- Chinese Academy of Medical Sciences (CAMS) Key Laboratory of Translational Research on Lung Cancer, State Key Laboratory of Molecular Oncology, Department of Medical Oncology, National Cancer Center/National Clinical Research Center for Cancer/Cancer Hospital, Chinese Academy of Medical Sciences & Peking Union Medical College, Beijing, China
Immunotherapy has changed the treatment strategy of non-small cell lung cancer (NSCLC) in recent years, among which anti-PD-1/PD-L1 antibodies are the most used. However, the majority of patients with NSCLC do not derive benefit from immune checkpoint inhibitors (ICIs). Vascular abnormalities are a hallmark of most solid tumors and facilitate immune evasion. Thus, combining antiangiogenic therapies might increase the effectiveness of anti-PD-1/PD-L1 antibodies. In this paper, the mechanisms of anti-angiogenic agents combined with anti-PD-1/PD-L1 antibodies are illustrated, moreover, relevant clinical studies and predictive immunotherapeutic biomarkers are summarized and analyzed, in order to provide more treatment options for NSCLC patients.
1 Introduction
Lung cancer is a disease that seriously endangers human health, and it also remains the leading cause of cancer death, including non-small cell lung cancer (NSCLC) and small cell lung cancer (SCLC) (1). NSCLC accounts for 80% - 85% of all lung cancers (2). In recent years, accurate treatment and combined therapies of lung cancer have made rapid progress, including immune checkpoint inhibitors (ICIs), ICIs combined with cytotoxic chemotherapies (Chemo), ICIs combined with ICIs and combined with anti-angiogenic therapy (3). Anti-PD-1/PD-L1 antibodies are the most used among ICIs (4). Additionally, the effects of ICIs + anti-angiogenic therapy are considerable, with accepted adverse events (AEs) (5). The results of a series of related clinical trials have been announced, which have been summarized here. Tumor angiogenesis provides nutrition for tumor growth, while lymphocytes infiltrate into the tumor through tumor vessels, and vascular growth interacts with tumor growth and tumor microenvironment (TME) to form a complex tumor ecological environment (6), so it is necessary to systematically review it. From this perspective, we described the mechanisms and summarized the latest progress of related clinical research of anti-angiogenic drugs combined with immunotherapies in NSCLC.
2 Crosstalk between tumor angiogenesis and immune microenvironment
2.1 Inhibiting effect of tumor angiogenic factors on tumor immune microenvironment
Abnormal vasculature is the hallmark of solid tumors, and is also involved in tumor immune escape (7). The abnormal vessels and impaired perfusion can also restrict the entry of cytotoxic drugs and immune cells from the circulation into tumors, limiting their anticancer activity. The TME consists of numerous pro-angiogenic factors, including vascular endothelial growth factor (VEGF), fibroblast growth factor (FGF), and platelet-derived growth factor (PDGF), which are secreted by tumor cells or tumor-infiltrating lymphocytes (TILs) or macrophages (8). These factors can activate pro-angiogenic signaling pathways to promote growth, invasion, and metastasis of tumor (9). Through the production of various cytokines and growth factors, such as VEGF, angiopoietin 2 (ANG-2), the immune cells work in concert with tumor cells lining abnormal tumor blood vessels to promote tumor angiogenesis and immunosuppression.
VEGF has suppressive effects on tumor immune microenvironment, by directly affect the differentiation, infiltration and cytotoxicity of various types of immune cells and other indirect mechanisms (10). VEGF could inhibit the differentiation of hematopoietic stem cells into CD4+ and CD8+ T cells in thymus. VEGF promotes the recruitment and proliferation of immunosuppressive cells such as regulatory T cells (Tregs), bone myeloid-derived suppressor cells (MDSCs), and M2-like tumor-associated macrophages (TAMs) (11). M2-like macrophages are similar in phenotype to TAMs, which promote tumor growth and metastasis, and are associated with poor prognosis of tumors (12). In addition, VEGF inhibits T cell proliferation and cytotoxicity, binding to VEGFR2 on T cell, and also up-regulates immune checkpoint molecules such as the programmed cell death protein 1 (PD-1), programmed cell death ligand 1 (PD-L1) and cytotoxic T lymphocyte-associated protein 4 (CTLA-4) to inhibit T cell activation (13). VEGF also can reduce the ability of immune cells to adhere and pass through the blood vessel, by down-regulating the integrin ligands intercellular adhesion molecule 1 (ICAM-1) and vascular cell adhesion protein 1 (VCAM-1) of endothelial cells or preventing them from accumulating on endothelial cells, thus prevent immune cells from entering the tumor (14). Dendritic cells (DCs) play a crucial role in T cell activation. However, VEGF-VEGFR2 signaling inhibits antigen presenting by interfering with DCs maturation, thus indirectly inhibiting T cell activity and resulting in decreased T cell-mediated anti-cancer activity (15). Moreover, VEGF could indirectly affect the biochemical properties of TME and promotes angiogenesis that results in an aberrant tumor vasculature, leading to hypoxia and a low pH in the TME, which in turn fosters immunosuppression both locally and systemically (16, 17).
In addition to VEGF, ANG-2 is another key vascular growth immunomodulator. Activated ANG-2 signals can induce immunosuppressive TME through a variety of mechanisms (18). ANG-2 binds to Tie-2 expressed on monocytes, which recruits monocytes and also stimulates monocytes to secrete IL-10. IL-10 suppresses CD8+ cytotoxic T lymphocytes (CTLs) proliferation and cytotoxicity, and enhances Tregs infiltration (19). Moreover, ANG-2 also inhibits the secretion of transforming growth factor α (TNF-α), thereby limiting the anti-cancer activity of monocytes (18) (Figure 1). Overall, abnormalities in cancer blood vessels can cause immunosuppressive TME.
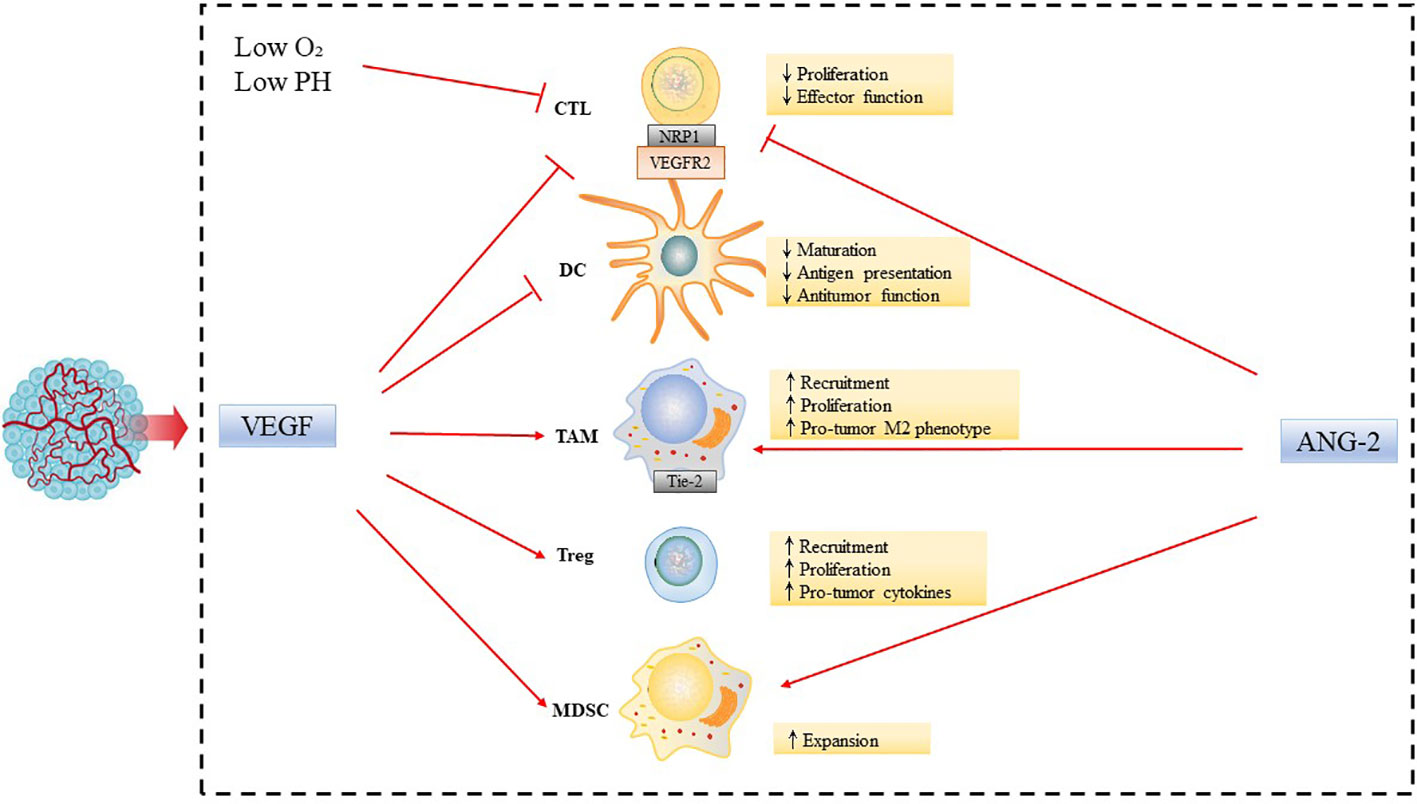
Figure 1 Inhibiting effect of tumor angiogenic factors on tumor immune microenvironment. Abnormalities in the tumor vasculature result in hypoxia and acidosis of the TME. Tumor angiogenic factors, such as VEGF and ANG-2, modulate the functions of immune cells leading to tumor immunosuppressive microenvironment, by increasing accumulation, activation, and expansion of Tregs; recruitment of inflammatory monocytes and TAMs and reprogramming of TAMs from an anticancer M1-like phenotype towards the pro-tumor M2 phenotype; suppression of DC maturation, which results in impaired antigen presentation and activation of CTLs, and expansion of MDSCs.
