- 1National Reference Laboratory for Animal Schistosomiasis, Shanghai Veterinary Research Institute, Chinese Academy of Agricultural Sciences, Shanghai, China
- 2Key Laboratory of Animal Parasitology of Ministry of Agriculture and Rural Affairs, Shanghai Veterinary Research Institute, Chinese Academy of Agricultural Sciences, Shanghai, China
Schistosomiasis is a neglected tropical disease caused by dioecious blood flukes of the genus Schistosoma and second to malaria as a parasitic disease with significant socio-economic impacts. Mating is essential for maturation of male and female schistosomes and for females to lay of eggs, which are responsible for the pathogenesis and propagation of the life cycle beyond the mammalian host. Single-sex schistosomes, which do not produce viable eggs without mating, have been overlooked given the symptomatic paucity of the single-sex schistosomiasis and limited diagnostic toolkit. Besides, single-sex schistosomes are less sensitive to praziquantel. Therefore, these issues should be considered to achieve the elimination of this infection disease. The aim of this review is to summarize current progress in research of single-sex schistosomes and host-parasite interactions.
Introduction
Schistosomiasis is a neglected tropical disease caused by parasitic flatworms (blood flukes) of the genus Schistosoma that affects about 250 million people mainly in tropical and subtropical regions and accounts for 1.4–3.3 million disability-adjusted life years annually (1, 2). The World Health Organization (WHO) currently recommends mass administration of praziquantel (PZQ) for the control, worm/egg burden reduction and elimination of schistosomiasis (3). The increasing attention to schistosomiasis over the past few decades has led to significant improvements in agricultural and irrigation practices, as well as sanitation and hygiene (4, 5). Data from the national surveillance sites for schistosomiasis in China show that there have been no cases of Schistosoma japonicum infection of humans, bovines, or aquatic snails as the intermediate host in 2021 (6), demonstrating remarkable achievements in the control of schistosomiasis. The considerable expansion of preventive chemotherapy in tandem with scientific progress have improved the global control of schistosomiasis (2, 7). A publication by the WHO, titled “Ending the neglect to attain the Sustainable Development Goals: a road map for neglected tropical diseases 2021–2030”, proposes more ambitious targets, including the elimination of schistosomiasis as a public health threat (8). Nevertheless, as a highly complex multi-host parasite, many questions remain unexplored.
Dioecious schistosomes mate and lay eggs within either the mesenteric or venules of the plexus venous of urogenital organs (depending on the species) (1). Most eggs are excreted in urine or feces, thereby contributing to propagate the life cycle. The presence of eggs in faecal or urine samples is considered the gold standard diagnostic tool (9). However, this approach is insufficient for detection of single-sex schistosomiasis (10). An opinion article published in 2018 proposed that exposure to single-sex schistosomes is an overlooked phenomenon, as no eggs are produced within the final host and infection is asymptomatic (11). Most importantly, a single-sex infection cannot be identified by traditional egg-based parasitological tests, which undoubtedly jeopardizes diagnostic accuracy of schistosomiasis (11). Other methods to theoretically detect egg-free single-sex schistosome infection include monitoring of schistosome gut-associated circulating anodic and cathodic antigens in serum or urine (12), and real-time polymerase chain reaction (PCR) analysis of schistosome DNA (13). When considering cost and convenience of rapid detection, circulating cathodic antigen is a direction worthy of further study in the future (14).
Both field and laboratory studies have reported cases of single-sex schistosome infections (15–18). Theoretically, single-sex schistosomes only mate in the host after encountering a worm of the opposite sex (17, 19). However, schistosomes produced by single-sex infections can also mate with the opposite sex of heterologous species and produce viable eggs (20–25). Another recent study indicated that both male and female S. japonicum can survive treatment with PZQ and retain normal reproductive potential (26). Therefore, it is necessary to address infections of single-sex schistosomes. Elucidation of the mechanisms underlying reproductive development of schistosomes and host-parasite interactions offers new perspectives for drug administration and therapies.
Single-sex schistosome infection occurs under natural conditions
Schistosomes mature only after mating and can subsequently survive in human hosts for 3–10 years (27, 28). Initial studies suggested that single-sex schistosome infections do not occur in nature but they can be generated in laboratory animals with cercariae produced from snails infected with single-sex miracidia (29, 30). However, subsequent field studies have found that single-sex schistosome infections do indeed occur in nature (15).
Single-sex miracidia can infect the tropical freshwater snail Oncomelania hupensis as the intermediate host (31). A field study conducted in the hilly areas of Anhui, China identified 67 (0.78%) of 8563 snails infected with schistosomes (32). Of the 46 snails selected for further studies, 21 (45.7%) were infected with female schistosomes, 23 (50.0%) with males, and only 2 (4.3%) with both sexes (32). Other field studies have reported similar results for S. mansoni and S. haematobium (31, 33). Besides, an ecological survey of definitive hosts conducted in 1993 found that 43 (20.8%) of 207 wild rats were infected with single-sex schistosomes, which included 38 (88.4%) infected with males and 5 (11.6%) with females (18). Another survey conducted along the Yangtze River (Hubei, China) identified 22 (5.5%) of 400 sentinel mice infected with schistosomes, of which 14 (63.6%) were infected with only males and 2 (9.1%) with only females (15). However, there is a lack of data from field investigations on how long single-sex schistosomes can survive in the host. Besides, schistosomes have numerous naturally permissive and non-adaptive hosts, thus surveillance is extremely difficult, which can potentially limit control measures (1). Moreover, single-sex schistosomes can remain “mating-ready” for up to 1 year in mice and still produce viable eggs (17, 34). These findings provide compelling proof that schistosomes can exist unpaired in natural environments.
Morphological differences between single-sex and bisexual worms
Morphological observations after dissection are commonly used to identify infections with single-sex schistosomes (35). Single-sex female (SF) worms and bisexual mated female (MF) worms have distinct morphological differences. In general, SF worms are about one-third of the length of MF worms, which renders detection relatively difficult (36). In S. japonicum, developed ovaries could be observed in MF worms at 18 day post infection (dpi) (2–3 days after mating) (37). From 21–25 dpi, MF worms continue to develop with proliferation of vitelline cells, while the ovaries and vitelline glands of SF worms were stunted which contains only stage I vitellocytes (38). An in vitro study found that when separated from the male, the female will lose the ability to produce viable eggs due to the loss of mature oocytes (39). Unlike females, there is no significant morphological difference between single-sex infected male worms (SM) and bisexual infected mated male worms (MM) (40, 41), although the testes of SM worms are slightly smaller (16).
Current omics studies of single-sex schistosomes
Laboratory models for stable single-sex infections can be established by infecting snails with single miracidia and identifying the sex of the cercariae released by individual snails by PCR (42). This model of single-sex infection has paved the way towards a better understanding of single-sex schistosomiasis in the mammalian host (42, 43).
Omics studies are useful to explore the reproductive development of schistosomes. Recent developments of sequence databases and improvements in proteomics and transcriptomics technologies have facilitated high-throughput studies of single-sex schistosomes and provided useful references for further studies of the reproductive mechanisms (44, 45).
