- 1Department of Medicine I, Division of Hematology and Hemostaseology, Medical University of Vienna, Vienna, Austria
- 2Cell Therapy Clinical Pharmacology and Modeling, Takeda, Boston, MA, United States
- 3Department of Hematology, Hospital Clínic de Barcelona, Barcelona, Spain
- 4Institut für Med. Mikrobiologie, Immunologie und Hygiene, Technische Universität Munich, Munich, Germany
- 5Hematology Department, Hospital Universitari Vall d’Hebron, Barcelona, Spain
- 6Experimental Hematology Unit, IRCCS San Raffaele Scientific Institute, Milano, Italy
- 7Bayer Aktiengesellschaft (AG), Business Development & Licensing & Open Innovation (OI), Pharmaceuticals, Berlin, Germany
- 8Lehrstuhl für Zelluläre Immuntherapie, Medizinische Klinik und Poliklinik II, Universitätsklinikum Würzburg, Würzburg, Germany
- 9ITTM S.A. (Information Technology for Translational Medicine), Esch-sur-Alzette, Luxembourg
- 10Institut de Recherches Internationales Servier, Suresnes, France
- 11Legal and Regulatory Affairs Committee of the European Society for Blood and Marrow Transplantation, Leiden, Netherlands
Lymphodepletion (LD) or conditioning is an essential step in the application of currently used autologous and allogeneic chimeric antigen receptor T-cell (CAR-T) therapies as it maximizes engraftment, efficacy and long-term survival of CAR-T. Its main modes of action are the depletion and modulation of endogenous lymphocytes, conditioning of the microenvironment for improved CAR-T expansion and persistence, and reduction of tumor load. However, most LD regimens provide a broad and fairly unspecific suppression of T-cells as well as other hematopoietic cells, which can also lead to severe side effects, particularly infections. We reviewed 1271 published studies (2011-2023) with regard to current LD strategies for approved anti-CD19 CAR-T products for large B cell lymphoma (LBCL). Fludarabine (Flu) and cyclophosphamide (Cy) (alone or in combination) were the most commonly used agents. A large number of different schemes and combinations have been reported. In the respective schemes, doses of Flu and Cy (range 75-120mg/m2 and 750-1.500mg/m2) and wash out times (range 2-5 days) differed substantially. Furthermore, combinations with other agents such as bendamustine (benda), busulfan or alemtuzumab (for allogeneic CAR-T) were described. This diversity creates a challenge but also an opportunity to investigate the impact of LD on cellular kinetics and clinical outcomes of CAR-T. Only 21 studies explicitly investigated in more detail the influence of LD on safety and efficacy. As Flu and Cy can potentially impact both the in vivo activity and toxicity of CAR-T, a more detailed analysis of LD outcomes will be needed before we are able to fully assess its impact on different T-cell subsets within the CAR-T product. The T2EVOLVE consortium propagates a strategic investigation of LD protocols for the development of optimized conditioning regimens.
Background
Adoptive cell transfer (ACT) for the treatment of malignancies has become one of the most active and fruitful developments in therapeutic advances against cancer in the past 3 decades. Not so long ago, a series of studies lead by Dr. Rosenberg opened a new field by proposing to re-infuse in vitro expanded and IL-2-stimulated tumor-infiltrating lymphocytes to overcome immunotolerance in patients with metastatic melanoma and achieve tumour-control (1). Three decades later, translational research has further developed procedures, including direct activation of immune-effector cells against specific targets in an MHC-independent manner (2, 3). In this respect, T-cells modified to express chimeric antigen receptors (CAR-T) demonstrated the potential to achieve deep and durable remissions across a wide variety of hematological malignancies (4–27) and are now established as an important part of antineoplastic therapies in lymphoproliferative diseases (28–30). Expansion to new hematologic indications, solid tumors, autoimmune and infectious diseases is on the horizon (31). As of September 2023, there are six FDA and EMA approved products, improving the lives of children and adults with B-Cell Acute Lymphoblastic Leukemia (B-ALL), various forms of B-cell Non-Hodgkin Lymphoma (NHL) and Multiple Myeloma (MM). Many more therapies are currently going through different phases of clinical trials. Approved CD19-CAR-T therapies show high response rates in B-ALL and B-NHL, depending on the CAR-T product, line of treatment and specific indication, with unprecedented long-term progression-free and overall survival in patients with relapsed or refractory disease (11, 12, 14–16, 32) The same holds true for B-cell maturation antigen (BCMA) directed CAR-T for MM (24, 25), but unfortunately a considerable proportion of these (high-risk) patients still relapse after or are refractory to CAR-T therapy. Hence, there is still a need to improve efficacy of many steps in the CAR-T treatment sequence including T-cell quality, product characteristics, manufacturing, lymphodepleting regimens as well as post-treatment management.
Lymphodepletion (LD) has been identified as a critical factor playing a major role in the outcomes achieved with CAR-T treatment (33).
The main purposes of LD are (1) the reduction of endogenous lymphocytes to prepare a niche for engraftment of CAR-T infusions and to support their long-term activity; (2) the reduction of tumor cells to avoid rapid exhaustion of CAR-T; (3) preparation and reprogramming of the microenvironment and soluble factors to ensure optimal engraftment, homing and long-term survival of CAR-T (Figure 1).
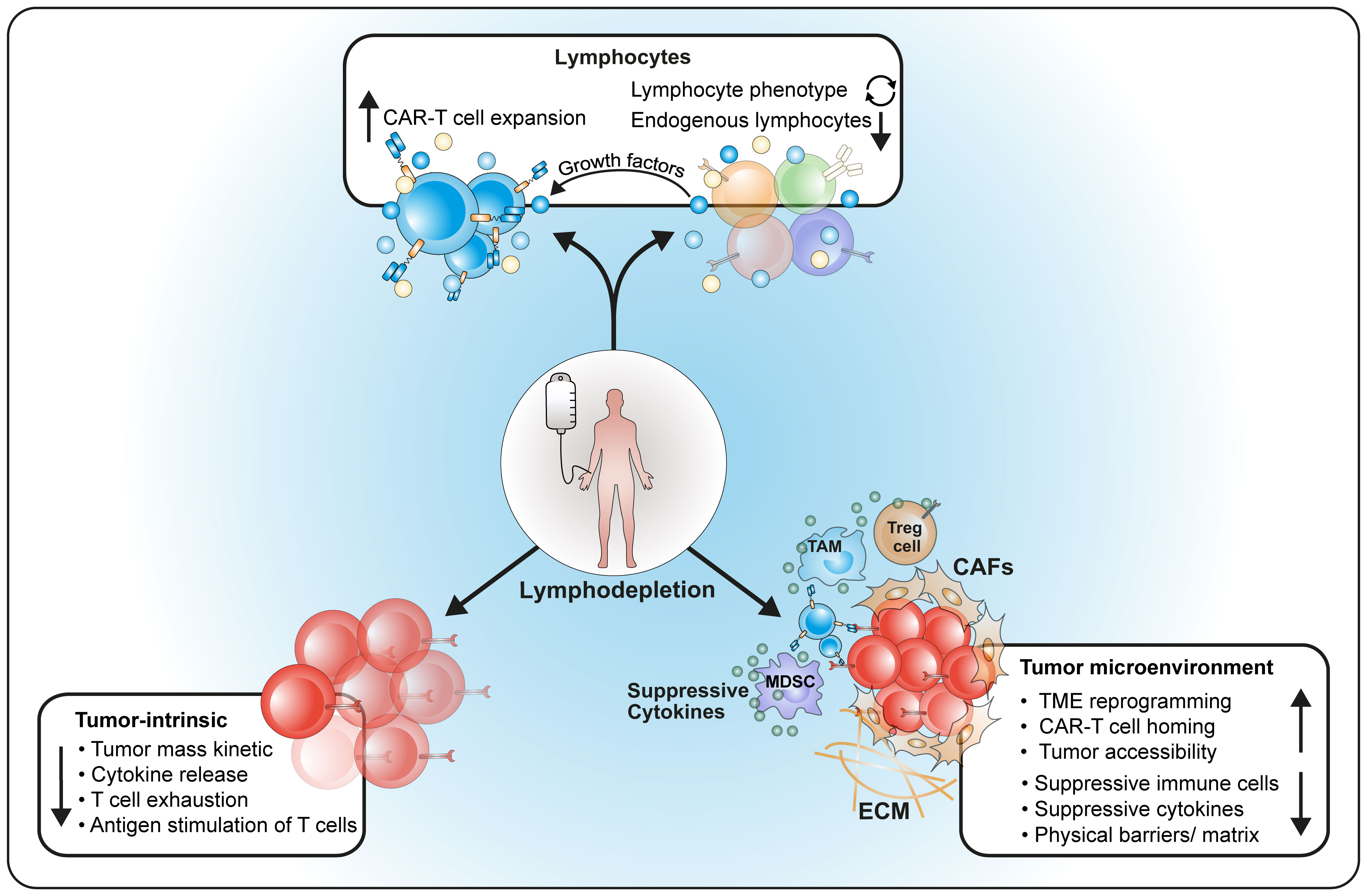
Figure 1 Major effects of lymphodepleting therapy on the host. LD influences (1) number and composition of host lymphocytes as well as cytokine production; (2) reduces the tumor and modulates its behavior; (3) reprograms the host microenvironment for better homing of CAR-T cells.
This is the reason why chemotherapeutic agents with both cytoreductive activity against tumor cells as well as T-cells are commonly used in LD. The most common schedules for LD (Fludarabine (Flu), cyclophosphamide (Cy) and combinations thereof) are based on the paradigm of pre-conditioning in small trials with tumor-infiltrating lymphocytes (34, 35) as well as for allogeneic hematopoietic stem cell transplantation (36).
Differences in LD regimens and dosages depend on the targeting disease (e.g. ALL, NHL, MM or solid tumors) as well as on the T-cell source (autologous vs. allogeneic). However, while almost every patient receives LD, the actual evidence for their activity as well as the underlying experimental data are surprisingly scarce (37, 38).
Moreover, different LD regimens are usually linked to specific CAR-T products in use, making evaluations in regard to their influence on clinical outcome nearly impossible. This is a major obstacle encountered in head-to-head comparisons and meta-analysis.
Recent evidence from population-based pharmacokinetic studies suggests that efficacy and toxicity of LD differ even within the same regimen (37–39).
Therefore, studies investigating the activity and toxicity of LD in depth are still needed.
Focusing on malignant diseases, we here review the rationale and current evidence for various LD regimens and dosages, adjustments and novel approaches with the intention to facilitate selection of research topics for this important aspect of CAR-T therapy.
Methods
This review is based on a systematic search of the literature, with the initial intention to perform a meta-analysis on LD.
The preliminary aim to identify publications on different doses of lymphodepletion was conducted on the 14.03.2023 via PubMed including the terms (“LYMPHODEPLETION” OR “CONDITIONING” OR “PREMEDICATION”), (“CART” OR “CAR-T” OR “CAR-T CELLS” OR “CHIMERIC ANTIGEN RECEPTOR T-CELLS”) and (“CD-19” OR “CD19”) delivering 21 studies of interest. While a more thorough search of those publications did not show the variety and comparability of lymphodepleting regimens, a broader analysis, including all 1271 published studies on approved anti-CD19 CART products for LBCL (axicabtagene ciloleucel, tisagenlecleucel and lisocabtagene maraleucel), only showed specific data on lymphodepleting regimens in 0.016% of those. A search of currently ongoing, planned or completed clinical trials was conducted on the 16.07.2023 using the search terms “CAR-T-Cells” and “Lymphodepletion” showed 171 results which are displayed in Table 1.
It became clear that due to the lack of conclusive data and the close link of individual LD regimens to specific CAR-T products, a meaningful meta-analysis of response and toxicity is currently not feasible.
Thus, instead of a meta-analysis of LD regimens, members of the T2EVOLVE work package 6 presents here a comprehensive literature review.
Currently used substances and lymphodepleting regimens
Table 1 shows the great variety of currently applied LD/conditioning regimens across clinical trials. These treatments differ in substances and their combinations, dosing and timing (even within LD regimens with identical doses). The regimens also differ between autologous and allogeneic CAR-T therapies. Depending on the indication (lymphomas, leukemias or solid tumors), various approaches have been proposed. The following substances or combinations thereof are the main stay of LD:
Fludarabine
Fludarabine (Flu) is a purine analogue, which has been a vital treatment option for Chronic Lymphocytic Leukemia (CLL) and indolent NHL for many years (40), is mainly used in combination with other lymphodepleting agents. Activity and toxicities are well studied in CLL and conditioning for allogeneic stem cell transplantation (41). Fludarabine seems to be particularly toxic for hemopoietic precursors – a fact that may account for some early toxicity after infusion (42, 43). The metabolism of the drug is dependent on glucuronidation by the UGT2B17-pathway, resulting in dependency on individual, sex- and ethnicity-related factors (44).
Cyclophosphamide
Cyclophosphamide (Cy) is an alkylating agent with efficacy in a wide range of lymphomas, Hodgkin´s lymphoma, aggressive as well as indolent NHLs (45–47). High-dosage Cy is used as a single lymphodepleting agent followed by CAR-T transfusion or in autologous stem cell transplantation. Dosages in the CAR-T setting up to 1800 mg/m2 for two days have been studied for efficacy (33).
Fludarabine and cyclophosphamide
Flu and Cy are used in combination with immunotherapy for CLL (48) and are currently the most commonly used lymphodepleting agents used for CAR-T cell therapy. Most LD regimens are based on the doses used for CLL therapy (49). Commercially available CAR –T products rely mostly on Flu + Cy for conditioning, except for tisa-cel (11) (Table 1). However, variations in dosages of Flu/Cy (25/250 mg/m2 up to 30/750 mg/m2) application duration (3 days up to 5 days of Flu and 1 up to 3 days of Cy respectively) and time until transfusion (1 day up to 14 days prior to transfusion) of CAR-T are common.
Bendamustine
Another alkylating agent is Bendamustine (Benda), used as a first- and second-line treatment option for CLL and other B- and T-cell lymphomas, either as single agent or in combination with targeted therapies (50–52).
While being included as an alternative to Flu/Cy in the JULIET trial (11) and showing fewer toxicities than Flu/Cy with similar outcomes (53), it is not included as an option for other products and only occasionally used in clinical trials regarding novel CAR-T. B seems to be a good option for patients with renal insufficiency (53).When used in clinical trials the dose ranges from 70 mg/m2 to 90 mg/m2 per day, for two consecutive days, as a single agent.
