- 1Instituto de Estudos do Xingu (IEX), Universidade Federal do Sul e Sudeste do Pará (UNIFESSPA), Unit of São Félix do Xingu, São Félix do Xingu, Brazil
- 2Laboratório de Evolução, Instituto de Estudos Costeiros (IECOS), Universidade Federal do Pará (UFPA), Bragança, Brazil
- 3Laboratório de Genética e Biotecnologia, Curso de Bacharelado em Ciências Biológicas, Universidade Federal da Amazônia (UFRA), Capanema, Brazil
- 4Grupo de Investigação Biológica Integrada (GIBI), Centro de Estudos Avançados da Biodiversidade (CEABIO), Universidade Federal do Pará, Belém, Brazil
- 5Laboratório de Genética e Biologia Molecular, Centro de Ciências Exatas Naturais e Tecnológicas (CCENT), Universidade Estadual da Região Tocantina do Maranhão (UEMASUL), Imperatriz, Brazil
- 6Laboratório de Genética e Biologia Molecular, Instituto de Estudos Costeiros (IECOS), Universidade Federal do Pará (UFPA), Bragança, Brazil
The Serra Spanish Mackerel, Scomberomorus brasiliensis is one of the principal fishery resources found in the tropical and subtropical waters of the western South Atlantic. Declining catches of S. brasiliensis off the coast of northeastern Brazil indicate that this species is being overfished. Despite the importance of this species to local fisheries, few data are available on its genetic diversity or population parameters. Given this scenario, the present study evaluated the genetic variability of S. brasiliensis off the coast of the western South Atlantic, based on mitochondrial genes. We compiled two databases, one with partial sequences of the MT-CYB gene (N = 105), and the other of the MT-ND4 gene of the Serra Spanish Mackerel, sampled from eight localities, ranging from Cumana in Venezuela to Paranaguá, in southern Brazil. The results of the analysis indicate low levels of genetic diversity in the study. Samples from Cumana, on the coast of Venezuela were the most differentiated, although the results of the AMOVA were not significant. The PhiST values, the genetic divergence, and the haplotype network all indicate the widespread sharing of haplotypes by the mackerel from all the different localities. Analyses of the historical demography, based on both markers, indicate the occurrence of past population expansion, coinciding with fluctuations in sea level that occurred 10,000 years ago. The demographic and phylogeographic analyses of the mitochondrial DNA revealed the existence of a single genetic stock of S. brasiliensis on the coast of the western South Atlantic according to. These findings are fundamental to the development of effective conservation strategies.
Introduction
The Serra Spanish mackerel, Scomberomorus brasiliensis (Collette et al., 1978), is a pelagic marine fish distributed in the Caribbean Sea and western South Atlantic, between southern Belize and the extreme south of Brazil, in Rio Grande do Sul (Collette and Russo, 1984). This mackerel is common near islands and open beaches and appears to be able to migrate long distances on a seasonal basis (Collette and Russo, 1984; Gold et al., 2010). The diet of S. brasiliensis is omnivorous, and includes a range of fish, crustaceans, and mollusks (Fonteles-Filho, 1988). Reproduction is oviparous, with pelagic eggs and larvae (Gesteira and Mesquita, 1976).
The Serra Spanish mackerel is one of the most important fishery resources in the western Atlantic. In Brazil alone, between 2009 and 2011, the annual catch of this fishery resource was approximately 9,500 t (Maia et al., 2015). In Brazil, 2,733,000 tons of S. brasiliensis were caught between 1970 and 2000 (FAO, 2000), with the state of Ceará contributing 40.7% of the total catch. The Brazilian bulletin of fishery and agricultural statistics reported a mean annual catch of 433,000 tons between 2009 and 2011 (MPA, 2011).
Nóbrega and Lessa (2009) found evidence of the overfishing of S. brasiliensis off the coast of the Brazilian Northeast, observing declining catches of this species and its congener, the King mackerel, Somberomorus cavalla (Gonçalves et al., 2003; Nóbrega and Lessa, 2009). In this case, the analysis of phylogeographic patterns and the genetic diversity of commercially exploited organisms is essential for the development of adequate conservation and management policies for these species (Oliveira et al., 2009, 2014; Reiss et al., 2009; Silva et al., 2015). There are a few studies of the genetic diversity of fish species exploited commercially in Brazil (Santa Brígida et al., 2007; Rodrigues et al., 2008; Silva et al., 2015) although none has contemplated S. brasiliensis. Using microsatellite markers, Gold et al. (2010) found evidence of structuring in the S. brasiliensis populations between the coast of Venezuela (Margarita Island and Cumana) and Trinidad and Tobago, which was corroborated by mitochondrial sequences. Up until now, however, there has been no molecular investigation of the Brazilian populations of S. brasiliensis, so it is not known whether these stocks are genetically distinct from those of the Caribbean region, or whether the populations of the western South Atlantic are genetically contiguous.
The present study evaluated in detail the phylogeographic patterns, population dynamics, and genetic diversity of S. brasiliensis on the western coast of the South Atlantic, based on the sequences of two distinct regions of the mitochondrial genome (including previously published data) – MT-CYB and MT-ND4 and compared the diversity indices with those available for the individuals from Brazil, Venezuela, and Trinidad and Tobago.
Materials and Methods
Ethics Statement
All Brazilian samples of S. brasiliensis were obtained directly from local fish markets or other retail outlets. The species is not subject to any legal restrictions in Brazil, according to the Brazilian Environment Institute (IBAMA, 2006).
Sampling and DNA Procedures
The S. brasiliensis specimens were obtained from three different locations on the Brazilian coast, that is, the municipalities of Macapá (Amapá state; N = 76) and Fortaleza (Ceará state; N = 12), and the port of Paranaguá (Paraná state; N = 17) (Figure 1A). The total DNA was extracted from the samples of muscle tissue using the standard phenol chloroform method, followed by precipitation with sodium acetate (Sambrook et al., 2001).