Transcriptomics
Alexis et al. (29) identified differentially expressed genes (DEGs) between 42-day S. mansoni SF and MF worms. The results of in situ hybridization studies found that DEGs were mainly localized in the vitellocytes and ovary of MF worms vs. the vitellocytes and subtegumental cells of SF worms (29). The various predicted functions of DEGs in MF worms include oocyte maturation, apoptosis, protein degradation, and interactions between vitellocytes (29). The predicted functions of the DEGs 6767 (GenBank no. CCD61090) and 15402 (GenBank no. XP_002573676) of SF worms involve interactions that occur during mating (46).
An in-depth study conducted by Lu et al. (47) applied RNA-sequencing (RNA-seq) analyses for comparisons of isolated complete ovaries and testes from paired (46-day) and unpaired (67-day) S. mansoni and the adult worm. The results identified 96 genes comparatively enriched in testes of MM worms and 147 in the testes of SM worms. However, the mating procedure resulted in nearly 15-fold more differentially expressed genes (DEGs) in the ovaries between SF and MF worms (47). This enormous discrepancy in quantity is probably, as mentioned above, closely related to the intuitive morphological differences (16). Reference to the Kyoto Encyclopedia of Genes and Genomes classified the DEGs to metabolic and regulatory pathways (48). The results revealed that 849 DEGs from the ovaries of MF worms were involved in the Akt-, MAPK-, and Ras-signaling pathways, ribosome biogenesis, RNA transport, and endocytosis, whereas 435 DEGs from the ovaries of SF worms were involved in focal adhesion, lysosome function, and the MAPK signaling pathway (47). The schistosome egg-shell precursor gene p14 (Smp_131110), which was up-regulated in MF worms, encodes a female-specific tyrosinase that plays a pivotal role in egg shell synthesis (49). Notably, the transcript profiles of SF worms were more similar to either SM or MM worms than MF worms, which might be associated with the evolutionary background (50).
A meta-analysis of RNA-seq studies offered valuable expression data across all life stages of S. mansoni (51). In addition, an interactive web portal was established (51) of not only RNA-seq data, but also conserved structural domains and related pathways. Combined with the WormBase ParaSite database (52), this web portal allows researchers to visualize and analyze data for genomic studies of schistosomes.
Single-cell RNA-seq has also been used to comprehensively describe tissue types and physiology of schistosomes (53). A study by Wendt et al. (53) classified 43642 cells from the adult S. mansoni into 68 distinct cell populations. These data will help to further clarify the development of various cellular lineages during the schistosome life cycle to facilitate the development of novel therapeutics.
Proteomics
Proteomics has been used extensively in schistosomiasis research and has facilitated the discovery of critical molecules involved in reproductive development and as potential vaccine targets (16, 37, 45, 54–58). Schistosomes are highly complex organisms, thus dynamic analysis of the developmental stages is warranted (59). Based on the life cycle of S. japonicum (mating at 15 or 16 dpi, eggshell formation at 22 dpi, and egg laying at 24 dpi) (1), the proteomic profiles of SF and MF worms at 18, 21, 23, and 25 dpi were elucidated by our group (37, 45). In total, 2835 differentially expressed proteins (DEPs) were identified between SF and MF worms at different developmental stages (37). Relative to SF worms, 402, 322, 415, and 505 DEPs were up-regulated, while 230, 267, 290, and 404 DEPs were down-regulated in MF worms at 18, 21, 23, and 25 dpi, respectively (37). Gene ontology functional annotations demonstrated that 34 DEPs down-regulated in MF worms at all four time points were mainly involved in actin-related cell cycle-related functions, whereas 44 DEPs up-regulated in MF worms were involved in protein folding and hydrolysis, redox reactions, translation, and calcium ion binding (37, 60, 61). Schistosoma japonicum translationally controlled tumor protein (SjTCTP), which was highly expressed in MF worms at 18, 21, 23, and 25 dpi, is essential to the development of S. japonicum and recombinant SjTCTP was reported to stimulate partial protective immunity against schistosome infection in BALB/c mice (37).
Similarly, our group conducted comparative analysis of the proteomic profiles of SM and MM worms at 18, 21, 23, and 25 dpi (16), which confirmed 674 DEPs at different developmental stages. As compared to studies of female worms, there were significantly fewer DEPs both overall and at single time points, similar to the transcriptome changes described above (47). However, the proteomics and transcriptomics profiles were not always consistent, possibly because of differences in the timing and loci of gene transcription and translation or posttranscriptional regulation of proteins, suggesting that these differences were not coincidental (62, 63). Interestingly, bioinformatics analysis identified some DEPs closely associated with tumor proliferation in mammals, suggesting that the biological function of a protein might have similar functions in different tissues or organisms and could possibly be involved in the growth and differentiation of some cells in schistosomes (64, 65). S. haematobium is classified as a class I carcinogen by the International Agency for Research on Cancer that is associated with squamous cell carcinoma of the urinary bladder. Proteomic studies that reveal the functional role of DEPs during tumour development in the context of urogenital schistosomiasis are critical to identify not only targets for control, but biomarkers. Promising proteomic studies looking at S. haematobium worm tegument and soluble egg proteins validate this statement (66).
miRNomics: MicroRNA biology and computational analysis
MicroRNAs (miRNAs) are small non-coding RNAs that can negatively regulate the expression of target genes at the post-transcriptional level by binding to the 3’- and 5’-untranslated regions and coding sequences in order to repress translation or initiate degradation (67). Schistosome-derived miRNAs have been implicated in schistosome development and host-parasite interactions in schistosomiasis (68–70). The miRNA expression profiles of SF and MF S. japonicum have been reported (71, 72). Sun et al. (71) investigated differentially expressed miRNAs of 18- and 23-day SF and MF, and found similar miRNA profiles in 18-day SF and MF worms, whereas in 23-day MF worms, sja-bantam was significantly up-regulated, while sja-miR-1, sja-miR-7, sja-miR-7-5p, and sja-miR-71 were significantly up-regulated in 23-day SF worms (71). The predicted target genes of sja-bantam are reportedly related to development of the embryo and primary sexual characteristics, while the four up-regulated miRNAs in 23-day SF worms are associated with ribonucleoprotein complex assembly and microtubule-based processes (71, 73). A previous study by our group identified differentially expressed miRNAs between 25-day SF and MF worms (72), where sja-bantam and 19 other miRNAs were up-regulated in 25-day MF worms, while sja-miR-1, sja-miR-7-5p, and 14 other miRNAs were up-regulated in 25-day SF worms (72). Furthermore, comparisons of the expression profiles of sma-miR-277, sma-miR-4989, and related target genes in single-sex infected schistosomes suggested that sma-miR-277 and sma-miR-4989 have pivotal roles during juvenile-to-adult transition (74). Collectively, these findings indicate that differentially expressed miRNAs and related target genes might regulate the sexual status of female worms.