Radiation
Radiation therapy (RT) is nowadays used in the treatment of localized lymphomas (54–56), as well as for transplant conditioning. Radiation of tumor masses shows benefits in animal studies as a pre-conditioning before CAR-T administration enabling them to mitigate antigen escape (57). In preclinical mouse models, total body irradiation has been used for a long time to induce lymphodepletion. In addition, low dose local radiation in combination with CAR-T is currently a promising field especially in the context of solid tumor therapies.
Targeted lymphodepletion
Alemtuzumab
As an alternative, targeting antigens on T-cells (and malignant cells) specifically has been explored. Alemtuzumab, alone or in combination with Flu/Cy is being used as conditioning agent for allogeneic CAR-T, particularly in lymphoproliferative diseases, where it seems to improve the clinical response rate over Flu/Cy (58). Higher toxicities should, however, be expected (58, 59).
Moreover, alemtuzumab due to its long half-life and pan-T activity can only be applied when CD52 has been knocked out in CAR-T.
Most of the regimens target both B as well as T-cells, making them particularly attractive to combine LD as well as tumor reduction in lymphomas. On the other hand, metabolism, route of elimination and half-life are different and may even vary between ethnicities. This may account for varying toxicities and efficacy (60). Serum concentrations as well as population genetic differences have been linked to these effects (39, 61, 62).
Differences in the total dose or effective serum levels of Flu/Cy may even account for outcome. It is interesting to note that tisa-cel which uses a lower dose of Flu/Cy has shown lower long-term progression-free survival and is the only agent failing in second-line trials (11, 13, 23, 63).
Variations in the interval between LD and CAR-T delivery may also be responsible for differences in toxicities and could even affect the growth and cellular kinetics of CAR-T, since very close timing to infusion may be associated with considerable serum concentrations of fludarabine after day+1 in special populations (39, 61, 62). Moreover, T cell subsets show different sensitivity to Flu, especially CD8+ effector T cells seem to be more sensitive (64). Therefore, exploring options targeting T cells or subsets thereof seems warranted.
Oxaliplatin/cyclophosphamide
This combination has been reported to enhance recruitment of CAR-T to solid tumors and to increase efficacy when combined with checkpoint blockade (65, 66).
Clofarabine
Clofarabine is well known for its activity as an anti-leukemic agent. It has successfully been used for LD together with cyclophosphamide before tisa-cel CD19+ CAR-T cell infusion leading to clinical remission (67, 68) Another potential indication is its use as conditioning for CAR-T reinfusion as in the case of ARI-0001 (22). Flu/Cy plus rituximab has been used for conditioning before a second CAR-T infusion (69).
Emerging strategies to avoid the need for LD
Several studies have demonstrated the potential of alternative strategies to enhance the in vivo activity of immune effectors without the need for lymphodepletion in CAR-T cell therapy. These strategies aim to overcome the limitations of lymphodepletion.
Such approaches seek to enhance CAR-T cell persistence and expansion by incorporating additional gene engineering besides the transfer of the CAR gene construct. This can be achieved by engineering CAR-T cells to also express certain cytokines that can help promote their survival and proliferation. These are considered “4th generation” CAR-T cells, or TRUCKs (“T cells redirected for antigen-unrestricted cytokine-initiated killing”) (70), an approach with a particular interest in solid tumors. Currently there are several TRUCK designs being tested in preclinical and early phase trials testing the incorporation of a wide variety of single or combinatory cytokines with autocrine and paracrine functions including IL-7, IL-12, IL-15, IL-18, and IL-23, among others.
Other complementary gene engineering candidates that could potentially allow for enhanced CAR-T cell persistence and expansion can also include the intervention of immune-checkpoints like PD-1 and CTLA-4. This could be achieved by either the incorporation of the capacity to secrete PD-1 and CTLA-4 blocking antibodies directed upon antigen-driven CAR signaling, or by directly disrupting PD-1 gene (71), an approach that potentially could prevent T-cell exhaustion and/or suppression.
Nevertheless, while these approaches may seem promising, they are still in very early stages of development, and all currently commercially available CAR-T cell products strongly rely on preconditioning, highlighting the need to identify optimal LD regimens to improve the safety and efficacy of adoptive cell therapy with engineered T-cells.
Evidence and rationale for dosing in humans
Lymphodepleting chemotherapy has a 3-decade long history of co-development with adoptive cell transfer. Right from the start, LD has proven to be of upmost importance as it drives a synergistic effect that outperforms the expected effect of what both, chemotherapeutic agents and immune-effector cells would achieve by themselves. In fact, initial reports in murine models showed how the prior administration of cyclophosphamide facilitated the elimination of an established tumor by eliminating tumour-induced suppressor T-cells (72). By doing this, the administration of cyclophosphamide unveiled the importance of reducing immunosuppression to boost T-cell mediated immunity. Thereby it was shown that cyclophosphamide administration could also unleash an antitumoral effect that was not directly related to LD itself.
While non-myeloablative conditioning prior to ACT significantly improves the efficacy of tumor-reactive T cells in pre-clinical models, complete ablation with higher conditioning doses can intensify these effects. In a non-myeloablated host the regulatory compartment is significantly reduced with increased cytokine availability of homeostatic cytokines like IL-7 and IL-15, which positively impacts the anti-tumor efficacy of adoptively transferred T cells. An intensified lymphodepletion up to the point at which HSC reconstitution is required, further promotes to the beneficial environment. Studies in the pmel-1 model for the treatment of established B16 melanoma showed that intensified myeloablative pre-conditioning up to 9 Gy in combination with HSC improves the efficacy by further reduction of homeostatic cytokine consuming host cells (73, 74). Nevertheless, the administration of higher conditioning intensities has to be balanced out with emerging toxicities (34).
The significance of conditioning intensity was further examined in the early stages of TIL therapy among patients with metastatic melanoma (75). Ninety-three participants with metastatic melanoma underwent TIL therapy following three distinct conditioning regimens in three non-randomized sequential studies. These regimens included a non-myeloablative chemotherapy-based approach with Cy/Flu (n=43) and two myeloablative regimens incorporating Cy/Flu along with either 2 or 12 Gy of TBI (both n=25). Based on previous studies in murine models indicating enhanced TIL efficacy with increased conditioning intensity reaching a myeloablative level, followed by autologous hematopoietic stem-cell rescue (34, 74), the clinical study (75) reported overall response rates of 48.8%, 52%, and 72% for non-myeloablative, 2-Gy myeloablative, and 12-Gy myeloablative conditioning, respectively. Additionally, complete response rates were 9.3%, 8%, and 16%, respectively. Despite the limitations of non-randomized sequential studies, a discernible positive impact on efficacy appears notable in patients conditioned with high-TBI myeloablative protocols.
Influence on the microenvironment
It was not long before the foundations of LD were laid, as it was discovered that most of the synergistic effects were not only mediated by the direct antitumoral effect itself, but rather on how LD modulates the environment in which the immune system acts (76, 77). Soon, the identification of several mechanisms on how LD was able to modulate the activity of transferred immune-effector cells was initiated, including its modulation of homeostatic cytokines such as IL-7 and IL-15 by abrogation of cellular cytokine ‘sinks’, or other immune cells that compete with transferred immune-effector cells for the consumption of stimulatory cytokines. Also, the impairment of regulatory T-cells (Treg) that suppress tumor-reactive T-cells contributes to polarization of the environment. Finally, the induction of tumor apoptosis and necrosis can potentially lead to an increased cellular immunity, driven by an increased tumor-antigen presentation. These findings were obtained when connecting increased tumor-regression with the absence of host lymphocytes. In fact, a link between LD and augmented immune function is suggested to be mediated by `homeostatic proliferation` (78–85), a process that drives increased T-cell proliferation after small numbers of them are transferred into a lymphopenic host (see also chapter pre-clinical experimental evidence). Evidence for the impact of competition for homeostatic cytokines between transferred immune-effector cells and host cells (or cellular cytokine ‘sinks’) has been described (78, 80, 85, 86), as the proliferation of transferred adoptive T-cells in lymphopenic hosts can be reduced in a dose-dependent manner by increasing the total number of either adoptive antigen-specific T-cells or by co-transferring an ‘irrelevant’ population of T-cells. Furthermore, the eradication of ‘cellular sinks’ by LD and the resultant increase in the availability of homeostatic cytokines has been described as a central mechanism driving the activation of antigen-specific CD8+ T cells (77). Finally, naturally occurring Treg can potently induce immunotolerance to self and foreign antigens, as the physiological function of Treg is thought to be the avoidance of autoimmunity by ensuring tolerance to self-antigens. Therefore, a close link between autoimmunity and tumor immunity suggests that Treg may have a crucial role in shaping a tolerogenic TME (87).
Intestinal microbiota are also involved in modulating the activity of cellular therapies including CAR-T cells. In addition to the LD regimen itself, antibiotic treatment as prophylactic intervention does cause dysbiotic states. Experimental approaches have shown that the microbial composition and commensal-derived metabolites crucially impact on host immunity, thereby shaping either tolerogenic or activating environments (88, 89). Further, recent studies have shown that the microbiome is capable of influencing the functionality of CD19-specific CAR-T cells in patients. Those correlated the use of antibiotics before CAR-T cell administration with decreased commensal diversity and worse survival highlighting a beneficial role of a rich microbiome in cellular therapy (90, 91). In that regard, it was demonstrated that commensal metabolites such as short-chain fatty acids might improve the function of adoptively transferred T-cells for cancer treatment (92). Interestingly, LD can also contribute to anticancer reactivity by causing bacterial translocation to other tissues than the intestine itself such as the mesenteric lymph nodes. There, activation of antigen-presenting cells by TLR ligands can enhance the stimulation of adoptively transferred T-cells with antitumoral activity (93). Translocation of commensals to the tumor site has been described as well. By local secretion of L-lactate, Lactobacillus iners is able to confer chemoradiation resistance in mouse and patients (94). In contrast, the related strain Lactobacillus reuteri releases a tryptophan metabolite intratumorally, thereby improving checkpoint inhibition (95). Hence, both the translocation of the microbiome to other sites as well as the modulatory capacity of bacterial metabolites should be considered as potential factors altering immunotherapy response which are in turn changed by LD and antibiotic regimens.
Reduction and modulation of lymphocytes
Further clinical evidence of the relevance of LD has been previously described in early trials of tumor-infiltrating lymphocytes (TIL) on patients with metastatic melanoma, where a LD regimen consisting of 2 days of cyclophosphamide 60 mg/kg followed by 5 days of 25mg/m2 fludarabine could effectively reduce leukocyte counts to fewer than 20 cells/mm3, allowing for TIL administration in a lymphodepleted status (35). Furthermore, this transferred adoptive immune-effector cell expansion could be boosted by the administration of the cytokine IL-2 (96). Similar outcomes were observed in another early TIL trial for patients with metastatic melanoma, where 10 patients received TIL treatment in two occasions, the first one without LD, and the second one following 5 days of fludarabine 25mg/m2, allowing for intra-patient comparison (97). Investigators observed that prior administration of fludarabine led to a 2.9-fold increase in the in vivo persistence, as well as also led to an increase in plasma levels of the homeostatic cytokines IL-7 and IL-15.
The importance of LD was later evaluated with the first clinical reports of administration of CAR-T, where a small group of patients received treatment with a first generation (CD3z) CD20-specific autologous CAR-T in the absence of LD. In the trial reported by Till and colleagues (98) the first 3 patients had a very limited expansion and persistence of the transduced cells (1-3 weeks) that was later increased up to 9 weeks after the addition of IL-2 administration. Due to the modest therapeutic effects observed in this trial, the construct was modified through the addition of two costimulatory domains (CD28-4-1BB-CD3z) and LD with 2 days of cyclophosphamide 1000 mg/m2 was followed by IL-2 administration (99). Using these modifications, the persistence of the transduced cells was detectable for up to 12 months, and 2 out of 4 patients achieved a complete response (CR). Consequently, at the nadir of LD, the absolute Treg levels, absolute CD3+ counts, and B cell counts were reduced by up to 96%, 93%, and 85%, respectively. However, despite the rapid lymphocyte expansion observed during IL-2 therapy, a possible counterproductive effect was detected as increased Treg levels were also seen after IL-2 injections. Therefore, IL-2 administrations were abandoned. Similar outcomes were observed in another early CAR-T report (100), this time with a more sophisticated construct composed of an anti-CD19 (FMC63), CD28-CD3z autologous product administered to a patient with lymphoma. The patient received an LD regimen consisting of 2 days of cyclophosphamide 60 mg/kg followed by 5 days of fludarabine 25 mg/m2. B cell aplasia has been observed for more than 3 years and the patient achieved a dramatic remission that lasted 32 weeks.
LD with Flu/Cy leads to changes in cytokine profiles and lymphocyte composition of the host, particularly when applied with growth factors such as GM-CSF (101). CD4+ T regulatory cells, CD8+ T suppressor cells, and T memory cells (CD8+ T central memory cells; T effector memory RA+ cells) are increased. Long-term changes of the T-cell and cytokine profiles are also observed with bendamustine (102).These long-term immunomodulatory effects of LD probably facilitate the development of opportunistic infections (103).
Reduction of rejection
The importance of the achievement of lymphodepletion was suggested in another early CAR-T trial (104) that observed how anti-transgene rejection responses contributed to limited persistence and efficacy of a first generation, dual (CD20/CD19 specific) CAR-T in a small group of 4 patients with Relapsed/Refractory (R/R) DLBCL and Follicular Lymphoma (FL). CAR-T were infused either following autologous Hematopoietic Stem Cell Transplantation (HSCT) or before fludarabine administration. Detection of transduced cells was reduced to a week or less, and anti-transgene immune rejection responses were detected in half of the patients. No patients were infused while lymphocyte counts were below normal range. This unveiled the possible role of immune rejection of the transgene as a major driver in the engraftment of the transferred cells, which in turn raised the interest in the immunosuppressive (IS) power of LD: an intensified LD might also lead to increased immunosuppression, consequently improving engraftment by avoiding anti-CAR-T-specific response.
