- 1Molecular Immunity Unit, Department of Medicine, University of Cambridge, Cambridge, United Kingdom
- 2NIHR Cambridge Biomedical Research Centre, Cambridge University Hospitals NHS Foundation Trust, Cambridge, United Kingdom
- 3Wellcome Sanger Institute, Hinxton, United Kingdom
To date, studies of tissue-resident immunity have mainly focused on innate immune cells and T cells, with limited data on B cells. B-1 B cells are a unique subset of B cells with innate-like properties, enriched in murine pleural and peritoneal cavities and distinct from conventional B-2 cells in their ontogeny, phenotype and function. Here we discuss how B-1 cells represent exemplar tissue-resident immune cells, summarizing the evidence for their long-term persistence & self-renewal within tissues, differential transcriptional programming shaped by organ-specific environmental cues, as well as their tissue-homeostatic functions. Finally, we review the emerging data supporting the presence and homeostatic role of B-1 cells across non-lymphoid organs (NLOs) both in mouse and human.
1 Introduction
Our understanding of mammalian immunity is largely based on studying immune cells in blood and lymphoid organs. However, there is a growing appreciation that some subsets of innate and adaptive immune cells reside permanently in non-lymphoid organs (NLOs) without recirculation. To date, studies of these tissue sentinels have mainly focused on innate immune cells and T cells, with limited data on B cells (1–5). Here we discuss B-1 cells, a subset of B cells with “innate-like” properties that have several features ascribed to tissue-resident lymphocytes. We review the current evidence for their presence and homeostatic role in NLOs in mouse and human.
B-1 B cells represent a unique subset of B cells that is distinct from conventional B-2 cells in terms of their ontogeny, phenotype and function (6, 7). Murine B-1 cells were first discovered as Ly-1+ (now CD5+) B cells in early 1980s by Kyoko Hayakawa in Herzenberg’s lab at Stanford University, fueled by the motivation to find the physiological counterpart to human CD5-expressing chronic B-cell leukemia (B-CLL) cells (8, 9). The “B-1” label denotes the fact that their development begins early in ontogeny - preceding B-2 cells. Although the original search for CD5+ B cells led to the identification of this unique subset, later studies reported B cells with B-1 characteristics but lacking CD5 expression. Thus, B-1 cells in mouse, identified as B220lowIgMhiCD23-CD43+IgDlow, have been further sub-classified as B-1a (CD5+) and B-1b (CD5-). While B-1a cells are preferentially produced by yolk-sac and foetal liver precursors, B-1b cells dominate the post-natal generation of B-1 cells in bone marrow (10, 11). Although some studies attempted to explain the existence of two B-1 subsets by the “division of labour” model, for example, the generation of natural versus antigen-driven antibody production in B-1a and B-1b, respectively, others did not observe such a functional split (12–14). Moreover, Savage et al. showed that upon toll-like receptor (TLR) stimulation B-1a B cells down-regulated their CD5 expression, suggesting B-1b cells being simply an activated form of B-1a cells rather than a distinct subset (15).
Although most of the evidence to date examining B-1 cells has utilized murine systems, and their existence in humans has been a matter of controversy, some recent studies applying single cell genomics to pre-natal human cells have clarified aspects of cross-species similarity (16). Here, we will initially focus on the body of work from mouse models, and then turn to human studies of innate-like B cells.
2 Tissue-residency of B-1 cells
Tissue-resident immune cells have several canonical features, exemplified and first demonstrated in macrophages, namely long-term persistence & self-renewal within tissues, differential transcriptional programming shaped by organ-specific environmental cues and tissue-homeostatic functions. These characteristics were subsequently described in tissue-resident T- and NK cells (reviewed by Fan and Rudensky (17)), but here we posit that B-1 cells also exhibit several of these features (Figure 1A).
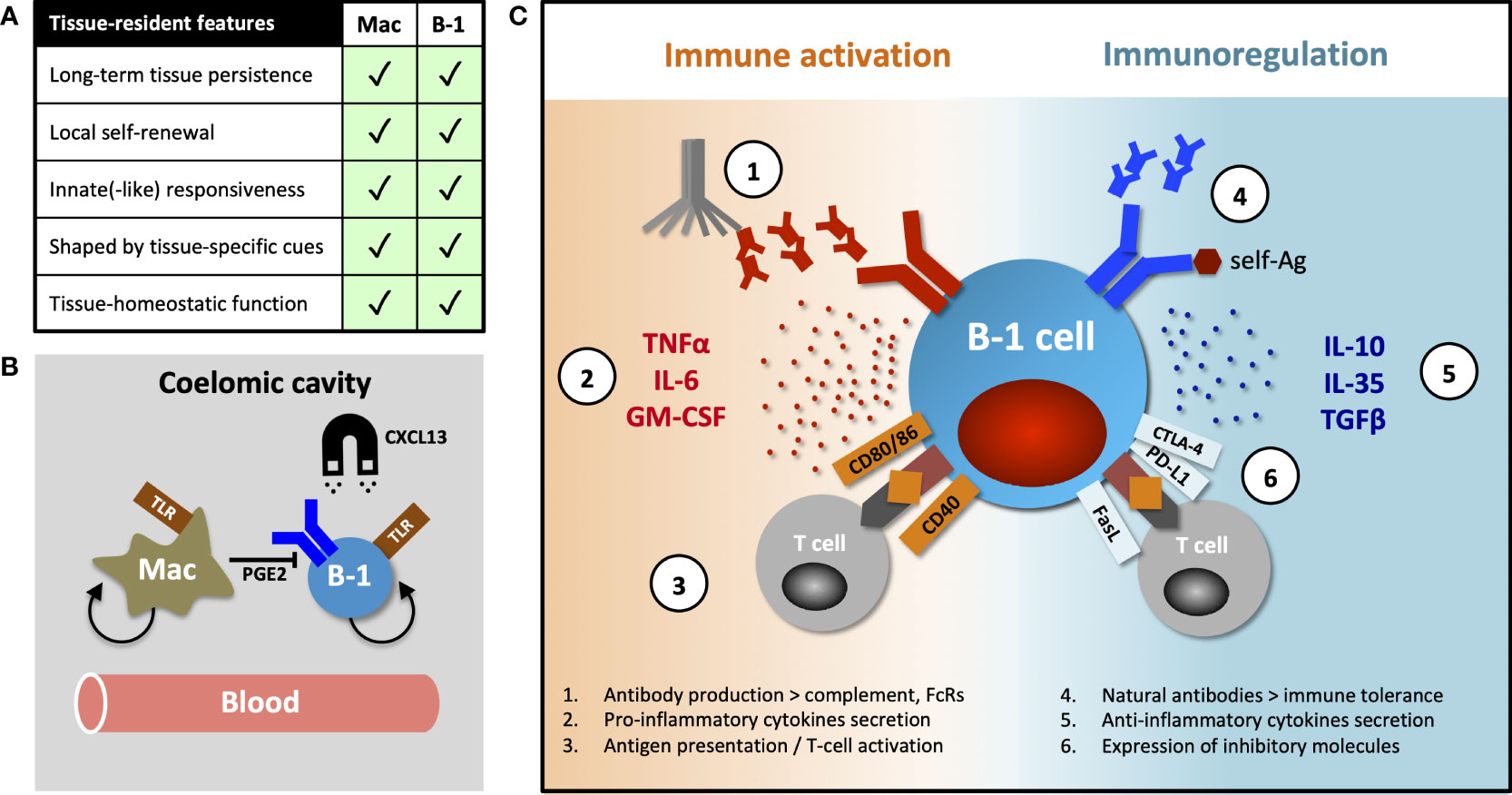
Figure 1 Tissue-resident features of B-1 cells and their diverse functions. (A) Table comparing canonical features of tissue residency between macrophages and B-1 cells. (B) B-1 cell innate-like responsiveness is mediated by TLR signaling and their tissue homing, at least in part, by CXCL13. Peritoneal macrophages influence nearby B-1 cells via prostaglandin E2 (PGE2), inhibiting their natural IgM production. (C) B-1 cells perform their diverse roles (including homeostatic functions) via both antibody-dependent and antibody–independent (cytokines secretion, antigen-specific activation or inhibition of T cells) mechanisms. They promote or regulate immune responses by other immune cells in direct contact-dependent or –independent (by-stander) mechanisms. Contact-dependent interaction with T cells can be antigen-specific, relying on coupling MHC/TCR coupling. While antibodies modulate immune response through complement activation and binding activating and inhibitory Fc-receptors (FcRs), they (in particular, natural antibodies) also play an essential role in maintaining both central and peripheral immune tolerance.
Murine B-1 cells predominantly reside in pleural and peritoneal cavities where they constitute 35-70% of local B cells. A smaller proportion of these cells can also be found in the spleen, bone marrow, lymph nodes and some NLOs (1, 6, 18, 19). Ansel et al. demonstrated that almost 70% of peritoneal B-1 cells remained sessile after 8-week parabiosis and that their homing into the peritoneal cavity was mediated, at least in part, by CXCL13 (20) (Figure 1B). The fact that ~30% of peritoneal B-1 cells exchanged in their parabiosis experiment, and that B-1 cells are detectable in peripheral blood, indicate that some B-1 cells undergo homeostatic re-circulation (6, 14, 20). However, little is known about their patterns of migration and the molecular mechanisms underpinning their dynamic behavior. Certainly, innate stimulation (e.g. via TLR), results in B-1 cell migration from body cavities into secondary lymphoid organs, a phenomenon that seems to be CD11b- and CD6-dependent (14, 21–23).
