- 1Laboratory of Malaria Immunology and Vaccinology, National Institute of Allergy and Infectious Diseases, National Institutes of Health, Bethesda, MD, United States
- 2Vagelos College of Physicians and Surgeons, Columbia University, New York, NY, United States
Malaria in pregnancy (MiP) poses a dangerous health risk to both mothers and their fetuses, causing severe outcomes such as preterm delivery, intrauterine growth restriction, miscarriage, stillbirth, and neonatal and maternal death. Plasmodium falciparum infected erythrocytes sequester in placental intervillous spaces causing placental malaria (PM), eliciting inflammatory responses associated with severe sequelae. Current MiP prevention strategies have improved pregnancy outcomes, but serious morbidity and mortality persist. Vaccines to prevent MiP and PM are under development and are expected to improve pregnancy outcomes. To prepare for safety and efficacy trials of these vaccines, the incidence of adverse pregnancy outcomes including those caused by MiP should be documented at clinical sites. This review summarizes reported key adverse pregnancy outcomes attributable to MiP, providing important baseline context to define measurable safety and efficacy endpoints for malaria vaccine trials in pregnancy.
Introduction
Despite progress in reducing malaria globally, malaria in pregnancy (MiP) remains a pervasive issue in endemic areas. According to the World Health Organization (WHO), malaria infection occurred in 32% of pregnancies from 38 moderate-to-high transmission African countries in 2021 (1). Exposure to malaria during pregnancy, even in women with pre-existing immunity, poses risks to both the mother and her fetus, as infection increases the risk of maternal death, severe maternal anemia, and fetal and neonatal death (2, 3). While pregnant women and children under age 5 are most vulnerable to severe malaria complications, poor pregnancy outcomes are often missed as malaria-related events, and generally not included in annual malaria burden reports such as malaria-related infant mortality estimates (4). Further, it has been demonstrated that malaria-endemic regions, such as countries in sub-Saharan Africa and South Asia, have pregnancies with the highest risk of death for the newborn but the lowest availability of data on adverse birth outcomes. This lack of data, particularly lack of national administrative data, poses difficulties in establishing baseline rates of adverse outcomes in regions where interventions are needed most (5). Current efforts to develop vaccines that prevent MiP will benefit from defined and measurable safety and efficacy endpoints including malaria-related adverse birth outcomes, such as embryonic, fetal, and neonatal deaths occurring from conception to the weeks following birth. In this review, we briefly review the pathogenesis of placental malaria and existing tools for prevention, and then focus on MiP-related pregnancy outcomes including miscarriage, stillbirth, perinatal death, preterm birth (PTB), and low birth weight (LBW) (Figure 1) and discuss their confounding or contributing factors, as a context to plan for future MiP vaccine trials.
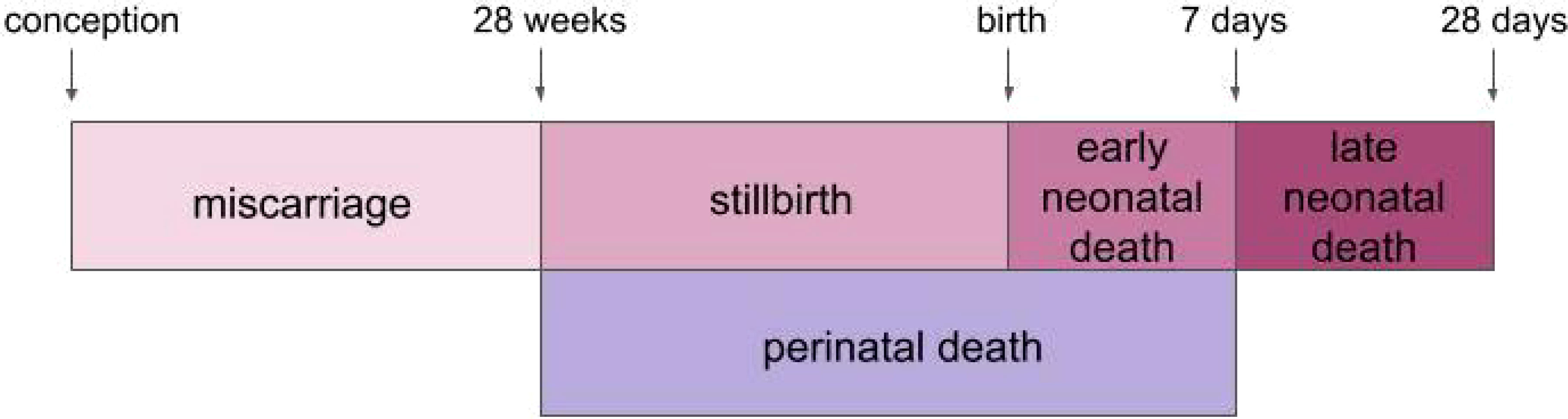
Figure 1 Timeline of adverse outcomes throughout pregnancy. Miscarriage, stillbirth, perinatal death, early neonatal death and late neonatal death are represented according to their timing during or after pregnancy.
Pathology of placental malaria
While adult residents of malaria-endemic regions typically enjoy immunity acquired over frequent exposures to malaria parasites, malaria poses a new risk to pregnant women. Women are particularly susceptible to malaria in their first pregnancy due to placental sequestration of Plasmodium falciparum infected erythrocytes. Parasites with binding affinity to the placental receptor chondroitin sulfate A (CSA) are responsible for this placental sequestration, defining a placental malaria (PM) syndrome (6). Nulligravidae lack immunity to CSA-binding parasites, but malaria-exposed women acquire protective antibodies to PM over successive pregnancies. These protective antibodies, including antibodies against the variant surface protein VAR2CSA, block parasite adhesion to CSA in the placenta (7). VAR2CSA is a distinctly structured member of the PfEMP1 family of variant surface antigens and mediates parasite adhesion to CSA in the placenta (8). The sequestration of parasite-infected erythrocytes within the intervillous spaces of the placenta elicits an inflammatory infiltrate and placental pathology that are associated with poor pregnancy outcomes.
