- 1Sidney Kimmel Cancer Center, Thomas Jefferson University, Philadelphia, PA, United States
- 2Department of Medical Oncology, Thomas Jefferson University, Philadelphia, PA, United States
- 3Department of Internal Medicine, The Ohio State University, Columbus, OH, United States
- 4Department of Pharmacology, Physiology and Cancer Biology, Thomas Jefferson University, Philadelphia, PA, United States
Cytokines are a vital component of the immune system that controls the activation and growth of blood cells. However, chronic overexpression of cytokines can trigger cellular events leading to malignant transformation. The cytokine interleukin-15 (IL-15) is of particular interest, which has been shown to contribute to the development and progression of various hematological malignancies. This review will provide an overview of the impact of the immunopathogenic function of IL-15 by studying its role in cell survival, proliferation, inflammation, and treatment resistance. We will also review therapeutic approaches for inhibiting IL-15 in blood cancers.
Introduction
Cytokines are small, secreted proteins essential for the activation and growth of immune cells and crucial in controlling cell signaling pathways in physiological homeostasis and disease states (1). Released by different types of immune and non-immune cells, they also regulate cell-to-cell communications, with complex regulatory functions in inflammation, cancer, and immunity. Their autocrine, paracrine, and endocrine functions are known to modulate and enhance the anti-tumor immune response and represent exploitable therapies alone or in combination with other agents (2, 3). However, chronic overexpression of cytokines can trigger cellular events leading to hyperproliferation and malignant transformation of immune cells (4). The cytokine interleukin-15 (IL-15) has multifaceted functions and controversial roles in the development and progression of various hematological malignancies but also has a crucial function in the cytotoxic boost against tumoral cells (5). Numerous IL-15-enhancing approaches have been used to treat different cancers, including leukemias and lymphomas, exploiting the main function of the cytokine to potentiate Natural Killer (NK) and T-cell responses and unleash immune-mediated cancer eradication. Nevertheless, the pathogenic role of IL-15 in lymphoproliferative tumors raises concerns about the counterintuitive use of these therapies in this group of malignancies. This review aims to: i. summarize the role of IL-15 deregulation in the pathogenesis of B- and T-cell neoplasms, ii. evaluate its potential as a therapeutic target, and iii. discuss the impact of IL-15 in the context of the new immunotherapeutic agents and adoptive cell therapies.
IL-15 functions and signaling
IL-15, a 14–15-kD 4-α helix bundle family cytokine member, belongs to the same family as IL-2 and was discovered in 1994 by two laboratories (6–8). It is a pleiotropic cytokine with a major role in the ontogeny, activation, and survival of CD8+ T cells, NKT cells, γδT cells, and NK cells (9, 10). It induces activated B-cells to produce immunoglobulins and is, thus, involved in the interface between nonspecific and acquired immunity (11, 12). IL-15 provides a fast and effective immunology burst to fight invading pathogens. However, compared to IL-2, IL-15 does not affect T-regulatory cells and activation-induced cell death (AICD) (13). IL-15 stimulates cell proliferation and differentiation, works as an anti-apoptotic factor, and its mRNA is expressed by many cell types; however, the protein expression of IL-15 is mainly restricted to activated myeloid cells such as monocytes, macrophages, and dendritic cells (14). Its translation is tightly regulated through multiple negative regulatory elements to limit its potency in eliciting and enhancing the inflammatory response. The large pool of untranslated IL-15 transcripts in different cells can be interpreted as emergency storage, ready to be released in case of a sudden invasion by pathogens or other exogenous insults (15).
The IL-15 receptor consists of a heterotrimeric structure within the plasma membrane, composed of a specific IL-15 receptor alpha subunit (IL-15Rα) (16), a beta subunit (IL-2R/15Rβ) shared with the IL-2 receptor, and a gamma subunit (γc) commonly shared with IL-2, IL-4, IL-7, IL-9, and IL-21 (17). Among the three subunits, IL-15Rα has a greater binding affinity for IL-15 than β and γc subunits; the soluble IL-15/IL15Rα complex is one of the IL-15 functional forms. Soluble and membrane-bound forms of IL-15 have also been described (18, 19). IL-15 can either signal in an autocrine manner by binding to IL-15Rα and IL-2/15R on the same cell (cis-presentation), or paracrine manner that involves presentation of IL-15 bound to IL-15Rα to another cell expressing the IL-2/15Rβγc transducing receptors (trans-presentation) (20–22). IL-15 binding induces conformational changes that lead to activation of the receptor-associated kinases, JAK1 and JAK3, resulting in the phosphorylation of STAT3 via the βc and of STAT5 via the γc. This causes activation of PI3K/Akt/mTOR and Ras/Raf/MAPK signaling cascades (Figure 1), resulting in a proliferative and anti-apoptotic transcriptional program, potentially leading to malignant transformation (23). In addition, PDGFR and IL-15/Akt/XBP1 signaling pathways have been recognized as mediators of the NK cell survival (24, 25). Another recently described mechanism of control from IL-15 on NK cell survival is the trans-endocytosis of the membrane-associated IL-15-IL-15Rα complexes into NK cells, which leads to the activation of STAT5 and subsequent induction of proliferation and survival signals (21). Recently, the IL-15-mediated upregulation of telomerase has been linked to the hyperproliferative program of tumors (26). Due to its complex functions, IL-15 has the potential as a tumor driver and an essential component of the anti-tumor immune response (27).
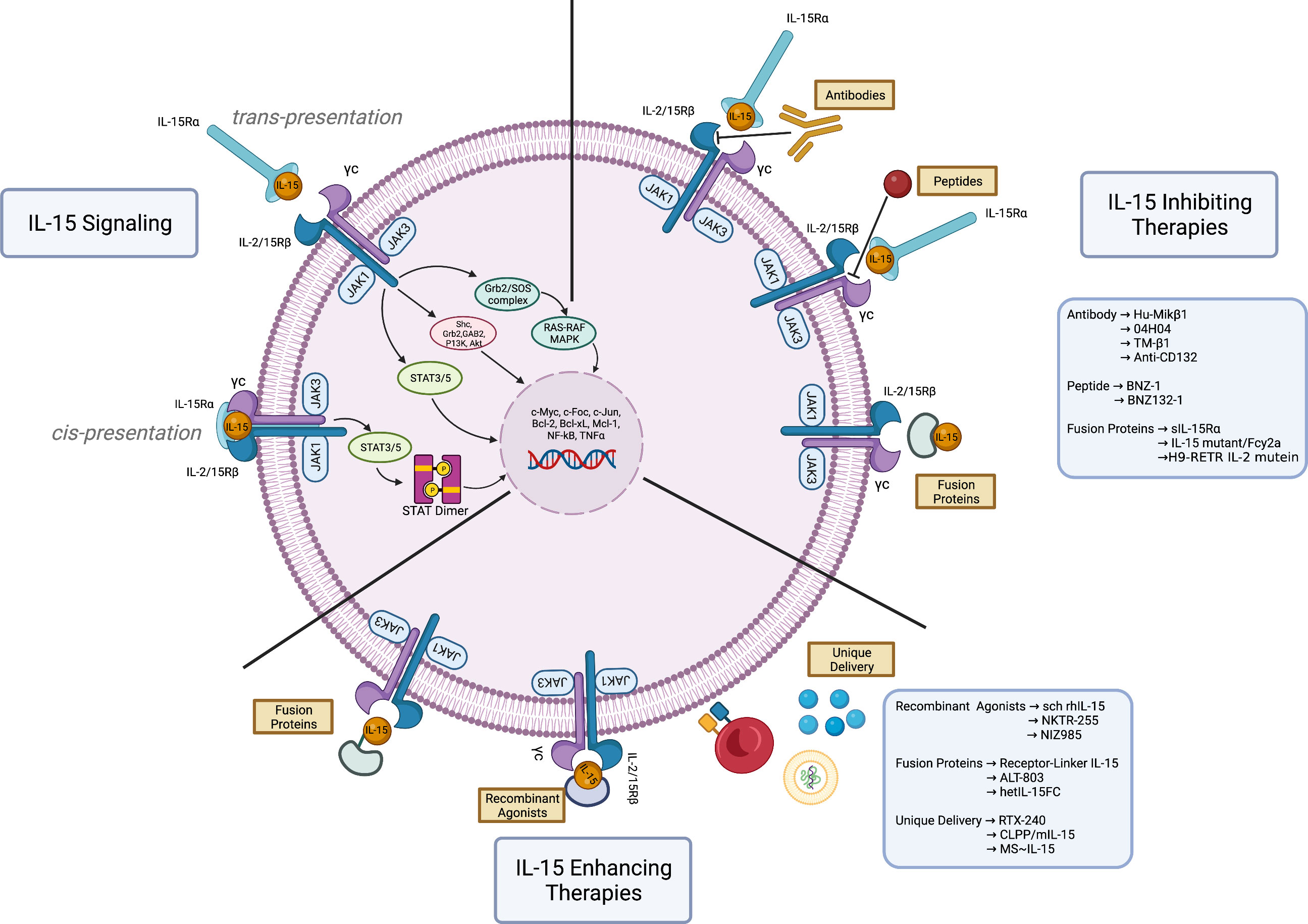
Figure 1 Therapeutic approaches on Interleukin-15 signaling. IL-15 signaling. Interleukin-15 (IL-15) plays a major role in the immune responses by serving as a growth factor for T- and NK-cells. Normal IL-15 signaling begins following the binding of IL-15, produced by dendritic cells, with its high affinity receptor IL-15Rα on the same cells (cis presentation). Upon binding, it is trans-presented to the IL-2R/15Rβ-γc receptor complex expressed by T- and NK-cells, which consequently activates JAK1/JAK3 along with STAT3/5, PI3K/Akt and Ras/Raf/MAPK. These signaling cascades trigger a transcriptional program aimed at boosting the anti-pathogens inflammatory response and enhancing the anti-tumoral immune response. Paradoxically, the overexpression of IL-15 can result in the development of hematological malignancies. IL-15 inhibiting therapies. Numerous interventions have been developed to block IL-15 signaling and the T- and NK-cells tumoral proliferation. Some examples are anti-IL-15 antibody (Hu-Mikβ1, 04H04, TM-β1, anti-CD-132), peptide antagonists (BNZ-1, BNZ132-1), and fusion proteins (soluble IL-15Rα-sIL15Rα, IL-15 mutant/Fcγ2a, H9-RETR IL-2 mutein). IL-15 enhancing therapies. Highly efficient immune response enhancing agents have been developed to leverage the ability of IL-15 to boost the anti-tumoral effector cells. The figure depicts recombinant agonists (sch rhIL-15, NKTR-255, NIZ985), fusion proteins (receptor linker IL-15 or RLI, ALT-803, hetIL-15FC), and unique delivery systems (RTX-240, CLPP/mIL-15, MS~IL-15). These compounds boost the immune response against tumoral cells and can potentiate the effect of immunotherapy and adoptive cell therapy. Figure made with Biorender.com.