In addition to high CD11b and low CD6 expression, peritoneal B-1 cells differ from their counterparts in secondary lymphoid organs in terms of their transcriptional profile, immune repertoire (for example, they have a higher frequency of Ighv11-encoded phosphatidyl-choline binders) and inability to secrete natural IgM antibodies (24–27). Adoptive transfer studies, together with the evidence for B-1 cell re-circulation, show site-specific differences in phenotype and function and indicate substantial B-1 cell plasticity and an ability to adapt to organ-specific cues, rather than the existence of several distinct B-1 subsets preferentially seeding different tissues (26, 27). Chace et al. provided a good example of such a tissue-specific influence, showing that peritoneal macrophages inhibit IgM secretion of nearby B-1 cells via prostaglandin E2 secretion (28) (Figure 1B).
3 Self-renewing capacity and innate-like responsiveness of B-1 cells
An important B-1 cell feature is the capacity to self-renew in situ, as suggested by the ability of adoptively transferred mature peritoneal B-1 cells to reconstitute all B-1 cell compartments (10, 29–32). Recently, Clark et al. showed that B-1 cell maintenance and self-renewal in the lipid-rich peritoneal cavity is dependent on autophagy and a specific metabolic programme characterized by fatty acid synthesis, oxidative phosphorylation, but also high levels of glycolysis (33). B-1 cells were able to utilize and store exogenous lipids available in the surrounding microenvironment, further demonstrating their metabolic adaptation to their tissue of residence (33). B-1 cells have unique intracellular signaling characteristics that distinguish them from B-2 cells and are essential for their long-term survival. These include a relative unresponsiveness to BCR crosslinking (evidenced by an inability to mobilize intracellular calcium and a lack of NF-κB activation and proliferation) and active basal tonic signaling downstream of the BCR with constitutive phosphorylation of SYK, ERK and STAT3, which occurs in B-2 cells only after BCR stimulation (34–36). This relative inhibition of BCR-induced proliferation is controlled by the balanced expression of positive (for example, CD19) and negative (for example, CD5, Siglec-G) BCR regulators, the perturbation of which also affected B-1 cell survival (32, 37–39). However, the ligands for these BCR regulators still remain to be found and/or confirmed. Anergic B-2 cells may share some of these characteristics (e.g. unresponsiveness of low-level self-reactive BCRs, constitutive ERK phosphorylation or CD5 expression) (40). However, in contrast to anergic B-2 cells, B-1 cells live longer (both in vitro and in vivo), express higher levels of surface IgM and co-stimulatory molecules, and upon BCR cross-linking phosphorylate AKT and upregulate MHCII (35, 40–42). Interestingly, unlike B-2 cells, B-1 cell development and survival does not seem to be dependent on the myeloid-derived cytokine BAFF (B cell activating factor of the TNF receptor family). Although Lam et al. demonstrated its enhancing role in B-1 cell activation, leading to increased expression of CD21/35 and NF-κB activation (43, 44).
One hallmark of tissue-resident lymphocytes is their innate-like ability to recognize danger and memory/memory-like phenotype, enabling rapid responses. B-1 cells vigorously respond to innate stimuli, for example mediated via TLR or interleukin (IL)5 receptor engagement, leading to their emigration from body cavities into secondary lymphoid organs and to rapid up-regulation of BLIMP1 and their subsequent differentiation into plasma cells, as also observed in marginal zone (MZ) B cells (21, 45, 46). The strong B-1 cell response to innate stimulation and relative BCR unresponsiveness might imply that B-1 cell activation is independent of BCR signaling. However, Savage et al. demonstrated that TLR stimulation in B-1 cells led to the reorganization of their BCR signalosome (including association with CD19 and loss of CD5) which, as a licensing step, released the initial BCR signaling block present in resting B-1 cells (15).
4 Homeostatic function of B-1 cells
One of the key functions of B-1 cells is their homeostatic “spontaneous” production of natural antibodies (predominantly IgM and IgG3), even in the absence of any foreign microbial stimuli (47). Recently, Zeng et al. reported the role of sex hormones in natural antibody production. They found that the female advantage in the clearance of enteropathogenic Escherichia coli was driven by oestrogen-dependent production of natural antibodies that bind the bacterium (48). IL-5, an inducer of BLIMP1 expression, is another factor shown to play a role in tonic antibody secretion (46). As noted above, natural antibodies are produced outside body cavities, mostly in the bone marrow and spleen (26). Savage et al. demonstrated that the population of natural antibody-secreting cells is heterogeneous, containing a significant proportion of non-terminally differentiated, BLIMP1neg cells (49). Recently, Benezech et al. added further complexity to this model of compartmentalized natural IgM secretion, exploring antibody production in fat-associated lymphoid clusters (FALCs) and milky spots located in serous cavities (50). These small anatomical niches are a source of CXCL13, IL33 (from stromal cells) and IL5 (from group 2 innate-lymphoid cells (ILC2), and sites of pleural and peritoneal B-1 cell activation, both during homeostasis and infection; allowing local production of IgM for protection of these body cavities (51, 52).
Natural antibodies are polyreactive, able to react with both self- (PtC, Thy1) and microbial (e.g. pneumococcus or influenza) antigens (13, 53, 54). They provide an instant defense against invading pathogens, for example, by direct neutralization or complement binding, but also promote B-2 antigen-specific responses, possibly by forming immune complexes that are more easily trapped by antigen presenting cells or follicular dendritic cells (13, 55). Natural antibodies also participate in tissue homeostasis by opsonizing and enhancing the clearance of apoptotic cells or low-density lipoproteins by mononuclear phagocytes (56, 57). There is a good body of evidence that B-1 cells also substantially contribute to the production of mucosal IgA, maintaining the symbiotic homeostatic relationship between the host and microbiome, as reviewed by Almut Meyer-Bahlburg (58). However, whether these antibodies are still “natural”, i.e. produced independently of microbial antigens, is hotly debated (58). In addition to the steady-state secretion of natural antibodies, B-1 cells can actively respond to a variety of pathogens or tumor antigens with increased (induced) IgM production following activation (59, 60). There is also some evidence that IgM natural antibodies enforce central tolerance and promote normal development of both B-1 and B-2 cells by a mechanism that is not completely clear, possibly by facilitating the tolerogenic presentation of autoantigens (61). On the other hand, natural antibodies can be an integral part of immunopathological processes such as ischaemia-reperfusion injury or the delayed hypersensitivity response in skin (62, 63).
B-1 cells have been shown to regulate immune response by mechanisms other than antibody secretion, including cytokine production. At steady state, a subset of peritoneal, and to a lesser extent splenic, B-1 cells spontaneously secretes the regulatory cytokine IL10 (64). A protective role for B-1 cell-derived IL10 has been demonstrated in an endotoxaemic-induced systemic inflammatory response and in autoimmune-prone mice (65–67). In addition to IL10, B-1 cells can also exert their regulatory function by secretion of IL35 and transforming growth factor beta (TGFβ) (68, 69) or in contact-dependent manner by expression of inhibitory molecules FasL, PD-L2 and CTLA-4 (in particular, B-1 cells with autoreactive specificities) (70–73).
Conversely, Rauch and Weber et al. described a B-1 cell subset they termed “innate response activator” (IRA) that upon TLR stimulation, migrated from body cavities to spleen or lung to enhance acute inflammatory responses via granulocyte macrophage colony stimulating factor (GM-CSF) and IL3 secretion, in addition to IgM (74, 75). Others observed that peritoneal B-1 cells stimulated with LPS increased both IL10 and IL6 secretion, proposing that the ratio between these cytokines ultimately determines whether their effect on co-cultured CD4 T cells is activating or inhibitory (76). Finally, B-1 cells may also act as efficient antigen-presenting cells and activators of T cells through their high constitutive expression of MHCII and CD80/86, as reviewed by Popi et al. (77).
Together these data indicate that B-1 cells are a functionally heterogeneous population capable of both immune suppression and stimulation (Figure 1C). It is unclear how much functional plasticity exist in any given B-1 cell for these two opposing roles and whether under certain circumstances all B-1 cells can eventually mount a pro-inflammatory response to infection.
5 Presence of B-1 cells in NLOs
Although some studies of tissue-resident memory B cells or natural antibody-producing plasma cells have identified B-1 B cells in NLOs at steady state (5, 78), these NLO-resident B-1 cells have received limited attention to date. However, evidence suggests the homeostatic presence of B-1 cells in skin, lungs, gut, liver and visceral fat, in addition to pleural and peritoneal cavities (Figure 2).