Placental parasitemia may contribute to these adverse outcomes in a number of ways. Healthy pregnancies are characterized by immunomodulatory phenomena and a predominant Th2 immunity, while inflammatory responses to PM including Th1 cytokines can alter this balance and contribute to sequelae (9, 10). For example, increased inflammatory responses in the P. falciparum infected placenta can lead to oxidative stress and apoptosis of placental cells (11). This stress on the placenta can have deleterious effects on embryo development and parturition. Placental structure can also be compromised, wherein women with MiP display decreased transport villi, increased placental lesions, and syncytial knotting (12, 13). Further, a Doppler ultrasound study in Kenya demonstrates that P. falciparum infection is associated with abnormal uterine artery blood flow (14)
Current strategies to prevent malaria in pregnancy
Diagnosis of placental malaria
Diagnosing malaria early during infection is important to prevent disease sequelae but is not easily accomplished. Many women carry parasitemia with few or no symptoms, owing to systemic immunity acquired during a lifetime of exposure. The microscopic detection of parasites on blood smears is the most widely used method to detect placental malaria due to long-standing clinical practice. However, parasites are often not detected on a peripheral blood smear, even when relatively large numbers of parasites are sequestered in the placenta (15). Thus, an individual with placental malaria may produce negative test results while infected, leaving them undiagnosed and untreated. For pregnant patients, diagnosis with rapid diagnostic tests (RDTs) serves as an alternative. This immunochromatographic antigen test reports sensitivities above 90%, making it potentially more effective than microscopy or clinical systems of detection (16). In addition, polymerase chain reaction (PCR) techniques have significantly helped to unravel the true burden of infection in pregnancy by detecting sub-microscopic infections that also contribute to poor pregnancy outcomes (17, 18).
Vector control strategies
Vector control strategies for MiP target the Anopheles mosquito to prevent transmission of Plasmodium parasites to pregnant women. WHO currently recommends deployment of insecticide-treated nets (ITNs) for pregnant women in endemic regions (19). Indoor residual spraying (IRS) is typically recommended in malaria-endemic regions but raises safety concerns, as insecticide residues have been found in breast milk and prenatal exposure to insecticides may impact human neurodevelopment (20, 21).
Genetic modification of mosquitoes provides options for more permanent vector control, through sex-specific sterilization, species replacement with genetically modified mosquitoes resistant to parasite infection, and gene drive approaches to spread disadvantageous genetic traits throughout mosquito populations (22). While potentially powerful, gene-based mosquito control strategies for malaria have not been widely implemented, due to many factors including uncertainty of ecological impact, genetic variance among Anopheles species, and difficulty of wide-scale implementation.
Intermittent preventive treatment in pregnancy
In addition to vector control strategies, WHO strongly recommends intermittent preventive treatment in pregnancy (IPTp) with sulfadoxine-pyrimethamine (IPTp-SP) in areas with moderate-to-high malaria transmission (23). IPTp-SP is administered to pregnant women up to once a month after the first trimester. While proven to significantly reduce several malaria-related adverse outcomes, IPTp is not recommended in the first trimester due to teratogenicity concerns, leaving early pregnancies at risk of malaria infection (24, 25). Alternatives to SP have also been explored in studies that investigated mefloquine (26) or dihydroartemisinin-piperaquine in both HIV-negative and -positive women (27, 28). The challenge for malaria control in early pregnancy is compounded by the difficulty in reaching women during this period, as women in endemic regions typically consult for antenatal care at mid-pregnancy or later (29). For instance, attendance to antenatal clinics in the first trimester ranges from 12% in Malawi to 15% in Kenya (30). Of note, parasites infecting women in the first trimester of pregnancy can display a placenta-binding phenotype. These early infections have been associated with adverse outcomes such as LBW (31–33), highlighting the importance of new tools like vaccines that can provide durable protection from before conception through the postpartum period.
Recommendations for antimalarials in the first trimester have been stymied due to concern of these drugs causing birth complications. WHO recommends quinine therapy for uncomplicated P. falciparum malaria in the first trimester despite ample evidence of superior efficacy by artemisinin-containing combination therapies (ACTs) (34). In animal models, artemisinin derivatives have been shown to have teratogenic and embryotoxic effects in early pregnancy (35). However, these toxic effects have not translated to discernible toxicity in humans as a meta-analysis of twenty studies found that treatment with artemisinin during pregnancy was not associated with miscarriage (35). Another meta-analysis of 30,618 pregnancies found no difference in the risk of miscarriage when comparing the use of artemisinin and quinine during the first trimester (36). Considering the ample safety evidence, it may be advantageous to regularly implement ACTs to combat adverse pregnancy outcomes such as miscarriage until vaccines for MiP are available.
Development of vaccines against malaria in pregnancy
Vaccine candidates in clinical trials
Women in endemic areas naturally acquire resistance to PM over successive pregnancies, and this is associated with acquisition of functional antibodies against placenta-binding parasites and the surface antigen VAR2CSA (37). High levels of antibodies against VAR2CSA have been associated with improved pregnancy outcomes, and VAR2CSA is the leading candidate for a placental malaria vaccine (38). PAMVAC and PRIMVAC are two VAR2CSA-based vaccine candidates tested in humans that have demonstrated a good safety profile and induced functional variant-specific but not variant-transcending antibodies (39, 40). More work must be done to develop a placental malaria vaccine effective against a wide range of VAR2CSA-expressing parasite variants, a major challenge given the extensive sequence variation of the protein (41).
While not specifically designed to prevent placental malaria, P. falciparum sporozoite (PfSPZ) Vaccine is an attenuated whole malaria sporozoite vaccine entering the early stages of development in pregnant women. In clinical trials with non-pregnant African adults, PfSPZ Vaccine has been remarkably well-tolerated and showed significant efficacy against P. falciparum infection (42, 43). Thus, the protective efficacy of PfSPZ Vaccine in preventing malaria infection warrants further consideration for trials in pregnant women (44). RTS,S/AS01 and R21/Matrix M™ are recombinant subunit virus-like-particle vaccines that target the same sporozoite surface protein (circumsporozoite protein, CSP) and prevent clinical malaria in African children. These are the only licensed malaria vaccines to-date; RTS,S (45) was recommended by WHO in October 2021 (46), and R21 was first approved for use in Ghana (47), then Nigeria (48), each with the indication to prevent clinical malaria in children aged 5 months to 3 years. These vaccines might also be assessed for benefits against pregnancy malaria. While less explored for MiP, monoclonal antibodies against malaria present another potential intervention. In phase 2 clinical trial, monoclonal antibody CIS43LS has demonstrated protection against P. falciparum infection of Malian adults (49).
Safety and efficacy endpoints for trials in pregnant women
Contemporary information on adverse pregnancy outcomes is needed to monitor safety and efficacy of MiP vaccines during development and implementation. Vaccine trial safety endpoints include number and grade of adverse events in study subjects. In general, safety endpoints for vaccines being tested in pregnant women classify adverse pregnancy outcomes as an adverse event (AE) that can dictate study progress. Maternal, fetal or neonatal death in particular are serious adverse events (SAEs) that can warrant halting a study, so documenting their pre-existing frequencies will be key to ensure safety while mitigating unwarranted delays in trial progress. In malaria endemic regions where adverse pregnancy events occur at high frequencies, baseline rates of AEs should be established before trial initiation. Baseline rates of adverse events provide safety boards with the information necessary to assess whether a vaccine is increasing the incidence of such events.
