- 1Ganjiang Chinese Medicine Innovation Center, Nanchang, China
- 2Division of Cancer Epidemiology and Genetics, National Cancer Institute, National Institutes of Health, Rockville, MD, United States
- 3CAS Key Laboratory of Separation Science for Analytical Chemistry, Dalian Institute of Chemical Physics, Chinese Academy of Sciences, Dalian, China
GPR35 is a G protein-coupled receptor with notable involvement in modulating inflammatory responses. Although the precise role of GPR35 in inflammation is not yet fully understood, studies have suggested that it may have both pro- and anti-inflammatory effects depending on the specific cellular environment. Some studies have shown that GPR35 activation can stimulate the production of pro-inflammatory cytokines and facilitate the movement of immune cells towards inflammatory tissues or infected areas. Conversely, other investigations have suggested that GPR35 may possess anti-inflammatory properties in the gastrointestinal tract, liver and certain other tissues by curbing the generation of inflammatory mediators and endorsing the differentiation of regulatory T cells. The intricate role of GPR35 in inflammation underscores the requirement for more in-depth research to thoroughly comprehend its functional mechanisms and its potential significance as a therapeutic target for inflammatory diseases. The purpose of this review is to concurrently investigate the pro-inflammatory and anti-inflammatory roles of GPR35, thus illuminating both facets of this complex issue.
Introduction
Inflammation is a multifaceted physiological reaction that serves a critical role in the immune response against infection, tissue injury, and other provocations. It orchestrates a series of cellular and molecular activities with the ultimate goals of eradicating pathogens, repairing damaged tissues, and reinstating homeostasis (1). However, a dysregulated or persistent inflammatory response can pave the way for numerous diseases, encompassing autoimmune disorders where the body mistakenly attacks its cells, chronic inflammatory conditions that endure over extended periods, and even cancer where abnormal cells proliferate uncontrollably (2–4). The link between inflammation and such diverse diseases underscores the importance of understanding and effectively managing inflammation.
G protein-coupled receptors (GPCRs) constitute a vast family of cell surface receptors that fundamentally mediate cellular responses to a myriad of stimuli, including inflammatory (5, 6). Among these receptors, GPR35 has recently drawn considerable interest due to its suspected role in inflammatory processes. Discovered in 1998, GPR35 is highly expressed in the intestine and various immune cells. Owing to its wide expression and the significance of its function, it is perceived as a prospective therapeutic target for various diseases (7–9).
The involvement of GPR35 in inflammation is complex and context-dependent, demonstrating both pro-inflammatory and anti-inflammatory effects in disparate cellular and tissue settings. GPR35 has been implicated in modulating an array of inflammatory responses, including the activation of immune cells, production of cytokines, and chemotactic movements (10–12). It interacts with several signaling pathways, such as MAPK, NF-κB and others, thereby modulating inflammatory signaling dynamics. Furthermore, the functionality of GPR35 can be influenced by various ligands, ranging from endogenous metabolites to synthetic compounds, thus impacting its downstream effects in the inflammation process (13). Through these complex interactions and functions, GPR35 illustrates the multifactorial nature of inflammation and the intricate molecular dance that enables the body to respond appropriately to internal and external stressors. Yet, it also underscores the delicate balance that must be maintained, as dysregulated signaling via pathways involving GPR35 could potentially lead to pathological inflammation, underscoring the need for further research to elucidate its precise role and regulatory mechanisms.
In this comprehensive review, we explored the current understanding of GPR35, with a particular focus on its role in inflammation. We began by presenting a detailed overview of GPR35, discussing aspects such as its expression pattern, signaling mechanisms, and the variety of ligands it interacts with. From there, we investigated the pro-inflammatory role of GPR35, and its activation influences the immune response, including the impact on immune cell activation, cytokine production, and chemotactic activity within various immune cells such as neutrophils, macrophages, and invariant natural killer T (iNKT) cells. Subsequently, we discussed an in-depth examination of the anti-inflammatory role of GPR35 and covered aspects such as its impact on immune cell signaling, cytokine generation, and the resolution of inflammation. In addition, we explored the intricate signaling pathways and mechanisms underpinning the pro-inflammatory and anti-inflammatory effects that are triggered following GPR35 activation. This comprehensive exploration will serve as a map guiding us through the complicated roles of GPR35 in inflammation, shedding light on its significance and potential therapeutic value.
Expression pattern of GPR35
GPR35 is classified as a class A orphan GPCR compromising nearly 85% of all GPCRs. GPCRs play a pivotal role in metabolite sensing within the intestine, acting as a significant connector linking the microbiota, immune system, and intestinal epithelium (14). Most GPCRs are predicted to be responsible for encoding olfactory receptors, while the rest are divided between receptors that bind with recognized endogenous compounds and those termed as orphan receptors because their specific endogenous activators remain unidentified (15).
Despite various endogenous ligands of GPR35, a definitive endogenous activator has not yet been firmly established, which maintains its status as an orphan receptor (13). Human GPR35 gene is transcribed and translated into three distinct variants (16). Both variants 2 and 3 encode the longer isoform GPR35b, which diverges from GPR35a by possessing an extended N-terminal sequence of 31 additional amino acids, thereby lengthening its extracellular domain. Despite this distinction, the subsequent sequences of the two isoforms are identical. mRNA expression of GPR35a and GPR35b have been identified in human tissues. Notably, GPR35b, which is highly expressed in gastric and colon cancer tissues, may have associations with carcinogenesis (17). Intriguingly, GPR35b demonstrates a lower agonist response efficacy than GPR35a (18). It was reported that the elongated N-terminus of the longer isoform may constrain G protein activation while boosting the interaction with β-arrestin (19).
GPR35 expression pattern suggests its pivotal role in immune responses and inflammation. GPR35 expression is particularly prominent in the small intestine and colon, with moderate but noticeable expression in the stomach, liver, spleen, kidney, and sympathetic neurons (20, 21). This widespread presence across various organ systems indicates its broad functionality within the body. Moreover, GPR35 expression is not limited to these organ systems. It also appears in various immune cells, including monocytes (CD14+), T-cells (CD3+), neutrophils, assorted dendritic cells, and invariant natural killer T cells (22–24), highlighting its integral role in immune responses. Among these, dendritic cells (CD103+/CD11b−) and macrophage clusters from the lamina propria and Peyer’s patch cells in mouse small intestine are noted for their high expression of GPR35 (25).
Furthermore, there is a significant upregulation of GPR35 in neutrophils and intestinal tissues during the invasion of pathogenic microbes, as well as in mast cells during the stimulation of IgE antibodies, indicating a pronounced inflammatory response (21, 26, 27). Additionally, GPR35 presence in epithelial and endothelial cells, which are key participants in the inflammatory response (28), further underscores broad impact across numerous cell types and physiological processes. This ubiquitous distribution and varied presence underscore GPR35 potential as a major player in immune responses and inflammation. GPR35 presents the intriguing features with tissue specificity and different isoforms, taking into consideration when examining the potential of GPR35 as a therapeutic target for inflammation-related conditions.
GPR35 involved inflammation-related pathways
G proteins serve as the primary effector proteins for GPCRs. Typically, G proteins exist as a trimeric complex composed of α, β, and γ subunits. Upon activation of the GPCR, it binds to the Gα subunit and facilitates the exchange of GDP for GTP on Gα. This process leads to the disassociation of Gα from the Gβγ dimer. Following this separation, both Gα and Gβγ can independently initiate their respective signaling pathways (29).
Four principal Gα protein families exist, and GPR35 has been found to couple predominantly with Gα12/13 and Gαi/o proteins (7, 24, 30) (Figure 1A). Gαi/o protein interacts with adenylate cyclase (AC), inhibiting its activity and consequently reducing intracellular cAMP levels, resulting in the dampening of the MAPK/ERK pathway (31). Interestingly, ERK may serve as an anti-inflammatory signal, suppressing the production of NF-κB dependent inflammatory factors through the inhibition of IKK activity (32). Gβγ dimer can activate phospholipase C beta (PLCβ), which results in phosphoinositide generation and activation of the PI3K/AKT pathway. This leads to the activation of a host of downstream transcription factors, notably NF-κB, a major player in the expression of genes encoding inflammatory factors (33). In the case of Gα12/13, activation leads to Rho-mediated cytoskeleton reorganization, collaborating with Gβγ to regulate immune cell chemotaxis to inflammation sites - these actions primarily result in pro-inflammatory effects (34, 35). Gαq coupled with GPR35 directly inhibits the PI3K catalytic subunit and then suppresses Akt activation. Additionally, Gαq plays a role in the activation of ERK through the PLCβ/Ca2+/Src signaling cascade (19, 36).