2.2 Promoting effect of tumor immune microenvironment on tumor angiogenesis
Tumor angiogenesis involves not only cancer cells but also a variety of immune cells, such as T cells, myeloid cell, and interstitial cell in TME. For the above immune cells, the release of proangiogenic cytokines is accompanied by a switch to an immunosuppressive behavior. T cells do not directly secrete VEGF, but they facilitate its effect by acquiring neuropilin 1 (NRP1) during interaction with DCs, which binds to VEGFA to promote angiogenesis (20). Both neutrophils and TAMs promote angiogenesis by secreting proangiogenic factors, such as VEGF, TNF-α, IL-8, and various chemokines including CXCR-2, 4 and 12, CXCL-3, 4, 8, 9, 10, and CCL2-5 (21). MDSC recruitment to the tumor can be induced by many different factors, such as CSF-3, IL-1β, and IL-6, and subsequently lead to activation of STAT3, rendering them potent as proangiogenic and immunosuppressive cells (22). Besides, Tregs indirectly inhibit tumor angiogenesis by inhibiting helper T cells (TH1) cells, which express IFN-γ (23). In short, immune cells in TME can promote tumor angiogenesis.
3 The mechanism of antiangiogenic drugs combined with immunotherapy
Anti-angiogenic drugs induced vascular normalization improve immune cell infiltration and promote the transformation of “cold tumors” into “hot tumors”, thus enhances the efficacy of immunotherapy (24, 25). Anti-angiogenic drugs promote immune cell maturation and improve infiltration by blocking the binding of VEGF to VEGFR2 on the surface of macrophages and T cells (26). Tumor vascular normalization relieves hypoxia and reduces the secretion of VEGF, thus reducing the recruitment of immunosuppressive cells such as MDSCs and Tregs, and also reduce the expression of PD-1, PD-L1, CTLA-4, TIM-3 and other immune checkpoint molecules on the surface of immunosuppressive cells (27, 28). Besides, the vascular normalization effect is associated with more efficient lymphocyte priming by antigen-presenting cells, TAM polarization to an M1-like phenotype, and accumulation of activated, IFN-γ expressing CD8+ T cells within the perivascular space (29, 30) M1-like TAMs are generally considered to be tumor-killing macrophages, primarily anti-tumor and immune-promoting (12) (Figure 2). In current clinical practice, the most used antiangiogenetic drugs include bevacizumab (targeting at VEGF-A), ramucirumab (targeting at VEGFR2) (31). Multiple therapeutic agents targeting VEGF and VEGF receptors have been developed and approved for use in cancers. Moreover, tyrosine kinase inhibitors (TKIs) can inhibit not only VEGFR but also FGFR, PDGFR and so on (32). The commonly used TKI drugs are lenvatinib, apatinib and anlotinib in NSCLC. Preclinical studies have proved that low-dose apatinib combined with PD-1/PD-L1 inhibitors can enhance its anti-tumor activity, and in the preliminary clinical application showed a good therapeutic effect (33).
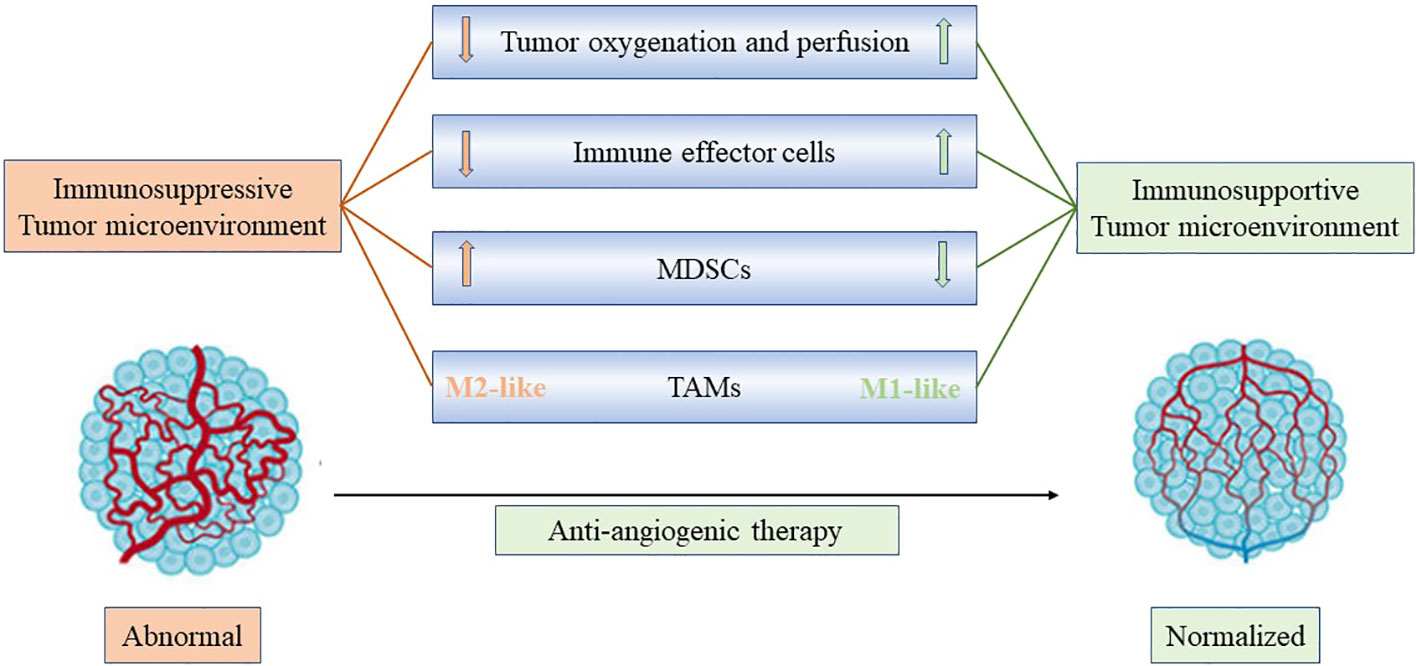
Figure 2 Anti-angiogenic treatment reprograms the tumor microenvironment from immunosuppressive to immunosupportive and improves effects of immunotherapy. Anti-angiogenic drugs induced vascular normalization improves immune cell infiltration and promotes the transformation of “cold tumors” into “hot tumors”, facilitating the infiltration of T effector cells while reducing MDSC accumulation. In addition, improved vascular perfusion polarizes TAMs to an immune stimulatory M1-like phenotype. Consequently, through anti-angiogenic treatment, vascular normalization could potentially enhance the effectiveness of immunotherapy.
For ICIs, these drugs have been thought to mainly affect T cells, which lead to activating T cells secret INF-γ which decreased endothelial VEGFA, and increased CXCL-9, CXCL-10 and CXCL-11, induced tumor vascular normalization (34, 35). Thus, vascular normalization in the setting of immune stimulation represents a novel mechanism for the antitumor effects of immune checkpoint blockade and provides a new understanding of tumor vascular remodeling and immune reprogramming. As for ICI, antibodies that block the interaction of PD-1 with its ligand PD-L1 and the binding of CTLA-4 to its receptor have been approved for clinical use (36). The former ones include atezolizumab and durvalumab, inhibiting PD-L1, and pembrolizumab, cemiplimab, nivolumab, camrelizumab, toripalimab, and sintilimab, inhibiting PD-1 receptors (Table 1).
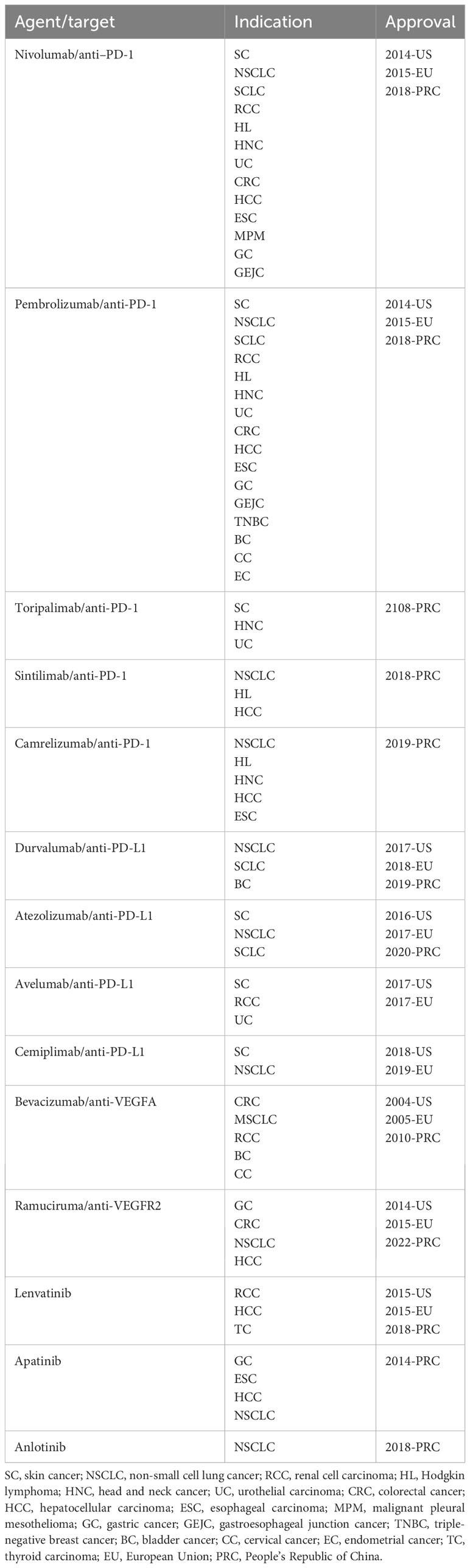
Table 1 The approved indications of anti-PD-1/PD-L1 antibodies and anti-angiogenic drugs in the globe.