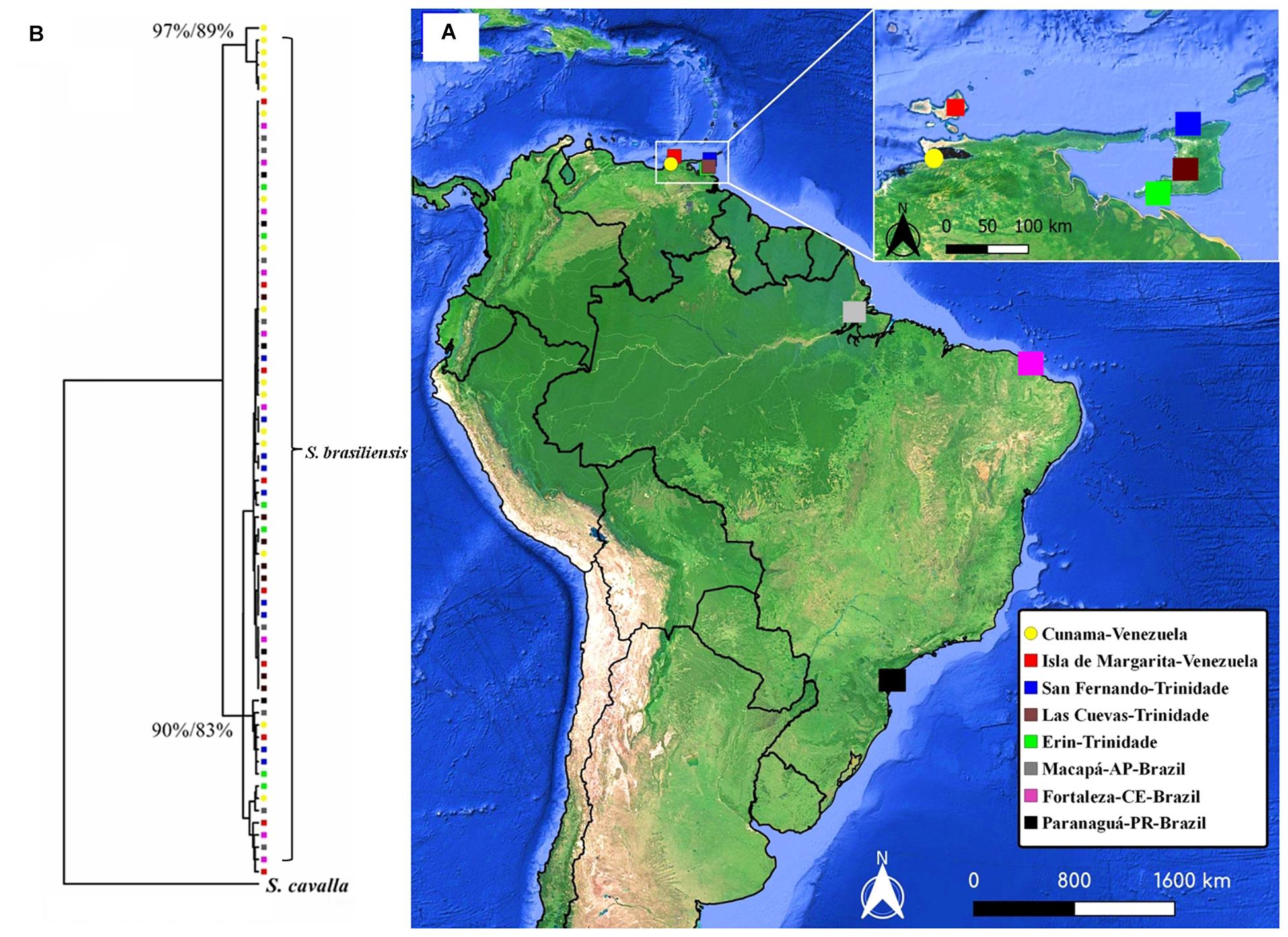
Figure 1. (A) Map of South America showing the eight sites from which the specimens of S. brasiliensis analyzed in the present study were obtained: 1- Cumana; 2- Isla de Margarita; 3- San Fernando; 4- Las Cuevas; 5- Erin; 6- Macapá; 7- Fortaleza and 8- Paranaguá. (B) Neighbor-Joining and Maximum Likelihood trees for the Scomberomorus brasiliensis haplotypes of the MT-ND4 gene. Each haplotype is represented by a different color, yellow circles = 1- Cumana, red squares= 2- Isla de Margarita, blue = 3- San Fernando, brown = 4- Las Cuevas, green = 5- Erin, gray = 6- Macapá, pink = 7- Fortaleza and black = 8- Paranaguá.
The primers used to amplify the MT-CYB (Cytochrome b) gene were TRNA-GluF (5′–CTYTAACCAGGACTAATGGCTTG–3′) and TRNA-ThrR (5′–CCTCCGACGTCCGGYTTACAAG–3′), which were designed specifically for the present study using FastPCR software (Kalendar et al., 2017). The PCR for the MT-CYB gene was run in a total volume of 10 μl containing 1.5 μl of the DNA (10–20 ng),1 μl of 10× buffer (InvitrogenTM; Tris-HCl, KCl, MgCl2, pH 7.8), 1.25 μl of MgCl2, 0.6 μl mix of dNTP; 0.1 μl of Taq polymerase, 0.5 μl of each primer (10 pmol/ μl), and 5.05 μl of sterile bidistilled water. The reactions were run in a Gene Amp PCR System 9700 thermocycler (Applied Biosystems), with a cycling protocol of 94°C for 3 min, followed by 30 cycles of 1 min at 94°C for denaturation and 1 min at 59°C for annealing, followed by 1 min extension at 72°C, with a final extension of 10 min at 72°C.
The primers used for the amplification of the MT-ND4 (Dehydrogenase subunit 4) gene were SbrND4F-(5′-CCACACTTATGCTCGTCC-3′) and SbrND4R-(5′-GCTTTGGGAAGTCATAGGT-3′) (Gold et al., 2010). In this case, the PCR protocol was also that described by Gold et al. (2010). The PCR products were purified using the ExoSAP-IT kit (Amersham Pharmacia Biotech) and sequenced using Big Dye 3.1 in an ABI 3100 automatic DNA sequencer, following the manufacturer’s instructions, and a GeneAmp® PCR System 9700 thermal cycler (Applied Biosystems, Foster City, CA, United States).
Two datasets were compiled for the present study. We obtained sequences of the MT-CTB fragment of 105 S. brasiliensis specimens. We generated 60 nucleotide sequences of the MT-ND4 fragment (Figure 1A), which were added to the 128 sequences published by Gold et al. (2010), which were obtained from GenBank (Supplementary Materials 1, 2).
Molecular Methods
The sequences were aligned using CLUSTAL W (Thompson et al., 1994), implemented in BioEdit V.7.0.4 (Hall, 1999). The phylogenetic relationships were analyzed in PAUP∗ 4.0b10 (Swofford, 2002) using the Neighbor-Joining (NJ) and Maximum Likelihood (ML) approaches. The nodes were estimated using 1,000 bootstrap pseudo-replicates (Felsenstein, 1985) and heuristic searches. The sister species Scomberomorus cavalla was used as the outgroup (Supplementary Material 1). The ML tree generated here was also used to construct the haplotype network in the Haploviewer program (Salzburger et al., 2011). The most adequate evolutionary models (TIM1 + I for MT-ND4 and HKY for MT-CYB) for the data were selected using jModelTest 2 (Darriba et al., 2012), based on the Akaike Information Criterion (AIC). We used the ARLEQUIN 3.5 software (Excoffier and Lischer, 2010) to estimate the haplotype (h; Nei, 1987) and nucleotide diversity (π; Nei, 1987) of each population and the neutrality tests. We calculated Tajima’s D (Tajima, 1989) and Fu’s Fs (Fu, 1997), which are the most sensitive indices for the detection of deviations from neutrality due to recent population expansion or bottlenecks. The genetic differentiation between pairs of populations was estimated using the Fst fixation index (Excoffier et al., 1992), with its significance being tested by 10,000 permutations. The genetic variability among populations was tested using a hierarchical Analysis of Molecular Variance, AMOVA (Excoffier et al., 1992) run in Arlequin v.3.5.