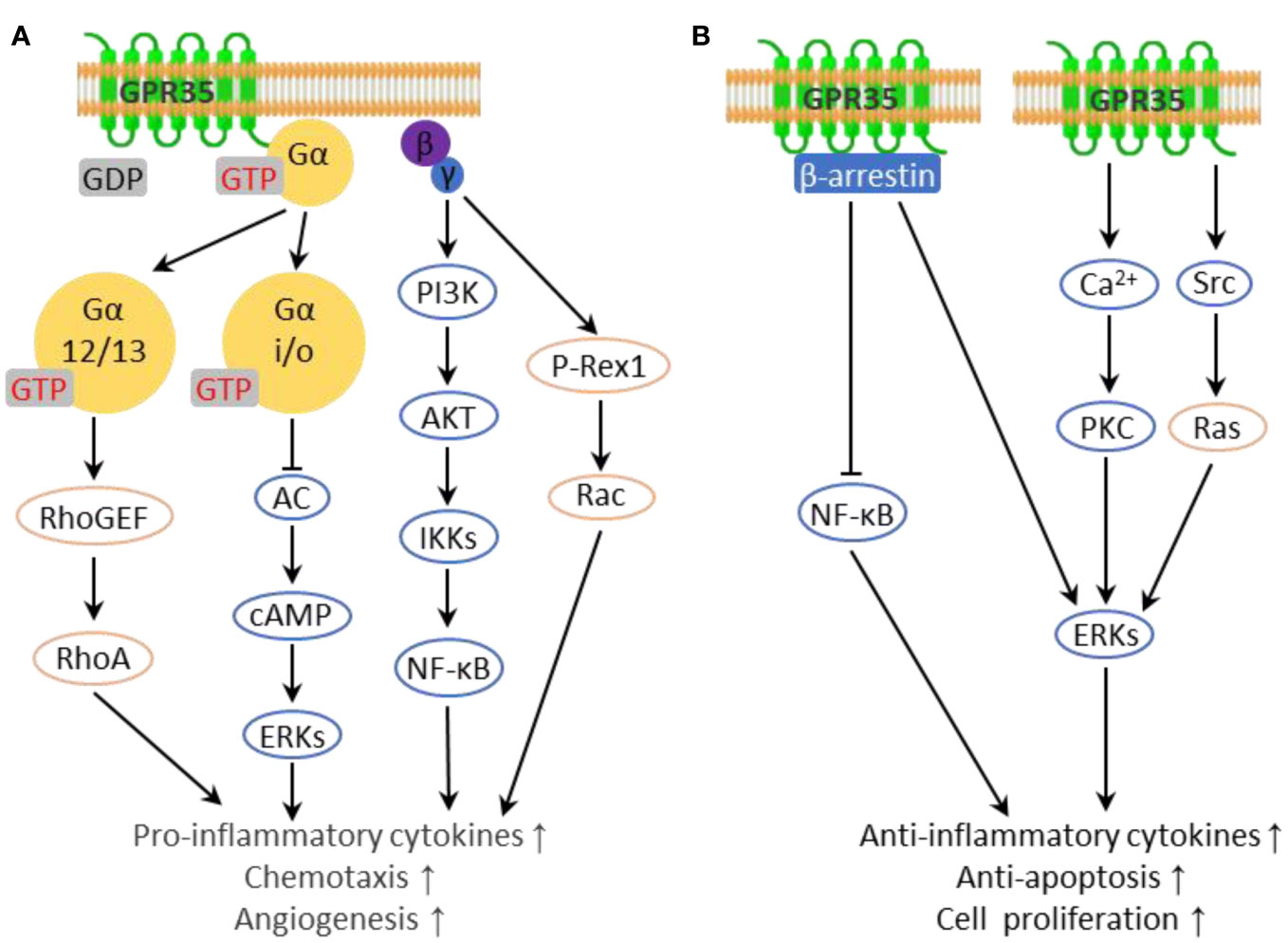
Figure 1 Inflammation-related downstream pathways of GPR35. (A) G proteins interacting with GPR35 transmit pro-inflammatory downstream pathways. (B) GPR35 transmits anti-inflammatory downstream pathways.
GPR35 has been demonstrated to directly engage with β-arrestin upon activation by agonists, leading to its internalization and desensitization (37). Beyond that, β-arrestins also function as signaling scaffolds, interacting with various pathways including the c-Jun N-terminal kinase, protein kinase B, and the extracellular signal-regulated kinase (ERK1/2) pathway in a G protein independent manner, leading to anti-inflammatory effects (38–40). In addition, β-arrestins interact with IκBα, leading to the suppression of NF-κB activation (41). It is worth noting that there are certain ligands capable of activating GPR35 without facilitating the interaction between GPR35 and β-arrestin, such as kynurenic acid (19, 42), 5-HIAA (42) and DHICA analogues (43). Moreover, GPR35 interacts with the sodium-potassium pump (Na/K-ATPase), a vital regulator of cellular electrochemical gradients and Src family kinase signaling (44). This interaction enhances Na/K-ATPase pump function, aiding in the regulation of Ca2+ homeostasis. Also, Na/K-ATPase directly activates the kinase Src, leading to ERK activation in macrophages and enterocytes (45). Given that elevated cytoplasmic Ca2+ levels promote ERK and NF-κB activation via PKC in inflammation, alterations in Ca2+ homeostasis have context-dependent impacts on inflammation (46, 47) (Figure 1B).
Furthermore, GPR35 has also been reported to modulate the production of reactive oxygen species (ROS), key mediators of inflammation. Under mechanical stress, GPR35 activation has been shown to enhance ROS production, and excessive ROS triggers further GPR35 expression (48). Conversely, GPR35 activation with Kynurenic acid in macrophages suppresses NLRP3 inflammasome activation and related inflammation by reducing mitochondrial damage and mitochondrial ROS production (49). These studies, despite showing divergent outcomes, reveal the significant role of GPR35 in modulating inflammation via ROS-mediated pathways.
In sum, a growing body of evidence proposes a sophisticated and intricate role of GPR35 in inflammation. It appears to exert both pro-inflammatory and anti-inflammatory influences, which depend on the type of cell, the specific signaling pathways, and the availability of ligands. The precise mechanisms through which GPR35 impacts inflammation-related pathways, however, remain somewhat elusive. Therefore, it necessitates additional research to comprehensively understand the subtleties of its signaling.
Primary ligands with activity at GPR35
A pivotal element influencing the activity of GPR35 are its ligands, otherwise referred to as agonists and antagonists. Agonists are molecules that initiate signaling by binding to the receptor, thereby triggering downstream cellular responses. Conversely, antagonists exert opposing effects. Furthermore, the activation of receptors is not always fully “on or off” scenario while some agonists can activate the corresponding receptors in a biased manner. For instance, in the case of the kynurenic acid/GPR35 axis, while kynurenic acid triggers the activation of G proteins downstream of GPR35, it scarcely facilitates the interaction between GPR35 and β-arrestin (42, 43).
There are multiple single nucleotide polymorphisms (SNPs) located within the GPR35 gene linked with various immune and inflammation-related diseases, such as inflammatory bowel diseases, ankylosing spondylitis, and primary sclerosing cholangitis (9). One of the most prevalent variants, rs3749171, induces the replacement of a threonine with methionine in transmembrane domain III (T108M), demonstrating a significant correlation with inflammatory bowel disease (50). Although most SNP-induced variations do not significantly affect the potency of agonist ligands, the V76M variant (rs13387859) does exhibit a reduction in agonist potency (51). While this variant is present at a 2% allele frequency, it has not been associated with any disease.
In recent years, a multitude of both endogenous and synthetic GPR35 ligands have been discovered, enriching our understanding of this involvement in inflammation and a host of other biological phenomena (Table 1).
Endogenous agonists
Kynurenic acid, a metabolite derived from tryptophan, is noted for its roles in the central nervous system, as well as anti-inflammatory property (73, 74). Its ability to activate GPR35 is significantly more potent in mice and rats compared to humans, where it requires 40- to 100-fold higher concentrations (20), occasionally failing to activate human GPR35 at high doses (27, 54). This species-selective aspect of the kynurenic acid/GPR35 axis has stirred discussion on whether this interaction is truly physiological, particularly in humans, leaving kynurenic acid as a potential endogenous ligand for GPR35 (7). Recently, it was demonstrated that pre-treatment with kynurenic acid mitigated the injuries sustained by both human iPS-cardiomyocytes and mouse cardiomyocytes following simulated ischemia/reperfusion (I/R) ex vivo (8). In mice, kynurenic acid stimulates the migration of CX3CR1+/GPR35+ macrophages in the small intestine, but not GPR35- macrophages (75).
Lysophosphatidic acid (LPA), an active phospholipid derivative, is present in cell membranes and can be produced extracellularly to activate six known GPCRs including LPAR1-6 (76). It was displayed that the Ca2+ response triggered by 2-acyl LPA was significantly stronger in GPR35-expressed HEK293 cells compared to the response in the control cells, which pronounced difference was not observed when 1-acyl LPA was applied (54). GPR35 deficiency was found to inhibit LPA-induced Ca2+ signaling in bone marrow-derived macrophages (BMDMs). However, it was posited that GPR35 deficiency might compromise LPA signaling via other LPA receptors (45). Recently, GPR35 was affirmed as a potential LPA receptor linked to an inhibitory G protein (Gi) (27). However, there LPA failed to activate GPR35 in other experimental settings, which leaves the question open on whether LPA acts as an endogenous agonist for GPR35 (26, 77).
CXCL17, a homeostatic chemokine in mucosa, attracts dendritic cells and macrophages but can be expressed elsewhere during inflammation (78). It was reported that CXCL17 influenced GPR35 at nanomolar levels within a physiological range, unlike kynurenic acid (56). However, subsequent studies failed to show that CXCL17 induced migration or signaling responses in GPR35-expressing cells (79, 80). Further, the actions of CXCL17 in a neuropathic pain model in mice were decreased by kynurenic acid and zaprinast, suggesting the presence of a CXCL17 receptor other than GPR35 (81).
Recent findings indicate that 5-Hydroxyindoleacetic acid (5-HIAA), a metabolite of 5-hydroxytryptamine generated by activated platelets and extravascular mast cells, can act as an agonist for GPR35, promoting the recruitment of neutrophils (26) and the migration of eosinophils (82). Interestingly, this perspective offers fresh insight into the role GPR35 regulating pain sensations due to the association of 5-HIAA with pain and sensory neurons (83). Additionally, GPR35 plays a role in modulating cAMP production and inhibiting N-type Ca2+ channels in neurons and astrocytes, showcasing its potential involvement in pain management treatments (84). Moreover, other endogenous molecules such as 5,6-dihydroxyindole-2-carboxylic acid (DHICA), reverse T3 (3,3,5- triiodothyronine), and cyclic guanosine 3′-5′ monophosphate (cGMP) have demonstrated a degree of activity towards GPR35, albeit with modest potency. However, these observations require further validation (40, 58).