To sum up, anti-angiogenesis therapy normalizes tumor blood vessels and also improves tumor immune microenvironment. ICIs can activate T lymphocytes to secrete IFN- γ, to reduce local hypoxia and promote the normalization of tumor vessels, which demonstrates the synergistic effect of anti-PD-1/PD-L1 antibodies combined with antiangiogenic drugs and provides a theoretical basis for their combination in the treatment of NSCLC (37, 38).
4 Clinical practices of anti-PD-1/PD-L1 antibodies plus antiangiogenic therapy in NSCLC
Based on published clinical studies, generally, anti-PD-1/PD-L1 antibodies combined with antiangiogenetic therapy showed better efficacy than ICIs alone, and prolonged progression free survival (PFS) and overall survival (OS). Here, we focused only on NSCLC, and summarized current clinical studies on combination therapy of anti-PD-1/PD-L1 antibodies plus antiangiogenesis in patients according to different clinical care scenarios.
4.1 Advanced first-line therapy
According to NCCN guidelines V1.2023 NSCLC, one of the recommended first-line therapy for advanced adenocarcinoma, large cell, and NSCLC not otherwise specified (NOS) patients is carboplatin + paclitaxel + bevacizumab + atezolizumab (ABCP) (39), with PD-L1 expression whether ≥50% or ranges between 1%-49%. The recommendation was based on the result of IMpower150, an international, open label, phase 3 study in chemo-naive NSCLC patients. Compared with becacizumab plus chemotherapy (BCP) group, ABCP group had longer median PFS (mPFS) (8.3 months vs. 6.8 months; HR, 0.62; 95% CI, 0.52 to 0.74; P < 0.001) and median OS (19.2 months vs. 14.7 months; HR, 0.78; 95% CI, 0.64 to 0.96; P = 0.02) (40, 41). The combination of pembrolizumab and ramucirumab for first-line treatment was investigated in an expansion cohort of JDVF trial, and the objective response rate (ORR) was 42.3% (42). Another cohort in a three-arm prospective study (anlotinib combined with erlotinib, carboplatin plus pemetrexed/gemcitabine, and sintilimab) adopting anlotinib combined with sintilimab in untreated locally advanced/metastatic NSCLC patients indicated low incidences of adverse events and better clinical benefits than previous reports with ORR 72.7%, and mPFS 15.6 months (43). Other clinical studies and their results were briefly listed in Table 2, and most of these researches showed positive evidence.
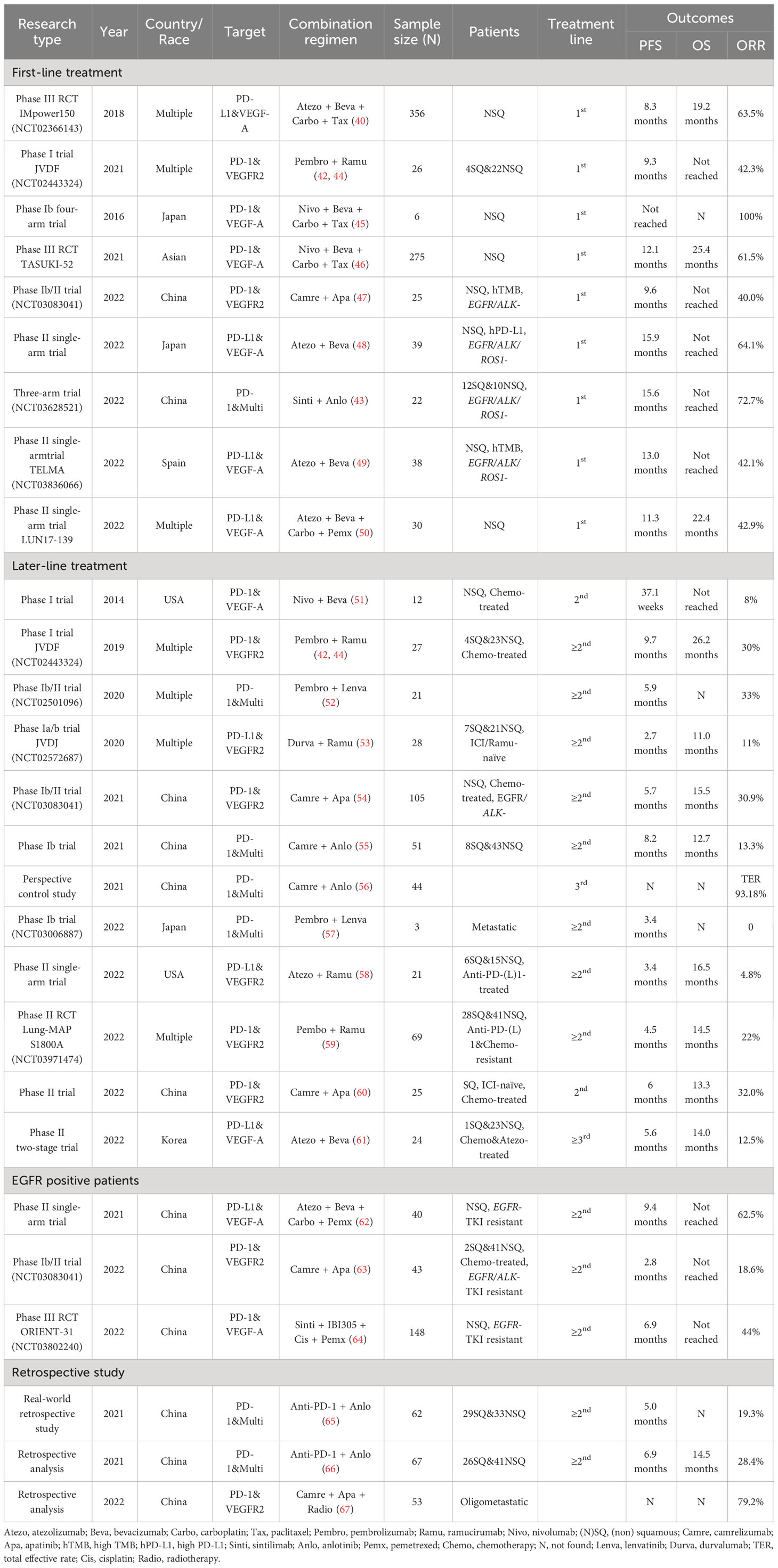
Table 2 Clinical studies of anti-PD-1/PD-L1 antibodies plus antiangiogenetic therapy in NSCLC patients.
However, in a meta-analysis that involved 8278 patients from 16 randomized controlled trials (RCTs) comparing efficacy and safety of different first-line immunotherapy combinations, including ABCP, pembrolizumab-chemo, atezolizumab-chemo, camrelizumab-chemo, tislelizumab-chemo, sintilimab-chemo, nivolumab-ipilimumab, nivolumab-ipilimumab-chemo, durvalumab-tremelimumab, and durva-tremelimumab-chemo, ABCP regimen showed best efficacy in PFS and ORR, but the advantages in OS and toxicity were not prominent (68). Similar findings were also revealed in a meta-analysis enrolling 19 phase II/III RCTs in which the ABCP regimen was ranked 5th among all 17 regimens including ABCP, 5 ICI-monotherapy regimens, 7 ICI-Chemo regimens, 2 dual-ICI strategies, 1 dual-ICI-Chemo combination, and 1 Beva-Chemo regimen, in which overall OS, PFS, safety, and ORR were taken into consideration (69). Furthermore, a cost-effectiveness analysis from the perspective of the US health care sector showed that adopting pembrolizumab monotherapy when PD-L1≥50%, pembrolizumab combined with chemotherapy when PD-L1 between 1%-49%, and nivolumab plus ipilimumab when PD-L1<1% were more cost-effective treatment options, whose performance was better than ABCP (QALY 2.39) (70). Similar results were revealed in several other cost-effectiveness analyses (71). It should be emphasized that comparing treatment strategies across studies is challenging, though, clinicians should be cautious, prudent, and comprehensive when considering anti-PD-1/PD-L1 antibodies combined with antiangiogenic treatment, especially when ICI monotherapy or dual-ICIs strategies are available at the same time.
4.2 Advanced later line therapy
Compared with traditional chemotherapy, immunotherapy has achieved good benefits in patients with advanced NSCLC, but the benefits are lower for patients receiving second or higher line treatment (72). The resistance mechanisms are probably multifactorial, including tumor expressing low levels of PD-L1 and immunosuppressive TME. Based on the theoretical synergistic mechanism between vascular normalization and immune promotion in TME, the combination of antiangiogenetic agent and anti-PD-1/PD-L1 antibodies may be able to show surprising antitumor activity in the subsequent line treatment. Furthermore, the resistance mechanism in the late stage of targeted therapy remains not well revealed (73). And the persistent toxicities after initial treatment with cytotoxic chemotherapy not only cause adverse events but also affect the response to later line therapy (44). In the randomized phase II Lung-MAP substudy (S1800A), 136 patients with progression after previous treatment with ICI and platinum-based chemotherapy randomly received pembrolizumab plus ramucirumab or standard care, and the former group showed an improved OS (14.5 months vs. 11.6 months) (59). Phase Ia/b JVDF trial assessed the safety and antitumor activity of ramucirumab combined with pembrolizumab treatment in previously treated solid tumor patients (advanced gastric or gastro-esophageal junction adenocarcinoma, non-small-cell lung cancer, or urothelial carcinoma). There was one, seven, fifteen, and three of 27 NSCLC patients achieved CR, PR, SD, and PD, respectively, with ORR 30% and mPFS 9.7 months, which was a favorable result compared with anti-PD-1/PD-L1 antibody treatment alone in other studies (44). Most randomized phase III clinical trials using combined immunotherapy were designed for first-line therapy, and there are few data for NSCLC patients in second-line or higher settings. Further explorations for subsequent line treatment of advanced NSCLC patients with multi-line resistance were needed.