A Bayesian Skyline Plot (BSP) was run in BEAST2 v2.0.3 (Bouckaert et al., 2014) for 200 million generations, with samples taken at intervals of 10,000 generations, using the default parameters and priors (HKY substitution model) for both markers (MT-CYB and MT-ND4), with the strict molecular clock model, tree prior and 10% of which were discarded as burn-in. The convergence and mixing of the chains were inspected visually in TRACER v1.6 (Rambaut et al., 2014). The convergence and mixing were appropriate when all of the Effective sample size (ESS) values for each of the parameters analyzed were above 200. For the MT-CYB gene with three groups and the piecewise-constant skyline model, auto-optimize operators, and log parameters every 10,000 interactions. For the MT-ND4, the plot had seven groups and a piecewise-constant skyline model, auto-optimize operators, and log parameters every 10,000 interactions. For this, two simulations were run, one based on a strict molecular clock, calibrated by the mutation rate, and the other with a relaxed, log-normal non-correlated clock. The results of the two analyses (strict and relaxed clocks) were compared using the Bayes factor, run in TRACER v1.6 (Rambaut et al., 2014). The strict model was the most adequate for our data (lnBayes Factor = 3.72) (Suchard et al., 2001), using a substitution rate of 1% per million years (Donaldson and Wilson, 1999; Craig et al., 2006), with a standard deviation of 10% for the two genes separately, MT-ND4 and MT-CYB.
Results
We obtained 105 MT-CYB sequences with total length of 410 bps from the S. brasiliensis specimens representing three Brazilian populations. Overall, 19 of the nucleotides were variable, and six sites were parsimonious. A total of 19 haplotypes were detected, including 11 singletons and eight shared haplotypes, one of which was very common, being shared by 54 (51%) of the specimens and all of the Brazilian populations analyzed (Figure 2A and Supplementary Material 2).
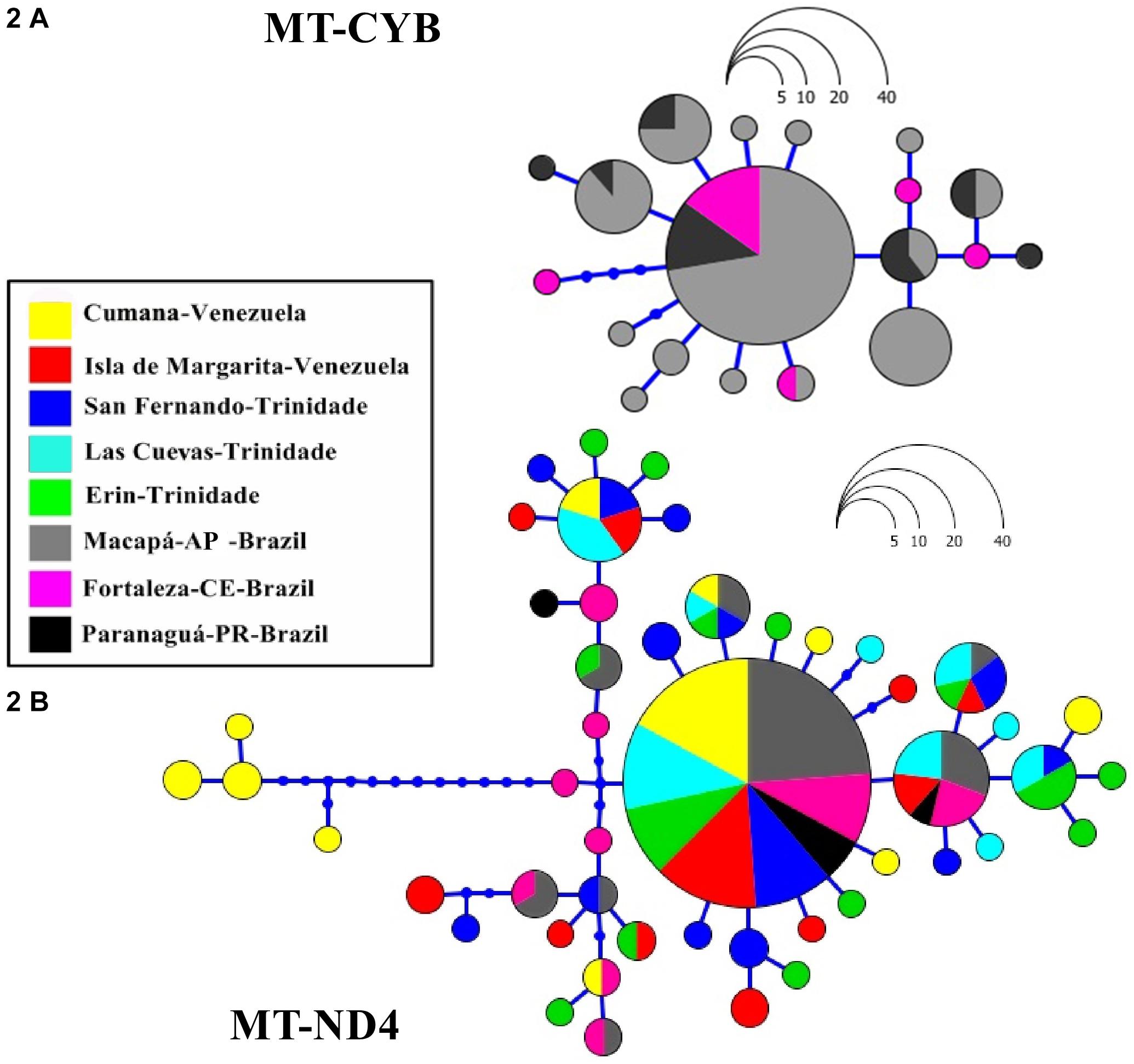
Figure 2. Haplotype genealogies derived from the Maximum Likelihood analysis of the mitochondrial MT-CYB (A) and MT-ND4 (B) genes of Scomberomorus brasiliensis, run in the Haploviewer software, based on the HKY model. Each locality is represented by a different color, and each circle denotes a specific haplotype, the frequency of which is proportional to the scale shown. The length of the branches are also proportional to the number of mutations that separate the respective haplotypes.
The three S. brasiliensis populations presented haplotype diversity (Hd) of between 0.701 and 0.808, while nucleotide diversity (π) ranged from 0.004 to 0.002 (Table 1). Only the total population and that from Macapá presented significantly negative deviations from neutrality, whereas the other populations returned negative, but non-significant values (Table 1). Divergence (P) varied between 0.0 and 1.5%, with a mean population divergence of 1%. Values for intra- and inter-population divergence were all below 1%. According to the PhiST values, none of the comparisons between populations were significant (Table 2). This is corroborated by the AMOVA, which indicated no significant structural arrangement. The overall analysis revealed that almost 99% of the observed variance was derived from within-population differentiation (Table 2).
