paeoniflorin inhibits high glucose-induced macrophage activation through TLR2-dependent signal pathways. J Ethnopharmacol (2016) 193:377–86. doi: 10.1016/j.jep.2016.08.035
164. Wu HX, Chen JY, Wang QT, Sun WY, Liu LH, Zhang LL, et al. Expression and function of β-arrestin 2 stimulated by IL-1β in human fibroblast-like synoviocytes and the effect of paeoniflorin. Int Immunopharmacol (2012) 12(4):701–6. doi: 10.1016/j.intimp.2012.01.018
165. Gu P, Zhu L, Liu Y, Zhang L, Liu J, Shen H. Protective effects of paeoniflorin on TNBS-induced ulcerative colitis through inhibiting NF-kappaB pathway and apoptosis in mice. Int Immunopharmacol (2017) 50:152–60. doi: 10.1016/j.intimp.2017.06.022
166. Weinreb O, Amit T, Mandel S, Youdim MB. Neuroprotective molecular mechanisms of (-)-epigallocatechin-3-gallate: a reflective outcome of its antioxidant, iron chelating and neuritogenic properties. Genes Nutr (2009) 4(4):283–96. doi: 10.1007/s12263-009-0143-4
167. Wu D, Wang J, Pae M, Meydani SN. Green tea EGCG, T cells, and T cell-mediated autoimmune diseases. Mol Aspects Med (2012) 33(1):107–18. doi: 10.1016/j.mam.2011.10.001
168. Clement Y. Can green tea do that? a literature review of the clinical evidence. Prev Med (2009) 49(2):83–7. doi: 10.1016/j.ypmed.2009.05.005
169. Khan N, Afaq F, Saleem M, Ahmad N, Mukhtar H. Targeting multiple signaling pathways by green tea polyphenol (–)-epigallocatechin-3-gallate. Cancer Res (2006) 66(1):2500–5. doi: 10.1158/0008-5472.CAN-05-3636
170. Riegsecker S, Wiczynski D, Kaplan MJ, Ahmed S. Potential benefits of green tea polyphenol EGCG in the prevention and treatment of vascular inflammation in rheumatoid arthritis. Life Sci (2013) 93(8):307–12. doi: 10.1016/j.lfs.2013.07.006
171. Ahmed. S. Green tea polyphenol epigallocatechin 3-gallate in arthritis: progress and promise. Arthritis Res Ther (2010) 12(2):208. doi: 10.1186/ar2982
172. Ahmed S, Rahman A, Hasnain A, Lalonde M, Goldberg VM, Haqqi TM. Green tea polyphenol epigallocatechin-3-gallate inhibits the IL-1 beta-induced activity and expression of cyclooxygenase-2 and nitric oxide synthase-2 in human chondrocytes. Free Radic Biol Med (2002) 33(2002):1097–105. doi: 10.1016/S0891-5849(02)01004-3
173. Singh R, Ahmed S, Islam N, Goldberg VM, Haqqi TM. Epigallocatechin-3-gallate inhibits interleukin-1beta-induced expression of nitric oxide synthase and production of nitric oxide in human chondrocytes: suppression of nuclear factor kappaB activation by degradation of the inhibitor of nuclear factor kappaB. Arthritis Rheum (2002) 46(1):2079–86. doi: 10.1002/art.10443
174. Singh R, Ahmed S, Malemud CJ, Goldberg VM, Haqqi TM. Epigallocatechin-3-gallate selectively inhibits interleukin-1beta-induced activation of mitogen activated protein kinase subgroup c-jun n-terminal kinase in human osteoarthritis chondrocytes. JOrthop Res (2003) 21(3):102–9. doi: 10.1016/S0736-0266(02)00089-X
175. Akhtar N, Haqqi TM. Epigallocatechin-3-gallate suppresses the global interleukin-1beta-induced inflammatory response in human chondrocytes. Arthritis Res Ther (2011) 13:R93. doi: 10.1186/ar3368
176. Adcocks C, Collin P, Buttle DJ. Catechins from green tea (Camellia sinensis) inhibit bovine and human cartilage proteoglycan and type II collagen degradation in vitro. JNutr (2002) 132(2):341–6. doi: 10.1093/jn/132.3.341
177. Kamon M, Zhao R, Sakamoto K. Green tea polyphenol (–)-epigallocatechin gallate suppressed the differentiation of murine osteoblastic MC3T3-E1 cells. Cell Biol Int (2010) 34(2):109–16. doi: 10.1042/CBI20090011
178. Lee JH, Jin H, Shim HE, Kim HN, Ha H, Lee ZH. Epigallocatechin-3-gallate inhibits osteoclastogenesis by down-regulating c-fos expression and suppressing the nuclear factor-kappaBsignal. MolPharmacol (2010) 77(1):17–25. doi: 10.1124/mol.109.057877
179. Liu D, Li P, Song S, Liu Y, Wang Q, Chang Y, et al. Pro-apoptotic effect of epigallo-catechin-3-gallate on b lymphocytes through regulating BAFF/PI3K/Akt/mTOR signaling in rats with collagen-induced arthritis. Eur J Pharmacol (2012) 690(1):214–25. doi: 10.1016/j.ejphar.2012.06.026
180. Ahmed S, Silverman MD, Marotte H, Kwan K, Matuszczak N, Koch AE. Down-regulation of myeloid cell leukemia 1 by epigallocatechin-3-gallate sensitizes rheumatoid arthritis synovial fibroblasts to tumor necrosis factor alpha-induced apoptosis. Arthritis Rheum (2009) 60(2):1282–93. doi: 10.1002/art.24488
181. Ahmed S, Pakozdi A, Koch AE. Regulation of interleukin-1beta-induced chemokine production and matrix metalloproteinase 2 activation by epigallocatechin-3-gallate in rheumatoid arthritis synovial fibroblasts. Arthritis Rheum (2006) 54(1):2393–401. doi: 10.1002/art.22023
182. Yun HJ, Yoo WH, Han MK, Lee YR, Kim JS, Lee SI. Epigallocatechin-3-gallate suppresses TNF-alpha -induced production of MMP-1 and -3 in rheumatoid arthritis synovial fibroblasts. Rheumatol Int (2008) 29(2):23–9. doi: 10.1007/s00296-008-0597-5
183. Zhou Y, Zhang H, Peng C. Puerarin: a review of pharmacological effects. Phytother Res (2014) 28:961–75. doi: 10.1002/ptr.5083
184. Kakehashi A, Yoshida M, Tago Y, Ishii N, Okuno T, Gi M, et al. Pueraria mirifica exerts estrogenic effects in the mammary gland and uterus and promotes mammary carcinogenesis in donryu rats. Toxins (2016) 8:275. doi: 10.3390/toxins8110275
185. Wei S, Chen Y, Xu X. Progress on the pharmacological research of puerarin: a review. Chin J Natural Medicines (2014) 12:407–14. doi: 10.1016/S1875-5364(14)60064-9
