- 1National Engineering Research Center for Marine Aquaculture, Institute of Innovation & Application, Zhejiang Ocean University, Zhoushan, Zhejiang, China
- 2State Key Laboratory of Southwestern Chinese Medicine Resources, School of Pharmacy, Chengdu University of Traditional Chinese Medicine, Chengdu, China
- 3C-MER Dennis Lam and Partners Eye Center, Hong Kong International Eye Care Group, Hong Kong, China
- 4Research Center for Preclinical Medicine, Southwest Medical University, Luzhou, China
- 5Department of Internal Medicine Oncology, Shandong Cancer Hospital and Institute, Shandong First Medical University and Shandong Academy of Medical Sciences, Jinan, China
Immunotherapy is a type of treatment that uses our own immune system to fight cancer. Studies have shown that traditional Chinese medicine (TCM) has antitumor activity and can enhance host immunity. This article briefly describes the immunomodulatory and escape mechanisms in tumors, as well as highlights and summarizes the antitumor immunomodulatory activities of some representative active ingredients of TCM. Finally, this article puts forward some opinions on the future research and clinical application of TCM, aiming to promote the clinical applications of TCM in tumor immunotherapy and to provide new ideas for the research of tumor immunotherapy using TCM.
1 Introduction
Malignant tumors are cancerous tumors that have cells growing uncontrollably. If the condition remains untreated, malignant cells can spread to distant sites via the lymphatic system and bloodstream and become life-threatening (1). The rate of new cases of malignant tumors has been increasing over the past years, and the incidence of early-onset cancers is on the rise worldwide (2, 3). Traditional tumor treatment methods, such as radiotherapy, chemotherapy and surgery, have drawbacks of easy recurrence, large side effects and low survival rate (4). Immune homeostasis is regulated and maintained by the immune system, which is made up of immune organs, immune tissues, immune cells and immune factors. On the other hand, immune disorders can lead to the occurrence and progression of different immune diseases (5–7). Tumor immunotherapy, which specifically kills and removes tumor cells by activating the autoimmune system and enhancing autoimmunity, is considered the only possible method to completely remove tumor cells (8, 9). Therefore, tumor treatment strategies that regulate immune activity have attracted increasing attention from researchers.
Traditional Chinese medicines (TCM) are known as a renowned source of medicinal compounds. They offer advantages of low cost, structural and functional diversity, and few side effects, and has a critical role in the long history of Chinese civilization (10, 11). However, the complex components of TCM, the unclear pharmacological mechanism of action, and the slow onset of action have significantly hindered its development (12, 13). With advances in science and technology and the improvement of the public’s understanding of TCM, there has been a worldwide upsurge in the research and development of TCM. Several studies have shown that some TCM are beneficial in improving the clinical symptoms of COVID-19 (14–16).
Currently, a variety of TCMs have been reported to have antitumor activity as well as to enhance immunity and survival rate of patients. Some studies suggest that TCM offer advantages over Western medicine at a certain stage of cancer treatment (17–19). Therefore, identifying the active substances in TCM and understanding their antitumor pharmacological mechanisms are crucial for the development of TCM in the future. This article describes the main regulatory mechanisms of tumor and body immunity, summarizes the representative active ingredients of TCM that play an immunoregulatory role in tumor treatment, and discusses their pharmacological mechanisms.
2 Immune regulation in tumors
Cancer immunoediting is the process of interaction and mutual influence between tumor cells and the body’s immune system (20, 21). Cancer immune regulation consists of three stages, namely, 1) elimination phase, 2) equilibrium phase and 3) escape phase (22, 23). During the elimination phase, the mutated “non-self” cells in the body are recognized and eliminated specifically by the surveillance function of the immune system (24). However, tumor cells with more mutations can alter their own characteristics and evade immune surveillance (25). As a result, the immune system cannot completely clear tumor cells. In this stage, tumor cells cannot significantly proliferate and expand due to the immune-mediated killing and immune stress (26, 27). The inhibitory activity of the immune system and the proliferative activity of tumor cells have reached a dynamic balance (28, 29). Subsequently, due to immunosuppression, exhaustion, or tumor cells mutation, the balance between the immune response and tumor activity is disrupted, allowing the mutated tumor cells to escape the immune pressure (30). During the escape phase, these tumor cells continue to clonally proliferate, eventually forming a clinically detectable, progressively growing tumor (31). In addition, tumor growth also establishes an immunosuppressive microenvironment, which further aids tumor cell escape and proliferation (32, 33).
2.1 Mechanisms of tumor immune escape
Accumulated evidence has shown that the immune escape mechanisms of tumors mainly include the following aspects:
2.1.1 Lower immunogenicity
Tumor cells with strong immunogenicity can induce antitumor immune responses and are easily eliminated (34). On the other hand, tumor cells with weak immunogenicity can escape the immune recognition and surveillance and achieve selective proliferation (35, 36). Therefore, reducing or losing the expression of MHC class I molecules on the surface of tumor cells is one of the main reasons for their immune escape (37, 38). Losing or downregulation of MHC class I molecules could result in a failure to present intracellular antigens on cell surface, and thus a failure to activate T cells (39, 40). Consequently, immune tolerance is achieved due to the non-response of T cell immunity, giving tumor cells the ability to evade surveillance of the immune system.
2.1.2 Tumor cell antigen modulation or antigen deletion
Some tumor cells express antigens similar to those of normal cells, which cannot induce the body to generate an effective antitumor immune response (41, 42). Furthermore, when the immune system recognizes and attacks the antigens that are originally expressed by tumor cells, some tumor cells can reduce or even eliminate these antigens through antibody-induced antigen internalization and mutation of the antigen itself, thereby escaping the fate of immune killing (43).
2.1.3 Abnormal tumor cell costimulatory signaling
In addition to TCR recognition, costimulatory signals expressed by tumor cells could also cause T cells activation (44). For example, the B7 family members are important costimulatory molecules responsible for regulating T cell proliferation and cytokine production (45). Many tumor cells lack B7 or other costimulatory signaling molecules and cannot activate T cells (46). Moreover, while positive costimulatory molecules (e.g. CD80 and CD86) are rarely expressed by tumor cells, negative costimulatory signals (e.g. PD-L1) are expressed. As a result, antitumor immune responses cannot be effectively induced (47).
2.1.4 Tumor-induced exemption regions
A variety of molecules, for example collagen, can be excreted by tumor cells and serve as a physical barrier around the tumor in order to prevent the entrance of antigen-presenting cells (APC) and lymphocytes into the tumor area (48).
2.1.5 Suppression of immune response
Tumor cells actively induce the body to produce tumor-associated macrophages, myeloid suppressor cells and regulatory T cells (Tregs) to suppress the body’s immune response (49).
2.1.6 Targeting T cells
Protein factors secreted by some tumor cells, such as PD-L1 and FasL can inhibit T cell proliferation or even induce T cell apoptosis (50, 51).
2.1.7 Tumor cell-induced immunosuppression
Immunosuppressive molecules produced by tumor cells, such as IL-10, TGF-β, IDO or PD-L1, can directly suppress the immune response (52, 53). Meanwhile, tumor cells can also recruit regulatory T cells that secrete immunosuppressive cytokines (54, 55).
2.2 Tumor microenvironment
Tumor microenvironment (TME) is the living environment of tumor cells, mainly including tumor cells and various surrounding cells, such as B lymphocytes, T lymphocytes, natural killer cells (NK cells), tumor-associated macrophages (TAMs), myeloid-derived suppressor cells (MDSCs), tumor-associated neutrophils (TANs), and dendritic cells (DCs)); blood and lymphatic vessels; as well as the intercellular substance, microvessels, and bioactive molecules infiltrating into the surrounding area (56). Hypoxia, acidification, interstitial hypertension, vascular hyperpermeability, inflammatory reactivity, and immunosuppression are the main features of TME (57). TME contains a variety of immunosuppressive molecules, which can protect tumor cells from cytotoxic T lymphocytes as well as enhance the immunosuppressive function of Tregs and MDSCs (58). This in turn promotes tumor growth and development.
2.3 Immune cells in the TME
2.3.1 Regulatory T cells
Tregs are a class of mature T cell subsets with immunosuppressive activity. They regulate the body’s immune system by actively regulating the proliferation and activation of autoreactive T cells. Treg cells are one of the important factors in maintaining the immune homeostasis of the body. When the immunosuppressive function of Treg cells is weakened or declined, the functions of killer T cells and helper T cells (Th cells) are enhanced, which can cause autoimmune diseases, such as systemic lupus erythematosus, multiple sclerosis, and rheumatoid arthritis (59). However, when the immunosuppressive function of Treg cells is too strong, the functions of killer T cells and Th cells are inhibited so that the immune system cannot effectively phagocytose pathogens, thereby causing diseases, such as viral infection and tumor escape (60).
2.3.2 Helper T cells
Th cells are a major component of the immune system, and their main surface marker is CD4 (61). Antigen receptors on Th cells surface can recognize fragments of antigens presented by MHC class II molecules of APC (62). They can also assist CD8+ T cells and B cells in producing cytokines, thereby activating or regulating stromal cells, epithelial cells and innate immune cells, among others (63). Activated Th cells regulate or assist immune responses by secreting cytokines (64).
2.3.3 Cytotoxic T cells
Cytotoxic T cells (CTL or Tc cells), also known as killer T cells, are surveillance cells that can directly attack tumor cells with heteroantigens, virus-infected cells and foreign cells (65). Tc cells can induce lysis or apoptosis of target cells by secreting perforin or granzyme (66). Memory T cells are antigen-experienced cells differentiated from Tc cells that have memory for antigen-bearing target cells. Once they encounter target cells with specific invading antigens, they stimulate Tc cells to produce effector T cells to destroy infected cells or cancer cells (67).
