- 1Department of Medical Oncology, The Affiliated Cancer Hospital of Zhengzhou University, Henan Cancer Hospital, Zhengzhou, China
- 2Department of Gastrointestinal Medical Oncology, Tianjin Medical University Cancer Institute and Hospital, National Clinical Research Center for Cancer, Tianjin’s Clinical Research Center for Cancer, Tianjin Key Laboratory of Digestive Cancer, Key Laboratory of Cancer Prevention and Therapy, Tianjin, China
- 3Department of Medical Oncology, Zhengzhou People’s Hospital, Zhengzhou, China
Introduction: Identifying predictive biomarkers for immune checkpoint inhibitor (ICI) treatment is critical for gastric cancer (GC) prognosis. C-X-C motif chemokine ligand 13(CXCL13) plays an important role in immune regulation by binding exclusively to its receptor CXCR5. However, its role, underlying mechanisms, and prognostic significance in ICI-treated GC patients remain controversial.
Methods: This study investigated the clinical significance of CXCL13 and its potential immunomodulatory function in GC patients. A total of 144 GC patients from two cohorts, who received a combination of chemotherapy and anti-PD-1 antibody, were analyzed. The expression of CXCL13 was assessed using immunohistochemistry (IHC) and enzyme-linked immunosorbent assay. Associations between CXCL13, CXCR5, CD8, and CD4 were assessed by IHC and immunofluorescence. Survival analysis was performed using the Kaplan–Meier method and Cox proportional hazards model. The treatment response to CXCL13 and anti-PD-1 antibody was investigated using a subcutaneous xenograft tumor mouse model.
Results: The results suggested that patients with high CXCL13 expression had prolonged survival. High CXCL13 expression exhibited increased infiltration of CXCR5+CD8+ T cells and was associated with better outcomes. The combined assessment of CXCL13, CXCR5, and CD8+ T cells served as an independent predictor of prognosis. Additionally, CXCR5 and CD8+ T cells were enriched in tertiary lymphoid structures (TLSs), which conferred a prognostic benefit in the presence of high CXCL13 expression. CXCL13, in combination with anti-PD-1 therapy, retarded tumor growth in vivo, resulting in increased infiltration of CXCR5+CD8+ T cells.
Discussion: This study identified CXCL13 as a prognostic factor in GC patients receiving ICI therapy, emphasizing its critical role in the antitumor microenvironment via CXCR5+CD8+ T cells.
Introduction
Gastric cancer (GC) ranks fifth in terms of incidence and fourth in mortality among cancers worldwide (1). Approximately 70% of patients in China are diagnosed with advanced or metastatic GC at initial presentation (2). Immune checkpoint inhibitors (ICIs) have transformed the treatment paradigm for advanced GC. The combination of anti-PD-1 antibodies and chemotherapy improves the survival of patients with advanced GC (3–5). Extensive research has been conducted on molecular markers associated with ICIs. In GC, HER-2 positivity (6), PD-L1 CPS ≥ 1 (7), microsatellite instability-high/mismatch repair protein deficiency (8, 9), tumor mutational burden-high (10), and Epstein–Barr virus(EBV) positivity (11) are good prognostic factors for immunotherapy. Unfortunately, the overall response rate of GC patients to immunotherapy remains below 15% (12). Consequently, the identification of biomarkers that predict immunotherapeutic responsiveness is of urgent importance.
The tumor microenvironment (TME) plays a crucial role in the immunotherapy response, particularly in individualized precision treatment strategies (13, 14). In our previous study, we assessed intratumoral transcriptomic changes in the TME at the single-cell level in GC patients receiving neoadjuvant anti-PD-1 antibody combined with mFOLFOX6. The results indicated that C-X-C motif chemokine ligand 13 (CXCL13)+CD8+ T cells were specifically enriched in responder patients (15). CXCL13 is a member of the chemokine CXC subfamily. Chemokines can be classified into CC, CXC, CX3C, and C subfamilies. They are predominantly 8- to 12-kDa secreted proteins that regulate directed cell migration (chemotaxis), adhesion, cell positioning, and cell–cell interactions by binding to chemokine receptors (16). In the TME, chemokines regulate immune cell trafficking and exert both pro- and antitumorigenic functions (17–19).
CXCL13 exerts antitumor effects by binding exclusively to the C-X-C motif chemokine receptor 5 (CXCR5) (20, 21) and plays a critical role in immune responses. Accumulating evidence has demonstrated that the CXCL13–CXCR5 axis significantly influences immune cell infiltration in the TME. Subpopulations of CD8-CXCL13 and CD4-CXCL13 T cells were significantly increased in liver metastatic samples of colon cancer, which exhibited high proliferative ability and tumor-activating characteristics, contributing to a better prognosis for patients (22). In ovarian cancer (23), high CXCL13 expression is associated with prolonged survival by shaping the anti-TME by facilitating the maintenance of CXCR5+CD8+ T cells. Nevertheless, the effects of CXCL13–CXCR5 maintenance on the TME of GC and its prognostic significance in ICI treatment remain unclear.
In this study, we aimed to elucidate the prognostic significance of CXCL13 and its functional association with CXCR5-expressing immune cells in GC patients. High expression of CXCL13 was associated with prolonged survival, and CXCL13 exerted its antitumor activity by recruiting CXCR5+CD8+ T cells in GC patients. Therefore, CXCL13 may serve as a valuable prognostic indicator and a potential therapeutic target for ICI treatment of GC.
Materials and methods
Patients and specimen collection
This study was conducted on two independent cohorts of GC patients from Tianjin Medical University Cancer Institute and Hospital and The Affiliated Cancer Hospital of Zhengzhou University. Formalin-fixed paraffin-embedded (FFPE) tumor tissues collected prior to ICI treatment from cohort 1 were used for immunohistochemical (IHC) and immunofluorescence staining. Serum samples were obtained from peripheral blood pre- and post-treatment (8–9 weeks following the first treatment) of patients in cohort 2. The selected patients received anti-PD-1 antibody combined with chemotherapy as a neoadjuvant or first-line treatment between 2020 and 2023. None of the enrolled patients had an autoimmune disease or a history of cancer, and none had received immunotherapy, chemotherapy, radiation, or any other antitumor therapy prior to the initiation of neoadjuvant or first-line treatment. Progression-free survival (PFS) was calculated from the first dose of first-line treatment to disease relapse, progression, or death. Overall survival (OS) was calculated from the first dose of first-line treatment to all-cause death or the last follow-up. Treatment responses were evaluated using the Response Evaluation Criteria in Solid Tumors version 1.1 (RECIST 1.1) and the College of American Pathologists Tumor Regression Grading System. Informed consent was obtained from all patients.
IHC staining
FFPE specimens from pre-treatment tumor tissues in cohort 1 were prepared for IHC analysis. The following primary antibodies were used: CXCL13 (dilution 1:500; Abcam), CD4 (dilution 1:1200; Abcam, USA), CD8 (working solution; Zhongshan Jinqiao, China), CD20 (dilution 1:300; Invitrogen, USA), and CXCR5 (dilution 1:200; CST, USA). A complete list of antibodies is available in Supplementary Table S1. Normal lymph node tissue was used as a positive control, while an isotype antibody, instead of the primary antibody, served as the negative control. IHC results were independently evaluated by two pathologists who were blinded to the patient’s clinical data. CXCL13 and CXCR5 expression levels were scored by combining the proportion of positively stained cells with staining intensity. Staining intensity was graded on a scale of 0 to 3 (absent = 0, weak = 1, moderate = 2, and strong = 3), and the percentage of positive cells (range, 0%–100%) was determined using the Image J (NIH) algorithm. The average CXCL13 or CXCR5 H-score (range, 0–300) was calculated using the following formula: % positive cells × staining intensity, across five randomly selected high-power fields (HPFs) (×400). CD4 and CD8 expression levels were evaluated based on the number of positively stained tumor-infiltrating lymphocytes. Each sample was examined under high magnification (×400) across five randomly selected fields and the average count was recorded. Median protein expression levels were used as the cut-off values for defining high- and low-level expression. The cut-off values were 25 for CXCL13, 40 for CXCR5, 15 cells/HPF for CD8, and 22 cells/HPF for CD4. Tertiary lymphoid structures (TLSs) were identified as organized dense lymphocyte aggregates in hematoxylin and eosin (HE)-stained samples, with simultaneous positive IHC staining for CD20 within these regions.
Immunofluorescence staining
Immunofluorescence was performed on pre-treatment tumor samples from 22 patients in cohort 1. The slides were incubated with goat anti-human CXCR5, rabbit anti-human CD8, or rabbit anti-human CD4 antibodies. Horseradish peroxidase (HRP)-conjugated goat anti-rabbit IgG and AlexaFluor®488-conjugated goat anti-rabbit secondary antibodies were used. Endogenous peroxidase activity was blocked with 3% H2O2 at room temperature for 15 min. The slides were then incubated with a rabbit recombinant anti-CD8 or anti-CD4 alpha antibody overnight at 4°C, followed by incubation with an HRP-conjugated goat antirabbit IgG antibody at room temperature for 50 min in the dark. The slides were subsequently stained with the TSA-CY3 solution for 10 min in the dark. Thereafter, the slides were incubated with a second primary antibody (recombinant anti-CXCR5 alpha antibody) overnight at 4°C. The tissue was then covered with a secondary antibody (AlexaFluor®488-conjugated goat anti-rabbit IgG) and incubated at room temperature for 50 min in the dark. The nuclei were counterstained with DAPI, and fluorescence microscopy was used for detection and image acquisition.