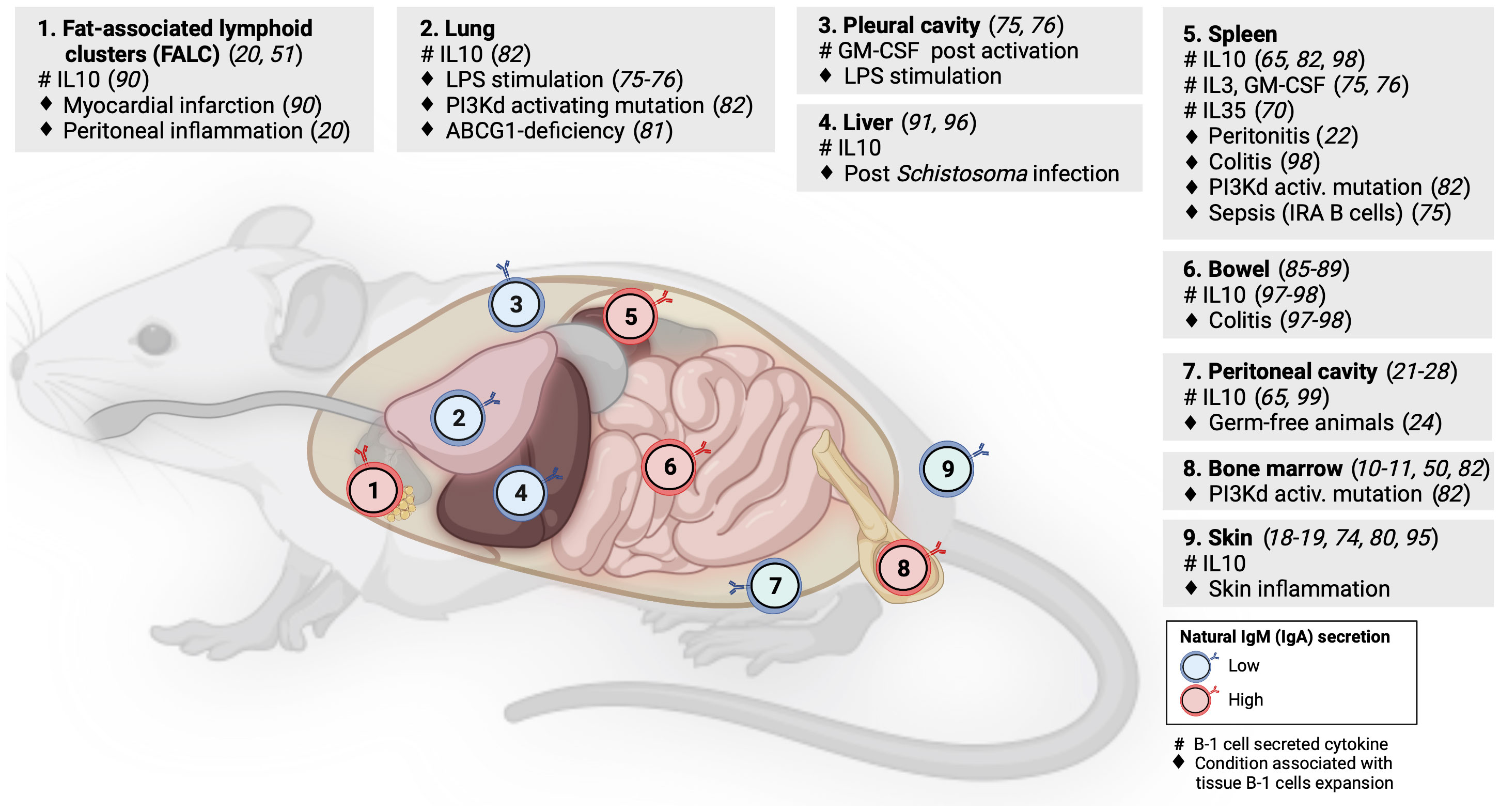
Figure 2 B-1 cell distribution across mouse lymphoid and non-lymphoid organs. Numbers within B-cell icons label mouse organs where B-1 cells have been documented. B-cell icon color indicates whether intra-organ production of natural antibodies is low (blue) or high (red). Grey panels show cytokines produced by tissue B-1 cells (#) and conditions associated with expansion of tissue B-1 cells (♦), linked literature references numbers are in brackets.
Geherin et al. used afferent lymphatic cannulation in sheep to demonstrate the presence of innate-like (IgMhiCD11bhi) B cells trafficking from uninflamed skin (18). Subsequently, they reported IL10+ B-1 B cells residing both in murine and human skin under normal conditions (1). Baldan et al. observed an expansion of B-1 cells in the lungs of ATP-binding cassette transporter G1 (ABCG1)-deficient mice as a result of lipid accumulation and chronic inflammation. These B-1 cells produced natural lipoprotein-binding antibodies that may protect against atherosclerosis (79). Stark et al. found a marked accumulation of aberrant B-1 cells in the lungs of mice with either global or B-cell specific activating mutation in the catalytic subunit of phosphoinositide-3-kinase δ (PI3Kd) that was demonstrated by others to increase the generation of B-1 cells in the bone marrow (80, 81). These lung B-1 cells secreted IL10 and were associated with higher susceptibility to bacterial pneumonia as seen in patients with activated PI3Kδ syndrome (80, 82). Using long-term B cell chimeras, Krosse et al. reported that almost half of IgA secreting plasma cells in the gut were derived from peritoneal B-1 B cells (83). However, this finding was subsequently disputed in gnotobiotic Ig allochimeric mice experiments (84) or by examining Igavh repertoire in lamina propria from L2 mice that lack B-2 cells (85). Recently, two studies re-examined this controversy: Bunker et al. performed a series of B-cell transfer experiments into Rag1-/- mice and found that commensal-specific IgA+ gut plasma cells originated from B-2 and, to a lesser extent, also from B-1b (but not B-1a) cells (86). Vergani et al. demonstrated that B-1a cells and IgA+ gut plasma cells shared the same haematopoietic progenitors in early life using two different lineage-tracing mouse models (87). However, there was no substantial clonal overlap between these two populations, suggesting an early bifurcation likely at the point of antigen selection (87). B-1 cells have been also identified in mouse visceral fat in steady state, forming special lymphoid clusters (19, 88). Zhang et al. reported IL10-producing B-1a cells in wild-type mouse liver but their quantification did not account for B cells from blood contamination (89). The presence of B-1 B cells in mouse liver and lung was indirectly supported also by experiments showing that a small number of cells from homogenized organs spontaneously secreted IgM on ELISPOT (49).
6 Evidence for homeostatic role of tissue-resident B-1 B cells in NLOs
As discussed above, B-1 cells secreting IL10 and natural antibodies have been identified across several NLOs in steady state and with increased abundance during inflammation, suggesting their anti-inflammatory homeostatic role protecting tissue integrity (Figure 2). Skin is an exemplar NLO to demonstrate such a B-1 cell function.
Using radioactive labeling, Geherin et al. showed that B-1 cells migrate from their natural reservoirs in the serous cavities into both non-inflamed, and upon innate stimulation, inflamed skin and intestine, in an α4β1 integrin-dependent manner to provide immunosurveillance and to suppress inflammation (1). The regulatory function of cutaneous B-1 cells is also consistent with several case reports of psoriasis exacerbation after therapeutic B-cell depletion with rituximab or α4-integrin blockade with natalizumab (90–92). Similarly, CD5+ IL10+ B cells were found to suppress contact hypersensitivity dermatitis in a mouse model (73, 93). A recent study by Wu et al. demonstrated the presence of IL10-producing CD5+ B-1a cells in pericardial fat in homeostasis that were subsequently shown to be capable of ameliorating damage associated with myocardial infarction (88). The ameliorating effect of IL10+ B-1 cells on local tissue inflammation was also found in the liver after Schistosoma infection (94) and in the gut during acute and chronic colitis (95, 96).
Although the body of evidence for the homeostatic role of IL10 produced by B-1 cells in NLOs organ immunity is increasing, its precise impact on other immune and non-immune cell subsets within tissues is an outstanding question that requires further investigation. In peritoneal cavity, Wong et al. identified that macrophages are polarized toward anti-inflammatory (M2) phenotype by the presence of B-1 cells both in vitro and in vivo and that this is driven by IL10 but not by IgM (97). They subsequently showed that such B-1 cell driven anti-inflammatory macrophage polarization was detrimental for a skin tumor clearance (97). The regulatory role of tissue innate-like B cells in homeostasis can increase organ susceptibility to infection or be even actively hijacked by pathogens (80, 82). Liu et al. reported that Listeria infection stimulates IL10 production by MZ B cells, which in turn, inhibits inducible nitric oxide synthase (iNOS) production in splenic macrophages, leading to an increased bacterial burden (98). In contrast to the anti-inflammatory function of B-1 cells in NLOs at steady state, there is also data for a pro-inflammatory/protective role of B-1 cells in local infection as exemplified by IRA B-1 cells relocating from the pleural cavity into the lung to protect against pneumonia via GM-CSF and IgM secretion (75).
As outlined above, most studies examining the role of natural antibodies in tissue homeostasis and protection were based on sampling blood or serous cavities. Savage et al. showed that the vast majority of natural IgM-secreting B-1 cells and plasma cells are located in bone marrow and spleen with only less than 5% found in serous cavities and NLOs (49). B-1 cells in peritoneal cavity (outside FALCs/milky spots) are largely suppressed in their natural antibody production by nearby macrophages (28, 50). Although the data on the homeostatic presence of B-1 cells in NLOs suggest some local secretion of natural antibodies, it is unclear if this secretion is inhibited by similar tissue-specific cues as seen in peritoneal cavity.
7 Evidence for B-1 cells in human NLOs
The identification of a human equivalent to murine B-1 cells has been a focus of ongoing study, and a source of heated debate and controversy in the field. Early attempts to find human B-1 cells were based on searching for CD5+ B cells. As noted previously, the vast majority of human B-CLL cells are CD5 positive. A more detailed analysis of these pathological CD5+ B cell clones revealed marked repertoire skewing for auto- and polyreactive BCRs, a feature of murine B-1 cells (99). Recently, Hayakawa et al. found a mouse B-1 cell clone with a non-mutated CDR3 region encoding a binding site for non-muscle myosin IIA, which is often a target of human B-CLL BCRs. These B-1 cells expanded with age and eventually became malignant, likely because of the chronic stimulation with a potent auto-antigen (100). Moreover, expanded polyreactive IgM+/IgA+CD5+ B cells were documented in human autoimmune conditions such as rheumatoid arthritis or IgA nephropathy (101–103).