One such study has been conducted in Mali with a cohort of women of child-bearing age who were monitored monthly for pregnancy via hCG testing. Women who became pregnant were followed thereafter to track their pregnancy outcome, thus providing data regarding the incidence of AEs across all trimesters including miscarriage, stillbirth, preterm delivery, and small for gestational age (50). Such data exemplify the types of information that can serve as benchmarks for efficacy and safety endpoints during vaccine trials. These endpoints are routinely used to assess the safety of any vaccine tested in pregnant women, but are also caused by malaria and therefore can be measured to assess vaccine efficacy.
Region-specific documented ranges of outcomes can provide reference for researchers to assess how vaccine candidates reduce or contribute to fetal or neonatal mortality, and these data can be used to create consensus on trial endpoints globally. Since rates for poor pregnancy outcomes can vary by site due to local factors, documentation of background rates of poor outcomes at a trial site is warranted. This is particularly relevant for early phase trials with small cohort sizes in which a single or few adverse events may be difficult to interpret and could prematurely halt trials. Well-documented background rates will provide an evidence base to more confidently assess the relationship of severe adverse events to the study product. This is especially important considering the relatively high frequency of adverse pregnancy outcomes that occur in malaria-endemic regions, whether due to malaria or other causes.
Pregnancy-specific endpoints require clear definitions that specify factors including timing of gestation during AEs and occurrence of congenital anomalies (44). Further, measurements of these outcomes should be thoroughly standardized so that data can be accurately synthesized across sites. For instance, precision of scales and gestational age assessment tools make a significant impact on reported rates of low birthweight and gestational age respectively (44). Creating vaccine endpoints for MiP requires a nuanced approach that considers multiple metrics with an understanding that the occurrence of AEs does not automatically disqualify a vaccine from being safe and effective. P. falciparum parasitemia is always treated in pregnant women owing to the strong association with poor pregnancy outcomes and is a relatively frequent event with which to assess vaccine efficacy. Thus, baseline rates of parasitemia in pregnant women are useful to calculate sample sizes for example for Phase 2 trials that assess efficacy.
Malaria-related adverse pregnancy outcomes
Miscarriage
Miscarriage is defined here as the death of a fetus during the first two trimesters, or before 28 weeks of pregnancy, per WHO’s definition. However, the definition of the gestational age of viability varies (between 24 and 28 weeks) and it has been general practice that pregnancy losses below that age are considered miscarriages. Preventing miscarriage is important for women and their future pregnancies as previous miscarriage is associated with an increased risk of PTB, fetal growth restriction, and other obstetric complications in subsequent pregnancies (51). Miscarriage is one of the lesser studied complications of MiP, partly due to low attendance at antenatal clinics early in pregnancy in endemic regions (29). In a study conducted on the Thai–Myanmar border, it was found that a single episode of symptomatic or asymptomatic falciparum or vivax malaria in the first-trimester can increase the risk of miscarriage (52). Incidence of miscarriage was highest in women infected with falciparum malaria with 16% of pregnancies resulting in miscarriage compared to 11% of women with vivax infection and 9% of noninfected women. In a cohort in Mali, 43 of 358 pregnancies resulted in miscarriage (12%) with 65.1% of these miscarriages occurring within the first trimester of pregnancy (50). This study did not evaluate malaria infection but demonstrates the burden of miscarriage in the first trimester of pregnancy in a malaria-endemic region of Mali. Additional risk factors for miscarriage in infected women include severe malaria [adjusted odds ratio (aOR) 3·63, 95% CI 1·15–11·46] and increased parasitaemia [aOR 1·49 (1·25–1·78) for each ten-fold increase in parasitaemia] (Table 1) (53).
Stillbirth
An estimated 2.6 million stillbirths occur annually, with 98% in low- and middle-income countries, and prenatal malaria is a major cause (54). An estimated 20% of stillbirths in sub-Saharan Africa are attributable to P. falciparum malaria in pregnancy (55). Pregnancy losses above the gestational age of viability are stillbirths, and here are defined as occurring from 28 weeks of pregnancy onward. Malaria-induced stillbirth may be caused by a myriad of sequelae that develop during infection of the placenta including impaired placental perfusion, maternal anemia, and preterm labor (56, 57).
In an observational study in Mali, malaria infection predicted increased risk of stillbirth (adjusted hazard ratio 3.87, P = .03) (18). In a meta-analysis of nineteen different countries, P. falciparum detected and treated during pregnancy was also associated with stillbirth (OR 1·47 [1·13-1·92], but to a lesser extent than untreated infection (OR 1·95 [95% CI 1·48-2·57]) (55). P. vivax malaria increased the odds of stillbirth when detected at delivery (OR 2·81 [0·77–10·22]), but not when detected and treated in pregnancy (OR 1·09 [0·76–1·57]). The timing of infection is also of interest; in Uganda, malaria within two weeks of delivery was found to be associated with a two-fold greater risk of stillbirth (OR 2.15 [1.04-4.46]) (Table 1) (58). In Sudan, the risk of stillbirth was significantly increased among women who reported malaria infection in the first and second trimester of pregnancy (OR 1.4 [1.1-1.9]). However, antenatal records of pregnant women from the Thai-Burmese border suggest that an episode of malaria in the first trimester does not predispose a pregnancy to later stillbirth (53). Thus, it is not completely clear how malaria in the first trimester is related to stillbirth, and whether this varies across parasite strains or populations.
Preventative measures contribute toward the reduction of stillbirth and related complications as IPTp and ITNs can reduce stillbirths by 22% (59). Antenatal care visits are also important; in areas of high malaria transmission in Malawi, stillbirth was associated with fewer than five antenatal care visits (aOR 3·1 [1·4–7·0]) (60). Nevertheless, these useful tools do not adequately address the MiP problem: in a longitudinal cohort of pregnant women in Mali, malaria was common and increased the risk of stillbirth nearly 4-fold among primigravidae despite widespread use of IPTp-SP (18).
Perinatal death
Perinatal death refers to death of a baby between the 28th week of pregnancy to 7 days after birth, encompassing the outcomes of stillbirth and early neonatal death. Rates of perinatal mortality are overall higher in malaria-endemic countries with an estimated perinatal mortality rate of 34.7 per 1000 births (61). However, actual perinatal mortality rates are believed to be even higher than reported since a large percentage of perinatal deaths are unreported in malaria-endemic countries (62).