Consolidation of efficacy
After these initial observations, several groups started reporting encouraging response rates and engraftment of CAR-T in patients with R/R B-ALL. A trial treating 16 adult ALL patients with Cy 1.5 to 3.0 g/m2 as LD followed by an anti-CD19 CD28-CD3z was able to achieve an unprecedented complete response rate (CRR) of 78% (105). Similar outcomes were observed in the pediatric setting in another trial (106) in which 30 patients were treated with a wide variety of LD regimens (Flu/Cy, Cyclophosphamide, Vincristine, and Prednisone [C/VP] and Cyclophosphamide, Vincristine, Doxorubicin, Dexamethasone [CVAD]) followed by CTL019 (later known as tisa-cel). In this trial, the CRR was 90%, with a 6-month rate of EFS and CTL019 persistence of 67% and 68%, respectively. Interestingly, most of the patients received LD, with at least half of them receiving Flu/Cy. Finally, a third trial (107) for R/R ALL treated 14 additional patients with LD consisting of 3 days Flu 25 mg/m2 and 1 day of Cy 900 mg/m2 followed by an anti-CD19 CD28-based CAR-T, achieving an 85% Minimal Residual Disease (MRD)-negative CRR.
Clinical evidence for the impact of fludarabine
Following the experience on the use of fludarabine in the context of allogeneic stem cell transplantation, new studies were designed to formally evaluate its impact on the LD regimen of patients with R/R ALL (108). On this trial, patients received a defined CD4+:CD8+ CAR-T composition with prior LD, either with Cy 2–4 g/m2 alone, Cy 2–3 g/m2 plus etoposide 100 mg/m2/d for 3 days, or Cy 60 mg/kg plus Flu 25 mg/m2/d for 3 or 5 days. Interestingly, patients receiving a fludarabine-based LD had improved CAR-T expansion, persistence and consistently with the increased CAR-T expansion, an improved disease-free survival.
Comparable outcomes were also observed in the pediatric setting in a study with 45 patients with R/R ALL treated with a defined CD4+:CD8+ CAR-T composition (4). Patients received LD either with cyclophosphamide alone or in combination with fludarabine. Patients that received Flu/Cy-based LD had a higher expansion peak, with a longer duration of B-cell aplasia in comparison to the ones that received Cy-based LD without Flu (2.1 vs 6.4 months). Finally, MRD-negative CRR was 100% and 90% for patients receiving LD with and without fludarabine.
Similar outcomes were obtained for patients with B cell lymphomas (109). In this study, patients who received Flu/Cy LD had a markedly increased CAR-T expansion and persistence, as well as higher response rates in comparison to the ones that received only cyclophosphamide-based LD (CRR of 50% vs 8%). Flu/Cy-based LD also led to higher serum concentrations of IL-7 and IL-15 on the day of CAR-T infusion. Furthermore, patients treated with cyclophosphamide based LD (without Flu) had an increased anti-CAR-T immune response, as second CAR-T administrations led to a lack of engraftment or measurable efficacy, as well as a detection of cytotoxic T cell responses specific for the murine single-chain fragment variable encoded by the transgene in 5/5 patients analyzed. This contrasts with the fact that 3/4 patients that received a second CAR-T infusion after previous exposure to Flu/Cy LD at their first CAR-T treatment were able to achieve a second engraftment which translated into tumor regression. These observations point towards to the importance of fludarabine to reduce the immune response to the transgene, allowing for longer persistence, efficacy, and eventually, for repetitive dosing of CAR-T cells.
Comparable outcomes were observed in a new study that focused on factors associated with durable event-free survival (EFS) in B-ALL patients after treatment with anti-CD19 CAR-T therapy (7). A total of 53 patients treated with a 4-1BB-based second generation product after being lymphodepleted with a variety of LD schemes. In this study, 85% of patients achieved a MRD-negative CR, and the multivariate modeling showed that the performance of LD regimens that incorporated fludarabine was associated with better EFS with a hazard ratio of 0.34. Interestingly, all CD19 negative relapses were observed in patients that received a fludarabine-based LD, and all relapses observed in patients whose LD did not contain fludarabine were CD19 positive. All this, together with the fact that a higher CAR-T AUC was seen in the Ful-based LD patients, is consistent with the fact that the magnitude of CAR-T expansion and persistence (and the LD performed) might influence the relapse phenotype.
Similarly, another study performed in 34 patients with R/R Hodgkin lymphoma analyzed the safety and efficacy of an autologous CAR-T targeting CD30 (27). In this trial, 3 different LD regimens were administered: Cy 500 mg/m2/day plus Flu 30 mg/m2/day for 3 days, bendamustine 90mg/m2/day for 2 days alone, and Benda 70 mg/m2/day and Flu 30 mg/m2/day for 3 days. Surprisingly, CRR varied between LD regimens to a point of achieving a 0% CRR in patients undergoing LD with Benda alone, which increased to 47% and 73% in patients lymphodepleted with Flu/Cy and Benda/Flu, respectively. These findings suggest that fludarabine seems to remain a fundamental drug in CAR-T LD therapy, which does not appear to be limited to products targeting B-cell malignancies.
The issue of intensity – influence on CAR-T cell kinetics
Focusing on dose intensity, another study further analyzed the impact of increasing the intensity of Flu/Cy-based LD in patients with B-cell lymphomas (33). In this study, patients with R/R aggressive B-cell lymphomas were treated with an anti-CD19 CAR-T product following 5 different LD categorized into ‘high-intensity’ vs ‘low-intensity’ LD based on the dose of Cy administered. ‘High-intensity’ LD consisted on Cy 60 mg/kg with either 3 or 5 days of Flu 25 mg/m2/day. On the other hand, ‘Low-intensity’ LD consisted on Cy 30 mg/kg or with 3 days Flu 25 mg/m2/day, 3 days of Cy 300 mg/m2/day with 3 days Flu 30 mg/m2/day, or 3 days of Cy 500 mg/m2/day with 3 days Flu 30 mg/m2/day (Table 1).
In this study, the best ORR was 51%, with 40% of patients achieving a CR and a 46% probability of estimated 2-year Progression Free Survival (PFS). However, patients that received ‘high-intensity’ LD achieved a more favorable cytokine profile, as defined by the levels of monocyte chemoattractant protein-1 (MCP-1) prior to infusion and peak interleukin-7 (IL-7). Those concentrations were above the median in patients that had a “high-intensity LD”, which in turn translated into higher CAR-T peak expansion, higher Cytokine Release Syndrome (CRS) intensity and severity, and a superior PFS (Figure 2B).
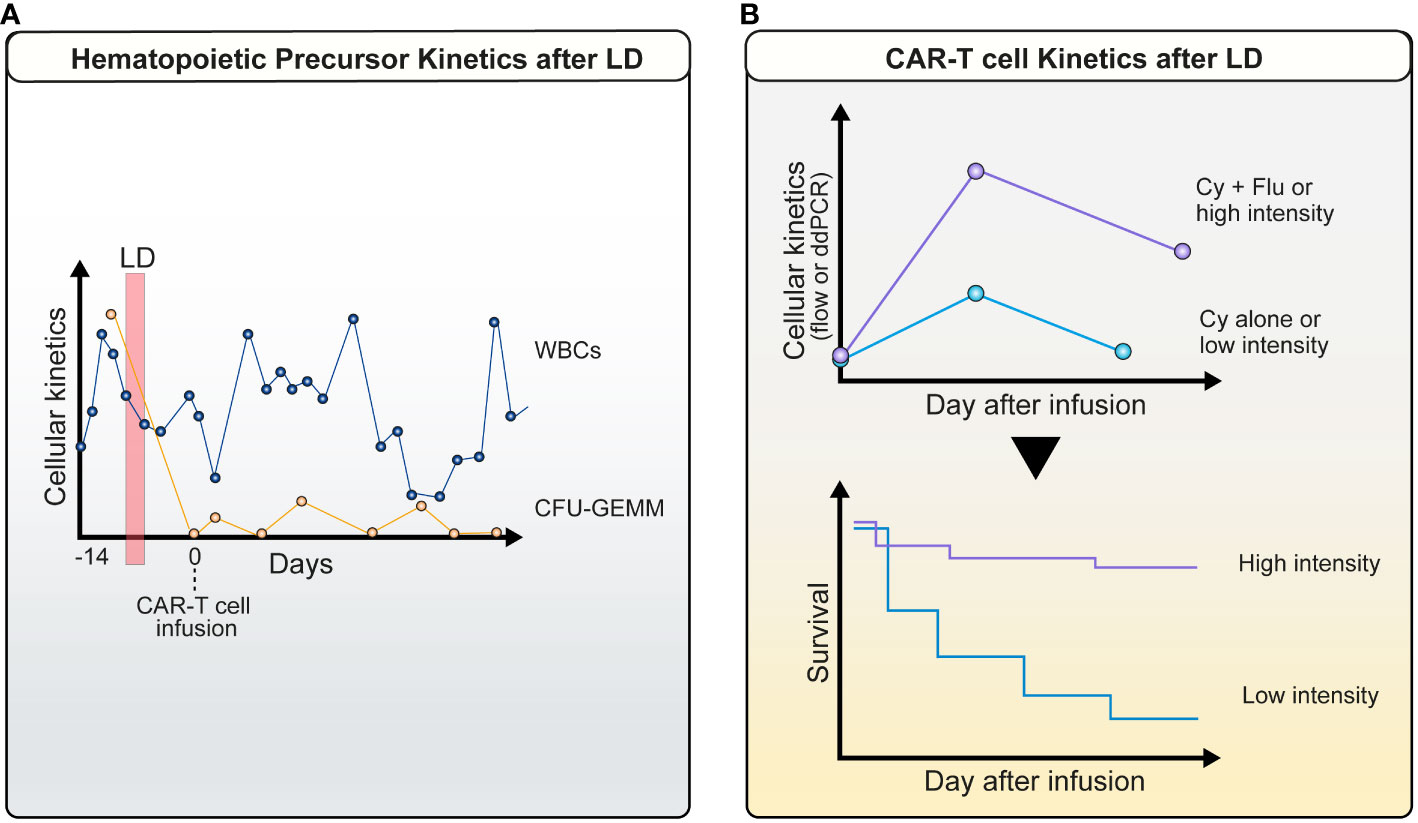
Figure 2 Cellular kinetics after lymphodepletion: (A) Profound decrease in host PB hematopoietic precursor cells. A marked drop in common myeloid progenitors CFU-GEMM (orange line) is observed between LD (red vertical bar) and CAR-T infusion in parallel with PB leukocyte counts (blue line) (patient example); (B) Kinetics of CAR-T cells is dependent on the intensity of LD. This is accompanied by an improved survival.
Nevertheless, an increase in intensity of LD might not always be associated with increased efficacy, as found in another study performed in R/R ALL (110)). 50 CAYA (children, adolescents, and young adult) patients were treated with a CD28-based anti-CD19 autologous CAR-T with the option of choosing the intensity of the LD regimen based on the disease burden observed prior infusion. This approach was designed to test the hypothesis that alternative LD regimens could reduce disease burden better prior to CAR-T infusion, with the objective of reducing CRS severity and potentially improve efficacy. ‘High-burden’ disease was defined by >25% bone marrow blasts, circulating peripheral blasts, or lymphomatous disease. Patients with ‘high-burden’ tended to receive an increased intensity LD either with (1) high-dose Flu (30 mg/m2 once a day x4 days) plus Cy (1,200 mg/m2 once a day x2 days), (2) FLAG, Flu (25 mg/m2 x 5 days) and cytarabine (2,000 mg/m2/day x5 days); and (3) ifosfamide (1,800 mg/m2 once a day x5 days) plus etoposide (100mg/m2 once a day x5 days), whereas patients with ‘low-burden’ disease received ‘standard-dose’ LD consisting of Flu (25 mg/m2 x 3 days) and Cy (900 mg/m2 x1 day). As for efficacy, MRD-negative CR was achieved in 56% of patients. CRR tended, however, to be significantly lower in patients that received increased intensity LD, with only 25% of patients achieving CR, which compares unfavorable with the 69% CRR observed in patients that received Flu/Cy-based LD. These outcomes suggest that increasing the intensity of LD might not be enough to overcome the bad prognosis associated with high-tumor burden.
All studies mentioned above indicate that the search for an optimal dose for LD for CAR-T rather reflected a ‘trial and error ‘strategy based on many drugs used in allogeneic stem cell transplantation and was not based on actual pharmacokinetic studies. Over the last decade, however, multiple studies showed a major impact of the individual pharmacokinetics for Anti-Thymocyte Globulin ATG (111), busulfan (112) but also fludarabine (61, 113) on the immune reconstitution of transplanted patient, leading to a proposal for optimal fludarabine dosages to be used (15-25 mg*h/l) to allow a well-balanced immune reconstitution with high efficacy and low toxicity. Therefore, prospective clinical trials are underway to test the impact of individual fludarabine dosing on immune reconstitution after allogenic stem cell transplantation (EudraCT Number: 2018-000356-18). Within this context, hypothesis-generating retrospective studies have been performed to assess the impact of fludarabine on CAR-T engraftment and clinical outcome, suggesting that intra-individual pharmacokinetics are an important factor to consider, even if patients receive at the first sight a similar dose. In the first study (114), CAYAs treated with commercial tisa-cel with prior on-label ‘conventional’ Flu/Cy LD had their cumulative total fludarabine exposure (area under the concentration-time curve [AUC]) measured. Blood samples were taken at time 0, + 1, +3, +7 and +11 hours after infusion of fludarabine on days 1 to 4 of LD. Concentrations of the circulating fludarabine metabolite were measured by a validated mass spectrometry method. Given the different needs of LD for allogeneic stem cell transplantation and CAR-T infusion, focus in this study was mainly on underexposure, as creating space for infused immune cells is the main goal. Patients were retrospectively categorized into ‘underexposure’ if the area under the AUC of fludarabine was lower than 14 mg*h/L. In the underexposed group, the median leukemia-free survival (LFS) was 1.8 months, which was lower compared with the 12.9 months observed in the group with an AUC of F ≥14 mg*h/L. Furthermore, the duration of B-cell aplasia within 6 months was shorter in the underexposed group (77.3% vs 37.3%). In addition, the cumulative incidence of CD19-positive relapse within 1 year following infusion was 100% in the underexposed group, which was significantly higher compared with 27.4% in the group with an AUC ≥14 mg*h/L. Finally, higher fludarabine exposure was associated with increased CAR-T expansion within the first 28 days after CAR T-cell infusion, with a mean peak CAR-T expansion in Peripheral Blood (PB) of 102 CAR-T cells/mL in the underexposed group vs the 295 CAR-T cells/mL in the group with an AUC ≥14 mg*h/L.
Comparable outcomes were observed by a second study that estimated the fludarabine exposure also as an AUC using a validated pharmacokinetic model in CAYAs treated with commercial tisa-cel with on-label, conventional’ Flu/Cy LD (62)). Fludarabine exposure was related to overall survival (OS), cumulative incidence of relapse (CIR), and a composite end point that included loss of B-cell aplasia and/or relapse. In this study, optimal fludarabine exposure was also similarly determined as an AUC ≥13.8 mg × h/L. In multivariable analyses, patients with AUC <13.8 mg × h/L had a 2.5-fold higher CIR and two-fold higher risk of relapse or loss of B-cell aplasia compared with those with optimal fludarabine exposure.
