- 1Department of Marine Biology, Xiamen Ocean Vocational College, Xiamen, China
- 2Fujian Key Laboratory of Marine Carbon Sequestration, Xiamen University, Xiamen, China
Microplastics can release dissolved organic matter (DOM) into seawater under solar radiation exposure. However, the molecular composition and bioavailability of this DOM remain to be investigated. Here, two popular microplastics, low-density polyethylene (LDPE) and polystyrene (PS), were exposed to solar radiation in an artificial seawater for 10 days. The solar-induced LDPE-DOM and PS-DOM were molecularly characterized using ultra-high-resolution mass spectrometry, and were further incubated in a coastal microbial assemblage to examine their bioavailability. Results showed that solar radiation stimulated release of DOM from the microplastics. Dissolved organic carbon concentration analysis indicated that approximately 19.03 µg C L–1 and 3.85 µg C L–1 were released from each gram of LDPE and PS per day, respectively. Molecular composition analysis showed that both the LDPE-DOM and PS-DOM comprised a proportion of nitrogen- and sulfur-bearing molecules, and that the LDPE-DOM molecules were associated with lower molecular abundance and values of double-equivalent-bond and aromatic-index, but higher average hydrogen-to-carbon ratio than that in the PS-DOM. In addition, a proportion of the assigned formulas in LDPE-DOM (22.3%) and PS-DOM (55.8%) could be found in a coastal-DOM sample, suggesting their potential contribution to coastal DOM pool. The further incubation experiment showed that nearly 18.7% of LDPE-DOM and 9.5% of PS-DOM were utilized or transformed within 30 days. Still, a fraction of the solar-induced LDPE-DOM and PS-DOM resisted rapid microbial utilization, remained as semi-labile DOM. These results underlined unaccounted consequences of microplastic-derived DOM in coastal DOM pool.
Introduction
Owing to the properties of low cost, excellent barrier properties, bio-recalcitrance, and light weight, plastics are globally used as packaging materials in various fields (Andrady, 2011). However, these materials are subjected to various biotic and abiotic processes, causing an emergence of microplastic pollutant. Microplastic is generally defined as plastic debris less than 5 mm in size and is ubiquitously found in marine environments, such as coastal seawaters and sediments, subtropical ocean gyres, and deep-sea sediments (Ling et al., 2017; Onink et al., 2019; Cunningham et al., 2020; Soursou et al., 2023). Annually, about 8-13 million tons of plastics could be transported into the ocean, mainly including polyethyelene, polystyrene, polypropylene, poly-ethylene terephthalate, and poly-vinyl chloride (Jambeck et al., 2015; Guo and Wang, 2019). The weathering of plastic produces micro- and nano- (plastic debris less than 1 μm in size) plastics, which have built up in the environment to an extent and have been considered as a planetary boundary threat (Villarrubia-Gomez et al., 2018; Arp et al., 2021). The plastic fragments and fibers are typically accumulated in coastal environments (Martellini et al., 2018; Luo et al., 2022). Accumulated microplastics could affect the marine fauna from small copepods to large mammals, causing stress, false satiation, reduced growth rates, and affecting reproduction (Romera-Castillo et al., 2018); another aspect is their potential roles as toxic inorganic trace elements and persistent organic pollutants (Soursou et al., 2023). In addition, microplastic-induced water column deoxygenation in some regions could take hundreds of years for recovery, indirectly affecting the ocean environments (Kvale and Oschlies, 2023). Therefore, microplastic has aroused global concerns since it was firstly introduced in nearly two decades ago (Thompson et al., 2004).
Microplastic in the seawater could be subjected to various biotic degradation (for example, microbial degradation) (Mishra et al., 2022; Ou et al., 2023) and abiotic processes (such as photo-degradation, photo-oxidation, thermal radiation degradation, and hydrolysis) (Andrady, 2011; Delacuvellerie et al., 2019; Birnstiel et al., 2022), which together determine the fate of microplastic in the seawater. Photo-degradation induced by solar radiation has been demonstrated as an efficient mechanism in plastic degradation (Andrady, 2011; Lee et al., 2020; Xu et al., 2022). When floating in seawater, photo-oxidation of microplastic leads to the formation of polar functional groups (for example carbonyl groups) in its polymers, potentially reducing the surface hydrophobicity of microplastic; leaching of additives could also occur (Arp et al., 2021). Previous studies have shown that microplastics can release dissolved organic matter (DOM) (Romera-Castillo et al., 2023), which could affect molecular composition of seawater DOM, or potentially becomes a part of the oceanic DOM pool (~662 Pg Carbon) (Hansell, 2013). Although numerous studies have investigated the occurrence and fate of microplastics in various environments, and recent studies have paid much attention in the characterization of plastic-leached DOM using mass spectrometry, fluorescence spectroscopy, and other analytical chemistry methods (Lee et al., 2020; Li Y. et al., 2022; Romera-Castillo et al., 2022b; Xu et al., 2022), limited efforts have been devoted toward exploring the high-resolution molecular composition of the microplastic-released DOM, together with its bioavailability in seawater. Therefore, it is essential to understand the fate of microplastics by concurrently studying the molecular composition and bioavailability of the plastic-derived organic matter.