4.3 Perioperative therapy
Apart from locally advanced or metastatic NSCLC patients, whether patients in relatively early stages can benefit from the combination therapy is also worth exploring. When investigating the efficacy of the combination therapy, stage III patients are often excluded, and there was little related research focusing on perioperative NSCLC patients. Apart from efficacy, it is also important to emphasize perioperative safety, as the inhibition of angiogenesis might affect wound healing (74). As far as currently known, an interval of ≥4 weeks between drug administration and surgery is recommended (75). The major concerns of perioperative patients such as neoadjuvant efficacy, postoperative recurrence and metastasis, and wound bleeding and healing complications, have yet not been well studied. As a theoretical basis, a pre-clinical study found that the combination therapy of pembrolizumab and bevacizumab could transform tumors into an inflamed condition, thus inhibiting tumor growth and preventing postoperative recurrence and metastasis in a humanized neoadjuvant mouse model (75).
The clinical studies that have been published so far on perioperative patients are mainly case reports. Toripalimab + apatinib + pemetrexed + nedaplatin has been reported for preoperative induction therapy in a patient who achieved a PFS of 7 months (76). Nivolumab + anlotinib and atezolizumab + bevacizumab + chemotherapy have both been reported for postoperative adjuvant therapy and have shown clinically favorable responses (77, 78). These reports revealed the feasibility of anti-PD-1/PD-L1 antibodies plus antiangiogenesis combination therapy as either neoadjuvant or adjuvant therapy, but still further clinical exploration is needed.
5 Immunotherapy combined with antiangiogenic therapy in EGFR positive patients
5.1 The tumor angiogenesis and immune microenvironment in EGFR positive patients
Epidermal growth factor receptor (EGFR)-activating mutations represent the most frequent targetable alteration with a prevalence of nearly 20% in Caucasians with lung adenocarcinomas (79). Moreover, EGFR mutations in NSCLC have immunosuppressive effects, and previous studies have reported that the EGFR mutations can modulate several factors to impact TME, such as TILs, Tregs, MDSCs, TAMs, and immunoregulatory cytokines (80, 81). Preclinical studies have revealed that the VEGF and EGFR pathways share common downstream signaling, and these pathways can function exclusively of one another during oncogenesis (82). Moreover, in EGFR-mutant NSCLCs, EGFR activation may drive VEGF expression as EGFR-mutant NSCLC cells constitutively up-regulate HIF-1α in a hypoxia-independent manner (83). Therefore, mutant EGFR-driven NSCLC has a unique immunosuppressive microenvironment, and EGFR activation promotes the expression of VEGF, which is expected to result in tumor angiogenesis.
5.2 Clinical practices
According to NCCN guidelines, results of molecular testing including ALK, BRAF, EGFR, etc., should be obtained before administering first-line immunotherapy if clinically feasible. Patients with metastatic NSCLC and high PD-L1 expression level who also have a molecular variant that targets the driver oncogene should receive first-line targeted therapy rather than first-line immunotherapy because the former choice yields higher response rates and is better tolerated (39, 84). However, acquired resistance to targeted drugs often occurs in these patients, and there is usually no good treatment for the posterior line. As previously stated, EGFR-positive patients have a special immunosuppressive TME. What’s more, EGFR mutation can be associated with increased VEGF expression (85), and a sharing downstream signaling pathways of VEGF and EGFR was revealed (82), which brought us interest in the efficacy of anti-PD-1/PD-L1 antibodies plus antiangiogenetic therapy in EGFR positive patients.
In the subgroup analysis of Impower150, ABCP brought longer PFS among patients with EGFR/ALK-mutant than BCP (40, 86). The phase 3 ORIENT-31 research recruited 444 locally advanced or metastatic NSCLC patients with EGFR-mutant who progressed after previous EGFR-TKI treatment and categorized them into sintilimab + IBI305 (a biosimilar of bevacizumab) + pemetrexed + cisplatin group, sintilimab + pemetrexed + cisplatin group, and chemotherapy alone group. After a median follow-up of 9.8 months, the first group exhibited prolonged PFS relative to the chemotherapy alone group (6.9 months vs. 4.3 months, HR 0.46) (64). Another phase Ib/II clinical trial enrolled 43 advanced NSCLC patients with EGFR+/ALK+ who had previously received targeted therapy. They received camrelizumab plus apatinib and showed a confirmed ORR of 18.6%, a clinical benefit response rate of 27.9%, and an mPFS of 2.8 months, assessed as moderate antitumor activity and acceptable safety profile (63). There was also research about uncommon EGFR mutations, enrolling 16 patients with EGFR mutations including Exon 18 (G719X, E709A), 20 insertion, 21 L861Q, and compound mutation and administrating atezolizumab + bevacizumab + carboplatin + (nab-)paclitaxel as first or further line treatment. ORR turned out to be 81.3%, disease control rate (DCR) was 87.5%, and mPFS reached 13.6 months (87). However, according to a meta-analysis evaluating the benefit-predicting factors of metastatic NSCLC, chemoimmunotherapy led to longer PFS regardless of EGFR or ALK status, whereas Impower130 exhibited no significant PFS superiority with non-bevacizumab-based regimens in EGFR/ALK+ subgroups (88, 89).
There were more studies enrolling patients with EGFR or with other driver gene mutations, included in Table 2. Overall, the efficacy of this combined regimen in treated or untreated EGFR+ patients has been proven, but further large-scale clinical trials are needed to confirm its prominent advantages.
6 Immunotherapy combined with antiangiogenic therapy in patients with distant organ metastases
Clinical trials have demonstrated the activity of immunotherapy in patients with brain and liver metastases (90, 91), and the activity was preliminarily confirmed by subgroup analyses of later clinical trials. The key subgroup analysis of Impower150 revealed a prolonged PFS in ABCP arm compared with BCP arm (13.2 months vs. 9.1 months) in liver metastatic ITT patients (92). Relatively prolonged mPFS was observed in patients with all bone, liver, and brain metastases in the phase III TASUKI-52 trial (46). However, brain metastasis could still be a significant risk factor for the combination therapy according to a phase Ib trial (55).
A retrospective study found the combination treatment of camrelizumab, apatinib, and radiotherapy was associated with lower levels of TRIM27, SCC-Ag, and CYFRA21-1, which might promote tumor infiltration, proliferation, and activation (67). As patients with oligometastases are in the transition from primary to extensive metastases, these patients will benefit from treatment effective for metastasis, which is worthy of further exploration.
Table 2 lists most searchable clinical studies on the combination of immunotherapy and antiangiogenic therapy. Only prospective trials and retrospective studies with more than 50 participants were selected.
7 Predictive biomarkers of ICI plus antiangiogenetic treatment
Immunotherapy has changed the treatment landscape of NSCLC and has shown durable response rates in some refractory tumors. However, some treated patients show no response and serious immune-related side effects. Therefore, there is an urgent need for immunotherapeutic markers to help select people who are likely to benefit from immunotherapy, Multiple biomarkers have been explored over the years, and some emerging biomarkers are also under exploration (31). There are relatively mature studies of biomarkers separately in the immunotherapy field and antiangiogenetic field. However, few biomarkers focus specifically on the field of combination therapy. Here we summarize all common biomarkers, hoping to shed light on future treatments, especially in the field of combination therapy.
7.1 PD-L1 expression
Based on extensive research, ICI treatment plays a better role in immune inflamed tumors compared with noninflamed types (93). Tumors under immune inflamed conditions are characterized by high PD-L1 expression, high CD8+ T cell density, or strong IFN-γ cytolytic T cell signature (94). The expression of PD-L1 is the first established biomarker that can predict the clinical efficacy of PD-1/PD-L1 inhibitors, which has shown guiding significance in the treatment of many tumors, and the NCCN treatment guidelines recommend PD-L1 expression as a biomarker for immunotherapy (39). Most clinical studies of anti-PD-1/PD-L1 antibodies plus antiangiogenetic therapy included PD-L1 subgroup analysis. In Impower150, PFS in ABCP group was 8.0 months among patients with PD-L1<50% and 12.6 months for the subgroup with high PD-L1 expression (40). In another camrelizumab plus apatinib trial, a trend of better efficacy was observed in PD-L1 positive subgroup defined as TPS ≥1%, but without statistical significance (ORR 36.0% vs. 22.7%, P=0.20; mPFS 6.8 vs. 5.1 months, P=0.29) (54). Although PD-L1 expression is not the optimal biomarker, and its predictive role varies between different studies, which might be attributed to different assay methods, reagents sources, and selected thresholds, it is the best available biomarker to assess whether a patient is a suitable candidate in current clinical practice (39, 95, 96).
7.2 Tumor mutation burden
Tumor mutation burden (TMB), defined as the number of non-synonymous somatic mutations in cancer cells, is used to be another NCCN guideline recommended predictor in ICI therapy (39). Patients with high TMB levels tend to express high neoantigen levels on the surface of tumor cells that will activate the killing effect of immune cells (39). In June 2020, the FDA approved pembrolizumab for patients with unresectable or metastatic solid tumors with high TMB levels (≥10muts/Mb) that had progressed after prior therapy (97), which represented FDA acceptance of TMB as an independent biomarker for immunotherapy. However, there is a lack of consensus on the predictive value of TMB in clinical research results (98), and the lack of agreement on cut-off value and measurement standardization in clinical application led to its removal from the recommended immune biomarker by the NCCN panel in 2020 (99). In a phase Ib trial of 22 NSCLC patients, sintilimab plus anlotinib exhibited an ORR showing no significant association with TMB level (43).