Similar outcomes were seen in patients with aggressive B-cell lymphomas treated with commercially available axicabtagene ciloleucel (115). In this study, a retrospective analysis of the impact of the fludarabine exposure on the safety and efficacy of axi-cel was measured in 199 adult patients. By estimating the fludarabine AUC using a validated pharmacokinetic model, three AUC categories were identified based on the results of P-splines curves: low (<18 mg*h/L), optimal (18-20 mg*h/L), and high (>20 mg*h/L). As previously described with tisa-cel in B-ALL, optimal fludarabine AUC was significantly associated with the most favorable PFS and OS. The 12-month PFS rate was 66% for the optimal AUC, which compared favorable to the 39% and 46% observed in the low and high groups, respectively. Also, the 12-month OS rate was 77% for the optimal AUC, which compared favorable to the 59% and 66% observed in the low and high groups, respectively. Given the different needs of LD for allogeneic stem cell transplantation and CAR-T infusion, focus in this study was mainly on underexposure, as creating space for infused immune cells is the main goal.
Animals/preclinical – experimental evidence
Murine model systems remain a powerful tool for the functional evaluation of adoptive cell therapy with immune-effector cells.
Xenograft models allow the transplantation of human tumor cells and primary human T cells to study the anti-tumor efficacy. To enable the successful engraftment of human cells, highly immunodeficient mice must be used as host. Especially mice with the NOD/shi-scid IL-2rγ(-/-) (NSG) background as well as the humanized version have proved to be reliable for the evaluation of efficacy and safety of CAR-T therapies. Due to the high immunodeficient state of the strains, these models are not, however, suited to investigate the complex interactions with other immune cell compartments influencing the outcome of the therapy in the context of lymphodepletion.
Therefore, a variety of syngeneic murine models has been developed to study the efficacy of adoptively transferred cells in the presence of the lymphoid compartment. Several decades ago, it was observed that the pre-conditioning with chemotherapeutic agents or total body irradiation has a significant impact on engraftment and therapy outcome (72, 116–118). This experimental evidence has been translated into the application of lymphopenia inducing conditioning as standard procedure to improve the engraftment and persistence of adoptively transferred cells in the clinic (35).
Typically, lymphodepletion in murine model systems is achieved by non-myeloablative total body irradiation (TBI). The irradiation dose to induce the desired pre-conditioning state before adoptive cell transfer can vary depending on the strain of mice and experimental requirements. Gattinoni et al. elucidated the effect of 5 Gy TBI in the context of T-cell Receptor (TCR) transgenic CD8+ T cells (pmel-1) targeting the self/tumor antigen gp100 in mice bearing established B16 melanoma. While the pre-conditioning with this intensity induced severe lymphopenia in treated mice, the anti-tumor efficacy was significantly increased compared to non-conditioned mice (77). Similar results were observed in pre-clinical settings with anti-murine CD19 CAR-T. Conditioning with 5-6 Gy provided optimal support for the key factors of efficacy like engraftment, proliferation and tumor eradication in mice challenged with 38c13 or A20 lymphoma and treated with CAR-T (119, 120). Therefore, the common irradiation doses in preclinical models for adoptive cell therapy, also applied in further tumor models, are usually based on a single-dose treatment between 4 to 6 Gray (121, 122).
Besides irradiation, clinically relevant chemotherapeutic agents such as cyclophosphamide and fludarabine as well as combination therapies are used in pre-clinical models to induce the conditioned state for adoptive transfer of T-cells. In a syngeneic C57Bl/6 mouse model of B-ALL Davila et al. applied escalating doses of cyclophosphamide and CAR-T doses (123). While the cyclophosphamide administration in moderate doses of 100 mg/kg improved the survival of treated mice and lead to a reduction in the B cell compartment, a dose escalation amplified the effect even further on the B cell level irrespective of the CAR-T cell dose administered. These results illustrate the central role of intensive pre-conditioning, rather than CAR-T dose escalation. This and other models apply typical cyclophosphamide conditioning from 50 mg/kg to 300 mg/kg in a single-dose treatment (123–126). Selected fludarabine doses are considerably lower ranging from 25 mg/kg to 100 mg/kg (77, 127, 128). Furthermore, not only individual therapy options are considered, Flu/Cy pre-treatments in pre-clinical models are also combined compared to the clinical regimens [16]. While a single-dose cyclophosphamide as well as Flu/Cy regimens induce strong leukopenia in mice, a single-dose fludarabine alone does not affect the leukocyte compartment (129).
While these studies demonstrate the importance of conditioning for engraftment and persistence of tumor reactive T cells in pre-clinical models, the effects of pre-conditioning on recipient mice that generate the most favorable milieu for cell transfer are complex and diverse.
In general, the combination of different mechanisms might be responsible for the advantage in engraftment and persistence of administered T cells in conditioned recipients rather than one mechanism alone.
Homeostatic expansion of T-cells has been one of the proposed mechanisms that drive anti-tumor immunity (86)(see also chapter influence of tumor microenvironment). The size of the immune compartment is precisely controlled through homeostatic regulation of the level and activation state of each individual cell type. Pre-conditioning induced lymphopenia triggers the expansion of T-cells by recognition of self-MHC/peptide ligands to restore the initial T-cell compartment size. In this process, naïve T-cells differentiate into activated/effector T-cells and gain effector functions characterized by interferon production. Surprisingly, in the pmel-1 transgenic mouse model challenged with B16 melanoma, the irradiation induced an increase in the functional capacity rather than the specific expansion of anti-tumor T-cells. While the compartment size of transferred T-cells was comparable between conditioned and non-conditioned mice, the interferon, tumor necrosis factor and IL-2 production were increased in irradiated mice (77). These results indicate a conditioning induced shift towards a reduced activation threshold of transferred T-cells.
In line with these findings, lymphodepletion has shown to reduce the levels of immune suppressive cells such as CD4+/CD25 +/FoxP3 + Treg and myeloid derived suppressor cells that contribute to immune tolerance by maintaining the activation threshold of effector T-cells (130). These cells maintain immunological tolerance to self/tumor antigens (131, 132) (see also chapter microenvironment).
In the pmel-1 mouse model, the adoptive transfer of CD4 T helper cells depleted by the regulatory CD25 type improved the anti-tumor response, while the transfer including the regulatory compartment significantly decreased anti-tumor efficacy indicating the critical role of lymphopenia inducing conditioning (133). Moreover, especially low dose cyclophosphamide is reported to selectively deplete Treg with a less suppressive capacity after recovery (126, 134, 135). Interestingly, it could be shown in a RAG-/- mice, which are naturally deficient in Treg cells, that non-myeloablative irradiation potentiates the efficacy of the treatment with tumor-reactive lymphocytes (77). Antibody mediated depletion of NK cells abolished this effect leading to similar tumor-control as in conditioned mice. Transferring the cells into tumor-bearing mice deficient in the homeostatic cytokines IL-7 or IL-15 impairs the treatment effect irrespective of conditioning. Based on these results, it was postulated, that lymphodepletion removes cellular compartments, that act as cytokine sinks and increases the cytokine availability of tumor-reactive transferred T cells (77).
While lymphodepletion with irradiation and Flu/Cy treatment significantly enhances adoptive cell therapy with tumor reactive lymphocytes in murine und human settings, the occurrence of life-threatening toxicities associated with pre-conditioning strategies demonstrates the need to overcome this hurdle.
Even though the transfer of in vitro activated and engineered T-cells in non-conditioned recipients remains challenging, engraftment of extremely low T-cell numbers, as low as a single naïve T-cell, can already be sufficient to develop into highly effective effector and memory T-cell subsets in non-lymphodepleted hosts (136). Several studies also investigated the potential of different phenotypic T-cell compositions on the potency to induce tumor destruction, a strategy that might also impact the dependence on the engraftment and persistence potential in lymphoreplete mouse models. Especially T-cell subsets of the stem cell memory (TSCM) and central memory (TCM) compartment possess superior functionality and persistence potential compared to more differentiated subsets (137–139). Therefore, ultra-short manufacturing protocols and the transfer of highly defined T cell subsets might be the strategy to preserve long-term persistence, while minimizing or preventing pre-conditioning requirements.
The co-engineering of T-cells to express tumor specific receptors in combination with interleukins is another promising strategy to overcome the conditioning obstacle. For example, a second generation anti-murine CD19 CAR construct co-expressing IL-12 was reported to successfully eliminate A20 lymphoma cells and to improve survival in lymphodepleted mice (125). Proposed mechanisms by which IL-12 co-expression leads to a functionality improvement even in the absence of pre-conditioning are the induction of proinflammatory cytokines, activation of NK and DC cells as well as an improvement in the cytotoxic effector function of T cells (140–142). Similar results were generated by the co-expression of the anti-murine CD19 CAR with IL-18 (143). As recombinant or transgenic cytokine delivery improves functionality, it comes at the cost of significant toxicities limiting clinical application. Therefore, the selective constitutive stimulation via engineered interleukin receptors like the IL-7 receptor or IL-2 incorporated in the CAR transgene as well as vaccination strategies provide a safer more promising approach for clinical translation (144–146). This co-engineering might also be a valuable approach to overcome CAR-T cell application in the solid tumor context moving more and more into the focus of the field (147). In summary murine model systems are essential in the toolbox for designing novel CAR-T products with reduced or even omitted LD requirements.
Impact of LD on CAR-T cellular kinetics in humans
Factors that impact toxicity and efficacy have been difficult to define because of differences in lymphodepletion regimens and heterogeneity of CAR-T administered to individuals. There have been many studies that investigated the effects of higher intensity vs. lower intensity of lymphodepletions on CD19 CAR-T expansion and persistence and clinical responses (Figure 2B). Patients, who received Flu/Cy lymphodepletion, had markedly increased CAR-T expansion and persistence (109). In addition, Curran et al., 2019 showed that higher dose intensity of conditioning chemotherapy and minimal pretreatment disease burden have a positive impact on response without a negative effect on toxicity (148). Higher intensity lymphodepletion clearly improves the cell expansion and persistence along with better efficacy rates; it is, however, usually associated with higher toxicity rates. Flu 25 mg/m2 x 2 + Cy 250 mg/m2 X 3 or Benda 90 mg/m2 x 2 (tisa-cel, DLBCL/FL) was also used in LBCL with tisa-cel as LD agent and results showed that Benda is as effective as Flu/Cy and validates a safer adverse event profile with reduced CRS, Immune Effector Cell-Associated Neurotoxicity Syndrome (ICANS), and hematological toxicities (53). These chemotherapeutic drugs can, however, increase the risk of infections and other side effects leading to the development of secondary malignancies.
Clinical effects
Impact on safety
It rapidly became clear that LD chemotherapy was not toxicity-free (149), as an early report describes how a patient with R/R CLL developed an unexpected toxic death (hypotension, dyspnea and renal failure) after adding LD with 1.5 g/m2 Cy before administering a 19-28z CAR-T. This was the first patient on this trial receiving CAR-T after LD, as the preceding 3 patients were safely treated without LD but with lack of CAR-T engraftment or efficacy. This experience made clear that LD might not only increase desired adoptive cell functions, but also their related toxicities. Later, further patients were treated in this trial (150).Almost no expansion and no clinical responses were seen among patients that received treatment without prior LD (0/3), in contrast to the 4/9 patients that did receive cyclophosphamide as LD that achieved better engraftment.
Almost at the same time, another case report (18)on a R/R CLL patient presented a patient treated with another second generation anti-CD19 (FMC63), 4-1BB-CD3z-based autologous CAR-T. The patient received prior LD with 600mg/m2 cyclophosphamide plus pentostatin 4mg/m2. These drugs were chosen due to their potential double effect (LD plus antitumor activity) as they already were being used to treat CLL (151). Surprisingly, the administration of what was considered a very low CAR-T dose (1.46×10e5 CAR-T cells/kg) led to an unprecedented MRD-negative response and deep B-cell aplasia. Similar outcomes were seen with 2 subsequent patients treated on the same trial that received LD with other agents (benda alone or with rituximab) (152).
Cytopenias and subsequent infections are one of the most important long-term side effects. Neutropenia and lymphopenia both contribute to the development of gram-negative or other opportunistic infections (153) Interestingly, a biphasic curve is observed for PB cell counts. The initial drop in white blood cells (within the first 2 weeks) is probably related to LD therapy (154). We have recently obtained evidence that not only mature myeloid or lymphatic cells are decreased during this phase, but that peripheral blood hematopoietic progenitors are also diminished (155) (Figure 2A). This indicates the marked toxicity of Flu/Cy - or benda-based regimens on all maturation grades of hematopoietic cells. Furthermore, it is unclear whether fludarabine levels remaining after CAR-T cell infusion (e.g. in patients with reduced kidney function) have detrimental effects on the number and function of CAR-T cells (61, 62). Recent evidence suggests that benda might be associated with fewer infections (53, 156).
While not being the main factor, LD is also likely to affect other known toxicities of CAR-T therapy including CRS and neurotoxicity. LD with Flu/Cy was identified as an independent variable of baseline characteristics together with high marrow tumor burden, higher CAR T-cell dose, thrombocytopenia before lymphodepletion, and manufacturing of CAR-T without selection of CD8+ central memory T cells as independent predictors of CRS (157).
The direct influence of LD on neurotoxicity is unclear, but parameters obtained at the start of LD predict severity of toxicity (158).
Due to the broad toxicity of Flu, Cy or benda, effects on other body systems such as the intestinal mucosa and the microbiome are also expected (159). This could facilitate the development of early gram-negative infections during neutropenia.
Impact on response to CAR-T cells
A direct influence of LD intensity on clinical response rates is well documented (see above). However, the strict connection between certain LD regimens and approved CAR-T products make a meta-analysis based on large datasets virtually impossible. However, it is interesting to note that in aggressive lymphoma the two products with higher Flu/Cy doses (axi-cel and liso-cel) have higher response rates and better long-term survival parameters then tisa-cel (63) Through their influence on cellular kinetics, LD regimens could also be involved in modulation of response.
Best current approaches
The best current approach may vary depending on indication and product. However, it seems that Flu/Cy at doses of 90mg/900-1500mg is a reasonable choice for most autologous settings. The data suggesting an optimal therapeutic window may lead to individualized dosing, particularly for fludarabine (115). Recent evidence suggests that bendamustin (180mg) is equally effective and possibly less toxic with both, tisa-cel and axi-cel in lymphoma and with cita-cel and ide-cel in multiple myeloma (53, 160, 161). Moreover, Benda is the preferred LD for patients with renal insufficiency or other comorbidities. For allogeneic CAR-T cells the evidence is less clear. Alemtuzumab based LD is a preferred option in the allogeneic setting, but has considerable toxicity.