Maternal malaria increased the risk of perinatal death and LBW (risk ratio = 12.4), in a study from the Democratic Republic of the Congo (known as Zaire at the time of the study) (63). On the Thai-Myanmar border, falciparum and vivax MiP increase the risk of mortality by 2.55-fold and 1.98-fold, respectively (64). Among pregnant women in rural Malawi, the risk of neonatal death increased as birth weight decreased (65). The risk of LBW is known to be increased by placental malaria infection, thereby highlighting a link between malaria and perinatal mortality (18). Further, in a study with pregnant women in Kenya, impaired uteroplacental blood flow has been found to be predictive of perinatal death (14). Malaria infection in the third trimester was associated with abnormal uterine artery flow velocity waveforms, suggesting impaired uteroplacental blood flow may be related to the pathology of infected maternal erythrocytes in the placenta.
Successful prevention of falciparum infections reduces the risk for perinatal mortality by 27% among primigravidae (66). When it was still effective as an antimalarial, chloroquine prophylaxis was shown to protect against perinatal death (risk ratio = 0.38) (63). While more comprehensive data on perinatal mortality are necessary to accurately determine the impact of preventative measures, new interventions are clearly necessary to reduce the burden of malaria sequelae during pregnancy.
Risk factors for fatal outcomes of neonates and infants
Intrauterine growth restriction (IUGR), PTB, and LBW are all risk factors for death at delivery or in early infancy (Table 1). IUGR, the condition in which a fetus does not grow to normal weight during pregnancy, is a major cause for LBW. High density parasitemia in placental smears has been associated with IUGR in Benin (67). Malaria both in early and late pregnancy is associated with IUGR. Women with early pregnancy malaria have 2.2 times the risk of IUGR measured on multiple ANC visits compared to women without early infection (95% CI: 1.1, 4.2) (68).
Malaria infection also increases the risk of PTB (hazard ratio = 2.41, p = 0.003) (18), the other major contributor to LBW in malaria exposed populations. PTB is related to stillbirth, with around three-quarters of stillbirths born preterm globally (5). MiP is estimated to be responsible for 36% of PTB and 70% of IUGR in areas with stable malaria transmission in Africa (58).
LBW, defined as a birthweight less than 2,500 grams, is a major risk factor for infant mortality. In malaria-endemic regions, an estimated 19% of LBW births are due to malaria, and an estimated 6% of overall infant deaths are due to malaria-induced LBW (69). MiP increases the risk of both LBW and prematurity, and some studies find that the risk is greater when the infection is acquired in early pregnancy (32, 70, 71). Frequency of infection is also a relevant factor as the risk of LBW increases as the number of malaria episodes during pregnancy increases [one episode: prevalence ratio (PR) 1.62 (95% CI 1.07–2.46); two episodes: PR 2.41 (95% CI 1.39–4.18)] (70). A study with pregnant women in Gambia found a four-fold risk of delivering LBW babies if mothers had parasitized placentae (72). Another cohort in Papua New Guinea found that histopathologically diagnosed chronic placental malaria is associated with both LBW and PTB (73).
Preventative measures are effective against adverse pregnancy outcomes. Three or more doses of IPTp-SP was associated with a 66% reduced risk of LBW (74). Further, a regimen of more than three doses IPTp-SP was associated with an improved birth weight compared to fewer doses of treatment. However, treatment with IPTp in the third trimester did not prevent IUGR, stressing the need to protect women from malaria from early in pregnancy (75). Furthermore, consistent antenatal care is of importance as PTB has been associated with <5 antenatal care visits (aOR 2.2, 95% CI 1.3-3.7) (60). Altogether, the evidence suggests measures focused directly on malaria prevention in conjunction with broader health care access are critical to address risk factors for MiP.
Limitations
There are contradicting data in the literature regarding which adverse birth outcomes are significantly related to malaria. This could be due to the lack of consensus on what markers of malaria should serve as surrogates of adverse birth outcomes. A 2020 study found that detection of parasites through placental histopathology was associated with an increased risk of adverse birth outcomes, while parasite detection by microscopy was not (76). The variation in sensitivity of malaria detection methods may contribute to discrepancies in reports of malaria impact on adverse birth outcomes.
Further, there is a lack of first trimester cohort studies in Africa, with most studies of first trimester pregnancies being conducted in Southeast Asia. This is at least partially attributable to the later gestational age at first ANC visit in most African sites, as well as a lack of established data collection sites in the region. Recent efforts to address this include a pregnancy registry established in Mali to provide baseline information on maternal and fetal outcomes in preparation for vaccine trials in pregnant women (44).
Finally, increased ANC monitoring and care (IPTp; bed-nets; clinic visits) during any vaccine or interventional trial in pregnancy will likely reduce the burden of parasitemia and therefore improve pregnancy outcomes. This benefit of study participation will thus reduce the endpoints needed to assess malaria vaccine efficacy, highlighting the need to estimate this effect a priori to ensure sufficient sample size and power for statistical analysis of the clinical benefit of the vaccine. On the other hand, fewer adverse pregnancy outcomes in the control group due to increased care and malaria prevention might facilitate identification of any concerning safety signals specifically related to the vaccine.
Future directions
There have been significant strides in reducing the burden of MiP through the distribution of ITNs, and implementation of IPTp/SP (Figure 2). However, with continued rates of maternal mortality, stillbirth, and other malaria-related sequelae, MiP persists as a perennial problem requiring stronger solutions. In light of growing resistance to current therapeutics, a more sustainable and effective approach to preventing pregnancy malaria is warranted (77). The development of a vaccine targeting MiP has possibly the greatest potential to prevent enduring adverse birth outcomes. By intensifying research on adverse pregnancy outcomes caused by MiP, we can best equip ourselves to confirm the safety and efficacy of vaccines against MiP and accelerate their ultimate deployment to end these preventable health consequences.