A latest study recommends several state-of-the-art techniques for characterizing plastic-leached DOM (Zhu et al., 2023), among them, the Fourier-transform ion cyclotron resonance mass spectrometry (FT-ICR MS) coupled with soft electrospray ionization (ESI) is a promising approach, since thousands of molecular formulas can be assigned to present high accuracy of DOM formulas and comprehensive understandings on DOM fingerprints via the FT-ICR MS (Kim et al., 2020). Although ESI-FT-ICR MS has been successfully applied on characterization of DOM in diverse ecosystems (Shi et al., 2021; He et al., 2022; He et al., 2023), limited studies have applied such state-of-the-art technique to provide high-resolution molecular composition on the plastics-leached DOM. Here, two popular microplastics, low-density polyethylene (LDPE) and polystyrene (PS), were selected for solar radiation degradation experiments, which aimed at revealing the effect of solar radiation on the molecular composition and bioavailability of microplastic-derived DOM in seawater. The LDPE is mainly used to produce plastic bags, six-pack rings, drinking straws, and wire cables, while the PS is applied to produce plastic utensils, medical bottle, meat trays, egg cartons, carry-out containers, and other food containers (Andrady, 2011; Guo and Wang, 2019). Studying the solar radiation effects on the DOM composition and bioavailability from these two popular microplastics should shed light into their fate in the ocean.
Materials and methods
Experimental setup for solar radiation degradation of microplastics
LDPE (~ 3 mm pellets, product number: 428043) and PS (~ 4 mm pellets, product number: 331651) were purchased from Sigma-Aldrich and used for solar radiation degradation experiment. Operationally, the two microplastics were sequentially rinsed by ultrapure water (Milli-Q) and designated artificial seawater (ASW) using pre-combusted (450 °C, 4 h) salts (NaCl, MgSO4, MgCl2, CaCl2, KCl, NaF, and KBr; anhydrous) and NaHCO3, H3BO3, NH4Cl, NaNO3, and KH2PO4 following the studies of Lechtenfeld et al. (2015) and Kester et al. (1967). The rinse would reduce contamination from the commercial microplastics. In addition, the ASW was filtered through 0.1 μm pore size PVDF filters (33 mm, Millipore) to remove microbes that might potentially utilize microplastics during the solar radiation experiment. For each treatment, ~ 16 grams of the microplastics were rinsed by ASW and then amended into 1 L of the filtered ASW in a 1.5 L quartz flask (450°C pre-combusted, 4h). The quartz flasks wrapped with aluminum were served as controls, while the non-wrapped flasks were marked as treatments. All the control and treatment flasks were then placed into a solar radiation simulator fitted with a 1 kW xenon lamp, to simulate sunlight reaching the seawater surface. Treatments were exposed to the solar simulator for 10 days, approximating the same time of clear-sky solar irradiance at the latitude of Xiamen city (China) during mid-September. The experiments were conducted in duplicate. On each incubation day, ~20 mL of water sample was collected from each incubation for DOC analysis. On day 10, ~ 1 mL of water sample was collected for bacteria abundance analysis (see below), to determine heterotrophic bacteria that potentially grew to utilize the microplastics.
Bioavailability examination of solar-induced DOM from microplastics
After the 10-day solar radiation experiment, the incubated ASW samples in treatments were filtered through pre-combusted (450°C for 4 h) GF/F glass fiber filters (0.75-μm, Whatman). Filtrates were subjected to further microbial incubation, in order to test bioavailability of the solar- induced DOM from the two microplastics. Prior to the microbial incubation experiment, a marine microbial assemblage was collected from coastal area (Supplementary Figure 1, 24°5793´ N, 118°3459´ E) near the Xiamen city on September 20, 2022. The filtrates were mixed with the seawater (v: v = 4: 1) to inoculate the microbial assemblage as treatments, while the ASW mixed with the same seawater (v: v = 4: 1) was served as controls. The experiment was conducted in duplicated in the dark and in a controlled temperature environment (24~26°C) for 30 days. In each incubation, subsamples were collected on days 0, 1, 4, 8, 15, and 30 for bacterial abundance analysis; subsamples for DOC concentration measurement were collected on days 0 and 30.
DOC concentration analysis
Water samples (aliquot of 20 mL) from the solar radiation incubation and microbial incubation experiment were filtered through GF/F filters (450°C pre-combusted, 4h) and collected into 40 mL pre-combusted glass vials (CNW, USA). The samples were then acidified to pH=2 with phosphoric acid (Guaranteed reagent GR, Sinopharm) and stored at −20°C until analysis. The DOC concentration was measured using a high-temperature combustion method using a Shimadzu TOC-LCPH Total Organic Carbon analyzer. Reference deep seawater and low-carbon water were provided by the Hansell Organic Biogeochemistry Laboratory at the University of Miami, and were used to calibrate the TOC analyzer.