2.3.4 B cells
B cells is a type of professional antigen-presenting cell derived from pluripotent stem cells of the bone marrow (68). Activated B cells bind to soluble antigens through their surface BCRs and present them to CD4+ T cells in the form of antigenic peptide-MHC molecule complexes (69). Activated B cells can generate a large number of cytokines and play a role in inflammatory response, immune regulation, and hematopoiesis (70). B cells infiltrating tumors can exert antitumor immunity by driving complement activation, phagocytosis and antibody-dependent cell-mediated cytotoxicity (71).
2.3.5 Macrophages
Macrophages are a type of white blood cell derived from bone marrow stem cells. They develop into monocytes and then distribute to various organs and tissues via the blood. Macrophages play an important role in engulfing and digesting pathogens and maintaining tissue homeostasis (72). TAMs infiltrating tumor tissues are highly plastic and heterogeneous. Proinflammatory cytokines, such as Toll-like receptor (TLR) agonists, can promote TAMs polarization to the M1 type, while colony-stimulating factor 1 and interleukin-4 (IL-4) induce polarization of TAMs toward the M2 type (73). The proinflammatory factors tumor necrosis factor (TNF-α), IL-6, IL-10, IL-23, nitric oxide (NO) and reactive oxygen species (ROS) secreted by M1 macrophages can significantly increase the inhibition of tumor cell proliferation. M2 macrophages secrete epidermal growth factor (EGF), matrix metalloprotein 9 (MMP-9) and the anti-inflammatory factor IL-10 to promote tumor progression (74).
2.3.6 Neutrophils
Neutrophils, the most abundant type of granulocyte that make up 40% to 70% of all white blood cells in humans, play a critical role in the innate immune system (75). Neutrophils have a variety of specific receptors (e.g. complement receptors), and can produce cytokines (e.g. IFN-γ and interleukins), chemokines, lectins, and other proteins (76). In addition, neutrophils express receptors for detection and adhesion of the endothelium and Fc receptors for opsonins (77). TNF-α and interferon-β induce neutrophils to polarize toward the antitumor N1 type with high immune activity and promote CD8+ T cell activation (78). IL-8 and TGF-β also promote the polarization of TANs to the tumor-promoting N2 type, thereby inhibiting tumor immunity and accelerating tumor progression (79).
2.3.7 Dendritic cells
CD34+ hematopoietic stem cell DCs derived from bone marrow are the most functional professional APC in the body, which can efficiently take up, process and present antigens, including surface-expressing antigen-presenting molecules (MHC class I, MHC class II and CD1 molecules), costimulatory molecules (B7-1, B7-2) and CD40 (80). In addition, DCs are the only APCs that can activate naïve T cells and play a key role in T cell activation and differentiation (81). Meanwhile, the growth factors and cytokines produced by DCs can enhance the activity of immune cells, such as NK cells and T cells, and establish a complete antitumor immune response (82).
2.3.8 Myeloid-derived suppressor cells
MDSCs, an immunosuppressive innate cell population composed of immature myeloid progenitors, is a class of cells that could not differentiate into macrophages, granulocytes and DCs (83). MDSCs can display potent immunosuppressive activity through multiple regulatory mechanisms, such as producing reactive oxygen species and nitrogen, inducing immunosuppressive cells, depleting metabolites critical for T cell function, blocking lymphocyte homing, expressing regulatory glands, expressing extracellular enzymes of glycoside metabolism, and expressing negative immune checkpoint molecules (84). Therefore, MDSCs are implicated in malignant tumors and are potential targets for tumor therapy.
2.3.9 Natural killer cells
NK cells are primarily involved in the body’s first line of defense against tumors. The molecular basis for NK cells to identify and eliminate transformed cells are based on the expression of MHC-I-specific inhibitory receptors on the NK cell surface. Tumor cells having down-modulated expression of surface MHC-I become susceptible to attack by NK cells (85). Furthermore, Fc receptors on the surface of NK cells can recognize the Fc portion of IgG antibodies and provide NK cells the ability to recognize and kill the IgG-coated tumor cells. NK cells also have natural cytotoxicity receptors (NCRs) that bind to antigens on the surface of tumor cells, thereby lysing tumor cells (86). NK cells and B cells can non-specifically kill tumor cells without antigen presentation (87). In addition, NK cells can help the response and formation of tumor-specific CD4+ and CD8+ T cells (88).
2.3.10 Mast cells
Mast cells, also known as mastocyte, are resident cell of connective tissue derived from CD34+ myeloid precursor cells. They circulate in blood and migrate to the vicinity of systemic lymphatics, blood vessels, and mucosal surfaces (such as the gastrointestinal tract and skin) and are involved in the coordination of innate and adaptive immunity (89). Mast cells have been found to have either protumor or antitumor effects, depending on the tumor type, cancer stage, mast cell activation status, the balance of tumor-promoting and antitumor effects on tumor cells, and the location of mast cells in the TME (90). Chemokines (e.g., CCL3 and CXCL8), cytokines, and other factors released by mast cells are able to recruit other immune cells (e.g. macrophages, MDSCs, neutrophils, NK cells, and DCs) into the TME and alter its activity or function (91).
3 Antitumor immunomodulatory activity of active ingredients of TCM
TCM is the crystallization of thousands of years of medical practice (92). The composition of TCM is complex. Although their active ingredients and pharmacological mechanism of action are unknown, clinical trials have shown that TCM can significantly prolong the postoperative survival time of tumor patients in the treatment and prevention of cancer recurrence and metastasis. At present, many studies have analyzed and verified the antitumor immunomodulatory activity of TCM monomers or single components, including polysaccharides, alkaloids, flavonoids, and terpenes (93–95). Due to the diversity of active ingredients in TCM and their promising pharmacological effects, representative compounds of TCM and their antitumor immunomodulatory activities are discussed in this section.
3.1 Alkaloids
Alkaloids are a class of natural nitrogen-containing alkaline compounds that possess a variety of physiological effects (96). Especially, alkaloids that contain complex nitrogen heterocyclic ring structure usually have significant biological activity and are important active ingredients in TCM. Alkaloid components in TCM are high-efficiency and low-toxicity antitumor compounds and are widely used in the medical field.
Berberine (BBR) is an alkaloid isolated from the TCM Coptis chinensis with significant antitumor activity (11, 97). Continuous DOX treatment can cause HL-60 cells to differentiate toward N2, resulting in chemoresistance. BBR can downregulate DOX-mediated expression of CD133 and CD309 in neutrophils, thereby inhibiting PD-1/PD-L1-mediated chemotherapy tolerance and immune rejection (98). Furthermore, BBR triggers PD-L1 ubiquitination and degradation through a ubiquitin (Ub)/proteasome-dependent pathway (97). Recent studies suggest that BBR also inhibits the expression of CD47, whose mediates immune escape, results in limited efficacy of rituximab and enhances phagocytosis by macrophages (99). In a melanoma model, BBR increased CD40 and MHC-II expression on macrophages, increased the number of IFNγ-producing CD4 T cells and the CTL activity. Previous studies also suggest that BBR can promote NK cell infiltration, increase the expression of inflammatory factors such as IL-6, and activate the apoptosis pathway (100). Therefore, the antitumor immunomodulatory activities of BBR mainly include targeting immune checkpoints, increasing NK cell activity, regulating the neutrophil phenotype, inducing macrophage polarization, and inhibiting the expression of inflammatory factors (Figure 1).