Given the preferential residence of murine B-1 cells in coelomic cavities, human peritoneal washings were also examined for the presence of CD5+ B cells. Although the relative proportion of these cells was higher than in blood, their absolute number was negligible compared to mouse (104, 105). Early studies reported a significantly increased frequency of CD5+ B cells in human umbilical cord blood, which clearly mirrored findings in mice, in particular with regards to the recently demonstrated role of Lin28b/Let7 as a switch between fetal and adult hematopoiesis, both in mouse and human (101, 102, 106). However, Lee et al. showed subsequently that the majority of CD5+ B cells in human blood represent “pre-naïve” B cells, having an intermediate phenotype between transitional and naïve B cells. Most of these cells lost their CD5 expression upon further differentiation into naïve cells (107). The fact that the frequency of CD5+ B cells in human adult blood is almost 20-times higher than in mice and that CD5 is also expressed by transitional, activated or anergic B-2 cells, further support the notion that CD5 is unlikely to be a useful marker for human B-1 cells (108–110).
One effort to define surface markers of putative human B-1 cells was presented by Griffin et al. (111). When profiling human umbilical cord blood they found an unexpectedly high percentage of CD27+ “memory” B cells (3-11% of CD20+ subset) that also expressed CD43, a canonical marker of murine B-1 cells. Subsequent functional examination of this FACS-sorted subset (CD20+CD27+CD43+) from both neonatal and adult blood confirmed three key B-1 cell characteristics – spontaneous IgM secretion, tonic signaling, and efficient stimulation of T cells (111). The proportion of these putative B-1 cells within CD20+CD27+ subset clearly declined with age, and only three quarters of these cells were CD5 positive. Since CD43 can be a marker of B-2 cell activation, the authors confirmed that other typical markers of activation (i.e. CD69, CD70) were not expressed by these cells (111). In a subsequent study, they further split CD20+CD27+CD43+ human “B-1” cells into CD11b- “secretor” cells (i.e. primarily secreting natural antibodies) and CD11b+ “orchestrator” cells that were expanded in SLE patients, and spontaneously secreted IL10, but could enhance T-cell proliferation and modulate T-cell activation (112, 113). Other groups have struggled to replicate these findings, suggesting potential issues with T cell contamination and doublets and confusion with B-2 pre-plasmablasts (114–116).
Geherin et al. identified B cells in healthy human skin, where 3.9 – 28.6% of them secreted IL10 after four-hour stimulation. Only 3.5% of total skin B cells expressed markers of putative human B-1 B cells (i.e. CD3-CD20+CD27+CD43+) discussed earlier (1). Nihal et al. found that B cells are rare in normal human skin, and hence difficult to phenotype, but using PCR-based Ig heavy chain rearrangements analysis, they showed that these B cells had a clonally-restricted BCR repertoire, potentially indicating antigen-driven clonal expansion. These finding suggested the potential existence of tissue-resident B-1 cells in normal human skin (117).
Recently, we identified putative B-1 cells in human embryonic and foetal tissues using single cell RNA sequencing (16) (Figure 3A). In line with previous reports, these IgMhiIgDlow B cells were CD27+CD43+CD5+ and shared functional characteristics with murine B-1 cells, including spontaneous antibody secretion and BCR features (reduced CDR3 junction length and NP additions and lower mutation frequency) (Figure 3B). Interestingly, by the third trimester, the ratio of B1/mature B cell significantly decreased in most organs except the thymus (Figure 3C) (16). The putative B-1 cells expressed also high levels of CCR10 that may play a role in their tissue homing and retention. CCL28, the ligand of CCR10, was expressed in bone marrow stromal cells, and in skin and gut epithelial cells (Figure 3B) (16).
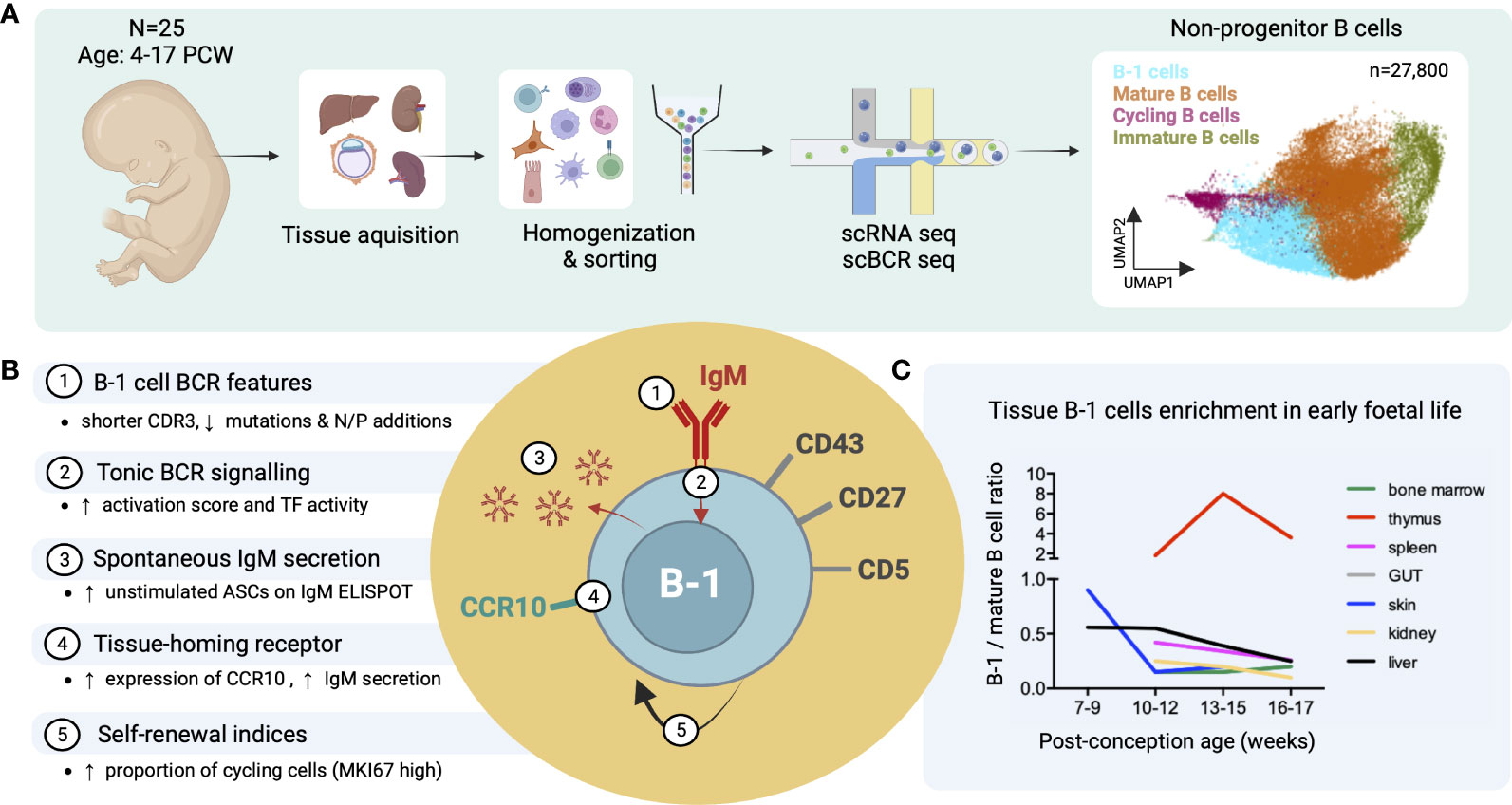
Figure 3 Identification of putative B-1 cells in prenatal human tissues by single cell RNA/BCR sequencing (adapted from Suo et al. (16)). (A) Study design schematic: Tissues from 25 human embryos/foetuses aged between 4 and 17 post-conception weeks (PCW) were homogenized into single cell (sc) suspensions, sorted for CD45+ live cells and subjected to sc RNA and BCR sequencing (10X Genomics). Total 27,800 non-progenitor B cells were recovered from all processed tissues following quality control (shown in UMAP plot, putative B-1 cells in light blue). (B) Characteristics of putative B-1 cells found in this study; mature (IgD+IgM+CD27-) B cells were used as the comparator. CDR3, complementarity-determining region 3; TF, transcription factors; ASC, antibody-secreting cell. (C) Plot showing the change of B-1/mature B cell number ratio across different tissues with post-conception age.
Asano et al. reported an accumulation of innate-like B cells in human kidney allografts during rejection that were transcriptionally similar to murine peritoneal B-1 cells, secreted autoantibodies directed against kidney (rather than donor-specific) antigens and expressed a signature gene, AHNAK (118). However, it is not clear whether these innate-like B cells expanded locally from cells residing in the kidney in homeostasis or infiltrated from the blood or other reservoir.