Synthetic agonists
Zaprinast (2-(2-propyloxyphenyl) -8-azapurin-6-one), originally identified as a cGMP phosphodiesterase inhibitor, is one of the earliest discovered GPR35 ligands. Notably, the effects of Zaprinast on GPR35 can be separated from its cGMP phosphodiesterase inhibition properties with the intracellular calcium mobilization (59). With its moderate-to-high potency across human, mouse, and rat orthologs, Zaprinast has established its status as a go-to reference agonist for GPR35 research (30, 72). Its efficacy and wide applicability across different species have aided in comparative studies and offered insights into the roles GPR35 plays in a myriad of biological processes.
Pamoic acid (5-nitro-2-(3-phenylproplyamino) benzoic acid) emerged as a potential GPR35 ligand following screening exercises within the Prestwick Chemical Library. However, its activity at rat and mouse orthologs of GPR35 is noticeably less potent compared to its interaction with the human version. This lower efficacy significantly impedes its usage in these preclinical studies (30, 60). Despite this limitation, the discovery of pamoic acid interaction with GPR35 has contributed to the expanding catalog of ligands and may potentially prompt further development of more effective analogs, thereby expanding our understanding of GPR35 physiological role.
YE120 (2-(3-cyano-5-(3,4-dichlorophenyl)-4,5-dimethylfuran-2(5H)-ylidene) malononitrile) is another compound that was identified as a GPR35 agonist through dynamic mass redistribution (DMR) assays performed in the native cell line HT-29. Remarkably, it has demonstrated superior potency compared to zaprinast (62). This discovery indicates the continuing progress in our understanding of GPR35, providing a new promising candidate for the functionality and potentially enabling the development of more effective therapeutic strategies targeting GPR35.
Lodoxamide, a widely used anti-inflammatory mast cell stabilizer, is another synthetic agonist that targets GPR35. Its application in the treatment of allergic keratoconjunctivitis attests to its significant role in modulating inflammatory responses. However, despite its high potency towards human and rat GPR35, the effectiveness of lodoxamide is notably diminished to for the mouse orthologue, with its potency being a 100-fold lower (51, 85). This highlights the importance of species-specific investigations in understanding the precise role of GPR35 and its ligands in mediating inflammatory responses. Recently, there was a significant development in understanding the structural attributes of GPR35 through its structure when bound to a GPR35 agonist lodoxamide, which shows a novel site for divalent cation coordination and a distinctive ionic regulatory mechanism. This helps understanding the affinity of GPR35 for other anti-asthma and anti-allergy agents, especially those featuring symmetric diacid structures like lodoxamide. providing a clear pathway for the binding process (86)
Aminosalicylates, a first-line treatment for inflammatory bowel diseases (IBDs), have shown activity on both human and mouse GPR35, although their exact target remains undefined. Of these, the pro-drug olsalazine exhibits the greatest potency in terms of GPR35 agonism, promoting ERK phosphorylation and the translocation of β-arrestin2. Notably, in a model of dextran sodium sulfate (DSS)-induced colitis, the protective effects of olsalazine on disease progression and its inhibitory effect on TNFα mRNA expression, as well as the NF-κB and JAK-STAT3 pathways, are significantly reduced in mice with GPR35 knockout, thus suggesting a critical role of GPR35 in these anti-inflammatory actions (70).
Recently, a group of 2H-chromen-2-one derivatives has been identified as agonists for GPR35 using dynamic mass redistribution assays in HT-29 cells. The compound 6-Bromo-7-hydroxy-8-nitro-3-(1H-tetrazol-5-yl)-2H-chromen-2-one (Compound 50) emerged as the most potent GPR35 agonist with an EC50 of 5.8 nM (71). In another development, GPR35 fluorescent probes were designed based on known GPR35 agonists. These serve as valuable tools for GPR35 research and for the discovery of new synthetic GPR35 agonists. The most promising compound from this series exhibited the highest binding potency, along with a minimal nonspecific Bioluminescence Resonance Energy Transfer (BRET) binding signal, with a Kd value of 3.9 nM (87).
Antagonists
CID2745687, known as methyl-5- [(tert-butylcarbamothioylhydrazinylidene)methyl] -1-(2,4- difluorophenyl)pyrazole-4-carboxylate, is a well-known antagonist of GPR35. It demonstrates the ability to obstruct the effects of the agonists pamoic acid and zaprinast in cells expressing either variant of human GPR35a or GPR35b. It is estimated that the inhibitor constant Ki is in the range of 10-20 nM, suggesting potential competitive function in a competitive manner (60). CID2745687 has a higher affinity for human GPR35 compared to its mouse and rat counterparts, limiting its application primarily to in vitro studies rather than rodent models (88). Another antagonist of GPR35 is ML-145, or 2-hydroxy-4- [4-(5Z)-5-[(E)-2-methyl-3- phenylprop-2-enylidene] -4-oxo-2-sulfanylidene-1,3- thiazolidin-3-yl] butanoylamino] benzoic acid. This compound also demonstrates high affinity for human GPR35 but shows appreciable affinity for the mouse and rat orthologues (72).
These antagonists and their specificity for the human GPR35 over rodent versions provide valuable insights into the diverse functionality and pharmacology of GPR35. They underline the challenges of studying GPR35 across different species but also offer opportunities for the development of novel therapeutic agents targeting the human GPR35 receptor.
Pro-inflammatory roles of GPR35
GPR35 activation has been demonstrated to induce a pro-inflammatory state within cells, leading to increased production of pro-inflammatory cytokines and chemokines, which act as signal carriers, promoting the recruitment and activation of specific types of immune cells to the inflammation site (Figure 2). This process, in essence, highlights a crucial role of GPR35 in orchestrating immune responses, contributing to the exacerbation of inflammation, and potentially influencing the progression of various inflammatory conditions and diseases. There have been two diseases that are connected to the pro-inflammatory effects of GPR35. GPR35 plays a vital role in detecting bacteroides fragilis toxin and triggering an immune response in bacteroides fragilis toxin-induced colitis (89), and GPR35 loss in nucleus pulposus cells significantly reduces intervertebral disc degeneration typically triggered by inflammation induced by ROS or mechanical stress (49). In addition, encephalomyelitis (EAE) is worsened through the accumulation of GPR35+/Ly6C+ macrophages in the small intestine, while the blockage of KYNA-GPR35 signaling can alleviate EAE (75).
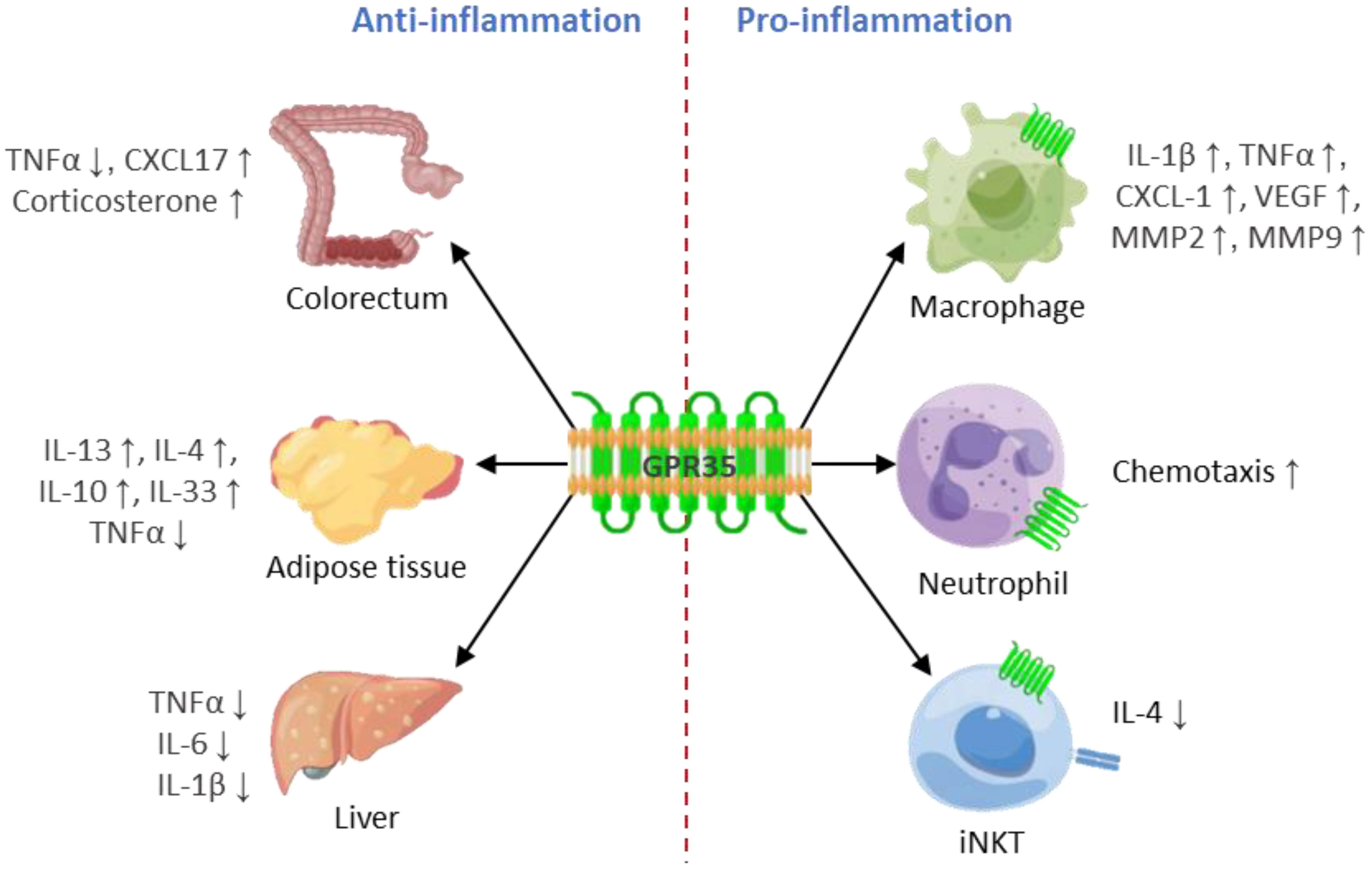
Figure 2 GPR35 plays a double-edged sword role in inflammation. GPR35 plays an anti-inflammatory role in colorectum, adipose tissue and liver, while plays a pro-inflammatory role in macrophages, neutrophils and iNKT.