Enzyme-linked immunosorbent assay
Enzyme-linked immunosorbent assay (ELISA) was performed to detect the expression of CXCL13, interferon-gamma (IFN-γ), tumor necrosis factor-alpha (TNF-α), Granzyme B (GZMB), interleukin (IL)-17A, and IL-2 using a commercially available ELISA kit (Liankebio, China). All assays were performed according to the manufacturer’s instructions. Cytokine concentrations were calculated based on standard curves generated using a specialized program for ELISA results evaluation.
Public database analysis
The Cancer Genome Atlas (TCGA) GC and GSE66229 transcriptome profiles were downloaded from TCGA Data Portal (http://tcga-data.nci.nih.gov/tcga/) and the NCBI public data platform, respectively. Comparisons between tumor and normal samples were performed using the Wilcoxon rank-sum test, and comparisons between different tumor stages were conducted using nonparametric Kruskal–Wallis tests. The Kaplan–Meier survival analysis was performed to compare the prognoses of the two datasets.
Animal experiments
Preparation of peripheral blood mononuclear cells
Fresh whole blood from healthy adults was collected using EDTA tubes. Peripheral blood mononuclear cells (PBMCs) were isolated using Ficoll lymphocyte gradient separation at 400×g for 30 min without acceleration. The second layer of the column was collected and washed with phosphate-buffered saline (PBS). After washing, the concentration of PBMCs was measured and adjusted to 1.0 × 107 cells/ml. PBMCs were stored in a liquid nitrogen tank until use.
PBMC injection process in M-NSG mice
The animal experiments complied with ethical standards and were approved by the Ethical Committee of Zhengzhou University(2023-YYY-044). Female M-NSG is the a mouse strain, and the full name of the mice of this strain is NOD.Cg-PrkdcscidIl2rgem1Smoc. Mice were obtained from Shanghai Model Organisms Center Inc. and were used at 4 weeks of age. Mice were maintained under specific pathogen-free (SPF) conditions according to the SPF guidelines (room temperature, 40%–60% humidity). Experiments were initiated after 1 week of adaptive feeding. PBMCs from healthy adults were removed from liquid nitrogen storage and immediately thawed in a 37°C water bath. The PBMCs were then washed once with Hank's Balanced Salt Solution (HBSS) (Gibco, USA) and resuspended by mixing 1.0 × 107 PBMCs with 200 μl of HBSS for intravenous injection into the tail vein of recipient M-NSG mice at 5 weeks of age (24, 25). CD45 expression in the peripheral blood was measured, and mice with over 25% CD45-positive cells were considered engrafted and humanized. Humanized M-NSG mice derived from different PBMC donors with varying engraftment levels were randomly assigned to each treatment group (Supplementary Figure S1A).
GC cells for tumor engraftment
The human GC cell line MKN-28 was purchased from ATCC (ATCC; Manassas, VA, USA) and cultured in standard RPMI 1640 medium supplemented with 10% fetal bovine serum and antibiotics (100 U/ml penicillin and 100 µg/ml streptomycin) under standard culture conditions (5% CO2, 37°C). The MKN-28 cell line demonstrated high CXCR5 expression, as confirmed by polymerase chain reaction (PCR) analysis (Supplementary Figure S1B). The PCR primer sequences used were 5′-TCAGTGGGCCCTATGTAGGAA-3′ (upper strand) and 5′-TGATGGCCTTGGCTGACTTT-3′-(lower strand). Hu-PBMC-NSG mice were subcutaneously injected with 1.0 × 106 MKN-28 cells under anesthesia at 6 weeks of age. The size of the subcutaneous xenograft tumors was measured every 3 days following injection. Tumor volume was calculated using the following formula: tumor volume = length × width × width/2.
Tumor experiments
Treatment was initiated when the tumors reached a volume of 50–100 mm3. Hu-PBMC-CDX mice were randomly divided into four groups (control, CXCL13, anti-PD-1 antibody, and CXCL13 plus anti-PD-1 antibody), with each group containing six mice. Mice were administered peritumoral injections of CXCL13 (1.25 µg per mouse) every other day until the end of the experiment (26). Anti-PD-1 antibody (camrelizumab, Jiangsu Hengrui, China) was administered intraperitoneally (200 µg per mouse) every 3 days for a total of six doses. Mice in the control group received intraperitoneal PBS injection (200 µg per mouse) every 3 days. Mice were killed 2 days after the final anti-PD-1 antibody dose. Subcutaneous tumors, spleens, and peripheral blood were harvested and processed into single-cell suspensions for flow cytometry analysis.
Flow cytometry
Single-cell suspensions were prepared from the subcutaneous tumors, spleens, and peripheral blood of Hu-PBMC-CDX-mice for flow cytometry. The cell suspensions were stained with a panel of fluorochrome-conjugated monoclonal antibodies (Supplementary Table S1). Staining was performed according to the manufacturer’s instructions (Beckman Coulter, USA). Flow cytometry was conducted using a FACS flow cytometer (Beckman Coulter), and data were analyzed using CytExpert (Beckman Coulter) and FlowJo software (Tree Star, USA).
Statistical analysis
Data were analyzed using GraphPad Prism 9.0 and SPSS software (version 26.0). Differences between the two groups were assessed using an independent t-test. Statistically significant differences among multiple groups were determined by one-way analysis of variance (ANOVA) or two-way ANOVA. Differences were considered statistically significant at the following levels: *p < 0.05; **p < 0.01; ***p < 0.001; and ****p < 0.0001. Survival analysis was performed using the Kaplan–Meier method with the log-rank test, along with univariate and multivariate Cox proportional hazards models. A Chi-square test was conducted to assess the correlation between molecular marker expression and clinical features. Statistical significance was set at a p-value < 0.05.
Results
High expression of CXCL13 is associated with a good clinical response to immunotherapy in GC
A total of 144 patients were enrolled across two cohorts, with 89 patients in cohort 1 (between 2020 and 2022) and 55 patients in cohort 2 (between April 2023 and December 2023). All enrolled patients received anti-PD-1 antibodies combined with chemotherapy as first-line or neoadjuvant treatment. The median age was 63 years (range: 28–82) in cohort 1 and 66 years (range: 32–78) in cohort 2. Among the patients in cohort 1, 69 (77.5%) received platinum-based chemotherapy, and seven (7.9%) received taxane-based chemotherapy. Among cohort 2 patients, 53 (96.4%) received platinum-based chemotherapy, and one (1.8%) received taxane-based chemotherapy. The baseline clinical characteristics of the two cohorts are presented in Table 1.
In cohort 1, patients were stratified into “high” and “low” expression groups based on the median IHC staining value for CXCL13 expression (Figure 1A). High CXCL13 expression was significantly correlated with longer PFS (median PFS: 20.0 months vs. 11.7 months, p = 0.042) and OS (median OS: 36.4 months vs. 18.7 months, p = 0.004) (Figure 1B). Furthermore, the evaluation of TGCA and GEO datasets confirmed that CXCL13 expression was higher in tumor tissues than in normal tissues. Upregulated CXCL13 expression was associated with prolonged survival in GC patients (Figure 1C).