Finally, there are several examples that illustrate marked inter-species differences in innate-like tissue-resident lymphocyte populations. For example, iNKT cells constitute the main lymphocyte subset in mouse liver, but they represent less than 1% of human liver lymphocytes (119). γδ T cells are more prominent in bovine skin than mouse or human and significantly differ in their oligoclonality and diversity (120). These studies suggest, that while the functions of innate-like tissue-resident lymphocytes are conserved across species, this common function is not necessarily conserved to specific cell types. Moreover, there is some evidence that innate-like lymphocytes, such as epidermal γδ T cells in mouse skin, might be replaced by Trm after an infection (121). It is plausible that similar phenomena could explain why the equivalent to murine B-1 cells cannot be convincingly identified in adult human tissues (including the peritoneal cavity) as they may have been functionally replaced by other innate-like subsets such as MZ B cells. Indeed, Weller et al. demonstrated marked phenotypic and functional similarity between “IgM memory” B cells in peripheral blood and human MZ B cells suggesting their re-circulation (122). Moreover, human MZ-like B cells were also described in the inner wall of the subcapsular sinus of lymph nodes, tonsillar crypts and mucosa-associated lymphoid tissue (MALT) (123–125).
8 Concluding remarks
B-1 cells with their unique combination of innate-like and adaptive functions are well suited to be efficient tissue-resident immune cells. In contrast to B-2 cells, they do not respond to activation by extensive clonal expansion, but rather rapidly migrate, re-distribute and differentiate in a process that seems to be controlled by the receipt of innate immune signals (20, 21, 126). B-1 cells represent an exemplar of how adaptive immune cells residing in NLOs adapt a hybrid functionality with innate and adaptive features, together contributing to the maintenance of local tissue immunity homeostasis and defense. Although tissue-resident innate-like B(-1) cells might have a clear therapeutic potential in a number of intra-organ pathologies (for example, cancer or transplant rejection), future studies should first convincingly delineate their equivalents in human.
Author contributions
OS wrote the manuscript and designed figures. MRC revised the manuscript and designed figures. All authors contributed to the article and approved the submitted version.
Funding
OS was an NIHR Clinical Lecturer funded by Health Education England (HEE)/NIHR (CL-2021-14-004) when completing this work. The views expressed in this publication are those of the authors and not necessarily those of the NIHR, NHS or the UK Department of Health and Social Care.
Acknowledgments
Figures 2, 3 were created using BioRender.com.
Conflict of interest
The authors declare that the research was conducted in the absence of any commercial or financial relationships that could be construed as a potential conflict of interest.
Publisher’s note
All claims expressed in this article are solely those of the authors and do not necessarily represent those of their affiliated organizations, or those of the publisher, the editors and the reviewers. Any product that may be evaluated in this article, or claim that may be made by its manufacturer, is not guaranteed or endorsed by the publisher.
References
1. Geherin SA, Gomez D, Glabman RA, Ruthel G, Hamann A, Debes GF. IL-10+ innate-like b cells are part of the skin immune system and require alpha4beta1 integrin to migrate between the peritoneum and inflamed skin. J Immunol (2016) 196(6):2514–25. doi: 10.4049/jimmunol.1403246
2. Li Y, Innocentin S, Withers DR, Roberts NA, Gallagher AR, Grigorieva EF, et al. Exogenous stimuli maintain intraepithelial lymphocytes via aryl hydrocarbon receptor activation. Cell (2011) 147(3):629–40. doi: 10.1016/j.cell.2011.09.025
3. Schenkel JM, Fraser KA, Beura LK, Pauken KE, Vezys V, Masopust D. T Cell memory. resident memory CD8 T cells trigger protective innate and adaptive immune responses. Science (2014) 346(6205):98–101. doi: 10.1126/science.1254536
4. Steinert EM, Schenkel JM, Fraser KA, Beura LK, Manlove LS, Igyarto BZ, et al. Quantifying memory CD8 T cells reveals regionalization of immunosurveillance. Cell (2015) 161(4):737–49. doi: 10.1016/j.cell.2015.03.031
5. Allie SR, Bradley JE, Mudunuru U, Schultz MD, Graf BA, Lund FE, et al. The establishment of resident memory b cells in the lung requires local antigen encounter. Nat Immunol (2019) 20(1):97–108. doi: 10.1038/s41590-018-0260-6
6. Baumgarth N. The double life of a b-1 cell: self-reactivity selects for protective effector functions. Nat Rev Immunol (2011) 11(1):34–46. doi: 10.1038/nri2901
7. Montecino-Rodriguez E, Dorshkind K. B-1 b cell development in the fetus and adult. Immunity (2012) 36(1):13–21. doi: 10.1016/j.immuni.2011.11.017
8. Hayakawa K, Hardy RR, Honda M, Herzenberg LA, Steinberg AD, Herzenberg LA. Ly-1 b cells: functionally distinct lymphocytes that secrete IgM autoantibodies. Proc Natl Acad Sci U S A (1984) 81(8):2494–8. doi: 10.1073/pnas.81.8.2494
9. Hayakawa K, Hardy RR, Parks DR, Herzenberg LA. The "Ly-1 b" cell subpopulation in normal immunodefective, and autoimmune mice. J Exp Med (1983) 157(1):202–18. doi: 10.1084/jem.157.1.202
10. Lalor PA, Herzenberg LA, Adams S, Stall AM. Feedback regulation of murine ly-1 b cell development. Eur J Immunol (1989) 19(3):507–13. doi: 10.1002/eji.1830190315
11. Hardy RR, Hayakawa K. Perspectives on fetal derived CD5+ B1 b cells. Eur J Immunol (2015) 45(11):2978–84. doi: 10.1002/eji.201445146
12. Haas KM, Poe JC, Steeber DA, Tedder TF. B-1a and b-1b cells exhibit distinct developmental requirements and have unique functional roles in innate and adaptive immunity to s. pneumoniae. Immunity (2005) 23(1):7–18. doi: 10.1016/j.immuni.2005.04.011
13. Baumgarth N, Herman OC, Jager GC, Brown L, Herzenberg LA, Herzenberg LA. Innate and acquired humoral immunities to influenza virus are mediated by distinct arms of the immune system. Proc Natl Acad Sci U S A (1999) 96(5):2250–5. doi: 10.1073/pnas.96.5.2250
14. Waffarn EE, Hastey CJ, Dixit N, Soo Choi Y, Cherry S, Kalinke U, et al. Infection-induced type I interferons activate CD11b on b-1 cells for subsequent lymph node accumulation. Nat Commun (2015) 6:8991. doi: 10.1038/ncomms9991
15. Savage HP, Klasener K, Smith FL, Luo Z, Reth M, Baumgarth N. TLR induces reorganization of the IgM-BCR complex regulating murine b-1 cell responses to infections. Elife. (2019) 8. doi: 10.7554/eLife.46997
16. Suo C, Dann E, Goh I, Jardine L, Kleshchevnikov V, Park JE, et al. Mapping the developing human immune system across organs. Sci (New York NY) (2022) 376(6597):eabo0510. doi: 10.1126/science.abo0510
17. Fan X, Rudensky AY. Hallmarks of tissue-resident lymphocytes. Cell. (2016) 164(6):1198–211. doi: 10.1016/j.cell.2016.02.048
18. Geherin SA, Fintushel SR, Lee MH, Wilson RP, Patel RT, Alt C, et al. The skin, a novel niche for recirculating b cells. J Immunol (2012) 188(12):6027–35. doi: 10.4049/jimmunol.1102639
19. Benezech C, Luu NT, Walker JA, Kruglov AA, Loo Y, Nakamura K, et al. Inflammation-induced formation of fat-associated lymphoid clusters. Nat Immunol (2015) 16(8):819–28. doi: 10.1038/ni.3215
20. Ansel KM, Harris RB, Cyster JG. CXCL13 is required for B1 cell homing, natural antibody production, and body cavity immunity. Immunity (2002) 16(1):67–76. doi: 10.1016/S1074-7613(01)00257-6
21. Ha SA, Tsuji M, Suzuki K, Meek B, Yasuda N, Kaisho T, et al. Regulation of B1 cell migration by signals through toll-like receptors. J Exp Med (2006) 203(11):2541–50. doi: 10.1084/jem.20061041
22. Enyindah-Asonye G, Li Y, Xin W, Singer NG, Gupta N, Fung J, et al. CD6 receptor regulates intestinal Ischemia/Reperfusion-induced injury by modulating natural IgM-producing B1a cell self-renewal. J Biol Chem (2017) 292(2):661–71. doi: 10.1074/jbc.M116.749804
23. Alam C, Bittoun E, Bhagwat D, Valkonen S, Saari A, Jaakkola U, et al. Effects of a germ-free environment on gut immune regulation and diabetes progression in non-obese diabetic (NOD) mice. Diabetologia (2011) 54(6):1398–406. doi: 10.1007/s00125-011-2097-5
24. Hardy RR, Carmack CE, Shinton SA, Riblet RJ, Hayakawa K. A single VH gene is utilized predominantly in anti-BrMRBC hybridomas derived from purified ly-1 b cells. definition of the VH11 family. J Immunol (1989) 142(10):3643–51.
25. Mercolino TJ, Arnold LW, Hawkins LA, Haughton G. Normal mouse peritoneum contains a large population of ly-1+ (CD5) b cells that recognize phosphatidyl choline. relationship to cells that secrete hemolytic antibody specific for autologous erythrocytes. J Exp Med (1988) 168(2):687–98.
26. Choi YS, Dieter JA, Rothaeusler K, Luo Z, Baumgarth N. B-1 cells in the bone marrow are a significant source of natural IgM. Eur J Immunol (2012) 42(1):120–9. doi: 10.1002/eji.201141890
27. Stoermann B, Kretschmer K, Duber S, Weiss S. B-1a cells are imprinted by the microenvironment in spleen and peritoneum. Eur J Immunol (2007) 37(6):1613–20. doi: 10.1002/eji.200636640
28. Chace JH, Fleming AL, Gordon JA, Perandones CE, Cowdery JS. Regulation of differentiation of peritoneal b-1a (CD5+) b cells. activated peritoneal macrophages release prostaglandin E2, which inhibits IgM secretion by peritoneal b-1a cells. J Immunol (Baltimore Md 1950). (1995) 154(11):5630–6.