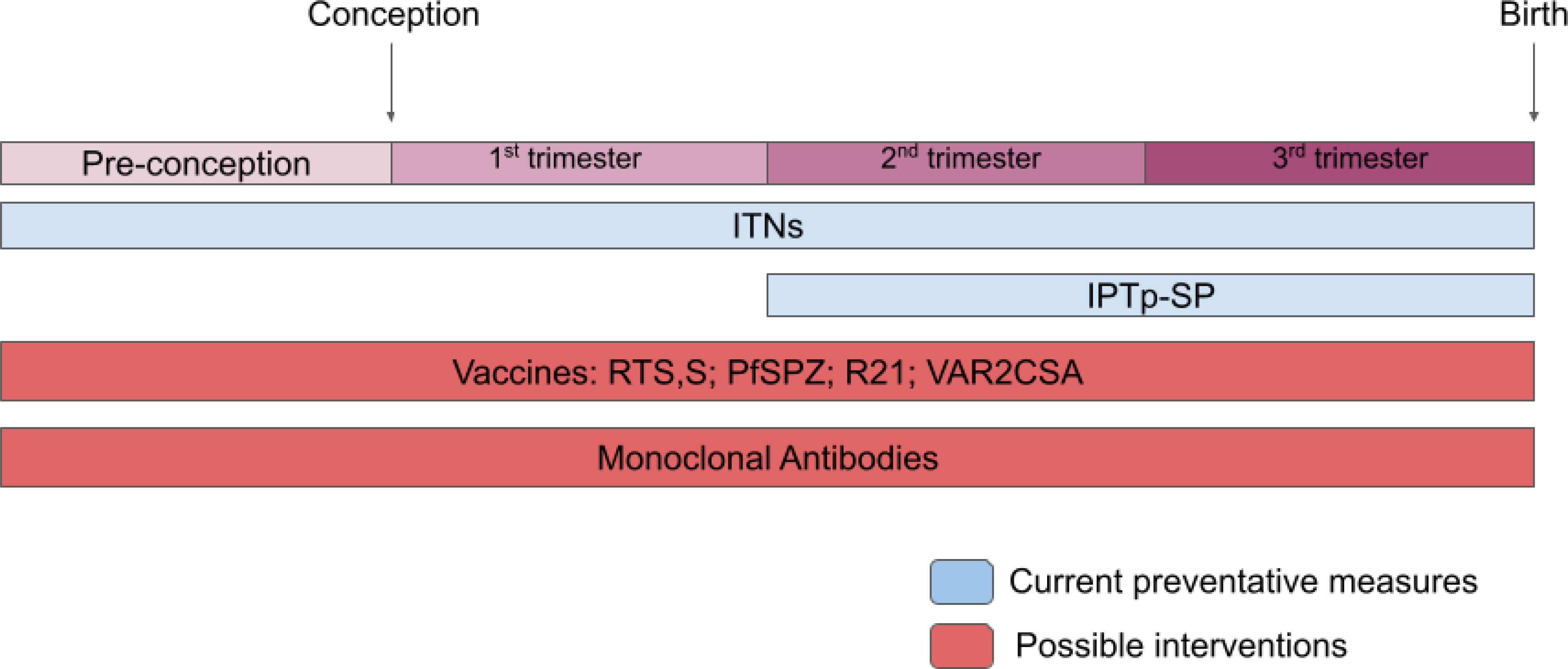
Figure 2 Current and future interventions for MiP. Summary of interventions for MiP from before conception to time of birth. Blue regions represent established interventions and red regions represent possible interventions for the future.
Author contributions
AB and JD wrote the original draft of the manuscript. PD reviewed and edited the manuscript. All authors read and approved the submitted version of the manuscript.
Funding
This work was supported by the Intramural Research Program of the National Institute of Allergy and Infectious Diseases, National Institutes of Health.
Acknowledgments
The authors thank J. Patrick Gorres for editing the manuscript.
Conflict of interest
The authors declare that the research was conducted in the absence of any commercial or financial relationships that could be construed as a potential conflict of interest.
The authors JD and PD declared that they were editorial board members of Frontiers, at the time of submission. This had no impact on the peer review process and the final decision.
Publisher’s note
All claims expressed in this article are solely those of the authors and do not necessarily represent those of their affiliated organizations, or those of the publisher, the editors and the reviewers. Any product that may be evaluated in this article, or claim that may be made by its manufacturer, is not guaranteed or endorsed by the publisher.
References
1. World Malaria Report (2022). Available at: https://www.who.int/teams/global-malaria-programme/reports/world-malaria-report-2022 (Accessed May 26, 2023).
2. Fried M, Duffy PE. Malaria during pregnancy. Cold Spring Harb Perspect Med (2017) 7. doi: 10.1101/cshperspect.a025551
3. Xiong X, Buekens P, Alexander S, Demianczuk N, Wollast E. Anemia during pregnancy and birth outcome: a meta-analysis. Am J Perinatol (2000) 17:137–46. doi: 10.1055/s-2000-9508
4. Taylor SM, Ter Kuile FO. Stillbirths: the hidden burden of malaria in pregnancy. Lancet Glob Health (2017) 5:e1052–3. doi: 10.1016/S2214-109X(17)30378-9
5. Lawn JE, Ohuma EO, Bradley E, Idueta LS, Hazel E, Okwaraji YB, et al. Small babies, big risks: global estimates of prevalence and mortality for vulnerable newborns to accelerate change and improve counting. Lancet (2023) 401:1707–19. doi: 10.1016/S0140-6736(23)00522-6
6. Fried M, Duffy PE. Adherence of Plasmodium falciparum to chondroitin sulfate A in the human placenta. Science (1996) 272:1502–4. doi: 10.1126/science.272.5267.1502
7. Doritchamou JYA, Renn JP, Jenkins B, Mahamar A, Dicko A, Fried M, et al. A single full-length VAR2CSA ectodomain variant purifies broadly neutralizing antibodies against placental malaria isolates. Elife (2022) 11:e76264. doi: 10.7554/eLife.76264
8. Fried M, Nosten F, Brockman A, Brabin BJ, Duffy PE. Maternal antibodies block malaria. Nature (1998) 395:851–2. doi: 10.1038/27570
9. Fried M, Muga RO, Misore AO, Duffy PE. Malaria elicits type 1 cytokines in the human placenta: IFN-gamma and TNF-alpha associated with pregnancy outcomes. J Immunol (1998) 160:2523–30. doi: 10.4049/jimmunol.160.5.2523
10. Wang W, Sung N, Gilman-Sachs A, Kwak-Kim J. T helper (Th) cell profiles in pregnancy and recurrent pregnancy losses: th1/th2/th9/th17/th22/tfh cells. Front Immunol (2020) 11:2025. doi: 10.3389/fimmu.2020.02025
11. Sharma L, Shukla G. Placental malaria: A new insight into the pathophysiology. Front Med (Lausanne) (2017) 4:117. doi: 10.3389/fmed.2017.00117
12. Crocker IP, Tanner OM, Myers JE, Bulmer JN, Walraven G, Baker PN. Syncytiotrophoblast degradation and the pathophysiology of the malaria-infected placenta. Placenta (2004) 25:273–82. doi: 10.1016/j.placenta.2003.09.010
13. Moeller SL, Nyengaard JR, Larsen LG, Nielsen K, Bygbjerg IC, Msemo OA, et al. Malaria in early pregnancy and the development of the placental vasculature. J Infect Dis (2019) 220:1425–34. doi: 10.1093/infdis/jiy735
14. Dorman EK, Shulman CE, Kingdom J, Bulmer JN, Mwendwa J, Peshu N, et al. Impaired uteroplacental blood flow in pregnancies complicated by falciparum malaria. Ultrasound Obstet Gynecol (2002) 19:165–70. doi: 10.1046/j.0960-7692.2001.00545.x
15. Schantz-Dunn J, Nour NM. Malaria and pregnancy: a global health perspective. Rev Obstet Gynecol (2009) 2:186–92. doi: 10.3909/riog0091
16. Minja DTR, Schmiegelow C, Oesterholt M, Magistrado PA, Boström S, John D, et al. Reliability of rapid diagnostic tests in diagnosing pregnancy-associated malaria in north-eastern Tanzania. Malar J (2012) 11:211. doi: 10.1186/1475-2875-11-211
17. Unger HW, Rosanas-Urgell A, Robinson LJ, Ome-Kaius M, Jally S, Umbers AJ, et al. Microscopic and submicroscopic Plasmodium falciparum infection, maternal anaemia and adverse pregnancy outcomes in Papua New Guinea: a cohort study. Malar J (2019) 18:302. doi: 10.1186/s12936-019-2931-7
18. Mahamar A, Andemel N, Swihart B, Sidibe Y, Gaoussou S, Barry A, et al. Malaria infection is common and associated with perinatal mortality and preterm delivery despite widespread use of chemoprevention in mali: an observational study 2010 to 2014. Clin Infect Dis (2021) 73:1355–61. doi: 10.1093/cid/ciab301
19. Malaria Vector Control. Available at: https://www.who.int/teams/global-malaria-programme/prevention/vector-control (Accessed May 26, 2023).