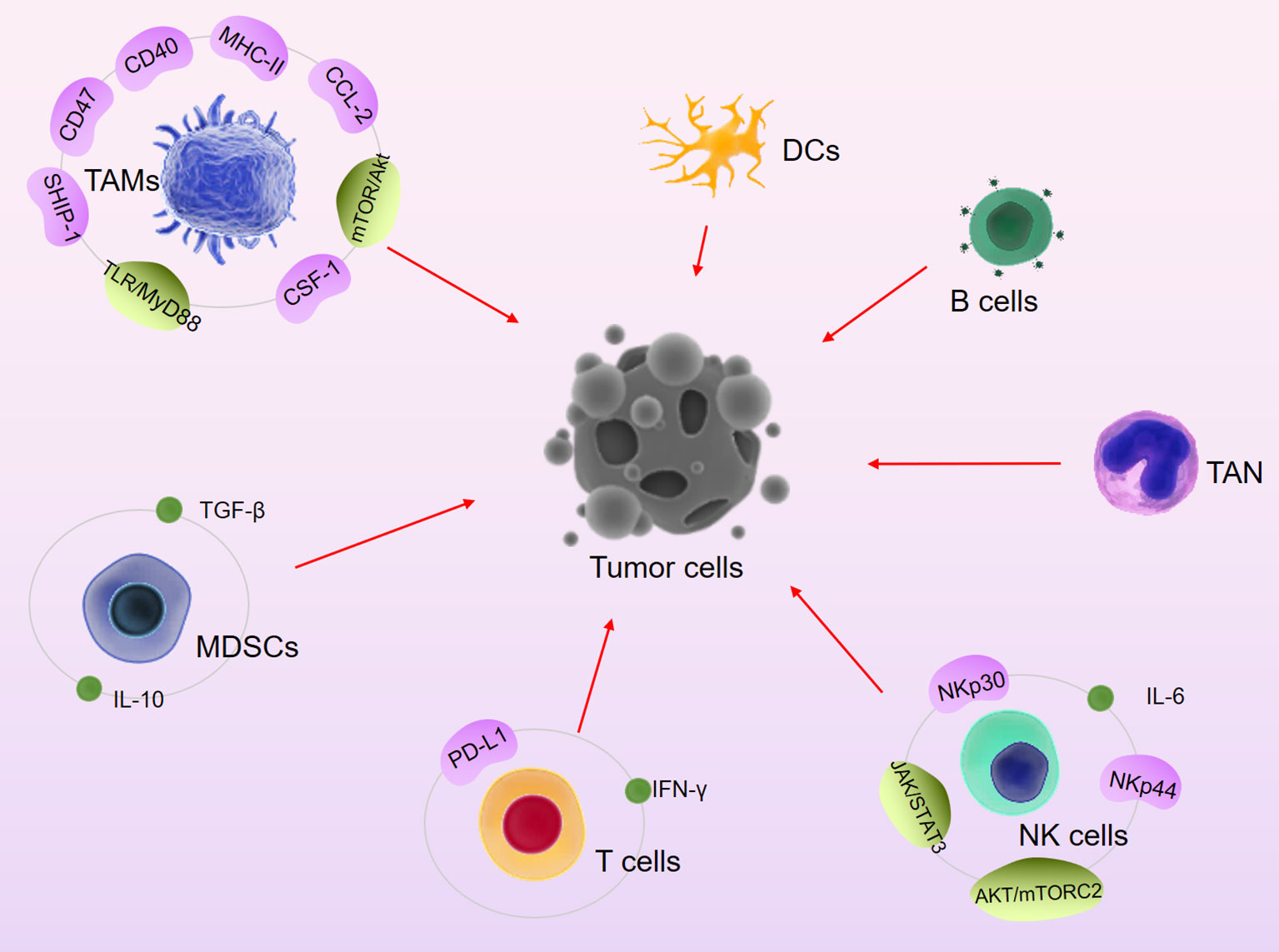
Figure 1 Major signaling pathways and immune cells involved in TCM-mediated anti-tumor immune activity.
Matrine is an alkaloid that possess various pharmacological activities and can be extracted from Sophora flavescens by organic solvents, such as ethanol. The antitumor activity of matrine includes inducing tumor cell differentiation and apoptosis, inhibiting tumor cell proliferation and metastasis, inhibiting telomerase activity, inhibiting tumor angiogenesis and inhibiting tumor drug resistance, as well as increasing the activity of white blood cells to enhance the body’s immunity.
Metastasis is responsible for nearly 90% of lung cancer deaths. Matrine inhibits lung cancer metastasis by enhancing T cell proliferation and inhibiting M2-like tumor-associated macrophage polarization (101). In leukemia treatment, matrine enhances the killing activity of NK cells on K562 cells by inhibiting IL-6-mediated JAK/STAT3 signaling in K562 cells. On the other hand, matrine significantly enhances antitumor immune activity by enhancing DC cell maturation, activation and differentiation (102). In addition, matrine promotes the secretion of inflammatory cytokines by regulating the TLR signaling pathway, which further enhances the body’s immune function (103).
3.2 Flavonoids
Flavonoids, a class of yellow pigments derived from flavonoids (2-phenylchromone), are present in a variety of fruits, vegetables, TCMs and other plants. Flavonoids possess a wide variety of biological activities, including anti-inflammatory, antioxidant, antitumor and hypoglycemic activities, as well as alleviation of vascular hyperplasia.
Epigallocatechin gallate (EGCG) is the most effective active ingredient in tea polyphenols and has been found to possess antioxidant, antiviral, antibacterial, antiarteriosclerosis, antithrombotic, antivascular proliferation, anti-inflammatory and antitumor effects. EGCG is an immune checkpoint inhibitor that can significantly downregulate the expression of PD-L1 induced by epidermal growth factor (EGF) or IFN-γ (104). Meanwhile, EGCG inhibits the JAK/STAT signaling pathway in tumor cells, activates T cells, and enhances the antitumor immune response (105). In an oral cancer study, it was found that EGCG significantly inhibited the expression of IDO by blockage of the IFN-γ-induced JAK-STAT1 signaling pathway (106). Therefore, EGCG can inhibit IDO-mediated immune escape to a certain extent or can be used as a potential targeted immunotherapy drug. In leukemia models, different doses of EGCG have different immunomodulatory activities, such as promoting natural killer cell activity, promoting phagocytosis of macrophages, and T cell proliferation (107). Furthermore, EGCG can inhibit M2 polarization and TAMs infiltration by inhibiting the expression of monocyte chemokines (CSF-1 and CCL-2) and HIF-1α, thereby inhibiting the development of breast cancer in mice (108, 109).
Apigenin, also known as chamomile, apigenol, apigenine, versulin, and others, can be found in a variety of vegetables, fruits, and plants. It has been confirmed that apigenin has biological activities, such as vasodilation; lowering blood pressure; lowering blood lipids; and acting as an anti-inflammatory, antitumor, and antioxidant factor.
After coculturing liver cancer patients-derived NK cells with apigenin, the expression levels of its activating receptors NKp44, NKp30 and NKG2D were significantly increased, while the level of TGF-β1 was decreased (110). Mechanistic studies have shown that apigenin increases the sensitivity of HCC cells to NK cells through HIF-1α. Apigenin can strongly inhibit STAT1 activation and IFN-γ-induced PD-L1 expression in tumor cells and enhance T cell-mediated killing (111, 112). In addition, apigenin inhibits the infiltration of TAMs and other leukocyte subsets in tumors, such as IL-1α, IL-6, granulocyte macrophage colony stimulating factor (GMCSF) and CCL2 (113–115). The expansion of immunosuppressive MDSCs and TAMs in the inflammatory TME results in ineffective immunotherapy for many tumors. Apigenin can enhance the proportion of tumor-killing macrophages by inducing the expression of SHIP-1, thereby enhancing the antitumor immune response (116, 117). Moreover, apigenin is also involved in maintaining T cell homeostasis in mouse pancreatic cancer (118). Other antitumor immune activities of apigenin have been reviewed by Huang et al. and are not discussed here (119).
3.3 Saponins
Saponins are naturally-ocurring glycosides with complex structures. They are widely distributed in all cells of legume plants. As an active ingredient in many TCMs, actions of saponins include anti-inflammation, inhibition of the growth of various tumor cells and improvement of the activity of immune cells.
Ginsenosides is a class of steroid glycoside present in ginseng. Ginsenoside has pharmacological activities, such as improving immunity, anti-aging, antibacterial, anti-fatigue, and antitumor properties. Ginsenosides can directly activate CD4+ T cells, promote T cells differentiation into Th cells, and enhance the immune response [30]. When tumor cells are killed by external stimuli, the process that the cells transform from a non-immunogenic to immunogenic state to mediate the body’s antitumor immune response is called immunogenic cell death (ICD). Ginsenosides can kill both immunogenic and nonimmunogenic tumor cells by enhancing DC function and inducing apoptosis (120). A recent study revealed that ginsenosides could attenuate the expression of cisplatin-inducing PD-L1 in non-small cell lung cancer cells (A549/DDP cells) and enhance the killing activity of T cells against tumor cells (121). When used in combination with other chemotherapeutic drugs, ginsenosides can enhance antitumor activity and reduce side effects. For example, cyclophosphamide (CTX) has unstable efficacy and serious side effects, and its application has been greatly limited. Wang et al. showed that ginsenosides could promote the antitumor activity of CTX by enhanacing the proliferation of intestinal probiotics and also alleviate CTX-induced intestinal mucositis by activating the Nrf2 signaling pathway (122). Furthermore, ginsenosides enhance macrophage innate immune responses by activating the LPS-induced mTOR/Akt signaling axis (123).
3.4 Terpenoids
Terpenoids are the most abundant class of compounds in natural substances and are the main components of the essences, resins, and pigments of certain plants. In addition, some animal hormones and vitamins also belong to terpenoids. Therefore, terpenoids are important compounds in TCM and are indispensable raw materials for the chemical and food industries.
Triptolide (TP) is an epoxy diterpene lactone compound that can be isolated from the roots, flowers, leaves and fruits of Tripterygium wilfordii. Tp is also a natural product with various pharmacological activities, such as anti-senile dementia, antioxidant, antitumor and antibacterial activities.
Recently, Jiang et al. discovered the mechanism by which TP remodels the TME. TP remodels the TME in the colorectum by downregulating the expression of CD206 and IL-10 and inducing macrophage polarization (124). Similar to other active ingredients of TCM, TP can inhibit the expression of PD-L1 induced by IFN-γ or chemotherapy resistance, significantly enhance the secretion of TNF-α and IL-2, and improve the NK cells activity (125–127). Chen et al. found that TP exerts tumor immunosuppressive effects by inhibiting DC maturation and trafficking (128). In addition, TP can enhance antitumor immunity by inhibiting the expression of Treg cells and IL-10 and TGFβ (129). TP was also found to significantly upregulate the cell populations of B cells (CD19), T cells (CD3), macrophages (Mac-3) and monocytes (CD11b) in leukemia cells, enhancing the phagocytosis of macrophages (130).
3.5 Phenols
Phenolic compounds are widely found in foods in the human diet as well as in plants, and their applications in human health has been widely studied.
Resveratrol, a phytoalexin produced by plants to resist external stress, is widely found in berries, peanuts, mulberries, grapes, and other fruits.