186. Xiao C, Li J, Dong X, He X, Niu X, Liu C, et al. Anti-oxidative and TNF-αα suppressive activities of puerarin derivative (4AC) in RAW264.7 cells and collagen-induced arthritic rats. Eur J Pharmacol (2011) 666:242–50. doi: 10.1016/j.ejphar.2011.05.061
187. Jeon Y, Lee J, Lee Y, Kim D. Puerarin inhibits inflammation and oxidative stress in dextran sulfate sodium-induced colitis mice model. Biomed Pharmacother (2020) 124:109847. doi: 10.1016/j.biopha.2020.109847
188. Zhu G, Wang X, Wu S, Li X, Li Q. Neuroprotective effects of puerarin on 1-methyl-4-phenyl-1,2,3,6-tetrahydropyridine induced parkinson’s disease model in mice. Phytother Res (2014) 28:179–86. doi: 10.1002/ptr.4975
189. Zhao M, Du Y, Yuan L, Wang N. Protective effect of puerarin on acute alcoholic liver injury. Am J Chin Med (2010) 38:241–9. doi: 10.1142/S0192415X10007816
190. Ahmad B, Khan S, Liu Y, Xue M, Nabi G. Molecular mechanisms of anticancer activities of puerarin. Cancer Manage Res (2020) 12:79–90. doi: 10.2147/CMAR.S233567
191. Chen X, Yu J, Shi J. Management of diabetes mellitus with puerarin, a natural isoflavone from pueraria lobata. Am J Chin Med (2018) 46:1771–89. doi: 10.1142/S0192415X18500891
192. Li X, Yuan T, Chen D, Chen Y, Sun S, Wang D, et al. Cardioprotective effects of puerarin-V on isoproterenol-induced myocardial infarction mice is associated with regulation of PPAR-Υ/NF-κB pathway. Molecules (2018) 23:3322. doi: 10.3390/molecules23123322
193. Bao L, Zhang Y, Wei G, Wang Y, Ma R, Cheng R, et al. The anti-atherosclerotic effects of puerarin on induced-atherosclerosis in rabbits. Biomed Papers Med Faculty Univ Palacky Olomouc Czechoslovakia (2015) 159:53–9. doi: 10.5507/bp.2013.096
194. Wu J, Zhang X, Zhang B. Efficacy and safety of puerarin injection in treatment of diabetic peripheral neuropathy: A systematic review and meta-analysis of randomized controlled trials. J Tradit Chin Med (2014) 34:401–10. doi: 10.1016/S0254-6272(15)30039-X
195. Yang Y, Chin A, Zhang L, Lu J, Wong RW. The role of traditional Chinese medicines in osteogenesis and angiogenesis. Phytother Res (2014) 28:1–8. doi: 10.1002/ptr.4959
196. Wang C, Wang W, Jin X, Shen J, Hu W, Jiang T. Puerarin attenuates inflammation and oxidation in mice with collagen antibody-induced arthritis via TLR4/NF-κB signaling. Mol Med Rep (2016) 14(2):1365–70. doi: 10.3892/mmr.2016.5357
197. Xiao C, Li J, Dong X, He X, Niu X, Liu C, et al. Anti-oxidative and TNF-α suppressive activities of puerarin derivative (4AC) in RAW264.7 cells and collagen-induced arthritic rats. Eur J Pharmacol (2011) 666(1-3):242–50. doi: 10.1016/j.ejphar.2011.05.061
198. Chen X, Huang C, Sun H, Hong H, Jin J, Bei C, et al. Puerarin suppresses inflammation and ECM degradation through Nrf2/HO-1 axis in chondrocytes and alleviates pain symptom in osteoarthritic mice. Food Funct (2021) 12(5):2075–89. doi: 10.1039/d0fo03076g
199. Singh AK, Jiang Y, Gupta S, Younus M, Ramzan M. Anti-inflammatory potency of nano-formulated puerarin and curcumin in rats subjected to the lipopolysaccharide-induced inflammation. J Med Food (2013) 16:899–911. doi: 10.1089/jmf.2012.0049
200. Chen X, Wang L, Fan S, Song S, Min H, Wu Y, et al. Puerarin acts on the skeletal muscle to improve insulin sensitivity in diabetic rats involving muopioid receptor. Eur J Pharmacol (2017) 818:115–23. doi: 10.1016/j.ejphar.2017.10.033
201. Dong F, Wang C, Duan J, Zhang W, Xiang D, Li M. Puerarin attenuates ovalbumin-induced lung inflammation and hemostatic unbalance in rat asthma model. Evid Based Complement Alternat Med (2014) 2014):726740. doi: 10.1155/2014/726740
202. Bao MH, Zhang YW, Lou XY, Xiao Y, Cheng Y, Zhou HH. Puerarin protects endothelial cells from oxidized low density lipoprotein induced injuries via the suppression of LOX-1 and induction of eNOS. Can J Physiol Pharmacol (2014) 92:299–306. doi: 10.1139/cjpp-2013-0322
203. Lu XL, Liu JX, Wu Q, Long SM, Zheng MY, Yao XL, et al. Protective effects of puerarin against Ass40-induced vascular dysfunction in zebrafish and human endothelial cells. Eur J Pharmacol (2014) 732:76–85. doi: 10.1016/j.ejphar.2014.03.030
204. Zhu LH, Wang L, Wang D, Jiang H, Tang QZ, Yan L, et al. Puerarin attenuates high-glucose-and diabetes-induced vascular smooth muscle cell proliferation by blocking PKCbeta2/Rac1-dependent signaling. Free Radic Biol Med (2010) 48:471–82. doi: 10.1016/j.freeradbiomed.2009.10.040
205. Tang L, Liu D, Yi X, Xu T, Liu Y, Luo Y, et al. The protective effects of puerarin in cardiomyocytes from anoxia/reoxygenation injury are mediated by PKCepsilon. Cell Biochem Funct (2014) 32:378–86. doi: 10.1002/cbf.3026
206. Liu X, Mei Z, Qian J, Zeng Y, Wang M. Puerarin partly counteracts the inflammatory response after cerebral ischemia/reperfusion via activating the cholinergic anti-inflammatory pathway. Neural Regeneration Res (2013) 8:3203–15. doi: 10.3969/j.issn.1673-5374.2013.34.004
207. Wang N, Zhang Y, Wu L, Wang Y, Cao Y, He L, et al. Puerarin protected the brain from cerebral ischemia injury via astrocyte apoptosis inhibition. Neuropharmacology (2014) 79:282–9. doi: 10.1016/j.neuropharm.2013.12.004
208. Ahmad S, Mittal S, Gulia R, Alam K, Saha TK, Arif Z, et al. Therapeutic role of hesperidin in collagen-induced rheumatoid arthritis through antiglycation and antioxidant activities. Cell Biochem Funct (2022) 40(5):473–80. doi: 10.1002/cbf.3708
209. Umar S, Kumar A, Sajad M, Zargan J, Ansari MM, Ahmad S, et al. Hesperidin inhibits collagen-induced arthritis possibly through suppression of free radical load and reduction in neutrophil activation and infiltration. Rheumatol Int (2013) 33(3):657–63. doi: 10.1007/s00296-012-2430-4