Open questions and future developments
While LD is firmly established in the routine CAR-T cell treatment cycle, it seems clear that improvements in this area are an unmet need. We note the paucity of systematic investigations of agents, dosage and combinations (animal and human data). This includes the lack of comparability between trials and real-world evidence data due to variability and close connection of LD to (approved) therapies. Further investigation of individual metabolism including comorbidities and ethnic differences will help to personalize LD. Optimal LD in solid tumors or non-malignant diseases has yet to be established. Finally, recent studies exploring ultra-short manufacturing protocols or in vivo gene editing indicate that with the advent of less in vitro cell culture-dependent strategies and technologies, the dependency of CAR-T engraftment, expansion and survival on LD is strongly reduced and could in certain situations even be omitted (162, 163). Furthermore, the co-engineering strategies with receptors or the implementation of vaccination strategies to selectively boost the proliferation and expansion of adoptively transferred T-cells represents another exciting approach to overcome hurdles associated with LD (144–146).
Conclusions
Lymphodepletion is essential for the success of currently used CAR-T therapies. Detailed laboratory investigations on drugs, dosage and timing are, however, still needed for informed selection of regimens and novel drugs. Clinical data on the specific effects of LD on cellular kinetics, efficacy as well as toxicity should be obtained through real world experience as well as dose finding studies. The T2EVOLVE consortium and others are currently addressing many of these question in a concerted action to pave the way for standardized and optimized designs of LD regimens, aiming to improve patient care and therapy success in the future.
Author contributions
BL: Writing – original draft, Writing – review & editing. LC: Writing – original draft, Writing – review & editing. VO-M: Writing – original draft, Writing – review & editing. LW: Writing – original draft, Writing – review & editing. PB: Writing – review & editing. MD: Writing – review & editing. DH: Writing – review & editing, Writing – original draft. MH: Writing – review & editing. AK: Writing – review & editing. JM: Writing – review & editing. MN: Writing – review & editing. HN: Writing – review & editing. CS: Writing – review & editing, Writing – original draft. PS: Writing – review & editing. RT: Writing – review & editing. DB: Writing – review & editing. JD: Writing – review & editing. JK: Writing – original draft, Writing – review & editing. ML: Writing – original draft, Writing – review & editing. UJ: Writing – original draft, Writing – review & editing.
Funding
The author(s) declare financial support was received for the research, authorship, and/or publication of this article. Supported by the Innovative Medicines Initiative (IMI) T2EVOLVE. The project receives funding from the Innovative Medicines Initiative 2 Joint Undertaking under grant agreement No 945393. This Joint Undertaking receives support from the European Union ´s Horizon 2020 research and innovation program and EFPIA.
Conflict of interest
MH and ML: inventors on patents related to CAR T- cell therapy filed by the University of Würzburg. MH is listed as an inventor on patent applications and granted patents related to CAR-T technologies that have been filed by the Fred Hutchinson Cancer Research Center, Seattle, WA and by the University of Würzburg, Würzburg, Germany. MH is a co-founder and equity owner of T-CURX GmbH, Würzburg, Germany. MH received honoraria from Celgene/BMS, Janssen, Kite/Gilead. VO-M: Travel grants: Kite, Celgene-BMS, Novartis, Roche, Takeda & Janssen; Consultant or advisory fees: Kite, Celgene-BMS, Miltenyi Biomedicine, Pfizer, Novartis & Janssen; Honoraria: Kite, Celgene-BMS & Janssen; Employment: Hospital Clínic de Barcelona. UJ: consultant and advisory fees from Novartis, BMS, Gilead, Janssen, Roche, Honoraria from Miltenyi Biomedicine. DB: research projects with Juno/BMS and Repairon Immuno GmbH; consultant and advisory fees from BMS and Immatics. JD: Employment: Hospital Clínic de Barcelona. DH is an employee and shareholder of Bayer AG. HN and RT: are employees of Servier IRIS. PB: Advisory Board and consultancy from Allogene, Amgen, BMS/Celgene, Kite/Gilead, Incyte, Miltenyi Biomedicine, Novartis, Nektar, Pfizer and Pierre FabreKite/Gilead, Incyte, Miltenyi Biomedicine, Novartis, Nektar, Pfizer and Pierre Fabre. JK: Received grants form Novartis, Miltenyi Biotech and Gadeta. JK is inventor and receives revenues on multiple patents on CAR T and TEG engineering. LC was employed by Takeda. AK was employed by ITTM S.A.
The remaining authors declare that the research was conducted in the absence of any commercial or financial relationships that could be construed as a potential conflict of interest.
Publisher’s note
All claims expressed in this article are solely those of the authors and do not necessarily represent those of their affiliated organizations, or those of the publisher, the editors and the reviewers. Any product that may be evaluated in this article, or claim that may be made by its manufacturer, is not guaranteed or endorsed by the publisher.
References
1. Rosenberg SA, Spiess P, Lafreniere R. A new approach to the adoptive immunotherapy of cancer with tumor-infiltrating lymphocytes. Science (1986) 233(4770):1318–21. doi: 10.1126/science.3489291
2. Gross G, Waks T, Eshhar Z. Expression of immunoglobulin-T-cell receptor chimeric molecules as functional receptors with antibody-type specificity. Proc Natl Acad Sci U.S.A. (1989) 86(24):10024–8. doi: 10.1073/pnas.86.24.10024
3. Eshhar Z, Gross G. Chimeric T cell receptor which incorporates the anti-tumour specificity of a monoclonal antibody with the cytolytic activity of T cells: a model system for immunotherapeutical approach. Br J Cancer Suppl (1990) 10:27–9.
4. Gardner RA, Finney O, Annesley C, Brakke H, Summers C, Leger K, et al. Intent-to-treat leukemia remission by CD19 CAR T cells of defined formulation and dose in children and young adults. Blood (2017) 129(25):3322–31. doi: 10.1182/blood-2017-02-769208
5. Park JH, Riviere I, Gonen M, Wang X, Senechal B, Curran KJ, et al. Long-term follow-up of CD19 CAR therapy in acute lymphoblastic leukemia. N Engl J Med (2018) 378(5):449–59. doi: 10.1056/NEJMoa1709919
6. Maude SL, Laetsch TW, Buechner J, Rives S, Boyer M, Bittencourt H, et al. Tisagenlecleucel in children and young adults with B-cell lymphoblastic leukemia. N Engl J Med (2018) 378(5):439–48. doi: 10.1056/NEJMoa1709866
7. Hay KA, Gauthier J, Hirayama AV, Voutsinas JM, Wu Q, Li D, et al. Factors associated with durable EFS in adult B-cell ALL patients achieving MRD-negative CR after CD19 CAR T-cell therapy. Blood (2019) 133(15):1652–63. doi: 10.1182/blood-2018-11-883710
8. Frey NV, Shaw PA, Hexner EO, Pequignot E, Gill S, Luger SM, et al. Optimizing chimeric antigen receptor T-cell therapy for adults with acute lymphoblastic leukemia. J Clin Oncol (2020) 38(5):415–22. doi: 10.1200/JCO.19.01892
9. Shah BD, Ghobadi A, Oluwole OO, Logan AC, Boissel N, Cassaday RD, et al. KTE-X19 for relapsed or refractory adult B-cell acute lymphoblastic leukaemia: phase 2 results of the single-arm, open-label, multicentre ZUMA-3 study. Lancet (2021) 398(10299):491–502. doi: 10.1016/S0140-6736(21)01222-8
10. Roddie C, Dias J, O'Reilly MA, Abbasian M, Cadinanos-Garai A, Vispute K, et al. Durable responses and low toxicity after fast off-rate CD19 chimeric antigen receptor-T therapy in adults with relapsed or refractory B-cell acute lymphoblastic leukemia. J Clin Oncol (2021) 39(30):3352–63. doi: 10.1200/JCO.21.00917
11. Schuster SJ, Bishop MR, Tam CS, Waller EK, Borchmann P, McGuirk JP, et al. Tisagenlecleucel in adult relapsed or refractory diffuse large B-cell lymphoma. N Engl J Med (2019) 380(1):45–56. doi: 10.1056/NEJMoa1804980
12. Neelapu SS, Locke FL, Bartlett NL, Lekakis LJ, Miklos DB, Jacobson CA, et al. Axicabtagene ciloleucel CAR T-cell therapy in refractory large B-cell lymphoma. N Engl J Med (2017) 377(26):2531–44. doi: 10.1056/NEJMoa1707447
13. Abramson JS, Palomba ML, Gordon LI, Lunning MA, Wang M, Arnason J, et al. Lisocabtagene maraleucel for patients with relapsed or refractory large B-cell lymphomas (TRANSCEND NHL 001): a multicentre seamless design study. Lancet (2020) 396(10254):839–52. doi: 10.1016/S0140-6736(20)31366-0
14. Wang M, Munoz J, Goy A, Locke FL, Jacobson CA, Hill BT, et al. KTE-X19 CAR T-cell therapy in relapsed or refractory mantle-cell lymphoma. N Engl J Med (2020) 382(14):1331–42. doi: 10.1056/NEJMoa1914347
15. Jacobson CA, Chavez JC, Sehgal AR, William BM, Munoz J, Salles G, et al. Axicabtagene ciloleucel in relapsed or refractory indolent non-Hodgkin lymphoma (ZUMA-5): a single-arm, multicentre, phase 2 trial. Lancet Oncol (2022) 23(1):91–103. doi: 10.1016/S1470-2045(21)00591-X
16. Fowler NH, Dickinson M, Dreyling M, Martinez-Lopez J, Kolstad A, Butler J, et al. Tisagenlecleucel in adult relapsed or refractory follicular lymphoma: the phase 2 ELARA trial. Nat Med (2022) 28(2):325–32. doi: 10.1038/s41591-021-01622-0
17. Porter DL, Levine BL, Kalos M, Bagg A, June CH. Chimeric antigen receptor-modified T cells in chronic lymphoid leukemia. N Engl J Med (2011) 365(8):725–33. doi: 10.1056/NEJMoa1103849
18. Porter DL, Hwang WT, Frey NV, Lacey SF, Shaw PA, Loren AW, et al. Chimeric antigen receptor T cells persist and induce sustained remissions in relapsed refractory chronic lymphocytic leukemia. Sci Transl Med (2015) 7(303):303ra139. doi: 10.1126/scitranslmed.aac5415
19. Turtle CJ, Hay KA, Hanafi LA, Li D, Cherian S, Chen X, et al. Durable molecular remissions in chronic lymphocytic leukemia treated with CD19-specific chimeric antigen receptor-modified T cells after failure of ibrutinib. J Clin Oncol (2017) 35(26):3010–20. doi: 10.1200/JCO.2017.72.8519
20. Gill S, Vides V, Frey NV, Hexner EO, Metzger S, O'Brien M, et al. Anti-CD19 CAR T cells in combination with ibrutinib for the treatment of chronic lymphocytic leukemia. Blood Adv (2022) 6(21):5774–85. doi: 10.1182/bloodadvances.2022007317
21. Siddiqi T, Soumerai JD, Dorritie KA, Stephens DM, Riedell PA, Arnason J, et al. Phase 1 TRANSCEND CLL 004 study of lisocabtagene maraleucel in patients with relapsed/refractory CLL or SLL. Blood (2022) 139(12):1794–806. doi: 10.1182/blood.2021011895
22. Ortiz-Maldonado V, Rives S, Castella M, Alonso-Saladrigues A, Benitez-Ribas D, Caballero-Banos M, et al. CART19-BE-01: A multicenter trial of ARI-0001 cell therapy in patients with CD19(+) relapsed/refractory Malignancies. Mol Ther (2021) 29(2):636–44. doi: 10.1016/j.ymthe.2020.09.027
23. Locke FL, Ghobadi A, Jacobson CA, Miklos DB, Lekakis LJ, Oluwole OO, et al. Long-term safety and activity of axicabtagene ciloleucel in refractory large B-cell lymphoma (ZUMA-1): a single-arm, multicentre, phase 1-2 trial. Lancet Oncol (2019) 20(1):31–42. doi: 10.1016/S1470-2045(18)30864-7
24. Berdeja JG, Madduri D, Usmani SZ, Jakubowiak A, Agha M, Cohen AD, et al. Ciltacabtagene autoleucel, a B-cell maturation antigen-directed chimeric antigen receptor T-cell therapy in patients with relapsed or refractory multiple myeloma (CARTITUDE-1): a phase 1b/2 open-label study. Lancet (2021) 398(10297):314–24. doi: 10.1016/S0140-6736(21)00933-8
25. Munshi NC, Anderson LD Jr., Shah N, Madduri D, Berdeja J, Lonial S, et al. Idecabtagene vicleucel in relapsed and refractory multiple myeloma. N Engl J Med (2021) 384(8):705–16. doi: 10.1056/NEJMoa2024850
26. Oliver-Caldes A, Gonzalez-Calle V, Cabanas V, Espanol-Rego M, Rodriguez-Otero P, Reguera JL, et al. Fractionated initial infusion and booster dose of ARI0002h, a humanised, BCMA-directed CAR T-cell therapy, for patients with relapsed or refractory multiple myeloma (CARTBCMA-HCB-01): a single-arm, multicentre, academic pilot study. Lancet Oncol (2023) 24(8):913–24. doi: 10.1016/S1470-2045(23)00222-X
27. Ramos CA, Grover NS, Beaven AW, Lulla PD, Wu MF, Ivanova A, et al. Anti-CD30 CAR-T cell therapy in relapsed and refractory hodgkin lymphoma. J Clin Oncol (2020) 38(32):3794–804. doi: 10.1200/JCO.20.01342