Although omics has provided tremendous insights into the mystery of single-sex schistosomes, relatively few studies have investigated epigenetics and small non-coding RNAs, other than miRNAs. A summary of recent studies of single-sex infected schistosomes is provided in Table 1.
Single-sex schistosomes regulate hepatic fibrosis in the host
Prolonged survival of schistosomes in the host can facilitate regulation of the host immune response through intricate mechanisms (81). Briefly, a T helper type 1 (Th1) response was generated during the initial phase of infection, which could target immature and mature migrating parasites (82). Schistosomes eggs trigger a dominate Th2 response regulated by regulatory T-cells (Tregs) (81). Granulomatous hypersensitivity to eggs trapped in the liver and intestinal tissues trigger the fundamental pathological causation of schistosomiasis, which could also be interpreted as a strong repair response (83) to suppress inflammation during the initial infection, but can also lead to tissue fibrosis (84). Mature female S. japonicum and S. mansoni produce hundreds of eggs per day, of which some become trapped in the liver, leading to hepatic inflammation, granuloma formation and fibrosis, and portal hypertension that leads to ascites (85). Without prompt treatment, schistosome-induced hepatic fibrosis is often irreversible (86). Therefore, targeting regulation of the host immune response could be useful for treatment or even reversal of schistosome-induced hepatic fibrosis.
Recent studies have investigated the immunomodulatory mechanisms of single-sex schistosomes in schistosome-induced hepatic fibrosis (75, 76, 78). Nicole et al. (75). demonstrated that primary infection of female S. mansoni (week 0–11) in secondary bisexually infected mice (week 12–19, then sacrificed) resulted in suppression of Th2-mediated granuloma and hepatic fibrosis, although no change in parasite load was observed. Mice with a primary infection of male S. mansoni also showed signs of reduced fibrosis, though not as evident as those initially infected with females (75), possibly due to high expression of cytotoxic T-lymphocyte-associated protein 4 (Ctla4) by Foxp3+ Tregs (87). Besides, the lack of relatively high production of Th1 cells implies that Th1 and Th2 responses might be regulated independently (75). Subsequent experiments indicated that Ctla4 had a preventive effect against schistosome-induced hepatic fibrosis (77).
When the timing of two infections were varied (primary infection at week 0–6 and secondary infection at week 7–14), protection against unisexual infection was not achieved when reinfection occurred more than 6 weeks later, thereby revealing the roles of male schistosomes in immune regulation (76). Male schistosomes triggered strong Th2 innate immune reactions during recurrent infection, which led to the strong recruitment of innate inflammatory cells, especially neutrophils and eosinophils, eventually resulting in reduced burdens of worms and eggs (76). However, the reduction to the parasite load in mice with primary infection of male schistosomes had a limited effect on granuloma size and hepatic fibrosis, which might be related to the high level of Th2 cytokines, such as interleukin-13, which promotes fibrosis (88).
A recent comparative transcriptomic analysis has helped to clarify the differences in immune regulation between male and female schistosomes (78). In this study, the number of DEGs in the spleens of mice infected with male schistosomes was more than two-fold greater than mice infected with females, suggesting greater involvement of males (78). Further analysis found that male schistosomes drove dendritic cell maturation and induced T cell differentiation via up-regulation of the costimulatory molecule CD86, whereas infection with unisexual female worms had little effect on the host immune system (78).
Overall, infection by single-sex schistosomes (either sex) can induce immune responses in the host, which subsequently causes a Th1/Th2 imbalance, although further studies are needed to better clarify the effects of female schistosomes. These findings provide promising targets for new immune modulatory strategies against schistosome-induced hepatic fibrosis and possibly other diseases. The different outcomes of single-sex schistosome-induced hepatic fibrosis are presented in Figure 1.
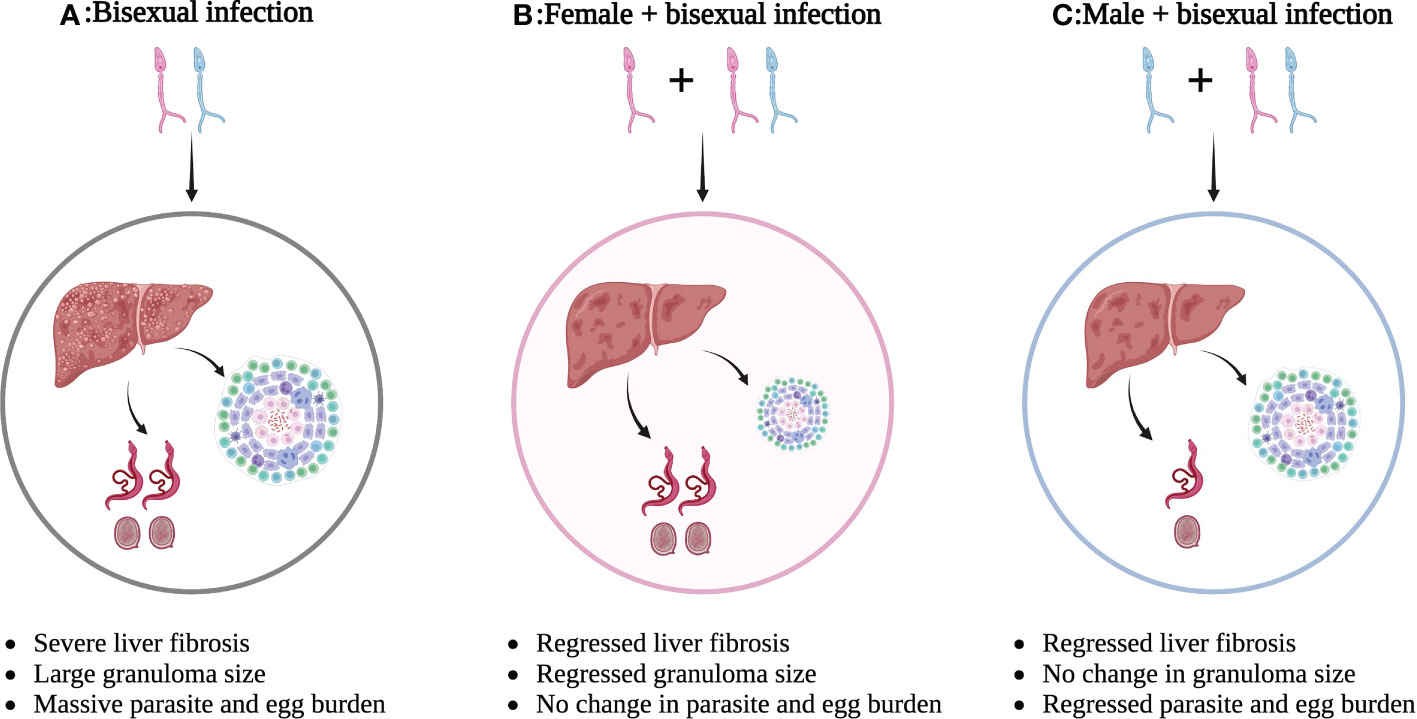
Figure 1 Different outcomes of single-sex schistosome-induced hepatic fibrosis. (A) Bisexual schistosome infection leads to severe liver fibrosis with large granuloma size and massive parasite/egg burden; (B) Bisexual schistosome infection after female infection leads to regressed liver fibrosis with smaller granuloma size, but no change in parasite/egg burden; (C) Bisexual schistosome infection after male infection leads to regressed liver fibrosis with regressed parasite/egg burden, but no change in granuloma size. This figure was created with Biorender.com. Arrows indicate the result of the next step.