Various IL-15 transgenic mouse models that carry cloned IL-15 under a variety of different foreign promoters have been instrumental in understanding the role of IL-15 in immunity and blood cancers. Depending on the promoters, genetic background and expression levels, IL-15 mice show either altered immunity or development of aggressive T-/NK- cell leukemia, and cutaneous T-cell lymphoma (13, 28–32). Conversely, IL-15 deficiency has been linked to leukemia development in an immunodeficient mouse model, suggesting a protective role of IL-15 against leukemia (31).
The pathogenic role of IL-15 in hematological malignancies
Adult T-cell leukemia (ATL), a rare and aggressive leukemia associated with human T-cell lymphotropic virus type 1 (HTLV-1), was the first model that allowed the discovery of some main functions of IL-15 in cancer (6). IL-15 and IL-15Rα mRNAs are overexpressed in T-cells infected with HTLV-1, the viral causative agent of ATL and are related to the proliferation of the malignant cells (14). The viral protein Tax induces the transcription of IL-15 and its receptor through an NF-kB motif (33, 34). Additional findings confirmed that IL-15 is involved in leukemia growth through an autocrine/paracrine mechanism (35). A link between ATL proliferation and the activation of the JAK/STAT pathway, one of the IL-15 downstream pathways, was established (36). The results of a JAK3 inhibitor in in vitro and in vivo models are promising (37) and clinical trials with drugs targeting the JAK/STAT pathway in ATL are ongoing (NCT01712659).
Cutaneous T-cell lymphoma (CTCL) is a heterogeneous group of lymphoproliferative disorders consisting of aberrant T-cell proliferation in the skin. Numerous studies have demonstrated the overexpression of IL-15 mRNA and protein in CTCL and the level of IL-15 is predictive of the disease severity (32, 38–40). An autocrine/paracrine mechanism has been described for CTCL and IL-15 (39), which allows the tumoral cells to create a self-sustaining microenvironment. IL-15 is responsible for the upregulation of c-myc, c-Jun and Bcl2 in CTCL, through the stimulation of the STAT transcription factors family (41). IL-15 modulates more than 1000 gene transcripts and activates JAK1, JAK3, STAT5, ERK, and PI3K/AKT (42). Another IL-15-dependent mechanism of proliferation in CTCL is the selective γc cytokine-mediated activation of the mTORC1 pathway (43).
Large granular lymphocytic leukemia (LGLL) is characterized by the clonal expansion of large granular lymphocytes (LGL) of both cytotoxic T-cell or NK type in the blood. The abnormal expression of IL-15 and serum IL-15Rα in LGLL is a major player in the pathogenesis of the disease due to their role in regulating T-and NK-cells activation and cytotoxicity, and overexpression of IL-15 in the cellular environment can easily initiate the progression of LGLL, as shown in several studies (44–46). Leukemia raised in IL-15 transgenic mice has been recognized as an in vivo model of LGLL, further confirming the cytokine’s pathogenetic role in this disorder (47). IL-15 drives normal large granular lymphocyte in vitro and in vivo transformation through myc-mediated activation of aurora kinases, DNA hypermethylation and chromosomal instability, leading to cytogenetic aberrancies (44). One of the mechanisms of IL-15-mediated leukemogenesis is the accelerated proteasomal degradation of BID (BH3-Interacting Domain Death Agonist), a pro-apoptotic Bcl-2 family member, that, together with the overexpression of Bcl2 anti-apoptotic genes, leads to the malignant cell aberrant survival (48).
IL-15 can have a role in the pathogenesis of the hematological complication of celiac disease (CD), namely enteropathy-associated T-cell lymphoma (EATL). Mucosa-associated T-cell subpopulations are mainly CD8+ T-cells and can proliferate and expand after an IL-15 stimulus. IL-15 is overexpressed in lamina propria and intestinal epithelium of patients affected by CD and manages intraepithelial lymphocyte homeostasis between their defense role and uncontrolled inflammation and malignant transformation (49, 50).
IL-15 sustains chronic inflammation, autoimmunity, and uncontrolled T-cell proliferation, leading to T-cell lymphoma development (51). The survival signals from IL-15 are mediated by anti-apoptotic factors BCL2/BCL-xL and require IL-15Rβ, JAK3, and STAT5 (52).
As a survival and anti-apoptotic factor for NK cells, IL-15 has a role in the survival and progression of NK cell neoplasms, stimulating tumor growth through the cytokine released by the tumor microenvironment (TME). Monocytes are the main source of IL-15 in NK-neoplasms TME and enhance cell growth by cell contact-dependent interaction via membrane-bound IL-15 (53). A large study recognized the prognostic role of serum IL-15 in extranodal NK/T cell lymphoma, but additional studies are needed to understand the impact of the cytokine on the pathogenesis of the disease (54).
Acute lymphoblastic leukemia (ALL) is characterized by the malignant transformation and proliferation of immature B- and T-cells. ALL constitutively expresses IL-15 and γc subunit (55). This expression is higher in the T-cell subtype, in BCR/ABL negative ALL and lymph node and mediastinal infiltration, and, most importantly, seems to have an impact on relapse-free survival (56). Overexpression of IL-15 correlates with disease severity and, more precisely, with Central Nervous System (CNS) involvement, through the upregulation of PSGL-1 and CXCR3, which mediate the homing of malignant cells to the CNS (57, 58), an immunologic sanctuary, where the NK cells are not present (59). IL-15, as a pro-inflammatory cytokine, can contribute to the increased permeability of the blood-brain barrier, facilitating the development of the CNS disease. Although the evidence suggests that IL-15 is associated with ALL development and progression, further studies are needed to identify the molecular mechanisms that are responsible for this observation. The available studies focus on the association between ALL, its clinical features and the level of IL-15 and are more observational. There is a need for more mechanistic investigations aiming to support the involvement of IL-15 in the pathogenesis of the disease.
Several studies have highlighted the importance of IL-15 single nucleotide polymorphisms (SNP) in ALL development, severity, and response to treatment (60–63), with some differences between T-ALL and B-ALL (64). In a cohort of 164 ALL patients, compared to 158 controls, the prevalence of the hetero- and homozygous variants of rs17007695, TC + CC genotype, was 79.6% in ALL patients and 62.7% in controls. The SNPs found in ALL are commonly located within the 3’UTR of the IL-15 gene and can lead to a reduced negative regulatory effect and, thus, an enhanced IL-15 transcription and translation efficiency (61, 65). High IL-15 expression can support ALL development and proliferation and can influence the tumor microenvironment interactions, leading to differences in minimal residual disease and treatment response.
In multiple myeloma (MM), a malignant plasma cell dyscrasia, unbalanced inflammatory cytokines, and strong microenvironment component characterize its pathogenesis. IL-15 and the receptor complex have been identified in MM cell lines and primary MM samples (66). Autocrine IL-15 stimulation is the microenvironment-independent mechanism through which MM cells avoid apoptosis and support their growth. Another study confirmed the role of IL-15 as a key regulator of proliferation and survival in MM (67). In a larger study, serum IL-15 levels were higher in 40 MM patients, compared to healthy controls, with a trend to be overexpressed in advanced stages (68). Further studies are needed to explore the pathogenetic role of IL-15 in MM.
In chronic lymphocytic leukemia (CLL), characterized by increasing accumulation of mature malignant B lymphocytes, IL-15 stimulates cell proliferation in vitro, by binding the IL-2R subunits β and γ (69, 70). B-cell proliferation is enhanced by the coexpression of IL-15 and CD40L and is induced by strong activation of STAT5 and ERK1/2 pathways (71). IL-15 can drive leukemogenesis through enhanced mutagenesis due to the suppression of key DNA damage response mediators and overexpression of cyclin D2, via PI3K and STAT5 upregulation (72).
IL-15, secreted by follicular dendritic cells, has been recognized in support of germinal center B cell proliferation, pointing out a potential involvement in germinal center-derived B-cell lymphoma pathogenesis (73). The cytokine can be trans-presented by surrounding cells, like macrophages and monocytes, and can promote STAT5-mediated B-cell proliferation and lymphomagenesis (74). In other cases, the monoclonal B-cells can aberrantly secrete the cytokine.
Hodgkin lymphoma (HL), a rare and potentially curable B-cell malignancy, is known to have a highly inflammatory microenvironment and to produce different types of cytokines: IL-15 and its receptor complex are aberrantly expressed, and IL-15 is secreted by the malignant cells and acts as an anti-apoptotic factor in HL and a mitogen through the phosphorylation of STAT5, and ERK1/2 (75). IL-15 is also able to induce resistance to standard chemotherapies. The assessment of IL-15 and IL-15 receptor complex in B-cell Burkitt’s lymphoma lines confirmed the overexpression of the cytokine, mainly in EBV+ cells (76).