210. Qi W, Lin C, Fan K, Chen Z, Liu L, Feng X, et al. Hesperidin inhibits synovial cell inflammation and macrophage polarization through suppression of the PI3K/AKT pathway in complete freund's adjuvant-induced arthritis in mice. Chem Biol Interact (2019) 306:19–28. doi: 10.1016/j.cbi.2019.04.002
211. Liu Y, Sun Z, Xu D, Liu J, Li X, Wu X, et al. Hesperidin derivative-11 inhibits fibroblast-like synoviocytes proliferation by activating secreted frizzled-related protein 2 in adjuvant arthritis rats. Eur J Pharmacol (2017) 794:173–83. doi: 10.1016/j.ejphar.2016.10.004
212. Li R, Cai L, Ren DY, Xie XF, Hu CM, Li J. Therapeutic effect of 7, 3'-dimethoxy hesperetin on adjuvant arthritis in rats through inhibiting JAK2-STAT3 signal pathway. Int Immunopharmacol (2012) 14(2):157–63. doi: 10.1016/j.intimp.2012.07.001
213. Li YS, Zhang J, Tian GH, Shang HC, Tang HB. Kirenol, darutoside and hesperidin contribute to the anti-inflammatory and analgesic activities of siegesbeckiapubescensmakino by inhibiting COX-2 expression and inflammatory cell infiltration. J Ethnopharmacol (2021) 268:113547. doi: 10.1016/j.jep.2020.113547
214. Ríos JL, Recio MC, Giner RM, Máñez S. An update review of saffron and its active constituents. Phytother Res (1996) 10(3):189–93. doi: 10.1002/(SICI)1099-1573(199605)10:3<189:AID-PTR754>3.0.CO;2-C
215. Mollazadeh H, Emami SA, Hosseinzadeh H. Razi's Al-hawi and saffron (Crocus sativus): a review. Iran J Basic Med Sci (2015) 18(2):1153–66.
216. Hosseinzadeh H, Nassiri-Asl M. Avicenna's (Ibn sina) the canon of medicine and saffron (Crocus sativus): a review. Phytother Res (2013) 27(4):475–83. doi: 10.1002/ptr.4784
217. Gohari AR, Saeidnia S, Mahmoodabadi MK. An overview on saffron, phytochemicals, and medicinal properties. Pharmacogn Rev (2013) 7(13):61–6. doi: 10.4103/0973-7847.112850
218. Baghishani F, Mohammadipour A, Hosseinzadeh H, Hosseini M, Ebrahimzadeh-Bideskan A. The effects of tramadol administration on hippocampal cell apoptosis, learning and memory in adult rats and neuroprotective effects of crocin. Metab Brain Dis (2018) 33(3):907–16. doi: 10.1007/s11011-018-0194-6
219. Haeri P, Mohammadipour A, Heidari Z, Ebrahimzadeh-Bideskan A. Neuroprotective effect of crocin on substantia nigra in MPTP-induced parkinson's disease model of mice. Anat Sci Int (2019) 94(1):119–27. doi: 10.1007/s12565-018-0457-7
220. Ebrahimi B, Vafaei S, Rastegar-Moghaddam SHR, Hosseini M, Tajik Yabr F, Mohammadipour A. Crocin administration from childhood to adulthood increases hippocampal neurogenesis and synaptogenesis in Male MiceJournal of kerman university of medical sciences. J Kerman Univ Med Sci (2021) 28(3):243–51. doi: 10.22062/jkmu.2021.91664
221. Shafiee M, Arekhi S, Omranzadeh A, Sahebkar A. Saffron in the treatment of depression, anxiety and other mental disorders: Current evidence and potential mechanisms of action. J Affect Disord (2018) 227:330–7. doi: 10.1016/j.jad.2017.11.020
222. Vafaei S, Motejaded F, Ebrahimzadeh-bideskan A. Protective effect of crocin on electromagnetic field-induced testicular damage and heat shock protein A2 expression in Male BALB/c mice. Iran J Basic Med Sci (2020) 23(1):102–10. doi: 10.22038/ijbms.2019.38896.9229
223. Hatziagapiou K, Kakouri E, Lambrou GI, Bethanis K, Tarantilis PA. Antioxidant properties of crocus sativus l. and its constituents and relevance to neurodegenerative diseases; focus on alzheimer's and parkinson's disease. Curr Neuropharmacol (2019) 17(4):377–402. doi: 10.2174/1570159x16666180321095705
224. Lv B, Huo F, Zhu Z, Xu Z, Dang X, Chen T. Crocin upregulates CX3CR1 expression by suppressing NF-Kb/yy1 signaling and inhibiting lipopolysaccharide-induced microglial activation. Neurochem Res (2016) 41(8):1949–57. doi: 10.1007/s11064-016-1905-1
225. Korani S, Korani M, Sathyapalan T, Sahebkar A. Therapeutic effects of crocin in autoimmune diseases: A review. Biofactors (2019) 45(6):835–43. doi: 10.1002/biof.1557
226. Hemshekhar M, Sebastin Santhosh M, Sunitha K, Thushara RM, Kemparaju K, Rangappa KS. A dietary colorant crocin mitigates arthritis and associated secondary complications by modulating cartilage deteriorating enzymes, inflammatory mediators and antioxidant status. Biochimie (2012) 94(12):2723–33. doi: 10.1016/j.biochi.2012.08.013
227. Hu Y, Liu X, Xia Q, Yin T, Bai C, Wang Z. Comparative anti-arthritic investigation of iridoid glycosides and crocetin derivatives from gardenia jasminoides Ellis in freund's complete adjuvant-induced arthritis in rats. Phytomedicine (2019) 53(2):223–33. doi: 10.1016/j.phymed.2018.07.005
228. Liu W, Sun Y, Cheng Z, Guo Y, Liu P, Wen Y. Crocin exerts anti-inflammatory and anti-arthritic effects on type II collagen-induced arthritis in rats. Pharm Biol (2019) 56(1):209–16. doi: 10.1080/13880209.2018.1448874
229. Li X, Jiang C, Zhu W. Crocin reduces the inflammation response in rheumatoid arthritis. biosci. biotechnol. Biochem (2017) 81(5):891–8. doi: 10.1080/09168451.2016.1263145
230. Li L, Zhang H, Jin S, Liu C. Effects of crocin on inflammatory activities in human fibroblast-like synoviocytes and collagen-induced arthritis in mice. Immunol Res (2018) 66(3):406–13. doi: 10.1007/s12026-018-8999-2
231. Wang JF, Xu HJ, He ZL, Yin Q, Cheng W. Crocin alleviates pain hyperalgesia in AIA rats by inhibiting the spinal Wnt5a/β-catenin signaling pathway and glial activation. Neural Plast (2020) 4297483. doi: 10.1155/2020/4297483
232. Mao QQ, Xu XY, Cao SY, Gan RY, Corke H, Beta T, et al. Bioactive compounds and bioactivities of ginger (Zingiber officinale Roscoe). Foods (2019) 8(6):185. doi: 10.3390/foods8060185