Challenges and future perspectives
Several unresolved challenges and problems remain due to insufficient knowledge of single-sex schistosomes, including (i) the application of traditional egg-based parasitological tests for detection of single-sex infections; (ii) detection of antigens or nucleic acids as diagnostic criteria, as positive results might be due to past schistosome exposure or cross-reactions, rather than a single-sex schistosome infection (89, 90), and the reliance on morphological observations after dissection, which can create logistical difficulties; (iii) greater resistance of long-lived single-sex schistosomes to PZQ than paired worms (26); and (iv) the application of Omics studies to identify potential diagnostic and therapeutic targets. Extracellular vesicles (EVs) released from schistosome eggs and worms at different developmental stages were identified and considered to be important vectors in the regulation of host-parasite interactions (70). Studies have reported that the cargo of schistosome-derived EVs include sja-miR-1 (91), sma-miR-10 (92), sja-miR-71a (69), sja-miR-125b (93), sja-miR-2162 (94), sja-bantam (93) and novel sja-miRNA-33 (95), which mediate cross-species host-parasite interactions. However, the expression profiles of the EVs of single-sex schistosomes remain unknown. Thus, further analysis is warranted to provide new insights into immune regulation by single-sex schistosomes.
The development of in vitro culture methods for schistosomes has helped to validate the reliability of targets screened from Omics studies (96). In fact, a recent transcriptomic study indicated that male schistosomes can stimulate synthesis of the pheromone β-alanyl-tryptamine via nonribosomal peptide synthetase to facilitate normal development and laying of eggs by female schistosomes cultured in vitro (79). The results of this study suggest avenues for therapeutic intervention and demonstrated the unlimited potential of single-sex schistosome research.
The recently proposed “hygiene hypothesis” has made it possible to apply the immunomodulatory effects of schistosomes for the prevention and treatment of autoimmune disorders, other than liver fibrosis (69, 94, 95, 97). Schistosome infection and schistosome-derived proteins, peptides, miRNAs and EVs have been reported for the treatment of human immune-related disorders, including allergic asthma (98), arthritis (99), colitis (100), diabetes (101), sepsis (102), cystitis (103), and cancers (104, 105). In addition, human models of male schistosome cercariae infection, which produced no eggs or associated pathology, may provide foundations for subsequent studies (12, 106, 107). Nonetheless, further studies are needed to elucidate the distinct immunomodulatory mechanisms of male and female schistosomes and potential impacts on human diseases.
Conclusions
Current control measures to reduce the infection rate of snails and definitive hosts could greatly increase the incidence of infection with single-sex schistosomes. These measures target the egg load in definitive hosts to reduce infection of snails, but also increase the risk for snails becoming hosts to mono-miracidia and production of only one sex of cercariae that can infect the definitive host (80).
Exposure to single-sex schistosomes is a neglected phenomenon that may become a threat of the control and elimination of schistosomiasis as a public health issue. The continued development of diagnostic and treatment protocols is imperative and further investigations of the reproductive development of schistosomes, as well as single-sex schistosomes, may provide essential references. In addition, future studies of the interactions between single-sex schistosomes and the host are warranted as a theoretical basis for autoimmune disorders, other than schistosomiasis.
Author contributions
YJ and HZ: conceptualization, validation, investigation, resources, and writing – review and editing. HZ: writing – original draft preparation and visualization. YJ: supervision, project administration, and funding acquisition. Both authors contributed to the article and approved the submitted version.
Funding
This work was supported by the Natural Science Foundation of Shanghai (no. 20ZR1469300) and the Chinese National Natural Science Foundation (no. 31672245).
Acknowledgments
We thank International Science Editing for revising the manuscript and their valuable comments.
Conflict of interest
The authors declare that the research was conducted in the absence of any commercial or financial relationships that could be construed as a potential conflict of interest.
Publisher’s note
All claims expressed in this article are solely those of the authors and do not necessarily represent those of their affiliated organizations, or those of the publisher, the editors and the reviewers. Any product that may be evaluated in this article, or claim that may be made by its manufacturer, is not guaranteed or endorsed by the publisher.
References
1. Colley DG, Bustinduy AL, Secor WE, King CH. Human schistosomiasis. Lancet (2014) 383(9936):2253–64. doi: 10.1016/s0140-6736(13)61949-2
2. Lo NC, Bezerra FSM, Colley DG, Fleming FM, Homeida M, Kabatereine N, et al. Review of 2022 who guidelines on the control and elimination of schistosomiasis. Lancet Infect Dis (2022) 22(11):e327–335. doi: 10.1016/s1473-3099(22)00221-3
3. Webster JP, Molyneux DH, Hotez PJ, Fenwick A. The contribution of mass drug administration to global health: past, present and future. Philos Trans R Soc Lond B Biol Sci (2014) 369(1645):20130434. doi: 10.1098/rstb.2013.0434
4. Zhou YB, Liang S, Jiang QW. Factors impacting on progress towards elimination of transmission of schistosomiasis japonica in China. Parasit Vectors (2012) 5:275. doi: 10.1186/1756-3305-5-275
5. Grover E, Paull S, Kechris K, Buchwald A, James K, Liu Y, et al. Predictors of bovine Schistosoma japonicum infection in rural sichuan, China. Int J Parasitol (2022) 52(8):485–96. doi: 10.1016/j.ijpara.2022.04.002
6. Zhang LJ, Xu ZM, Yang F, He JY, Dang H, Li YL, et al. Progress of schistosomiasis control in people's republic of China in 2021. Zhongguo Xue Xi Chong Bing Fang Zhi Za Zhi (2022) 34(4):329–36. doi: 10.16250/j.32.1374.2022132
7. Kokaliaris C, Garba A, Matuska M, Bronzan RN, Colley DG, Dorkenoo AM, et al. Effect of preventive chemotherapy with praziquantel on schistosomiasis among school-aged children in sub-Saharan Africa: a spatiotemporal modelling study. Lancet Infect Dis (2022) 22(1):136–49. doi: 10.1016/s1473-3099(21)00090-6
8. WHO. Ending the neglect to attain the sustainable development goals. a global strategy on water, sanitation, and hygiene to combat neglected tropical diseases 2021–2030. Geneva: World Health Organization (2021).