IL-15 can have a mitogenic and anti-apoptotic function in selected subsets of acute myeloid leukemia (AML), confirmed by the expression of the IL-2Rβγ complex by blasts (77). Chronic overexposure to IL-15 of NK cells in AML has been recently described: this leads to NK exhaustion and reduced anti-tumor activity and metabolic defects (78). Some of these studies were conducted with longer durations or higher doses of IL-15. These may have affected the outcome of the investigations in different ways, including in animal models and clinical trials. It is important to note that the exposure times and intensity of the IL-15 doses are important factors that can affect the clinical and biological effects of this cytokine.
Additionally, in both primary myelofibrosis (PMF) and myelodysplastic syndromes (MDS), higher levels of IL-15 were detected. but it is only in PMF that it was regarded as a prognostic factor (79, 80). Overexpression of IL-15 in MDS is indicated to cause the growth of memory T-cells in patients (81).
Therapeutic interventions on IL-15 signaling
The multi-faceted nature of IL-15 encompasses its anti-tumorigenic abilities as a booster of the immune response, and its pathogenic function as a driver of malignant cell proliferation, through the activation of crucial signaling pathways. Both sides of IL-15 function can be exploited in therapeutic approaches against cancer. A list of IL-15-related therapeutic interventions is provided in Table 1. Agents that inhibit IL-15 include soluble IL-15Rα, antibodies against IL-15 or components of the heterotrimeric receptor, and steric antagonists of the receptor in the form of a modified IL-2 (Figure 1). These approaches showed variable clinical activity and further evaluations of efficacy and toxicity in different hematological malignancies are needed.
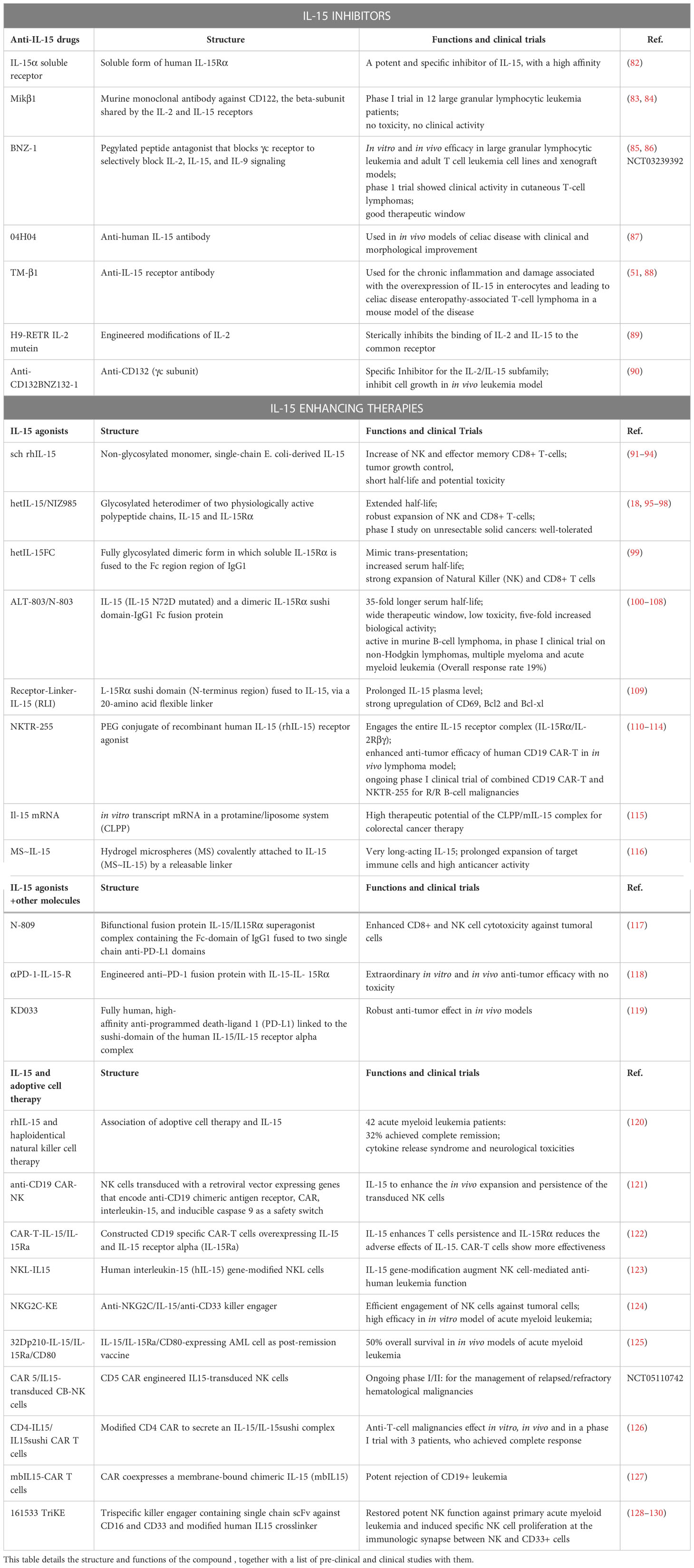
Table 1 IL-15-related therapeutic interventions: IL-15 is used in hematologic malignancies using anti-IL-15 drugs, IL-15 agonists, chimeric compounds of IL-15 and other molecules, and a combination of IL-15 with adoptive cell therapies.
IL-15 has the highest potential as a therapeutic tool when administered with the purpose of eliciting tumor eradication through the activation of NK cells and T-lymphocytes. Major challenges in employing IL-15 as an anti-tumoral agent are its limiting pharmacokinetics and the risk of toxicities. With an in vivo half-life of 2.5 hours and its unstable protein structure, the IL-15 has demonstrated a limited effect, nevertheless safe for infusion therapy in clinical use (91, 131). With a deeper understanding of IL-15 biology, new therapeutic agents have been developed to improve in vivo pharmacokinetics, mimic physiologic IL-15:IL-15Rα trans-presentation and reduce the risk of toxicity.
The results of preclinical and clinical studies have brought to the conclusion that IL-15 is not enough as a single agent and produces the best effects when in combination with other agents, namely checkpoint inhibitors and monoclonal antibodies (132–139). IL-15 treatment is currently being evaluated in several ongoing clinical studies in conjunction with immune checkpoint blockade (#NCT02523469, #NCT04261439, #NCT03905135), monoclonal antibody (#NCT02689453) and bispecific monoclonal antibodies (#NCT02384954).
The most recent publications on IL-15 concern its use in the field of cellular adoptive therapies, both as an adjuvant in the in vitro expansion of CAR-T and CAR-NK cells and in combination with the cellular infusion to propagate and maintain the cellular therapies in vivo. In the phase I/II clinical trial of CD19 CAR-T for B-cell malignancies (NCT01865617), low serum IL-15 post-CAR-T infusion concentration was related to inferior CAR-T kinetics and, analogously, high levels of IL-15 in the serum were associated with post-CAR-T cell favorable outcomes, highlighting the importance of this cytokine in the adoptive cell therapy response (140). Novel approaches have integrated the IL-15 gene into the adoptive cells to allow them to secrete the cytokine and auto-sustain their growth, expansion, and cytotoxic function against the tumoral cells (123, 124, 141), with promising clinical activity. The newest engineered cell products are listed in Table 1.
Discussion and conclusions
This minireview aimed to picture a general update on the role of IL-15 in the pathogenesis of hematological malignancies and to briefly describe the use of IL-15-based therapies.
The complex transcriptional and posttranscriptional regulatome of IL-15 represents a major challenge in assessing IL-15 expression in hematological malignancies. There is a need for a systematic evaluation of IL-15 protein expression in B- and T-cell neoplasms, especially considering its eminent role as a combination agent for immunotherapy and adoptive cell therapy.
Of relevance is the association between IL-15-driven malignancies and autoimmunity: LGLL and EATL shared a background of autoimmune diseases and are CD8+ T-cell malignancies. IL-15 differs from IL-2 in its ability to inhibit AICD and have no effect on the Treg subpopulation. Overexpression of IL-15 can be, thus, directly responsible of the persistence of self-reactive lymphocytes that lead to autoimmunity. IL-15 also creates a microenvironment of chronic inflammation, facilitating the malignant transformation of the proliferating lymphocytes. Studying the biology and function of IL-15 can lead to a better understanding of the still unexplored relationship between lymphoproliferative disorders and autoimmunity.
The major trend in the recent literature involves using IL-15 agonists and cytokine-receptor complexes as enhancers of anti-tumor immune response and as combination agents with immunotherapy and adoptive cell therapy. From the results of the most recent clinical trials, the latest option is preferred since the efficacy of IL-15 as monotherapy seems inadequate to induce cancer regression.
However, IL-15 can have a pathogenic role in the development and progression of B- and T-cell neoplasms and different strategies have been established to block its downstream signaling pathways to inhibit malignant cell proliferation. The fact that in hematological malignancies, the tumoral cells are also immune cells can explain this paradoxical role of IL-15. An undetermined aspect is the effect of the administration of exogenous IL-15 for the treatment of known IL-15-driven malignancies: the autocrine and paracrine production of IL-15 from the tumoral cells and the tumoral microenvironment can have opposite effects compared to the exogenous cytokine, and it can be even curative through the inhibition of the IL-15 endogenous production. Further studies are needed, with the aim of avoiding disease progression and systemic toxicities. In this context, the use of a standardized laboratory test to monitor the levels of IL-15 before, during and after IL-15-based therapies is of paramount importance. In addition to this, factors such as the technical and biological variables that affect the commutability of the IL-15 should also be considered. Different levels of IL-15 should be measured between different groups of patients or over time using the same procedure and platform. The data collected from the profiling of IL-15 should be correlated with the results of nucleic acid amplification and imaging observations to obtain an accurate assessment of the disease progression.