20. Bouwman H, Kylin H. Malaria control insecticide residues in breast milk: the need to consider infant health risks. Environ Health Perspect (2009) 117:1477–80. doi: 10.1289/ehp.0900605
21. Eskenazi B, An S, Rauch SA, Coker ES, Maphula A, Obida M, et al. Prenatal exposure to DDT and pyrethroids for malaria control and child neurodevelopment: the VHEMBE cohort, South Africa. Environ Health Perspect (2018) 126:047004. doi: 10.1289/EHP2129
22. Hammond A, Galizi R, Kyrou K, Simoni A, Siniscalchi C, Katsanos D, et al. A CRISPR-Cas9 gene drive system targeting female reproduction in the malaria mosquito vector Anopheles gambiae. Nat Biotechnol (2016) 34:78–83. doi: 10.1038/nbt.3439
23. WHO Policy Brief for the Implementation of Intermittent Preventive Treatment of Malaria in Pregnancy Using Sulfadoxine-Pyrimethamine (IPTp-SP). Available at: https://www.who.int/publications-detail-redirect/WHO-HTM-GMP-2014.4 (Accessed May 26, 2023).
24. Kayentao K, Garner P, van Eijk AM, Naidoo I, Roper C, Mulokozi A, et al. Intermittent preventive therapy for malaria during pregnancy using 2 vs 3 or more doses of sulfadoxine-pyrimethamine and risk of low birth weight in Africa: systematic review and meta-analysis. JAMA (2013) 309:594–604. doi: 10.1001/jama.2012.216231
25. Peters PJ, Thigpen MC, Parise ME, Newman RD. Safety and toxicity of sulfadoxine/pyrimethamine. Drug-Safety (2007) 30:481–501. doi: 10.2165/00002018-200730060-00003
26. González R, Mombo-Ngoma G, Ouédraogo S, Kakolwa MA, Abdulla S, Accrombessi M, et al. Intermittent preventive treatment of malaria in pregnancy with mefloquine in HIV-negative women: a multicentre randomized controlled trial. PloS Med (2014) 11:e1001733. doi: 10.1371/journal.pmed.1001733
27. González R, Nhampossa T, Mombo-Ngoma G, Mischlinger J, Esen M, Tchouatieu A-M, et al. Evaluation of the safety and efficacy of dihydroartemisinin-piperaquine for intermittent preventive treatment of malaria in HIV-infected pregnant women: protocol of a multicentre, two-arm, randomised, placebo-controlled, superiority clinical trial (MAMAH project). BMJ Open (2021) 11:e053197. doi: 10.1136/bmjopen-2021-053197
28. Madanitsa M, Barsosio HC, Minja DTR, Mtove G, Kavishe RA, Dodd J, et al. Effect of monthly intermittent preventive treatment with dihydroartemisinin-piperaquine with and without azithromycin versus monthly sulfadoxine-pyrimethamine on adverse pregnancy outcomes in Africa: a double-blind randomised, partly placebo-controlled trial. Lancet (2023) 401:1020–36. doi: 10.1016/S0140-6736(22)02535-1
29. Huynh B-T, Cottrell G, Cot M, Briand V. Burden of malaria in early pregnancy: a neglected problem? Clin Infect Dis (2015) 60:598–604. doi: 10.1093/cid/ciu848
30. Pell C, Meñaca A, Were F, Afrah NA, Chatio S, Manda-Taylor L, et al. Factors affecting antenatal care attendance: results from qualitative studies in Ghana, Kenya and Malawi. PloS One (2013) 8:e53747. doi: 10.1371/journal.pone.0053747
31. Doritchamou J, Bertin G, Moussiliou A, Bigey P, Viwami F, Ezinmegnon S, et al. First-trimester Plasmodium falciparum infections display a typical “placental” phenotype. J Infect Dis (2012) 206:1911–9. doi: 10.1093/infdis/jis629
32. Huynh B-T, Fievet N, Gbaguidi G, Dechavanne S, Borgella S, Guezo-Mevo B, et al. Influence of the timing of malaria infection during pregnancy on birth weight and on maternal anemia in Benin. Am J Trop Med Hygiene (2011) 85:214–20. doi: 10.4269/ajtmh.2011.11-0103
33. Valea I, Tinto H, Drabo MK, Huybregts L, Sorgho H, Ouedraogo J-B, et al. An analysis of timing and frequency of malaria infection during pregnancy in relation to the risk of low birth weight, anaemia and perinatal mortality in Burkina Faso. Malar J (2012) 11:71. doi: 10.1186/1475-2875-11-71
34. Burger RJ, van Eijk AM, Bussink M, Hill J, Ter Kuile FO. Artemisinin-based combination therapy versus quinine or other combinations for treatment of uncomplicated plasmodium falciparum malaria in the second and third trimester of pregnancy: A systematic review and meta-analysis. Open Forum Infect Dis (2016) 3:ofv170. doi: 10.1093/ofid/ofv170
35. Kovacs SD, van Eijk AM, Sevene E, Dellicour S, Weiss NS, Emerson S, et al. The safety of artemisinin derivatives for the treatment of malaria in the 2nd or 3rd trimester of pregnancy: A systematic review and meta-analysis. PloS One (2016) 11:e0164963. doi: 10.1371/journal.pone.0164963
36. Dellicour S, Sevene E, McGready R, Tinto H, Mosha D, Manyando C, et al. First-trimester artemisinin derivatives and quinine treatments and the risk of adverse pregnancy outcomes in Africa and Asia: A meta-analysis of observational studies. PloS Med (2017) 14:e1002290. doi: 10.1371/journal.pmed.1002290
37. Ndam NT, Denoeud-Ndam L, Doritchamou J, Viwami F, Salanti A, Nielsen MA, et al. Protective Antibodies against Placental Malaria and Poor Outcomes during Pregnancy, Benin. Emerging Infect Dis (2015) 21:813–23. doi: 10.3201/eid2105.141626
38. McLean ARD, Opi DH, Stanisic DI, Cutts JC, Feng G, Ura A, et al. High antibodies to VAR2CSA in response to malaria infection are associated with improved birthweight in a longitudinal study of pregnant women. Front Immunol (2021) 12:644563. doi: 10.3389/fimmu.2021.644563