Blood-based TMB (bTMB) provides a new way to measure TMB in patients who are difficult to obtain tumor tissue samples. Since high bTMB level can predict PFS benefit but not OS benefit in previous studies, Wang et al. proposed the concept of low allele frequence-bTMB (LAF-bTMB) and verified the prediction efficiency of LAF-bTMB algorithm through several international clinical cohorts (100). However, the predictor appears to have limited value at times. In a pilot study, bTMB could not stratify the PFS among 22 cases of second or further line camrelizumab plus apatinib treatment (95).
7.3 VEGF and ANG-2
Solid predictive biomarkers for VEGF signaling pathway targeted treatment have not been identified in tumor patients, whereas VEGF and angiopoietins are the key targets in related therapies (18). In the field of NSCLC, ANG-2, and βFGF was found to contribute to worse prognosis (101, 102), and higher plasma VEGF level might predict a better response to bevacizumab (102). VEGF-related molecules need to be further investigated as a potential predictive biomarker as data in response to anti-VEGF therapy or antiangiogenetic combination therapy are scarce.
7.4 Mutations in specific genes
Tumor driver genes can affect the tumor immune microenvironment and the response of immunotherapy. For example, KRAS and TP53 mutated populations tend to express higher levels of PD-L1, and the combined treatment of ABCP led to OS improvements in patients with KRAS and TP53 co-mutations in a subgroup analysis of Impower150. On the contrary, in KRAS, STK11, and KEAP1 co-mutated patients, the expression of PD-L1 was reduced, and no apparent OS improvement was observed (103). However, the addition of apatinib to camrelizumab was reported to have better clinical outcomes in patients with mutation of STK11 and/or KEAP1 in another research, which indicated that the addition of antiangiogenetic drugs to ICI might also bring reverse effect against mono-agent immunotherapy (54, 104). The underlying reason might be attributed to the involvement of STK11 and KEAP1 in angiogenesis.
7.5 Liquid biopsy biomarkers
Other circulating markers such as circulating tumor DNA (ctDNA), circulating tumor cells (CTCs), exosomes, and circulating immune cells have also attracted research interest as surrogate markers of tumor burden (94). The concentration of ctDNA was identified as one of the independent risk factors of camrelizumab plus apatinib therapy in a multivariate Cox regression analysis, while ctDNA mutational status indicated a trend of difference but was not independent (95). Although many studies have explored the predictive value of liquid biopsy in immunotherapy, there is less exploration of combination therapy.
7.6 Others
There are also other genes, proteins, cells, or indexes that describe their status that have been proposed as biomarkers in mono-agent immunotherapy, including TIL, MSI-H/dMMR, CTLA4 expression, diversity of gut microbiome, etc. (94, 96). But rarely were reported in the field of combination immunotherapy. It also appears that no anti-PD-1/PD-L1 antibody plus antiangiogenetic specific biomarker have been identified. Further research on predictive biomarkers will be necessary.
8 Conclusion
In summary, a series of clinical studies have shown that anti-angiogenic drugs and ICIs have synergistic anti-tumor effects. On the one hand, anti-angiogenic drugs can reverse the immunosuppressive state of TME and enhance the efficacy of ICIs through immune reprogramming. On the other hand, ICIs can restore the immunosupportive microenvironment, promote vascular normalization, and enhance the efficacy of anti-angiogenic drugs. ICIs combined with antiangiogenic drugs have been widely and increasingly prescribed, but they also have drug resistance leading to treatment failure, and vascular normalization has a short specific time window. Therefore, how to delay the generation of drug-resistant cells is one of the urgent problems to be solved at present. Additionally, how to extend the normalization window effectively and explore the administration time, sequence, and optimal dosage of each drug in combination therapy may become the key for further research. Last but not least, how to select the most appropriate treatment plan for different patients to achieve the purpose of precise personalized treatment is of great importance, so it is also the direction of future research to find relevant biomarkers to predict the efficacy of patients and identify potential benefit groups. To sum up, more in-depth basic experiments and clinical studies are needed to optimize the treatment regimen of ICIs combined with anti-angiogenic drugs, so as to further improve patient prognosis with NSCLC.
Author contributions
DH: Writing – original draft, Writing – review & editing. LW: Writing – original draft, Writing – review & editing. JX: Writing – review & editing. JZ: Writing – review & editing. HB: Writing – review & editing. JW: Funding acquisition, Writing – review & editing.
Funding
This work was supported by National key R&D program of China (2022YFC2505000); NSFC general program (82272796); NSFC special program (82241229); CAMS Innovation Fund for Medical Sciences (CIFMS 2022-I2M-1-009; CAMS Key Laboratory of Translational Research on Lung Cancer (2018PT31035); Ministry of Education Innovation Team development project (3332018002) and Aiyou foundation (KY201701).
Conflict of interest
The authors declare that the research was conducted in the absence of any commercial or financial relationships that could be construed as a potential conflict of interest.
Publisher’s note
All claims expressed in this article are solely those of the authors and do not necessarily represent those of their affiliated organizations, or those of the publisher, the editors and the reviewers. Any product that may be evaluated in this article, or claim that may be made by its manufacturer, is not guaranteed or endorsed by the publisher.
References
1. Howlader N, Forjaz G, Mooradian MJ, Meza R, Kong CY, Cronin KA, et al. The effect of advances in lung-cancer treatment on population mortality. N Engl J Med (2020) 383(7):640–9. doi: 10.1056/NEJMoa1916623
2. Siegel RL, Miller KD, Fuchs HE, Jemal A. Cancer statistics, 2022. CA Cancer J Clin (2022) 72(1):7–33. doi: 10.3322/caac.21708
3. Galluzzi L, Humeau J, Buqué A, Zitvogel L, Kroemer G. Immunostimulation with chemotherapy in the era of immune checkpoint inhibitors. Nat Rev Clin Oncol (2020) 17(12):725–41. doi: 10.1038/s41571-020-0413-z
4. Atanackovic D, Luetkens T. Biomarkers for checkpoint inhibition in hematologic Malignancies. Semin Cancer Biol (2018) 52(Pt 2):198–206. doi: 10.1016/j.semcancer.2018.05.005
5. Huinen ZR, Huijbers EJM, van Beijnum JR, Nowak-Sliwinska P, Griffioen AW. Anti-angiogenic agents - overcoming tumour endothelial cell anergy and improving immunotherapy outcomes. Nat Rev Clin Oncol (2021) 18(8):527–40. doi: 10.1038/s41571-021-00496-y
6. Lugano R, Ramachandran M, Dimberg A. Tumor angiogenesis: causes, consequences, challenges and opportunities. Cell Mol Life Sci (2020) 77(9):1745–70. doi: 10.1007/s00018-019-03351-7
7. Ren S, Xiong X, You H, Shen J, Zhou P. The combination of immune checkpoint blockade and angiogenesis inhibitors in the treatment of advanced non-small cell lung cancer. Front Immunol (2021) 12:689132. doi: 10.3389/fimmu.2021.689132
8. Komi DEA, Redegeld FA. Role of mast cells in shaping the tumor microenvironment. Clin Rev Allergy Immunol (2020) 58(3):313–25. doi: 10.1007/s12016-019-08753-w
9. Jiang X, Wang J, Deng X, Xiong F, Zhang S, Gong Z, et al. The role of microenvironment in tumor angiogenesis. J Exp Clin Cancer Res (2020) 39(1):204. doi: 10.1186/s13046-020-01709-5
10. Cho HD, Kim JH, Park JK, Hong SM, Kim DH, et al. Kochia scoparia seed extract suppresses VEGF-induced angiogenesis via modulating VEGF receptor 2 and PI3K/AKT/mTOR pathways. Pharm Biol (2019) 57(1):684–93. doi: 10.1080/13880209.2019.1672753
11. Mao X, Xu J, Wang W, Liang C, Hua J, Liu J, et al. Crosstalk between cancer-associated fibroblasts and immune cells in the tumor microenvironment: new findings and future perspectives. Mol Cancer (2021) 20(1):131. doi: 10.1186/s12943-021-01428-1
12. Locati M, Curtale G, Mantovani A. Diversity, mechanisms, and significance of macrophage plasticity. Annu Rev Pathol (2020) 15:123–47. doi: 10.1146/annurev-pathmechdis-012418-012718