9. Chen C, Guo Q, Fu Z, Liu J, Lin J, Xiao K, et al. Reviews and advances in diagnostic research on Schistosoma japonicum. Acta Trop (2021) 213:105743. doi: 10.1016/j.actatropica.2020.105743
10. Nation CS, Da'dara AA, Marchant JK, Skelly PJ. Schistosome migration in the definitive host. PloS Negl Trop Dis (2020) 14(4):e0007951. doi: 10.1371/journal.pntd.0007951
11. Lu DB, Deng Y, Ding H, Liang YS, Webster JP. Single-sex schistosome infections of definitive hosts: implications for epidemiology and disease control in a changing world. PloS Pathog (2018) 14(3):e1006817. doi: 10.1371/journal.ppat.1006817
12. Casacuberta-Partal M, van Lieshout L, van Diepen A, Sijtsma JC, Ozir-Fazalalikhan A, Koopman JPR, et al. Excretion patterns of Schistosoma mansoni antigens CCA and CAA by adult male and female worms, using a mouse model and ex vivo parasite cultures. Parasitology (2022) 149(3):306–13. doi: 10.1017/S0031182021001839
13. Sun MT, Gu MM, Zhang JY, Yu QF, Lamberton PHL, Lu DB. Meta-analysis of variable-temperature PCR technique performance for diagnosising Schistosoma japonicum infections in humans in endemic areas. PloS Negl Trop Dis (2022) 16(1):e0010136. doi: 10.1371/journal.pntd.0010136
14. Sousa-Figueiredo JC, Betson M, Kabatereine NB, Stothard JR. The urine circulating cathodic antigen (Cca) dipstick: a valid substitute for microscopy for mapping and point-of-Care diagnosis of intestinal schistosomiasis. PloS Negl Trop Dis (2013) 7(1):e2008. doi: 10.1371/journal.pntd.0002008
15. Tu ZW, Cai SX, Huang XB, Su ZM, Gao H, Chen YY, et al. Surveillance and forecast for schistosome infectivity of Yangtze river and hanbeihe river during flooding in hubei province. Zhongguo Xue Xi Chong Bing Fang Zhi Za Zhi (2012) 24(2):193–5,9.
16. Zhong H, Wu L, Ren Y, Qin F, Jin Y. Comparative proteomic profiles of Schistosoma japonicum male worms derived from single-sex and bisexual infections. Int J Parasitol (2022) 52(13-14):815–28. doi: 10.1016/j.ijpara.2022.09.005
17. Lu DB, Yu QF, Zhang JY, Sun MT, Gu MM, Webster JP, et al. Extended survival and reproductive potential of single-sex male and female Schistosoma japonicum within definitive hosts. Int J Parasitol (2021) 51(11):887–91. doi: 10.1016/j.ijpara.2021.03.005
18. Morand S, Pointier J, Borel G, Theron A. Pairing probability of schistosomes related to their distribution among the host population. Ecology (1993) 74(8):2444–9.
19. Qiu L, Zhang Y, Chen W, Li H, Xue H. Relationship between the sex of Schistosoma japonicum and circulating antigen detection. Zhongguo ji sheng chong xue yu ji sheng chong bing za zhi (1994) 12(3):221–2.
20. Khalil SB, Mansour NS. Worm development in hamsters infected with unisex and cross-mated Schistosoma mansoni and Schistosoma haematobium. J Parasitol (1995) 81(1):8–11.
21. Tchuente LA, Imbert-Establet D, Southgate VR, Jourdane J. Interspecific stimulation of parthenogenesis in Schistosoma intercalatum and S. mansoni. J Helminthol (1994) 68(2):167–73. doi: 10.1017/s0022149x00013717
22. Tchuem Tchuente LA, Southgate VR, Imbert-Establet D, Jourdane J. Change of mate and mating competition between males of Schistosoma intercalatum and S. mansoni. Parasitology (1995) 110(Pt 1):45–52. doi: 10.1017/s0031182000081038
23. Cosgrove CL, Southgate VR. Mating interactions between Schistosoma mansoni and S. margrebowiei. Parasitology (2002) 125(Pt 3):233–43. doi: 10.1017/s0031182002002111
24. Cosgrove CL, Southgate VR. Interactions between Schistosoma intercalatum (zaire strain) and S. mansoni. J Helminthol (2003) 77(3):209–18. doi: 10.1079/JOH2002165
25. Webster BL, Southgate VR, Tchuem Tchuenté LA. Mating interactions between Schistosoma haematobium and S. mansoni. J Helminthol (1999) 73(4):351–6. doi: 10.1017/s0022149x99000591
26. Wang N, Peng HQ, Gao CZ, Cheng YH, Sun MT, Qu GL, et al. In vivo efficiency of praziquantel treatment of single-sex Schistosoma japonicum aged three months old in mice. Int J Parasitol Drugs Drug Resist (2022) 20:129–34. doi: 10.1016/j.ijpddr.2022.11.002
27. Warren KS, Mahmoud AA, Cummings P, Murphy DJ, Houser HB. Schistosomiasis mansoni in Yemeni in California: duration of infection, presence of disease, therapeutic management. Am J Trop Med Hyg (1974) 23(5):902–9. doi: 10.4269/ajtmh.1974.23.902
28. Chabasse D, Bertrand G, Leroux JP, Gauthey N, Hocquet P. Developmental bilharziasis caused by Schistosoma mansoni discovered 37 years after infestation. Bull la Societe pathologie exotique ses filiales (1985) 78(5):643–7.
29. Cogswell AA, Kommer VP, Williams DL. Transcriptional analysis of a unique set of genes involved in Schistosoma mansoni female reproductive biology. PloS Negl Trop Dis (2012) 6(11):e1907. doi: 10.1371/journal.pntd.0001907
30. Shaw JR. Schistosoma mansoni: Pairing in vitro and development of females from single sex infections. Exp Parasitol (1977) 41(1):54–65. doi: 10.1016/0014-4894(77)90129-1
31. Woolhouse ME, Chandiwana SK, Bradley M. On the distribution of schistosome infections among host snails. Int J Parasitol (1990) 20(3):325–7. doi: 10.1016/0020-7519(90)90147-f
32. Shi HP, Lu DB, Shen L, Shi T, Gu J. Single- or mixed-sex Schistosoma japonicum infections of intermediate host snails in hilly areas of anhui, China. Parasitol Res (2014) 113(2):717–21. doi: 10.1007/s00436-013-3700-0
33. Eppert A, Lewis FA, Grzywacz C, Coura-Filho P, Caldas I, Minchella DJ. Distribution of schistosome infections in molluscan hosts at different levels of parasite prevalence. J Parasitol (2002) 88(2):232–6. doi: 10.1645/0022-3395(2002)088[0232:Dosiim]2.0.Co;2
34. Shaw JR, Erasmus DA. Schistosoma Mansoni: An examination of the reproductive status of females from single sex infections. Parasitology (1981) 82(1):121–4. doi: 10.1017/s0031182000041913
35. Smithers SR, Terry RJ. The infection of laboratory hosts with cercariae of Schistosoma mansoni and the recovery of the adult worms. Parasitology (1965) 55(4):695–700. doi: 10.1017/s0031182000086248
36. Li P. The development of Schistosoma japonicum in unisexual and bisexual infections. Acta Zoologica Sin (1959) 11(4):499–506.