Resveratrol has been found to enhance antitumor immune activity by inhibiting PD-1 expression or blocking the PD-1 signaling pathway in various tumors (131, 132). Meanwhile, downregulated PD-1 may amplify the Th1 immune response and promote the activity of CD8+ T cells (133). Resveratrol treatment can enhance/restore the killing activity of NK cells in human and mouse whole blood and significantly inhibit growth and metastasis of tumor (134). It was suggested that resveratrol upregulates the expression of c-Myb by activation of the AKT/mTORC2 signaling pathway, thereby activating NK cells (135). In addition, resveratrol can enhance the susceptibility of breast cancer cells to NK cells through inhibiting the expression of c-Myc (136). Furthermore, resveratrol can reduce the number of CD8+CD122+ T cells and M2 TAMs by inhibiting the secretion of cytokines (IL-10 and TGF-β1) and the phosphorylation of STAT3 while inhibiting M2 macrophage polarization (137–139).
3.6 Polysaccharides
Polysaccharides are the most abundant naturally occurring macromolecular compounds, which are widely present in higher plants, algae, fungi and animals. Polysaccharides have various pharmacological activities and are widely used as clinical drugs and nutritional health care products.
Astragalus polysaccharide (APS), a type of water-soluble polysaccharide, is one of the most important natural active ingredients derived from Astragalus. In recent years, APS has attracted much attention due to their promising activities in anti-aging, regulating blood sugar levels, and immune activity.
Currently, APS is used as a synergistic immune enhancer in breast cancer treatment. Further mechanistic studies have shown that APS regulates immunity by activating multiple signaling pathways, including the activation of macrophages and the TLR4-mediated MyD88 signaling pathway (140, 141). APS can be used as an immune adjuvant in tumor chemotherapy because it can enhance lymphocyte proliferation and macrophage phagocytosis and, when combined with 5-FU, it reduces the immunosuppressive activity of 5-FU (142). Huang et al. shows that APS inhibits cytokines (IL-4, IL-6, IL-13, IL-17, IL-1β, IFN-γ and GM-CSF), and immunosuppressive agent (IL-10 and IL-12) expression, which helps in improving the quality of life of patients with advanced cancer (143). In APS-treated melanoma mice, it was found that the number of MDSCs and the expression of related factors (TGF-β, IL-10 and Arg-1) were significantly downregulated, and the activities of CD8+ T cells were significantly upregulated (144). γδT cell, which is the main subgroup of intraepithelial lymphocytes, has a critical role in maintaining intestinal mucosal homeostasis and immune regulation. APS also involves in the promotion of γδT cells proliferation and activation of γδT cells in vivo (145). Furthermore, APS can enhance the antitumor immune activity of T cells by regulating M2 macrophage polarization and promoting DC maturation (146).
4 Conclusion and Outlook
In recent years, the significance of the body’s immunomodulatory activities in tumor treatment has been evidently supported, thus promoting the advent of a variety of promising new tumor immunotherapies. While TCM are rich sources of medicinal compounds and have good biological activities, no modulators for clinical use in tumor immunotherapy have been approved. This article summarizes the immunomodulatory activity and mechanism of action of various active components of TCM in tumor therapy. The mechanisms of TCM active components in tumor immune regulation, including the promotion of T cell proliferation, enhancement of the activity of T cells and NK cells, inhibition of immune checkpoints, enhancement of the activity of tumor-associated macrophages, regulation of the polarization of tumor-associated macrophages, promotion of DC maturation, and inhibition of MDSC, are complex and diverse (Table 1).
In addition to the active compounds listed in this paper, more immunomodulatory activities of the active components of TCM have also been reported (17, 147–152). However, the targets of these active compounds and their immunomodulatory activities remain unclear. On the other hand, the active ingredients of TCM that have been discovered nowadays only represent a small portion of TCM, and more research is needed to explore other active ingredients and new action targets/mechanisdms of TCM. The constantly updated cutting-edge technologies, such as spatial transcriptome, single-cell sequencing, and nano-delivery technology, have also paved the way for the exploration of new targets for immune regulation and the clinical application of TCM (153–156).
Author contributions
CY and KW conceived and designed the whole project. CY and DL drafted the manuscript. C-NK and HW revised the manuscript. All authors contributed to the article and approved the submitted version.
Funding
The work was supported by the National Natural Science Foundation of China (82104477, U19A2010 and 81891012), National Interdisciplinary Innovation Team of Traditional Chinese Medicine (ZYYCXTD-D-202209).
Conflict of interest
The authors declare that the research was conducted in the absence of any commercial or financial relationships that could be construed as a potential conflict of interest.
Publisher’s note
All claims expressed in this article are solely those of the authors and do not necessarily represent those of their affiliated organizations, or those of the publisher, the editors and the reviewers. Any product that may be evaluated in this article, or claim that may be made by its manufacturer, is not guaranteed or endorsed by the publisher.
References
1. Zhu L, Zhao J, Guo Z, Liu Y, Chen H, Chen Z, et al. Applications of aptamer-bound nanomaterials in cancer therapy. Biosens (Basel) (2021) 11. doi: 10.3390/bios11090344
2. White B, Renzi C, Rafiq M, Abel GA, Jensen H, Lyratzopoulos G. Does changing healthcare use signal opportunities for earlier detection of cancer? a review of studies using information from electronic patient records. Cancer Epidemiol (2022) 76:102072. doi: 10.1016/j.canep.2021.102072
3. Zhu Z, Wang Q, Chen X, Wang Q, Yan C, Zhao X, et al. An enzyme-activatable aggregation-Induced-Emission probe: Intraoperative pathological fluorescent diagnosis of pancreatic cancer via specific cathepsin e. Adv Mater (2022) 34:e2107444. doi: 10.1002/adma.202107444
4. Debela DT, Muzazu SG, Heraro KD, Ndalama MT, Mesele BW, Haile DC, et al. New approaches and procedures for cancer treatment: Current perspectives. SAGE Open Med (2021) 9. doi: 10.1177/20503121211034366
5. Rankin LC, Artis D. Beyond host defense: Emerging functions of the immune system in regulating complex tissue physiology. Cell (2018) 173:554–67. doi: 10.1016/j.cell.2018.03.013
6. Zheng D, Liwinski T, Elinav E. Interaction between microbiota and immunity in health and disease. Cell Res (2020) 30:492–506. doi: 10.1038/s41422-020-0332-7
7. Tong R, Pan L, Zhang X, Li Y. Neuroendocrine-immune regulation mechanism in crustaceans: A review. Int J Mol Sci (2022) 14:378–98. doi: 10.1111/raq.12603
8. Rossin A, Miloro G, Hueber AO. TRAIL and FasL functions in cancer and autoimmune diseases: Towards an increasing complexity. Cancers (Basel) (2019) 11. doi: 10.3390/cancers11050639
9. Wong HY, Schwarz H. CD137 / CD137 ligand signalling regulates the immune balance: A potential target for novel immunotherapy of autoimmune diseases. J Autoimmun (2020) 112:102499. doi: 10.1016/j.jaut.2020.102499
10. Xu HY, Zhang YQ, Liu ZM, Chen T, Lv CY, Tang SH, et al. ETCM: an encyclopaedia of traditional Chinese medicine. Nucleic Acids Res (2019) 47:D976–d982. doi: 10.1093/nar/gky987
11. Li D, Yang C, Zhu JZ, Lopez E, Zhang T, Tong Q, et al. Berberine remodels adipose tissue to attenuate metabolic disorders by activating sirtuin 3. Acta Pharmacol Sin (2022) 43:1285–98. doi: 10.1038/s41401-021-00736-y
12. Luo YX, Wang XY, Huang YJ, Fang SH, Wu J, Zhang YB, et al. Systems pharmacology-based investigation of sanwei ganjiang prescription: related mechanisms in liver injury. Chin J Nat Med (2018) 16:756–65. doi: 10.1016/s1875-5364(18)30115-8
13. Chen Z, Yan G, Yang Y, Sun H, Zhang A, Han Y, et al. Rapid characterization of chemical constituents in naoling pian by LC-MS combined with data processing techniques. J Sep Sci (2022) 45:3431–42. doi: 10.1002/jssc.202200244
14. Zhang XR, Li TN, Ren YY, Zeng YJ, Lv HY, Wang J, et al. The important role of volatile components from a traditional Chinese medicine dayuan-yin against the COVID-19 pandemic. Front Pharmacol (2020) 11:583651. doi: 10.3389/fphar.2020.583651
15. An X, Zhang Y, Duan L, Jin D, Zhao S, Zhou R, et al. The direct evidence and mechanism of traditional Chinese medicine treatment of COVID-19. BioMed Pharmacother (2021) 137:111267. doi: 10.1016/j.biopha.2021.111267
16. Wu X, Li W, Qin Z, Xue L, Huang G, Luo Z, et al. Traditional Chinese medicine as an adjunctive therapy for mild and common COVID-19: A systematic review and network meta-analysis. Med (Baltimore) (2021) 100:e27372. doi: 10.1097/md.0000000000027372
17. Wang Y, Zhang Q, Chen Y, Liang CL, Liu H, Qiu F, et al. Antitumor effects of immunity-enhancing traditional Chinese medicine. BioMed Pharmacother (2020) 121:109570. doi: 10.1016/j.biopha.2019.109570
18. Yao CL, Zhang JQ, Li JY, Wei WL, Wu SF, Guo DA. Traditional Chinese medicine (TCM) as a source of new anticancer drugs. Nat Prod Rep (2021) 38:1618–33. doi: 10.1039/d0np00057d
19. Li Y, Li Y, Zhang J, Ji L, Li M, Sun X, et al. Current perspective of traditional Chinese medicines and active ingredients in the therapy of hepatocellular carcinoma. J Hepatocell Carcinoma (2022) 9:41–56. doi: 10.2147/jhc.S346047
20. Berezhnaya NM. Interaction between tumor and immune system: the role of tumor cell biology. Exp Oncol (2010) 32:159–66.