233. Lindblad AJ, Koppula S. Ginger for nausea and vomiting of pregnancy. Can Fam Physician. (2016) 62(2):145.
234. Kiyama R. Nutritional implications of ginger: chemistry, biological activities and signaling pathways. J Nutr Biochem (2020) 86:108486. doi: 10.1016/j.jnutbio.2020.108486
235. Letarouilly JG, Sanchez P, Nguyen Y, Sigaux J, Czernichow S, Flipo RM, et al. Efficacy of spice supplementation in rheumatoid arthritis: A systematic literature review. Nutrients (2020) 12(12):3800. doi: 10.3390/nu12123800
236. Kiuchi F, Iwakami S, Shibuya M, Hanaoka F, Sankawa U. Inhibition of prostaglandin and leukotriene biosynthesis by gingerols and diarylheptanoids. Chem Pharm Bull (1992) 40(2):387–91. doi: 10.1248/cpb.40.387
237. Ribel-Madsen S, Bartels EM, Stockmarr A, Borgwardt A, Cornett C, Danneskiold-Samsøe B, et al. A synoviocyte model for osteoarthritis and rheumatoid arthritis: response to ibuprofen, betamethasone, and ginger extract-a cross-sectional in vitro study. Arthritis (2012) 2012:505842. doi: 10.1155/2012/505842
238. Young HY, Luo YL, Cheng HY, Hsieh WC, Liao JC, Peng WH. Analgesic and anti-inflammatory activities of [6]-gingerol. J Ethnopharmacol (2005) 96(1-2):207–10. doi: 10.1016/j.jep.2004.09.009
239. Ojewole JA. Analgesic, antiinflammatory and hypoglycaemic effects of ethanol extract of zingiber officinale (Roscoe) rhizomes (Zingiberaceae) in mice and rats. Phytother Res (2006) 20(9):764–72. doi: 10.1002/ptr.1952
240. Srivastava KC, Mustafa T. Ginger (Zingiber officinale) in rheumatism and musculoskeletal disorders. Med Hypotheses (1992) 39(4):342–8. doi: 10.1016/0306-9877(92)90059-l
241. van Breemen RB, Tao Y, Li W. Cyclooxygenase-2 inhibitors in ginger (Zingiber officinale). Fitoterapia (2011) 82(1):38–43. doi: 10.1016/j.fitote.2010.09.004
242. Grzanna R, Lindmark L, Frondoza CG. Ginger–an herbal medicinal product with broad anti-inflammatory actions. J Med Food (2005) 8(2):125–32. doi: 10.1089/jmf.2005.8.125
243. Nurtjahja-Tjendraputra E, Ammit AJ, Roufogalis BD, Tran VH, Duke CC. Effective anti-platelet and COX-1 enzyme inhibitors from pungent constituents of ginger. Thromb Res (2003) 111(4-5):259–65. doi: 10.1016/j.thromres.2003.09.009
244. Tripathi S, Bruch D, Kittur DS. Ginger extract inhibits LPS induced macrophage activation and function. BMC Complement Altern Med (2008) 8:1. doi: 10.1186/1472-6882-8-1
245. Lee HY, Park SH, Lee M. 1-Dehydro-[10]-gingerdione from ginger inhibits IKKβ activity for NF-κB activation and suppresses NF-κB-regulated expression of inflammatory genes. Br J Pharmacol (2012) 167(1):128–40. doi: 10.1111/j.1476-5381.2012.01980.x
246. Hariri M, Ghiasvand R. Cinnamon and chronic diseases. Adv Exp Med Biol (2016) 929:1–24. doi: 10.1007/978-3-319-41342-6_1
247. Gruenwald J, Freder J, Armbruester N. Cinnamon and health. Crit Rev Food Sci Nutr (2010) 50(9):822–34. doi: 10.1080/10408390902773052
248. Cao H, Urban JF Jr, Anderson RA. Cinnamon polyphenol extract affects immune responses by regulating anti- and proinflammatory and glucose transporter gene expression in mouse macrophages. J Nutr (2008) 138(5):833–40. doi: 10.1093/jn/138.5.833
249. Sharma S, Mandal A, Kant R, Jachak S, Jagzape M. Is cinnamon efficacious for glycaemic control in type-2 diabetes mellitus? J Pak Med Assoc (2020) 70(11):2065–9.