GPR35 plays a pro-inflammatory role in neutrophils
Neutrophils, the most populous immune cells in human blood, act as first responders in the face of tissue damage and pathogenic infections. The journey of neutrophils from blood vessels to target tissues is complex, traditionally classified into four stages: 1. The egress of neutrophils from bone marrow into the bloodstream. 2. A multi-step adhesion cascade resulting in the congregation of adherent neutrophils in microvessels. 3. Diapedesis of neutrophils across the vascular wall, granting access to the extravascular space. 4. Interstitial migration of neutrophils to their ultimate target sites (90). Various small molecules and cytokines orchestrate these stages, resulting in a highly redundant regulatory system, which complicates the task of identifying dominant chemokines (91).
Interestingly, GPR35 expression is observed to be relatively low in inactive neutrophils, while it increases substantially in activated neutrophils, which aligns with the role of GPR35 in promoting neutrophil recruitment to inflammatory tissues (26). While GPR35 deficiency was found to somewhat dampen neutrophil adherence to blood vessel walls, this effect was not significantly observed in animal studies. Nevertheless, the role of GPR35 in neutrophil diapedesis has been identified as non-redundant (92). Moreover, 5-HIAA produced by activated platelets and extravascular mast cells, can activate GPR35 as an agonist, thus encouraging the recruitment of neutrophils (93).
In summary, through the complex interactions of these various mechanisms, GPR35 plays a significant role in promoting an inflammatory response in neutrophils by facilitating neutrophil recruitment, reinforcing its central role in the body response to inflammation.
GPR35 plays a pro-inflammatory role in macrophages
Macrophages are specialized cells derived from monocytes in the bloodstream, and they play crucial roles in identifying, engulfing, and destroying bacteria, thereby initiating inflammation. These activated macrophages are classified into two types based on their involvement in inflammation: pro-inflammatory (M1) and anti-inflammatory (M2). M1 macrophages secrete pro-inflammatory cytokines such as IL-1, IL-6, TNF-α, as well as metalloproteinases MMP-2 and MMP-9, which help to reshape the extracellular matrix at inflammation sites. However, M2 macrophages produce IL-10, PDGF, IGF-1, TGF-β, and other cytokines that suppress inflammation and foster tissue repair (94–96).
In vitro experiments reveal that the absence of GPR35 does not influence the differentiation of macrophages into M1 and M2 subtypes. However, the level of secreted substances such as CXCL-1, VEGF, MMP2, and MMP9 is reduced in GPR35-deficient macrophages derived from mice with GPR35 deletion, specifically the LysM+ cells (44, 45). When observing macrophages in the colon, a notable difference in cytokine expression was seen between GPR35+/+ and GPR35-/- macrophages. Proinflammatory cytokines like IL-1 and TNF were highly expressed in GPR35+/+ macrophages. Moreover, GPR35 was predominantly expressed in monocyte subgroups exhibiting high to medium levels of Ly6C in the intestine, with the level of intestinal TNF significantly dropping in GPR35-/- macrophages (27). Ly6C+ macrophages, differentiated from monocytes expressing high to medium levels of Ly6C, are known to be pro-inflammatory (97).
Additionally, GPR35 deficiency hinders the adhesion of human peripheral monocytes induced by kynurenic acid (20). In contrast, the activation of GPR35 elevates the infiltration level of macrophages in gastric tissues (11). Taken together, it becomes evident that GPR35 contributes to the pro-inflammatory phenotype of macrophages.
GPR35 has pro-inflammatory function in iNKT cells
Human iNKT cells constitute a unique subset of T cells that feature an invariant alpha-beta T-cell receptor (TCR) along with numerous surface molecules characteristic of natural killer (NK) cells. These iNKT cells can become activated either directly through the engagement of the invariant TCR with glycolipid antigens and CD1d, or indirectly through activated antigen-presenting cell (98). Notably, iNKT cells are critical immunoregulatory components capable of rapid, abundant cytokine production, thus influencing the behavior of other immune cells (99). iNKT cells have been found to express GPR35 at high levels. Following receptor activation, GPR35 undergoes internalization within these iNKT cells. It has been observed that specific GPR35 agonists significantly decrease the release of Interleukin-4 (IL-4), but not Interferon gamma (IFN-γ) (22). IL-4 secretion by iNKT cells plays a crucial role in anti-inflammatory processes, such as M2 polarization of macrophages, the transition of monocytes (from Ly6Chi to Ly6Clo), and tissue repair, particularly in the liver (100–102). Thus, the activation of GPR35 in iNKT cells, which in turn reduces the release of IL-4, has the potential to stimulate inflammation.
Overall, it is increasingly apparent that GPR35 can play a pro-inflammatory role under certain circumstances. This is evidenced by the role of GPR35 in the stimulation of pro-inflammatory cytokines and chemokines production, the activation of inflammatory signaling pathways, and the amplification of pro-inflammatory responses in particular cell types. However, it is crucial to emphasize that the pro-inflammatory effects of GPR35 may be modulated by various factors. These include the microenvironment in which the receptor operates, the existence of other receptor types and signaling pathways, as well as the specific cellular type expressing GPR35. Further in-depth research is required to unravel the underlying mechanisms and conclusively establish the exact role of GPR35 within the context of inflammation. The complexity of these dynamics underscores the intricate interplay of processes within our immune responses and the need for a comprehensive understanding of GPR35.
Anti-inflammatory role of GPR35
Although GPR35 is predominantly linked with pro-inflammatory actions across a range of immune cells, recent studies reveal its potential anti-inflammatory capabilities under certain circumstances. This perspective brings to light the anti-inflammatory roles of GPR35 (Figure 2), enriching our understanding of its part in maintaining immune homeostasis and potentially revealing novel therapeutic targets for treating various inflammatory conditions.
GPR35 displays an anti-inflammatory role, particularly in colonic inflammation. It is prominently expressed in the gastrointestinal tract, playing a pivotal role in regulating the healing process of gastrointestinal injuries. This means GPR35 can inhibit inflammation by maintaining gastrointestinal homeostasis. In fact, GPR35 demonstrates protective qualities in scenarios such as DSS-induced colitis (27, 63, 103). GPR35 agonists such as YE120, zaprinast, and pamoic acid have been shown to expedite wound repair in mouse colon epithelial cells by boosting cell migration through the augmentation of fibronectin expression and ERK phosphorylation (63). Interestingly, the recruitment of β-arrestin 2 and the activation of ERK1/2, mediated by pamoic acid, can be obstructed by the GPR35 antagonist CID2745687. The endogenous ligand of GPR35, 5-HIAA, has been recognized to mitigate the symptoms of ulcerative colitis, while the precise role of GPR35 was not delineated further (104). This insight suggests a potential therapeutic strategy, hinting that manipulating the activity of GPR35 could control the inflammatory response in colonic tissues (60).
GPR35 deficiency in intestinal epithelial cells leads to the reduction in both the number of goblet cells and the expression of Muc2, which occurs due to an increase in the pyroptosis of goblet cells (77). Pyroptosis is a form of cell death that contributes to inflammation, thus exacerbating inflammatory conditions. In consequence of this, the epithelial barrier is weakened, leading to increased susceptibility to infections such as those caused by Citrobacter rodentium (105, 106). In DSS-induced colitis mouse models, the specific deletion of GPR35 in macrophages resulted in elevated inflammation associated with a decrease in TNF production in macrophages (27). Although TNF is generally recognized for its pro-inflammatory role and is routinely targeted in treatments for IBD (107), it also serves an anti-inflammatory function. Specifically, it can induce the production of corticosterone in intestinal epithelial cells, a hormone that plays a key role in mitigating inflammation. The interplay between GPR35 and TNF provides new insights into the mechanisms through which inflammation can be regulated and controlled in gastrointestinal disorders (108).
Recently, it was discovered that when GPR35 was eliminated in the liver of non-alcoholic fatty liver disease (NAFLD) mice, there was an increase in inflammatory cytokines such as TNFα, IL6, and IL1β in the liver. This led to a worsening of hepatitis. However, the overexpression of GPR35 in the liver reversed these effects, hinting at its potential anti-inflammatory role in liver disease (109). Additionally, Kynurenic acid was found to improve energy metabolism and reduce inflammation in mice fed with a high-fat diet. However, TNF in adipose tissue increased in the absence of GPR35, and the anti-inflammatory effects of kynurenic acid were abolished via reducing anti-inflammatory cytokines such as IL-13, IL-4, IL-10, and IL-33 (74). This finding suggests that GPR35 might also exhibit anti-inflammatory effects in adipose tissue. Therefore, understanding the precise function of GPR35 could provide novel insights into inflammation in diseases like NAFLD and obesity. Interestingly, it is worth noting that kynurenic acid is recognized for its anti-inflammatory effects (74, 110, 111). However, kynurenic acid is known to interact with multiple receptors, not just GPR35, including α7 nicotinic acetylcholine receptor (a7nAChR), the aryl hydrocarbon receptor (AhR), and ionotropic glutamate receptors (112). Therefore, it is challenging to discern the specific influence of GPR35 in the observed anti-inflammatory effects of kynurenic acid.