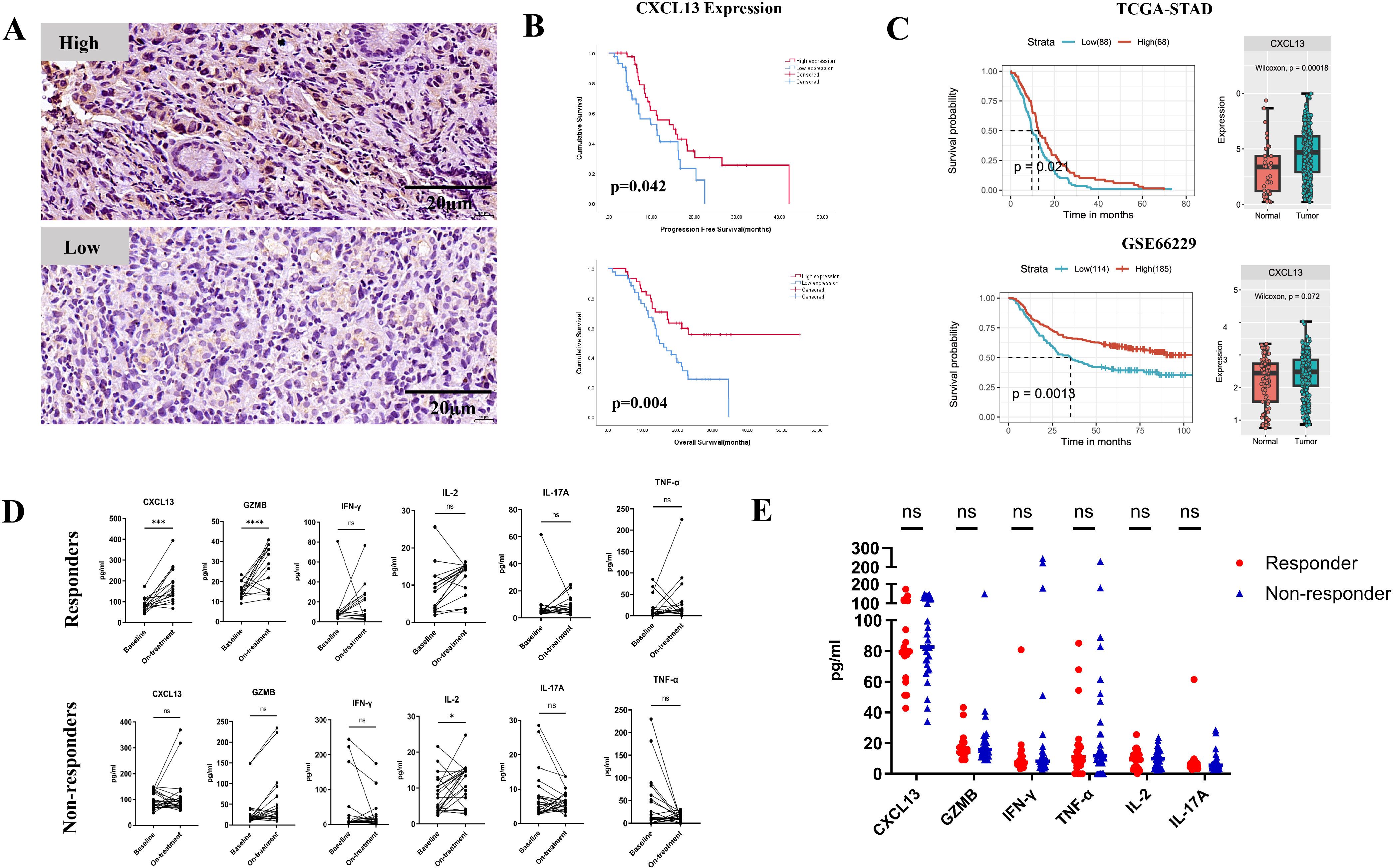
Figure 1. (A) Representative images showing high and low expression of CXCL13 by IHC staining. (B) Kaplan–Meier survival curves for PFS (top) and OS (bottom) in cohort 1 patients stratified by CXCL13 expression (n = 89; log-rank test, p-values shown). (C) Survival analysis of CXCL13 expression in TCGA and GEO datasets (left); expression of CXCL13 in tumor tissues vs. normal tissues (right). (D) Pre- and on-treatment (8–9 weeks from the first treatment) expression changes of CXCL13, GZMB, IFN-γ, IL-2, IL-17A, and TNF-α in serum samples between responders and non-responders in cohort 2 patients. (E) Baseline expression of CXCL13, GZMB, IFN-γ, IL-2, IL-17A, and TNF-α in responders and non-responders. (* p < 0.05; ***p < 0.001; **** p < 0.0001; ns, not significant).
In cohort 2, patients who achieved a complete response (CR), partial response (PR), or tumor regression grade (TRG) 0–1 were defined as the responder group (n = 21). Those with stable disease (SD), progressive disease (PD), or TRG 2–3 were defined as the non-responder group (n = 34). Among responders, CXCL13 expression was markedly elevated before treatment. Furthermore, an increase in the effector cytokine GZMB was observed in the responder group but not in the non-responders (Figure 1D). No statistically significant differences in baseline CXCL13 or effector cytokine expression levels were observed between responders and non-responders before treatment (Figure 1E). These results suggest that the upregulation of CXCL13 expression may enhance the efficacy and improve the survival outcomes of anti-PD-1 treatment in GC patients.
CXCL13 expression was significantly correlated with intratumoral infiltration of CXCR5+CD8+ T cells
CXCL13 is the exclusive ligand for CXCR5. Therefore, we hypothesized that CXCR5+ lymphocytes might participate in CXCL13-mediated immunotherapy responses. To investigate this, we first analyzed the correlation between CXCL13 expression and the presence of CXCR5+ immune cells. Simultaneous high expression of CXCR5 and CD8 was defined as the CXCR5HCD8H group, whereas simultaneous high expression of CXCR5 and CD4 was defined as the CXCR5HCD4H group. Low expression of both markers or high expression of only one marker was categorized as the “other expression” group. A strong correlation was observed between CXCL13 expression and both CXCR5HCD8H and CXCR5HCD4H expression. No significant associations were found between CXCR5HCD8H or CXCR5HCD4H and other clinical characteristics (Supplementary Table S2). Moreover, patients with CXCR5HCD8H expression demonstrated significantly better PFS (p = 0.027) and OS (p = 0.001) than those in the other expression group (Figure 2A). The same phenomenon was observed in the external databases. As single markers, neither CXCR5 nor CD8 expression was significantly associated with GC survival outcomes (Supplementary Figure S2). Patients with CXCR5HCD4H expression exhibited improved OS (p = 0.044) (Supplementary Figure S3A). Intriguingly, double-label immunofluorescence staining revealed that CXCR5-expressing cells colocalized with CD8 and CD4 in CR and PR patients (Figure 2B; Supplementary Figure S3B).
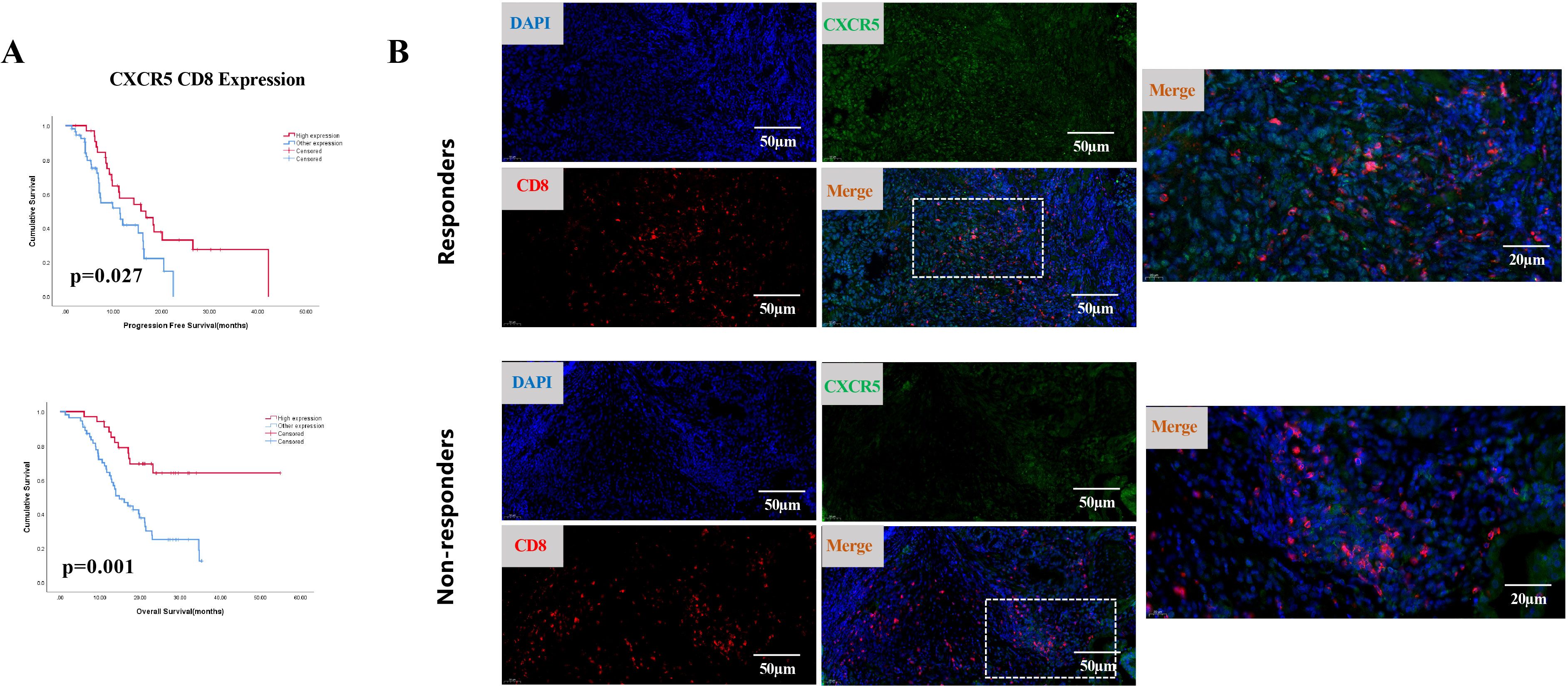
Figure 2. (A) Kaplan-Meier survival curves for PFS (top) and OS (bottom) in cohort 1, comparing patients with simultaneous high expression of CXCR5 and CD8 (CXCR5HCD8H) to other expression groups (n = 89; log-rank test, p-values shown). (B) Representative IF staining of responders and non-responders. Samples were stained for CXCR5 (green), CD8 (red), and DAPI (blue).