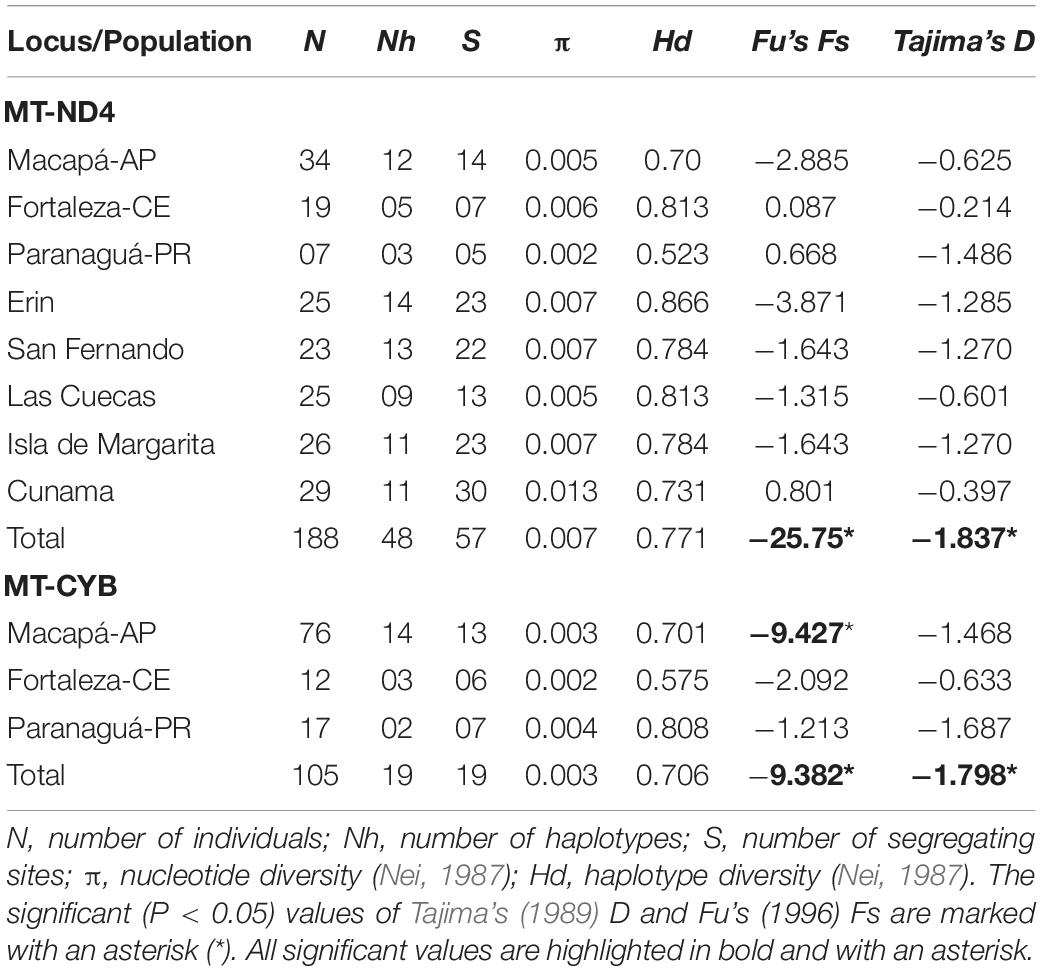
Table 1. Genetic diversity and the results of the neutrality tests for the different Scomberomorus brasiliensis localities, based on two mitochondrial genes.
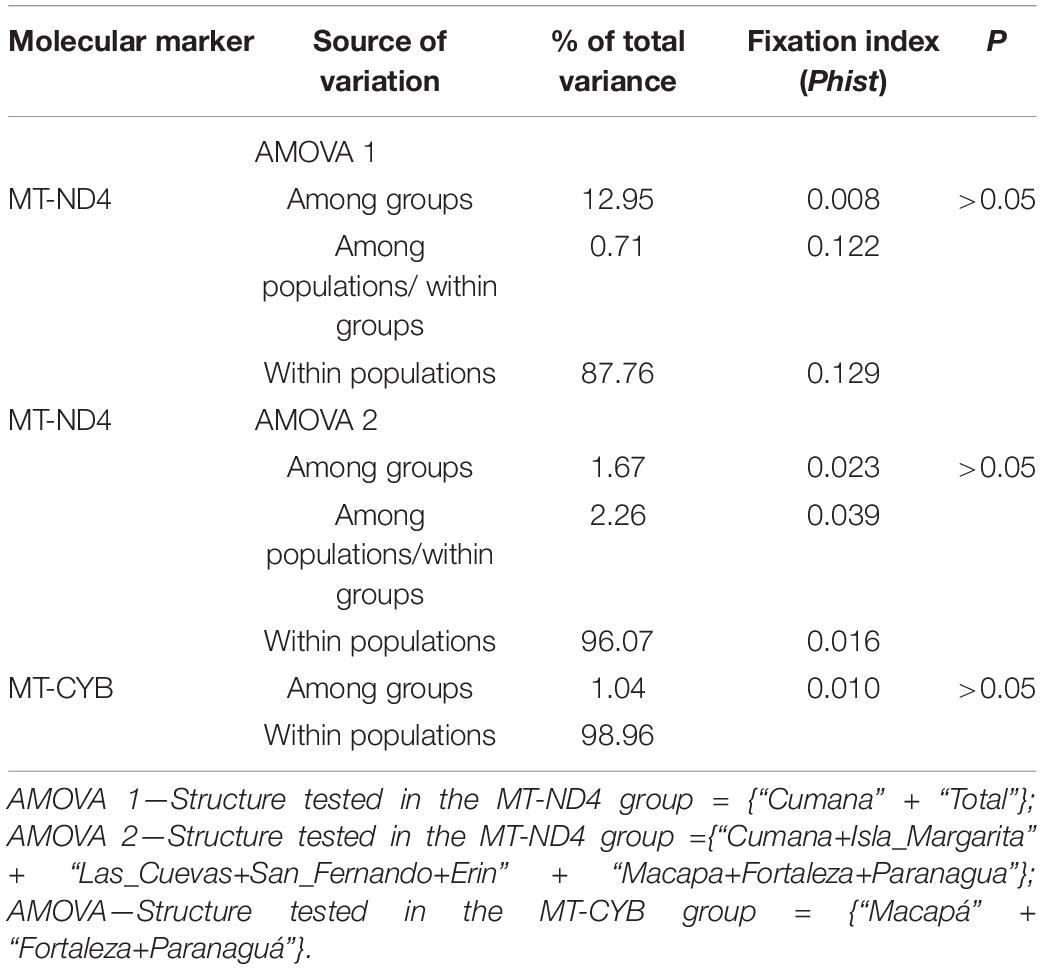
Table 2. Results of the Analysis of Molecular Variance (AMOVA) of S. brasiliensis from Brazil, Venezuela, and Trinidad and Tobago.
A dataset of 188 sequences of 500 bps was compiled for the MT-ND4 gene, based on the 188 S. brasiliensis specimens representing the South American distribution of the species (Figure 1B and Supplementary Material 2). In this case, haplotype diversity (Hd) ranged between 0.523 and 0.866, and nucleotide diversity (π) varied from 0.002 to 0.013 (Table 1). A total of 48 haplotypes were identified, one of which was quite common, being shared by 88 (47%) of the specimens and found in all of the populations analyzed (Figure 2B and Supplementary Material 2). The individuals from Cumana were the most variable of all the study localities, with some haplotypes being separated by up to 12 mutations (Figure 2B). The Fst (Table 3), AMOVA, and haplotype network all indicate the existence of a discrete genetic structure in this region.