13. Bouzin C, Brouet A, De Vriese J, Dewever J, Feron O. Effects of vascular endothelial growth factor on the lymphocyte-endothelium interactions: identification of caveolin-1 and nitric oxide as control points of endothelial cell anergy. J Immunol (2007) 178(3):1505–11. doi: 10.4049/jimmunol.178.3.1505
14. Hack SP, Zhu AX, Wang Y. Augmenting anticancer immunity through combined targeting of angiogenic and PD-1/PD-L1 pathways: challenges and opportunities. Front Immunol (2020) 11:598877. doi: 10.3389/fimmu.2020.598877
15. Mennitto A, Huber V, Ratta R, Sepe P, de Braud F, Procopio G, et al. Angiogenesis and immunity in renal carcinoma: can we turn an unhappy relationship into a happy marriage? J Clin Med (2020) 9(4):930. doi: 10.3390/jcm9040930
16. Huang Y, Goel S, Duda DG, Fukumura D, Jain RK. Vascular normalization as an emerging strategy to enhance cancer immunotherapy. Cancer Res (2013) 73(10):2943–8. doi: 10.1158/0008-5472.CAN-12-4354
17. Shweiki D, Itin A, Soffer D, Keshet E. Vascular endothelial growth factor induced by hypoxia may mediate hypoxia-initiated angiogenesis. Nature (1992) 359(6398):843–5. doi: 10.1038/359843a0
18. Fukumura D, Kloepper J, Amoozgar Z, Duda DG, Jain RK. Enhancing cancer immunotherapy using antiangiogenics: opportunities and challenges. Nat Rev Clin Oncol (2018) 15(5):325–40. doi: 10.1038/nrclinonc.2018.29
19. Riera-Domingo C, Audigé A, Granja S, Cheng WC, Ho PC, Baltazar F, et al. Immunity, hypoxia, and metabolism-the ménage à Trois of cancer: implications for immunotherapy. Physiol Rev (2020) 100(1):1–102. doi: 10.1152/physrev.00018.2019
20. Chuckran CA, Liu C, Bruno TC, Workman CJ, Vignali D. Neuropilin-1: a checkpoint target with unique implications for cancer immunology and immunotherapy. J Immunother Cancer (2020) 8(2):967–78. doi: 10.1136/jitc-2020-000967
21. Hao NB, Lü MH, Fan YH, Cao YL, Zhang ZR, Yang SM. Macrophages in tumor microenvironments and the progression of tumors. Clin Dev Immunol 2012. (2012) p:948098. doi: 10.1155/2012/948098
22. Kumar V, Patel S, Tcyganov E, Gabrilovich DI. The nature of myeloid-derived suppressor cells in the tumor microenvironment. Trends Immunol (2016) 37(3):208–20. doi: 10.1016/j.it.2016.01.004
23. De Palma M, Biziato D, Petrova TV. Microenvironmental regulation of tumour angiogenesis. Nat Rev Cancer (2017) 17(8):457–74. doi: 10.1038/nrc.2017.51
24. Huang Y, Kim BYS, Chan CK, Hahn SM, Weissman IL, Jiang W. Improving immune-vascular crosstalk for cancer immunotherapy. Nat Rev Immunol (2018) 18(3):195–203. doi: 10.1038/nri.2017.145
25. Liu YT, Sun ZJ. Turning cold tumors into hot tumors by improving T-cell infiltration. Theranostics (2021) 11(11):5365–86. doi: 10.7150/thno.58390
26. Palazon A, Tyrakis PA, Macias D, Veliça P, Rundqvist H, Fitzpatrick S, et al. An HIF-1α/VEGF-A axis in cytotoxic T cells regulates tumor progression. Cancer Cell (2017) 32(5):669–683.e5. doi: 10.1016/j.ccell.2017.10.003
27. Pircher A, Wolf D, Heidenreich A, Hilbe W, Pichler R, Heidegger I. Synergies of targeting tumor angiogenesis and immune checkpoints in non-small cell lung cancer and renal cell cancer: from basic concepts to clinical reality. Int J Mol Sci (2017) 18(11):2291–305. doi: 10.3390/ijms18112291
28. Viallard C, Larrivée B. Tumor angiogenesis and vascular normalization: alternative therapeutic targets. Angiogenesis (2017) 20(4):409–26. doi: 10.1007/s10456-017-9562-9
29. Schmittnaegel M, Rigamonti N, Kadioglu E, Cassará A, Wyser Rmili C, Kiialainen A, et al. Dual angiopoietin-2 and VEGFA inhibition elicits antitumor immunity that is enhanced by PD-1 checkpoint blockade. Sci Transl Med (2017) 9(385):eaak9670. doi: 10.1126/scitranslmed.aak9670
30. Kloepper J, Riedemann L, Amoozgar Z, Seano G, Susek K, Yu V, et al. Ang-2/VEGF bispecific antibody reprograms macrophages and resident microglia to anti-tumor phenotype and prolongs glioblastoma survival. Proc Natl Acad Sci U.S.A. (2016) 113(16):4476–81. doi: 10.1073/pnas.1525360113
31. Yi M, Jiao D, Qin S, Chu Q, Wu K, Li A. Synergistic effect of immune checkpoint blockade and anti-angiogenesis in cancer treatment. Mol Cancer (2019) 18(1):60. doi: 10.1186/s12943-019-0974-6
32. Wu SG, Shih JY. Management of acquired resistance to EGFR TKI-targeted therapy in advanced non-small cell lung cancer. Mol Cancer (2018) 17(1):38. doi: 10.1186/s12943-018-0777-1
33. Zhao S, Ren S, Jiang T, Zhu B, Li X, Zhao C, et al. Low-dose apatinib optimizes tumor microenvironment and potentiates antitumor effect of PD-1/PD-L1 blockade in lung cancer. Cancer Immunol Res (2019) 7(4):630–43. doi: 10.1158/2326-6066.CIR-17-0640
34. Tian L, Goldstein A, Wang H, Ching Lo H, Sun Kim I, Welte T, et al. Mutual regulation of tumour vessel normalization and immunostimulatory reprogramming. Nature (2017) 544(7649):250–4. doi: 10.1038/nature21724
35. Kammertoens T, Friese C, Arina A, Idel C, Briesemeister D, Rothe M, et al. Tumour ischaemia by interferon-γ resembles physiological blood vessel regression. Nature (2017) 545(7652):98–102. doi: 10.1038/nature22311
36. Zhao Y, Guo S, Deng J, Shen J, Du F, Wu X, et al. VEGF/VEGFR-targeted therapy and immunotherapy in non-small cell lung cancer: targeting the tumor microenvironment. Int J Biol Sci (2022) 18(9):3845–58. doi: 10.7150/ijbs.70958
37. Zhou C, Chen G, Huang Y, Zhou J, Lin L, Feng J, et al. Camrelizumab plus carboplatin and pemetrexed versus chemotherapy alone in chemotherapy-naive patients with advanced non-squamous non-small-cell lung cancer (CameL): a randomised, open-label, multicentre, phase 3 trial. Lancet Respir Med (2021) 9(3):305–14. doi: 10.1016/S2213-2600(20)30365-9
38. Herbst RS, Giaccone G, de Marinis F, Reinmuth N, Vergnenegre A, Barrios CH, et al. Atezolizumab for first-line treatment of PD-L1-selected patients with NSCLC. N Engl J Med (2020) 383(14):1328–39. doi: 10.1056/NEJMoa1917346
40. Socinski MA, Nishio M, Jotte RM, Cappuzzo F, Orlandi F, Stroyakovskiy D, et al. Atezolizumab for first-line treatment of metastatic nonsquamous NSCLC. N Engl J Med (2018) 378(24):2288–301. doi: 10.1056/NEJMoa1716948
41. Socinski MA, Nishio M, Jotte RM, Cappuzzo F, Orlandi F, Stroyakovskiy D, et al. IMpower150 final overall survival analyses for atezolizumab plus bevacizumab and chemotherapy in first-line metastatic nonsquamous NSCLC. J Thorac Oncol (2021) 16(11):1909–24. doi: 10.1016/j.jtho.2021.07.009
42. Herbst RS, Arkenau HT, Bendell J, Arrowsmith E, Wermke M, Soriano A, et al. Phase 1 expansion cohort of ramucirumab plus pembrolizumab in advanced treatment-naive NSCLC. J Thorac Oncol (2021) 16(2):289–98. doi: 10.1016/j.jtho.2020.10.004
43. Chu T, Zhong R, Zhong H, Zhang B, Zhang W, Shi C, et al. Phase 1b study of sintilimab plus anlotinib as first-line therapy in patients with advanced NSCLC. J Thorac Oncol (2021) 16(4):643–52. doi: 10.1016/j.jtho.2020.11.026
44. Herbst RS, Arkenau HT, Santana-Davila R, Calvo E, Paz-Ares L, Cassier PA, et al. Ramucirumab plus pembrolizumab in patients with previously treated advanced non-small-cell lung cancer, gastro-oesophageal cancer, or urothelial carcinomas (JVDF): a multicohort, non-randomised, open-label, phase 1a/b trial. Lancet Oncol (2019) 20(8):1109–23. doi: 10.1016/S1470-2045(19)30458-9
45. Kanda S, Goto K, Shiraishi H, Kubo E, Tanaka A, Utsumi H, et al. Safety and efficacy of nivolumab and standard chemotherapy drug combination in patients with advanced non-small-cell lung cancer: a four arms phase Ib study. Ann Oncol (2016) 27(12):2242–50. doi: 10.1093/annonc/mdw416
46. Sugawara S, Lee JS, Kang JH, Kim HR, Inui N, Hida T, et al. Nivolumab with carboplatin, paclitaxel, and bevacizumab for first-line treatment of advanced nonsquamous non-small-cell lung cancer. Ann Oncol (2021) 32(9):1137–47. doi: 10.1016/j.annonc.2021.06.004
47. Ren S, He J, Fang Y, Chen G, Ma Z, Chen J, et al. Camrelizumab plus apatinib in treatment-naive patients with advanced nonsquamous NSCLC: A multicenter, open-label, single-arm, phase 2 trial. JTO Clin Res Rep (2022) 3(5):100312. doi: 10.1016/j.jtocrr.2022.100312
48. Seto T, Nosaki K, Shimokawa M, Toyozawa R, Sugawara S. Phase II study of atezolizumab with bevacizumab for non-squamous non-small cell lung cancer with high PD-L1 expression (@Be Study). J Immunother Cancer (2022) 10(2):4025–33. doi: 10.1136/jitc-2021-004025