DOM composition analysis
An aliquot of 500 mL water sample from each incubation of the solar radiation experiment was collected on days 0 and 10, and was filtered through pre-combusted GF/F glass fiber filters. The filtrates were acidified to pH=2 using 30% HCl (HPLC grade, Merck), then ready for solid-phase extraction. The solid-phase extraction was conducted using 100-mg Bond Elut PPL cartridges (Agilent) followed the procedures reported in Dittmar et al. (2008). Additional 500 mL of the coastal seawater was collected at the location (Supplementary Figure 1) where the coastal microbial assemblage was collected for bioavailability incubation. DOM of this coastal seawater was also solid-phase extracted for molecular composition analysis.
The DOM extracts were adjusted to yield an approximate DOC concentration of 25 mmol C L-1 and analyzed using a Bruker Solarix 2XR Fourier transform ion cyclotron resonance mass spectrometer (FT-ICR MS) equipped with a 7 T superconducting magnet. The samples were infused via an Apollo II electrospray ion source (ESI) at 200 μL h–1 with a syringe pump. Typical operating conditions for negative ESI were as follows: spray shield voltage 3.5 kV, capillary column introduce voltage 4 kV, and capillary column end voltage -320 V. The ion transformation parameter for the quadrupole was optimized at m/z 300. The mass range was set to m/z 200–800. The 2M word size was selected for the time domain signal acquisition. A total of 128 time-domain signals were added together to enhance the signal-to-noise ratio and dynamic range. A molecular formula calculator generated matching formulae according to elemental combinations of 12C1−60, 13C0-1, 1H1−120, 14N0−3, 15N0-1, 16O0−30, 18O0-1, 32S0−1, and 34S0-1 (He et al., 2020). The mass accuracy window was set to 1.0 ppm in the formula assignment section. All assigned formulas had to meet the following basic chemical criteria: (1) the number of H atoms should be at least 1/3 that of C atoms and cannot exceed 2C + N + 2; (2) the sum of H and N atoms must be even (the “nitrogen rule”); and (3) the number of N or O atoms cannot exceed the number of C atoms (Koch and Dittmar, 2006; Kujawinski and Behn, 2006). Additional DOM assignment criteria followed the study of Yi et al. (2023).
Because this study focuses on molecular composition of only the solar radiation induced DOM from LDPE and PS. Therefore, any formula appeared in the day 0 incubation did not belong to the microplastic-DOM, was then removed from the DOM samples extracted on day 10. Average values of double-equivalent-bond (DBEa) (Koch and Dittmar, 2006), a modified aromatic index (AImoda) (Koch and Dittmar, 2016), hydrogen to carbon ratios (H/Ca), and oxygen to carbon ratios (O/Ca) were calculated for the solar-induced and photochemical transformed DOM (hereafter defined as LDPE-DOM and PS-DOM) and Coastal-DOM.
Bacteria abundance analysis
Bacterial cells of 1 mL were preserved in sterile cryotubes at each sampling day and glutaraldehyde was added at a final concentration of 1% (v/v). All the cryotubes were directly frozen with liquid nitrogen and stored in -80°C before analysis. Bacterial cells were stained with SYBR green (Invitrogen, USA) and determined using an Accuri C6 Flow Cytometer (BD Biosciences, USA).
Results and discussion
DOC leached from microplastics during the solar radiation experiment
DOC concentrations were measured in the solar radiation treatments of LDPE (LDPE-light) and PS (PS-light) and dark incubations of LDPE (LDPE-dark) and PS (PS-dark) during the 10-day period, to investigate how microplastics released DOC into saline water under solar radiation exposure. DOC concentrations in the LDPE-dark and PS-dark incubations remained almost invariant, while DOC concentrations in the LDPE-light and PS-light incubations started to increase after one day (Figure 1), indicating that solar radiation stimulated release of DOC from the microplastics. After 10 days, DOC concentration in the LDPE-light incubations was 253.72 ± 13.8 µmol C L–1, which is higher than that in the PS-light incubations (51.28 ± 0.47 µmol C L–1). During this period, the average DOC release rates were approximately 19.03 and 3.85 µg C L–1 day-1 from each gram of the LDPE and PS pellets, respectively. After the 10-day incubation, both the incubated waters were collected from the light and dark incubation of LDPE and PS for flow cytometer analysis, to assess potential growth of heterotrophic bacteria during the incubation period. Almost no living bacteria could be detected in all the light incubations, indicating that biological activities could be excluded from the incubation. Overall, by comparing the light and dark incubation, irradiation significantly enhanced the release of DOC from both microplastics.