28. Sadelain M, Riviere I, Riddell S. Therapeutic T cell engineering. Nature (2017) 545(7655):423–31. doi: 10.1038/nature22395
29. June CH, O'Connor RS, Kawalekar OU, Ghassemi S, Milone MC. CAR T cell immunotherapy for human cancer. Science (2018) 359(6382):1361–5. doi: 10.1126/science.aar6711
30. Cappell KM, Kochenderfer JN. Long-term outcomes following CAR T cell therapy: what we know so far. Nat Rev Clin Oncol (2023) 20(6):359–71. doi: 10.1038/s41571-023-00754-1
31. Daei Sorkhabi A, Mohamed Khosroshahi L, Sarkesh A, Mardi A, Aghebati-Maleki A, Aghebati-Maleki L, et al. The current landscape of CAR T-cell therapy for solid tumors: Mechanisms, research progress, challenges, and counterstrategies. Front Immunol (2023) 14:1113882. doi: 10.3389/fimmu.2023.1113882
32. Westin JR, Kersten MJ, Salles G, Abramson JS, Schuster SJ, Locke FL, et al. Efficacy and safety of CD19-directed CAR-T cell therapies in patients with relapsed/refractory aggressive B-cell lymphomas: Observations from the JULIET, ZUMA-1, and TRANSCEND trials. Am J Hematol (2021) 96(10):1295–312. doi: 10.1002/ajh.26301
33. Hirayama AV, Gauthier J, Hay KA, Voutsinas JM, Wu Q, Gooley T, et al. The response to lymphodepletion impacts PFS in patients with aggressive non-Hodgkin lymphoma treated with CD19 CAR T cells. Blood (2019) 133(17):1876–87. doi: 10.1182/blood-2018-11-887067
34. Muranski P, Boni A, Wrzesinski C, Citrin DE, Rosenberg SA, Childs R, et al. Increased intensity lymphodepletion and adoptive immunotherapy–how far can we go? Nat Clin Pract Oncol (2006) 3(12):668–81. doi: 10.1038/ncponc0666
35. Dudley ME, Wunderlich JR, Robbins PF, Yang JC, Hwu P, Schwartzentruber DJ, et al. Cancer regression and autoimmunity in patients after clonal repopulation with antitumor lymphocytes. Science (2002) 298(5594):850–4. doi: 10.1126/science.1076514
36. Gyurkocza B, Sandmaier BM. Conditioning regimens for hematopoietic cell transplantation: one size does not fit all. Blood (2014) 124(3):344–53. doi: 10.1182/blood-2014-02-514778
37. Neelapu SS. CAR-T efficacy: is conditioning the key? Blood (2019) 133(17):1799–800. doi: 10.1182/blood-2019-03-900928
38. Amini L, Silbert SK, Maude SL, Nastoupil LJ, Ramos CA, Brentjens RJ, et al. Preparing for CAR T cell therapy: patient selection, bridging therapies and lymphodepletion. Nat Rev Clin Oncol (2022) 19(5):342–55. doi: 10.1038/s41571-022-00607-3
39. Scordo M, Flynn JR, Devlin SM, Gonen M, Parascondola A, Alarcon Tomas A, et al. Population pharmacokinetic model identifies an optimal fludarabine exposure for improved outcomes after CD19-directed CAR T cell therapy for aggressive B-NHL: analysis from the cell therapy consortium. Blood (2022) 140(Supplement 1):1588–91. doi: 10.1182/blood-2022-164674
40. Adkins JC, Peters DH, Markham A. Fludarabine. An update of its pharmacology and use in the treatment of haematological Malignancies. Drugs (1997) 53(6):1005–37. doi: 10.2165/00003495-199753060-00007
41. Hallek M, Al-Sawaf O. Chronic lymphocytic leukemia: 2022 update on diagnostic and therapeutic procedures. Am J Hematol (2021) 96(12):1679–705. doi: 10.1002/ajh.26367
42. Janikova A, Koristek Z, Vinklarkova J, Pavlik T, Sticha M, Navratil M, et al. Efficacious but insidious: a retrospective analysis of fludarabine-induced myelotoxicity using long-term culture-initiating cells in 100 follicular lymphoma patients. Exp Hematol (2009) 37(11):1266–73. doi: 10.1016/j.exphem.2009.07.011
43. Richard Cl, Maguer-Satta Vr, Berger J, Jeanpierre S, Nicolini FE, Tournilhac O, et al. In vitro toxicity of fludarabine on normal bone marrow (BM) progenitors suggests its involvement in the impairment of hematopoietic progenitor (HP) mobilization. Blood (2007) 110(11):1192–. doi: 10.1182/blood.V110.11.1192.1192
44. Gruber M, Bellemare J, Hoermann G, Gleiss A, Porpaczy E, Bilban M, et al. Overexpression of uridine diphospho glucuronosyltransferase 2B17 in high-risk chronic lymphocytic leukemia. Blood (2013) 121(7):1175–83. doi: 10.1182/blood-2012-08-447359
45. Kolstad A, Nome O, Delabie J, Lauritzsen GF, Fossa A, Holte H. Standard CHOP-21 as first line therapy for elderly patients with Hodgkin's lymphoma. Leuk Lymphoma (2007) 48(3):570–6. doi: 10.1080/10428190601126610
46. Coiffier B, Lepage E, Briere J, Herbrecht R, Tilly H, Bouabdallah R, et al. CHOP chemotherapy plus rituximab compared with CHOP alone in elderly patients with diffuse large-B-cell lymphoma. N Engl J Med (2002) 346(4):235–42. doi: 10.1056/NEJMoa011795
47. Hiddemann W, Kneba M, Dreyling M, Schmitz N, Lengfelder E, Schmits R, et al. Frontline therapy with rituximab added to the combination of cyclophosphamide, doxorubicin, vincristine, and prednisone (CHOP) significantly improves the outcome for patients with advanced-stage follicular lymphoma compared with therapy with CHOP alone: results of a prospective randomized study of the German Low-Grade Lymphoma Study Group. Blood (2005) 106(12):3725–32. doi: 10.1182/blood-2005-01-0016
48. Hallek M, Eichhorst BF. Chemotherapy combination treatment regimens with fludarabine in chronic lymphocytic leukemia. Hematol J (2004) 5 Suppl 1:S20–30. doi: 10.1038/sj.thj.6200388
49. Cramer P, Isfort S, Bahlo J, Stilgenbauer S, Dohner H, Bergmann M, et al. Outcome of advanced chronic lymphocytic leukemia following different first-line and relapse therapies: a meta-analysis of five prospective trials by the German CLL Study Group (GCLLSG). Haematologica (2015) 100(11):1451–9. doi: 10.3324/haematol.2015.124693
50. Cheson BD, Brugger W, Damaj G, Dreyling M, Kahl B, Kimby E, et al. Optimal use of bendamustine in hematologic disorders: Treatment recommendations from an international consensus panel - an update. Leuk Lymphoma (2016) 57(4):766–82. doi: 10.3109/10428194.2015.1099647
51. Flinn IW, van der Jagt R, Kahl BS, Wood P, Hawkins TE, Macdonald D, et al. Randomized trial of bendamustine-rituximab or R-CHOP/R-CVP in first-line treatment of indolent NHL or MCL: the BRIGHT study. Blood (2014) 123(19):2944–52. doi: 10.1182/blood-2013-11-531327
52. Fischer K, Cramer P, Busch R, Stilgenbauer S, Bahlo J, Schweighofer CD, et al. Bendamustine combined with rituximab in patients with relapsed and/or refractory chronic lymphocytic leukemia: a multicenter phase II trial of the German Chronic Lymphocytic Leukemia Study Group. J Clin Oncol (2011) 29(26):3559–66. doi: 10.1200/JCO.2010.33.8061
53. Ghilardi G, Chong EA, Svoboda J, Wohlfarth P, Nasta SD, Williamson S, et al. Bendamustine is safe and effective for lymphodepletion before tisagenlecleucel in patients with refractory or relapsed large B-cell lymphomas. Ann Oncol (2022) 33(9):916–28. doi: 10.1016/j.annonc.2022.05.521
54. Specht L, Yahalom J, Illidge T, Berthelsen AK, Constine LS, Eich HT, et al. Modern radiation therapy for Hodgkin lymphoma: field and dose guidelines from the international lymphoma radiation oncology group (ILROG). Int J Radiat Oncol Biol Phys (2014) 89(4):854–62. doi: 10.1016/j.ijrobp.2013.05.005
55. Illidge T, Specht L, Yahalom J, Aleman B, Berthelsen AK, Constine L, et al. Modern radiation therapy for nodal non-Hodgkin lymphoma-target definition and dose guidelines from the International Lymphoma Radiation Oncology Group. Int J Radiat Oncol Biol Phys (2014) 89(1):49–58. doi: 10.1016/j.ijrobp.2014.01.006
56. Yahalom J, Illidge T, Specht L, Hoppe RT, Li YX, Tsang R, et al. Modern radiation therapy for extranodal lymphomas: field and dose guidelines from the International Lymphoma Radiation Oncology Group. Int J Radiat Oncol Biol Phys (2015) 92(1):11–31. doi: 10.1016/j.ijrobp.2015.01.009
57. DeSelm C, Palomba ML, Yahalom J, Hamieh M, Eyquem J, Rajasekhar VK, et al. Low-dose radiation conditioning enables CAR T cells to mitigate antigen escape. Mol Ther (2018) 26(11):2542–52. doi: 10.1016/j.ymthe.2018.09.008
58. Benjamin R, Jain N, Maus MV, Boissel N, Graham C, Jozwik A, et al. UCART19, a first-in-class allogeneic anti-CD19 chimeric antigen receptor T-cell therapy for adults with relapsed or refractory B-cell acute lymphoblastic leukaemia (CALM): a phase 1, dose-escalation trial. Lancet Haematol (2022) 9(11):e833–e43. doi: 10.1016/S2352-3026(22)00245-9
59. Mailankody S, Matous JV, Chhabra S, Liedtke M, Sidana S, Oluwole OO, et al. Allogeneic BCMA-targeting CAR T cells in relapsed/refractory multiple myeloma: phase 1 UNIVERSAL trial interim results. Nat Med (2023) 29(2):422–9. doi: 10.1038/s41591-022-02182-7
60. Wood AC, Perez AP, Arciola B, Patel K, Johnson G, DiMaggio E, et al. Outcomes of CD19-targeted chimeric antigen receptor T cell therapy for patients with reduced renal function including dialysis. Transplant Cell Ther (2022) 28(12):829 e1– e8. doi: 10.1016/j.jtct.2022.09.009
61. Langenhorst JB, Dorlo TPC, van Maarseveen EM, Nierkens S, Kuball J, Boelens JJ, et al. Population pharmacokinetics of fludarabine in children and adults during conditioning prior to allogeneic hematopoietic cell transplantation. Clin Pharmacokinet (2019) 58(5):627–37. doi: 10.1007/s40262-018-0715-9
62. Fabrizio VA, Boelens JJ, Mauguen A, Baggott C, Prabhu S, Egeler E, et al. Optimal fludarabine lymphodepletion is associated with improved outcomes after CAR T-cell therapy. Blood Adv (2022) 6(7):1961–8. doi: 10.1182/bloodadvances.2021006418
63. Bachy E, Le Gouill S, Di Blasi R, Sesques P, Manson G, Cartron G, et al. A real-world comparison of tisagenlecleucel and axicabtagene ciloleucel CAR T cells in relapsed or refractory diffuse large B cell lymphoma. Nat Med (2022) 28(10):2145–54. doi: 10.1038/s41591-022-01969-y
64. Woodahl EL, Wang J, Heimfeld S, Sandmaier BM, O'Donnell PV, Phillips B, et al. A novel phenotypic method to determine fludarabine triphosphate accumulation in T-lymphocytes from hematopoietic cell transplantation patients. Cancer Chemother Pharmacol (2009) 63(3):391–401. doi: 10.1007/s00280-008-0748-0
65. Davies DM, Maher J. Crosstown traffic: lymphodepleting chemotherapy drives CAR T cells. Cancer Cell (2021) 39(2):138–40. doi: 10.1016/j.ccell.2020.12.019
66. Srivastava S, Furlan SN, Jaeger-Ruckstuhl CA, Sarvothama M, Berger C, Smythe KS, et al. Immunogenic chemotherapy enhances recruitment of CAR-T cells to lung tumors and improves antitumor efficacy when combined with checkpoint blockade. Cancer Cell (2021) 39(2):193–208 e10. doi: 10.1016/j.ccell.2020.11.005
67. Foti A, Stein D, Seidel D, Levine J, Hijiya N, Williams O, et al. Clofarabine for lymphodepletion before CAR-T-cell infusion: A brief case report. J Pediatr Hematol Oncol (2023) 45(4):e551–e4. doi: 10.1097/MPH.0000000000002668
68. Ramiz S, Elhaj O, Siddiqui K, Khan S, AlSaedi H, AlAnazi A, et al. Clofarabine in pediatric acute relapsed or refractory leukemia: where do we stand on the bridge to hematopoietic stem cell transplantation? J Hematol (2023) 12(1):16–26. doi: 10.14740/jh1065
69. Ortiz-Maldonado V, Martinez-Cibrian N, Español-Rego M, Muñoz-Sanchez G, Navarro S, Alserawan L, et al. P1394: rituximab based lymphodepletion may increase engraftment and efficacy of second infusions of cart19 cells in B-cell acute lymphoblastic leukemia. Hemasphere (2023) 7(Suppl):e97357cb. doi: 10.1097/01.HS9.0000972464.97357.cb
70. Chmielewski M, Abken H. TRUCKS, the fourth-generation CAR T cells: Current developments and clinical translation. Expert Opin Biol Ther (2020) 3(3):e84. doi: 10.1002/acg2.84
71. Abdoli Shadbad M, Hemmat N, Khaze Shahgoli V, Derakhshani A, Baradaran F, Brunetti O, et al. A systematic review on PD-1 blockade and PD-1 gene-editing of CAR-T cells for glioma therapy: from deciphering to personalized medicine. Front Immunol (2021) 12:788211. doi: 10.3389/fimmu.2021.788211
72. North RJ. Cyclophosphamide-facilitated adoptive immunotherapy of an established tumor depends on elimination of tumor-induced suppressor T cells. J Exp Med (1982) 155(4):1063–74. doi: 10.1084/jem.155.4.1063
73. Wrzesinski C, Restifo NP. Less is more: lymphodepletion followed by hematopoietic stem cell transplant augments adoptive T-cell-based anti-tumor immunotherapy. Curr Opin Immunol (2005) 17(2):195–201. doi: 10.1016/j.coi.2005.02.002