49. Provencio M, Ortega AL, Coves-Sarto J, Calvo V, Marsé-Fabregat R, Dómine M, et al. Atezolizumab plus bevacizumab as first-line treatment for patients with metastatic nonsquamous non-small cell lung cancer with high tumor mutation burden: A nonrandomized controlled trial. JAMA Oncol (2022) 9(3):344–53. doi: 10.1001/jamaoncol.2022.5959
50. Ardeshir-Larijani F, Althouse SK, Leal T, Feldman LE, Hejleh TA. A phase II trial of atezolizumab plus carboplatin plus pemetrexed plus bevacizumab in the treatment of patients with stage IV non-squamous non-small cell lung cancer: big ten cancer research consortium (BTCRC)- LUN 17-139. Clin Lung Cancer (2022) 23(7):578–84. doi: 10.1016/j.cllc.2022.07.001
51. Rizvi NA, Antonia SJ, Shepherd FA, Chow LQ, Goldman J, Shen Y. Nivolumab (Anti-PD-1; BMS-936558, ONO-4538) maintenance as monotherapy or in combination with bevacizumab (BEV) for non-small cell lung cancer (NSCLC) previously treated with chemotherapy: metastatic non-small cell lung cancer. Int J Radiat OncologyBiologyPhysics (2014) 90(5, Supplement):S32. doi: 10.1016/j.ijrobp.2014.08.206
52. Taylor MH, Lee CH, Makker V, Rasco D, Dutcus CE, Wu J, et al. Phase IB/II trial of lenvatinib plus pembrolizumab in patients with advanced renal cell carcinoma, endometrial cancer, and other selected advanced solid tumors. J Clin Oncol (2020) 38(11):1154–63. doi: 10.1200/JCO.19.01598
53. Bang YJ, Golan T, Dahan L, Fu S, Moreno V, Park K, et al. Ramucirumab and durvalumab for previously treated, advanced non-small-cell lung cancer, gastric/gastro-oesophageal junction adenocarcinoma, or hepatocellular carcinoma: An open-label, phase Ia/b study (JVDJ). Eur J Cancer (2020) 137:272–84. doi: 10.1016/j.ejca.2020.06.007
54. Zhou C, Wang Y, Zhao J, Chen G, Liu Z, Gu K, et al. Efficacy and biomarker analysis of camrelizumab in combination with apatinib in patients with advanced nonsquamous NSCLC previously treated with chemotherapy. Clin Cancer Res (2021) 27(5):1296–304. doi: 10.1158/1078-0432.CCR-20-3136
55. Zhou N, Jiang M, Li T, Zhu J, Liu K, Hou H, et al. Anlotinib combined with anti-PD-1 antibody, camrelizumab for advanced NSCLCs after multiple lines treatment: An open-label, dose escalation and expansion study. Lung Cancer (2021) 160:111–7. doi: 10.1016/j.lungcan.2021.08.006
56. Wang Y, Shi X, Qi Q, Ye B, Zou Z. Safety of anlotinib capsules combined with PD-1 inhibitor camrelizumab in the third-line treatment of advanced non-small-cell lung cancer and their effect on serum tumor markers. J Healthc Eng (2021) 2021:2338800. doi: 10.1155/2021/2338800
57. Kitano S, Fujiwara Y, Shimizu T, Iwasa S, Yonemori K, Kondo S, et al. A feasibility study of lenvatinib plus pembrolizumab in Japanese patients with advanced solid tumors. Cancer Chemother Pharmacol (2022) 90(6):523–9. doi: 10.1007/s00280-022-04480-w
58. Herzog BH, Waqar SN, Devarakonda S, Ward JP, Gao F, Govindan R, et al. Ramucirumab plus atezolizumab in patients with stage IV non-small cell lung cancer previously treated with immune checkpoint inhibitors. Lung Cancer (2022) 173:101–6. doi: 10.1016/j.lungcan.2022.09.011
59. Reckamp KL, Redman MW, Dragnev KH, Minichiello K VII. Phase II randomized study of ramucirumab and pembrolizumab versus standard of care in advanced non-small-cell lung cancer previously treated with immunotherapy-lung-MAP S1800A. J Clin Oncol (2022) 40(21):2295–306. doi: 10.1200/JCO.22.00912
60. Gao G, Zhao J, Ren S, Wang Y, Chen G, Chen J, et al. Efficacy and safety of camrelizumab plus apatinib as second-line treatment for advanced squamous non-small cell lung cancer. Ann Transl Med (2022) 10(8):441. doi: 10.21037/atm-21-4792
61. Lee J, Koh J, Kim HK, Hong S, Kim K, Park S, et al. Bevacizumab plus atezolizumab after progression on atezolizumab monotherapy in pretreated patients with NSCLC: an open-label, two-stage, phase 2 trial. J Thorac Oncol (2022) 17(7):900–8. doi: 10.1016/j.jtho.2022.04.001
62. Lam TC, Tsang KC, Choi HC, Lee VH, Lam KO, Chiang CL, et al. Combination atezolizumab, bevacizumab, pemetrexed and carboplatin for metastatic EGFR mutated NSCLC after TKI failure. Lung Cancer (2021) 159:18–26. doi: 10.1016/j.lungcan.2021.07.004
63. Gao G, Ni J, Wang Y, Ren S, Liu Z, Chen G, et al. Efficacy and safety of camrelizumab plus apatinib in previously treated patients with advanced non-small cell lung cancer harboring EGFR or ALK genetic aberration. Transl Lung Cancer Res (2022) 11(6):964–74. doi: 10.21037/tlcr-22-22
64. Lu S, Wu L, Jian H, Chen Y, Wang Q, Fang J, et al. Sintilimab plus bevacizumab biosimilar IBI305 and chemotherapy for patients with EGFR-mutated non-squamous non-small-cell lung cancer who progressed on EGFR tyrosine-kinase inhibitor therapy (ORIENT-31): first interim results from a randomised, double-blind, multicentre, phase 3 trial. Lancet Oncol (2022) 23(9):1167–79. doi: 10.1016/S1470-2045(22)00382-5
65. Zhang X, Zeng L, Li Y, Xu Q, Yang H, Lizaso A, et al. Anlotinib combined with PD-1 blockade for the treatment of lung cancer: a real-world retrospective study in China. Cancer Immunol Immunother (2021) 70(9):2517–28. doi: 10.1007/s00262-021-02869-9
66. Wang P, Fang X, Yin T, Tian H, Yu J, Teng F. Efficacy and safety of anti-PD-1 plus anlotinib in patients with advanced non-small-cell lung cancer after previous systemic treatment failure-A retrospective study. Front Oncol (2021) 11:628124. doi: 10.3389/fonc.2021.628124
67. Ye W, Song Z, Lin Z. Camrelizumab and apatinib combined with radiotherapy is effective in advanced oligometastatic non-small-cell lung cancer. Evid Based Complement Alternat Med (2022) 2022:5067402. doi: 10.1155/2022/5067402
68. Liu L, Bai H, Wang C, Seery S, Wang Z, Duan J, et al. Efficacy and safety of first-line immunotherapy combinations for advanced NSCLC: A systematic review and network meta-analysis. J Thorac Oncol (2021) 16(7):1099–117. doi: 10.1016/j.jtho.2021.03.016
69. Siciliano MA, Caridà G, Ciliberto D, d'Apolito M, Pelaia C, Caracciolo D. Efficacy and safety of first-line checkpoint inhibitors-based treatments for non-oncogene-addicted non-small-cell lung cancer: a systematic review and meta-analysis. ESMO Open (2022) 7(3):100465. doi: 10.1016/j.esmoop.2022.100465
70. Yang SC, Ou HT, Su WC, Wang SY. Cost-effectiveness of first-line immunotherapies for advanced non-small cell lung cancer. Cancer Med (2023) 12(7):8838–50. doi: 10.1002/cam4.5632
71. Criss SD, Mooradian MJ, Watson TR, Gainor JF, Reynolds KL, Kong CY. Cost-effectiveness of atezolizumab combination therapy for first-line treatment of metastatic nonsquamous non-small cell lung cancer in the United States. JAMA Netw Open (2019) 2(9):e1911952. doi: 10.1001/jamanetworkopen.2019.11952
72. Rittmeyer A, Barlesi F, Waterkamp D, Park K, Ciardiello F, von Pawel J. Atezolizumab versus docetaxel in patients with previously treated non-small-cell lung cancer (OAK): a phase 3, open-label, multicentre randomised controlled trial. Lancet (2017) 389(10066):255–65. doi: 10.1016/S0140-6736(16)32517-X
73. Leonetti A, Sharma S, Minari R, Perego P, Giovannetti E, Tiseo M. Resistance mechanisms to osimertinib in EGFR-mutated non-small cell lung cancer. Br J Cancer (2019) 121(9):725–37. doi: 10.1038/s41416-019-0573-8
74. Bose D, Meric-Bernstam F, Hofstetter W, Reardon DA, Flaherty KT, Ellis LM. Vascular endothelial growth factor targeted therapy in the perioperative setting: implications for patient care. Lancet Oncol (2010) 11(4):373–82. doi: 10.1016/S1470-2045(09)70341-9
75. Qiao T, Zhao J, Xin X, Xiong Y, Guo W, Meng F, et al. Combined pembrolizumab and bevacizumab therapy effectively inhibits non-small-cell lung cancer growth and prevents postoperative recurrence and metastasis in humanized mouse model. Cancer Immunol Immunother (2022) 72(5):1169–81. doi: 10.1007/s00262-022-03318-x
76. Zhai P, Niu X, Zheng K. A dramatic response to toripalimab with chemotherapy and antiangiogenic agent followed by surgery in a stage IIIB lung adenocarcinoma patient with an uncommon EGFR mutation: A case report. Front Oncol (2022) 12:915628. doi: 10.3389/fonc.2022.915628
77. Jin C, Yang B. Dramatic response of pulmonary sarcomatoid carcinoma to nivolumab combined with anlotinib: A case report. Case Rep Oncol (2020) 13(2):601–5. doi: 10.1159/000507568
78. Akaba T, Shiota Y, Onizawa F, Isaka T, Nagashima Y, Tagaya E. Recurrent spindle cell carcinoma of the lung successfully treated by chemoimmunotherapy. Respirol Case Rep (2021) 9(6):e00757. doi: 10.1002/rcr2.757