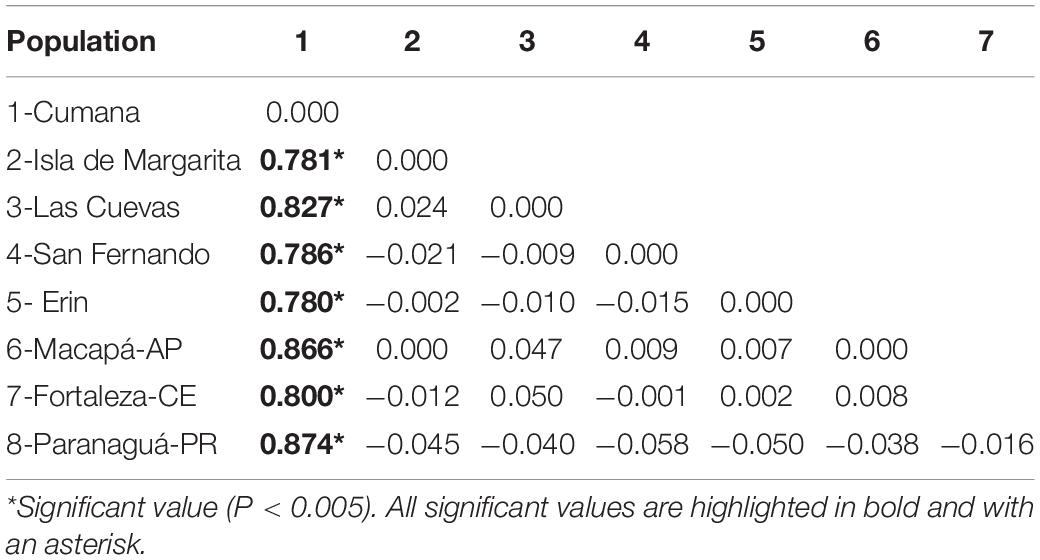
Table 3. Matrix of the values of the Fst fixation index for the MT-ND4 gene sequences from eight S. brasiliensis localities on the coast of Venezuela, Trinidade and Tobago, and Brazil.
All the results of the Analysis of Molecular Variance (AMOVA) indicated that the variation occurs primarily within populations, rather than between them, with low and non-significant PhiST values being obtained (Table 2). However, in the specific case of the MT-ND4 gene, when we tested Cumana and all the other regions (AMOVA 1), 12.9% of the variation was explained by the differences between this region and the other localities (Table 2). This result is supported by the Fst indices between localities, which were significant (Table 3).
In the case of the neutrality tests, both Fu’s F and Tajima’s D were significant only when the S. brasiliensis individuals were grouped within a single population for both mitochondrial genes. In accordance with Fu’s F and Tajima’s D for the total population, the Bayesian Skyline Plot presented evidence of demographic expansion in the S. brasiliensis population, dated to the Holocene in both markers. The phylogenetic analyses were run only for the MT-ND4 gene. The phylogeographic reconstructions based on the NJ and ML approaches identified two strongly supported groups of haplotypes. The first clade was formed by the four haplotypes from Cumana, which is represented by the yellow circles to highlight that there are two divergent groups (bootstrap NJ 97% and 80% ML), while the second clade contains the remaining S. brasiliensis haplotypes (Figure 1B).
Discussion
Scomberomorus brasiliensis is one of the most important fishery resources in the central Atlantic and northern Brazil, which may be suffering the effects of overfishing in some Brazilian regions (Nóbrega and Lessa, 2009). The present study analyzed datasets obtained from two distinct regions of the mitochondrial genome of S. brasiliensis specimens from the Brazilian coast, MT-CYB and MT-ND4. The MT-ND4 sequences were obtained from 188 S. brasiliensis specimens representing the known distribution of the species at South American.
The MT-ND4 sequences were structured in the S. brasiliensis specimens from Cunama, as indicated by the formation of two well-supported haplotype groups in the NJ and ML trees (Figure 1B). The Fst indices (Table 3) also indicated a greater degree of differentiation of these haplotypes, in comparison with the other localities. Gold et al. (2010) found genetic structuring in the Cumana specimens, a pattern like that observed in the present study, which was supported by the phylogeographic reconstructions (Figure 1B). One reason for the divergence of the Cumana population may be its geographic location (Figure 1A), which is relatively isolated from the influence of adjacent marine environments, a situation that may limit its contact with other populations. Even so, fidelity to spawning sites, adaptations to specific oceanographic conditions, or feeding preferences may also be relevant here (Gold et al., 2010; López et al., 2010; Zhu et al., 2014; Córdova-Alarcón et al., 2019).
It is important to note, however, that no evidence from either the population analyses or the haplotype network indicated any structuring among the other sites analyzed. According to the mitochondrial data, then, there is a single genetic stock of S. brasiliensis between eastern Venezuela and southern Brazil (Figure 1B and Table 3). In the MT-ND4 network, the most frequent haplotype was found in 47% of the specimens, and in all the S. brasiliensis populations (Figure 2B). As for the MT-ND4 gene, no population structuring was observed in the MT-CYB sequences, and the extremely low Fst values recorded among the three localities further reinforce the lack of population structure.
In the case of the MT-CYB, all three populations presented high levels of haplotype diversity (Hd: 0.701–0.808), but while low levels of nucleotide diversity were recorded in Macapá and Fortaleza, this value was relatively high in Paranaguá (Table 1). High levels of haplotype diversity (Hd: 0.523–0.866) were also recorded in the MT-ND4 gene, with low levels of nucleotide diversity (π: 0.002–0.013). All these values are relatively high in comparison with the sister species, S. cavalla, for which, Santa Brígida et al. (2007) recorded a Hd of 0.704.
This observed genetic homogeneity may be related to the dispersal capacity of the members of the family Scombridae (Collette and Russo, 1984; Moyle and Cech, 2004; López et al., 2010). The results of the present study are consistent with those of Batista and Fabré (2001), who concluded that the coast of the Brazilian state of Maranhão is a part of the species’ migratory circuit, which may exceed 300 nautical miles. Few physical barriers to dispersal exist in the ocean, and the dispersal of pelagic eggs and larvae on ocean currents, such as the Brazilian and North Brazil currents (see Rocha-Olivares et al., 2000; Broughton et al., 2002; Córdova-Alarcón et al., 2019), may also have contributed to the genetic homogeneity observed in the present study.
A similar pattern of genetic connectivity has been observed in other fish species, such as the King mackerel, Scomberomorus cavalla, on the northern and northeastern coasts of Brazil (Santa Brígida et al., 2007), and the red snapper, Lutjanus campechanus, and Acoupa weakfish, Cynoscion acoupa, populations of the Brazilian coast, in which no structuring was found in mitochondrial markers (Broughton et al., 2002; Gomes et al., 2008; Rodrigues et al., 2008; Silva et al., 2015). Furthermore, Siccha-Ramírez et al. (2018) utilizing SNPs (Single Nucleotide Polymorphisms) analyze samples from two commercially important species (S. brasiliensis and Thunnus albacares), where the results suggest the absence of any genetic structure among the local populations for both species, despite the sampling points are distant approximately 3,000 km.
As migratory pelagic fish generally present low levels of geographic differentiation across their distribution in the oceans due to their way of life as pelagic larval life with external fertilization and the swimming ability of the adults, strong genetic heterogeneity has nevertheless been detected at the regional scale in Scomberomorus commerson (López et al., 2010; Fauvelot and Borsa, 2011). Further studies of the Brazilian S. brasiliensis populations, based on more variable markers, such as microsatellites and new generation sequencing, may nevertheless reveal yet undetected structuring.
The BSP reconstructions indicate that the population of S. brasiliensis expanded between approximately 7 and 10 million years ago. The MT-CYB data indicate that the Ne of the Brazilian population of S. brasiliensis increased around 10,000 years ago (Figure 3A). In the case of the MT-ND4 gene, the whole population (Ne = 29 × 1014) expanded at approximately 7.5 million years ago (Figure 3B). It is important to treat the estimates obtained in the present study with caution, however, because the divergence rates of the mitochondrial genes are highly variable in fish (Donaldson and Wilson, 1999; Waters et al., 2007).