A discussed challenge about the use of IL-15 as anti-cancer therapy is the probable effect of chronic exposure of NK cells to IL-15, which can produce a paradoxical reduction in efficacy, due to progressive exhaustion of the target cells, through the cell cycle arrest and decreased cell survival (142). This conclusion is a debate because other groups demonstrated unchanged cell viability and function of NK cells after sustained IL-15 exposure in vivo (143). It is also important to note that the effects of systemic administration of IL-15 on autologous or allogeneic NK cells have been explored in AML patients. IL-15 can trigger the expansion of recipient CD8+ T cells, which can accelerate donor NK rejection. Indeed, the use of systemic IL-15 in combination with allogeneic cell therapy could limit the clinical activity and therapeutic window of these therapies. Two independent clinical trials on relapse/refractory acute myeloid leukemia cohorts treated with NK cell therapy disclosed a lower clinical activity when systemic IL-15 (IL-15; N-803) was used for support, compared with IL-2 (144).
In summary, harnessing IL-15 as an anti-cancer drug should be considered with caution and its appropriateness for a particular type of cancer, especially hematological malignancies, must be carefully assessed.
Author contributions
PS, HP, and AM planned and conceptualized the review. PS and HP wrote the initial draft and have contributed equally. PS, HP, CI, AM, JB, NC, and PP contributed to writing, reviewing, and revision. All authors contributed to the article and approved the submitted version.
Funding
American Society of Hematology to AM; NIH/NCI to AM. PS is funded by T-cell Leukemia/Lymphoma Initiative, Philadelphia.
Conflict of interest
The authors declare that the research was conducted in the absence of any commercial or financial relationships that could be construed as a potential conflict of interest.
Publisher’s note
All claims expressed in this article are solely those of the authors and do not necessarily represent those of their affiliated organizations, or those of the publisher, the editors and the reviewers. Any product that may be evaluated in this article, or claim that may be made by its manufacturer, is not guaranteed or endorsed by the publisher.
References
1. Amin MN, Siddiqui SA, Ibrahim M, Hakim ML, Ahammed M, Kabir A, et al. Inflammatory cytokines in the pathogenesis of cardiovascular disease and cancer. SAGE Open Med (2020) 8:205031212096575. doi: 10.1177/2050312120965752
2. Dranoff G. Cytokines in cancer pathogenesis and cancer therapy. Nat Rev Cancer (2004) 4(1):11–22. doi: 10.1038/nrc1252
3. Landskron G, de la Fuente M, Thuwajit P, Thuwajit C, Hermoso MA. Chronic inflammation and cytokines in the tumor microenvironment. J Immunol Res (2014) 2014:1–19. doi: 10.1155/2014/149185
4. Zamarron BF, Chen W. Dual roles of immune cells and their factors in cancer development and progression. Int J Biol Sci (2011) 7(5):651–8. doi: 10.7150/ijbs.7.651
5. Fiore PF, Di Matteo S, Tumino N, Mariotti FR, Pietra G, Ottonello S, et al. Interleukin-15 and cancer: Some solved and many unsolved questions. J Immunother Cancer (2020) 8(2):e001428. doi: 10.1136/jitc-2020-001428
6. Burton JD, Bamford RN, Peters C, Grant AJ, Kurys G, Goldman CK, et al. A lymphokine, provisionally designated interleukin T and produced by a human adult T-cell leukemia line, stimulates T-cell proliferation and the induction of lymphokine-activated killer cells. Proc Natl Acad Sci (1994) 91(11):4935–9. doi: 10.1073/pnas.91.11.4935
7. Grabstein KH, Eisenman J, Shanebeck K, Rauch C, Srinivasan S, Fung V, et al. Cloning of a T cell growth factor that interacts with the β chain of the interleukin-2 receptor. Science (1994) 264(5161):965–8. doi: 10.1126/science.8178155
8. Waldmann TA, Miljkovic MD, Conlon KC. Interleukin-15 (dys)regulation of lymphoid homeostasis: Implications for therapy of autoimmunity and cancer. J Exp Med (2020) 217(1):e20191062. doi: 10.1084/jem.20191062
9. Van Acker HH, Anguille S, Willemen Y, Van den Bergh JM, Berneman ZN, Lion E, et al. Interleukin-15 enhances the proliferation, stimulatory phenotype, and antitumor effector functions of human gamma delta T cells. J Hematol OncolJ Hematol Oncol (2016) 9(1):101. doi: 10.1186/s13045-016-0329-3
10. Wang X, Zhao XY. Transcription factors associated with IL-15 cytokine signaling during NK cell development. Front Immunol (2021) 12:610789. doi: 10.3389/fimmu.2021.610789
11. Kennedy MK, Glaccum M, Brown SN, Butz EA, Viney JL, Embers M, et al. Reversible defects in natural killer and memory Cd8 T cell lineages in interleukin 15–deficient mice. J Exp Med (2000) 191(5):771–80. doi: 10.1084/jem.191.5.771
12. Lodolce JP, Burkett PR, Koka RM, Boone DL, Ma A. Regulation of lymphoid homeostasis by interleukin-15. Cytokine Growth Factor Rev (2002) 13(6):429–39. doi: 10.1016/S1359-6101(02)00029-1
13. Marks-Konczalik J, Dubois S, Losi JM, Sabzevari H, Yamada N, Feigenbaum L, et al. IL-2-induced activation-induced cell death is inhibited in IL-15 transgenic mice. Proc Natl Acad Sci (2000) 97(21):11445–50. doi: 10.1073/pnas.200363097
14. Bamford RN, Battiata AP, Burton JD, Sharma H, Waldmann TA. Interleukin (IL) 15/IL-T production by the adult T-cell leukemia cell line HuT-102 is associated with a human T-cell lymphotrophic virus type I region/IL-15 fusion message that lacks many upstream AUGs that normally attenuates IL-15 mRNA translation. Proc Natl Acad Sci (1996) 93(7):2897–902. doi: 10.1073/pnas.93.7.2897
15. Bamford RN, DeFilippis AP, Azimi N, Kurys G, Waldmann TA. The 5′ untranslated region, signal peptide, and the coding sequence of the carboxyl terminus of IL-15 participate in its multifaceted translational control. J Immunol (1998) 160(9):4418–26. doi: 10.4049/jimmunol.160.9.4418
16. Giri JG, Ahdieh M, Eisenman J, Shanebeck K, Grabstein K, Kumaki S, et al. Utilization of the beta and gamma chains of the IL-2 receptor by the novel cytokine IL-15. EMBO J (1994) 13(12):2822–30. doi: 10.1002/j.1460-2075.1994.tb06576.x
17. Fehniger TA, Caligiuri MA. Interleukin 15: Biology and relevance to human disease. Blood (2001) 97(1):14–32. doi: 10.1182/blood.V97.1.14
18. Bergamaschi C, Bear J, Rosati M, Beach RK, Alicea C, Sowder R, et al. Circulating IL-15 exists as heterodimeric complex with soluble IL-15Rα in human and mouse serum. Blood (2012) 120(1):e1–8. doi: 10.1182/blood-2011-10-384362
19. Giron-Michel J, Giuliani M, Fogli M, Brouty-Boyé D, Ferrini S, Baychelier F, et al. Membrane-bound and soluble IL-15/IL-15Rα complexes display differential signaling and functions on human hematopoietic progenitors. Blood (2005) 106(7):2302–10. doi: 10.1182/blood-2005-01-0064
20. Burkett PR, Koka R, Chien M, Chai S, Boone DL, Ma A. Coordinate expression and trans presentation of interleukin (IL)-15Rα and IL-15 supports natural killer cell and memory CD8+ T cell homeostasis. J Exp Med (2004) 200(7):825–34. doi: 10.1084/jem.20041389
21. Anton OM, Peterson ME, Hollander MJ, Dorward DW, Arora G, Traba J, et al. Trans -endocytosis of intact IL-15Rα–IL-15 complex from presenting cells into NK cells favors signaling for proliferation. Proc Natl Acad Sci (2020) 117(1):522–31. doi: 10.1073/pnas.1911678117
22. Huntington ND, Legrand N, Alves NL, Jaron B, Weijer K, Plet A, et al. IL-15 trans-presentation promotes human NK cell development and differentiation in vivo. J Exp Med (2009) 206(1):25–34. doi: 10.1084/jem.20082013
23. Shenoy AR, Kirschnek S, Häcker G. IL-15 regulates bcl-2 family members bim and mcl-1 through JAK/STAT and PI3K/AKT pathways in T cells: Molecular immunology. Eur J Immunol (2014) 44(8):2500–7. doi: 10.1002/eji.201344238
24. Ma S, Tang T, Wu X, Mansour AG, Lu T, Zhang J, et al. PDGF-D–PDGFRβ signaling enhances IL-15–mediated human natural killer cell survival. Proc Natl Acad Sci (2022) 119(3):e2114134119. doi: 10.1073/pnas.2114134119
25. Wang Y, Zhang Y, Yi P, Dong W, Nalin AP, Zhang J, et al. The IL-15–AKT–XBP1s signaling pathway contributes to effector functions and survival in human NK cells. Nat Immunol (2019) 20(1):10–7. doi: 10.1038/s41590-018-0265-1