21. Demkow U. Neutrophil extracellular traps (NETs) in cancer invasion, evasion and metastasis. Cancers (Basel) (2021) 13. doi: 10.3390/cancers13174495
22. Chen WW, Liu W, Li Y, Wang J, Ren Y, Wang G, et al. Deciphering the immune-tumor interplay during early-stage lung cancer development via single-cell technology. Front Oncol (2021) 11:716042. doi: 10.3389/fonc.2021.716042
23. Wilczyński JR, Nowak M. Cancer immunoediting: Elimination, equilibrium, and immune escape in solid tumors. Exp Suppl (2022) 113:1–57. doi: 10.1007/978-3-030-91311-3_1
24. McComb S, Thiriot A, Akache B, Krishnan L, Stark F. Introduction to the immune system. Methods Mol Biol (2019) 2024:1–24. doi: 10.1007/978-1-4939-9597-4_1
25. Roetman JJ, Apostolova MKI, Philip M. Viral and cellular oncogenes promote immune evasion. Oncogene (2022) 41:921–9. doi: 10.1038/s41388-021-02145-1
26. Smith HA, Kang Y. The metastasis-promoting roles of tumor-associated immune cells. J Mol Med (Berl) (2013) 91:411–29. doi: 10.1007/s00109-013-1021-5
27. Jennings VA, Scott GB, Rose AMS, Scott KJ, Migneco G, Keller B, et al. Potentiating oncolytic virus-induced immune-mediated tumor cell killing using histone deacetylase inhibition. Mol Ther (2019) 27:1139–52. doi: 10.1016/j.ymthe.2019.04.008
28. Wang HF, Wang SS, Huang MC, Liang XH, Tang YJ, Tang YL. Targeting immune-mediated dormancy: A promising treatment of cancer. Front Oncol (2019) 9:498. doi: 10.3389/fonc.2019.00498
29. Zhong L, Luo N, Zhong X, Xu T, Hao P. The immunoregulatory effects of natural products on psoriasis via its action on Th17 cells versus regulatory T cells balance. Int Immunopharmacol (2022) 110:109032. doi: 10.1016/j.intimp.2022.109032
30. Liu Y, Cao X. Immunosuppressive cells in tumor immune escape and metastasis. J Mol Med (Berl) (2016) 94:509–22. doi: 10.1007/s00109-015-1376-x
31. Philip M, Schietinger A. CD8(+) T cell differentiation and dysfunction in cancer. Nat Rev Immunol (2022) 22:209–23. doi: 10.1038/s41577-021-00574-3
32. Lu C, Rong D, Zhang B, Zheng W, Wang X, Chen Z, et al. Current perspectives on the immunosuppressive tumor microenvironment in hepatocellular carcinoma: challenges and opportunities. Mol Cancer (2019) 18:130. doi: 10.1186/s12943-019-1047-6
33. Li X, Liu R, Su X, Pan Y, Han X, Shao C, et al. Harnessing tumor-associated macrophages as aids for cancer immunotherapy. Mol Cancer (2019) 18:177. doi: 10.1186/s12943-019-1102-3
34. Qi J, Jin F, Xu X, Du Y. Combination cancer immunotherapy of nanoparticle-based immunogenic cell death inducers and immune checkpoint inhibitors. Int J Nanomed (2021) 16:1435–56. doi: 10.2147/ijn.S285999
35. Yi M, Xu L, Jiao Y, Luo S, Li A, Wu K. The role of cancer-derived microRNAs in cancer immune escape. J Hematol Oncol (2020) 13:25. doi: 10.1186/s13045-020-00848-8
36. Costoya JA, Arce VM. Cancer cells escape the immune system by increasing stemness through epigenetic reprogramming. Cell Mol Immunol (2022) 20:6–7. doi: 10.1038/s41423-022-00953-3
37. Morrison BJ, Steel JC, Morris JC. Reduction of MHC-I expression limits T-lymphocyte-mediated killing of cancer-initiating cells. BMC Cancer (2018) 18:469. doi: 10.1186/s12885-018-4389-3
38. Dhatchinamoorthy K, Colbert JD, Rock KL. Cancer immune evasion through loss of MHC class I antigen presentation. Front Immunol (2021) 12:636568. doi: 10.3389/fimmu.2021.636568
39. Gabor F, Jahn G, Sedmak DD, Sinzger C. In vivo downregulation of MHC class I molecules by HCMV occurs during all phases of viral replication but is not always complete. Front Cell Infect Microbiol (2020) 10:283. doi: 10.3389/fcimb.2020.00283
40. Kumagai S, Koyama S, Nishikawa H. Antitumour immunity regulated by aberrant ERBB family signalling. Nat Rev Cancer (2021) 21:181–97. doi: 10.1038/s41568-020-00322-0
41. Ebrahimi N, Akbari M, Ghanaatian M, Roozbahani Moghaddam P, Adelian S, Borjian Boroujeni M, et al. Development of neoantigens: from identification in cancer cells to application in cancer vaccines. Expert Rev Vaccines (2022) 21:941–55. doi: 10.1080/14760584.2021.1951246
42. Li Z, Mo F, Wang Y, Li W, Chen Y, Liu J, et al. Enhancing gasdermin-induced tumor pyroptosis through preventing ESCRT-dependent cell membrane repair augments antitumor immune response. Nat Commun (2022) 13:6321. doi: 10.1038/s41467-022-34036-8
43. Meeusen E, Lim E, Mathivanan S. Secreted tumor antigens - immune biomarkers for diagnosis and therapy. Proteomics (2017) 17:23–24. doi: 10.1002/pmic.201600442
44. Wu L, Wei Q, Brzostek J, Gascoigne NRJ. Signaling from T cell receptors (TCRs) and chimeric antigen receptors (CARs) on T cells. Cell Mol Immunol (2020) 17:600–12. doi: 10.1038/s41423-020-0470-3
45. Harris NL, Ronchese F. The role of B7 costimulation in T-cell immunity. Immunol Cell Biol (1999) 77:304–11. doi: 10.1046/j.1440-1711.1999.00835.x
46. Liao P, Wang H, Tang YL, Tang YJ, Liang XH. The common costimulatory and coinhibitory signaling molecules in head and neck squamous cell carcinoma. Front Immunol (2019) 10:2457. doi: 10.3389/fimmu.2019.02457
47. Carenza C, Calcaterra F, Oriolo F, Di Vito C, Ubezio M, Della Porta MG, et al. Costimulatory molecules and immune checkpoints are differentially expressed on different subsets of dendritic cells. Front Immunol (2019) 10:1325. doi: 10.3389/fimmu.2019.01325
48. Accolla RS, Ramia E, Tedeschi A, Forlani G. CIITA-driven MHC class II expressing tumor cells as antigen presenting cell performers: Toward the construction of an optimal anti-tumor vaccine. Front Immunol (2019) 10:1806. doi: 10.3389/fimmu.2019.01806
49. Yang Y, Li C, Liu T, Dai X, Bazhin AV. Myeloid-derived suppressor cells in tumors: From mechanisms to antigen specificity and microenvironmental regulation. Front Immunol (2020) 11:1371. doi: 10.3389/fimmu.2020.01371
50. Zhu J, Petit PF, Van Den Eynde BJ. Apoptosis of tumor-infiltrating T lymphocytes: a new immune checkpoint mechanism. Cancer Immunol Immunother (2019) 68:835–47. doi: 10.1007/s00262-018-2269-y
51. Kang M, Hong J, Jung M, Kwon SP, Song SY, Kim HY, et al. T-Cell-Mimicking nanoparticles for cancer immunotherapy. Adv Mater (2020) 32:e2003368. doi: 10.1002/adma.202003368
52. You L, Wu W, Wang X, Fang L, Adam V, Nepovimova E, et al. The role of hypoxia-inducible factor 1 in tumor immune evasion. Med Res Rev (2021) 41:1622–43. doi: 10.1002/med.21771
53. Abd El-Fattah EE, Selim HM. Reprograming immune microenvironment modulates CD47 cancer stem cells in hepatocellular carcinoma. Int Immunopharmacol (2022) 113:109475. doi: 10.1016/j.intimp.2022.109475
54. Wang YA, Li XL, Mo YZ, Fan CM, Tang L, Xiong F, et al. Effects of tumor metabolic microenvironment on regulatory T cells. Mol Cancer (2018) 17:168. doi: 10.1186/s12943-018-0913-y
55. Togashi Y, Shitara K, Nishikawa H. Regulatory T cells in cancer immunosuppression - implications for anticancer therapy. Nat Rev Clin Oncol (2019) 16:356–71. doi: 10.1038/s41571-019-0175-7
56. Menter T, Tzankov A, Dirnhofer S. The tumor microenvironment of lymphomas: Insights into the potential role and modes of actions of checkpoint inhibitors. Hematol Oncol (2021) 39:3–10. doi: 10.1002/hon.2821
57. Vito A, El-Sayes N, Mossman K. Hypoxia-driven immune escape in the tumor microenvironment. Cells (2020) 9. doi: 10.3390/cells9040992
58. Li K, Shi H, Zhang B, Ou X, Ma Q, Chen Y, et al. Myeloid-derived suppressor cells as immunosuppressive regulators and therapeutic targets in cancer. Signal Transduct Target Ther (2021) 6:362. doi: 10.1038/s41392-021-00670-9
59. Shevyrev D, Tereshchenko V. Treg heterogeneity, function, and homeostasis. Front Immunol (2019) 10:3100. doi: 10.3389/fimmu.2019.03100
60. Li C, Jiang P, Wei S, Xu X, Wang J. Regulatory T cells in tumor microenvironment: new mechanisms, potential therapeutic strategies and future prospects. Mol Cancer (2020) 19:116. doi: 10.1186/s12943-020-01234-1
61. Zhu X, Zhu J. CD4 T helper cell subsets and related human immunological disorders. Int J Mol Sci (2020) 21. doi: 10.3390/ijms21218011
62. Rossjohn J, Gras S, Miles JJ, Turner SJ, Godfrey DI, Mccluskey J. T Cell antigen receptor recognition of antigen-presenting molecules. Annu Rev Immunol (2015) 33:169–200. doi: 10.1146/annurev-immunol-032414-112334
63. Volarić I, Vičić M, Prpić-Massari L. The role of CD8+ T-cells and their cytokines in the pathogenesis of psoriasis. Acta Dermatovenerol Croat (2019) 27:159–62.