74. Wrzesinski C, Paulos CM, Gattinoni L, Palmer DC, Kaiser A, Yu Z, et al. Hematopoietic stem cells promote the expansion and function of adoptively transferred antitumor CD8 T cells. J Clin Invest (2007) 117(2):492–501. doi: 10.1172/JCI30414
75. Dudley ME, Yang JC, Sherry R, Hughes MS, Royal R, Kammula U, et al. Adoptive cell therapy for patients with metastatic melanoma: evaluation of intensive myeloablative chemoradiation preparative regimens. J Clin Oncol (2008) 26(32):5233–9. doi: 10.1200/JCO.2008.16.5449
76. Klebanoff CA, Khong HT, Antony PA, Palmer DC, Restifo NP. Sinks, suppressors and antigen presenters: how lymphodepletion enhances T cell-mediated tumor immunotherapy. Trends Immunol (2005) 26(2):111–7. doi: 10.1016/j.it.2004.12.003
77. Gattinoni L, Finkelstein SE, Klebanoff CA, Antony PA, Palmer DC, Spiess PJ, et al. Removal of homeostatic cytokine sinks by lymphodepletion enhances the efficacy of adoptively transferred tumor-specific CD8+ T cells. J Exp Med (2005) 202(7):907–12. doi: 10.1084/jem.20050732
78. Wu Z, Bensinger SJ, Zhang J, Chen C, Yuan X, Huang X, et al. Homeostatic proliferation is a barrier to transplantation tolerance. Nat Med (2004) 10(1):87–92. doi: 10.1038/nm965
79. Murali-Krishna K, Ahmed R. Cutting edge: naive T cells masquerading as memory cells. J Immunol (2000) 165(4):1733–7. doi: 10.4049/jimmunol.165.4.1733
80. Ernst B, Lee DS, Chang JM, Sprent J, Surh CD. The peptide ligands mediating positive selection in the thymus control T cell survival and homeostatic proliferation in the periphery. Immunity (1999) 11(2):173–81. doi: 10.1016/S1074-7613(00)80092-8
81. Rocha B, von Boehmer H. Peripheral selection of the T cell repertoire. Science (1991) 251(4998):1225–8. doi: 10.1126/science.1900951
82. Bell EB, Sparshott SM, Drayson MT, Ford WL. The stable and permanent expansion of functional T lymphocytes in athymic nude rats after a single injection of mature T cells. J Immunol (1987) 139(5):1379–84. doi: 10.4049/jimmunol.139.5.1379
83. Oehen S, Brduscha-Riem K. Naive cytotoxic T lymphocytes spontaneously acquire effector function in lymphocytopenic recipients: A pitfall for T cell memory studies? Eur J Immunol (1999) 29(2):608–14. doi: 10.1002/(SICI)1521-4141(199902)29:02<608::AID-IMMU608>3.0.CO;2-A
84. Goldrath AW, Bogatzki LY, Bevan MJ. Naive T cells transiently acquire a memory-like phenotype during homeostasis-driven proliferation. J Exp Med (2000) 192(4):557–64. doi: 10.1084/jem.192.4.557
85. Cho BK, Rao VP, Ge Q, Eisen HN, Chen J. Homeostasis-stimulated proliferation drives naive T cells to differentiate directly into memory T cells. J Exp Med (2000) 192(4):549–56. doi: 10.1084/jem.192.4.549
86. Dummer W, Ernst B, LeRoy E, Lee D, Surh C. Autologous regulation of naive T cell homeostasis within the T cell compartment. J Immunol (2001) 166(4):2460–8. doi: 10.4049/jimmunol.166.4.2460
87. Shimizu J, Yamazaki S, Sakaguchi S. Induction of tumor immunity by removing CD25+CD4+ T cells: a common basis between tumor immunity and autoimmunity. J Immunol (1999) 163(10):5211–8. doi: 10.4049/jimmunol.163.10.5211
88. Luu M, Pautz S, Kohl V, Singh R, Romero R, Lucas S, et al. The short-chain fatty acid pentanoate suppresses autoimmunity by modulating the metabolic-epigenetic crosstalk in lymphocytes. Nat Commun (2019) 10(1):760. doi: 10.1038/s41467-019-08711-2
89. Romero R, Zarzycka A, Preussner M, Fischer F, Hain T, Herrmann JP, et al. Selected commensals educate the intestinal vascular and immune system for immunocompetence. Microbiome (2022) 10(1):158. doi: 10.1186/s40168-022-01353-5
90. Smith M, Dai A, Ghilardi G, Amelsberg KV, Devlin SM, Pajarillo R, et al. Author Correction: Gut microbiome correlates of response and toxicity following anti-CD19 CAR T cell therapy. Nat Med (2022) 29(11):2954. doi: 10.1038/s41591-022-02069-7
91. Stein-Thoeringer CK, Saini NY, Zamir E, Blumenberg V, Schubert ML, Mor U, et al. A non-antibiotic-disrupted gut microbiome is associated with clinical responses to CD19-CAR-T cell cancer immunotherapy. Nat Med (2023) 29(4):906–16. doi: 10.1038/s41591-023-02234-6
92. Luu M, Riester Z, Baldrich A, Reichardt N, Yuille S, Busetti A, et al. Microbial short-chain fatty acids modulate CD8(+) T cell responses and improve adoptive immunotherapy for cancer. Nat Commun (2021) 12(1):4077. doi: 10.1038/s41467-021-24331-1
93. Paulos CM, Wrzesinski C, Kaiser A, Hinrichs CS, Chieppa M, Cassard L, et al. Microbial translocation augments the function of adoptively transferred self/tumor-specific CD8+ T cells via TLR4 signaling. J Clin Invest (2007) 117(8):2197–204. doi: 10.1172/JCI32205
94. Colbert LE, El Alam MB, Wang R, Karpinets T, Lo D, Lynn EJ, et al. Tumor-resident Lactobacillus iners confer chemoradiation resistance through lactate-induced metabolic rewiring. Cancer Cell (2023) 41(11):1945–62 e11. doi: 10.1016/j.ccell.2023.09.012
95. Bender MJ, McPherson AC, Phelps CM, Pandey SP, Laughlin CR, Shapira JH, et al. Dietary tryptophan metabolite released by intratumoral Lactobacillus reuteri facilitates immune checkpoint inhibitor treatment. Cell (2023) 186(9):1846–62 e26. doi: 10.1016/j.cell.2023.03.011
96. Dudley ME, Wunderlich JR, Yang JC, Sherry RM, Topalian SL, Restifo NP, et al. Adoptive cell transfer therapy following non-myeloablative but lymphodepleting chemotherapy for the treatment of patients with refractory metastatic melanoma. J Clin Oncol (2005) 23(10):2346–57. doi: 10.1200/JCO.2005.00.240
97. Wallen H, Thompson JA, Reilly JZ, Rodmyre RM, Cao J, Yee C. Fludarabine modulates immune response and extends in vivo survival of adoptively transferred CD8 T cells in patients with metastatic melanoma. PloS One (2009) 4(3):e4749. doi: 10.1371/journal.pone.0004749
98. Till BG, Jensen MC, Wang J, Chen EY, Wood BL, Greisman HA, et al. Adoptive immunotherapy for indolent non-Hodgkin lymphoma and mantle cell lymphoma using genetically modified autologous CD20-specific T cells. Blood (2008) 112(6):2261–71. doi: 10.1182/blood-2007-12-128843
99. Till BG, Jensen MC, Wang J, Qian X, Gopal AK, Maloney DG, et al. CD20-specific adoptive immunotherapy for lymphoma using a chimeric antigen receptor with both CD28 and 4-1BB domains: pilot clinical trial results. Blood (2012) 119(17):3940–50. doi: 10.1182/blood-2011-10-387969
100. Kochenderfer JN, Wilson WH, Janik JE, Dudley ME, Stetler-Stevenson M, Feldman SA, et al. Eradication of B-lineage cells and regression of lymphoma in a patient treated with autologous T cells genetically engineered to recognize CD19. Blood (2010) 116(20):4099–102. doi: 10.1182/blood-2010-04-281931
101. Gunturu KS, Meehan KR, Mackenzie TA, Crocenzi TS, McDermott D, Usherwood EJ, et al. Cytokine working group study of lymphodepleting chemotherapy, interleukin-2, and granulocyte-macrophage colony-stimulating factor in patients with metastatic melanoma: clinical outcomes and peripheral-blood cell recovery. J Clin Oncol (2010) 28(7):1196–202. doi: 10.1200/JCO.2009.24.8153
102. Stokes J, Molina MS, Hoffman EA, Simpson RJ, Katsanis E. Immunomodulatory effects of bendamustine in hematopoietic cell transplantation. Cancers (Basel) (2021) 13(7):1702. doi: 10.3390/cancers13071702
103. Cona A, Tesoro D, Chiamenti M, Merlini E, Ferrari D, Marti A, et al. Disseminated cytomegalovirus disease after bendamustine: a case report and analysis of circulating B- and T-cell subsets. BMC Infect Dis (2019) 19(1):881. doi: 10.1186/s12879-019-4545-7
104. Jensen MC, Popplewell L, Cooper LJ, DiGiusto D, Kalos M, Ostberg JR, et al. Antitransgene rejection responses contribute to attenuated persistence of adoptively transferred CD20/CD19-specific chimeric antigen receptor redirected T cells in humans. Biol Blood Marrow Transplant (2010) 16(9):1245–56. doi: 10.1016/j.bbmt.2010.03.014
105. Davila ML, Riviere I, Wang X, Bartido S, Park J, Curran K, et al. Efficacy and toxicity management of 19-28z CAR T cell therapy in B cell acute lymphoblastic leukemia. Sci Transl Med (2014) 6(224):224ra25. doi: 10.1126/scitranslmed.3008226
106. Maude SL, Frey N, Shaw PA, Aplenc R, Barrett DM, Bunin NJ, et al. Chimeric antigen receptor T cells for sustained remissions in leukemia. N Engl J Med (2014) 371(16):1507–17. doi: 10.1056/NEJMoa1407222
107. Lee DW, Kochenderfer JN, Stetler-Stevenson M, Cui YK, Delbrook C, Feldman SA, et al. T cells expressing CD19 chimeric antigen receptors for acute lymphoblastic leukaemia in children and young adults: a phase 1 dose-escalation trial. Lancet (2015) 385(9967):517–28. doi: 10.1016/S0140-6736(14)61403-3
108. Turtle CJ, Hanafi LA, Berger C, Gooley TA, Cherian S, Hudecek M, et al. CD19 CAR-T cells of defined CD4+:CD8+ composition in adult B cell ALL patients. J Clin Invest (2016) 126(6):2123–38. doi: 10.1172/JCI85309
109. Turtle CJ, Hanafi LA, Berger C, Hudecek M, Pender B, Robinson E, et al. Immunotherapy of non-Hodgkin's lymphoma with a defined ratio of CD8+ and CD4+ CD19-specific chimeric antigen receptor-modified T cells. Sci Transl Med (2016) 8(355):355ra116. doi: 10.1126/scitranslmed.aaf8621
110. Shah NN, Lee DW, Yates B, Yuan CM, Shalabi H, Martin S, et al. Long-term follow-up of CD19-CAR T-cell therapy in children and young adults with B-ALL. J Clin Oncol (2021) 39(15):1650–9. doi: 10.1200/JCO.20.02262
111. Admiraal R, Nierkens S, de Witte MA, Petersen EJ, Fleurke GJ, Verrest L, et al. Association between anti-thymocyte globulin exposure and survival outcomes in adult unrelated haemopoietic cell transplantation: a multicentre, retrospective, pharmacodynamic cohort analysis. Lancet Haematol (2017) 4(4):e183–e91. doi: 10.1016/S2352-3026(17)30029-7
112. Langenhorst JB, Boss J, van Kesteren C, Lalmohamed A, Kuball J, Egberts ACG, et al. A semi-mechanistic model based on glutathione depletion to describe intra-individual reduction in busulfan clearance. Br J Clin Pharmacol (2020) 86(8):1499–509. doi: 10.1111/bcp.14256
113. Langenhorst JB, van Kesteren C, van Maarseveen EM, Dorlo TPC, Nierkens S, Lindemans CA, et al. Fludarabine exposure in the conditioning prior to allogeneic hematopoietic cell transplantation predicts outcomes. Blood Adv (2019) 3(14):2179–87. doi: 10.1182/bloodadvances.2018029421
114. Dekker L, Calkoen FG, Jiang Y, Blok H, Veldkamp SR, De Koning C, et al. Fludarabine exposure predicts outcome after CD19 CAR T-cell therapy in children and young adults with acute leukemia. Blood Adv (2022) 6(7):1969–76. doi: 10.1182/bloodadvances.2021006700
115. Scordo M, Flynn JR, Gonen M, Devlin SM, Parascondola A, Alarcon Tomas A, et al. Identifying an Optimal Fludarabine Exposure for Improved Outcomes after CD19 CAR T cell therapy for Aggressive B-NHL. Blood Adv (2023) 7(18):5579–85. doi: 10.1182/blood-2022-164674
116. Berenson JR, Einstein AB Jr., Fefer A. Syngeneic adoptive immunotherapy and chemoimmunotherapy of a Friend leukemia: requirement for T cells. J Immunol (1975) 115(1):234–8. doi: 10.4049/jimmunol.115.1.234
117. Cheever MA, Greenberg PD, Fefer A. Specificity of adoptive chemoimmunotherapy of established syngeneic tumors. J Immunol (1980) 125(2):711–4. doi: 10.4049/jimmunol.125.2.711
118. Wang LX, Shu S, Plautz GE. Host lymphodepletion augments T cell adoptive immunotherapy through enhanced intratumoral proliferation of effector cells. Cancer Res (2005) 65(20):9547–54. doi: 10.1158/0008-5472.CAN-05-1175
119. Cheadle EJ, Hawkins RE, Batha H, O'Neill AL, Dovedi SJ, Gilham DE. Natural expression of the CD19 antigen impacts the long-term engraftment but not antitumor activity of CD19-specific engineered T cells. J Immunol (2010) 184(4):1885–96. doi: 10.4049/jimmunol.0901440
120. Kochenderfer JN, Yu Z, Frasheri D, Restifo NP, Rosenberg SA. Adoptive transfer of syngeneic T cells transduced with a chimeric antigen receptor that recognizes murine CD19 can eradicate lymphoma and normal B cells. Blood (2010) 116(19):3875–86. doi: 10.1182/blood-2010-01-265041
121. Ward-Kavanagh LK, Zhu J, Cooper TK, Schell TD. Whole-body irradiation increases the magnitude and persistence of adoptively transferred T cells associated with tumor regression in a mouse model of prostate cancer. Cancer Immunol Res (2014) 2(8):777–88. doi: 10.1158/2326-6066.CIR-13-0164
122. Hanada KI, Yu Z, Chappell GR, Park AS, Restifo NP. An effective mouse model for adoptive cancer immunotherapy targeting neoantigens. JCI Insight (2019) 4(10):e124405. doi: 10.1172/jci.insight.124405