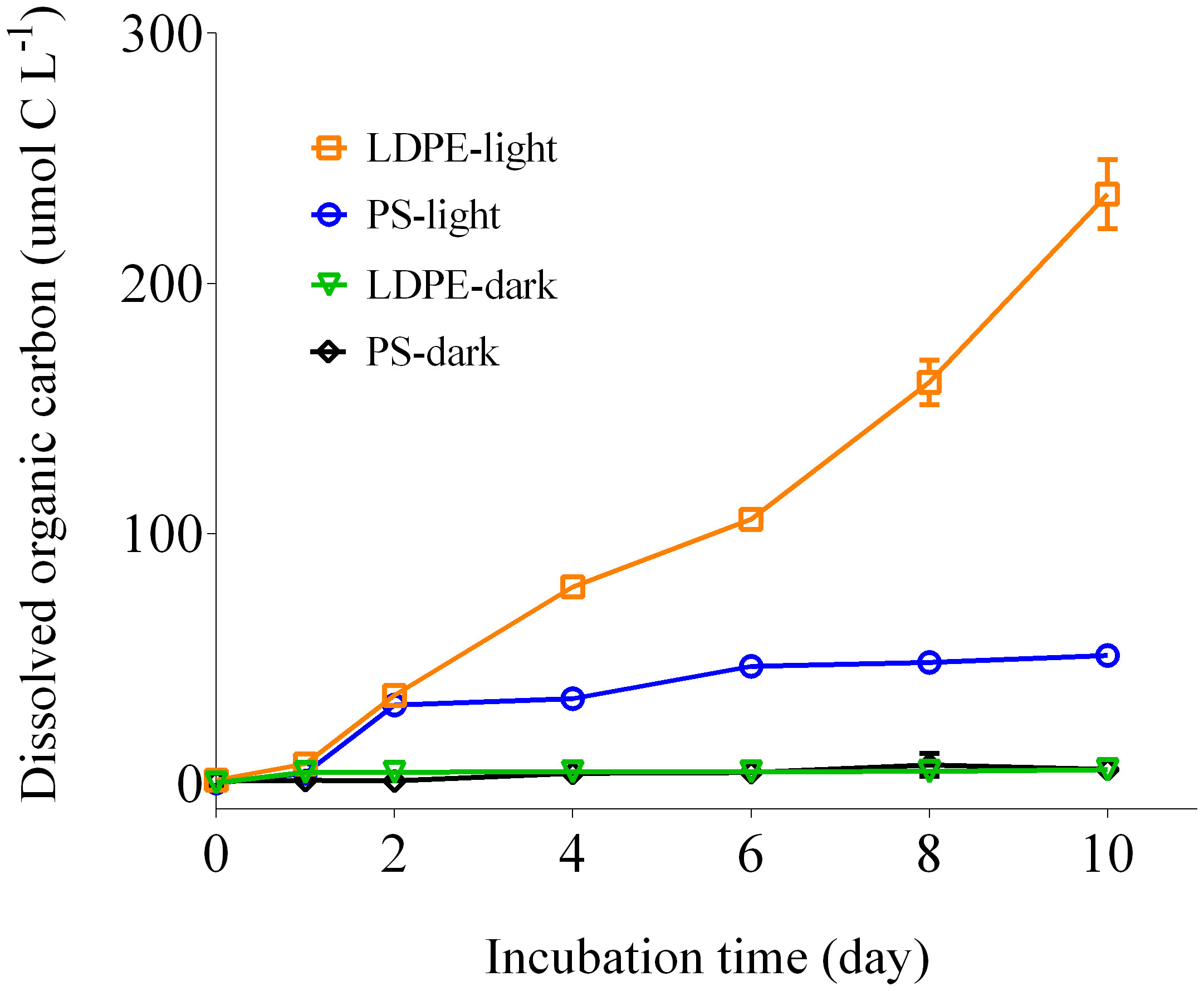
Figure 1 Temporal variations in dissolved organic carbon (DOC) concentration leached from microplastics over 10 days of incubation (mean ± SD, n = 2). DOC concentration in the solar radiation treatments of low-density polyethylene (LDPE-light) and polystyrene (PS-light) and dark incubation of low-density polyethylene (LDPE-dark) and polystyrene (PS-dark) was measured during the 10-day incubation.
The LDPE released relatively higher amount of DOC than PS in the solar radiation experiment (Figure 1), this result is consistent with previous studies (Romera-Castillo et al., 2022a; Romera-Castillo et al., 2022b). The LDPE is polymerized by ethylene and generally applied to produce thin containers. Compared to PS, LDPE is composed of short branches of carbon chains in molecular structure and is made in lower density (Delacuvellerie et al., 2019). Likely, the ultraviolet rays and other radiations could easily penetrate into the lower density of the polymeric structure of LDPE, accelerating aging of LDPE pellets and DOC release. PS is a benzene-based plastic and could be photo-active than PE, because aromatic structures are the potential photo-oxidation to take place (Medeiros et al., 2015). However, the PS released much lower content of DOC than PS in our experiment. Supplementary Figure 2 shows that the appearance of PS pellets was photochemically modified as light yellow from initially transparent. It is unclear whether such modification inhibited DOC release, because DOC concentration in the PS-light incubation remained almost invariant since day 2, whereas the DOC concentration was gradually increased in the LDPE-light incubation (Figure 1). Our study suggests that although PS could be photo-active, higher photo-susceptibility does not necessarily mean higher release of DOC. However, a latest study shows that postconsumer PS granules released higher content of DOC than standard PE in a photochemical reaction experiment (Stubbins et al., 2023), opposing our experimental results. Microplastics usually contain varied types and contents of additives that can significantly affect their photochemical aging and fragmentation process in seawater (Wu et al., 2021). Different additives and photo-reactive contaminants affect photochemical degradation of microplastic (Wu et al., 2021; Yaseen et al., 2021), and potentially determine DOC leaching during photochemical reaction of microplastics, yet the latter requires further comparison experiments.