250. Wu SJ, Ng LT, Lin CC. Cinnamaldehyde-induced apoptosis in human PLC/PRF/5 cells through activation of the proapoptotic bcl-2 family proteins and MAPK pathway. Life Sci (2005) 77(8):938–51. doi: 10.1016/j.lfs.2005.02.005
251. Reddy AM, Seo JH, Ryu SY, Kim YS, Kim YS, Min KR, et al. Cinnamaldehyde and 2-methoxycinnamaldehyde as NF-kappaB inhibitors from cinnamomum cassia. Planta Med (2004) 70(9):823–7. doi: 10.1055/s-2004-827230
252. Youn HS, Lee JK, Choi YJ, Saitoh SI, Miyake K, Hwang DH, et al. Cinnamaldehyde suppresses toll-like receptor 4 activation mediated through the inhibition of receptor oligomerization. Biochem Pharmacol (2008) 75(2):494–502. doi: 10.1016/j.bcp.2007.08.033
253. Kowalska J, Tyburski J, Matysiak K, Jakubowska M, Łukaszyk J, Krzymińska J. Cinnamon as a useful preventive substance for the care of human and plant health. Molecules (2021) 26(17):5299. doi: 10.3390/molecules26175299
254. Cho N, Lee KY, Huh J, Choi JH, Yang H, Jeong EJ, et al. Cognitive-enhancing effects of rhusverniciflua bark extract and its active flavonoids with neuroprotective and anti-inflammatory activities. Food Chem Toxicol (2013) 58:355–61. doi: 10.1016/j.fct.2013.05.007
255. Cao H, Anderson RA. Cinnamon polyphenol extract regulates tristetraprolin and related gene expression in mouse adipocytes. J Agric Food Chem (2011) 59(6):2739–44. doi: 10.1021/jf103527x
256. Azab KS, Mostafa AHA, Ali EM, Abdel-Aziz MA. Cinnamon extract ameliorates ionizing radiation-induced cellular injury in rats. Ecotoxicol Environ Saf (2011) 74(8):2324–9. doi: 10.1016/j.ecoenv.2011.06.016
257. Rathi B, Bodhankar S, Mohan V, Thakurdesai P. Ameliorative effects of a polyphenolic fraction of cinnamomum zeylanicum l. bark in animal models of inflammation and arthritis. Sci Pharm (2013) 81(2):567–90. doi: 10.3797/scipharm.1301-16
258. Lee BJ, Kim YJ, Cho DH, Sohn NW, Kang H. Immunomodulatory effect of water extract of cinnamon on anti-CD3-induced cytokine responses and p38, JNK, ERK1/2, and STAT4 activation. Immunopharmacol Immunotoxicol (2011) 33(4):714–22. doi: 10.3109/08923973.2011.564185
259. Mateen S, Rehman MT, Shahzad S, Naeem SS, Faizy AF, Khan AQ, et al. Anti-oxidant and anti-inflammatory effects of cinnamaldehyde and eugenol on mononuclear cells of rheumatoid arthritis patients. Eur J Pharmacol (2019) 852:14–24. doi: 10.1016/j.ejphar.2019.02.031
260. Wang H, Wang Q, Shi R, Zhang M, Liu S, Fan X, et al. Effects of cinnamic aldehyde and total glucosides of paeony on synovial inflammatory response of osteoarthritis. Zhonghua J Tradit Chin Med (2018) 33(8):3356–60.
261. Wang H, Tang X, Ding H, Zhang M, Yang L, Dong Z, et al. Experimental study on effects of cinnamic aldehyde on synovial inflammation in osteoarthritic based on the MicroRNA-146a Interference. World Chin Med (2017) 12(10):2408–13. doi: 10.3969/j.issn.1673-7202.2017.10.034
262. Liu P, Wang J, Wen W, Pan T, Chen H, Fu Y, et al. Cinnamaldehyde suppresses NLRP3 derived IL-1β via activating succinate/HIF-1 in rheumatoid arthritis rats. Int Immunopharmacol (2020) 84:106570. doi: 10.1016/j.intimp.2020.106570
263. Wan Y, Li H, Fu G, Chen X, Chen F, Xie M. The relationship of antioxidant components and antioxidant activity of sesame seed oil. J Sci Food Agric (2015) 95(13):2571–8. doi: 10.1002/jsfa.7035
264. Sankar D, Rao MR, Sambandam G, Pugalendi KV. A pilot study of open label sesame oil in hypertensive diabetics. J Med Food (2006) 9(3):408–12. doi: 10.1089/jmf.2006.9.408
265. Sankar D, Ali A, Sambandam G, Rao R. Sesame oil exhibits synergistic effect with anti-diabetic medication in patients with type 2 diabetes mellitus. Clin Nutr (2011) 30(3):351–8. doi: 10.1016/j.clnu.2010.11.005
266. Nakano D, Kurumazuka D, Nagai Y, Nishiyama A, Kiso Y, Matsumura Y. Dietary sesamin suppresses aortic NADPH oxidase in DOCA salt hypertensive rats. Clin Exp Pharmacol Physiol (2008) 35(3):324–6. doi: 10.1111/j.1440-1681.2007.04817.x
267. Miyawaki T, Aono H, Toyoda-Ono Y, Maeda H, Kiso Y, Moriyama K. Antihypertensive effects of sesamin in humans. J Nutr Sci Vitaminol (Tokyo) (2009) 55(1):87–91. doi: 10.3177/jnsv.55.87
268. Rogi T, Tomimori N, Ono Y, Kiso Y. The mechanism underlying the synergetic hypocholesterolemic effect of sesamin and α-tocopherol in rats fed a high-cholesterol diet. J Pharmacol Sci (2011) 115(3):408–16. doi: 10.1254/jphs.10287fp
269. Yuliana ND, Iqbal M, Jahangir M, Wijaya CH, Korthout H, Kottenhage M, et al. Screening of selected Asian spices for anti obesity-related bioactivities. Food Chem (2011) 126(4):1724–9. doi: 10.1016/j.foodchem.2010.12.066
270. Abdel-Daim MM, Taha R, Ghazy EW, El-Sayed YS. Synergistic ameliorative effects of sesame oil and alpha-lipoic acid against subacute diazinon toxicity in rats: hematological, biochemical, and antioxidant studies. Can J Physiol Pharmacol (2016) 94(1):81–8. doi: 10.1139/cjpp-2015-0131
271. Hashempour-Baltork F, Torbati M, Azadmard-Damirchi S, Savage GP. Vegetable oil blending: A review of physicochemical, nutritional and health effects. Trends Food Sci Technol (2016) 57(1):52–8. doi: 10.1016/j.tifs.2016.09.007
272. Abdel-Daim MM, Taha R, Ghazy EW, El-Sayed YS. Synergistic ameliorative effects of sesame oil and alpha-lipoic acid against subacute diazinon toxicity in rats: Hematological, biochemical, and antioxidant studies. Can J Physiol Pharmacol (2015) 94(1):81–8. doi: 10.1139/cjpp-2015-0131