26. Watkinson F, Nayar SK, Rani A, Sakellariou CA, Elhage O, Papaevangelou E, et al. IL-15 upregulates telomerase expression and potently increases proliferative capacity of NK, NKT-like, and CD8 T cells. Front Immunol (2021) 11:594620. doi: 10.3389/fimmu.2020.594620
27. Santana Carrero RM, Beceren-Braun F, Rivas SC, Hegde SM, Gangadharan A, Plote D, et al. IL-15 is a component of the inflammatory milieu in the tumor microenvironment promoting antitumor responses. Proc Natl Acad Sci (2019) 116(2):599–608. doi: 10.1073/pnas.1814642116
28. Fehniger TA, Suzuki K, Ponnappan A, VanDeusen JB, Cooper MA, Florea SM, et al. Fatal leukemia in interleukin 15 transgenic mice follows early expansions in natural killer and memory phenotype CD8+ T cells. J Exp Med. (2001) 193(2):219–31. doi: 10.1084/jem.193.2.219
29. Katano I, Nishime C, Ito R, Kamisako T, Mizusawa T, Ka Y, et al. Long-term maintenance of peripheral blood derived human NK cells in a novel human IL-15- transgenic NOG mouse. Sci Rep (2017) 7(1):17230. doi: 10.1038/s41598-017-17442-7
30. Ju C, Zhang M, Wu D, Tang J, Li S, Zhao J, et al. Human interleukin 15 (IL15) humanized NCG mice support the human natural killer cells reconstitution and development. Blood (2019) 134(Supplement_1):4871–1. doi: 10.1182/blood-2019-123661
31. Bobbala D, Kandhi R, Chen X, Mayhue M, Bouchard E, Yan J, et al. Interleukin-15 deficiency promotes the development of T-cell acute lymphoblastic leukemia in non-obese diabetes mice with severe combined immunodeficiency. Leukemia (2016) 30(8):1749–52. doi: 10.1038/leu.2016.28
32. Mishra A, La Perle K, Kwiatkowski S, Sullivan LA, Sams GH, Johns J, et al. Mechanism, consequences, and therapeutic targeting of abnormal IL15 signaling in cutaneous T-cell lymphoma. Cancer Discov (2016) 6(9):986–1005. doi: 10.1158/2159-8290.CD-15-1297
33. Azimi N, Brown K, Bamford RN, Tagaya Y, Siebenlist U, Waldmann TA. Human T cell lymphotropic virus type I tax protein trans-activates interleukin 15 gene transcription through an NF-κB site. Proc Natl Acad Sci (1998) 95(5):2452–7. doi: 10.1073/pnas.95.5.2452
34. Mariner JM, Lantz V, Waldmann TA, Azimi N. Human T cell lymphotropic virus type I tax activates IL-15Rα gene expression through an NF-κB site. J Immunol (2001) 166(4):2602–9. doi: 10.4049/jimmunol.166.4.2602
35. Kukita T, Arima N, Matsushita K, Arimura K, Ohtsubo H, Sakaki Y, et al. Autocrine and/or paracrine growth of adult T-cell leukaemia tumour cells by interleukin 15: IL-15-induced growth of ATL cells. Br J Haematol (2002) 119(2):467–74. doi: 10.1046/j.1365-2141.2002.03813.x
36. Takemoto S, Mulloy JC, Cereseto A, Migone TS, Patel BKR, Matsuoka M, et al. Proliferation of adult T cell leukemia/lymphoma cells is associated with the constitutive activation of JAK/STAT proteins. Proc Natl Acad Sci (1997) 94(25):13897–902. doi: 10.1073/pnas.94.25.13897
37. Ju W, Zhang M, Jiang Jk, Thomas CJ, Oh U, Bryant BR, et al. CP-690,550, a therapeutic agent, inhibits cytokine-mediated Jak3 activation and proliferation of T cells from patients with ATL and HAM/TSP. Blood (2011) 117(6):1938–46. doi: 10.1182/blood-2010-09-305425
38. Leroy S, Dubois S, Tenaud I, Chebassier N, Godard A, Jacques Y, et al. Interleukin-15 expression in cutaneous T-cell lymphoma (mycosis fungoides and sezary syndrome). Br J Dermatol (2001) 144(5):1016–23. doi: 10.1046/j.1365-2133.2001.04192.x
39. Döbbeling U, Dummer R, Laine E, Potoczna N, Qin JZ, Burg G. Interleukin-15 is an Autocrine/Paracrine viability factor for cutaneous T-cell lymphoma cells. Blood (1998) 92(1):252–8. doi: 10.1182/blood.V92.1.252.413k08_252_258
40. Asadullah K, Haeußler-Quade A, Gellrich S, Hanneken S, Hansen-Hagge TE, Döcke WD, et al. IL-15 and IL-16 overexpression in cutaneous T-cell lymphomas: stage-dependent increase in mycosis fungoides progression: Expression of IL-15 & IL-16 in cutaneous T-cell lymphomas. Exp Dermatol (2000) 9(4):248–51. doi: 10.1034/j.1600-0625.2000.009004248.x
41. Qin JZ, Kamarashev J, Zhang CL, Dummer R, Burg G, Döbbeling U. Constitutive and interleukin-7- and interleukin-15-Stimulated DNA binding of STAT and novel factors in cutaneous T cell lymphoma cells. J Invest Dermatol (2001) 117(3):583–9. doi: 10.1046/j.0022-202x.2001.01436.x
42. Marzec M, Halasa K, Kasprzycka M, Wysocka M, Liu X, Tobias JW, et al. Differential effects of interleukin-2 and interleukin-15 versus interleukin-21 on CD4+ cutaneous T-cell lymphoma cells. Cancer Res (2008) 68(4):1083–91. doi: 10.1158/0008-5472.CAN-07-2403
43. Marzec M, Liu X, Kasprzycka M, Witkiewicz A, Raghunath PN, El-Salem M, et al. IL-2– and IL-15–induced activation of the rapamycin-sensitive mTORC1 pathway in malignant CD4+ T lymphocytes. (2008) 111(4):2181–9. doi: 10.1182/blood-2007-06-095182
44. Zambello R, Facco M, Trentin L, Sancetta R, Tassinari C, Perin A, et al. Interleukin-15 triggers the proliferation and cytotoxicity of granular lymphocytes in patients with lymphoproliferative disease of granular lymphocytes. Blood (1997) 89(1):201–11. doi: 10.1182/blood.V89.1.201
45. Mishra A, Liu S, Sams GH, Curphey DP, Santhanam R, Rush LJ, et al. Aberrant overexpression of IL-15 initiates Large granular lymphocyte leukemia through chromosomal instability and DNA hypermethylation. Cancer Cell (2012) 22(5):645–55. doi: 10.1016/j.ccr.2012.09.009
46. Chen J, Petrus M, Bamford R, Shih JH, Morris JC, Janik JE, et al. Increased serum soluble IL-15Rα levels in T-cell large granular lymphocyte leukemia. Blood (2012) 119(1):137–43. doi: 10.1182/blood-2011-04-346759
47. Yokohama A, Mishra A, Mitsui T, Becknell B, Johns J, Curphey D, et al. A novel mouse model for the aggressive variant of NK cell and T cell large granular lymphocyte leukemia. Leuk Res (2010) 34(2):203–9. doi: 10.1016/j.leukres.2009.06.031
48. Hodge DL, Yang J, Buschman MD, Schaughency PM, Dang H, Bere W, et al. Interleukin-15 enhances proteasomal degradation of bid in normal lymphocytes: Implications for Large granular lymphocyte leukemias. Cancer Res (2009) 69(9):3986–94. doi: 10.1158/0008-5472.CAN-08-3735
49. James OJ, Vandereyken M, Marchingo JM, Singh F, Bray SE, Wilson J, et al. IL-15 and PIM kinases direct the metabolic programming of intestinal intraepithelial lymphocytes. Nat Commun (2021) 12(1):4290. doi: 10.1038/s41467-021-24473-2
50. Mention JJ, Ben Ahmed M, Bègue B, Barbe U, Verkarre V, Asnafi V, et al. Interleukin 15: a key to disrupted intraepithelial lymphocyte homeostasis and lymphomagenesis in celiac disease. Gastroenterology (2003) 125(3):730–45. doi: 10.1016/S0016-5085(03)01047-3
51. Yokoyama S, Takada K, Hirasawa M, Perera LP, Hiroi T. Transgenic mice that overexpress human IL-15 in enterocytes recapitulate both b and T cell-mediated pathologic manifestations of celiac disease. J Clin Immunol (2011) 31(6):1038–44. doi: 10.1007/s10875-011-9586-7
52. Malamut G, El Machhour R, Montcuquet N, Martin-Lannerée S, Dusanter-Fourt I, Verkarre V, et al. IL-15 triggers an antiapoptotic pathway in human intraepithelial lymphocytes that is a potential new target in celiac disease–associated inflammation and lymphomagenesis. J Clin Invest (2010) 120(6):2131–43. doi: 10.1172/JCI41344
53. Ishii H, Takahara M, Nagato T, Kis LL, Nagy N, Kishibe K, et al. Monocytes enhance cell proliferation and LMP1 expression of nasal natural killer/T-cell lymphoma cells by cell contact-dependent interaction through membrane-bound IL-15. Int J Cancer (2012) 130(1):48–58. doi: 10.1002/ijc.25969
54. Wang H, Zhu J, Liu Cc, Zhu My, Wang Jh, Geng Qr, et al. Increased serum levels of interleukin-15 correlate with negative prognostic factors in extranodal NK/T cell lymphoma. Med Oncol (2015) 32(1):370. doi: 10.1007/s12032-014-0370-4
55. Kebelmann-Betzing C, Körner G, Badiali L, Buchwald D, Möricke A, Korte A, et al. Characterization of cytokine, growth factor receptor, costimulatory and adhesion molecule expression patterns of bone marrow blasts in relapsed childhood b cell precursor all. Cytokine (2001) 13(1):39–50. doi: 10.1006/cyto.2000.0794