Next, we investigated the prognostic significance of the combined expression of CXCL13, CXCR5, and CD8 or CD4. The results indicated that concurrent high expression of CXCL13, CXCR5, and CD8 (CXCL13HCXCR5HCD8H) predicted superior survival outcomes (Figure 3A). Concurrent high expression of these three markers was an independent prognostic factor, correlating with better PFS (HR: 0.475, 95% CI: 0.254–0.888) and OS (HR: 0.358, 95% CI: 0.177–0.723) in cohort 1 patients (Figure 3B). Patients with concurrent high expression of CXCL13, CXCR5, and CD4 (CXCL13HCXCR5HCD4H) demonstrated prolonged OS (HR: 0.481, 95% CI: 0.231–1.001) (Figures 3C, D).
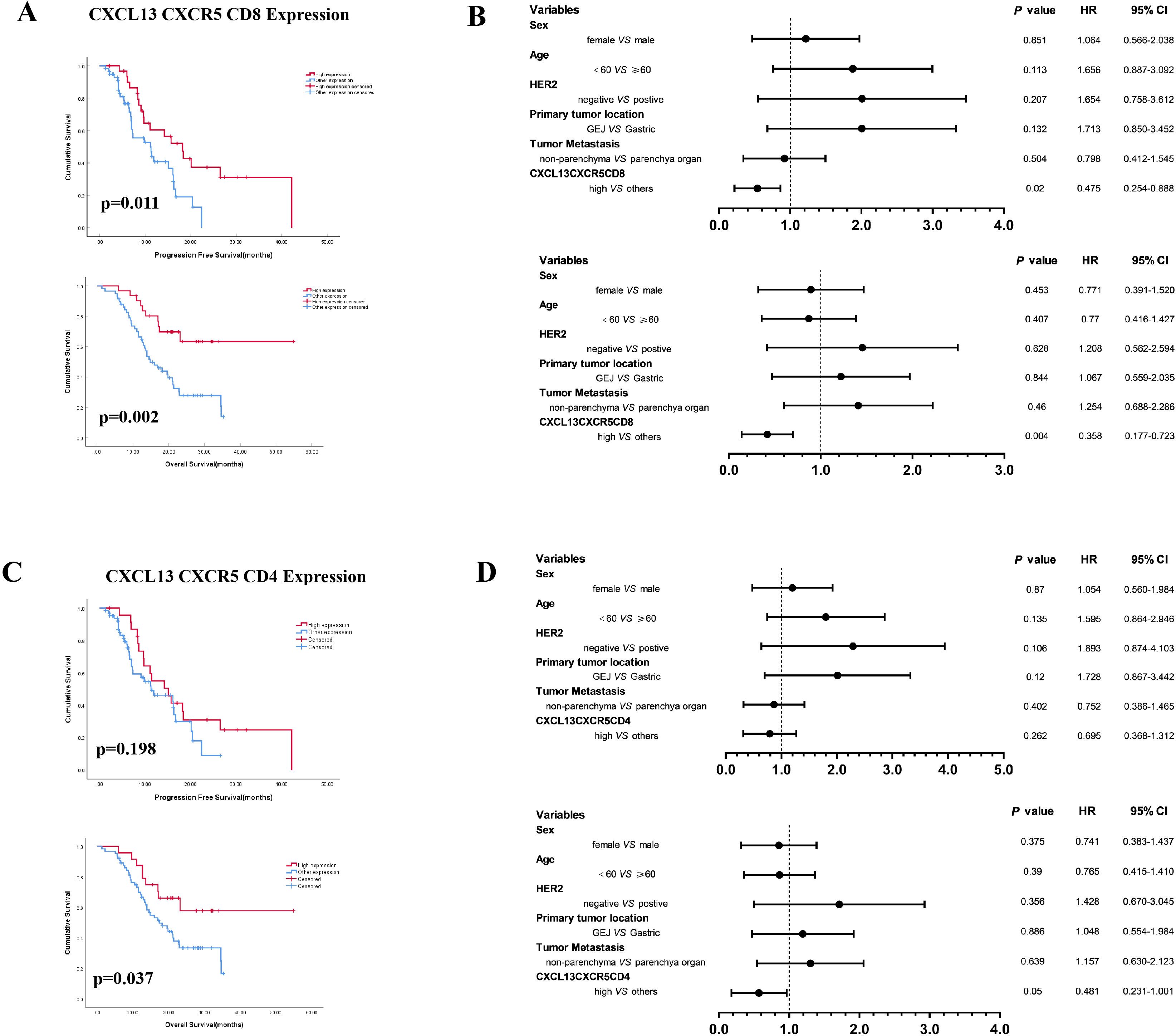
Figure 3. (A) Kaplan–Meier survival curves for PFS and OS according to concurrent high expression of CXCL13, CXCR5, and CD8 (CXCL13HCXCR5HCD8H vs. other expression). (B) Multivariate analysis based on clinicopathological characteristics and the expression of CXCL13, CXCR5, and CD8 in cohort 1 patients. (C) CXCL13, CXCR5, and CD4 (CXCL13HCXCR5HCD4H vs. other expression). (D) Multivariate analysis based on clinicopathological characteristics and the expression of CXCL13, CXCR5, and CD4 in cohort 1 patients (log-rank test for Kaplan–Meier curves. HR, hazard ratio; CI, confidence interval).
CXCR5 expression was correlated with the presence of TLSs in GC patients
Previous studies have demonstrated that TLSs may influence the efficacy of immunotherapy by regulating immune cell infiltration. The CXCL13–CXCR5 axis jointly contributes to the formation of TLSs in malignant melanoma, and the presence of TLSs is associated with increased benefits from ICI treatment (27). In our study, TLSs were identified in 51 of the 88 GC specimens. Survival analysis indicated that the presence of TLSs improved the immunotherapy outcomes. The median PFS was 16.1 months in patients with TLSs compared with 9.1 months in those without TLSs (p = 0.062). The median OS was 22.9 months vs. 17.0 months, respectively (p = 0.085) (Figure 4A). Among the patients with TLSs, 24 exhibited high CXCL13 expression and prolonged PFS (p = 0.039) and OS (p < 0.001) (Table 2). Of these 24 patients, 19 (79.2%) showed concurrent high expression of CXCR5 and CD8 (CXCR5HCD8H) (Figure 4C). IHC results further revealed significant enrichment of CXCR5 within TLSs (Figure 4B). In contrast, among patients with low CXCL13 expression, the proportion of CXCR5HCD8H was lower, regardless of the presence of TLSs (Figure 4C). These findings suggest that the CXCL13–CXCR5 axis may contribute to TLS formation, potentially regulating CD8+ T-cell infiltration and influencing clinical responses to immunotherapy in GC.
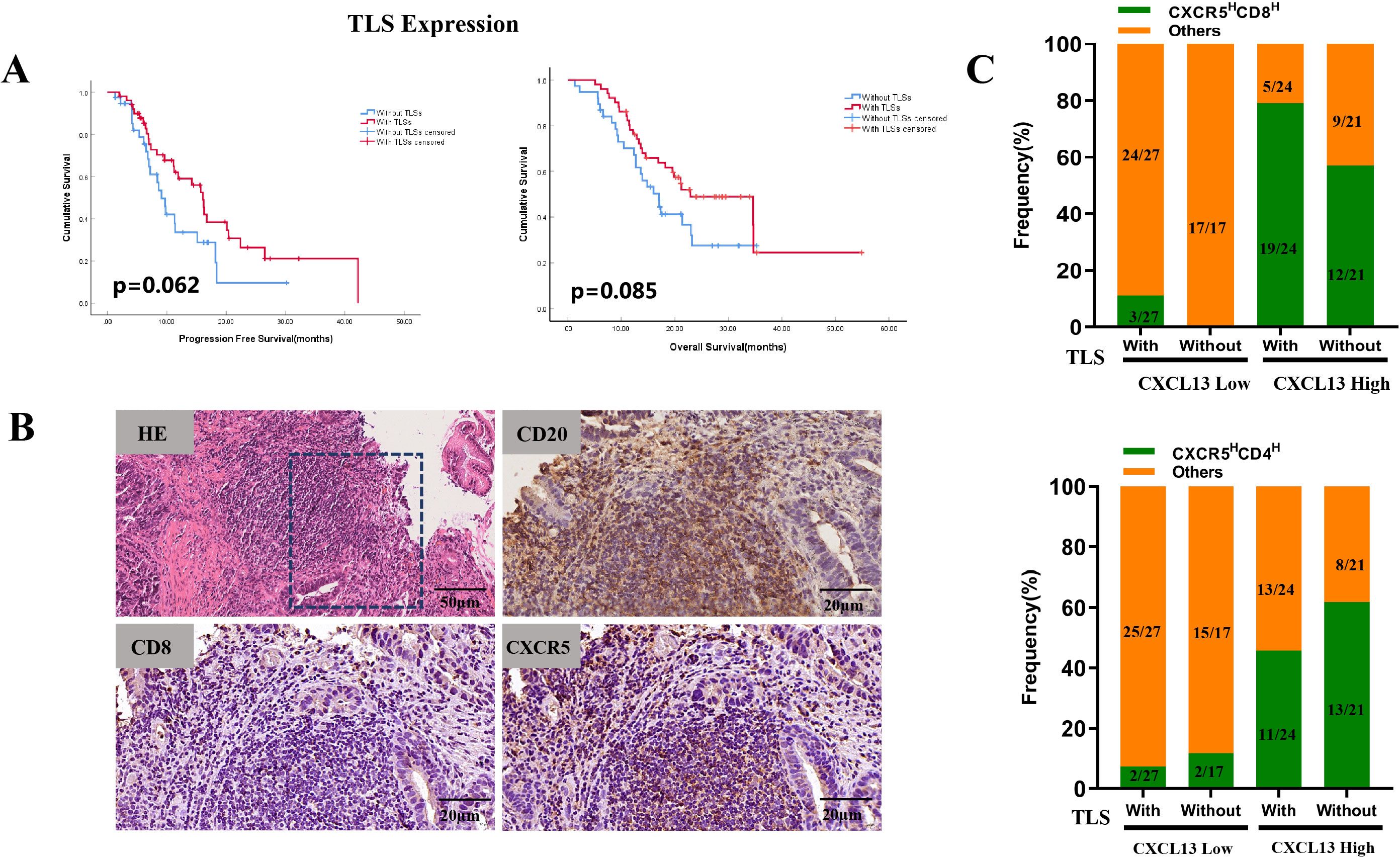
Figure 4. (A) Kaplan–Meier survival curves for PFS (left) and OS (right) in cohort 1 patients stratified by the presence of TLSs. (B) Representative images of TLSs (H&E staining) and expression of CD20, CD8, and CXCR5 within TLSs. (C) Frequencies of CXCR5HCD8H and CXCR5HCD4H expression were compared to other expression groups, based on TLS presence and CXCL13 expression levels.