64. Dong C. Cytokine regulation and function in T cells. Annu Rev Immunol (2021) 39:51–76. doi: 10.1146/annurev-immunol-061020-053702
65. Martínez-Lostao L, Anel A, Pardo J. How do cytotoxic lymphocytes kill cancer cells? Clin Cancer Res (2015) 21:5047–56. doi: 10.1158/1078-0432.Ccr-15-0685
66. Govendir MA, Kempe D, Sianati S, Cremasco J, Mazalo JK, Colakoglu F, et al. T Cell cytoskeletal forces shape synapse topography for targeted lysis via membrane curvature bias of perforin. Dev Cell (2022) 57:2237–2247.e2238. doi: 10.1016/j.devcel.2022.08.012
67. Oh DY, Fong L. Cytotoxic CD4(+) T cells in cancer: Expanding the immune effector toolbox. Immunity (2021) 54:2701–11. doi: 10.1016/j.immuni.2021.11.015
68. Mashima H, Zhang R, Kobayashi T, Tsukamoto H, Liu T, Iwama T, et al. Improved safety of induced pluripotent stem cell-derived antigen-presenting cell-based cancer immunotherapy. Mol Ther Methods Clin Dev (2021) 21:171–9. doi: 10.1016/j.omtm.2021.03.002
69. Ghosh D, Jiang W, Mukhopadhyay D, Mellins ED. New insights into b cells as antigen presenting cells. Curr Opin Immunol (2021) 70:129–37. doi: 10.1016/j.coi.2021.06.003
70. Chekol Abebe E, Asmamaw Dejenie T, Mengie Ayele T, Dagnew Baye N, Agegnehu Teshome A, Tilahun Muche Z. The role of regulatory b cells in health and diseases: A systemic review. J Inflammation Res (2021) 14:75–84. doi: 10.2147/jir.S286426
71. Pan Y, Yu Y, Wang X, Zhang T. Tumor-associated macrophages in tumor immunity. Front Immunol (2020) 11:583084. doi: 10.3389/fimmu.2020.583084
72. Xu R, Zhang F, Chai R, Zhou W, Hu M, Liu B, et al. Exosomes derived from pro-inflammatory bone marrow-derived mesenchymal stem cells reduce inflammation and myocardial injury via mediating macrophage polarization. J Cell Mol Med (2019) 23:7617–31. doi: 10.1111/jcmm.14635
73. Zhu S, Yi M, Wu Y, Dong B, Wu K. Roles of tumor-associated macrophages in tumor progression: implications on therapeutic strategies. Exp Hematol Oncol (2021) 10:60. doi: 10.1186/s40164-021-00252-z
74. Lin CY, Chen WL, Huang YC, Lim CL, Yang CH. Gum Arabic in combination with IFN-γ promotes the M1 polarization in macrophage. Int J Biol Macromol (2022) 209:506–12. doi: 10.1016/j.ijbiomac.2022.04.024
75. Ronchetti L, Boubaker NS, Barba M, Vici P, Gurtner A, Piaggio G. Neutrophil extracellular traps in cancer: not only catching microbes. J Exp Clin Cancer Res (2021) 40:231. doi: 10.1186/s13046-021-02036-z
76. Metzemaekers M, Gouwy M, Proost P. Neutrophil chemoattractant receptors in health and disease: double-edged swords. Cell Mol Immunol (2020) 17:433–50. doi: 10.1038/s41423-020-0412-0
77. Lőrincz Á M, Bartos B, Szombath D, Szeifert V, Timár CI, Turiák L, et al. Role of mac-1 integrin in generation of extracellular vesicles with antibacterial capacity from neutrophilic granulocytes. J Extracell Vesicles (2020) 9:1698889. doi: 10.1080/20013078.2019.1698889
78. Wang C, Fiering SN, Steinmetz NF. Cowpea mosaic virus promotes anti-tumor activity and immune memory in a mouse ovarian tumor model. Adv Ther (Weinh) (2019) 2. doi: 10.1002/adtp.201900003
79. Xue VW, Chung JY, Córdoba CAG, Cheung AH, Kang W, Lam EW, et al. Transforming growth factor-β: A multifunctional regulator of cancer immunity. Cancers (Basel) (2020) 12:1–18. doi: 10.3390/cancers12113099
80. Sonoda Y. Human CD34-negative hematopoietic stem cells: The current understanding of their biological nature. Exp Hematol (2021) 96:13–26. doi: 10.1016/j.exphem.2021.02.004
81. Hua Z, Hou B. The role of b cell antigen presentation in the initiation of CD4+ T cell response. Immunol Rev (2020) 296:24–35. doi: 10.1111/imr.12859
82. Mao X, Xu J, Wang W, Liang C, Hua J, Liu J, et al. Crosstalk between cancer-associated fibroblasts and immune cells in the tumor microenvironment: new findings and future perspectives. Mol Cancer (2021) 20:131. doi: 10.1186/s12943-021-01428-1
83. Wang Y, Johnson KCC, Gatti-Mays ME, Li Z. Emerging strategies in targeting tumor-resident myeloid cells for cancer immunotherapy. J Hematol Oncol (2022) 15:118. doi: 10.1186/s13045-022-01335-y
84. Groth C, Hu X, Weber R, Fleming V, Altevogt P, Utikal J, et al. Immunosuppression mediated by myeloid-derived suppressor cells (MDSCs) during tumour progression. Br J Cancer (2019) 120:16–25. doi: 10.1038/s41416-018-0333-1
85. Burster T, Gärtner F, Bulach C, Zhanapiya A, Gihring A, Knippschild U. Regulation of MHC I molecules in glioblastoma cells and the sensitizing of NK cells. Pharm (Basel) (2021) 14. doi: 10.3390/ph14030236
86. Dixon KJ, Wu J, Walcheck B. Engineering anti-tumor monoclonal antibodies and fc receptors to enhance ADCC by human NK cells. Cancers (Basel) (2021) 13. doi: 10.3390/cancers13020312
87. Ahmad G, Amiji MM. Chapter eight - cellular therapeutics in immuno-oncology. In: Amiji MM, Milane LS, editors. Cancer immunology and immunotherapy. Academic Press (2022). p. 237–65.
88. Kim H, Kim J, Sa JK, Ryu BK, Park KJ, Kim J, et al. Calcipotriol, a synthetic vitamin d analog, promotes antitumor immunity via CD4+T-dependent CTL/NK cell activation. BioMed Pharmacother (2022) 154:113553. doi: 10.1016/j.biopha.2022.113553
89. Elieh Ali Komi D, Wöhrl S, Bielory L. Mast cell biology at molecular level: a comprehensive review. Clin Rev Allergy Immunol (2020) 58:342–65. doi: 10.1007/s12016-019-08769-2
90. Lichterman JN, Reddy SM. Mast cells: A new frontier for cancer immunotherapy. Cells (2021) 10. doi: 10.3390/cells10061270
91. Zalpoor H, Aziziyan F, Liaghat M, Bakhtiyari M, Akbari A, Nabi-Afjadi M, et al. The roles of metabolic profiles and intracellular signaling pathways of tumor microenvironment cells in angiogenesis of solid tumors. Cell Commun Signal (2022) 20:186. doi: 10.1186/s12964-022-00951-y
92. Zhang Y, Xie H, He ZM, Zhang F, Li LL, Wang N, et al. Medical therapy of hearing impairment and tinnitus with Chinese medicine: An overview. Chin J Integr Med (2022). doi: 10.1007/s11655-022-3678-5
93. Ali Reza ASM, Nasrin MS, Hossen MA, Rahman MA, Jantan I, Haque MA, et al. Mechanistic insight into immunomodulatory effects of food-functioned plant secondary metabolites. Crit Rev Food Sci Nutr (2021), 1–31. doi: 10.1080/10408398.2021.2021138
94. Liang L, Li C, Wang Y, Yue Y, Zhang H, Yang M, et al. Physalis alkekengi l. var. franchetii (Mast.) makino: A review of the pharmacognosy, chemical constituents, pharmacological effects, quality control, and applications. Phytomedicine (2022) 105:154328. doi: 10.1016/j.phymed.2022.154328
95. Qian H, Wang L, Li Y, Wang B, Li C, Fang L, et al. The traditional uses, phytochemistry and pharmacology of abrus precatorius l.: A comprehensive review. J Ethnopharmacol (2022) 296:115463. doi: 10.1016/j.jep.2022.115463
96. Ferreira MU. Alkaloids in future drug discovery. Molecules (2022) 27. doi: 10.3390/molecules27041347
97. Liu Y, Liu X, Zhang N, Yin M, Dong J, Zeng Q, et al. Berberine diminishes cancer cell PD-L1 expression and facilitates antitumor immunity via inhibiting the deubiquitination activity of CSN5. Acta Pharm Sin B (2020) 10:2299–312. doi: 10.1016/j.apsb.2020.06.014
98. Zhang S, Zhou L, Zhang M, Wang Y, Wang M, Du J, et al. Berberine maintains the neutrophil N1 phenotype to reverse cancer cell resistance to doxorubicin. Front Pharmacol (2019) 10:1658. doi: 10.3389/fphar.2019.01658
99. Shah D, Challagundla N, Dave V, Patidar A, Saha B, Nivsarkar M, et al. Berberine mediates tumor cell death by skewing tumor-associated immunosuppressive macrophages to inflammatory macrophages. Phytomedicine (2021) 99:153904. doi: 10.1016/j.phymed.2021.153904
100. Ma W, Zhang Y, Yu M, Wang B, Xu S, Zhang J, et al. In-vitro and in-vivo anti-breast cancer activity of synergistic effect of berberine and exercise through promoting the apoptosis and immunomodulatory effects. Int Immunopharmacol (2020) 87:106787. doi: 10.1016/j.intimp.2020.106787
101. Zhao B, Hui X, Wang J, Zeng H, Yan Y, Hu Q, et al. Matrine suppresses lung cancer metastasis via targeting M2-like tumour-associated-macrophages polarization. Am J Cancer Res (2021) 11:4308–28.