29. Hayakawa K, Hardy RR, Herzenberg LA, Herzenberg LA. Progenitors for ly-1 b cells are distinct from progenitors for other b cells. J Exp Med (1985) 161(6):1554–68. doi: 10.1084/jem.161.6.1554
30. Lalor PA, Stall AM, Adams S, Herzenberg LA. Permanent alteration of the murine ly-1 b repertoire due to selective depletion of ly-1 b cells in neonatal animals. Eur J Immunol (1989) 19(3):501–6. doi: 10.1002/eji.1830190314
31. Hayakawa K, Hardy RR, Stall AM, Herzenberg LA, Herzenberg LA. Immunoglobulin-bearing b cells reconstitute and maintain the murine ly-1 b cell lineage. Eur J Immunol (1986) 16(10):1313–6.
32. Krop I, de Fougerolles AR, Hardy RR, Allison M, Schlissel MS, Fearon DT. Self-renewal of b-1 lymphocytes is dependent on CD19. Eur J Immunol (1996) 26(1):238–42.
33. Clarke AJ, Riffelmacher T, Braas D, Cornall RJ, Simon AK. B1a b cells require autophagy for metabolic homeostasis and self-renewal. J Exp Med (2018) 215(2):399–413. doi: 10.1084/jem.20170771
34. Chumley MJ, Dal Porto JM, Cambier JC. The unique antigen receptor signaling phenotype of b-1 cells is influenced by locale but induced by antigen. J Immunol (2002) 169(4):1735–43. doi: 10.4049/jimmunol.169.4.1735
35. Holodick NE, Tumang JR, Rothstein TL. Continual signaling is responsible for constitutive ERK phosphorylation in b-1a cells. Mol Immunol (2009) 46(15):3029–36. doi: 10.1016/j.molimm.2009.06.011
36. Morris DL, Rothstein TL. Abnormal transcription factor induction through the surface immunoglobulin m receptor of b-1 lymphocytes. J Exp Med (1993) 177(3):857–61. doi: 10.1084/jem.177.3.857
37. Bikah G, Carey J, Ciallella JR, Tarakhovsky A, Bondada S. CD5-mediated negative regulation of antigen receptor-induced growth signals in b-1 b cells. Science. (1996) 274(5294):1906–9. doi: 10.1126/science.274.5294.1906
38. Jellusova J, Duber S, Guckel E, Binder CJ, Weiss S, Voll R, et al. Siglec-G regulates B1 cell survival and selection. J Immunol (2010) 185(6):3277–84. doi: 10.4049/jimmunol.1001792
39. Jellusova J, Wellmann U, Amann K, Winkler TH, Nitschke L. CD22 x siglec-G double-deficient mice have massively increased B1 cell numbers and develop systemic autoimmunity. J Immunol (2010) 184(7):3618–27. doi: 10.4049/jimmunol.0902711
40. Wong SC, Chew WK, Tan JE, Melendez AJ, Francis F, Lam KP. Peritoneal CD5+ b-1 cells have signaling properties similar to tolerant b cells. J Biol Chem (2002) 277(34):30707–15. doi: 10.1074/jbc.M202460200
41. Bell SE, Goodnow CC. A selective defect in IgM antigen receptor synthesis and transport causes loss of cell surface IgM expression on tolerant b lymphocytes. EMBO J (1994) 13(4):816–26.
42. Durand CA, Hartvigsen K, Fogelstrand L, Kim S, Iritani S, Vanhaesebroeck B, et al. Phosphoinositide 3-kinase p110 delta regulates natural antibody production, marginal zone and b-1 b cell function, and autoantibody responses. J Immunol (2009) 183(9):5673–84. doi: 10.4049/jimmunol.0900432
43. Ng LG, Ng CH, Woehl B, Sutherland AP, Huo J, Xu S, et al. BAFF costimulation of toll-like receptor-activated b-1 cells. Eur J Immunol (2006) 36(7):1837–46. doi: 10.1002/eji.200635956
44. Rauch M, Tussiwand R, Bosco N, Rolink AG. Crucial role for BAFF-BAFF-R signaling in the survival and maintenance of mature b cells. PloS One (2009) 4(5):e5456. doi: 10.1371/journal.pone.0005456
45. Genestier L, Taillardet M, Mondiere P, Gheit H, Bella C, Defrance T. TLR agonists selectively promote terminal plasma cell differentiation of b cell subsets specialized in thymus-independent responses. J Immunol (2007) 178(12):7779–86. doi: 10.4049/jimmunol.178.12.7779
46. Moon BG, Takaki S, Miyake K, Takatsu K. The role of IL-5 for mature b-1 cells in homeostatic proliferation, cell survival, and ig production. J Immunol (2004) 172(10):6020–9. doi: 10.4049/jimmunol.172.10.6020
47. Hooijkaas H, Benner R, Pleasants JR, Wostmann BS. Isotypes and specificities of immunoglobulins produced by germ-free mice fed chemically defined ultrafiltered "antigen-free" diet. Eur J Immunol (1984) 14(12):1127–30. doi: 10.1002/eji.1830141212
48. Zeng Z, Surewaard BGJ, Wong CHY, Guettler C, Petri B, Burkhard R, et al. Sex-hormone-driven innate antibodies protect females and infants against EPEC infection. Nat Immunol (2018) 19(10):1100–11. doi: 10.1038/s41590-018-0211-2
49. Savage HP, Yenson VM, Sawhney SS, Mousseau BJ, Lund FE, Baumgarth N. Blimp-1-dependent and -independent natural antibody production by b-1 and b-1-derived plasma cells. J Exp Med (2017) 214(9):2777–94. doi: 10.1084/jem.20161122
50. Jackson-Jones LH, Bénézech C. Control of innate-like b cell location for compartmentalised IgM production. Curr Opin Immunol (2018) 50:9–13. doi: 10.1016/j.coi.2017.10.006
51. Jackson-Jones LH, Duncan SM, Magalhaes MS, Campbell SM, Maizels RM, McSorley HJ, et al. Fat-associated lymphoid clusters control local IgM secretion during pleural infection and lung inflammation. Nat Commun (2016) 7:12651. doi: 10.1038/ncomms12651
52. Moro K, Yamada T, Tanabe M, Takeuchi T, Ikawa T, Kawamoto H, et al. Innate production of T(H)2 cytokines by adipose tissue-associated c-Kit(+)Sca-1(+) lymphoid cells. Nature. (2010) 463(7280):540–4. doi: 10.1038/nature08636
53. Hayakawa K, Carmack CE, Hyman R, Hardy RR. Natural autoantibodies to thymocytes: origin, VH genes, fine specificities, and the role of thy-1 glycoprotein. J Exp Med (1990) 172(3):869–78. doi: 10.1084/jem.172.3.869
54. Martin F, Oliver AM, Kearney JF. Marginal zone and B1 b cells unite in the early response against T-independent blood-borne particulate antigens. Immunity (2001) 14(5):617–29. doi: 10.1016/S1074-7613(01)00129-7
55. Boes M, Esau C, Fischer MB, Schmidt T, Carroll M, Chen J. Enhanced b-1 cell development, but impaired IgG antibody responses in mice deficient in secreted IgM. J Immunol (Baltimore Md 1950). (1998) 160(10):4776–87. doi: 10.4049/jimmunol.160.10.4776
56. Boes M, Schmidt T, Linkemann K, Beaudette BC, Marshak-Rothstein A, Chen J. Accelerated development of IgG autoantibodies and autoimmune disease in the absence of secreted IgM. Proc Natl Acad Sci U S A (2000) 97(3):1184–9. doi: 10.1073/pnas.97.3.1184
57. Shaw PX, Horkko S, Chang MK, Curtiss LK, Palinski W, Silverman GJ, et al. Natural antibodies with the T15 idiotype may act in atherosclerosis, apoptotic clearance, and protective immunity. J Clin Invest (2000) 105(12):1731–40. doi: 10.1172/JCI8472
58. Meyer-Bahlburg A. B-1 cells as a source of IgA. Ann N Y Acad Sci (2015) 1362:122–31. doi: 10.1111/nyas.12801
59. Smith FL, Baumgarth N. B-1 cell responses to infections. Curr Opin Immunol (2019) 57:23–31. doi: 10.1016/j.coi.2018.12.001
60. Haro MA, Dyevoich AM, Phipps JP, Haas KM. Activation of b-1 cells promotes tumor cell killing in the peritoneal cavity. Cancer Res (2019) 79(1):159–70. doi: 10.1158/0008-5472.CAN-18-0981
61. Nguyen TT, Elsner RA, Baumgarth N. Natural IgM prevents autoimmunity by enforcing b cell central tolerance induction. J Immunol (Baltimore Md 1950). (2015) 194(4):1489–502. doi: 10.4049/jimmunol.1401880
62. Kerfoot SM, Szczepanik M, Tung JW, Askenase PW. Identification of initiator b cells, a novel subset of activation-induced deaminase-dependent b-1-like cells that mediate initiation of contact sensitivity. J Immunol (2008) 181(3):1717–27. doi: 10.4049/jimmunol.181.3.1717
63. Kulik L, Fleming SD, Moratz C, Reuter JW, Novikov A, Chen K, et al. Pathogenic natural antibodies recognizing annexin IV are required to develop intestinal ischemia-reperfusion injury. J Immunol (2009) 182(9):5363–73. doi: 10.4049/jimmunol.0803980
64. O'Garra A, Chang R, Go N, Hastings R, Haughton G, Howard M. Ly-1 b (B-1) cells are the main source of b cell-derived interleukin 10. Eur J Immunol (1992) 22(3):711–7. doi: 10.1002/eji.1830220314