37. Zhong H, Ren Y, Qin F, Li X, Hou L, Gu S, et al. Schistosoma japonicum translationally controlled tumour protein, which is associated with the development of female worms, as a target for control of schistosomiasis. Int J Parasitol (2022) 52(8):569–79. doi: 10.1016/j.ijpara.2022.01.005
38. Neves RH, Costa-Silva M, Martinez EM, Branquinho TB, de Oliveira RM, Lenzi HL, et al. Phenotypic plasticity in adult worms of Schistosoma mansoni (trematoda:schistosomatidae) evidenced by brightfield and confocal laser scanning microscopies. Mem Inst Oswaldo Cruz (2004) 99(2):131–6. doi: 10.1590/s0074-02762004000200003
39. Popiel I, Cioli D, Erasmus DA. The morphology and reproductive status of female Schistosoma mansoni following separation from male worms. Int J Parasitol (1984) 14(2):183–90. doi: 10.1016/0020-7519(84)90047-x
40. Armstrong JC. Mating behavior and development of schistosomes in the mouse. J Parasitol (1965) 51:605–16.
41. Beckmann S, Quack T, Burmeister C, Buro C, Long T, Dissous C, et al. Schistosoma mansoni: Signal transduction processes during the development of the reproductive organs. Parasitology (2010) 137(3):497–520. doi: 10.1017/S0031182010000053
42. Liu S, Piao X, Hou N, Cai P, Ma Y, Chen Q. Duplex real-time PCR for sexing Schistosoma japonicum cercariae based on w chromosome-specific genes and its applications. PloS Negl Trop Dis (2020) 14(8):e0008609. doi: 10.1371/journal.pntd.0008609
43. Boissier J, Durand P, Moné H. PCR effectiveness for sexing Schistosoma mansoni cercariae: application for sexing clonal cercarial populations. Mol Biochem Parasitol (2001) 112(1):139–41. doi: 10.1016/s0166-6851(00)00344-3
44. Zhang M, Hong Y, Han Y, Han H, Peng J, Qiu C, et al. Proteomic analysis of tegument-exposed proteins of female and male Schistosoma japonicum worms. J Proteome Res (2013) 12(11):5260–70. doi: 10.1021/pr400476a
45. Li X, Qiao H, Qin F, Cheng G, Liu J, Li H, et al. Comparative analysis of ITRAQ-based proteome profiles of Schistosoma japonicum female worms coming from single-sex infections and bisexual infections. J Proteomics (2020) 213:103597. doi: 10.1016/j.jprot.2019.103597
46. Protasio AV, Tsai IJ, Babbage A, Nichol S, Hunt M, Aslett MA, et al. A systematically improved high quality genome and transcriptome of the human blood fluke Schistosoma mansoni. PloS Negl Trop Dis (2012) 6(1):e1455. doi: 10.1371/journal.pntd.0001455
47. Lu Z, Sessler F, Holroyd N, Hahnel S, Quack T, Berriman M, et al. Schistosome sex matters: a deep view into gonad-specific and pairing-dependent transcriptomes reveals a complex gender interplay. Sci Rep (2016) 6:31150. doi: 10.1038/srep31150
48. Kanehisa M, Goto S. Kegg: kyoto encyclopedia of genes and genomes. Nucleic Acids Res (2000) 28(1):27–30. doi: 10.1093/nar/28.1.27
49. Fitzpatrick JM, Hirai Y, Hirai H, Hoffmann KF. Schistosome egg production is dependent upon the activities of two developmentally regulated tyrosinases. FASEB J (2007) 21(3):823–35. doi: 10.1096/fj.06-7314com
50. Platt TR, Brooks DR. Evolution of the schistosomes (Digenea: schistosomatoidea): the origin of dioecy and colonization of the venous system. J Parasitol (1997) 83(6):1035–44.
51. Lu Z, Zhang Y, Berriman M. A web portal for gene expression across all life stages of. Schistosoma mansoni. bioRxiv (2018) 308213(11). doi: 10.1101/308213
52. Howe KL, Bolt BJ, Cain S, Chan J, Chen WJ, Davis P, et al. Wormbase 2016: expanding to enable helminth genomic research. Nucleic Acids Res (2016) 44(D1):D774–80. doi: 10.1093/nar/gkv1217
53. Wendt G, Zhao L, Chen R, Liu C, O'Donoghue AJ, Caffrey CR, et al. A single-cell RNA-seq atlas of Schistosoma mansoni identifies a key regulator of blood feeding. Science (2020) 369(6511):1644–9. doi: 10.1126/science.abb7709
54. Shang Z, Guo Q, Zhou X, Yue Y, Zhou K, Tang L, et al. Characterization of aspartyl aminopeptidase from Schistosoma japonicum. Acta Trop (2022) 232:106519. doi: 10.1016/j.actatropica.2022.106519
55. Li X, Cheng G, Qin F, Liu J, Li H, Jin Y. Function of the lesswright (Lwr) gene in the growth, development, and reproduction of Schistosoma japonicum. Vet Parasitol (2019) 272:31–9. doi: 10.1016/j.vetpar.2019.06.010
56. Cheng G, Li X, Qin F, Xu R, Zhang Y, Liu J, et al. Functional analysis of the frzb2 gene in Schistosoma japonicum. Vet Res (2019) 50(1):108. doi: 10.1186/s13567-019-0716-1
57. Hong Y, Sun A, Zhang M, Gao F, Han Y, Fu Z, et al. Proteomics analysis of differentially expressed proteins in schistosomula and adult worms of Schistosoma japonicum. Acta Trop (2013) 126(1):1–10. doi: 10.1016/j.actatropica.2012.12.009
58. Liu M, Ju C, Du XF, Shen HM, Wang JP, Li J, et al. Proteomic analysis on cercariae and schistosomula in reference to potential proteases involved in host invasion of Schistosoma japonicum larvae. J Proteome Res (2015) 14(11):4623–34. doi: 10.1021/acs.jproteome.5b00465
59. Wang J, Yu Y, Shen H, Qing T, Zheng Y, Li Q, et al. Dynamic transcriptomes identify biogenic amines and insect-like hormonal regulation for mediating reproduction in Schistosoma japonicum. Nat Commun (2017) 8:14693. doi: 10.1038/ncomms14693
60. Conesa A, Götz S, García-Gómez JM, Terol J, Talón M, Robles M. Blast2go: a universal tool for annotation, visualization and analysis in functional genomics research. Bioinformatics (2005) 21(18):3674–6. doi: 10.1093/bioinformatics/bti610
61. Zhong H, Qin F, Ren Y, Li X, Hou L, Gu S, et al. Functional characterization of differentially expressed proteins coming from unisexual and bisexual infected Schistosoma japonicum female worms. Exp Parasitol (2023) 248:108504. doi: 10.1016/j.exppara.2023.108504
62. Zhai Q, Fu Z, Hong Y, Yu X, Han Q, Lu K, et al. ITRAQ-based comparative proteomic analysis of adult Schistosoma japonicum from water buffalo and yellow cattle. Front Microbiol (2018) 9:99. doi: 10.3389/fmicb.2018.00099