123. Davila ML, Kloss CC, Gunset G, Sadelain M. CD19 CAR-targeted T cells induce long-term remission and B Cell Aplasia in an immunocompetent mouse model of B cell acute lymphoblastic leukemia. PloS One (2013) 8(4):e61338. doi: 10.1371/journal.pone.0061338
124. Proietti E, Greco G, Garrone B, Baccarini S, Mauri C, Venditti M, et al. Importance of cyclophosphamide-induced bystander effect on T cells for a successful tumor eradication in response to adoptive immunotherapy in mice. J Clin Invest (1998) 101(2):429–41. doi: 10.1172/JCI1348
125. Kueberuwa G, Kalaitsidou M, Cheadle E, Hawkins RE, Gilham DE. CD19 CAR T cells expressing IL-12 eradicate lymphoma in fully lymphoreplete mice through induction of host immunity. Mol Ther Oncolytics (2018) 8:41–51. doi: 10.1016/j.omto.2017.12.003
126. Ueki T, Murata S, Kitamura N, Mekata E, Tani T. Pre-treatment with cyclophosphamide or OX40 (CD134) costimulation targeting regulatory T cell function enhances the anti-tumor immune effect of adoptively transferred CD8+ T cells from wild-type mice. Mol Med Rep (2009) 2(4):615–20. doi: 10.3892/mmr_00000146
127. Gassner FJ, Weiss L, Geisberger R, Hofbauer JP, Egle A, Hartmann TN, et al. Fludarabine modulates composition and function of the T cell pool in patients with chronic lymphocytic leukaemia. Cancer Immunol Immunother (2011) 60(1):75–85. doi: 10.1007/s00262-010-0920-3
128. Koike N, Pilon-Thomas S, Mule JJ. Nonmyeloablative chemotherapy followed by T-cell adoptive transfer and dendritic cell-based vaccination results in rejection of established melanoma. J Immunother (2008) 31(4):402–12. doi: 10.1097/CJI.0b013e31816cabbb
129. Johnson CB, May BR, Riesenberg BP, Suriano S, Mehrotra S, Garrett-Mayer E, et al. Enhanced lymphodepletion is insufficient to replace exogenous IL2 or IL15 therapy in augmenting the efficacy of adoptively transferred effector CD8(+) T cells. Cancer Res (2018) 78(11):3067–74. doi: 10.1158/0008-5472.CAN-17-2153
130. Antony PA, Paulos CM, Ahmadzadeh M, Akpinarli A, Palmer DC, Sato N, et al. Interleukin-2-dependent mechanisms of tolerance and immunity in vivo. J Immunol (2006) 176(9):5255–66. doi: 10.4049/jimmunol.176.9.5255
131. Curiel TJ, Coukos G, Zou L, Alvarez X, Cheng P, Mottram P, et al. Specific recruitment of regulatory T cells in ovarian carcinoma fosters immune privilege and predicts reduced survival. Nat Med (2004) 10(9):942–9. doi: 10.1038/nm1093
132. Woo EY, Yeh H, Chu CS, Schlienger K, Carroll RG, Riley JL, et al. Cutting edge: Regulatory T cells from lung cancer patients directly inhibit autologous T cell proliferation. J Immunol (2002) 168(9):4272–6. doi: 10.4049/jimmunol.168.9.4272
133. Antony PA, Piccirillo CA, Akpinarli A, Finkelstein SE, Speiss PJ, Surman DR, et al. CD8+ T cell immunity against a tumor/self-antigen is augmented by CD4+ T helper cells and hindered by naturally occurring T regulatory cells. J Immunol (2005) 174(5):2591–601. doi: 10.4049/jimmunol.174.5.2591
134. Lutsiak ME, Semnani RT, De Pascalis R, Kashmiri SV, Schlom J, Sabzevari H. Inhibition of CD4(+)25+ T regulatory cell function implicated in enhanced immune response by low-dose cyclophosphamide. Blood (2005) 105(7):2862–8. doi: 10.1182/blood-2004-06-2410
135. Ghiringhelli F, Larmonier N, Schmitt E, Parcellier A, Cathelin D, Garrido C, et al. CD4+CD25+ regulatory T cells suppress tumor immunity but are sensitive to cyclophosphamide which allows immunotherapy of established tumors to be curative. Eur J Immunol (2004) 34(2):336–44. doi: 10.1002/eji.200324181
136. Stemberger C, Huster KM, Koffler M, Anderl F, Schiemann M, Wagner H, et al. A single naive CD8+ T cell precursor can develop into diverse effector and memory subsets. Immunity (2007) 27(6):985–97. doi: 10.1016/j.immuni.2007.10.012
137. Sabatino M, Hu J, Sommariva M, Gautam S, Fellowes V, Hocker JD, et al. Generation of clinical-grade CD19-specific CAR-modified CD8+ memory stem cells for the treatment of human B-cell Malignancies. Blood (2016) 128(4):519–28. doi: 10.1182/blood-2015-11-683847
138. Fraietta JA, Lacey SF, Orlando EJ, Pruteanu-Malinici I, Gohil M, Lundh S, et al. Determinants of response and resistance to CD19 chimeric antigen receptor (CAR) T cell therapy of chronic lymphocytic leukemia. Nat Med (2018) 24(5):563–71. doi: 10.1038/s41591-018-0010-1
139. Sommermeyer D, Hudecek M, Kosasih PL, Gogishvili T, Maloney DG, Turtle CJ, et al. Chimeric antigen receptor-modified T cells derived from defined CD8+ and CD4+ subsets confer superior antitumor reactivity in vivo. Leukemia (2016) 30(2):492–500. doi: 10.1038/leu.2015.247
140. Tatsumi T, Takehara T, Kanto T, Miyagi T, Kuzushita N, Sugimoto Y, et al. Administration of interleukin-12 enhances the therapeutic efficacy of dendritic cell-based tumor vaccines in mouse hepatocellular carcinoma. Cancer Res (2001) 61(20):7563–7.
141. Liu J, Cao S, Kim S, Chung EY, Homma Y, Guan X, et al. Interleukin-12: an update on its immunological activities, signaling and regulation of gene expression. Curr Immunol Rev (2005) 1(2):119–37. doi: 10.2174/1573395054065115
142. Portielje JE, Lamers CH, Kruit WH, Sparreboom A, Bolhuis RL, Stoter G, et al. Repeated administrations of interleukin (IL)-12 are associated with persistently elevated plasma levels of IL-10 and declining IFN-gamma, tumor necrosis factor-alpha, IL-6, and IL-8 responses. Clin Cancer Res (2003) 9(1):76–83.
143. Avanzi MP, Yeku O, Li X, Wijewarnasuriya DP, van Leeuwen DG, Cheung K, et al. Engineered tumor-targeted T cells mediate enhanced anti-tumor efficacy both directly and through activation of the endogenous immune system. Cell Rep (2018) 23(7):2130–41. doi: 10.1016/j.celrep.2018.04.051
144. Aspuria PJ, Vivona S, Bauer M, Semana M, Ratti N, McCauley S, et al. An orthogonal IL-2 and IL-2Rbeta system drives persistence and activation of CAR T cells and clearance of bulky lymphoma. Sci Transl Med (2021) 13(625):eabg7565. doi: 10.1126/scitranslmed.abg7565
145. Shum T, Omer B, Tashiro H, Kruse RL, Wagner DL, Parikh K, et al. Constitutive signaling from an engineered IL7 receptor promotes durable tumor elimination by tumor-redirected T cells. Cancer Discovery (2017) 7(11):1238–47. doi: 10.1158/2159-8290.CD-17-0538
146. Reinhard K, Rengstl B, Oehm P, Michel K, Billmeier A, Hayduk N, et al. An RNA vaccine drives expansion and efficacy of claudin-CAR-T cells against solid tumors. Science (2020) 367(6476):446–53. doi: 10.1126/science.aay5967
147. Adachi K, Kano Y, Nagai T, Okuyama N, Sakoda Y, Tamada K. IL-7 and CCL19 expression in CAR-T cells improves immune cell infiltration and CAR-T cell survival in the tumor. Nat Biotechnol (2018) 36(4):346–51. doi: 10.1038/nbt.4086
148. Curran KJ, Margossian SP, Kernan NA, Silverman LB, Williams DA, Shukla N, et al. Toxicity and response after CD19-specific CAR T-cell therapy in pediatric/young adult relapsed/refractory B-ALL. Blood (2019) 134(26):2361–8. doi: 10.1182/blood.2019001641
149. Brentjens R, Yeh R, Bernal Y, Riviere I, Sadelain M. Treatment of chronic lymphocytic leukemia with genetically targeted autologous T cells: case report of an unforeseen adverse event in a phase I clinical trial. Mol Ther (2010) 18(4):666–8. doi: 10.1038/mt.2010.31
150. Brentjens RJ, Riviere I, Park JH, Davila ML, Wang X, Stefanski J, et al. Safety and persistence of adoptively transferred autologous CD19-targeted T cells in patients with relapsed or chemotherapy refractory B-cell leukemias. Blood (2011) 118(18):4817–28. doi: 10.1182/blood-2011-04-348540
151. Lamanna N, Kalaycio M, Maslak P, Jurcic JG, Heaney M, Brentjens R, et al. Pentostatin, cyclophosphamide, and rituximab is an active, well-tolerated regimen for patients with previously treated chronic lymphocytic leukemia. J Clin Oncol (2006) 24(10):1575–81. doi: 10.1200/JCO.2005.04.3836
152. Kalos M, Levine BL, Porter DL, Katz S, Grupp SA, Bagg A, et al. T cells with chimeric antigen receptors have potent antitumor effects and can establish memory in patients with advanced leukemia. Sci Transl Med (2011) 3(95):95ra73. doi: 10.1126/scitranslmed.3002842
153. Rejeski K, Subklewe M, Aljurf M, Bachy E, Balduzzi AC, Barba P, et al. Immune effector cell-associated hematotoxicity (ICAHT): EHA/EBMT consensus grading and best practice recommendations. Blood (2023) 142(10):865–77. doi: 10.1182/blood.2023020578
154. Rejeski K, Perez A, Sesques P, Hoster E, Berger C, Jentzsch L, et al. CAR-HEMATOTOX: a model for CAR T-cell-related hematologic toxicity in relapsed/refractory large B-cell lymphoma. Blood (2021) 138(24):2499–513. doi: 10.1182/blood.2020010543
155. Saxenhuber NAA. Das Verhalten Peripherer Hämatopoietischer Stammzellen von antiCD19+ CAR-T-Zelltherapie Patient:innen. The Behavior of Peripheral Hematopoietic Progenitor Cells in Patients receiving anti-CD19+ CAR-T-Cell Therapy. (Vienna: Medical Universit Vienna) (2022). p. 1, (66 Seiten), Diagramme.
156. Ghilardi G, Chong EA, Svoboda J, Wohlfarth P, Dwivedy Nasta S, Williamson S, et al. Bendamustine is a safe and effective regimen for lymphodepletion before tisagenlecleucel in patients with large B-cell lymphomas. Blood (2021) 138(Supplement 1):1438–. doi: 10.1182/blood-2021-151766
157. Hay KA, Hanafi LA, Li D, Gust J, Liles WC, Wurfel MM, et al. Kinetics and biomarkers of severe cytokine release syndrome after CD19 chimeric antigen receptor-modified T-cell therapy. Blood (2017) 130(21):2295–306. doi: 10.1182/blood-2017-06-793141
158. Pennisi M, Sanchez-Escamilla M, Flynn JR, Shouval R, Alarcon Tomas A, Silverberg ML, et al. Modified EASIX predicts severe cytokine release syndrome and neurotoxicity after chimeric antigen receptor T cells. Blood Adv (2021) 5(17):3397–406. doi: 10.1182/bloodadvances.2020003885
159. Blijlevens NM, Donnelly JP, de Pauw BE. Microbiologic consequences of new approaches to managing hematologic Malignancies. Rev Clin Exp Hematol (2005) 9(2):E2.
160. Ong SY, Pak S, Mei M, Wang Y, Popplewell L, Baird JH, et al. Bendamustine lymphodepletion is a well-tolerated alternative to fludarabine and cyclophosphamide lymphodepletion for axicabtagene ciloleucel therapy for aggressive B-cell lymphoma. Am J Hematol (2023) 98(11):1751–61. doi: 10.1002/ajh.27069
161. Sidana S, Hosoya H, Jensen A, Liu L, Goyal A, Hovanky V, et al. Bendamustine vs. fludarabine/cyclophosphamide lymphodepletion prior to BCMA CAR-T cell therapy in multiple myeloma. Blood Cancer J (2023) 13(1):158. doi: 10.1038/s41408-023-00929-0
162. Rurik JG, Tombacz I, Yadegari A, Mendez Fernandez PO, Shewale SV, Li L, et al. CAR T cells produced in vivo to treat cardiac injury. Science (2022) 375(6576):91–6. doi: 10.1126/science.abm0594
Keywords: CAR-T cells, lymphodepletion, conditioning, optimization, efficacy, toxicity
Citation: Lickefett B, Chu L, Ortiz-Maldonado V, Warmuth L, Barba P, Doglio M, Henderson D, Hudecek M, Kremer A, Markman J, Nauerth M, Negre H, Sanges C, Staber PB, Tanzi R, Delgado J, Busch DH, Kuball J, Luu M and Jäger U (2023) Lymphodepletion – an essential but undervalued part of the chimeric antigen receptor T-cell therapy cycle. Front. Immunol. 14:1303935. doi: 10.3389/fimmu.2023.1303935
Received: 28 September 2023; Accepted: 05 December 2023;
Published: 22 December 2023.
Edited by:
Uday Kishore, United Arab Emirates University, United Arab EmiratesReviewed by:
Pawel Muranski, Columbia University, United StatesSimon Völkl, University Hospital Erlangen, Germany
Usama Gergis, Thomas Jefferson University, United States
Copyright © 2023 Lickefett, Chu, Ortiz-Maldonado, Warmuth, Barba, Doglio, Henderson, Hudecek, Kremer, Markman, Nauerth, Negre, Sanges, Staber, Tanzi, Delgado, Busch, Kuball, Luu and Jäger. This is an open-access article distributed under the terms of the Creative Commons Attribution License (CC BY). The use, distribution or reproduction in other forums is permitted, provided the original author(s) and the copyright owner(s) are credited and that the original publication in this journal is cited, in accordance with accepted academic practice. No use, distribution or reproduction is permitted which does not comply with these terms.
*Correspondence: Ulrich Jäger, dWxyaWNoLmphZWdlckBtZWR1bml3aWVuLmFjLmF0
†These authors share first authorship
‡These authors share senior authorship