In various tissues, different types of cells contribute to the anti-inflammatory effects orchestrated by GPR35. In the colorectum, it mainly operates through epithelial cells and goblet cells to exert anti-inflammatory effects. While it is known that GPR35 also facilitates anti-inflammatory in both the adipose tissue and the liver, the specific cell types involved in these processes have yet to be identified. When looking at immune cells, it was highlighted that the activation of GPR35 with kynurenic acid in macrophages leads to the suppression of NLRP3 inflammasome activation, consequently reducing related inflammation (49). Moreover, it was revealed that GPR35, along with its platelet- and mast-cell-derived ligand 5-HIAA, facilitates eosinophils recruitment to the lungs infected with cryptococcus neoformans and exacerbation of disease (82).
Overall, activation of GPR35 has been demonstrated to suppress the production of pro-inflammatory cytokines, stimulate the output of anti-inflammatory cytokines in a variety of tissues and organs, and fortify the integrity of the gut barrier. These findings highlight the multifaceted and context-dependent nature of GPR35 in inflammation. Nonetheless, a full understanding of the intricacies involved in GPR35 in counteracting anti-inflammatory demands further investigation.
Conclusion and discussion
GPR35 is a distinctive G protein-coupled receptor with a significant role in the regulation of inflammation, demonstrating both pro-inflammatory and anti-inflammatory properties. Its pro-inflammatory effects have been witnessed in neutrophils, macrophages, and iNKT cells, while its anti-inflammatory attributes have been noted in varying contexts, including those in the colorectum, adipose tissue, and liver. Furthermore, GPR35 is increasingly recognized for its role in cancer and the related immune response. It facilitates angiogenesis within the tumor microenvironment, and GPR35 elimination in macrophages results in reduced immune cell infiltration in colon tumors (44). It was found that activating GPR35 in group 2 innate lymphoid cells fosters an immunosuppressive environment in lung cancer, thereby advancing the progression of lung cancer (113, 114). Consequently, GPR35 exhibits promising potential as a therapeutic target in cancer immunotherapy.
The question arises, “Could GPR35 be a potential target for treating inflammation-associated diseases?” The dual role of GPR35 in inflammation could have considerable consequences for devising therapeutic strategies centered on this receptor. For instance, the use of GPR35 agonists or antagonists could serve to regulate inflammatory responses in diverse diseases. In conditions characterized by excessive inflammation, such as IBD, inhibiting the pro-inflammatory actions of GPR35 could be advantageous. Conversely, in conditions requiring inflammation, such as wound healing or tissue repair, augmenting the anti-inflammatory effects of GPR35 activation may be beneficial. However, considering the intricate nature of GPR35 in inflammation, more comprehensive research is required to determine its exact impact and potential as a therapeutic target.
Currently, only two drugs targeting GPR35 have progressed to clinical trials. The first, Lodoxamide, has been employed for the treatment of allergic keratocon junctivitis (51), while the other, sodium cromoglycate (also known as RVT-1601 or PA101), is being used for treating idiopathic pulmonary fibrosis and chronic cough (115). Despite this, the potential for GPR35-focused treatment remains promising. GPR35 offers several advantages as a therapeutic target. Firstly, as a receptor situated on the cell surface, it is at the top of the signal transduction pathway. This is particularly significant given the numerous redundancies and compensatory mechanisms that exist within inflammation-related signal pathways, which can minimize these effects at the starting point.
Furthermore, GPR35 exhibits a tissue-specific expression pattern with high expression levels observed in immune cells and the gastrointestinal tract. The robust expression of GPR35 in immune cells aligns with its role in the regulation of inflammation. The substantial presence of GPR35 in the gastrointestinal tract, along with the strong correlation between GPR35 mutation and IBD, has led to the consideration of GPR35 as a potential target for IBD treatment. However, it is important to acknowledge the dual role GPR35 plays in IBD. While inhibiting GPR35 activity can diminish the proinflammatory response of macrophages and neutrophils, it may concurrently hinder the repair of gastrointestinal damage. Consequently, the risk-benefit balance of targeting GPR35 for IBD treatment remains somewhat elusive and requires further investigation.
The precise mechanisms driving the dual roles of GPR35 in promoting and inhibiting inflammation across various cells and tissues remain unclear and necessitate more in-depth research. The effects of GPR35 activation on inflammatory responses are likely shaped by numerous factors. These include cell types, tissue microenvironments, and the co-existence of other signaling pathways. Additionally, the expression levels and functional activity of GPR35 agonists may also be instrumental in steering the direction of the inflammatory response. In conclusion, GPR35 is a versatile receptor that can adopt either pro-inflammatory or anti-inflammatory stances within distinct immune cells and tissues. This warrants additional comprehensive research into GPR35 intricacies. Recently, the unveiling of the first protein structure of GPR35 marked a significant advancement in our understanding of GPR35 (86). As our knowledge of GPR35 deepens, we can anticipate the development and application of a broader range of GPR35-targeted drugs in the future.
Author contributions
YW: Writing – original draft. PZ: Writing – original draft, Writing – review & editing. HF: Writing – review & editing. CZ: Writing – review & editing. PY: Writing – review & editing. XL: Conceptualization, Supervision. YC: Writing – review & editing, Conceptualization, Funding acquisition, Supervision.
Funding
The author(s) declare financial support was received for the research, authorship, and/or publication of this article. This work was supported by the Natural Science Foundation of Jiangxi, China (20232BBH80012, 20224ACB216012), Natural Science Foundation of Liaoning, China (2022-MS-017) and Dalian High-Level Talent Support Program (2021RQ003).
Conflict of interest
The authors declare that the research was conducted in the absence of any commercial or financial relationships that could be construed as a potential conflict of interest.
Publisher’s note
All claims expressed in this article are solely those of the authors and do not necessarily represent those of their affiliated organizations, or those of the publisher, the editors and the reviewers. Any product that may be evaluated in this article, or claim that may be made by its manufacturer, is not guaranteed or endorsed by the publisher.
References
1. Weavers H, Martin P. The cell biology of inflammation: From common traits to remarkable immunological adaptations. J Cell Biol (2020) 219(7):e202004003. doi: 10.1083/jcb.202004003
2. Pagan AJ, Ramakrishnan L. The formation and function of granulomas. Annu Rev Immunol (2018) 36:639–65. doi: 10.1146/annurev-immunol-032712-100022
3. Gurevich DB, French KE, Collin JD, Cross SJ, Martin P. Live imaging the foreign body response in zebrafish reveals how dampening inflammation reduces fibrosis. J Cell Sci (2019) 133(5). doi: 10.1242/jcs.236075
4. Sofias AM, De Lorenzi F, Pena Q, Azadkhah Shalmani A, Vucur M, Wang JW, et al. Therapeutic and diagnostic targeting of fibrosis in metabolic, proliferative and viral disorders. Adv Drug Delivery Rev (2021) 175:113831. doi: 10.1016/j.addr.2021.113831
5. Seyedabadi M, Ghahremani MH, Albert PR. Biased signaling of G protein coupled receptors (GPCRs): Molecular determinants of GPCR/transducer selectivity and therapeutic potential. Pharmacol Ther (2019) 200:148–78. doi: 10.1016/j.pharmthera.2019.05.006
6. Insel PA, Snead A, Murray F, Zhang L, Yokouchi H, Katakia T, et al. GPCR expression in tissues and cells: are the optimal receptors being used as drug targets? Br J Pharmacol (2012) 165(6):1613–6. doi: 10.1111/j.1476-5381.2011.01434.x