Molecular composition of the solar radiation induced DOM
Microplastics released DOM into the ambient ASW under solar radiation (Figure 1), some of the DOM molecules could be photochemically transformed because the microplastic pellets and waters continuously received solar radiation during the 10 days. Molecular characteristics of the solar-induced and photochemically transformed DOM (LDPE-DOM and PS-DOM) were then analyzed using high resolution FT-ICR MS. In addition, DOM extracted from a natural coastal seawater (Supplementary Figure 1) was also analyzed for comparison. Molecular formulas were assigned and their basic characteristics (formula counts, average values of O/Ca, H/Ca, DBEa, and AImoda) were shown in Supplementary Table 1. The assigned molecular formulas and their corresponding abundances were shown as van Krevelen diagrams (Figures 2A–C), which indicated a clear difference of molecular pattern between the LDPE-DOM and PS-DOM. The molecular composition of these two DOM samples also differed from the coastal seawater DOM sample, suggesting that different DOM molecules were produced from different microplastics when exposed to solar radiation.
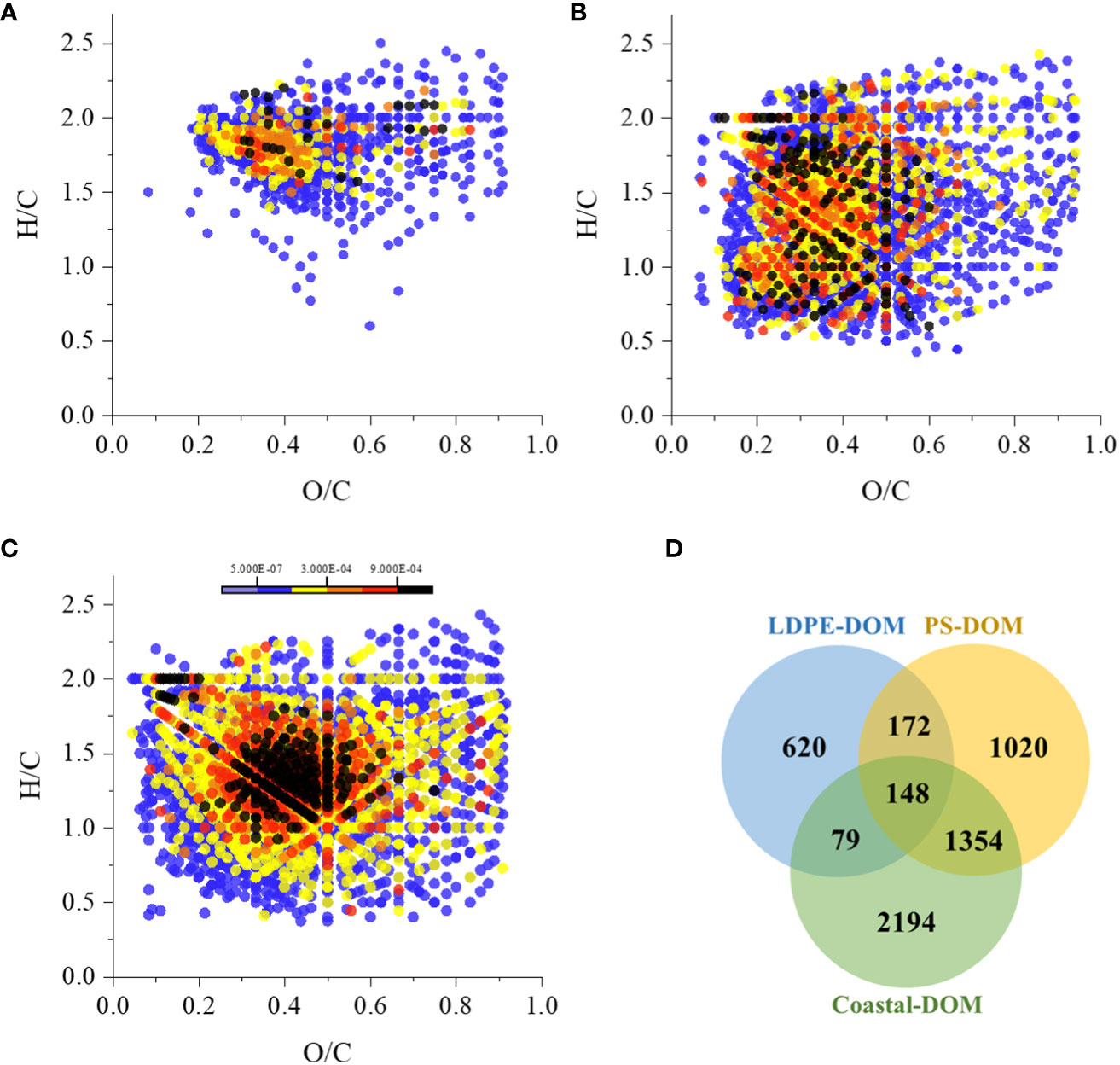
Figure 2 Molecular analyses of DOM revealed by ultra-high-resolution FT-ICR MS. van Krevelen diagrams showing the molecular distribution of the solar radiation induced LDPE-DOM (A) and PS-DOM (B), and a natural DOM extracted from a coastal seawater (Coastal-DOM, C). The color bar represents the relative abundance of the formulas in each DOM extract. A Venn diagram (D) shows the molecular overlap of LDPE-DOM, PS-DOM, and Coastal-DOM. Numbers in areas of overlap are the formula count that appears in both and three of the LDPE-DOM, PS-DOM, and Coastal DOM. Numbers in areas with no overlap are of formulas that are unique to that individual sample.