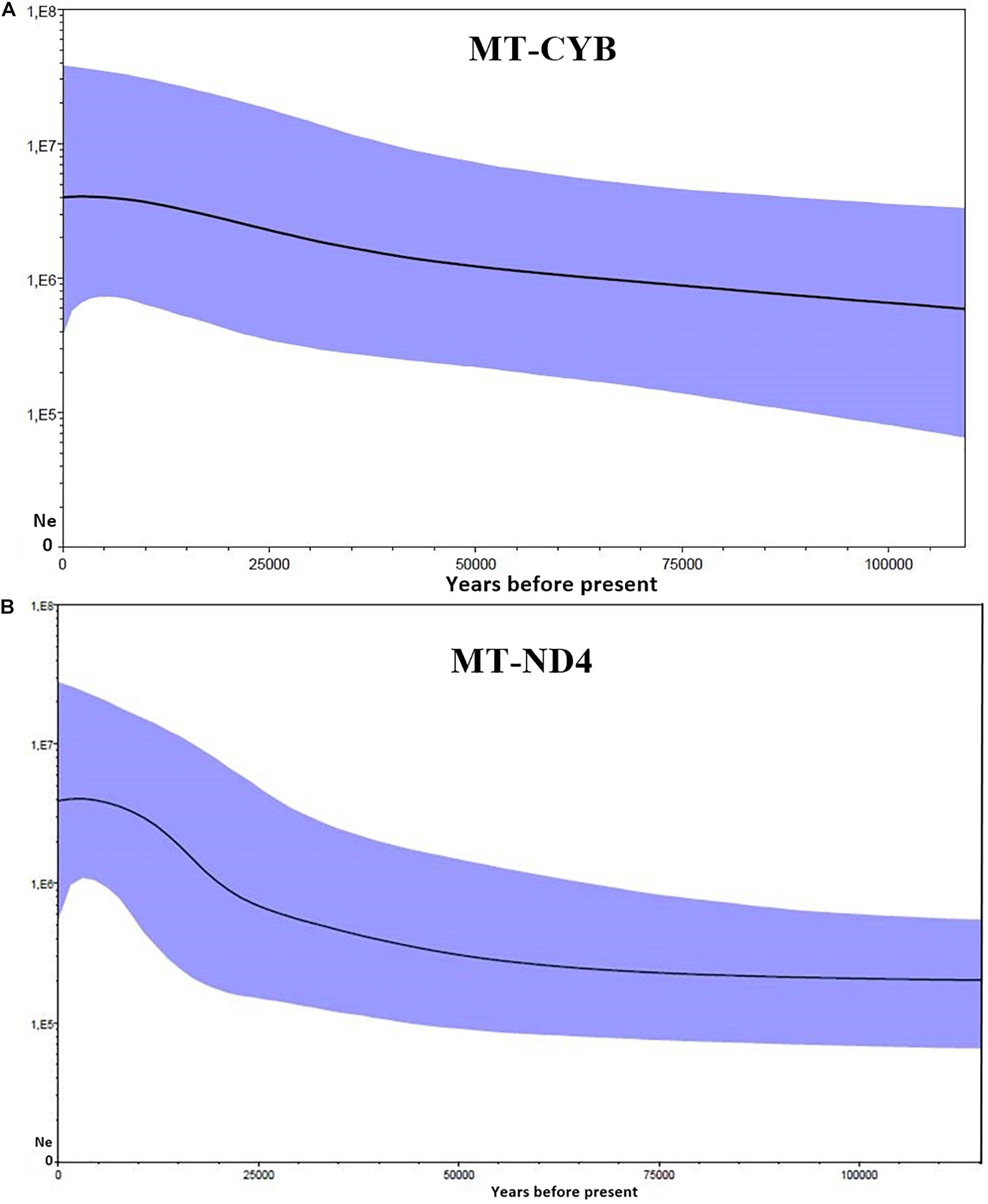
Figure 3. Bayesian Skyline plots showing changes in the effective population size over time in Scomberomorus brasiliensis, based on mtDNA sequences. The x axis shows time before present in years, going backward from left to right. The y axis shows the effective population size. (A) The posterior distribution of the effective population size over time based on the MT-CYB gene at three Brazilian localities, and (B) The posterior distribution of the effective population size over time based on the MT-ND4 gene from seven localities in the western South Atlantic. The dark line represents the mean, and the blue band represents the 95% highest posterior density.
The Holocene is characterized by an interglacial period that began approximately 10,000 years ago, when sea levels began to rise following the glaciations of the Pleistocene (Lambeck et al., 2002). The Serra Spanish mackerel is associated with areas of high productivity in which larval growth is favored (Lauth and Olson, 1996), and the increase in sea levels may have contributed to an increase in primary productivity, favoring the expansion of the S. brasiliensis populations into the tropical and subtropical waters of the Atlantic (Lee et al., 1995; Lambeck et al., 2002). This probable expansion of the population is supported by the negative and highly significant Fs and D values, which indicate a highly stable population with a long evolutionary history (Table 1).
Given the fact of S. brasiliensis is one of the most harvested marine fish species on the Brazilian coast we suggest that population of the southwestern Atlantic may correspond to a single genetic stock, and should thus be treated as a unit. The genetic variability observed in the S. brasiliensis population sampled in the present study is important for the definition of effective conservation strategies, with the primary objective of mediating the decline of the population and guaranteeing the sustainability of this fishery resource (Ward, 2000; López et al., 2010; Souza-Shibatta et al., 2018; Córdova-Alarcón et al., 2019). Given this, further research on the S. brasiliensis populations should adopt a multi-locus approach integrating microsatellites, SNPs, and RADseq (see Siccha-Ramírez et al., 2018), which would complement the findings of the present study, based on two mtDNA loci. The findings of the present study provide important insights into the genetic diversity and the potential distribution of the stocks of S. brasiliensis and provide a fundamental contribution to the development of effective, long-term management strategies for the populations of the species.
Data Availability Statement
The datasets generated for this study can be found in the online repositories. The names of the repository/repositories and accession number(s) can be found in the article/Supplementary Material.
Ethics Statement
Ethical review and approval was not required for the animal study because all Brazilian samples of S. brasiliensis were obtained directly from local fish markets or other retail outlets. The species is not subject to any legal restrictions in Brazil, according to the Brazilian Environment Institute (IBAMA).
Author Contributions
DC: acquisition of samples, laboratory procedures, analysis and interpretation of the data, development of the intellectual content, drafting and writing the manuscript, and the approval of the final version. PR: collected samples and drafted the manuscript. CQ: acquisition of samples, laboratory procedures, analysis and interpretation of data, draft of the manuscript, and the development of intellectual content. LR-F: analysis and interpretation of data, development of intellectual content, manuscript writing, and final version approval. JL: analysis and interpretation of data, draft work, development of intellectual content, writing of the manuscript, and approval of the final version. IS: interpretation of data, development of intellectual content, manuscript writing, and approval of the final version. MV: interpretation of data, development of intellectual content, manuscript writing, and final version approval. All authors contributed to the article and approved the submitted version.