65. Aziz M, Holodick NE, Rothstein TL, Wang P. B-1a cells protect mice from sepsis: Critical role of CREB. J Immunol (2017) 199(2):750–60. doi: 10.4049/jimmunol.1602056
66. Aziz M, Ode Y, Zhou M, Ochani M, Holodick NE, Rothstein TL, et al. B-1a cells protect mice from sepsis-induced acute lung injury. Mol Med (2018) 24(1):26. doi: 10.1186/s10020-018-0029-2
67. Scapini P, Lamagna C, Hu Y, Lee K, Tang Q, DeFranco AL, et al. B cell-derived IL-10 suppresses inflammatory disease in Lyn-deficient mice. Proc Natl Acad Sci U S A (2011) 108(41):E823–32. doi: 10.1073/pnas.1107913108
68. Natarajan P, Singh A, McNamara JT, Secor ER Jr., Guernsey LA, Thrall RS, et al. Regulatory b cells from hilar lymph nodes of tolerant mice in a murine model of allergic airway disease are CD5+, express TGF-β, and co-localize with CD4+Foxp3+ T cells. Mucosal Immunol (2012) 5(6):691–701. doi: 10.1038/mi.2012.42
69. Shen P, Roch T, Lampropoulou V, O'Connor RA, Stervbo U, Hilgenberg E, et al. IL-35-producing b cells are critical regulators of immunity during autoimmune and infectious diseases. Nature. (2014) 507(7492):366–70. doi: 10.1038/nature12979
70. Zhong X, Tumang JR, Gao W, Bai C, Rothstein TL. PD-L2 expression extends beyond dendritic cells/macrophages to B1 cells enriched for V(H)11/V(H)12 and phosphatidylcholine binding. Eur J Immunol (2007) 37(9):2405–10. doi: 10.1002/eji.200737461
71. Yang Y, Li X, Ma Z, Wang C, Yang Q, Byrne-Steele M, et al. CTLA-4 expression by b-1a b cells is essential for immune tolerance. Nat Commun (2021) 12(1):525. doi: 10.1038/s41467-020-20874-x
72. Lundy SK, Boros DL. Fas ligand-expressing b-1a lymphocytes mediate CD4(+)-t-cell apoptosis during schistosomal infection: induction by interleukin 4 (IL-4) and IL-10. Infect Immun. (2002) 70(2):812–9. doi: 10.1128/IAI.70.2.812-819.2002
73. Yanaba K, Bouaziz JD, Haas KM, Poe JC, Fujimoto M, Tedder TF. A regulatory b cell subset with a unique CD1dhiCD5+ phenotype controls T cell-dependent inflammatory responses. Immunity (2008) 28(5):639–50. doi: 10.1016/j.immuni.2008.03.017
74. Rauch PJ, Chudnovskiy A, Robbins CS, Weber GF, Etzrodt M, Hilgendorf I, et al. Innate response activator b cells protect against microbial sepsis. Science. (2012) 335(6068):597–601. doi: 10.1126/science.1215173
75. Weber GF, Chousterman BG, Hilgendorf I, Robbins CS, Theurl I, Gerhardt LM, et al. Pleural innate response activator b cells protect against pneumonia via a GM-CSF-IgM axis. J Exp Med (2014) 211(6):1243–56. doi: 10.1084/jem.20131471
76. Margry B, Kersemakers SC, Hoek A, Arkesteijn GJ, Wieland WH, van Eden W, et al. Activated peritoneal cavity b-1a cells possess regulatory b cell properties. PloS One (2014) 9(2):e88869. doi: 10.1371/journal.pone.0088869
77. Popi AF, Longo-Maugeri IM, Mariano M. An overview of b-1 cells as antigen-presenting cells. Front Immunol (2016) 7:138. doi: 10.3389/fimmu.2016.00138
78. Allie SR, Bradley JE, Mudunuru U, Randall TD. Identification of antigen-specific, lung resident memory b cells after influenza infection. J Immunol (2017) 198(1 Supplement):153.19–.19. doi: 10.4049/jimmunol.198.Supp.153.19
79. Baldan A, Gonen A, Choung C, Que X, Marquart TJ, Hernandez I, et al. ABCG1 is required for pulmonary b-1 b cell and natural antibody homeostasis. J Immunol (2014) 193(11):5637–48. doi: 10.4049/jimmunol.1400606
80. Stark A-K, Chandra A, Chakraborty K, Alam R, Carbonaro V, Clark J, et al. PI3Kδ hyper-activation promotes development of b cells that exacerbate streptococcus pneumoniae infection in an antibody-independent manner. Nat Commun (2018) 9(1):3174. doi: 10.1038/s41467-018-05674-8
81. Wray-Dutra MN, Al Qureshah F, Metzler G, Oukka M, James RG, Rawlings DJ. Activated PIK3CD drives innate b cell expansion yet limits b cell-intrinsic immune responses. J Exp Med (2018) 215(10):2485–96. doi: 10.1084/jem.20180617
82. Angulo I, Vadas O, Garçon F, Banham-Hall E, Plagnol V, Leahy TR, et al. Phosphoinositide 3-kinase δ gene mutation predisposes to respiratory infection and airway damage. Sci (New York NY) (2013) 342(6160):866–71. doi: 10.1126/science.1243292
83. Kroese FG, Butcher EC, Stall AM, Lalor PA, Adams S, Herzenberg LA. Many of the IgA producing plasma cells in murine gut are derived from self-replenishing precursors in the peritoneal cavity. Int Immunol (1989) 1(1):75–84. doi: 10.1093/intimm/1.1.75
84. Thurnheer MC, Zuercher AW, Cebra JJ, Bos NA. B1 cells contribute to serum IgM, but not to intestinal IgA, production in gnotobiotic ig allotype chimeric mice. J Immunol (2003) 170(9):4564–71. doi: 10.4049/jimmunol.170.9.4564
85. Roy B, Agarwal S, Brennecke AM, Krey M, Pabst O, Duber S, et al. B-1-cell subpopulations contribute differently to gut immunity. Eur J Immunol (2013) 43(8):2023–32. doi: 10.1002/eji.201243070
86. Bunker JJ, Flynn TM, Koval JC, Shaw DG, Meisel M, McDonald BD, et al. Innate and adaptive humoral responses coat distinct commensal bacteria with immunoglobulin a. Immunity (2015) 43(3):541–53. doi: 10.1016/j.immuni.2015.08.007
87. Vergani S, Muleta KG, Da Silva C, Doyle A, Kristiansen TA, Sodini S, et al. A self-sustaining layer of early-life-origin b cells drives steady-state IgA responses in the adult gut. Immunity (2022) 55(10):1829–42 e6. doi: 10.1016/j.immuni.2022.08.018
88. Wu L, Dalal R, Cao CD, Postoak JL, Yang G, Zhang Q, et al. IL-10-producing b cells are enriched in murine pericardial adipose tissues and ameliorate the outcome of acute myocardial infarction. Proc Natl Acad Sci U S A. (2019) 116(43):21673–84. doi: 10.1073/pnas.1911464116
89. Zhang H, Stolz DB, Chalasani G, Thomson AW. Hepatic b cells are readily activated by toll-like receptor-4 ligation and secrete less interleukin-10 than lymphoid tissue b cells. Clin Exp Immunol (2013) 173(3):473–9. doi: 10.1111/cei.12126
90. Millan-Pascual J, Turpin-Fenoll L, Del Saz-Saucedo P, Rueda-Medina I, Navarro-Munoz S. Psoriasis during natalizumab treatment for multiple sclerosis. J Neurol (2012) 259(12):2758–60. doi: 10.1007/s00415-012-6713-1
91. Dass S, Vital EM, Emery P. Development of psoriasis after b cell depletion with rituximab. Arthritis Rheumatol (2007) 56(8):2715–8. doi: 10.1002/art.22811
92. Guidelli GM, Fioravanti A, Rubegni P, Feci L. Induced psoriasis after rituximab therapy for rheumatoid arthritis: a case report and review of the literature. Rheumatol Int (2013) 33(11):2927–30. doi: 10.1007/s00296-012-2581-3
93. Nakashima H, Hamaguchi Y, Watanabe R, Ishiura N, Kuwano Y, Okochi H, et al. CD22 expression mediates the regulatory functions of peritoneal b-1a cells during the remission phase of contact hypersensitivity reactions. J Immunol (2010) 184(9):4637–45. doi: 10.4049/jimmunol.0901719
94. Yong L, Tang Y, Ren C, Liu M, Shen J, Hou X. B1 cells protect against schistosoma japonicum-induced liver inflammation and fibrosis by controlling monocyte infiltration. PloS Negl Trop Dis. (2019) 13(6):e0007474. doi: 10.1371/journal.pntd.0007474
95. Mizoguchi A, Mizoguchi E, Takedatsu H, Blumberg RS, Bhan AK. Chronic intestinal inflammatory condition generates IL-10-producing regulatory b cell subset characterized by CD1d upregulation. Immunity (2002) 16(2):219–30. doi: 10.1016/S1074-7613(02)00274-1
96. Yanaba K, Yoshizaki A, Asano Y, Kadono T, Tedder TF, Sato S. IL-10-producing regulatory B10 cells inhibit intestinal injury in a mouse model. Am J Pathology (2011) 178(2):735–43. doi: 10.1016/j.ajpath.2010.10.022
97. Wong SC, Puaux AL, Chittezhath M, Shalova I, Kajiji TS, Wang X, et al. Macrophage polarization to a unique phenotype driven by b cells. Eur J Immunol (2010) 40(8):2296–307. doi: 10.1002/eji.200940288