Compared to the PS-DOM, lower molecular abundance (Figures 2A, B) and values of DBEa and AImoda, but higher H/Ca (Supplementary Table 1) were found in the LDPE-DOM. The AImoda was first calculated and applied for the identification of polyaromatic hydrocarbons (Koch and Dittmar, 2006; Koch and Dittmar, 2016). Generally, higher value of AImoda indicates a higher number of aromatic rings fused in DOM molecules (Koch and Dittmar, 2006). The DBE generally represents the sum of unsaturation plus rings in a molecule. DBEa is also used to support AImoda in evaluating the contribution of aromatic and condensed aromatic structures in DOM samples (Cai and Jiao, 2023). Similarly, the H/C ratio has been applied to evaluate the general unsaturated state of a molecule (Cai and Jiao, 2023). The lower value of H/Ca ratios accompanied with higher values of DBEa and AImoda indicates a higher unsaturated state of the molecule in the PS-DOM, suggesting that the PS-DOM could contain higher content of aromatic structures in DOM molecules (Koch and Dittmar, 2006). Due to the benzene ring in styrene could be potential photo-oxidation to take place, PS pellets could then leach high abundance of aromatic structures containing DOM molecules at the early stage of solar radiation exposure. We further speculated that the DBEa and AImoda could be decreased in the PS-DOM, if the PS-DOM continuously received additional solar radiation, because some aromatic rings containing molecules or unsaturated molecules are generally sensitive to ultraviolet light (Bostick et al., 2021). The highly aromatic structures can be bleached or broken down after photochemical degradation, or can be transformed into unsaturated aliphatic molecules and/or volatile organic compounds and carbon monoxide, increasing the bioavailability of the originally bio-recalcitrant DOM (Mopper et al., 1991; Stedmon et al., 2007). Therefore, solar radiation accompanied with microbial degradation could accelerate elimination of microplastics in the seawater, and promote the cycling of the microplastic-derived DOM in aquatic environments.
A proportion of the solar-induced DOM shared same molecular formulas with the natural seawater DOM (Figure 2D). About 22.3% of the LDPE-DOM formulas existed in the natural coastal DOM, while nearly 55.8% of the PS-DOM formulas were commonly assigned in the natural coastal DOM sample (Figure 2D), suggesting that a fraction of the solar-induced DOM molecules could contribute to natural seawater DOM. Nevertheless, we noted that these common formulas in natural seawater are not necessarily from microplastics, because an assigned formula could represent molecules with different chemical structures. Compared to the LDPE-Coastal common molecules, molecular characteristics of the LDPE-DOM unique molecules were characterized as lower O/Ca, DBEa, and AImoda, but higher H/Ca ratio (Supplementary Figure 3 and Table 1). Similar trends of these parameters (except the DBEa) were found in the comparison between the PS-DOM unique molecules and PS-Coastal common molecules. Generally, DOM with higher H/Ca ratio and lower values of DBEa and AImoda indicates its overall state of relatively higher saturated, and has been found to accompany relatively higher Δ14C values (Flerus et al., 2012). DOM with these molecular properties could be relatively bio-labile (Cai et al., 2019; Lian et al., 2021; Li H. et al., 2022). Therefore, some of the LDPE-DOM unique and PS-DOM unique molecules might be preferentially utilized by microbes in natural seawater.