273. McKay DL, Blumberg JB. Cranberries (Vaccinium macrocarpon) and cardiovascular disease risk factors. Nutr Rev (2007) 65:490–502. doi: 10.1301/nr.2007.nov.490-502
274. Pedersen CB, Kyle J, Jenkinson AM, Gardner PT, McPhail DB, Duthie GG. Effects of blueberry and cranberry juice consumption on the plasma antioxidant capacity of healthy female volunteers. Eur J Clin Nutr (2000) 54:405–8. doi: 10.1038/sj.ejcn.1600972
275. Ruel G, Couillard C. Evidences of the cardioprotective potential of fruits: the case of cranberries. Mol Nutr Food Res (2007) 51:692–701. doi: 10.1002/mnfr.200600286
276. Ruel G, Pomerleau S, Couture P, Lamarche B, Couillard C. Changes in plasma antioxidant capacity and oxidized low-density lipoprotein levels in men after short-term cranberry juice consumption. Metabolism (2005) 54:856–61. doi: 10.1016/j.metabol.2005.01.031
277. Ruel G, Pomerleau S, Couture P, Lemieux S, Lamarche B, Couillard C. Low-calorie cranberry juice supplementation reduces plasma oxidized LDL and cell adhesion molecule concentrations in men. Br J Nutr (2008) 99:352–9. doi: 10.1017/S0007114507811986
278. Lee IT, Chan YC, Lin CW, Lee WJ, Sheu WH-H. Effect of cranberry extracts on lipid profiles in subjects with type 2 diabetes. Diabetes Med (2008) 25:1473–7. doi: 10.1111/j.1464-5491.2008.02588.x
279. Basu A, Betts NM, Ortiz J, Simmons B, Wu M, Lyons TJ. Low-energy cranberry juice decreases lipid oxidation and increases plasma antioxidant capacity in women with metabolic syndrome. Nutr Res (2011) 31:190–6. doi: 10.1016/j.nutres.2011.02.003
280. Martínez-Florez S, Guti errez-Fern andez B, Sanchez-Campos S, Gonz alez-Gallego J, Tun~on MJ. Quercetin attenuates nuclear factor- kB activation and nitric oxide production in interleukin-1bactivated rat hepatocytes. J Nutr (2005) 135:1359–65. doi: 10.1093/jn/135.6.1359
281. Ditano-Vázquez P, Torres-Peña JD, Galeano-Valle F, Pérez-Caballero AI, Demelo-Rodríguez P, Lopez-Miranda J, et al. The fluid aspect of the Mediterranean diet in the prevention and management of cardiovascular disease and diabetes: The role of polyphenol content in moderate consumption of wine and olive oil. Nutrients (2019) 11(11):2833. doi: 10.3390/nu11112833
282. Finicelli M, Squillaro T, Galderisi U, Peluso G. Polyphenols, the healthy brand of olive oil: Insights and perspectives. Nutrients (2021) 13(11):3831. doi: 10.3390/nu13113831
283. Romani A, Ieri F, Urciuoli S, Noce A, Marrone G, Nediani C. Health effects of phenolic compounds found in extra-virgin olive oil, by-products, and leaf of OleaeuropaeaL. Nutrients (2019) 11(1):1–33. doi: 10.3390/nu11081776
284. Mahdavi Siuki M, Fazel Nasab N, Barati E, NezafatFirizi M, Jalilvand T, Namdar Ahmadabad H. The protective effect of berberine against lipopolysaccharide-induced abortion by modulation of inflammatory/immune responses. Immunopharmacol Immunotoxicol (2018) 40(1):333–7. doi: 10.1080/08923973.2018.1485155
285. Rosillo MÁ, Alarcón-de-la-Lastra C, Castejón ML, Montoya T, Cejudo-Guillén M, Sánchez-Hidalgo M. Polyphenolic extract from extra virgin olive oil inhibits the inflammatory response in IL-1β -activated synovial fibroblasts. Br J Nutr (2019) 121(1):55–62. doi: 10.1017/S0007114518002829
286. Romani A, Ieri F, Urciuoli S, Noce A, Marrone G, Nediani C. Health effects of phenolic compounds found in extra-virgin olive oil, by-products, and leaf of OleaeuropaeaL. Nutrients (2019) 11(2):1–33. doi: 10.3390/nu11081776
287. Gorzynik-Debicka M, Przychodzen P, Cappello F, Kuban-Jankowska A, Marino Gammazza A, Knap N, et al. Potential health benefits of olive oil and plant polyphenols. Int J Mol Sci (2018) 19(3):686. doi: 10.3390/ijms19030686
288. Santangelo C, Vari R, Scazzocchio B, De Sanctis P, Giovannini C, D'Archivio M, et al. Anti-inflammatory activity of extra virgin olive oil polyphenols: Which role in the prevention and treatment of immune-mediated inflammatory diseases? Endocr Metab Immune Disord Drug Targets (2018) 18(1):36–50. doi: 10.2174/1871530317666171114114321
289. Zia A, Farkhondeh T, Pourbagher-Shahri AM, Samarghandian S. The role of curcumin in aging and senescence: Molecular mechanisms. BioMed Pharmacother (2021) 134:111119. doi: 10.1016/j.biopha.2020.111119
290. Jabczyk M, Nowak J, Hudzik B, Zubelewicz-Szkodzińska B. Curcumin in metabolic health and disease. Nutrients (2021) 13(12):4440. doi: 10.3390/nu13124440
291. Pourhabibi-Zarandi F, Shojaei-Zarghani S, Rafraf M. Curcumin and rheumatoid arthritis: A systematic review of literature. Int J Clin Pract (2021) 75(10):e14280. doi: 10.1111/ijcp.14280
292. MohammadianHaftcheshmeh S, Khosrojerdi A, Aliabadi A, Lotfi S, Mohammadi A, Momtazi-Borojeni AA. Immunomodulatory effects of curcumin in rheumatoid arthritis: Evidence from molecular mechanisms to clinical outcomes. Rev Physiol Biochem Pharmacol (2021) 179:1–29. doi: 10.1007/112_2020_54
293. Makuch S, Więcek K, Woźniak M. The immunomodulatory and anti-inflammatory effect of curcumin on immune cell populations, cytokines, and In vivo models of rheumatoid arthritis. Pharm (Basel) (2021) 14(4):309. doi: 10.3390/ph14040309
294. Bagherniya M, Darand M, Askari G, Guest PC, Sathyapalan T, Sahebkar A. The clinical use of curcumin for the treatment of rheumatoid arthritis: A systematic review of clinical trials. Adv Exp Med Biol (2021) 1291:251–63. doi: 10.1007/978-3-030-56153-6_15