98. Liu D, Yin X, Olyha SJ, Nascimento MSL, Chen P, White T, et al. IL-10-Dependent crosstalk between murine marginal zone b cells, macrophages, and CD8alpha(+) dendritic cells promotes listeria monocytogenes infection. Immunity (2019) 51(1):64–76 e7. doi: 10.1016/j.immuni.2019.05.011
99. Herve M, Xu K, Ng YS, Wardemann H, Albesiano E, Messmer BT, et al. Unmutated and mutated chronic lymphocytic leukemias derive from self-reactive b cell precursors despite expressing different antibody reactivity. J Clin Invest (2005) 115(6):1636–43. doi: 10.1172/JCI24387
100. Hayakawa K, Formica AM, Colombo MJ, Shinton SA, Brill-Dashoff J, Morse Iii HC, et al. Loss of a chromosomal region with synteny to human 13q14 occurs in mouse chronic lymphocytic leukemia that originates from early-generated b-1 b cells. Leukemia. (2016) 30(7):1510–9. doi: 10.1038/leu.2016.61
101. Casali P, Burastero SE, Nakamura M, Inghirami G, Notkins AL. Human lymphocytes making rheumatoid factor and antibody to ssDNA belong to leu-1+ b-cell subset. Science. (1987) 236(4797):77–81. doi: 10.1126/science.3105056
102. Hardy RR, Kishimoto T, Hayakawa K. Differentiation of b cell progenitors in vitro: generation of surface IgM+ b cells, including ly-1 b cells, from thy-1- asialoGM1+ cells in newborn liver. Eur J Immunol (1987) 17(12):1769–74. doi: 10.1002/eji.1830171214
103. Yuling H, Ruijing X, Xiang J, Yanping J, Lang C, Li L, et al. CD19+CD5+ b cells in primary IgA nephropathy. J Am Soc Nephrol (2008) 19(11):2130–9. doi: 10.1681/ASN.2007121303
104. Donze HH, Lue C, Julian BA, Kutteh WH, Kantele A, Mestecky J. Human peritoneal b-1 cells and the influence of continuous ambulatory peritoneal dialysis on peritoneal and peripheral blood mononuclear cell (PBMC) composition and immunoglobulin levels. Clin Exp Immunol (1997) 109(2):356–61. doi: 10.1046/j.1365-2249.1997.4541352.x
105. Nisitani S, Murakami M, Akamizu T, Okino T, Ohmori K, Mori T, et al. Preferential localization of human CD5+ b cells in the peritoneal cavity. Scand J Immunol (1997) 46(6):541–5. doi: 10.1046/j.1365-3083.1997.d01-166.x
106. Yuan J, Nguyen CK, Liu X, Kanellopoulou C, Muljo SA. Lin28b reprograms adult bone marrow hematopoietic progenitors to mediate fetal-like lymphopoiesis. Science (2012) 335(6073):1195–200. doi: 10.1126/science.1216557
107. Lee J, Kuchen S, Fischer R, Chang S, Lipsky PE. Identification and characterization of a human CD5+ pre-naive b cell population. J Immunol (2009) 182(7):4116–26. doi: 10.4049/jimmunol.0803391
108. Freedman AS, Freeman G, Whitman J, Segil J, Daley J, Nadler LM. Studies of in vitro activated CD5+ b cells. Blood. (1989) 73(1):202–8. doi: 10.1182/blood.V73.1.202.202
109. Sims GP, Ettinger R, Shirota Y, Yarboro CH, Illei GG, Lipsky PE. Identification and characterization of circulating human transitional b cells. Blood. (2005) 105(11):4390–8. doi: 10.1182/blood-2004-11-4284
110. Hippen KL, Tze LE, Behrens TW. CD5 maintains tolerance in anergic b cells. J Exp Med (2000) 191(5):883–90. doi: 10.1084/jem.191.5.883
111. Griffin DO, Holodick NE, Rothstein TL. Human B1 cells in umbilical cord and adult peripheral blood express the novel phenotype CD20+ CD27+ CD43+ CD70. J Exp Med (2011) 208(1):67–80. doi: 10.1084/jem.20101499
112. Griffin DO, Rothstein TL. A small CD11b(+) human B1 cell subpopulation stimulates T cells and is expanded in lupus. J Exp Med (2011) 208(13):2591–8. doi: 10.1084/jem.20110978
113. Griffin DO, Rothstein TL. Human "orchestrator" CD11b(+) B1 cells spontaneously secrete interleukin-10 and regulate T-cell activity. Mol Med (2012) 18:1003–8. doi: 10.2119/molmed.2012.00203
114. Covens K, Verbinnen B, Geukens N, Meyts I, Schuit F, Van Lommel L, et al. Characterization of proposed human b-1 cells reveals pre-plasmablast phenotype. Blood (2013) 121(26):5176–83. doi: 10.1182/blood-2012-12-471953
115. Descatoire M, Weill JC, Reynaud CA, Weller S. A human equivalent of mouse b-1 cells? J Exp Med (2011) 208(13):2563–4. doi: 10.1084/jem.20112232
116. Suchanek O, Sadler R, Bateman EA, Patel SY, Ferry BL. Immunophenotyping of putative human B1 b cells in healthy controls and common variable immunodeficiency (CVID) patients. Clin Exp Immunol (2012) 170(3):333–41. doi: 10.1111/j.1365-2249.2012.04656.x
117. Nihal M, Mikkola D, Wood GS. Detection of clonally restricted immunoglobulin heavy chain gene rearrangements in normal and lesional skin: analysis of the b cell component of the skin-associated lymphoid tissue and implications for the molecular diagnosis of cutaneous b cell lymphomas. J Mol Diagn (2000) 2(1):5–10. doi: 10.1016/S1525-1578(10)60609-5
118. Asano Y, Daccache J, Jain D, Ko K, Kinloch A, Veselits M, et al. Innate-like self-reactive b cells infiltrate human renal allografts during transplant rejection. Nat Commun (2021) 12(1):4372. doi: 10.1038/s41467-021-24615-6
119. Kenna T, Golden-Mason L, Porcelli SA, Koezuka Y, Hegarty JE, O'Farrelly C, et al. NKT cells from normal and tumor-bearing human livers are phenotypically and functionally distinct from murine NKT cells. J Immunol (2003) 171(4):1775–9. doi: 10.4049/jimmunol.171.4.1775
120. Hein WR, Dudler L. TCR gamma delta+ cells are prominent in normal bovine skin and express a diverse repertoire of antigen receptors. Immunology. (1997) 91(1):58–64. doi: 10.1046/j.1365-2567.1997.00224.x
121. Zaid A, Mackay LK, Rahimpour A, Braun A, Veldhoen M, Carbone FR, et al. Persistence of skin-resident memory T cells within an epidermal niche. Proc Natl Acad Sci U S A (2014) 111(14):5307–12. doi: 10.1073/pnas.1322292111
122. Weller S, Braun MC, Tan BK, Rosenwald A, Cordier C, Conley ME, et al. Human blood IgM "memory" b cells are circulating splenic marginal zone b cells harboring a prediversified immunoglobulin repertoire. Blood. (2004) 104(12):3647–54. doi: 10.1182/blood-2004-01-0346
123. Dono M, Zupo S, Leanza N, Melioli G, Fogli M, Melagrana A, et al. Heterogeneity of tonsillar subepithelial b lymphocytes, the splenic marginal zone equivalents. J Immunol (2000) 164(11):5596–604. doi: 10.4049/jimmunol.164.11.5596
124. Spencer J, Finn T, Pulford KA, Mason DY, Isaacson PG. The human gut contains a novel population of b lymphocytes which resemble marginal zone cells. Clin Exp Immunol (1985) 62(3):607–12.
125. Tierens A, Delabie J, Michiels L, Vandenberghe P, De Wolf-Peeters C. Marginal-zone b cells in the human lymph node and spleen show somatic hypermutations and display clonal expansion. Blood (1999) 93(1):226–34. doi: 10.1182/blood.V93.1.226
Keywords: B-1 cells, innate-like B cells, tissue-residency, homeostasis, non-lymphoid organ, tissue immunity
Citation: Suchanek O and Clatworthy MR (2023) Homeostatic role of B-1 cells in tissue immunity. Front. Immunol. 14:1106294. doi: 10.3389/fimmu.2023.1106294
Received: 23 November 2022; Accepted: 27 February 2023;
Published: 08 September 2023.
Edited by:
Marilia Cascalho, University of Michigan, United StatesReviewed by:
Munir Akkaya, The Ohio State University, United StatesAmit Kumar Jaiswal, University of California, Los Angeles, United States
Copyright © 2023 Suchanek and Clatworthy. This is an open-access article distributed under the terms of the Creative Commons Attribution License (CC BY). The use, distribution or reproduction in other forums is permitted, provided the original author(s) and the copyright owner(s) are credited and that the original publication in this journal is cited, in accordance with accepted academic practice. No use, distribution or reproduction is permitted which does not comply with these terms.
*Correspondence: Ondrej Suchanek, b3MzMzRAY2FtLmFjLnVr