63. Lv L, Wang Y, Feng W, Hernandez JA, Huang W, Zheng Y, et al. ITRAQ-based differential proteomic analysis in mongolian gerbil brains chronically infected with Toxoplasma gondii. J Proteomics (2017) 160:74–83. doi: 10.1016/j.jprot.2017.03.012
64. Bommer UA, Telerman A. Dysregulation of TCTP in biological processes and diseases. Cells (2020) 9(7):1632. doi: 10.3390/cells9071632
65. Lee SR, Hong ST, Choi KW. Regulation of epithelial integrity and organ growth by tctp and coracle in Drosophila. PloS Genet (2020) 16(6):e1008885. doi: 10.1371/journal.pgen.1008885
66. Sotillo J, Pearson MS, Becker L, Mekonnen GG, Amoah AS, van Dam G, et al. In-depth proteomic characterization of Schistosoma haematobium: towards the development of new tools for elimination. PloS Negl Trop Dis (2019) 13(5):e0007362. doi: 10.1371/journal.pntd.0007362
67. Bartel DP. MicroRNAs: genomics, biogenesis, mechanism, and function. Cell (2004) 116(2):281–97. doi: 10.1016/s0092-8674(04)00045-5
68. Yu J, Yu Y, Li Q, Chen M, Shen H, Zhang R, et al. Comprehensive analysis of miRNA profiles reveals the role of Schistosoma japonicum miRNAs at different developmental stages. Vet Res (2019) 50(1):23. doi: 10.1186/s13567-019-0642-2
69. Wang L, Liao Y, Yang R, Yu Z, Zhang L, Zhu Z, et al. Sja-mir-71a in schistosome egg-derived extracellular vesicles suppresses liver fibrosis caused by schistosomiasis via targeting semaphorin 4d. J Extracell Vesicles (2020) 9(1):1785738. doi: 10.1080/20013078.2020.1785738
70. Zhong H, Jin Y. Multifunctional roles of microRNAs in schistosomiasis. Front Microbiol (2022) 13:925386. doi: 10.3389/fmicb.2022.925386
71. Sun J, Wang S, Li C, Ren Y, Wang J. Novel expression profiles of microRNAs suggest that specific miRNAs regulate gene expression for the sexual maturation of female Schistosoma japonicum after pairing. Parasit Vectors (2014) 7:177. doi: 10.1186/1756-3305-7-177
72. Han Y, Feng J, Ren Y, Wu L, Li H, Liu J, et al. Differential expression of microRNA between normally developed and underdeveloped female worms of Schistosoma japonicum. Vet Res (2020) 51(1):126. doi: 10.1186/s13567-020-00851-4
73. Fitzpatrick JM, Hoffmann KF. Dioecious Schistosoma mansoni express divergent gene repertoires regulated by pairing. Int J Parasitol (2006) 36(10-11):1081–9. doi: 10.1016/j.ijpara.2006.06.007
74. Protasio AV, van Dongen S, Collins J, Quintais L, Ribeiro DM, Sessler F, et al. Mir-277/4989 regulate transcriptional landscape during juvenile to adult transition in the parasitic helminth Schistosoma mansoni. PloS Negl Trop Dis (2017) 11(5):e0005559. doi: 10.1371/journal.pntd.0005559
75. Koslowski N, Sombetzki M, Loebermann M, Engelmann R, Grabow N, Osterreicher CH, et al. Single-sex infection with female Schistosoma mansoni cercariae mitigates hepatic fibrosis after secondary infection. PloS Negl Trop Dis (2017) 11(5):e0005595. doi: 10.1371/journal.pntd.0005595
76. Sombetzki M, Koslowski N, Rabes A, Seneberg S, Winkelmann F, Fritzsche C, et al. Host defense versus immunosuppression: unisexual infection with male or female Schistosoma mansoni differentially impacts the immune response against invading cercariae. Front Immunol (2018) 9:861. doi: 10.3389/fimmu.2018.00861
77. Sombetzki M, Rabes A, Bischofsberger M, Winkelmann F, Koslowski N, Schulz C, et al. Preventive ctla-4-Ig treatment reduces hepatic egg load and hepatic fibrosis in Schistosoma mansoni-infected mice. BioMed Res Int (2019) 2019:1704238. doi: 10.1155/2019/1704238
78. Winkelmann F, Rabes A, Reinholdt C, Koslowski N, Koczan D, Reisinger EC, et al. Sex-specific modulation of the host transcriptome in the spleen of Schistosoma mansoni-infected mice. Front Cell Infect Microbiol (2022) 12:893632. doi: 10.3389/fcimb.2022.893632
79. Chen R, Wang J, Gradinaru I, Vu HS, Geboers S, Naidoo J, et al. A male-derived nonribosomal peptide pheromone controls female schistosome development. Cell (2022) 185(9):1506–20.e17. doi: 10.1016/j.cell.2022.03.017
80. Reinholdt C, Winkelmann F, Koslowski N, Reisinger EC, Sombetzki M. Unisexual infection with Schistosoma mansoni in mice has the potential to boost the immune response against eggs after challenge infection. Front Immunol (2023) 14:1125912. doi: 10.3389/fimmu.2023.1125912
81. Pearce EJ, MacDonald AS. The immunobiology of schistosomiasis. Nat Rev Immunol (2002) 2(7):499–511. doi: 10.1038/nri843
82. Wynn TA, Thompson RW, Cheever AW, Mentink-Kane MM. Immunopathogenesis of schistosomiasis. Immunol Rev (2004) 201:156–67. doi: 10.1111/j.0105-2896.2004.00176.x
83. Zhong H, Gui X, Hou L, Lv R, Jin Y. From inflammation to fibrosis: novel insights into the roles of high mobility group protein box 1 in schistosome-induced liver damage. Pathogens (2022) 11(3):289. doi: 10.3390/pathogens11030289
84. Wilson MS, Mentink-Kane MM, Pesce JT, Ramalingam TR, Thompson R, Wynn TA. Immunopathology of schistosomiasis. Immunol Cell Biol (2007) 85(2):148–54. doi: 10.1038/sj.icb.7100014
85. Chuah C, Jones MK, Burke ML, McManus DP, Gobert GN. Cellular and chemokine-mediated regulation in schistosome-induced hepatic pathology. Trends Parasitol (2014) 30(3):141–50. doi: 10.1016/j.pt.2013.12.009
86. Schwartz C, Fallon PG. Schistosoma "eggs-iting" the host: granuloma formation and egg excretion. Front Immunol (2018) 9:2492. doi: 10.3389/fimmu.2018.02492
87. Taylor MD, Harris A, Babayan SA, Bain O, Culshaw A, Allen JE, et al. Ctla-4 and Cd4+ Cd25+ regulatory T cells inhibit protective immunity to filarial parasites. vivo. J Immunol (2007) 179(7):4626–34. doi: 10.4049/jimmunol.179.7.4626
88. Fichtner-Feigl S, Strober W, Kawakami K, Puri RK, Kitani A. Il-13 signaling through the il-13alpha2 receptor is involved in induction of TGF-beta1 production and fibrosis. Nat Med (2006) 12(1):99–106. doi: 10.1038/nm1332