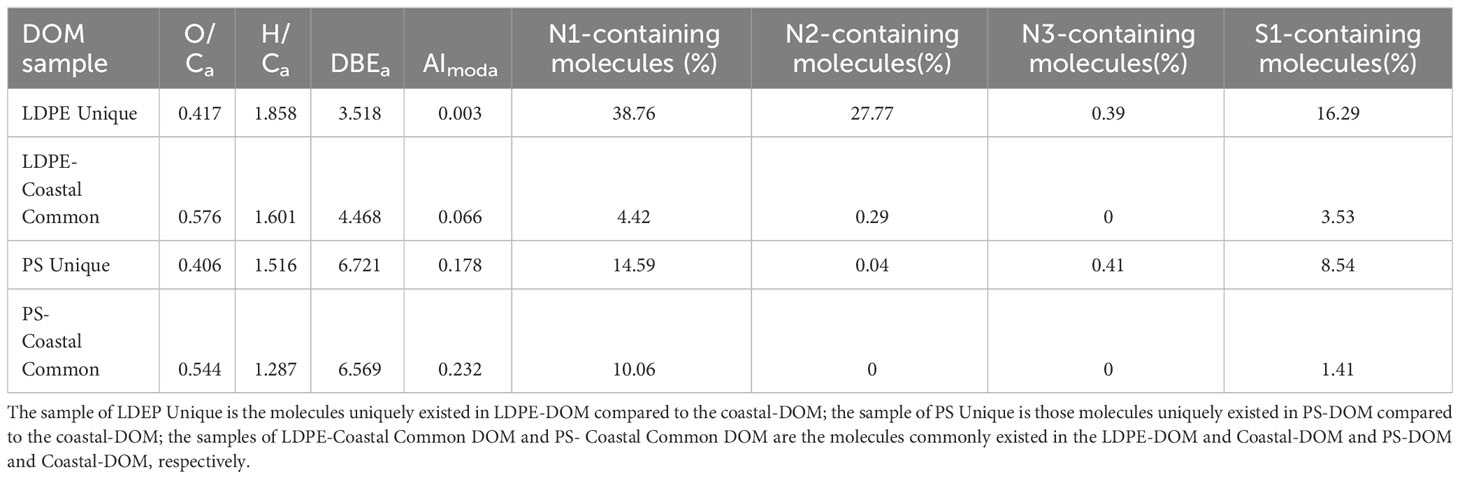
Table 1 Averages of the oxygen to carbon ratios (O/Ca), hydrogen to carbon ratios (H/Ca), double-bound equivalent (DBEa), and a modified aromatic index (AImoda), and relative abundance (%) of one, two, three nitrogen-containing molecules and one sulphur-containing molecules in each sample were calculated.
Compared to the common formulas, the LDPE-DOM unique molecules and PS-DOM unique molecules were characterized as higher abundance of nitrogen- and sulfur-containing molecules (Table 1). Specifically, both the LDPE-DOM unique and PS-DOM unique molecules contained much higher abundance of molecules with one nitrogen containing in a molecule, compared to that in the LDPE-Coastal common DOM and PS-Coastal common DOM. About 0.29% of the LDPE-Coastal common molecules was characterized with two nitrogen atoms containing in a molecule, while none of DOM contained three nitrogen atoms that could be characterized in the LDPE-Coastal common or the PS-Coastal common DOM (Table 1). Overall, the LDPE-DOM contained much higher abundance of heteroatoms-containing DOM molecules than PS-DOM, especially the nitrogen-containing molecules (Table 1). Considering that total dissolved nitrogen concentration was higher in the LDPE-light incubations than that in the PS-light incubations on day 10 (Supplementary Figure 4), and that relatively diversified of additives are generally added to produce polyethylene (Fauser et al., 2022). Thus some heteroatoms-containing molecules in LDPE-DOM could be derived from the plastic additives. If this is true, a microplastic obtained from different origins could differ in additives and contaminants, then resulting in distinct molecular composition in biotic and abiotic plastic degradation experiments. However, this assumption requires experimental evidence in future studies. These heteroatoms containing molecules in the LDPE-DOM unique and PS-DOM unique samples are mostly non-natural DOM molecules (Figure 2) and could have unaccounted consequences for marine microbes and DOM cycling, as a recent study shows an example indicating that solar radiation could result in production of organic acids and CO2 from plastics, further leading to ocean acidification (Romera-Castillo et al., 2023). Future studies are required to investigate the potential effects of those non-natural DOM in aquatic environments.
Bioavailability of the microplastics-derived DOM
Bioavailability of the solar-induced LDPE-DOM and PS-DOM was examined in a coastal seawater microbial assemblage (Supplementary Figure 1), based on the variations in bacterial abundance (Supplementary Figure 5) and DOC concentration (Figure 3) during the 30-day incubation. At the beginning, bacterial abundance in the control was approximately 1.26 ± 0.08 × 106 cells mL-1 on average. It increased in response to the addition of the PS-DOM on day 1 and reached its peak at ~ 5.25 × 107 cells mL-1 on day 4. Bacterial abundance in the treatment of LDPE-DOM started to increase on day 4 and reached its peak at ~ 2.88 × 108 cells mL-1 on day 8. The maximum value of bacterial abundance in the LDPE-DOM amended incubations was approximately 5 times higher than that in the PS-DOM amended incubations. Therefore, although lower diversity of DOM was characterized in the LDPE-DOM, which included a higher proportion of labile DOM, indicated by lower values of AImoda and DBEa but higher average H/Ca (Supplementary Table 1), as well as higher DOC released from LDPE, then stimulating the growth of seawater bacteria. After 15 days, the bacterial abundance in both the treatments of PS-DOM and LDPE-DOM declined (Supplementary Figure 5).