295. Zeng L, Yang T, Yang K, Yu G, Li J, Xiang W, et al. Curcumin and curcuma longa extract in the treatment of 10 types of autoimmune diseases: A systematic review and meta-analysis of 31 randomized controlled trials. Front Immunol (2022) 13:896476. doi: 10.3389/fimmu.2022.896476
296. Yang M, Akbar U, Mohan C. Curcumin in autoimmune and rheumatic diseases. Nutrients (2019) 11(5):1004. doi: 10.3390/nu11051004
297. Kloesch B, Becker T, Dietersdorfer E, Kiener H, Steiner G. Anti-inflammatory and apoptotic effects of the polyphenol curcumin on human fibroblast-like synoviocytes. Int Immunopharmacol (2013) 15(2):400–5. doi: 10.1016/j.intimp.2013.01.003
298. Ahn JK, Kim S, Hwang J, Kim J, Lee YS, Koh EM, et al. Metabolomic elucidation of the effects of curcumin on fibroblast-like synoviocytes in rheumatoid arthritis. PloS One (2015) 10:e0145539. doi: 10.1371/journal.pone.0145539
299. Moon DO, Kim MO, Choi YH, Park YM, Kim GY. Curcumin attenuates inflammatory response in IL-1beta-induced human synovial fibroblasts and collagen-induced arthritis in mouse model. Int Immunopharmacol (2010) 10(5):605–10. doi: 10.1016/j.intimp.2010.02.011
300. Fan Z, Li J, Liu J, Jiao H, Liu B. Anti-inflammation and joint lubrication dual effects of a novel hyaluronic acid/curcumin nanomicelle improve the efficacy of rheumatoid arthritis therapy. ACS Appl Mater Interfaces (2018) 10:23595–604. doi: 10.1021/acsami.8b06236
301. Dai Q, Zhou D, Xu L, Song X. Curcumin alleviates rheumatoid arthritis-induced inflammation and synovial hyperplasia by targeting mTOR pathway in rats. Drug Des Devel Ther (2018) 12:4095–105. doi: 10.2147/DDDT.S175763
302. Wang Q, Ye C, Sun S, Li R, Shi X, Wang S, et al. Curcumin attenuates collageninduced rat arthritis via anti-inflammatory and apoptotic effects. Int Immunopharmacol (2019) 72:292–300. doi: 10.1016/j.intimp.2019.04.027
303. Zheng Z, Sun Y, Liu Z, Zhang M, Li C, Cai H. The effect of curcumin and its nanoformulation on adjuvant-induced arthritis in rats. Drug Des Devel Ther (2015) 9:4931–42. doi: 10.2147/DDDT.S90147
304. Li X, Xu DQ, Sun DY, Zhang T, He X, Xiao DM. Curcumin ameliorates monosodium urate-induced gouty arthritis through nod-like receptor 3 inflammasome mediation via inhibiting nuclear factor-kappa b signaling. J Cell Biochem (2018) 120:6718–28. doi: 10.1002/jcb.27969
305. Okamoto Y, Tanaka M, Fukui T, Masuzawa T. Inhibition of interleukin 17 production by curcumin in mice with collagen-induced arthritis. BioMed Res (2011) 22:299–304.
306. Capini C, Jaturanpinyo M, Chang HI, Mutalik S, McNally A, Street S. Antigenspecific suppression of inflammatory arthritis using liposomes. J Immunol (2009) 182:3556–65. doi: 10.4049/jimmunol.0802972
307. Sung S, Kwon D, Um E, Kim B. Could polyphenols help in the control of rheumatoid arthritis? Molecules (2019) 24(8):1589. doi: 10.3390/molecules24081589
308. Yahfoufi N, Alsadi N, Jambi M, Matar C. The immunomodulatory and anti-inflammatory role of polyphenols. Nutrients (2018) 10(11):1618. doi: 10.3390/nu10111618
309. Dini I, Grumetto L. Recent advances in natural polyphenol research. Molecules (2022) 27(24):8777. doi: 10.3390/molecules27248777
310. Liu X, Wang Z, Qian H, Tao W, Zhang Y, Hu C, et al. Natural medicines of targeted rheumatoid arthritis and its action mechanism. Front Immunol (2022) 13:945129. doi: 10.3389/fimmu.2022.945129
311. Kim KH, Tsao R, Yang R, Cui SW. Phenolic acid profiles and antioxidant activities of wheat bran extracts and the effect of hydrolysis conditions. Food Chem (2006) 95(3):466–73. doi: 10.1016/j.foodchem.2005.01.032
312. Francini-Pesenti F, Spinella P, Calò LA. Potential role of phytochemicals in metabolic syndrome prevention and therapy. Diabetes Metab Syndrome Obesity: Targets Ther (2019) 12:1987–2002. doi: 10.2147/DMSO.S214550
313. Nagula RL, Wairkar S. Recent advances in topical delivery of flavonoids: A review. J Controlled Release (2019) 296:190–201. doi: 10.1016/j.jconrel.2019.0
314. Kaleem M, Ahmad A. Flavonoids as nutraceuticals. In: Therapeutic, probiotic, and unconventional foods. Cambridge, MA: Academic Press (2018). p. 137–55. doi: 10.1016/B978-0-12-814625-5.00008-X
315. Gautam RK, Roy K, Thapa G, Arora D, Parashar S, Gurung B, et al. Perspective of plant medicine in therapy of rheumatoid arthritis. Indian J Pharm Sci (2020) 82(5):741–65. doi: 10.36468/pharmaceutical-sciences.703
316. Behl T, Upadhyay T, Singh S, Chigurupati S, Alsubayiel AM, Mani V, et al. Polyphenols targeting MAPK mediated oxidative stress and inflammation in rheumatoid arthritis. Molecules (2021) 26(21):6570. doi: 10.3390/molecules26216570
317. Behl T, Mehta K, Sehgal A, Singh S, Sharma N, Ahmadi A, et al. Exploring the role of polyphenols in rheumatoid arthritis. Crit Rev Food Sci Nutr (2022) 62(19):5372–93. doi: 10.1080/10408398.2021.1924613
318. Behl T, Chadha S, Sehgal A, Singh S, Sharma N, Kaur R, et al. Exploring the role of cathepsin in rheumatoid arthritis. Saudi J Biol Sci (2022) 29(1):402–10. doi: 10.1016/j.sjbs.2021.09.014
319. Khan H, Sureda A, Belwal T, Çetinkaya S, Süntar İ, Tejada S, et al. Polyphenols in the treatment of autoimmune diseases. Autoimmun Rev (2019) 18(7):647–57. doi: 10.1016/j.autrev.2019.05.001
320. Mateen S, Moin S, Zafar A, Khan AQ. Redox signaling in rheumatoid arthritis and the preventive role of polyphenols. Clin Chim Acta (2016) 463:4–10. doi: 10.1016/j.cca.2016.10.007