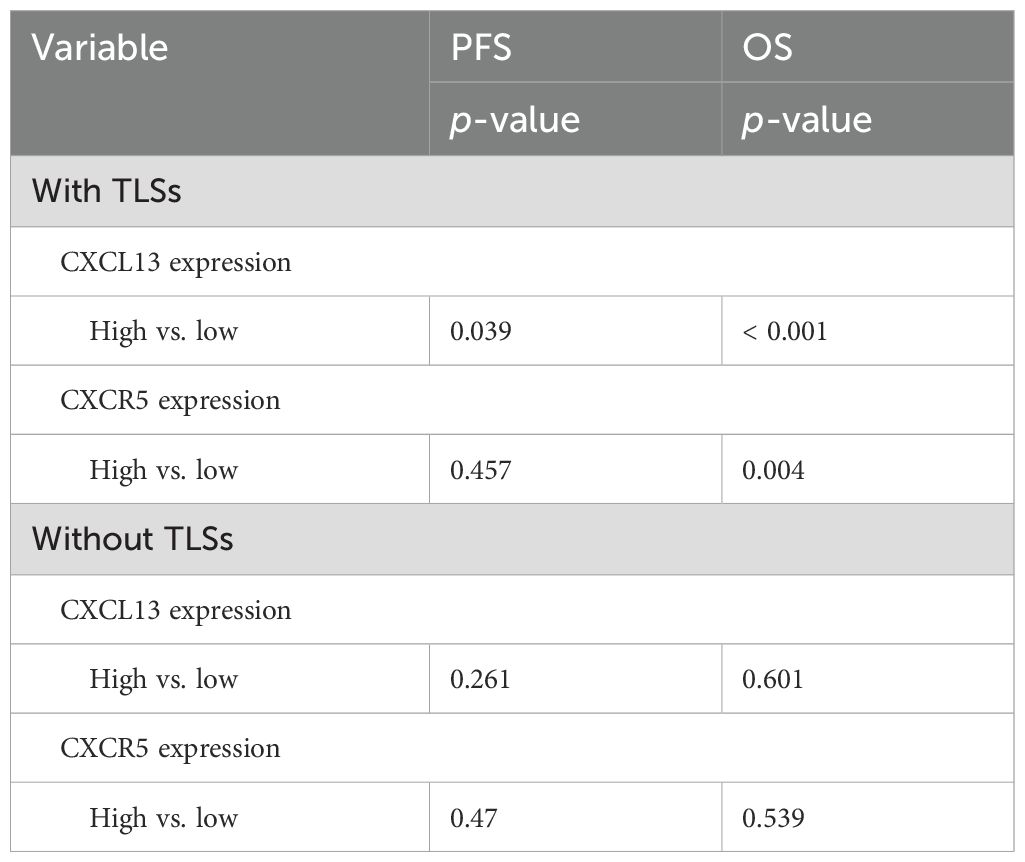
Table 2. Analysis of the correlation between molecular marker expression with/without TLSs and survival.
CXCL13 enhances the response to anti-PD-1 therapy in a humanized subcutaneous GC mouse model
Given our findings that concurrent high expression of CXCL13, CXCR5, and CD8+ T cells predicts a favorable clinical response to ICIs in patients with GC and that CXCR5+CD8+ T cells may play a pivotal role during ICI treatment, we further investigated the impact of CXCL13 on anti-PD-1 therapy. Xenograft tumor models were established using the GC cell line MKN-28 in immunodeficient NOD/SCID mice, which were humanized via the engraftment of PBMCs (Figure 5A). Combination treatment with CXCL13 and anti-PD-1 antibody significantly suppressed tumor growth compared to both the monotherapy and control groups (Figures 5B, C). Furthermore, the combination therapy increased the proportion of CXCR5+CD8+ T cells and reduced the ratio of PD-1+CD8+ T cells in the peripheral blood (Figures 6A, B). No significant differences in immune cell infiltration were observed in the spleen or tumor tissues across the groups. Additionally, combination treatment significantly promoted the expression of the effector cytokines GZMB and IFN-γ compared to other groups (Figure 6C). These findings suggest that CXCL13 may enhance the response to anti-PD-1 antibody therapy by expanding the population of CXCR5+CD8+ T cells.
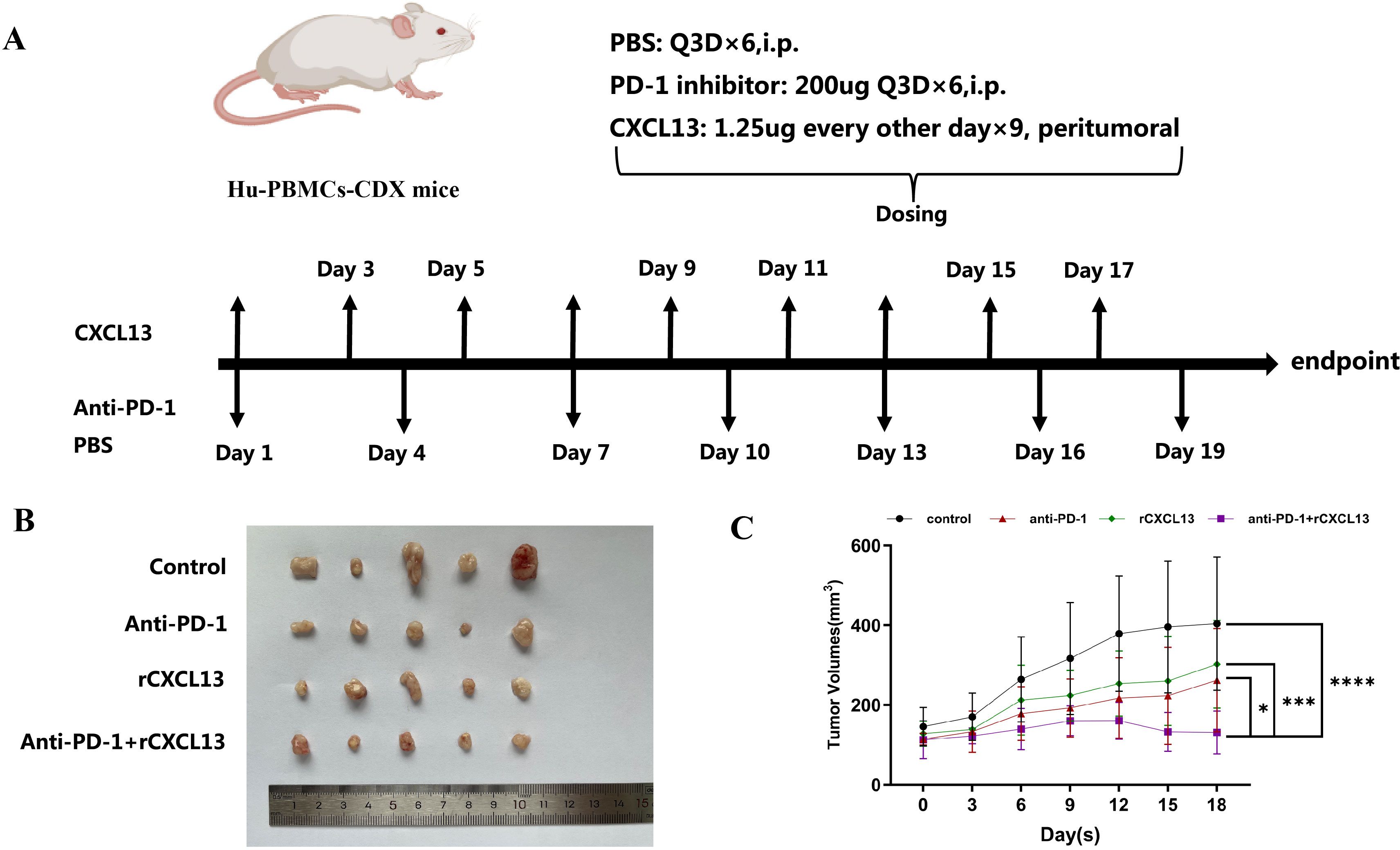
Figure 5. (A) Schematic depiction of the animal experiments and treatment administration details. (B) Combined treatment with CXCL13 and anti-PD-1 antibody effectively suppressed subcutaneous tumor growth. (C) Tumor growth curves comparing different treatment groups. (*p < 0.05; ***p < 0.001; ****p < 0.0001).