39. Mordmüller B, Sulyok M, Egger-Adam D, Resende M, de Jongh WA, Jensen MH, et al. First-in-human, randomized, double-blind clinical trial of differentially adjuvanted PAMVAC, A vaccine candidate to prevent pregnancy-associated malaria. Clin Infect Dis (2019) 69:1509–16. doi: 10.1093/cid/ciy1140
40. Sirima SB, Richert L, Chêne A, Konate AT, Campion C, Dechavanne S, et al. PRIMVAC vaccine adjuvanted with Alhydrogel or GLA-SE to prevent placental malaria: a first-in-human, randomised, double-blind, placebo-controlled study. Lancet Infect Dis (2020) 20:585–97. doi: 10.1016/S1473-3099(19)30739-X
41. Doritchamou JYA, Suurbaar J, Ndam NT. Progress and new horizons toward a VAR2CSA-based placental malaria vaccine. Expert Rev Vaccines (2021) 20:215–26. doi: 10.1080/14760584.2021.1878029
42. Sissoko MS, Healy SA, Katile A, Omaswa F, Zaidi I, Gabriel EE, et al. Safety and efficacy of PfSPZ Vaccine against Plasmodium falciparum via direct venous inoculation in healthy malaria-exposed adults in Mali: a randomised, double-blind phase 1 trial. Lancet Infect Dis (2017) 17:498–509. doi: 10.1016/S1473-3099(17)30104-4
43. Sissoko MS, Healy SA, Katile A, Zaidi I, Hu Z, Kamate B, et al. Safety and efficacy of a three-dose regimen of Plasmodium falciparum sporozoite vaccine in adults during an intense malaria transmission season in Mali: a randomised, controlled phase 1 trial. Lancet Infect Dis (2022) 22:377–89. doi: 10.1016/S1473-3099(21)00332-7
44. Healy SA, Fried M, Richie T, Bok K, Little M, August A, et al. Malaria vaccine trials in pregnant women: An imperative without precedent. Vaccine (2019) 37:763–70. doi: 10.1016/j.vaccine.2018.12.025
45. Laurens MB. RTS,S/AS01 vaccine (MosquirixTM): an overview. Hum Vaccin Immunother (2020) 16:480–9. doi: 10.1080/21645515.2019.1669415
46. WHO Recommends Groundbreaking Malaria Vaccine for Children at Risk. Available at: https://www.who.int/news/item/06-10-2021-who-recommends-groundbreaking-malaria-vaccine-for-children-at-risk (Accessed May 26, 2023).
47. Davies L. Ghana is first country to approve Oxford malaria vaccine (2023). The Guardian. Available at: https://www.theguardian.com/global-development/2023/apr/13/ghana-is-first-country-to-approve-oxford-r21-malaria-vaccine (Accessed May 26, 2023).
48. Erezi D. Nigeria approves Oxford malaria vaccine, to take immunisation action (2023). The Guardian Nigeria News - Nigeria and World News. Available at: https://guardian.ng/news/nigeria-approves-oxford-malaria-vaccine-to-action-immunisation/ (Accessed May 26, 2023).
49. Kayentao K, Ongoiba A, Preston AC, Healy SA, Doumbo S, Doumtabe D, et al. Safety and efficacy of a monoclonal antibody against malaria in Mali. N Engl J Med (2022) 387:1833–42. doi: 10.1056/NEJMoa2206966
50. Gaoussou S, Attaher O, Swihart B, Traore M, Diarra S, Soumbounou IH, et al. Pregnancy outcomes in a malaria-exposed Malian cohort of women of child-bearing age. Front Med (Lausanne) (2022) 9:1061538. doi: 10.3389/fmed.2022.1061538
51. The Lancet null. Miscarriage: worldwide reform of care is needed. Lancet (2021) 397:1597. doi: 10.1016/S0140-6736(21)00954-5
52. Moore KA, Simpson JA, Paw MK, Pimanpanarak M, Wiladphaingern J, Rijken MJ, et al. Safety of artemisinins in first trimester of prospectively followed pregnancies: an observational study. Lancet Infect Dis (2016) 16:576–83. doi: 10.1016/S1473-3099(15)00547-2
53. McGready R, Lee SJ, Wiladphaingern J, Ashley EA, Rijken MJ, Boel M, et al. Adverse effects of falciparum and vivax malaria and the safety of antimalarial treatment in early pregnancy: a population-based study. Lancet Infect Dis (2012) 12:388–96. doi: 10.1016/S1473-3099(11)70339-5
54. Lawn JE, Blencowe H, Waiswa P, Amouzou A, Mathers C, Hogan D, et al. Stillbirths: rates, risk factors, and acceleration towards 2030. Lancet (2016) 387:587–603. doi: 10.1016/S0140-6736(15)00837-5
55. Moore KA, Simpson JA, Scoullar MJL, McGready R, Fowkes FJI. Quantification of the association between malaria in pregnancy and stillbirth: a systematic review and meta-analysis. Lancet Glob Health (2017) 5:e1101–12. doi: 10.1016/S2214-109X(17)30340-6
56. Watson-Jones D, Weiss HA, Changalucha JM, Todd J, Gumodoka B, Bulmer J, et al. Adverse birth outcomes in United Republic of Tanzania–impact and prevention of maternal risk factors. Bull World Health Organ (2007) 85:9–18. doi: 10.2471/blt.06.033258
57. Yatich NJ, Funkhouser E, Ehiri JE, Agbenyega T, Stiles JK, Rayner JC, et al. Malaria, intestinal helminths and other risk factors for stillbirth in Ghana. Infect Dis Obstet Gynecol (2010) 2010:350763. doi: 10.1155/2010/350763
58. De Beaudrap P, Turyakira E, White LJ, Nabasumba C, Tumwebaze B, Muehlenbachs A, et al. Impact of malaria during pregnancy on pregnancy outcomes in a Ugandan prospective cohort with intensive malaria screening and prompt treatment. Malar J (2013) 12:139. doi: 10.1186/1475-2875-12-139
59. Ishaque S, Yakoob MY, Imdad A, Goldenberg RL, Eisele TP, Bhutta ZA. Effectiveness of interventions to screen and manage infections during pregnancy on reducing stillbirths: a review. BMC Public Health (2011) 11(Suppl 3):S3. doi: 10.1186/1471-2458-11-S3-S3