79. Planchard D, Popat S, Kerr K, Novello S, Smit EF, Faivre-Finn C, et al. Metastatic non-small cell lung cancer: ESMO Clinical Practice Guidelines for diagnosis, treatment and follow-up. Ann Oncol (2018) 29(Suppl 4):iv192–237. doi: 10.1093/annonc/mdy275
80. Jia Y, Li X, Jiang T, Zhao S, Zhao C, Zhang L, et al. EGFR-targeted therapy alters the tumor microenvironment in EGFR-driven lung tumors: Implications for combination therapies. Int J Cancer (2019) 145(5):1432–44. doi: 10.1002/ijc.32191
81. Dong ZY, Zhang JT, Liu SY, Su J, Zhang C, Xie Z, et al. EGFR mutation correlates with uninflamed phenotype and weak immunogenicity, causing impaired response to PD-1 blockade in non-small cell lung cancer. Oncoimmunology (2017) 6(11):e1356145. doi: 10.1080/2162402X.2017.1356145
82. Le X, Nilsson M, Goldman J, Reck M, Nakagawa K, Kato T, et al. Dual EGFR-VEGF pathway inhibition: A promising strategy for patients with EGFR-mutant NSCLC. J Thorac Oncol (2021) 16(2):205–15. doi: 10.1016/j.jtho.2020.10.006
83. Xu L, Nilsson MB, Saintigny P, Cascone T, Herynk MH, Du Z, et al. Epidermal growth factor receptor regulates MET levels and invasiveness through hypoxia-inducible factor-1alpha in non-small cell lung cancer cells. Oncogene (2010) 29(18):2616–27. doi: 10.1038/onc.2010.16
84. Mazieres J, Drilon A, Lusque A, Mhanna L, Cortot AB, Mezquita L, et al. Immune checkpoint inhibitors for patients with advanced lung cancer and oncogenic driver alterations: results from the IMMUNOTARGET registry. Ann Oncol (2019) 30(8):1321–8. doi: 10.1093/annonc/mdz167
85. Reinmuth N, Jauch A, Xu EC, Muley T, Granzow M, Hoffmann H, et al. Correlation of EGFR mutations with chromosomal alterations and expression of EGFR, ErbB3 and VEGF in tumor samples of lung adenocarcinoma patients. Lung Cancer (2008) 62(2):193–201. doi: 10.1016/j.lungcan.2008.03.011
86. Reck M, Mok TSK, Nishio M, Jotte RM, Cappuzzo F, Orlandi F, et al. Atezolizumab plus bevacizumab and chemotherapy in non-small-cell lung cancer (IMpower150): key subgroup analyses of patients with EGFR mutations or baseline liver metastases in a randomised, open-label phase 3 trial. Lancet Respir Med (2019) 7(5):387–401. doi: 10.1016/S2213-2600(19)30084-0
87. Trummer A, Bethge A, Dickgreber N, Dittrich I, Golpon H, Hoffknecht P. NSCLC with uncommon EGFR mutations treated with atezolizumab plus bevacizumab and chemotherapy. Lung Cancer (2022) 174:141–5. doi: 10.1016/j.lungcan.2022.11.006
88. El-Osta HE, Mott FE, Burt BM, Wang DY, Sabichi AL. Predictors of benefits from frontline chemoimmunotherapy in stage IV non-small-cell lung cancer: a meta-analysis. Oncoimmunology (2019) 8(12):e1665974. doi: 10.1080/2162402X.2019.1665974
89. West H, McCleod M, Hussein M, Morabito A, Rittmeyer A, Conter HJ, et al. Atezolizumab in combination with carboplatin plus nab-paclitaxel chemotherapy compared with chemotherapy alone as first-line treatment for metastatic non-squamous non-small-cell lung cancer (IMpower130): a multicentre, randomised, open-label, phase 3 trial. Lancet Oncol (2019) 20(7):924–37. doi: 10.1016/S1470-2045(19)30167-6
90. Goldberg SB, Schalper KA, Gettinger SN, Mahajan A, Herbst RS, et al. Pembrolizumab for management of patients with NSCLC and brain metastases: long-term results and biomarker analysis from a non-randomised, open-label, phase 2 trial. Lancet Oncol (2020) 21(5):655–63. doi: 10.1016/S1470-2045(20)30111-X
91. Gadgeel S, Rodríguez-Abreu D, Speranza G, Esteban E, Felip E, Dómine M, et al. Updated analysis from KEYNOTE-189: pembrolizumab or placebo plus pemetrexed and platinum for previously untreated metastatic nonsquamous non-small-cell lung cancer. J Clin Oncol (2020) 38(14):1505–17. doi: 10.1200/JCO.19.03136
92. Socinski MA, Mok TS, Nishio M, Jotte RM, Cappuzzo F, Orlandi F, et al. Abstract CT216: IMpower150 final analysis: Efficacy of atezolizumab (atezo) + bevacizumab (bev) and chemotherapy in first-line (1L) metastatic nonsquamous (nsq) non-small cell lung cancer (NSCLC) across key subgroups. Cancer Res (2020) 80(16_Supplement):CT216–6. doi: 10.1158/1538-7445.AM2020-CT216
93. Yagi T, Baba Y, Ishimoto T, Iwatsuki M, Miyamoto Y, Yoshida N, et al. PD-L1 expression, tumor-infiltrating lymphocytes, and clinical outcome in patients with surgically resected esophageal cancer. Ann Surg (2019) 269(3):471–8. doi: 10.1097/SLA.0000000000002616
94. Hegde PS, Karanikas V, Evers S. The where, the when, and the how of immune monitoring for cancer immunotherapies in the era of checkpoint inhibition. Clin Cancer Res (2016) 22(8):1865–74. doi: 10.1158/1078-0432.CCR-15-1507
95. Chen Y, Li X, Liu G, Chen S, Xu M, Song L, et al. ctDNA concentration, MIKI67 mutations and hyper-progressive disease related gene mutations are prognostic markers for camrelizumab and apatinib combined multiline treatment in advanced NSCLC. Front Oncol (2020) 10:1706. doi: 10.3389/fonc.2020.01706
96. Herbst RS, Soria JC, Kowanetz M, Fine GD, Hamid O, Gordon MS, et al. Predictive correlates of response to the anti-PD-L1 antibody MPDL3280A in cancer patients. Nature (2014) 515(7528):563–7. doi: 10.1038/nature14011
97. Nassar AH, Adib E, Abou Alaiwi S, El Zarif T, Groha S, Akl EW, et al. Ancestry-driven recalibration of tumor mutational burden and disparate clinical outcomes in response to immune checkpoint inhibitors. Cancer Cell (2022) 40(10):1161–1172.e5. doi: 10.1016/j.ccell.2022.08.022
98. Sholl LM, Hirsch FR, Hwang D, Botling J, Lopez-Rios F, Bubendorf L, et al. The promises and challenges of tumor mutation burden as an immunotherapy biomarker: A perspective from the international association for the study of lung cancer pathology committee. J Thorac Oncol (2020) 15(9):1409–24. doi: 10.1016/j.jtho.2020.05.019
99. Ettinger DS, Wood DE, Aisner DL, Akerley W, Bauman JR, Bharat A, et al. NCCN guidelines insights: non-small cell lung cancer, version 2.2021. J Natl Compr Canc Netw (2021) 19(3):254–66. doi: 10.6004/jnccn.2021.0013
100. Wang Z, Duan J, Wang G, Zhao J, Xu J, Han J, et al. Allele frequency-adjusted blood-based tumor mutational burden as a predictor of overall survival for patients with NSCLC treated with PD-(L)1 inhibitors. J Thorac Oncol (2020) 15(4):556–67. doi: 10.1016/j.jtho.2019.12.001
101. Coelho AL, Gomes MP, Catarino RJ, Rolfo C, Medeiros RM, Araújo AM. CSF-1 and Ang-2 serum levels - prognostic and diagnostic partners in non-small cell lung cancer. ESMO Open (2018) 3(5):e000349. doi: 10.1136/esmoopen-2018-000349
102. Farhat FS, Tfayli A, Fakhruddin N, Mahfouz R, Otrock ZK, Alameddine RS, et al. Expression, prognostic and predictive impact of VEGF and bFGF in non-small cell lung cancer. Crit Rev Oncol Hematol (2012) 84(2):149–60. doi: 10.1016/j.critrevonc.2012.02.012
103. West HJ, McCleland M, Cappuzzo F, Reck M, Mok TS, Jotte RM, et al. Clinical efficacy of atezolizumab plus bevacizumab and chemotherapy in KRAS-mutated non-small cell lung cancer with STK11, KEAP1, or TP53 comutations: subgroup results from the phase III IMpower150 trial. J Immunother Cancer (2022) 10(2):3027–38. doi: 10.1136/jitc-2021-003027
Keywords: lung cancer, immunology, anti-angiogeneic therapy, research advance, immune checkpoint inhibitors
Citation: He D, Wang L, Xu J, Zhao J, Bai H and Wang J (2023) Research advances in mechanism of antiangiogenic therapy combined with immune checkpoint inhibitors for treatment of non-small cell lung cancer. Front. Immunol. 14:1265865. doi: 10.3389/fimmu.2023.1265865
Received: 24 July 2023; Accepted: 26 September 2023;
Published: 16 October 2023.
Edited by:
Yutian Zou, Sun Yat-sen University Cancer Center (SYSUCC), ChinaReviewed by:
Jean-Marc Barret, GamaMabs Pharma, FranceMarion Ferreira, Centre Hospitalier Universitaire de Tours, France
Copyright © 2023 He, Wang, Xu, Zhao, Bai and Wang. This is an open-access article distributed under the terms of the Creative Commons Attribution License (CC BY). The use, distribution or reproduction in other forums is permitted, provided the original author(s) and the copyright owner(s) are credited and that the original publication in this journal is cited, in accordance with accepted academic practice. No use, distribution or reproduction is permitted which does not comply with these terms.
*Correspondence: Jie Wang, emxodXhpQDE2My5jb20=; Hua Bai, YmFpaHVhaGJAc2luYS5jb20=
†These authors have contributed equally to this work and share first authorship