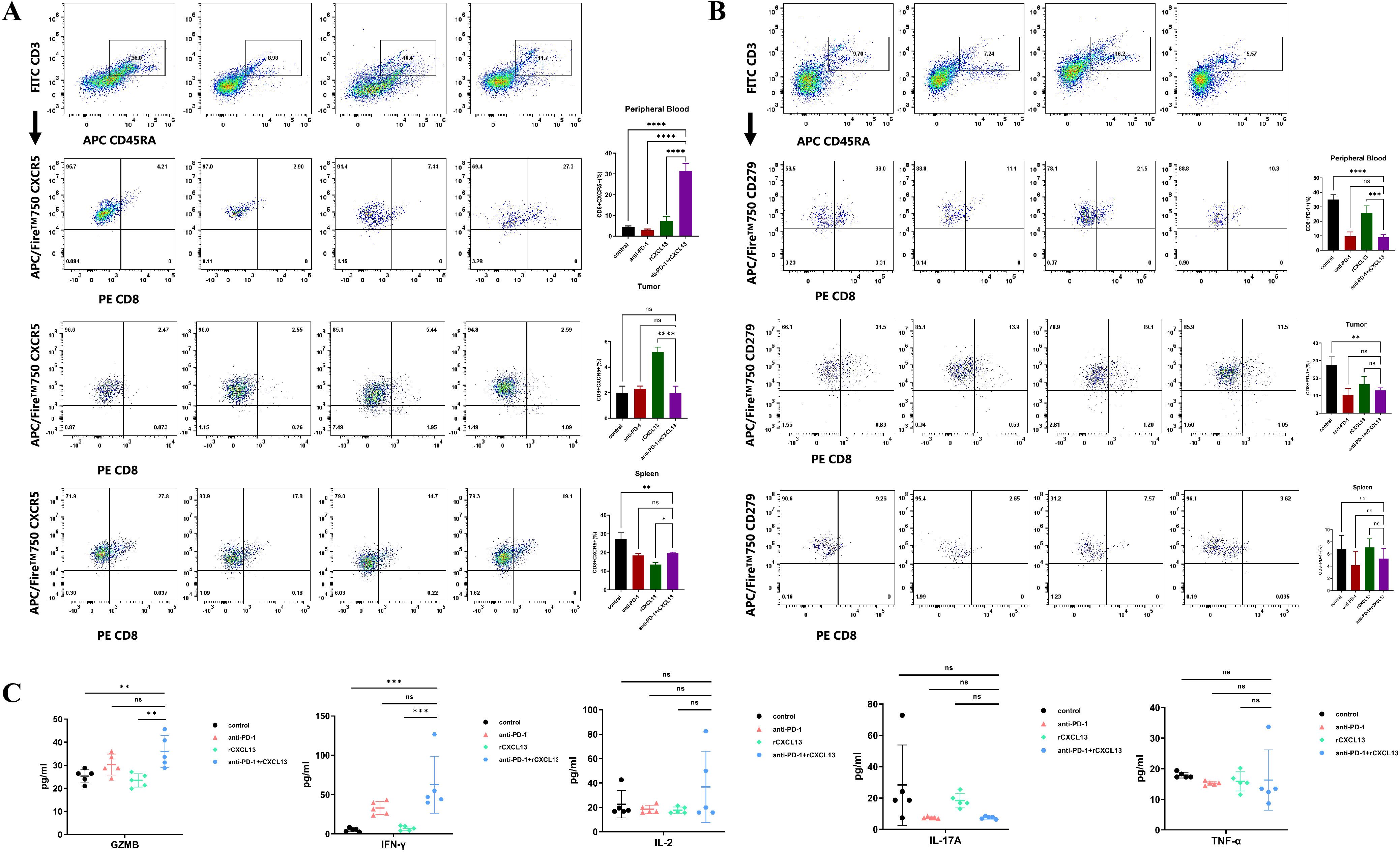
Figure 6. (A) Percentage of CXCR5+CD8+ T cells and (B) PD-1+CD8+ T cells in peripheral blood, subcutaneous tumor, and spleen across the four indicated treatment groups (n = 5 per group). (C) Percentage of effector cytokines (GZMB, IFN-γ, IL-2, IL-17A, and TNF-α) in peripheral blood across the four indicated groups (n = 5 per group). (Bar plots represent mean ± SD; **p < 0.01; ***p < 0.001; ****p < 0.0001; ns, not significant).
Discussion
This study identified the regulatory functions of CXCL13 in immune cell infiltration within the TME of GC patients and highlighted its potential role as a molecular marker for immunotherapy. Elevated CXCL13 expression was associated with prolonged survival. The combined evaluation of CXCL13, CD8, and CXCR5 was confirmed to be an independent prognostic factor in GC patients who received ICI therapy. Previous research has shown that, in EBV-positive GC patients, the clonal expansion of CXCL13+CD8+ T cells is substantially increased in responders after immunotherapy (28). CXCL13 has been identified as a critical regulator of immune cell recruitment and differentiation within the TME in GC patients with signet ring cell carcinoma (29). Additionally, CXCL13 may attract CXCR5+CD8+ T cells to the TME and enhance cytotoxic T-lymphocyte function by regulating the expression of GZMB, TNF-α, IFN-γ, and other cytokines (30). Concordantly, our study also observed significantly elevated GZMB and IFN-γ levels during immunotherapy, which may indicate the activation of CXCR5+CD8+ T cells. These results suggest that CXCL13 plays a vital role in PD-1-based therapies for GC.
CXCL13 influences the efficacy of ICI treatment by modulating immune cell infiltration in various solid cancers. High levels of pre-treatment CXCL13+ T cells are associated with pro-inflammatory macrophage activity and predict favorable responses in patients with triple-negative breast cancer receiving paclitaxel combined with a PD-L1 monoclonal antibody (31). Interactions between follicular helper T cells (CD4 TFH), tissue-resident T cells (CD8 TRM), and B cells mediated by the CXCL13–CXCR5 axis are crucial for anti-tumor immunity in EGFR-mutated non-small cell lung cancer (NSCLC). Dysregulation of TFH-B and TRM-B crosstalk may contribute to poor responses to PD-1 blockade in EGFR-mutated NSCLC (32). In ovarian cancer, combination therapy with CXCL13 and an anti-PD-1 antibody inhibited tumor growth in a CXCR5+CD8+ T-cell expansion-dependent manner (23). Similarly, tumor growth in NSCLC was effectively suppressed by combination treatment (26). In bladder cancer, data from the CheckMate-275 and IMvigor210 trials demonstrated that patients with ARID1A mutations and high CXCL13 expression had favorable outcomes following ICI treatment (33).
In addition to exerting anti-tumor functions through immunomodulatory effects on the TME, studies have reported that CXCL13 may directly affect tumor cells. In prostate cancer, activation of the nonclassical NF-κB signaling pathway promotes the secretion of CXCL13 in tumor cells. Elevated CXCL13 secretion, in turn, enhances tumor growth, migration, and invasion through CXCR5 on the tumor cell surface (34). Furthermore, CXCL13 increases the expression of EVT4 in pancreatic ductal adenocarcinoma (PDAC), and EVT4 promotes PDAC invasion and metastasis by binding to CXCR5 on the tumor cell surface (35). These results suggest that CXCL13 may exert a direct non-chemokine effect on tumor cells. However, further confirmation is needed due to inconsistencies between the study findings.