7. Milligan G. Orthologue selectivity and ligand bias: translating the pharmacology of GPR35. Trends Pharmacol Sci (2011) 32(5):317–25. doi: 10.1016/j.tips.2011.02.002
8. Wyant GA, Yu W, Doulamis IP, Nomoto RS, Saeed MY, Duignan T, et al. Mitochondrial remodeling and ischemic protection by G protein-coupled receptor 35 agonists. Science (2022) 377(6606):621–9. doi: 10.1126/science.abm1638
9. Quon T, Lin LC, Ganguly A, Tobin AB, Milligan G. Therapeutic opportunities and challenges in targeting the orphan G protein-coupled receptor GPR35. ACS Pharmacol Transl Sci (2020) 3(5):801–12. doi: 10.1021/acsptsci.0c00079
10. Im DS. Recent advances in GPR35 pharmacology; 5-HIAA serotonin metabolite becomes a ligand. Arch Pharm Res (2023) 46(6):550–63. doi: 10.1007/s12272-023-01449-y
11. Shu C, Wang C, Chen S, Huang X, Cui J, Li W, et al. ERR-activated GPR35 promotes immune infiltration level of macrophages in gastric cancer tissues. Cell Death Discovery (2022) 8(1):444. doi: 10.1038/s41420-022-01238-4
12. Kaya B, Melhem H, Niess JH. GPR35 in intestinal diseases: from risk gene to function. Front Immunol (2021) 12:717392. doi: 10.3389/fimmu.2021.717392
13. Milligan G. GPR35: from enigma to therapeutic target. Trends Pharmacol Sci (2023) 44(5):263–73. doi: 10.1016/j.tips.2023.03.001
14. Feng Z, Sun R, Cong Y, Liu Z. Critical roles of G protein-coupled receptors in regulating intestinal homeostasis and inflammatory bowel disease. Mucosal Immunol (2022) 15(5):819–28. doi: 10.1038/s41385-022-00538-3
15. Zhou Q, Yang D, Wu M, Guo Y, Guo W, Zhong L, et al. Common activation mechanism of class A GPCRs. Elife (2019) 8:e50279. doi: 10.7554/eLife.50279
16. Okumura S, Baba H, Kumada T, Nanmoku K, Nakajima H, Nakane Y, et al. Cloning of a G-protein-coupled receptor that shows an activity to transform NIH3T3 cells and is expressed in gastric cancer cells. Cancer Sci (2004) 95(2):131–5. doi: 10.1111/j.1349-7006.2004.tb03193.x
17. Ali H, AbdelMageed M, Olsson L, Israelsson A, Lindmark G, Hammarstrom ML, et al. Utility of G protein-coupled receptor 35 expression for predicting outcome in colon cancer. Tumour Biol (2019) 41(6):1010428319858885. doi: 10.1177/1010428319858885
18. Marti-Solano M, Crilly SE, Malinverni D, Munk C, Harris M, Pearce A, et al. Combinatorial expression of GPCR isoforms affects signalling and drug responses. Nature (2020) 587(7835):650–6. doi: 10.1038/s41586-020-2888-2
19. Schihada H, Klompstra TM, Humphrys LJ, Cervenka I, Dadvar S, Kolb P, et al. Isoforms of GPR35 have distinct extracellular N-termini that allosterically modify receptor-transducer coupling and mediate intracellular pathway bias. J Biol Chem (2022) 298(9):102328. doi: 10.1016/j.jbc.2022.102328
20. Barth MC, Ahluwalia N, Anderson TJ, Hardy GJ, Sinha S, Alvarez-Cardona JA, et al. Kynurenic acid triggers firm arrest of leukocytes to vascular endothelium under flow conditions. J Biol Chem (2009) 284(29):19189–95. doi: 10.1074/jbc.M109.024042
21. Yang Y, Lu JY, Wu X, Summer S, Whoriskey J, Saris C, et al. G-protein-coupled receptor 35 is a target of the asthma drugs cromolyn disodium and nedocromil sodium. Pharmacology (2010) 86(1):1–5. doi: 10.1159/000314164
22. Fallarini S, Magliulo L, Paoletti T, de Lalla C, Lombardi G. Expression of functional GPR35 in human iNKT cells. Biochem Biophys Res Commun (2010) 398(3):420–5. doi: 10.1016/j.bbrc.2010.06.091
23. O'Dowd BF, Nguyen T, Marchese A, Cheng R, Lynch KR, Heng HH, et al. Discovery of three novel G-protein-coupled receptor genes. Genomics (1998) 47(2):310–3. doi: 10.1006/geno.1998.5095
24. Guo J, Williams DJ, Puhl HL 3rd, Ikeda SR. Inhibition of N-type calcium channels by activation of GPR35, an orphan receptor, heterologously expressed in rat sympathetic neurons. J Pharmacol Exp Ther (2008) 324(1):342–51. doi: 10.1124/jpet.107.127266
25. Xu H, Ding J, Porter CBM, Wallrapp A, Tabaka M, Ma S, et al. Transcriptional atlas of intestinal immune cells reveals that neuropeptide alpha-CGRP modulates group 2 innate lymphoid cell responses. Immunity (2019) 51(4):696–708 e9. doi: 10.1016/j.immuni.2019.09.004
26. De Giovanni M, Tam H, Valet C, Xu Y, Looney MR, Cyster JG. GPR35 promotes neutrophil recruitment in response to serotonin metabolite 5-HIAA. Cell (2022) 185(5):815–30 e19. doi: 10.1016/j.cell.2022.01.010
27. Kaya B, Donas C, Wuggenig P, Diaz OE, Morales RA, Melhem H, et al. Lysophosphatidic acid-mediated GPR35 signaling in CX3CR1(+) macrophages regulates intestinal homeostasis. Cell Rep (2020) 32(5):107979. doi: 10.1016/j.celrep.2020.107979
28. Divorty N, Mackenzie AE, Nicklin SA, Milligan G. G protein-coupled receptor 35: an emerging target in inflammatory and cardiovascular disease. Front Pharmacol (2015) 6:41. doi: 10.3389/fphar.2015.00041
29. Sriram K, Insel PA. G protein-coupled receptors as targets for approved drugs: how many targets and how many drugs? Mol Pharmacol (2018) 93(4):251–8. doi: 10.1124/mol.117.111062
30. Jenkins L, Brea J, Smith NJ, Hudson BD, Reilly G, Bryant NJ, et al. Identification of novel species-selective agonists of the G-protein-coupled receptor GPR35 that promote recruitment of beta-arrestin-2 and activate Galpha13. Biochem J (2010) 432(3):451–9. doi: 10.1042/BJ20101287
31. Belcheva MM, Coscia CJ. Diversity of G protein-coupled receptor signaling pathways to ERK/MAP kinase. Neurosignals (2002) 11(1):34–44. doi: 10.1159/000057320
32. Maeng YS, Min JK, Kim JH, Yamagishi A, Mochizuki N, Kwon JY, et al. ERK is an anti-inflammatory signal that suppresses expression of NF-kappaB-dependent inflammatory genes by inhibiting IKK activity in endothelial cells. Cell Signal (2006) 18(7):994–1005. doi: 10.1016/j.cellsig.2005.08.007
33. Vuong B, Hogan-Cann AD, Alano CC, Stevenson M, Chan WY, Anderson CM, et al. NF-kappaB transcriptional activation by TNFalpha requires phospholipase C, extracellular signal-regulated kinase 2 and poly(ADP-ribose) polymerase-1. J Neuroinflammation. (2015) 12:229. doi: 10.1186/s12974-015-0448-8
34. Sun L, Ye RD. Role of G protein-coupled receptors in inflammation. Acta Pharmacol Sin (2012) 33(3):342–50. doi: 10.1038/aps.2011.200
35. Guo P, Tai Y, Wang M, Sun H, Zhang L, Wei W, et al. Galpha(12) and galpha(13): versatility in physiology and pathology. Front Cell Dev Biol (2022) 10:809425. doi: 10.3389/fcell.2022.809425
36. Zhang L, Shi G. Gq-coupled receptors in autoimmunity. J Immunol Res (2016) 2016:3969023. doi: 10.1155/2016/3969023
37. Jenkins L, Alvarez-Curto E, Campbell K, de Munnik S, Canals M, Schlyer S, et al. Agonist activation of the G protein-coupled receptor GPR35 involves transmembrane domain III and is transduced via Galpha(1)(3) and beta-arrestin-2. Br J Pharmacol (2011) 162(3):733–48. doi: 10.1111/j.1476-5381.2010.01082.x
38. Jean-Charles PY, Kaur S, Shenoy SK. G protein-coupled receptor signaling through beta-arrestin-dependent mechanisms. J Cardiovasc Pharmacol (2017) 70(3):142–58. doi: 10.1097/FJC.0000000000000482
39. Eishingdrelo H, Sun W, Li H, Wang L, Eishingdrelo A, Dai S, et al. ERK and beta-arrestin interaction: a converging point of signaling pathways for multiple types of cell surface receptors. J Biomol Screen. (2015) 20(3):341–9. doi: 10.1177/1087057114557233
40. Deng H, Hu H, Fang Y. Multiple tyrosine metabolites are GPR35 agonists. Sci Rep (2012) 2:373. doi: 10.1038/srep00373
41. Gu YJ, Sun WY, Zhang S, Wu JJ, Wei W. The emerging roles of beta-arrestins in fibrotic diseases. Acta Pharmacol Sin (2015) 36(11):1277–87. doi: 10.1038/aps.2015.74
42. De Giovanni M, Chen H, Li X, Cyster JG. GPR35 and mediators from platelets and mast cells in neutrophil migration and inflammation. Immunol Rev (2023) 317(1):187–202. doi: 10.1111/imr.13194
43. Deng H, Fang Y. Synthesis and agonistic activity at the GPR35 of 5,6-dihydroxyindole-2-carboxylic acid analogues. ACS Med Chem Lett (2012) 3(7):550–4. doi: 10.1021/ml300076u
44. Pagano E, Elias JE, Schneditz G, Saveljeva S, Holland LM, Borrelli F, et al. Activation of the GPR35 pathway drives angiogenesis in the tumour microenvironment. Gut (2022) 71(3):509–20. doi: 10.1136/gutjnl-2020-323363
45. Schneditz G, Elias JE, Pagano E, Zaeem Cader M, Saveljeva S, Long K, et al. GPR35 promotes glycolysis, proliferation, and oncogenic signaling by engaging with the sodium potassium pump. Sci Signal (2019) 12(562):eaau9048. doi: 10.1126/scisignal.aau9048