56. Wu S, Fischer L, Gökbuget N, Schwartz S, Burmeister T, Notter M, et al. Expression of interleukin 15 in primary adult acute lymphoblastic leukemia. Cancer (2010) 116(2):387–92. doi: 10.1002/cncr.24729
57. Cario G, Izraeli S, Teichert A, Rhein P, Skokowa J, Möricke A, et al. High interleukin-15 expression characterizes childhood acute lymphoblastic leukemia with involvement of the CNS. J Clin Oncol (2007) 25(30):4813–20. doi: 10.1200/JCO.2007.11.8166
58. Williams MTS, Yousafzai Y, Cox C, Blair A, Carmody R, Sai S, et al. Interleukin-15 enhances cellular proliferation and upregulates CNS homing molecules in pre-b acute lymphoblastic leukemia. Blood (2014) 123(20):3116–27. doi: 10.1182/blood-2013-05-499970
59. Frishman-Levy L, Shemesh A, Bar-Sinai A, Ma C, Ni Z, Frenkel S, et al. Central nervous system acute lymphoblastic leukemia: role of natural killer cells. Blood (2015) 125(22):3420–31. doi: 10.1182/blood-2014-08-595108
60. Aly RM, Taalab MM, Ghazy HF. Influence of interleukin-15 polymorphism on the survival of adult patients with acute lymphoblastic leukemia in Egypt. Leuk Lymphoma (2015) 56(1):151–6. doi: 10.3109/10428194.2014.910659
61. Lu Y, Kham SKY, Ariffin H, Oei AMI, Lin HP, Tan AM, et al. Host genetic variants of ABCB1 and IL15 influence treatment outcome in paediatric acute lymphoblastic leukaemia. Br J Cancer (2014) 110(6):1673–80. doi: 10.1038/bjc.2014.7
62. Yang JJ, Cheng C, Yang W, Pei D, Cao X, Fan Y, et al. Genome-wide interrogation of germline genetic variation associated with treatment response in childhood acute lymphoblastic leukemia. JAMA (2009) 301(4):393–403. doi: 10.1001/jama.2009.7
63. Rots D, Kreile M, Nikulshin S, Kovalova Z, Gailite L. Influence of IL15 gene variations on the clinical features, treatment response and risk of developing childhood acute lymphoblastic leukemia in Latvian population. Pediatr Hematol Oncol (2018) 35(1):37–44. doi: 10.1080/08880018.2018.1440334
64. Lin D, Liu C, Xue M, Liu R, Jiang L, Yu X, et al. The role of interleukin-15 polymorphisms in adult acute lymphoblastic leukemia. PloS One (2010) 5(10):e13626. doi: 10.1371/journal.pone.0013626
65. Zhang XJ, Yan KL, Wang ZM, Yang S, Zhang GL, Fan X, et al. Polymorphisms in interleukin-15 gene on chromosome 4q31.2 are associated with psoriasis vulgaris in Chinese population. J Invest Dermatol (2007) 127(11):2544–51. doi: 10.1038/sj.jid.5700896
66. Tinhofer I, Marschitz I, Henn T, Egle A, Greil R. Expression of functional interleukin-15 receptor and autocrine production of interleukin-15 as mechanisms of tumor propagation in multiple myeloma. Blood (2000) 95(2):610–8. doi: 10.1182/blood.V95.2.610
67. Hjorth-Hansen H, Waage A, Börset M. Interleukin-15 blocks apoptosis and induces proliferation of the human myeloma cell line OH-2 and freshly isolated myeloma cells: IL-15 promotes growth and inhibits apoptosis in myeloma. Br J Haematol (1999) 106(1):28–34. doi: 10.1046/j.1365-2141.1999.01510.x
68. Pappa C, Miyakis S, Tsirakis G, Sfiridaki A, Alegakis A, Kafousi M, et al. Serum levels of interleukin-15 and interleukin-10 and their correlation with proliferating cell nuclear antigen in multiple myeloma. Cytokine (2007) 37(2):171–5. doi: 10.1016/j.cyto.2007.02.022
69. Trentin L, Cerutti A, Zambello R, Sancretta R, Tassinari C, Facco M, et al. Interleukin-15 promotes the growth of leukemic cells of patients with b- cell chronic lymphoproliferative disorders. Blood (1996) 87(8):3327–35. doi: 10.1182/blood.V87.8.3327.bloodjournal8783327
70. Mongini PKA, Gupta R, Boyle E, Nieto J, Lee H, Stein J, et al. TLR-9 and IL-15 synergy promotes the In vitro clonal expansion of chronic lymphocytic leukemia b cells. J Immunol (2015) 195(3):901–23. doi: 10.4049/jimmunol.1403189
71. de Totero D, Meazza R, Capaia M, Fabbi M, Azzarone B, Balleari E, et al. The opposite effects of IL-15 and IL-21 on CLL b cells correlate with differential activation of the JAK/STAT and ERK1/2 pathways. Blood (2008) 111(2):517–24. doi: 10.1182/blood-2007-04-087882
72. Gupta R, Li W, Yan XJ, Barrientos J, Kolitz JE, Allen SL, et al. Mechanism for IL-15–driven b cell chronic lymphocytic leukemia cycling: Roles for AKT and STAT5 in modulating cyclin D2 and DNA damage response proteins. J Immunol (2019) 202(10):2924–44. doi: 10.4049/jimmunol.1801142
73. Park CS, Yoon SO, Armitage RJ, Choi YS. Follicular dendritic cells produce IL-15 that enhances germinal center b cell proliferation in membrane-bound form. J Immunol (2004) 173(11):6676–83. doi: 10.4049/jimmunol.173.11.6676
74. Epron G, Ame-Thomas P, Le Priol J, Pangault C, Dulong J, Lamy T, et al. Monocytes and T cells cooperate to favor normal and follicular lymphoma b-cell growth: role of IL-15 and CD40L signaling. Leukemia (2012) 26(1):139–48. doi: 10.1038/leu.2011.179
75. Ullrich K, Blumenthal-Barby F, Lamprecht B, Köchert K, Lenze D, Hummel M, et al. The IL-15 cytokine system provides growth and survival signals in Hodgkin lymphoma and enhances the inflammatory phenotype of HRS cells. Leukemia (2015) 29(5):1213–8. doi: 10.1038/leu.2014.345
76. Tsukamoto K, Huang YC, Dorsey WC, Carns B, Sharma V. Juxtacrine function of interleukin-15/interleukin-15 receptor system in tumour derived human b-cell lines. Clin Exp Immunol (2006) 146(3):559–66. doi: 10.1111/j.1365-2249.2006.03240.x
77. Meazza R, Basso S, Gaggero A, Detotero D, Trentin L, Pereno R, et al. Interleukin (IL)-15 induces survival and proliferation of the growth factor-dependent acute myeloid leukemia m-07e through the IL-2 receptor β/γ. Int J Cancer (1998) 78(2):189–95. doi: 10.1002/(SICI)1097-0215(19981005)78:2<189::AID-IJC12>3.0.CO;2-6
78. Bou-Tayeh B, Laletin V, Salem N, Just-Landi S, Fares J, Leblanc R, et al. Chronic IL-15 stimulation and impaired mTOR signaling and metabolism in natural killer cells during acute myeloid leukemia. Front Immunol (2021) 12:730970. doi: 10.3389/fimmu.2021.730970
79. Tefferi A, Vaidya R, Caramazza D, Finke C, Lasho T, Pardanani A. Circulating interleukin (IL)-8, IL-2R, IL-12, and IL-15 levels are independently prognostic in primary myelofibrosis: A comprehensive cytokine profiling study. J Clin Oncol (2011) 29(10):1356–63. doi: 10.1200/JCO.2010.32.9490
80. Pardanani A, Finke C, Lasho TL, Al-Kali A, Begna KH, Hanson CA, et al. IPSS-independent prognostic value of plasma CXCL10, IL-7 and IL-6 levels in myelodysplastic syndromes. Leukemia (2012) 26(4):693–9. doi: 10.1038/leu.2011.251
81. Dong W, Ding T, Wu L, Ren X, Epling-Burnette PK, Yang L. Effect of IL-7 and IL-15 on T cell phenotype in myelodysplastic syndromes. Oncotarget (2016) 7(19):27479–88. doi: 10.18632/oncotarget.8459
82. Mortier E, Bernard J, Plet A, Jacques Y. Natural, proteolytic release of a soluble form of human IL-15 receptor α-chain that behaves as a specific, high affinity IL-15 antagonist. J Immunol (2004) 173(3):1681–8. doi: 10.4049/jimmunol.173.3.1681
83. Morris JC, Janik JE, White JD, Fleisher TA, Brown M, Tsudo M, et al. Preclinical and phase I clinical trial of blockade of IL-15 using Mikβ1 monoclonal antibody in T cell large granular lymphocyte leukemia. Proc Natl Acad Sci (2006) 103(2):401–6. doi: 10.1073/pnas.0509575103
84. Waldmann TA, Conlon KC, Stewart DM, Worthy TA, Janik JE, Fleisher TA, et al. Phase 1 trial of IL-15 trans presentation blockade using humanized mik-Beta-1 mAb in patients with T-cell large granular lymphocytic leukemia. Blood (2013) 121(3):476–84. doi: 10.1182/blood-2012-08-450585
85. Wang TT, Yang J, Zhang Y, Zhang M, Dubois S, Conlon KC, et al. IL-2 and IL-15 blockade by BNZ-1, an inhibitor of selective γ-chain cytokines, decreases leukemic T-cell viability. Leukemia (2019) 33(5):1243–55. doi: 10.1038/s41375-018-0290-y
86. Frohna PA, Ratnayake A, Doerr N, Basheer A, Al-Mawsawi LQ, Kim WJ, et al. Results from a first-in-Human study of BNZ-1, a selective multicytokine inhibitor targeting members of the common gamma (γc) family of cytokines. J Clin Pharmacol (2020) 60(2):264–73. doi: 10.1002/jcph.1522