89. Cai YC, Xu JF, Steinmann P, Chen SH, Chu YH, Tian LG, et al. Field comparison of circulating antibody assays versus circulating antigen assays for the detection of schistosomiasis japonica in endemic areas of China. Parasit Vectors (2014) 7:138. doi: 10.1186/1756-3305-7-138
90. Lamberton PH, Kabatereine NB, Oguttu DW, Fenwick A, Webster JP. Sensitivity and specificity of multiple kato-Katz thick smears and a circulating cathodic antigen test for Schistosoma mansoni diagnosis pre- and post-repeated-praziquantel treatment. PloS Negl Trop Dis (2014) 8(9):e3139. doi: 10.1371/journal.pntd.0003139
91. Wang Y, Fan X, Lei N, He X, Wang X, Luo X, et al. A microRNA derived from Schistosoma japonicum promotes schistosomiasis hepatic fibrosis by targeting host secreted frizzled-related protein 1. Front Cell Infect Microbiol (2020) 10:101. doi: 10.3389/fcimb.2020.00101
92. Meningher T, Barsheshet Y, Ofir-Birin Y, Gold D, Brant B, Dekel E, et al. Schistosomal extracellular vesicle-enclosed miRNAs modulate host T helper cell differentiation. EMBO Rep (2020) 21(1):e47882. doi: 10.15252/embr.201947882
93. Liu J, Zhu L, Wang J, Qiu L, Chen Y, Davis RE, et al. Schistosoma japonicum extracellular vesicle miRNA cargo regulates host macrophage functions facilitating parasitism. PloS Pathog (2019) 15(6):e1007817. doi: 10.1371/journal.ppat.1007817
94. He X, Wang Y, Fan X, Lei N, Tian Y, Zhang D, et al. A schistosome miRNA promotes host hepatic fibrosis by targeting transforming growth factor beta receptor III. J Hepatol (2020) 72(3):519–27. doi: 10.1016/j.jhep.2019.10.029
95. Wang Y, Gong W, Zhou H, Hu Y, Wang L, Shen Y, et al. A novel miRNA from egg-derived exosomes of Schistosoma japonicum promotes liver fibrosis in murine schistosomiasis. Frontier Immunol (2022) 13:860807. doi: 10.3389/fimmu.2022.860807
96. Wang J, Chen R, Collins JJ 3rd. Systematically improved in vitro culture conditions reveal new insights into the reproductive biology of the human parasite Schistosoma mansoni. PloS Biol (2019) 17(5):e3000254. doi: 10.1371/journal.pbio.3000254
97. Alexandre-Silva GM, Brito-Souza PA, Oliveira ACS, Cerni FA, Zottich U, Pucca MB. The hygiene hypothesis at a glance: early exposures, immune mechanism and novel therapies. Acta Trop (2018) 188:16–26. doi: 10.1016/j.actatropica.2018.08.032
98. Liu P, Li J, Yang X, Shen Y, Zhu Y, Wang S, et al. Helminth infection inhibits airway allergic reaction and dendritic cells are involved in the modulation process. Parasite Immunol (2010) 32(1):57–66. doi: 10.1111/j.1365-3024.2009.01161.x
99. Osada Y, Horie Y, Nakae S, Sudo K, Kanazawa T. Stat6 and il-10 are required for the anti-arthritic effects of Schistosoma mansoni via different mechanisms. Clin Exp Immunol (2019) 195(1):109–20. doi: 10.1111/cei.13214
100. Liu Y, Ye Q, Liu YL, Kang J, Chen Y, Dong WG. Schistosoma japonicum Attenuates dextran sodium sulfate-induced colitis in mice via reduction of endoplasmic reticulum stress. World J Gastroenterol (2017) 23(31):5700–12. doi: 10.3748/wjg.v23.i31.5700
101. Yan K, Wang B, Zhou H, Luo Q, Shen J, Xu Y, et al. Amelioration of type 1 diabetes by recombinant fructose-1,6-bisphosphate aldolase and cystatin derived from Schistosoma japonicum in a murine model. Parasitol Res (2020) 119(1):203–14. doi: 10.1007/s00436-019-06511-7
102. Tang H, Liang YB, Chen ZB, Du LL, Zeng LJ, Wu JG, et al. Soluble egg antigen activates M2 macrophages via the stat6 and pi3k pathways, and Schistosoma japonicum alternatively activates macrophage polarization to improve the survival rate of septic mice. J Cell Biochem (2017) 118(12):4230–9. doi: 10.1002/jcb.26073
103. Mbanefo EC, Le L, Zee R, Banskota N, Ishida K, Pennington LF, et al. Ipse, a urogenital parasite-derived immunomodulatory protein, ameliorates ifosfamide-induced hemorrhagic cystitis through downregulation of pro-inflammatory pathways. Sci Rep (2019) 9(1):1586. doi: 10.1038/s41598-018-38274-z
104. Jiang P, Wang J, Zhu S, Hu C, Lin Y, Pan W. Identification of a Schistosoma japonicum microRNA that suppresses hepatoma cell growth and migration by targeting host fzd4 gene. Front Cell Infect Microbiol (2022) 12:786543. doi: 10.3389/fcimb.2022.786543
105. Mu Y, McManus DP, Hou N, Cai P. Schistosome infection and schistosome-derived products as modulators for the prevention and alleviation of immunological disorders. Front Immunol (2021) 12:619776. doi: 10.3389/fimmu.2021.619776
106. Janse JJ, Langenberg MCC, Kos-Van Oosterhoud J, Ozir-Fazalalikhan A, Brienen EAT, Winkel BMF, et al. Establishing the production of male Schistosoma mansoni cercariae for a controlled human infection model. J Infect Dis (2018) 218(7):1142–6. doi: 10.1093/infdis/jiy275
Keywords: schistosome, single-sex infection, omics, host-parasite interaction, immune regulation
Citation: Zhong H and Jin Y (2023) Single-sex schistosomiasis: a mini review. Front. Immunol. 14:1158805. doi: 10.3389/fimmu.2023.1158805
Received: 04 February 2023; Accepted: 10 April 2023;
Published: 19 April 2023.
Edited by:
Roberta Lima Caldeira, René Rachou Institute, Oswaldo Cruz Foundation (FIOCRUZ), BrazilReviewed by:
Gabriel Rinaldi, Aberystwyth University, United KingdomWannaporn Ittiprasert, George Washington University, United States
Copyright © 2023 Zhong and Jin. This is an open-access article distributed under the terms of the Creative Commons Attribution License (CC BY). The use, distribution or reproduction in other forums is permitted, provided the original author(s) and the copyright owner(s) are credited and that the original publication in this journal is cited, in accordance with accepted academic practice. No use, distribution or reproduction is permitted which does not comply with these terms.
*Correspondence: Yamei Jin, yameijin@shvri.ac.cn