46. Zophel D, Hof C, Lis A. Altered ca(2+) homeostasis in immune cells during aging: role of ion channels. Int J Mol Sci (2020) 22(1):110. doi: 10.3390/ijms22010110
47. Iamartino L, Brandi ML. The calcium-sensing receptor in inflammation: Recent updates. Front Physiol (2022) 13:1059369. doi: 10.3389/fphys.2022.1059369
48. Sun T, Xie R, He H, Xie Q, Zhao X, Kang G, et al. Kynurenic acid ameliorates NLRP3 inflammasome activation by blocking calcium mobilization via GPR35. Front Immunol (2022) 13:1019365. doi: 10.3389/fimmu.2022.1019365
49. Chen Z, Jiao Y, Zhang Y, Wang Q, Wu W, Zheng J, et al. G-protein coupled receptor 35 induces intervertebral disc degeneration by mediating the influx of calcium ions and upregulating reactive oxygen species. Oxid Med Cell Longev (2022) 2022:5469220. doi: 10.1155/2022/5469220
50. Venkateswaran S, Prince J, Cutler DJ, Marigorta UM, Okou DT, Prahalad S, et al. Enhanced contribution of HLA in pediatric onset ulcerative colitis. Inflammation Bowel Dis (2018) 24(4):829–38. doi: 10.1093/ibd/izx084
51. MacKenzie AE, Caltabiano G, Kent TC, Jenkins L, McCallum JE, Hudson BD, et al. The antiallergic mast cell stabilizers lodoxamide and bufrolin as the first high and equipotent agonists of human and rat GPR35. Mol Pharmacol (2014) 85(1):91–104. doi: 10.1124/mol.113.089482
52. Dantzer R. Role of the kynurenine metabolism pathway in inflammation-induced depression: preclinical approaches. Curr Top Behav Neurosci (2017) 31:117–38. doi: 10.1007/7854_2016_6
53. Wang J, Simonavicius N, Wu X, Swaminath G, Reagan J, Tian H, et al. Kynurenic acid as a ligand for orphan G protein-coupled receptor GPR35. J Biol Chem (2006) 281(31):22021–8. doi: 10.1074/jbc.M603503200
54. Oka S, Ota R, Shima M, Yamashita A, Sugiura T. GPR35 is a novel lysophosphatidic acid receptor. Biochem Biophys Res Commun (2010) 395(2):232–7. doi: 10.1016/j.bbrc.2010.03.169
55. Mathew D, Torres RM. Lysophosphatidic acid is an inflammatory lipid exploited by cancers for immune evasion via mechanisms similar and distinct from CTLA-4 and PD-1. Front Immunol (2020) 11:531910. doi: 10.3389/fimmu.2020.531910
56. Maravillas-Montero JL, Burkhardt AM, Hevezi PA, Carnevale CD, Smit MJ, Zlotnik A. Cutting edge: GPR35/CXCR8 is the receptor of the mucosal chemokine CXCL17. J Immunol (2015) 194(1):29–33. doi: 10.4049/jimmunol.1401704
57. Xiao S, Xie W, Zhou L. Mucosal chemokine CXCL17: What is known and not known. Scand J Immunol (2021) 93(2):e12965. doi: 10.1111/sji.12965
58. Southern C, Cook JM, Neetoo-Isseljee Z, Taylor DL, Kettleborough CA, Merritt A, et al. Screening beta-arrestin recruitment for the identification of natural ligands for orphan G-protein-coupled receptors. J Biomol Screen. (2013) 18(5):599–609. doi: 10.1177/1087057113475480
59. Taniguchi Y, Tonai-Kachi H, Shinjo K. Zaprinast, a well-known cyclic guanosine monophosphate-specific phosphodiesterase inhibitor, is an agonist for GPR35. FEBS Lett (2006) 580(21):5003–8. doi: 10.1016/j.febslet.2006.08.015
60. Zhao P, Sharir H, Kapur A, Cowan A, Geller EB, Adler MW, et al. Targeting of the orphan receptor GPR35 by pamoic acid: a potent activator of extracellular signal-regulated kinase and beta-arrestin2 with antinociceptive activity. Mol Pharmacol (2010) 78(4):560–8. doi: 10.1124/mol.110.066746
61. De Leo F, Rossi A, De Marchis F, Cigana C, Melessike M, Quilici G, et al. Pamoic acid is an inhibitor of HMGB1.CXCL12 elicited chemotaxis and reduces inflammation in murine models of Pseudomonas aeruginosa pneumonia. Mol Med (2022) 28(1):108. doi: 10.1186/s10020-022-00535-z
62. Deng H, Hu H, He M, Hu J, Niu W, Ferrie AM, et al. Discovery of 2-(4-methylfuran-2(5H)-ylidene)malononitrile and thieno[3,2-b]thiophene-2-carboxylic acid derivatives as G protein-coupled receptor 35 (GPR35) agonists. J Med Chem (2011) 54(20):7385–96. doi: 10.1021/jm200999f
63. Tsukahara T, Hamouda N, Utsumi D, Matsumoto K, Amagase K, Kato S. G protein-coupled receptor 35 contributes to mucosal repair in mice via migration of colonic epithelial cells. Pharmacol Res (2017) 123:27–39. doi: 10.1016/j.phrs.2017.06.009
64. Neetoo-Isseljee Z, MacKenzie AE, Southern C, Jerman J, McIver EG, Harries N, et al. High-throughput identification and characterization of novel, species-selective GPR35 agonists. J Pharmacol Exp Ther (2013) 344(3):568–78. doi: 10.1124/jpet.112.201798
65. Funke M, Thimm D, Schiedel AC, Muller CE. 8-Benzamidochromen-4-one-2-carboxylic acids: potent and selective agonists for the orphan G protein-coupled receptor GPR35. J Med Chem (2013) 56(12):5182–97. doi: 10.1021/jm400587g
66. Yang Y, Fu A, Wu X, Reagan JD. GPR35 is a target of the loop diuretic drugs bumetanide and furosemide. Pharmacology (2012) 89(1-2):13–7. doi: 10.1159/000335127
67. Deng H, Hu H, Fang Y. Tyrphostin analogs are GPR35 agonists. FEBS Lett (2011) 585(12):1957–62. doi: 10.1016/j.febslet.2011.05.026
68. Deng H, Hu J, Hu H, He M, Fang Y. Thieno[3,2-b]thiophene-2-carboxylic acid derivatives as GPR35 agonists. Bioorg Med Chem Lett (2012) 22(12):4148–52. doi: 10.1016/j.bmcl.2012.04.057
69. Deng H, Fang Y. Anti-inflammatory gallic Acid and wedelolactone are G protein-coupled receptor-35 agonists. Pharmacology (2012) 89(3-4):211–9. doi: 10.1159/000337184
70. Otkur W, Wang J, Hou T, Liu F, Yang R, Li Y, et al. Aminosalicylates target GPR35, partly contributing to the prevention of DSS-induced colitis. Eur J Pharmacol (2023) 949:175719. doi: 10.1016/j.ejphar.2023.175719
71. Wei L, Wang J, Zhang X, Wang P, Zhao Y, Li J, et al. Discovery of 2H-chromen-2-one derivatives as G protein-coupled receptor-35 agonists. J Med Chem (2017) 60(1):362–72. doi: 10.1021/acs.jmedchem.6b01431
72. Jenkins L, Harries N, Lappin JE, MacKenzie AE, Neetoo-Isseljee Z, Southern C, et al. Antagonists of GPR35 display high species ortholog selectivity and varying modes of action. J Pharmacol Exp Ther (2012) 343(3):683–95. doi: 10.1124/jpet.112.198945
73. Vecsei L, Szalardy L, Fulop F, Toldi J. Kynurenines in the CNS: recent advances and new questions. Nat Rev Drug Discovery (2013) 12(1):64–82. doi: 10.1038/nrd3793
74. Agudelo LZ, Ferreira DMS, Cervenka I, Bryzgalova G, Dadvar S, Jannig PR, et al. Kynurenic acid and gpr35 regulate adipose tissue energy homeostasis and inflammation. Cell Metab (2018) 27(2):378–92 e5. doi: 10.1016/j.cmet.2018.01.004
75. Miyamoto K, Sujino T, Harada Y, Ashida H, Yoshimatsu Y, Yonemoto Y, et al. The gut microbiota-induced kynurenic acid recruits GPR35-positive macrophages to promote experimental encephalitis. Cell Rep (2023) 42(8):113005. doi: 10.1016/j.celrep.2023.113005
76. Ye X, Chun J. Lysophosphatidic acid (LPA) signaling in vertebrate reproduction. Trends Endocrinol Metab (2010) 21(1):17–24. doi: 10.1016/j.tem.2009.08.003
77. Melhem H, Kaya B, Kaymak T, Wuggenig P, Flint E, Roux J, et al. Epithelial GPR35 protects from Citrobacter rodentium infection by preserving goblet cells and mucosal barrier integrity. Mucosal Immunol (2022) 15(3):443–58. doi: 10.1038/s41385-022-00494-y
78. Burkhardt AM, Tai KP, Flores-Guiterrez JP, Vilches-Cisneros N, Kamdar K, Barbosa-Quintana O, et al. CXCL17 is a mucosal chemokine elevated in idiopathic pulmonary fibrosis that exhibits broad antimicrobial activity. J Immunol (2012) 188(12):6399–406. doi: 10.4049/jimmunol.1102903
79. Binti Mohd Amir NAS, Mackenzie AE, Jenkins L, Boustani K, Hillier MC, Tsuchiya T, et al. Evidence for the existence of a CXCL17 receptor distinct from GPR35. J Immunol (2018) 201(2):714–24. doi: 10.4049/jimmunol.1700884
80. Park SJ, Lee SJ, Nam SY, Im DS. GPR35 mediates lodoxamide-induced migration inhibitory response but not CXCL17-induced migration stimulatory response in THP-1 cells; is GPR35 a receptor for CXCL17? Br J Pharmacol (2018) 175(1):154–61. doi: 10.1111/bph.14082
81. Rojewska E, Ciapala K, Mika J. Kynurenic acid and zaprinast diminished CXCL17-evoked pain-related behaviour and enhanced morphine analgesia in a mouse neuropathic pain model. Pharmacol Rep (2019) 71(1):139–48. doi: 10.1016/j.pharep.2018.10.002
82. De Giovanni M, Dang EV, Chen KY, An J, Madhani HD, Cyster JG. Platelets and mast cells promote pathogenic eosinophil recruitment during invasive fungal infection via the 5-HIAA-GPR35 ligand-receptor system. Immunity (2023) 56(7):1548–60 e5. doi: 10.1016/j.immuni.2023.05.006