87. Sestak K, Dufour JP, Liu DX, Rout N, Alvarez X, Blanchard J, et al. Beneficial effects of human anti-Interleukin-15 antibody in gluten-sensitive rhesus macaques with celiac disease. Front Immunol (2018) 9:1603. doi: 10.3389/fimmu.2018.01603
88. Yokoyama S, Watanabe N, Sato N, Perera PY, Filkoski L, Tanaka T, et al. Antibody-mediated blockade of IL-15 reverses the autoimmune intestinal damage in transgenic mice that overexpress IL-15 in enterocytes. Proc Natl Acad Sci (2009) 106(37):15849–54. doi: 10.1073/pnas.0908834106
89. Mitra S, Ring AM, Amarnath S, Spangler JB, Li P, Ju W, et al. Interleukin-2 activity can be fine tuned with engineered receptor signaling clamps. Immunity (2015) 42(5):826–38. doi: 10.1016/j.immuni.2015.04.018
90. Nata T, Basheer A, Cocchi F, van Besien R, Massoud R, Jacobson S, et al. Targeting the binding interface on a shared receptor subunit of a cytokine family enables the inhibition of multiple member cytokines with selectable target spectrum. J Biol Chem (2015) 290(37):22338–51. doi: 10.1074/jbc.M115.661074
91. Sneller MC, Kopp WC, Engelke KJ, Yovandich JL, Creekmore SP, Waldmann TA, et al. IL-15 administered by continuous infusion to rhesus macaques induces massive expansion of CD8+ T effector memory population in peripheral blood. Blood (2011) 118(26):6845–8. doi: 10.1182/blood-2011-09-377804
92. Conlon KC, Lugli E, Welles HC, Rosenberg SA, Fojo AT, Morris JC, et al. Redistribution, hyperproliferation, activation of natural killer cells and CD8 T cells, and cytokine production during first-in-Human clinical trial of recombinant human interleukin-15 in patients with cancer. J Clin Oncol (2015) 33(1):74–82. doi: 10.1200/JCO.2014.57.3329
93. Waldmann TA, Lugli E, Roederer M, Perera LP, Smedley JV, Macallister RP, et al. Safety (toxicity), pharmacokinetics, immunogenicity, and impact on elements of the normal immune system of recombinant human IL-15 in rhesus macaques. Blood (2011) 117(18):4787–95. doi: 10.1182/blood-2010-10-311456
94. Vyas VV, Esposito D, Sumpter TL, Broadt TL, Hartley J, Knapp GC IV, et al. Clinical manufacturing of recombinant human interleukin 15. i. production cell line development and protein expression in e. coli with stop codon optimization. Biotechnol Prog (2012) 28(2):497–507. doi: 10.1002/btpr.746
95. Conlon K, Watson DC, Waldmann TA, Valentin A, Bergamaschi C, Felber BK, et al. Phase I study of single agent NIZ985, a recombinant heterodimeric IL-15 agonist, in adult patients with metastatic or unresectable solid tumors. J Immunother Cancer (2021) 9(11):e003388. doi: 10.1136/jitc-2021-003388
96. Chertova E, Bergamaschi C, Chertov O, Sowder R, Bear J, Roser JD, et al. Characterization and favorable in vivo properties of heterodimeric soluble IL-15·IL-15Rα cytokine compared to IL-15 monomer*. J Biol Chem (2013) 288(25):18093–103. doi: 10.1074/jbc.M113.461756
97. Ng SSM, Nagy BA, Jensen SM, Hu X, Alicea C, Fox BA, et al. Heterodimeric IL15 treatment enhances tumor infiltration, persistence, and effector functions of adoptively transferred tumor-specific T cells in the absence of lymphodepletion. Clin Cancer Res (2017) 23(11):2817–30. doi: 10.1158/1078-0432.CCR-16-1808
98. Thaysen-Andersen M, Chertova E, Bergamaschi C, Moh ESX, Chertov O, Roser J, et al. Recombinant human heterodimeric IL-15 complex displays extensive and reproducible n- and O-linked glycosylation. Glycoconj J (2016) 33(3):417–33. doi: 10.1007/s10719-015-9627-1
99. Dubois S, Patel HJ, Zhang M, Waldmann TA, Müller JR. Preassociation of IL-15 with IL-15Rα-IgG1-Fc enhances its activity on proliferation of NK and CD8+/CD44high T cells and its antitumor action. J Immunol (2008) 180(4):2099–106. doi: 10.4049/jimmunol.180.4.2099
100. Rubinstein MP, Williams C, Mart C, Beall J, MacPherson L, Azar J, et al. Phase I trial characterizing the pharmacokinetic profile of n-803, a chimeric IL-15 superagonist, in healthy volunteers. J Immunol (2022) 208(6):1362–70. doi: 10.4049/jimmunol.2100066
101. Liu B, Kong L, Han K, Hong H, Marcus WD, Chen X, et al. A novel fusion of ALT-803 (Interleukin (IL)-15 superagonist) with an antibody demonstrates antigen-specific antitumor responses. J Biol Chem (2016) 291(46):23869–81. doi: 10.1074/jbc.M116.733600
102. Xu W, Jones M, Liu B, Zhu X, Johnson CB, Edwards AC, et al. Efficacy and mechanism-of-Action of a novel superagonist interleukin-15: Interleukin-15 receptor αSu/Fc fusion complex in syngeneic murine models of multiple myeloma. Cancer Res (2013) 73(10):3075–86. doi: 10.1158/0008-5472.CAN-12-2357
103. Romee R, Cooley S, Berrien-Elliott MM, Westervelt P, Verneris MR, Wagner JE, et al. First-in-human phase 1 clinical study of the IL-15 superagonist complex ALT-803 to treat relapse after transplantation. Blood (2018) 131(23):2515–27. doi: 10.1182/blood-2017-12-823757
104. Foltz JA, Hess BT, Bachanova V, Bartlett NL, Berrien-Elliott MM, McClain E, et al. Phase I trial of n-803, an IL15 receptor agonist, with rituximab in patients with indolent non-Hodgkin lymphoma. Clin Cancer Res (2021) 27(12):3339–50. doi: 10.1158/1078-0432.CCR-20-4575
105. Bailey CP, Budak-Alpdogan T, Sauter CT, Panis MM, Buyukgoz C, Jeng EK, et al. New interleukin-15 superagonist (IL-15SA) significantly enhances graft-versus-tumor activity. Oncotarget (2017) 8(27):44366–78. doi: 10.18632/oncotarget.17875
106. Rosario M, Liu B, Kong L, Collins LI, Schneider SE, Chen X, et al. The IL-15-Based ALT-803 complex enhances FcγRIIIa-triggered NK cell responses and In vivo clearance of b cell lymphomas. Clin Cancer Res (2016) 22(3):596–608. doi: 10.1158/1078-0432.CCR-15-1419
107. Van der Meer JMR, Maas RJA, Guldevall K, Klarenaar K, de Jonge PKJD, Evert JSHv, et al. IL-15 superagonist n-803 improves IFNγ production and killing of leukemia and ovarian cancer cells by CD34+ progenitor-derived NK cells. Cancer Immunol Immunother (2021) 70(5):1305–21. doi: 10.1007/s00262-020-02749-8
108. Zhu X, Marcus WD, Xu W, Lee H, Han K, Egan JO, et al. Novel human interleukin-15 agonists. J Immunol (2009) 183(6):3598–607. doi: 10.4049/jimmunol.0901244
109. Mortier E, Quéméner A, Vusio P, Lorenzen I, Boublik Y, Grötzinger J, et al. Soluble interleukin-15 receptor α (IL-15Rα)-sushi as a selective and potent agonist of IL-15 action through IL-15Rβ/γ. J Biol Chem (2006) 281(3):1612–9. doi: 10.1074/jbc.M508624200
110. Robinson TO, Hegde SM, Chang A, Gangadharan A, Rivas S, Madakamutil L, et al. NKTR-255 is a polymer-conjugated IL-15 with unique mechanisms of action on T and natural killer cells. J Clin Invest (2021) 131(19):e144365. doi: 10.1172/JCI144365
111. Fernandez RA, Mayoral JE, Pierre-Louis L, Yao Y, Xu Y, Mu S, et al. Improving NK cell function in multiple myeloma with NKTR-255, a novel polymer-conjugated human IL-15. Blood Adv (2023) 7(1):9–19. doi: 10.1182/bloodadvances.2022007985
112. Hirayama AV, Chou CK, Miyazaki T, Steinmetz RN, Di HA, Fraessle SP, et al. A novel polymer-conjugated human IL-15 improves efficacy of CD19-targeted CAR-T cell immunotherapy. Blood Adv (2022). doi: 10.1182/bloodadvances.2022008697
113. Shah N, Perales MA, Turtle CJ, Cairo MS, Cowan AJ, Saeed H, et al. Phase I study protocol: NKTR-255 as monotherapy or combined with daratumumab or rituximab in hematologic malignancies. Future Oncol (2021) 17(27):3549–60. doi: 10.2217/fon-2021-0576
114. Miyazaki T, Maiti M, Hennessy M, Chang T, Kuo P, Addepalli M, et al. NKTR-255, a novel polymer-conjugated rhIL-15 with potent antitumor efficacy. J Immunother Cancer (2021) 9(5):e002024. doi: 10.1136/jitc-2020-002024
115. Lei S, Zhang X, Men K, Gao Y, Yang X, Wu S, et al. Efficient colorectal cancer gene therapy with IL-15 mRNA nanoformulation. Mol Pharm (2020) 17(9):3378–91. doi: 10.1021/acs.molpharmaceut.0c00451
116. Hangasky JA, Chen W, Dubois SP, Daenthanasanmak A, Müller JR, Reid R, et al. A very long-acting IL-15: implications for the immunotherapy of cancer. J Immunother Cancer (2022) 10(1):e004104. doi: 10.1136/jitc-2021-004104