102. Zhou N, Li S, Zhang F, Chen C, Li Y. Matrine combined with mammalian target of rapamycin inhibitor enhances anti-tumor efficacy of dendritic cell vaccines in hepatocellular carcinoma. Bioengineered (2022) 13:9274–83. doi: 10.1080/21655979.2022.2037855
103. Wang JK, Zhao BS, Wang M, Liu CY, Li YQ, Ma QT, et al. Anti-tumor and phenotypic regulation effect of matrine on dendritic cells through regulating TLRs pathway. Chin J Integr Med (2021) 27:520–6. doi: 10.1007/s11655-020-3433-8
104. Rawangkan A, Wongsirisin P, Namiki K, Iida K, Kobayashi Y, Shimizu Y, et al. Green tea catechin is an alternative immune checkpoint inhibitor that inhibits PD-L1 expression and lung tumor growth. Molecules (2018) 23. doi: 10.3390/molecules23082071
105. Ravindran Menon D, Li Y, Yamauchi T, Osborne DG, Vaddi PK, Wempe MF, et al. EGCG inhibits tumor growth in melanoma by targeting JAK-STAT signaling and its downstream PD-L1/PD-L2-PD1 axis in tumors and enhancing cytotoxic T-cell responses. Pharm (Basel) (2021) 14. doi: 10.3390/ph14111081
106. Cheng CW, Shieh PC, Lin YC, Chen YJ, Lin YH, Kuo DH, et al. Indoleamine 2,3-dioxygenase, an immunomodulatory protein, is suppressed by (-)-epigallocatechin-3-gallate via blocking of gamma-interferon-induced JAK-PKC-delta-STAT1 signaling in human oral cancer cells. J Agric Food Chem (2010) 58:887–94. doi: 10.1021/jf903377e
107. Huang AC, Cheng HY, Lin TS, Chen WH, Lin JH, Lin JJ, et al. Epigallocatechin gallate (EGCG), influences a murine WEHI-3 leukemia model in vivo through enhancing phagocytosis of macrophages and populations of T- and b-cells. In Vivo (2013) 27:627–34.
108. Jang JY, Lee JK, Jeon YK, Kim CW. Exosome derived from epigallocatechin gallate treated breast cancer cells suppresses tumor growth by inhibiting tumor-associated macrophage infiltration and M2 polarization. BMC Cancer (2013) 13:421. doi: 10.1186/1471-2407-13-421
109. Yang N, Li X. Epigallocatechin gallate relieves asthmatic symptoms in mice by suppressing HIF-1α/VEGFA-mediated M2 skewing of macrophages. Biochem Pharmacol (2022) 202:115112. doi: 10.1016/j.bcp.2022.115112
110. Lee HH, Cho H. Apigenin increases natural killer cytotoxicity to human hepatocellular carcinoma expressing HIF-1α through high interaction of CD95/CD95L. J Microbiol Biotechnol (2022) 32:397–404. doi: 10.4014/jmb.2201.01010
111. Coombs MRP, Harrison ME, Hoskin DW. Apigenin inhibits the inducible expression of programmed death ligand 1 by human and mouse mammary carcinoma cells. Cancer Lett (2016) 380:424–33. doi: 10.1016/j.canlet.2016.06.023
112. Xu L, Zhang Y, Tian K, Chen X, Zhang R, Mu X, et al. Apigenin suppresses PD-L1 expression in melanoma and host dendritic cells to elicit synergistic therapeutic effects. J Exp Clin Cancer Res (2018) 37:261. doi: 10.1186/s13046-018-0929-6
113. Bauer D, Redmon N, Mazzio E, Soliman KF. Apigenin inhibits TNFα/IL-1α-induced CCL2 release through IKBK-epsilon signaling in MDA-MB-231 human breast cancer cells. PloS One (2017) 12:e0175558. doi: 10.1371/journal.pone.0175558
114. Bauer D, Mazzio E, Soliman KFA. Whole transcriptomic analysis of apigenin on TNFα immuno-activated MDA-MB-231 breast cancer cells. Cancer Genomics Proteomics (2019) 16:421–31. doi: 10.21873/cgp.20146
115. Bauer D, Mazzio E, Hilliard A, Oriaku ET, Soliman KFA. Effect of apigenin on whole transcriptome profile of TNFα-activated MDA-MB-468 triple negative breast cancer cells. Oncol Lett (2020) 19:2123–32. doi: 10.3892/ol.2020.11327
116. Villalobos-Ayala K, Ortiz Rivera I, Alvarez C, Husain K, Deloach D, Krystal G, et al. Apigenin increases SHIP-1 expression, promotes tumoricidal macrophages and anti-tumor immune responses in murine pancreatic cancer. Cancers (Basel) (2020) 12. doi: 10.3390/cancers12123631
117. Husain K, Villalobos-Ayala K, Laverde V, Vazquez OA, Miller B, Kazim S, et al. Apigenin targets MicroRNA-155, enhances SHIP-1 expression, and augments anti-tumor responses in pancreatic cancer. Cancers (Basel) (2022) 14. doi: 10.3390/cancers14153613
118. Nelson N, Szekeres K, Iclozan C, Rivera IO, Mcgill A, Johnson G, et al. Apigenin: Selective CK2 inhibitor increases ikaros expression and improves T cell homeostasis and function in murine pancreatic cancer. PloS One (2017) 12:e0170197. doi: 10.1371/journal.pone.0170197
119. Huang J, Chen X, Chang Z, Xiao C, Najafi M. Boosting anti-tumour immunity using adjuvant apigenin. Anticancer Agents Med Chem (2022). doi: 10.2174/1871520622666220523151409
120. Son KJ, Choi KR, Lee SJ, Lee H. Immunogenic cell death induced by ginsenoside Rg3: Significance in dendritic cell-based anti-tumor immunotherapy. Immune Netw (2016) 16:75–84. doi: 10.4110/in.2016.16.1.75
121. Jiang Z, Yang Y, Yang Y, Zhang Y, Yue Z, Pan Z, et al. Ginsenoside Rg3 attenuates cisplatin resistance in lung cancer by downregulating PD-L1 and resuming immune. BioMed Pharmacother (2017) 96:378–83. doi: 10.1016/j.biopha.2017.09.129
122. Zhu H, He YS, Ma J, Zhou J, Kong M, Wu CY, et al. The dual roles of ginsenosides in improving the anti-tumor efficiency of cyclophosphamide in mammary carcinoma mice. J Ethnopharmacol (2021) 265:113271. doi: 10.1016/j.jep.2020.113271
123. Wang Y, Liu Y, Zhang XY, Xu LH, Ouyang DY, Liu KP, et al. Ginsenoside Rg1 regulates innate immune responses in macrophages through differentially modulating the NF-κB and PI3K/Akt/mTOR pathways. Int Immunopharmacol (2014) 23:77–84. doi: 10.1016/j.intimp.2014.07.028
124. Jiang X, Cao G, Gao G, Wang W, Zhao J, Gao C. Triptolide decreases tumor-associated macrophages infiltration and M2 polarization to remodel colon cancer immune microenvironment via inhibiting tumor-derived CXCL12. J Cell Physiol (2021) 236:193–204. doi: 10.1002/jcp.29833
125. Liang M, Fu J. Triptolide inhibits interferon-gamma-induced programmed death-1-ligand 1 surface expression in breast cancer cells. Cancer Lett (2008) 270:337–41. doi: 10.1016/j.canlet.2008.05.025
126. Hu H, Huang G, Wang H, Li X, Wang X, Feng Y, et al. Inhibition effect of triptolide on human epithelial ovarian cancer via adjusting cellular immunity and angiogenesis. Oncol Rep (2018) 39:1191–6. doi: 10.3892/or.2017.6158
127. Kuo CS, Yang CY, Lin CK, Lin GJ, Sytwu HK, Chen YW. Triptolide suppresses oral cancer cell PD-L1 expression in the interferon-γ-modulated microenvironment in vitro, in vivo, and in clinical patients. BioMed Pharmacother (2021) 133:111057. doi: 10.1016/j.biopha.2020.111057
128. Chen X, Murakami T, Oppenheim JJ, Howard OM. Triptolide, a constituent of immunosuppressive Chinese herbal medicine, is a potent suppressor of dendritic-cell maturation and trafficking. Blood (2005) 106:2409–16. doi: 10.1182/blood-2005-03-0854
129. Liu B, Zhang H, Li J, Lu C, Chen G, Zhang G, et al. Triptolide downregulates treg cells and the level of IL-10, TGF-β, and VEGF in melanoma-bearing mice. Planta Med (2013) 79:1401–7. doi: 10.1055/s-0033-1350708