Funding
This research was supported by the CNPq (Grants 306233/2009-6 to IS, 306233/2009-6 to IS and MCT/CNPq 14 – 2010 to MV), the FAPESPA (PRONEX 2007 to IS), and the CAPES/FCT 244/09 to MV. WT received a master’s stipend from CNPq. The authors are also grateful to CNPq for a research fellowship.
Conflict of Interest
The authors declare that the research was conducted in the absence of any commercial or financial relationships that could be construed as a potential conflict of interest.
Supplementary Material
The Supplementary Material for this article can be found online at: https://www.frontiersin.org/articles/10.3389/fmars.2020.558902/full#supplementary-material
References
Batista, V. S., and Fabré, N. N. (2001). Temporal and Spatial Patterns on Serra, Scomberomorus brasiliensis (Teleostei, Scombridae), Catches from the Fisheries on the Maranhão coast, Brazil. Braz. Jour. Biol. 61, 541–546. doi: 10.1590/s1519-69842001000400003
Bouckaert, R., Heled, J., Kuhnert, D., Vaughan, T., Wu, C., Xie, D., et al. (2014). BEAST 2: a software platform for Bayesian evolutionary analysis. PLoS. Comput. Biol. 10, 1–6. doi: 10.1371/journal.pcbi.1003537
Broughton, R. E., Stewart, L. B., and Gold, J. R. (2002). Microssatelite variation suggest substâncial gene flow between king mackerel (Scomberomorus cavalla) in the western Atlântic Ocean and Gulf of Mexico. Fisheries Res. 54, 305–316. doi: 10.1016/s0165-7836(01)00275-2
Collette, B. B., and Russo, J. L. (1984). Morphology, systematics, and biology of the Spanish mackerels (Scomberomorus maculatus, Scombridae). Fish. Bull. 82, 545–692.
Collette, B. B., Russo, J. L., and Zavala-Cumin, L. A. (1978). Scomberomorus brasiliensis. A new species of Spanish mackerel from the Western Atlantic. Fish. Bull. U.S. 76, 273–280.
Córdova-Alarcón, V. R., Araneda, C., Jilberto, F., Magnolfi, P., Toledo, M. I., and Lam, N. (2019). Genetic Diversity and Population Structure of Genypterus chilensis, a Commercial Benthic Marine Species of the South Pacific. Front. Mar. Sci. 6:748. doi: 10.3389/fmars.2019.00748
Craig, M. T., Hastings, P. A., Pondella, D. J. I. I., Robertson, D. R., and Casian, J. A. R. (2006). Phylogeography of the flag cabrilla (Epinephelus labriformis, Serranidae): implications for the biogeography of the Tropical Eastern Pacific and the early stages of speciation in a marine shore fish. Jour. Biog. 33, 969–979. doi: 10.1111/j.1365-2699.2006.01467.x
Darriba, D., Taboada, G. L., Doallo, R., and Posada, D. (2012). jModelTest 2: more models, new heuristics and parallel computing. Nat. Methods. 9:772. doi: 10.1038/nmeth.2109
Donaldson, K. A., and Wilson, R. R. (1999). Amphi-Panamaic geminates of snook (Percoidei: Centropomidae) provide a calibration of the divergence rates in the mitochondrial DNA control region of fishes. Mole. Phylogenet. Evol. 13, 208–213. doi: 10.1006/mpev.1999.0625
Excoffier, L., and Lischer, H. E. L. (2010). Arlequin suite ver 3.5: a new series of programs to perform population genetics analyses under Linux and Windows. Mol. Ecol. Resour. 10, 564–567. doi: 10.1111/j.1755-0998.2010.02847.x
Excoffier, L., Smouse, P. E., and Quattro, J. M. (1992). Analysis of molecular variance inferred from metric distances among DNA haplotypes: application to human mitochondrial DNA restriction data. Genetics 131, 479–491.
Fauvelot, C., and Borsa, P. (2011). Patterns of genetic isolation in narrow-barred Spanish mackerel (Scomberomorus commerson) across the Indo-West Pacific. Biol. Jour. Linn. Soc. 104, 886–902. doi: 10.1111/j.1095-8312.2011.01754.x
Felsenstein, J. (1985). Confidence limits on phylogenies: an approach using the bootstrap. Evolution 39, 783–791. doi: 10.1111/j.1558-5646.1985.tb00420.x
Fonteles-Filho, A. A. (1988). Sinopse de informações sobre a cavala, Scomberomoruscavalla, e a serra, S. brasiliensis, no Estado do Ceará. Arq. Ciên. Mar. 27, 21–48.
Fu, Y. X. (1997). Statistical tests of neutrality of mutations against population growth, hitchhiking and background selection. Genetics 147, 915–925.
Gesteira, T. C. V., and Mesquita, A. L. L. (1976). Época de reprodução, tamanho e idade na primeira desova da cavala e da serra, na costa do Estado do Ceará (Brasil). Arq. Ciên. Mar. 16, 83–88.
Gold, J. R., Jobity, A. M. C., Saillant, E., and Renshaw, M. A. (2010). Population structure of carite (Scomberomorus brasiliensis) in waters offshore of Trinidad and northern Venezuela. Fish. Res. 103, 30–39. doi: 10.1016/j.fishres.2010.01.009
Gomes, G., Schneider, H., Vallinoto, M., Santos, S., Orti, G., and Sampaio, I. (2008). Can Lutjanus purpureus (South red snapper) be “legally” considered a red snapper (Lutjanus campechanus)? Genet. Mole. Biol. 31, 372–376. doi: 10.1590/s1415-47572008000200035
Gonçalves, A. P., Dourado, E. C. S., Castro, A. C. L., and Tavares, R. G. C. F. (2003). Aspectos da dinâmica populacional da serra, Scomberomorus brasiliensis (Teleostei, Scombridae), no estado do maranhão, Brasil. Bol. Lab. Hidro. 16, 1982–6421.
Hall, T. A. (1999). BioEdit: a user-friendly biological sequence alignment editor and analysis program for Windows 95/98/NT. Nucl. Acids. Symp. Ser. 41, 95–98.
Instituto Brasileiro do Meio Ambiente e dos Recursos Naturais Renováveis [IBAMA] (2006). Available online at: http://www.ibama.gov.br/rec_pesqueiros/download.php?id_menu=93 (accessed 23 de Janeiro 2006).
Kalendar, R., Khassenov, B., Ramankulov, Y., Samuilova, O., and Ivanov, K. I. (2017). FastPCR: an in silico tool for fast primer and probe design and advanced sequence analysis. Genomics 109, 312–319. doi: 10.1016/j.ygeno.2017.05.005
Lambeck, K., Esat, T. M., and Potter, E. K. (2002). Links between climate and sea levels for the past three million years. Nature 419, 199–206. doi: 10.1038/nature01089
Lauth, R. R., and Olson, R. J. (1996). Distribution and abundance of larval scombridae in relation to the physical environment in the north-western Panama Bight. Inter. Am. Trop. Tuna Comm. Bull 21, 125–167.
Lee, W., Conroy, J., Howell, W. H., and Kocher, T. D. (1995). Structure and evolution of teleost mitochondrial control region. J. Mole. Evol. 41, 54–66.