321. Tian J, Chen JW, Gao JS, Li L, Xie X. Resveratrol inhibits TNF-α-induced IL-1β, MMP-3 production in human rheumatoid arthritis fibroblast-like synoviocytes via modulation of PI3kinase/Akt pathway. Rheumatol Int (2013) 33(7):1829–35. doi: 10.1007/s00296-012-2657-0
322. Wang G, Xie X, Yuan L, Qiu J, Duan W, Xu B, et al. Resveratrol ameliorates rheumatoid arthritis via activation of SIRT1-Nrf2 signaling pathway. Biofactors (2020) 46(3):441–53. doi: 10.1002/biof.1599
323. Zhou Y, Jiang Z, Lu H, Xu Z, Tong R, Shi J, et al. Recent advances of natural polyphenols activators for Keap1-Nrf2 signaling pathway. Chem Biodivers (2019) 16(11):e1900400. doi: 10.1002/cbdv.201900400
324. Xu B, Wang G, Zhang J, Cao W, Chen X. Resveratrol decreases FoXO protein expression through PI3K-akt-dependent pathway inhibition in H2O2-treated synoviocytes. Histol Histopathol (2017) 32(12):1305–15. doi: 10.14670/HH-11-884
325. Xu Q, Cao Z, Xu J, Dai M, Zhang B, Lai Q, et al. Effects and mechanisms of natural plant active compounds for the treatment of osteoclast-mediated bone destructive diseases. J Drug Targeting (2022) 30(4):394–412. doi: 10.1080/1061186X.2021.2013488
326. Xu X, Wang M, Wang Z, Chen Q, Chen X, Xu Y, et al. The bridge of the gut-joint axis: Gut microbial metabolites in rheumatoid arthritis. Front Immunol (2022) 13:1007610. doi: 10.3389/fimmu.2022.1007610
327. Zhao Y, Cheng M, Zou L, Yin L, Zhong C, Zha Y, et al. Hidden link in gut-joint axis: gut microbes promote rheumatoid arthritis at early stage by enhancing ascorbate degradation. Gut (2022) 71(5):1041–3. doi: 10.1136/gutjnl-2021-325209
328. Zaiss MM, Joyce Wu HJ, Mauro D, Schett G, Ciccia F. The gut-joint axis in rheumatoid arthritis. Nat Rev Rheumatol (2021) 17(4):224–37. doi: 10.1038/s41584-021-00585-3
329. Zhao T, Wei Y, Zhu Y, Xie Z, Hai Q, Li Z, et al. Gut microbiota and rheumatoid arthritis: From pathogenesis to novel therapeutic opportunities. Front Immunol (2022) 13:1007165. doi: 10.3389/fimmu.2022.1007165
330. Maeda Y, Kurakawa T, Umemoto E, Motooka D, Ito Y, Gotoh K, et al. Dysbiosis contributes to arthritis development via activation of autoreactive T cells in the intestine. Arthritis Rheumatol (2016) 68(11):2646–61. doi: 10.1002/art.39783
331. Aarts J, Boleij A, Pieters BCH, Feitsma AL, van Neerven RJJ, Ten Klooster JP, et al. Flood control: How milk-derived extracellular vesicles can help to improve the intestinal barrier function and break the gut-joint axis in rheumatoid arthritis. Front Immunol (2021) 12:703277. doi: 10.3389/fimmu.2021.703277
332. Yan H, Su R, Xue H, Gao C, Li X, Wang C. Pharmacomicrobiology of methotrexate in rheumatoid arthritis: Gut microbiome as predictor of therapeutic response. Front Immunol (2021) 12:789334. doi: 10.3389/fimmu.2021.789334
333. Hou T, Zhang L, Yang X. Ferulic acid, a natural polyphenol, protects against osteoporosis by activating SIRT1 and NF-κB in neonatal rats with glucocorticoid-induced osteoporosis. BioMed Pharmacother (2019) 120:109205. doi: 10.1016/j.biopha.2019.109205
334. Yang YJ, Zhu Z, Wang DT, Zhang XL, Liu YY, Lai WX, et al. Tanshinol alleviates impaired bone formation by inhibiting adipogenesis via KLF15/PPARγ2 signaling in GIO rats. Acta Pharmacol Sin (2018) 39(4):633–41. doi: 10.1038/aps.2017.134
335. Razali N, Agarwal R, Agarwal P, Tripathy M, Kapitonova MY, Kutty MK, et al. Topical trans-resveratrol ameliorates steroid-induced anterior and posterior segment changes in rats. Exp Eye Res (2016) 143:9–16. doi: 10.1016/j.exer.2015.09.014
336. Wang L, Li Q, Yan H, Jiao G, Wang H, Chi H, et al. Resveratrol protects osteoblasts against dexamethasone-induced cytotoxicity through activation of AMP-activated protein kinase. Drug Des Devel Ther (2020) 14:4451–63. doi: 10.2147/DDDT.S266502
337. Zhai JL, Weng XS, Wu ZH, Guo SG. Effect of resveratrol on preventing steroid-induced osteonecrosis in a rabbit model. Chin Med J (2016) 129(7):824–30. doi: 10.4103/0366-6999.178952. (Engl).
Keywords: dietary polyphenols, rheumatoid arthritis, randomized controlled trial, systematic review, meta-analysis
Citation: Long Z, Xiang W, He Q, Xiao W, Wei H, Li H, Guo H, Chen Y, Yuan M, Yuan X, Zeng L, Yang K, Deng Y and Huang Z (2023) Efficacy and safety of dietary polyphenols in rheumatoid arthritis: A systematic review and meta-analysis of 47 randomized controlled trials. Front. Immunol. 14:1024120. doi: 10.3389/fimmu.2023.1024120
Received: 21 August 2022; Accepted: 27 January 2023;
Published: 22 March 2023.
Edited by:
Rashmi Singh, Banaras Hindu University, IndiaReviewed by:
Jia Xiong, North Carolina State University, United StatesKatarina Bauerova, Slovak Academy of Sciences (SAS), Slovakia
Vikas Kumar, Technology and Sciences, India
Tapan Behl, University of Petroleum and Energy Studies, India
Copyright © 2023 Long, Xiang, He, Xiao, Wei, Li, Guo, Chen, Yuan, Yuan, Zeng, Yang, Deng and Huang. This is an open-access article distributed under the terms of the Creative Commons Attribution License (CC BY). The use, distribution or reproduction in other forums is permitted, provided the original author(s) and the copyright owner(s) are credited and that the original publication in this journal is cited, in accordance with accepted academic practice. No use, distribution or reproduction is permitted which does not comply with these terms.
*Correspondence: Zhiyong Long, MjIxMjQ3MTQzOEBxcS5jb20=; Wang Xiang, NjA5ODM0NTUxQHFxLmNvbQ==; Wei Xiao, eGlhb3czMjEyQDEyNi5jb20=; Zhen Huang, emhodWFuZzMzMjFAMTI2LmNvbQ==
†These authors share first authorship