TLSs serve as prognostic and predictive biomarkers (36) and are linked to higher objective responses to PD-1 blockade in various solid tumors. During tumorigenesis, TLSs act as effective sites for tumor-immune interactions within the TME, triggering inflammatory responses by immune cell infiltration (37). In patients with stage IV GC, responders exhibited a higher percentage of TLSs and increased infiltration of CXCL13+CD160+CD8+ T cells following immunochemotherapy (38). Furthermore, upregulation of CXCL13 expression facilitates the recruitment of CXCR5+ B cells and the formation of TLSs. Alternatively, one study found that CXCL13+CD103+CD8+ Trm cells within TLSs were associated with a better response to anti-PD-1 therapy (39). This suggests a significant role for CXCL13-dependent TLS formation in the efficacy of ICI treatment (23, 40). In our study, patients with TLSs showed improved outcomes from immunotherapy. CXCL13 increased the expression of CXCR5 and CD8+ T cells, particularly in patients with TLSs. TLSs are composed of various immune cell types, and the characteristics of these cells are crucial for TLS maturation (41). Mature TLSs are more likely to show significant clinical benefits after ICI treatment (42). Therefore, multiple factors likely contribute to the antitumor immunity of TLSs, and the specific regulatory mechanisms underlying CXCL13-dependent TLS formation warrant further investigation.
This study has several limitations. First, the sample size is relatively small. Second, most of the patients had stage IV diseases, which led to clinically heterogeneous findings. Third, further mechanistic investigation is needed, including additional molecular biology experiments to verify the mechanism by which CXCL13 recruits CXCR5+CD8+ T cells to the TME and promotes the formation of TLSs, as well as the co-expression of the above indicators in GC. Despite these limitations, our findings highlight the clinical predictive value of CXCL13 in GC and suggest that its underlying mechanisms warrant further exploration.
In summary, our offer insights into how CXCL13 promotes the response of GC patients to ICI therapy. We identified CXCL13 as a prognostic marker for GC and demonstrated that its critical role in the antitumor microenvironment is mediated through CXCR5+CD8+ T cells.
Data availability statement
The original contributions presented in the study are included in the article/Supplementary Material. Further inquiries can be directed to the corresponding authors.
Ethics statement
The study protocol and all amendments were reviewed and approved by the Institutional Review Board and Ethics Committee of Tianjin Medical University Cancer Institute and Hospital and The Affiliated Cancer Hospital of Zhengzhou University (ethical approval number: E2019401; 2019209). The studies were conducted in accordance with the local legislation and institutional requirements. The participants provided their written informed consent to participate in this study. The animal testing procedures have been approved by the Code of Ethics and reviewed and implemented according to the guidelines of the Animal Care and Use Committee of Zhengzhou University (number: 2023-YYY-044). The study was conducted in accordance with the local legislation and institutional requirements.
Author contributions
SX: Data curation, Writing – review & editing. DL: Data curation, Formal analysis, Writing – original draft, Writing – review & editing. TN: Data curation, Writing – review & editing. YaL: Data curation, Writing – review & editing. YS: Data curation, Writing – review & editing. HB: Data curation, Writing – review & editing. LQ: Writing – review & editing, Data curation. TD: Conceptualization, Writing – review & editing. YiL: Conceptualization, Writing – review & editing.
Funding
The author(s) declare that no financial support was received for the research and/or publication of this article.
Acknowledgments
We thank all the patients and their families for participating in this study. We also thank the doctors from the Department of Pathology for their valuable support.
Conflict of interest
The authors declare that the research was conducted in the absence of any commercial or financial relationships that could be construed as a potential conflict of interest.
Generative AI statement
The author(s) declare that no Generative AI was used in the creation of this manuscript.
Publisher’s note
All claims expressed in this article are solely those of the authors and do not necessarily represent those of their affiliated organizations, or those of the publisher, the editors and the reviewers. Any product that may be evaluated in this article, or claim that may be made by its manufacturer, is not guaranteed or endorsed by the publisher.
Supplementary material
The Supplementary Material for this article can be found online at: https://www.frontiersin.org/articles/10.3389/fimmu.2025.1551259/full#supplementary-material.
References
1. Sung H, Ferlay J, Siegel RL, Laversanne M, Soerjomataram I, Jemal A, et al. Global cancer statistics 2020: GLOBOCAN estimates of incidence and mortality worldwide for 36 cancers in 185 countries. CA Cancer J Clin. (2021) 71:209–49. doi: 10.3322/caac.21660
2. Chen W, Zheng R, Baade PD, Zhang S, Zeng H, Bray F, et al. Cancer statistics in China, 2015. CA Cancer J Clin. (2016) 66:115–32. doi: 10.3322/caac.21338
3. Janjigian YY, Shitara K, Moehler M, Garrido M, Salman P, Shen L, et al. First-line nivolumab plus chemotherapy versus chemotherapy alone for advanced gastric, gastro-oesophageal junction, and oesophageal adenocarcinoma (CheckMate 649): a randomised, open-label, phase 3 trial. Lancet. (2021) 398:27–40. doi: 10.1016/S0140-6736(21)00797-2
4. Xu J, Jiang H, Pan Y, Gu K, Cang S, Han L, et al. LBA53 Sintilimab plus chemotherapy (chemo) versus chemo as first-line treatment for advanced gastric or gastroesophageal junction (G/GEJ) adenocarcinoma (ORIENT-16): First results of a randomized, double-blind, phase III study. Ann Oncol. (2021) 32:S1331. doi: 10.1016/j.annonc.2021.08.2133
5. Rha SY, Oh D-Y, Yañez P, Bai Y, Ryu MH, Lee J, et al. Pembrolizumab plus chemotherapy versus placebo plus chemotherapy for HER2-negative advanced gastric cancer (KEYNOTE-859): a multicentre, randomised, double-blind, phase 3 trial. Lancet Oncol. (2023) 24:1181–95. doi: 10.1016/S1470-2045(23)00515-6
6. Janjigian YY, Kawazoe A, Yanez P, Li N, Lonardi S, Kolesnik O, et al. The KEYNOTE-811 trial of dual PD-1 and HER2 blockade in HER2-positive gastric cancer. Nature. (2021) 600:727–30. doi: 10.1038/s41586-021-04161-3
7. Yoon HH, Jin Z, Kour O, Kankeu Fonkoua LA, Shitara K, Gibson MK, et al. Association of PD-L1 expression and other variables with benefit from immune checkpoint inhibition in advanced gastroesophageal cancer: systematic review and meta-analysis of 17 phase 3 randomized clinical trials. JAMA Oncol. (2022) 8:1456–65. doi: 10.1001/jamaoncol.2022.3707
8. Chao J, Fuchs CS, Shitara K, Tabernero J, Muro K, Van Cutsem E, et al. Assessment of pembrolizumab therapy for the treatment of microsatellite instability–high gastric or gastroesophageal junction cancer among patients in the KEYNOTE-059, KEYNOTE-061, and KEYNOTE-062 clinical trials. JAMA Oncol. (2021) 7:895–902. doi: 10.1001/jamaoncol.2021.0275
9. Pietrantonio F, Randon G, Di Bartolomeo M, Luciani A, Chao J, Smyth EC, et al. Predictive role of microsatellite instability for PD-1 blockade in patients with advanced gastric cancer: a meta-analysis of randomized clinical trials. ESMO Open. (2021) 6:100036. doi: 10.1016/j.esmoop.2020.100036
10. Wang F, Wei XL, Wang FH, Xu N, Shen L, Dai GH, et al. Safety, efficacy and tumor mutational burden as a biomarker of overall survival benefit in chemo-refractory gastric cancer treated with toripalimab, a PD-1 antibody in phase Ib/II clinical trial NCT02915432. Ann Oncol. (2019) 30:1479–86. doi: 10.1093/annonc/mdz197
11. Xie T, Liu Y, Zhang Z, Zhang X, Gong J, Qi C, et al. Positive status of epstein-barr virus as a biomarker for gastric cancer immunotherapy: A prospective observational study. J Immunotherapy. (2020) 43:139–44. doi: 10.1097/CJI.0000000000000316