83. Chen Y, Palm F, Lesch KP, Gerlach M, Moessner R, Sommer C. 5-hydroxyindolacetic acid (5-HIAA), a main metabolite of serotonin, is responsible for complete Freund's adjuvant-induced thermal hyperalgesia in mice. Mol Pain. (2011) 7:21. doi: 10.1186/1744-8069-7-21
84. Ciapala K, Mika J, Rojewska E. The kynurenine pathway as a potential target for neuropathic pain therapy design: from basic research to clinical perspectives. Int J Mol Sci (2021) 22(20):11055. doi: 10.3390/ijms222011055
85. Kim MJ, Park SJ, Nam SY, Im DS. Lodoxamide attenuates hepatic fibrosis in mice: involvement of GPR35. Biomol Ther (Seoul). (2020) 28(1):92–7. doi: 10.4062/biomolther.2018.227
86. Duan J, Liu Q, Yuan Q, Ji Y, Zhu S, Tan Y, et al. Insights into divalent cation regulation and G(13)-coupling of orphan receptor GPR35. Cell Discovery (2022) 8(1):135. doi: 10.1038/s41421-022-00499-8
87. Wei L, Xiang K, Kang H, Yu Y, Fan H, Zhou H, et al. Development and characterization of fluorescent probes for the G protein-coupled receptor 35. ACS Med Chem Lett (2023) 14(4):411–6. doi: 10.1021/acsmedchemlett.2c00461
88. Mackenzie AE, Quon T, Lin LC, Hauser AS, Jenkins L, Inoue A, et al. Receptor selectivity between the G proteins Galpha(12) and Galpha(13) is defined by a single leucine-to-isoleucine variation. FASEB J (2019) 33(4):5005–17. doi: 10.1096/fj.201801956R
89. Boleij A, Fathi P, Dalton W, Park B, Wu X, Huso D, et al. G-protein coupled receptor 35 (GPR35) regulates the colonic epithelial cell response to enterotoxigenic Bacteroides fragilis. Commun Biol (2021) 4(1):585. doi: 10.1038/s42003-021-02014-3
90. Ley K, Laudanna C, Cybulsky MI, Nourshargh S. Getting to the site of inflammation: the leukocyte adhesion cascade updated. Nat Rev Immunol (2007) 7(9):678–89. doi: 10.1038/nri2156
91. Petri B, Sanz MJ. Neutrophil chemotaxis. Cell Tissue Res (2018) 371(3):425–36. doi: 10.1007/s00441-017-2776-8
92. Gonzalez RJ, von Andrian UH. Quo vadis, neutrophil? Cell (2022) 185(5):759–61. doi: 10.1016/j.cell.2022.02.009
93. Bordon Y. Platelet-derived 5-HIAA helps neutrophils enter tissue. Nat Rev Immunol (2022) 22(4):206–7. doi: 10.1038/s41577-022-00699-z
94. Martinez FO, Gordon S. The M1 and M2 paradigm of macrophage activation: time for reassessment. F1000Prime Rep (2014) 6:13. doi: 10.12703/P6-13
95. Ploeger DT, Hosper NA, Schipper M, Koerts JA, de Rond S, Bank RA. Cell plasticity in wound healing: paracrine factors of M1/ M2 polarized macrophages influence the phenotypical state of dermal fibroblasts. Cell Commun Signal (2013) 11(1):29. doi: 10.1186/1478-811X-11-29
96. Nguyen-Chi M, Laplace-Builhe B, Travnickova J, Luz-Crawford P, Tejedor G, Phan QT, et al. Identification of polarized macrophage subsets in zebrafish. Elife (2015) 4:e07288. doi: 10.7554/eLife.07288
97. Kratofil RM, Kubes P, Deniset JF. Monocyte conversion during inflammation and injury. Arterioscler Thromb Vasc Biol (2017) 37(1):35–42. doi: 10.1161/ATVBAHA.116.308198
98. Godfrey DI, MacDonald HR, Kronenberg M, Smyth MJ, Van Kaer L. NKT cells: what's in a name? Nat Rev Immunol (2004) 4(3):231–7. doi: 10.1038/nri1309
99. Van Kaer L, Parekh VV, Wu L. Invariant natural killer T cells: bridging innate and adaptive immunity. Cell Tissue Res (2011) 343(1):43–55. doi: 10.1007/s00441-010-1023-3
100. Liew PX, Lee WY, Kubes P. iNKT cells orchestrate a switch from inflammation to resolution of sterile liver injury. Immunity (2017) 47(4):752–65 e5. doi: 10.1016/j.immuni.2017.09.016
101. Ahn S, Jeong D, Oh SJ, Ahn J, Lee SH, Chung DH. GM-CSF and IL-4 produced by NKT cells inversely regulate IL-1beta production by macrophages. Immunol Lett (2017) 182:50–6. doi: 10.1016/j.imlet.2017.01.003
102. Yoshimoto T. The hunt for the source of primary interleukin-4: how we discovered that natural killer T cells and basophils determine T helper type 2 cell differentiation in vivo. Front Immunol (2018) 9:716. doi: 10.3389/fimmu.2018.00716
103. Farooq SM, Hou Y, Li H, O'Meara M, Wang Y, Li C, et al. Disruption of GPR35 exacerbates dextran sulfate sodium-induced colitis in mice. Dig Dis Sci (2018) 63(11):2910–22. doi: 10.1007/s10620-018-5216-z
104. Zhang Q, Wang Q, Li X, Wu M, Wu X, Zhao Q, et al. Gut microbiota-derived 5-hydroxyindoleacetic acid from pumpkin polysaccharides supplementation alleviates colitis through epac/rap1 signaling activation. (2023). doi: 10.21203/rs.3.rs-3123790/v1.
105. Lei-Leston AC, Murphy AG, Maloy KJ. Epithelial cell inflammasomes in intestinal immunity and inflammation. Front Immunol (2017) 8:1168. doi: 10.3389/fimmu.2017.01168
106. Churchill MJ, Mitchell PS, Rauch I. Epithelial pyroptosis in host defense. J Mol Biol (2022) 434(4):167278. doi: 10.1016/j.jmb.2021.167278
107. Hanauer SB, Feagan BG, Lichtenstein GR, Mayer LF, Schreiber S, Colombel JF, et al. Maintenance infliximab for Crohn's disease: the ACCENT I randomised trial. Lancet (2002) 359(9317):1541–9. doi: 10.1016/S0140-6736(02)08512-4
108. Noti M, Corazza N, Mueller C, Berger B, Brunner T. TNF suppresses acute intestinal inflammation by inducing local glucocorticoid synthesis. J Exp Med (2010) 207(5):1057–66. doi: 10.1084/jem.20090849
109. Wei X, Yin F, Wu M, Xie Q, Zhao X, Zhu C, et al. G protein-coupled receptor 35 attenuates nonalcoholic steatohepatitis by reprogramming cholesterol homeostasis in hepatocytes. Acta Pharm Sin B (2023) 13(3):1128–44. doi: 10.1016/j.apsb.2022.10.011
110. Zheng X, Hu M, Zang X, Fan Q, Liu Y, Che Y, et al. Kynurenic acid/GPR35 axis restricts NLRP3 inflammasome activation and exacerbates colitis in mice with social stress. Brain Behav Immun (2019) 79:244–55. doi: 10.1016/j.bbi.2019.02.009
111. Wang D, Li D, Zhang Y, Chen J, Zhang Y, Liao C, et al. Functional metabolomics reveal the role of AHR/GPR35 mediated kynurenic acid gradient sensing in chemotherapy-induced intestinal damage. Acta Pharm Sin B (2021) 11(3):763–80. doi: 10.1016/j.apsb.2020.07.017
112. Cervenka I, Agudelo LZ, Ruas JL. Kynurenines: Tryptophan's metabolites in exercise, inflammation, and mental health. Science (2017) 357(6349):eaaf9794. doi: 10.1126/science.aaf9794
113. Mackiewicz T, Wlodarczyk J, Zielinska M, Wlodarczyk M, Durczynski A, Hogendorf P, et al. Increased GPR35 expression in human colorectal and pancreatic cancer samples: A preliminary clinical validation of a new biomarker. Adv Clin Exp Med (2023) 32(7):783–9. doi: 10.17219/acem/157291
114. Yue J, Guo H, Xu P, Ma J, Wu Y. Activation of the GPR35 on ILC2 drives immunosuppression to promote lung cancer progression. Am J Cancer Res (2023) 13(6):2426–38.
115. Birring SS, Wijsenbeek MS, Agrawal S, van den Berg JWK, Stone H, Maher TM, et al. A novel formulation of inhaled sodium cromoglicate (PA101) in idiopathic pulmonary fibrosis and chronic cough: a randomised, double-blind, proof-of-concept, phase 2 trial. Lancet Respir Med (2017) 5(10):806–15. doi: 10.1016/S2213-2600(17)30310-7
Keywords: GPR35, pro-inflammatory, anti-inflammatory, inflammatory diseases, therapeutic target
Citation: Wu Y, Zhang P, Fan H, Zhang C, Yu P, Liang X and Chen Y (2023) GPR35 acts a dual role and therapeutic target in inflammation. Front. Immunol. 14:1254446. doi: 10.3389/fimmu.2023.1254446
Received: 07 July 2023; Accepted: 02 November 2023;
Published: 16 November 2023.
Edited by:
Yan Huang, Anhui Medical University, ChinaReviewed by:
Hua Ren, East China Normal University, ChinaMarco De Giovanni, University of California, San Francisco, United States
Berna Kaya, Massachusetts General Hospital and Harvard Medical School, United States
Copyright © 2023 Wu, Zhang, Fan, Zhang, Yu, Liang and Chen. This is an open-access article distributed under the terms of the Creative Commons Attribution License (CC BY). The use, distribution or reproduction in other forums is permitted, provided the original author(s) and the copyright owner(s) are credited and that the original publication in this journal is cited, in accordance with accepted academic practice. No use, distribution or reproduction is permitted which does not comply with these terms.
*Correspondence: Yang Chen, Y2hlbnlhbmcyODA4QGRpY3AuYWMuY24=; Xinmiao Liang, bGlhbmd4bUBkaWNwLmFjLmNu
†These authors have contributed equally to this work and share first authorship