117. Jochems C, Tritsch SR, Knudson KM, Gameiro SR, Rumfield CS, Pellom ST, et al. The multi-functionality of n-809, a novel fusion protein encompassing anti-PD-L1 and the IL-15 superagonist fusion complex. OncoImmunology (2019) 8(2):e1532764. doi: 10.1080/2162402X.2018.1532764
118. Shen J, Zou Z, Guo J, Cai Y, Xue D, Liang Y, et al. An engineered concealed IL-15-R elicits tumor-specific CD8+T cell responses through PD-1-cis delivery. J Exp Med (2022) 219(12):e20220745. doi: 10.1084/jem.20220745
119. Martomo SA, Lu D, Polonskaya Z, Luna X, Zhang Z, Feldstein S, et al. Single-dose anti–PD-L1/IL-15 fusion protein KD033 generates synergistic antitumor immunity with robust tumor-immune gene signatures and memory responses. Mol Cancer Ther (2021) 20(2):347–56. doi: 10.1158/1535-7163.MCT-20-0457
120. Cooley S, He F, Bachanova V, Vercellotti GM, DeFor TE, Curtsinger JM, et al. First-in-human trial of rhIL-15 and haploidentical natural killer cell therapy for advanced acute myeloid leukemia. Blood Adv (2019) 3(13):1970–80. doi: 10.1182/bloodadvances.2018028332
121. Liu E, Marin D, Banerjee P, Macapinlac HA, Thompson P, Basar R, et al. Use of CAR-transduced natural killer cells in CD19-positive lymphoid tumors. N Engl J Med (2020) 382(6):545–53. doi: 10.1056/NEJMoa1910607
122. Zhang Y, Zhuang Q, Wang F, Zhang C, Xu C, Gu A, et al. Co-Expression IL-15 receptor alpha with IL-15 reduces toxicity via limiting IL-15 systemic exposure during CAR-T immunotherapy. J Transl Med (2022) 20(1):432. doi: 10.1186/s12967-022-03626-x
123. Jiang W, Zhang C, Tian Z, Zhang J. hIL-15-gene modified human natural killer cells (NKL-IL15) exhibit anti-human leukemia functions. J Cancer Res Clin Oncol (2018) 144(7):1279–88. doi: 10.1007/s00432-018-2654-0
124. Chiu E, Felices M, Cichocki F, Davis Z, Wang H, Tuninga K, et al. Anti-NKG2C/IL-15/anti-CD33 killer engager directs primary and iPSC-derived NKG2C+ NK cells to target myeloid leukemia. Mol Ther (2021) 29(12):3410–21. doi: 10.1016/j.ymthe.2021.06.018
125. Shi Y, Dincheva-Vogel L, Ayemoba CE, Fung JP, Bergamaschi C, Pavlakis GN, et al. IL-15/IL-15Ra/CD80-expressing AML cell vaccines eradicate minimal residual disease in leukemic mice. Blood Adv (2018) 2(22):3177–92. doi: 10.1182/bloodadvances.2018019026
126. Feng J, Xu H, Cinquina A, Wu Z, Zhang W, Sun L, et al. Treatment of aggressive T-cell lymphoma/leukemia with anti-CD4 CAR T cells. Front Immunol (2022) 13:997482. doi: 10.3389/fimmu.2022.997482
127. Hurton LV, Singh H, Najjar AM, Switzer KC, Mi T, Maiti S, et al. Tethered IL-15 augments antitumor activity and promotes a stem-cell memory subset in tumor-specific T cells. Proc Natl Acad Sci (2016) 113(48):E7788–97. doi: 10.1073/pnas.1610544113
128. Vallera DA, Felices M, McElmurry R, McCullar V, Zhou X, Schmohl JU, et al. IL15 trispecific killer engagers (TriKE) make natural killer cells specific to CD33+ targets while also inducing persistence, In vivo expansion, and enhanced function. Clin Cancer Res (2016) 22(14):3440–50. doi: 10.1158/1078-0432.CCR-15-2710
129. Felices M, Kodal B, Hinderlie P, Kaminski MF, Cooley S, Weisdorf DJ, et al. Novel CD19-targeted TriKE restores NK cell function and proliferative capacity in CLL. Blood Adv (2019) 3(6):897–907. doi: 10.1182/bloodadvances.2018029371
130. Felices M, Lenvik TR, Kodal B, Lenvik AJ, Hinderlie P, Bendzick LE, et al. Potent cytolytic activity and specific IL15 delivery in a second-generation trispecific killer engager. Cancer Immunol Res (2020) 8(9):1139–49. doi: 10.1158/2326-6066.CIR-19-0837
131. Dubois SP, Miljkovic MD, Fleisher TA, Pittaluga S, Hsu-Albert J, Bryant BR, et al. Short-course IL-15 given as a continuous infusion led to a massive expansion of effective NK cells: Implications for combination therapy with antitumor antibodies. J Immunother Cancer (2021) 9(4):e002193. doi: 10.1136/jitc-2020-002193
132. Zhang M, Wen B, Anton OM, Yao Z, Dubois S, Ju W, et al. IL-15 enhanced antibody-dependent cellular cytotoxicity mediated by NK cells and macrophages. Proc Natl Acad Sci (2018) 115(46):E10915–24. doi: 10.1073/pnas.1811615115
133. Moga E, Cantó E, Vidal S, Juarez C, Sierra J, Briones J. Interleukin-15 enhances rituximab-dependent cytotoxicity against chronic lymphocytic leukemia cells and overcomes transforming growth factor beta-mediated immunosuppression. Exp Hematol (2011) 39(11):1064–71. doi: 10.1016/j.exphem.2011.08.006
134. Yu P, Steel JC, Zhang M, Morris JC, Waldmann TA. Simultaneous blockade of multiple immune system inhibitory checkpoints enhances antitumor activity mediated by interleukin-15 in a murine metastatic colon carcinoma model. Clin Cancer Res (2010) 16(24):6019–28. doi: 10.1158/1078-0432.CCR-10-1966
135. Waldmann TA, Dubois S, Miljkovic MD, Conlon KC. IL-15 in the combination immunotherapy of cancer. Front Immunol (2020) 11:868. doi: 10.3389/fimmu.2020.00868
136. Laprevotte E, Voisin G, Ysebaert L, Klein C, Daugrois C, Laurent G, et al. Recombinant human IL-15 Trans -presentation by b leukemic cells from chronic lymphocytic leukemia induces autologous NK cell proliferation leading to improved anti-CD20 immunotherapy. J Immunol (2013) 191(7):3634–40. doi: 10.4049/jimmunol.1300187
137. Miljkovic M, Müller J, Ma E, Waldmann T. Interleukin-15 augments NK cell-mediated ADCC of alemtuzumab in patients with CD52+ T-cell malignancies. Blood Adv (2023) 7(3):384–94. doi: 10.1182/bloodadvances.2021006440
138. Wrangle JM, Velcheti V, Patel MR, Garrett-Mayer E, Hill EG, Ravenel JG, et al. ALT-803, an IL-15 superagonist, in combination with nivolumab in patients with metastatic non-small cell lung cancer: a non-randomised, open-label, phase 1b trial. Lancet Oncol (2018) 19(5):694–704. doi: 10.1016/S1470-2045(18)30148-7
139. Shin SJ, O’Sullivan Coyne G, Streicher H, Takebe N, Bruns A, Sharon E, et al. Abstract CT147: Phase 1 study of recombinant interleukin 15 (rhIL-15) in combination with checkpoint inhibitors nivolumab and ipilimumab in subjects with refractory cancers. Cancer Res (2022) 82(12_Supplement):CT147–7. doi: 10.1158/1538-7445.AM2022-CT147
140. Kochenderfer JN, Somerville RPT, Lu T, Shi V, Bot A, Rossi J, et al. Lymphoma remissions caused by anti-CD19 chimeric antigen receptor T cells are associated with high serum interleukin-15 levels. J Clin Oncol (2017) 35(16):1803–13. doi: 10.1200/JCO.2016.71.3024
141. Christodoulou I, Ho WJ, Marple A, Ravich JW, Tam A, Rahnama R, et al. Engineering CAR-NK cells to secrete IL-15 sustains their anti-AML functionality but is associated with systemic toxicities. J Immunother Cancer (2021) 9(12):e003894. doi: 10.1136/jitc-2021-003894
142. Felices M, Lenvik AJ, McElmurry R, Chu S, Hinderlie P, Bendzick L, et al. Continuous treatment with IL-15 exhausts human NK cells via a metabolic defect. JCI Insight (2018) 3(3):e96219. doi: 10.1172/jci.insight.96219
143. Conlon KC, Miljkovic MD, Waldmann TA. Cytokines in the treatment of cancer. J Interferon Cytokine Res (2019) 39(1):6–21. doi: 10.1089/jir.2018.0019
Keywords: IL-15, treatment, blood, cytokine, cancer
Citation: Sindaco P, Pandey H, Isabelle C, Chakravarti N, Brammer JE, Porcu P and Mishra A (2023) The role of interleukin-15 in the development and treatment of hematological malignancies. Front. Immunol. 14:1141208. doi: 10.3389/fimmu.2023.1141208
Received: 10 January 2023; Accepted: 22 February 2023;
Published: 20 April 2023.
Edited by:
Sheela Ramanathan, Université de Sherbrooke, CanadaReviewed by:
Kevin Charles Conlon, Clinical Center (NIH), United StatesCopyright © 2023 Sindaco, Pandey, Isabelle, Chakravarti, Brammer, Porcu and Mishra. This is an open-access article distributed under the terms of the Creative Commons Attribution License (CC BY). The use, distribution or reproduction in other forums is permitted, provided the original author(s) and the copyright owner(s) are credited and that the original publication in this journal is cited, in accordance with accepted academic practice. No use, distribution or reproduction is permitted which does not comply with these terms.
*Correspondence: Anjali Mishra, QW5qYWxpLk1pc2hyYUBqZWZmZXJzb24uZWR1
†These authors have contributed equally to this work