12. Janjigian YY, Bendell J, Calvo E, Kim JW, Ascierto PA, Sharma P, et al. CheckMate-032 study: efficacy and safety of nivolumab and nivolumab plus ipilimumab in patients with metastatic esophagogastric cancer. J Clin Oncol. (2018) 36:2836–44. doi: 10.1200/JCO.2017.76.6212
13. Bejarano L, Jordao MJC, Joyce JA. Therapeutic targeting of the tumor microenvironment. Cancer Discov. (2021) 11:933–59. doi: 10.1158/2159-8290.CD-20-1808
14. Sadeghi Rad H, Monkman J, Warkiani ME, Ladwa R, O'Byrne K, Rezaei N, et al. Understanding the tumor microenvironment for effective immunotherapy. Med Res Rev. (2021) 41:1474–98. doi: 10.1002/med.21765
15. Li S, Li K, Tian F, Li H, Xia Q, Li T, et al. A high interferon gamma signature of CD8(+) T cells predicts response to neoadjuvant immunotherapy plus chemotherapy in gastric cancer. Front Immunol. (2022) 13:1056144. doi: 10.3389/fimmu.2022.1056144
16. Ozga AJ, Chow MT, Luster AD. Chemokines and the immune response to cancer. Immunity. (2021) 54:859–74. doi: 10.1016/j.immuni.2021.01.012
17. Märkl F, Huynh D, Endres S, Kobold S. Utilizing chemokines in cancer immunotherapy. Trends Cancer. (2022) 8:670–82. doi: 10.1016/j.trecan.2022.04.001
18. Yang H, Qiu B, Chen S, Xun Y, Pan Y, Chen M, et al. Soluble CXCL16 promotes TNF-α-induced apoptosis in DLBCL via the AMAD10-NF-κB regulatory feedback loop. Cell Biol Int. (2019) 43:863–74. doi: 10.1002/cbin.11154
19. Liu D, Qi F, Liu W, Liu W, Liu J, Wang J, et al. Integrated analysis of 14 lymphoma datasets revealed high expression of CXCL14 promotes cell migration in mantle cell lymphoma. Aging (Albany NY). (2022) 14:3346–463. doi: 10.18632/aging.204022
20. Wang B, Wang M, Ao D, Wei X. CXCL13-CXCR5 axis: Regulation in inflammatory diseases and cancer. Biochim Biophys Acta Rev Cancer. (2022) 1877:188799. doi: 10.1016/j.bbcan.2022.188799
21. Nagarsheth N, Wicha MS, Zou W. Chemokines in the cancer microenvironment and their relevance in cancer immunotherapy. Nat Rev Immunol. (2017) 17:559–72. doi: 10.1038/nri.2017.49
22. Wang F, Long J, Li L, Wu ZX, Da TT, Wang XQ, et al. Single-cell and spatial transcriptome analysis reveals the cellular heterogeneity of liver metastatic colorectal cancer. Sci Advances. (2023) 9:eadf5464. doi: 10.1126/sciadv.adf5464
23. Yang M, Lu J, Zhang G, Wang Y, He M, Xu Q, et al. CXCL13 shapes immunoactive tumor microenvironment and enhances the efficacy of PD-1 checkpoint blockade in high-grade serous ovarian cancer. J Immunother Cancer. (2021) 9:e001136. doi: 10.1136/jitc-2020-001136
24. Wu C, Wang X, Shang H, Wei H. Construction of a humanized PBMC-PDX model to study the efficacy of a bacterial marker in lung cancer immunotherapy. Dis Markers. (2022) 2022:1479246. doi: 10.1155/2022/1479246
25. Pyo KH, Kim JH, Lee JM, Kim SE, Cho JS, Lim SM, et al. Promising preclinical platform for evaluation of immuno-oncology drugs using Hu-PBL-NSG lung cancer models. Lung Cancer. (2019) 127:112–21. doi: 10.1016/j.lungcan.2018.11.035
26. Sorin M, Karimi E, Rezanejad M, Yu MW, Desharnais L, McDowell SAC, et al. Single-cell spatial landscape of immunotherapy response reveals mechanisms of CXCL13 enhanced antitumor immunity. J Immunother Cancer. (2023) 11. doi: 10.1136/jitc-2022-005545
27. Cabrita R, Lauss M, Sanna A, Donia M, Skaarup Larsen M, Mitra S, et al. Tertiary lymphoid structures improve immunotherapy and survival in melanoma. Nature. (2020) 577:561–5. doi: 10.1038/s41586-019-1914-8
28. Qiu MZ, Wang C, Wu Z, Zhao Q, Zhao Z, Huang CY, et al. Dynamic single-cell mapping unveils Epstein–Barr virus-imprinted T-cell exhaustion and on-treatment response. Signal Transduct Target Ther. (2023) 8:370. doi: 10.1038/s41392-023-01622-1
29. Chen J, Liu K, Luo Y, Kang M, Wang J, Chen G, et al. Single-cell profiling of tumor immune microenvironment reveals immune irresponsiveness in gastric signet-ring cell carcinoma. Gastroenterology. (2023) 165:88–103. doi: 10.1053/j.gastro.2023.03.008
30. E J, Yan F, Kang Z, Zhu L, Xing J, Yu E. CD8+CXCR5+ T cells in tumor-draining lymph nodes are highly activated and predict better prognosis in colorectal cancer. Hum Immunol. (2018) 79:446–52. doi: 10.1016/j.humimm.2018.03.003
31. Zhang Y, Chen H, Mo H, Hu X, Gao R, Zhao Y, et al. Single-cell analyses reveal key immune cell subsets associated with response to PD-L1 blockade in triple-negative breast cancer. Cancer Cell. (2021) 39:1578–1593 e1578. doi: 10.1016/j.ccell.2021.09.010
32. Cho JW, Park S, Kim G, Han H, Shim HS, Shin S, et al. Dysregulation of T(FH)-B-T(RM) lymphocyte cooperation is associated with unfavorable anti-PD-1 responses in EGFR-mutant lung cancer. Nat Commun. (2021) 12:6068. doi: 10.1038/s41467-021-26362-0
33. Goswami S, Chen Y, Anandhan S, Szabo PM, Basu S, Blando JM, et al. ARID1A mutation plus CXCL13 expression act as combinatorial biomarkers to predict responses to immune checkpoint therapy in mUCC. Sci Trans Med. (2020) 12:eabc4220. doi: 10.1126/scitranslmed.abc4220
34. Garg R, Blando JM, Perez CJ, Abba MC, Benavides F, Kazanietz MG. Protein kinase C epsilon cooperates with PTEN loss for prostate tumorigenesis through the CXCL13-CXCR5 pathway. Cell Rep. (2017) 19:375–88. doi: 10.1016/j.celrep.2017.03.042
35. Gao X, Jiang M, Chu Y, Han Y, Jin Y, Zhang W, et al. ETV4 promotes pancreatic ductal adenocarcinoma metastasis through activation of the CXCL13/CXCR5 signaling axis. Cancer Lett. (2022) 524:42–56. doi: 10.1016/j.canlet.2021.09.026
36. Schumacher TN, Thommen DS. Tertiary lymphoid structures in cancer. Science. (2022) 375:eabf9419. doi: 10.1126/science.abf9419
37. Teillaud J-L, Houel A, Panouillot M, Riffard C, Dieu-Nosjean MC. Tertiary lymphoid structures in anticancer immunity. Nat Rev Cancer. (2024) 24:629–46. doi: 10.1038/s41568-024-00728-0
38. Wang J, Liang Y, Xue A, Xiao J, Zhao X, Cao S, et al. Intratumoral CXCL13+CD160+CD8+T cells promote the formation of tertiary lymphoid structures to enhance the efficacy of immunotherapy in advanced gastric cancer. J ImmunoTherapy Cancer. (2024) 12. doi: 10.1136/jitc-2024-009603
39. Hu C, You W, Kong D, Huang Y, Lu J, Zhao M, et al. Tertiary lymphoid structure-associated B cells enhance CXCL13+CD103+CD8+ Tissue-resident memory T-cell response to programmed cell death protein 1 blockade in cancer immunotherapy. Gastroenterology. (2024) 166:1069–84. doi: 10.1053/j.gastro.2023.10.022
40. Groeneveld CS, Fontugne J, Cabel L, Bernard-Pierrot I, Radvanyi F, Allory Y, et al. Tertiary lymphoid structures marker CXCL13 is associated with better survival for patients with advanced-stage bladder cancer treated with immunotherapy. Eur J Cancer. (2021) 148:181–9. doi: 10.1016/j.ejca.2021.01.036
41. Sautès-Fridman C, Petitprez F, Calderaro J, Fridman WH. Tertiary lymphoid structures in the era of cancer immunotherapy. Nat Rev Cancer. (2019) 19:307–25. doi: 10.1038/s41568-019-0144-6
Keywords: gastric cancer, immunotherapy, prognosis markers, CXCL13, CXCR5
Citation: Xu S, Li D, Ning T, Lu Y, Sun Y, Bai H, Qiao L, Deng T and Liu Y (2025) High expression of CXCL13 predicts a favorable response to immunotherapy by upregulating CXCR5+CD8+ T-cell infiltration in gastric cancer. Front. Immunol. 16:1551259. doi: 10.3389/fimmu.2025.1551259
Received: 25 December 2024; Accepted: 14 April 2025;
Published: 08 May 2025.
Edited by:
Qing Xi, First Affiliated Hospital of Guangzhou Medical University, ChinaReviewed by:
Wenxing Li, Columbia University, United StatesXiang-Xu Wang, Air Force Medical University, China
Maoshu Bai, Dazhou Integrated Traditional Chinese Medicine and Western Medicine Hospital, China
Copyright © 2025 Xu, Li, Ning, Lu, Sun, Bai, Qiao, Deng and Liu. This is an open-access article distributed under the terms of the Creative Commons Attribution License (CC BY). The use, distribution or reproduction in other forums is permitted, provided the original author(s) and the copyright owner(s) are credited and that the original publication in this journal is cited, in accordance with accepted academic practice. No use, distribution or reproduction is permitted which does not comply with these terms.
*Correspondence: Ying Liu, emx5eWxpdXlpbmcxNjY0QHp6dS5lZHUuY24=; Ting Deng, eHltY2Rlbmd0aW5nQDEyNi5jb20=
†These authors have contributed equally to this work