60. Kalanda BF, Verhoeff FH, Chimsuku L, Harper G, Brabin BJ. Adverse birth outcomes in a malarious area. Epidemiol Infect (2006) 134:659–66. doi: 10.1017/S0950268805005285
61. Breman JG, Alilio MS, Mills A. Conquering the intolerable burden of malaria: what’s new, what’s needed: a summary. Am J Trop Med Hyg (2004) 71:1–15. doi: 10.4269/ajtmh.2004.71.2_suppl.0700001
62. van Geertruyden J-P, Thomas F, Erhart A, D’Alessandro U. The contribution of malaria in pregnancy to perinatal mortality. Am J Trop Med Hyg (2004) 71:35–40. doi: 10.4269/ajtmh.2004.71.35
63. Nyirjesy P, Kavasya T, Axelrod P, Fischer PR. Malaria during pregnancy: neonatal morbidity and mortality and the efficacy of chloroquine chemoprophylaxis. Clin Infect Dis (1993) 16:127–32. doi: 10.1093/clinids/16.1.127
64. Moore KA, Fowkes FJI, Wiladphaingern J, Wai NS, Paw MK, Pimanpanarak M, et al. Mediation of the effect of malaria in pregnancy on stillbirth and neonatal death in an area of low transmission: observational data analysis. BMC Med (2017) 15:98. doi: 10.1186/s12916-017-0863-z
65. McDermott JM, Wirima JJ, Steketee RW, Breman JG, Heymann DL. The effect of placental malaria infection on perinatal mortality in rural Malawi. Am J Trop Med Hyg (1996) 55:61–5. doi: 10.4269/ajtmh.1996.55.61
66. Desai M, ter Kuile FO, Nosten F, McGready R, Asamoa K, Brabin B, et al. Epidemiology and burden of malaria in pregnancy. Lancet Infect Dis (2007) 7:93–104. doi: 10.1016/S1473-3099(07)70021-X
67. Ibhanesebhor SE, Okolo AA. Placental malaria and pregnancy outcome. Int J Gynaecol Obstet (1992) 37:247–52. doi: 10.1016/0020-7292(92)90324-c
68. Griffin JB, Lokomba V, Landis SH, Thorp JM, Herring AH, Tshefu AK, et al. Plasmodium falciparum parasitaemia in the first half of pregnancy, uterine and umbilical artery blood flow, and foetal growth: a longitudinal Doppler ultrasound study. Malar J (2012) 11:319. doi: 10.1186/1475-2875-11-319
69. Guyatt HL, Snow RW. Impact of malaria during pregnancy on low birth weight in sub-Saharan Africa. Clin Microbiol Rev (2004) 17:760–9. doi: 10.1128/CMR.17.4.760-769.2004
70. Kalilani L, Mofolo I, Chaponda M, Rogerson SJ, Meshnick SR. The effect of timing and frequency of Plasmodium falciparum infection during pregnancy on the risk of low birth weight and maternal anemia. Trans R Soc Trop Med Hyg (2010) 104:416–22. doi: 10.1016/j.trstmh.2010.01.013
71. Taha Te-T, Gray RH. Malaria and perinatal mortality in central Sudan. Am J Epidemiol (1993) 138:563–8. doi: 10.1093/oxfordjournals.aje.a116896
72. Okoko BJ, Ota MO, Yamuah LK, Idiong D, Mkpanam SN, Avieka A, et al. Influence of placental malaria infection on foetal outcome in the Gambia: twenty years after Ian Mcgregor. J Health Popul Nutr (2002) 20:4–11.
73. Stanisic DI, Moore KA, Baiwog F, Ura A, Clapham C, King CL, et al. Risk factors for malaria and adverse birth outcomes in a prospective cohort of pregnant women resident in a high malaria transmission area of Papua New Guinea. Trans R Soc Trop Med Hyg (2015) 109:313–24. doi: 10.1093/trstmh/trv019
74. Mlugu EM, Minzi O, Asghar M, Färnert A, Kamuhabwa AAR, Aklillu E. Effectiveness of sulfadoxine-pyrimethamine for intermittent preventive treatment of malaria and adverse birth outcomes in pregnant women. Pathogens (2020) 9:207. doi: 10.3390/pathogens9030207
75. Briand V, Saal J, Ghafari C, Huynh B-T, Fievet N, Schmiegelow C, et al. Fetal growth restriction is associated with malaria in pregnancy: A prospective longitudinal study in Benin. J Infect Dis (2016) 214:417–25. doi: 10.1093/infdis/jiw158
76. Ategeka J, Kakuru A, Kajubi R, Wasswa R, Ochokoru H, Arinaitwe E, et al. Relationships between measures of malaria at delivery and adverse birth outcomes in a high-transmission area of Uganda. J Infect Dis (2020) 222:863–70. doi: 10.1093/infdis/jiaa156
Keywords: malaria, pregnancy, vaccine, perinatal death, pre-term birth (PTB), miscarriage, stillbirth, low birth weight (LBW)
Citation: Berhe AD, Doritchamou JYA and Duffy PE (2023) Malaria in pregnancy: adverse pregnancy outcomes and the future of prevention. Front. Trop. Dis 4:1229735. doi: 10.3389/fitd.2023.1229735
Received: 26 May 2023; Accepted: 12 July 2023;
Published: 14 August 2023.
Edited by:
Tais Nobrega De Sousa, Oswaldo Cruz Foundation (Fiocruz), BrazilReviewed by:
Ghyslain Mombo-Ngoma, Centre de Recherche Médicales de Lambaréné, GabonFlor Martinez Espinosa, Oswaldo Cruz Foundation, Brazil
Copyright © 2023 Berhe, Doritchamou and Duffy. This is an open-access article distributed under the terms of the Creative Commons Attribution License (CC BY). The use, distribution or reproduction in other forums is permitted, provided the original author(s) and the copyright owner(s) are credited and that the original publication in this journal is cited, in accordance with accepted academic practice. No use, distribution or reproduction is permitted which does not comply with these terms.
*Correspondence: Patrick E. Duffy, cGF0cmljay5kdWZmeUBuaWguZ292; eWFpLmRvcml0Y2hhbW91QG5paC5nb3Y=