130. Chan SF, Chen YY, Lin JJ, Liao CL, Ko YC, Tang NY, et al. Triptolide induced cell death through apoptosis and autophagy in murine leukemia WEHI-3 cells in vitro and promoting immune responses in WEHI-3 generated leukemia mice in vivo. Environ Toxicol (2017) 32:550–68. doi: 10.1002/tox.22259
131. Yang M, Li Z, Tao J, Hu H, Li Z, Zhang Z, et al. Resveratrol induces PD-L1 expression through snail-driven activation of wnt pathway in lung cancer cells. J Cancer Res Clin Oncol (2021) 147:1101–13. doi: 10.1007/s00432-021-03510-z
132. Chen J, Huang ST, Chen JG, He JH, Lin WM, Huang ZH, et al. Resveratrol reduces lactate production and modifies the ovarian cancer immune microenvironment. Neoplasma (2022) 69:1129–37. doi: 10.4149/neo_2022_220414N410
133. Han X, Zhao N, Zhu W, Wang J, Liu B, Teng Y. Resveratrol attenuates TNBC lung metastasis by down-regulating PD-1 expression on pulmonary T cells and converting macrophages to M1 phenotype in a murine tumor model. Cell Immunol (2021) 368:104423. doi: 10.1016/j.cellimm.2021.104423
134. Lee Y, Shin H, Kim J. In vivo anti-cancer effects of resveratrol mediated by NK cell activation. J Innate Immun (2021) 13:94–106. doi: 10.1159/000510315
135. Lee YJ, Kim J. Resveratrol activates natural killer cells through akt- and mTORC2-mediated c-myb upregulation. Int J Mol Sci (2020) 21. doi: 10.3390/ijms21249575
136. Pan J, Shen J, Si W, Du C, Chen D, Xu L, et al. Resveratrol promotes MICA/B expression and natural killer cell lysis of breast cancer cells by suppressing c-Myc/miR-17 pathway. Oncotarget (2017) 8:65743–58. doi: 10.18632/oncotarget.19445
137. Kimura Y, Sumiyoshi M. Resveratrol prevents tumor growth and metastasis by inhibiting lymphangiogenesis and M2 macrophage activation and differentiation in tumor-associated macrophages. Nutr Cancer (2016) 68:667–78. doi: 10.1080/01635581.2016.1158295
138. Sun L, Chen B, Jiang R, Li J, Wang B. Resveratrol inhibits lung cancer growth by suppressing M2-like polarization of tumor associated macrophages. Cell Immunol (2017) 311:86–93. doi: 10.1016/j.cellimm.2016.11.002
139. Davoodvandi A, Darvish M, Borran S, Nejati M, Mazaheri S, Reza Tamtaji O, et al. The therapeutic potential of resveratrol in a mouse model of melanoma lung metastasis. Int Immunopharmacol (2020) 88:106905. doi: 10.1016/j.intimp.2020.106905
140. Zhou L, Liu Z, Wang Z, Yu S, Long T, Zhou X, et al. Astragalus polysaccharides exerts immunomodulatory effects via TLR4-mediated MyD88-dependent signaling pathway in vitro and in vivo. Sci Rep (2017) 7:44822. doi: 10.1038/srep44822
141. Li W, Song K, Wang S, Zhang C, Zhuang M, Wang Y, et al. Anti-tumor potential of astragalus polysaccharides on breast cancer cell line mediated by macrophage activation. Mater Sci Eng C Mater Biol Appl (2019) 98:685–95. doi: 10.1016/j.msec.2019.01.025
142. Li W, Hu X, Wang S, Jiao Z, Sun T, Liu T, et al. Characterization and anti-tumor bioactivity of astragalus polysaccharides by immunomodulation. Int J Biol Macromol (2020) 145:985–97. doi: 10.1016/j.ijbiomac.2019.09.189
143. Huang WC, Kuo KT, Bamodu OA, Lin YK, Wang CH, Lee KY, et al. Astragalus polysaccharide (PG2) ameliorates cancer symptom clusters, as well as improves quality of life in patients with metastatic disease, through modulation of the inflammatory cascade. Cancers (Basel) (2019) 11. doi: 10.3390/cancers11081054
144. Ding G, Gong Q, Ma J, Liu X, Wang Y, Cheng X. Immunosuppressive activity is attenuated by astragalus polysaccharides through remodeling the gut microenvironment in melanoma mice. Cancer Sci (2021) 112:4050–63. doi: 10.1111/cas.15078
145. Sun S, Zheng K, Zhao H, Lu C, Liu B, Yu C, et al. Regulatory effect of astragalus polysaccharides on intestinal intraepithelial γδT cells of tumor bearing mice. Molecules (2014) 19:15224–36. doi: 10.3390/molecules190915224
146. Bamodu OA, Kuo KT, Wang CH, Huang WC, Wu ATH, Tsai JT, et al. Astragalus polysaccharides (PG2) enhances the M1 polarization of macrophages, functional maturation of dendritic cells, and T cell-mediated anticancer immune responses in patients with lung cancer. Nutrients (2019) 11. doi: 10.3390/nu11102264
147. Wang S, Long S, Deng Z, Wu W. Positive role of Chinese herbal medicine in cancer immune regulation. Am J Chin Med (2020) 48:1577–92. doi: 10.1142/s0192415x20500780
148. Wang Y, Lu J, Jiang B, Guo J. The roles of curcumin in regulating the tumor immunosuppressive microenvironment. Oncol Lett (2020) 19:3059–70. doi: 10.3892/ol.2020.11437
149. Chen L, Musa AE. Boosting immune system against cancer by resveratrol. Phytother Res (2021) 35:5514–26. doi: 10.1002/ptr.7189
150. Chhabra G, Singh CK, Amiri D, Akula N, Ahmad N. Recent advancements on immunomodulatory mechanisms of resveratrol in tumor microenvironment. Molecules (2021) 26. doi: 10.3390/molecules26051343
151. Paul S, Sa G. Curcumin as an adjuvant to cancer immunotherapy. Front Oncol (2021) 11:675923. doi: 10.3389/fonc.2021.675923
152. Wang Y, Zhang X, Wang Y, Zhao W, Li H, Zhang L, et al. Application of immune checkpoint targets in the anti-tumor novel drugs and traditional Chinese medicine development. Acta Pharm Sin B (2021) 11:2957–72. doi: 10.1016/j.apsb.2021.03.004
153. Fu X, Zhang G, Zhang Y, Sun H, Yang S, Ni S, et al. Co-Delivery of anticancer drugs and cell penetrating peptides for improved cancer therapy. Chin Chem Lett (2021) 32:1559–62. doi: 10.1016/j.cclet.2020.10.011
154. Zeng H, Qi Y, Zhang Z, Liu C, Peng W, Zhang Y. Nanomaterials toward the treatment of alzheimer’s disease: Recent advances and future trends. Chin Chem Lett (2021) 32:1857–68. doi: 10.1016/j.cclet.2021.01.014
155. Ko CN, Zang S, Zhou Y, Zhong Z, Yang C. Nanocarriers for effective delivery: modulation of innate immunity for the management of infections and the associated complications. J Nanobiotechnol (2022) 20:380. doi: 10.1186/s12951-022-01582-8
Keywords: tumor immunity, immunomodulation, traditional Chinese medicine, active ingredients, antitumor immunotherapy, natural products
Citation: Yang C, Li D, Ko C-N, Wang K and Wang H (2023) Active ingredients of traditional Chinese medicine for enhancing the effect of tumor immunotherapy. Front. Immunol. 14:1133050. doi: 10.3389/fimmu.2023.1133050
Received: 28 December 2022; Accepted: 28 February 2023;
Published: 10 March 2023.
Edited by:
Wen Tan, Lanzhou University, ChinaReviewed by:
Xianfeng Hui, Xinxiang Medical University, ChinaHuayi Yang, Macau University of Science and Technology, China
Copyright © 2023 Yang, Li, Ko, Wang and Wang. This is an open-access article distributed under the terms of the Creative Commons Attribution License (CC BY). The use, distribution or reproduction in other forums is permitted, provided the original author(s) and the copyright owner(s) are credited and that the original publication in this journal is cited, in accordance with accepted academic practice. No use, distribution or reproduction is permitted which does not comply with these terms.
*Correspondence: Chung-Nga Ko, Y2h1bmduZ2Frb0BnbWFpbC5jb20=; Kai Wang, d2FuZ2thaUBzd211LmVkdS5jbg==; Haiyong Wang, d2FuZ2hhaXlvbmc2Njg4QDEyNi5jb20=
†These authors have contributed equally to this work