López, M. D., Alcocer, M. U., and Jaimes, P. D. (2010). Phylogeography and historical demography of the Pacific Sierra mackerel (Scomberomorus sierra) in the Eastern Pacific. BMC Genet. 11:34. doi: 10.1186/1471-2156-11-34
Maia, R. C. N., Silva, B. B., Holanda, F., Pereira, L. J. G., and Alberto, F. C. (2015). Pesca comercial e estrutura populacional da serra, Scomberomorus brasiliensis (Collette, Russo & Zavala, 1978), desembarcada em um pólo pesqueiro na Costa Norte do Brasil. Biota Amazônia. 5, 99–106. doi: 10.18561/2179-5746/biotaamazonia.v5n2p99-106
Moyle, P. B., and Cech, J. J. (2004). Fishes: an introduction to ichthyology. Upper Saddle River, NJ: Prentice-Hall. 593.
Nóbrega, M. F., and Lessa, R. P. (2009). Age and growth of spanish mackerel (Scomberomorus brasiliensis) off the Northeastern Coast of Brazil. Neot. Ichthy 7, 667–676. doi: 10.1590/S1679-62252009000400016
Oliveira, C., Foresti, F., and Hilsdorf, A. W. S. (2009). Genetics of neotropical fish: from chromosomes to populations. Fish. Physiol. Biochem. 35, 81–100. doi: 10.1007/s10695-008-9250-1
Oliveira, J. N., Gomes, G., Rêgo, P. S., Moreira, S., Sampaio, I., Schneider, H., et al. (2014). Molecular Data Indicate the Presence of a Novel Species of Centropomus (Centropomidae - Perciformes) in the Western Atlantic. Mol. Phyl. Evol. 77, 275–280. doi: 10.1016/j.ympev.2014.04.019
Rambaut, A., Suchard, M. A., Xie, D., and Drummond, A. J. (2014). Tracer v1.6. Available online at: http://beast.bio.ed.ac.uk/Tracer (accessed January 28, 2020).
Reiss, H., Hoarau, G., Dickey-Collas, M., and Wolff, W. J. (2009). Genetic population structure of marine fishes: mismatch between biological and fisheries management units. Fish Fisheries 10, 359–478.
Rocha-Olivares, A., Garber, N. M., and Stuck, K. C. (2000). High genetic diversity, large inter-oceanic divergence and historical demography of the striped mullet. J. Fish Biol. 57, 1134–1149. doi: 10.1111/j.1095-8649.2000.tb00476.x
Rodrigues, R., Schneider, H., Santos, S., Vallinoto, M., Saint-Paul, U., and Sampaio, I. (2008). Low levels of genetic diversity depicted from mitochondrial DNA sequences in a heavily exploited marine fish (Cynoscion acoupa, Sciaenidae) from the Northern coast of Brazil. Genet. Mole. Biol. 31, 487–492. doi: 10.1590/s1415-47572008000300015
Salzburger, W., Ewing, G. B., and Von Haeseler, A. (2011). The performance of phylogenetic algorithms in estimating haplotype genealogies with migration. Mole. Ecol. 20, 1952–1963. doi: 10.1111/j.1365-294x.2011.05066.x
Sambrook, J., Maccallum, P., and Russel, D. (2001). Molecular cloning: A laboratory manual, 3nd ed, New York, NY: Cold Springs Harbour Press,Google Scholar
Santa Brígida, E. L., Cunha, D. B., Rego, P. S., Sampaio, I., Schneider, H., and Vallinoto, M. (2007). Population analysis of Scomberomorus cavalla (Cuvier 1829) (Perciformes, Scombridae) from the Northern and Northeastern coast of Brazil. Braz. J. Biol. 67, 919–924. doi: 10.1590/s1519-69842007000500016
Siccha-Ramírez, Z., Maroso, F., Pardo, B. G., Fernández, C., Martínez, P., and Oliveira, C. (2018). SNP identification and validation on genomic DNA for studying genetic diversity in Thunnus albacares and Scomberomorus brasiliensis by combining RADseq and long read high throughput sequencing. Fisheries Res. 198, 189–194. doi: 10.1016/j.fishres.2017.09.002
Silva, R., Veneza, I., Sampaio, I., Araripe, J., Schneider, H., and Gomes, G. (2015). High Levels of Genetic Connectivity among Populations of Yellowtail Snapper, Ocyurus chrysurus (Lutjanidae – Perciformes), in the Western South Atlantic Revealed through Multilocus Analysis. PLoS One 10:0122173. doi: 10.1371/journal.pone.0122173
Souza-Shibatta, L., Kotelok-Diniz, T., Ferreira, D. G., Shibatta, O. A., Sofia, S. H., de Assumpção, L., et al. (2018). Genetic Diversity of the Endangered Neotropical Cichlid Fish (Gymnogeophagus setequedas) in Brazil. Front. Genet. 9:13. doi: 10.3389/fgene.2018.00013
Suchard, M. A., Weiss, R. E., and Sinsheimer, J. S. (2001). Bayesian selection of continuous-time Markov chain evolutionary models. Mole. Biol. Evol. 18, 1001–1013. doi: 10.1093/oxfordjournals.molbev.a003872
Swofford, D. L. (2002). PAUP∗: Phylogenetic Analysis Using Parsimony and other Methods Version 4. Sundeland, Ma: Sinauer Associates, Inc.
Tajima, F. (1989). Statistical method for testing the neutral mutation hypothesis by DNA polymorphism. Genetics 123, 585–595.
Thompson, J. D., Higgins, D. G., and Gibson, T. J. (1994). Clustal W: improving the sensitivity of progressive multiple sequence alignment through sequence weighting, position-specific gap penalties and weight matrix choice. Nucleic. Acids. Res. 22, 4673–4680. doi: 10.1093/nar/22.22.4673
Ward, R. D. (2000). Genetics in fisheries management. Hydrobiology 420, 191–201. doi: 10.1007/978-94-017-2184-4_18
Waters, J. M., Rowe, D. L., Apte, S., King, T. M., Wallis, G. P., et al. (2007). Geological Dates and Molecular Rates: Rapid Divergence of Rivers and Their Biotas. Syst. Biol. 56, 271–282. doi: 10.1080/10635150701313855
Keywords: demographic history, genetic diversity, western South Atlantic, mitochondrial genes, S. brasiliensis
Citation: da Cunha DB, Rodrigues-Filho LFS, de Luna Sales JB, Rêgo P, Queiroz C, Sampaio I and Vallinoto M (2020) Molecular Inferences on Scomberomorus brasiliensis, From the Western South Atlantic, Based on Two Mitochondrial Genes. Front. Mar. Sci. 7:558902. doi: 10.3389/fmars.2020.558902
Received: 04 May 2020; Accepted: 16 October 2020;
Published: 04 November 2020.
Edited by:
Salvatore Siciliano, Oswaldo Cruz Foundation (Fiocruz), BrazilReviewed by:
Maria Carolina Viana, Campinas State University, BrazilVictor Hugo Valiati, University of the Rio dos Sinos Valley, Brazil
Copyright © 2020 da Cunha, Rodrigues-Filho, de Luna Sales, Rêgo, Queiroz, Sampaio and Vallinoto. This is an open-access article distributed under the terms of the Creative Commons Attribution License (CC BY). The use, distribution or reproduction in other forums is permitted, provided the original author(s) and the copyright owner(s) are credited and that the original publication in this journal is cited, in accordance with accepted academic practice. No use, distribution or reproduction is permitted which does not comply with these terms.
*Correspondence: Divino B. da Cunha, divinobruno@yahoo.com.br