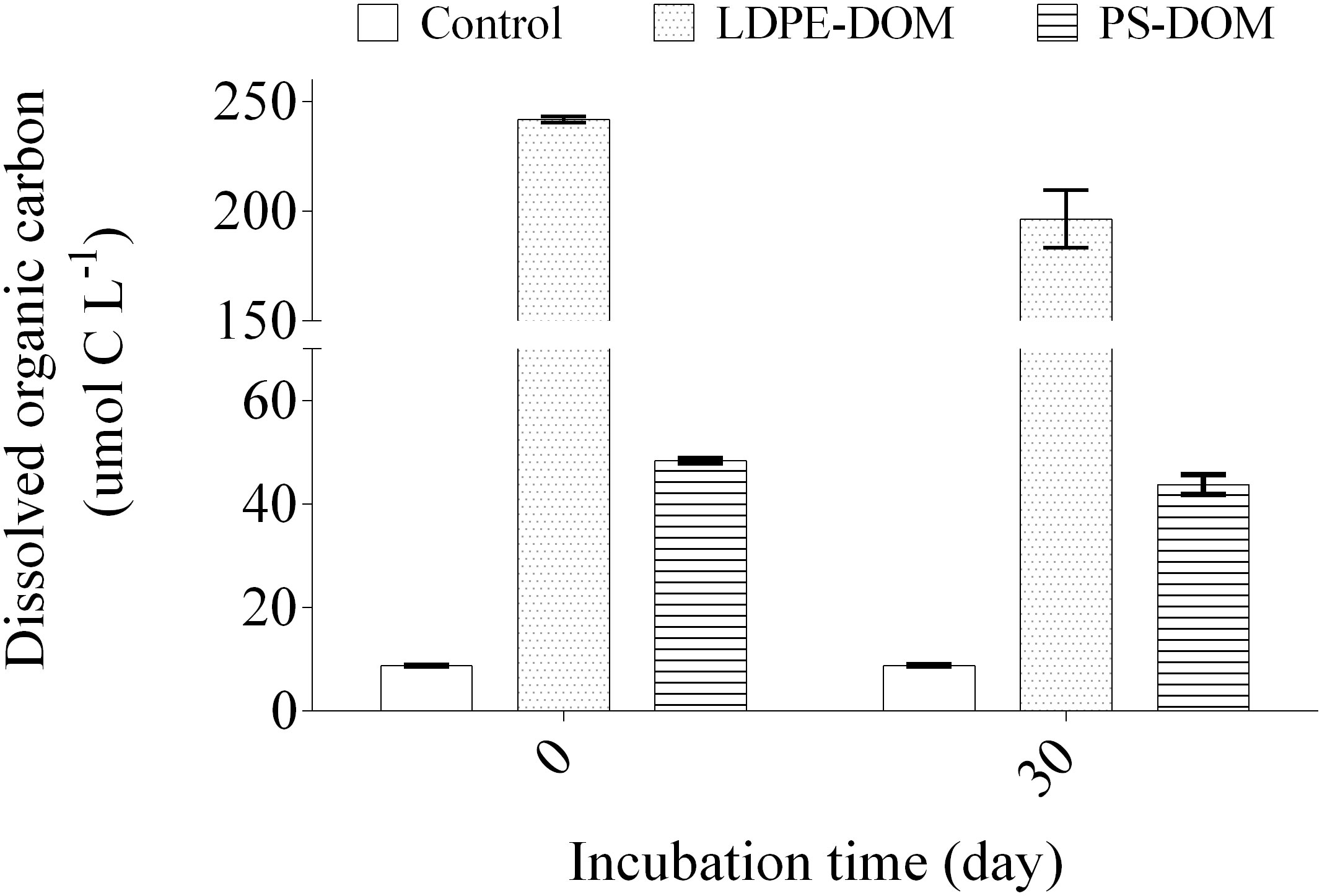
Figure 3 Consumption of DOC in the controls and LDPE- and PS-DOM amended treatments of the coastal seawaters during the 30-day period. The experiment was conducted in duplicate.
The higher bioavailability of LDPE-DOM was also demonstrated by its higher DOC utilization during the incubation. The starting DOC concentration was 241.7 ± 1.44 µmol C L–1 and 48.4 ± 0.41 µmol C L–1 in the LDPE-DOM and PS-DOM amended incubations, respectively. After the 30 days, DOC concentration decreased to 196.5 ± 13.03 µmol C L–1 and 43.8 ± 1.90 µmol C L–1 in the LDPE-DOM and PS-DOM amended incubations, respectively. Nevertheless, DOC concentration in the control remained almost invariant (Figure 3). During this period, approximately 18.7% and 9.5% of the amended DOC were utilized or transformed in the treatments of LDPE-DOM and PS-DOM, respectively. According to a previous definition, the most bioactive DOM could be utilized by heterotrophic bacteria in hours and days (Hansell, 2013), then residue DOM in both the LDPE-DOM and PS-DOM after the 30 days resisted rapid microbial degradation, could be at least of semi-labile DOM. We noted that change of DOM composition was not detected during this bioavailability examination experiment, due to that the inoculation of the costal microbial community brought a suite of natural DOM molecules, which could not be excluded during the extraction of DOM and the measurement of DOM using ESI FT-ICR MS. Nevertheless, the residue DOM molecules in the treatments are likely to be subjected to further biotic and abiotic transformation in the natural water environments, therefore, in-depth experiments and in situ observations are required in future studies.
Conclusion
This study investigated the effects of solar radiation on DOM released from two popular microplastics (LDPE and PS), by measuring quality and quantity of the released DOM and its bioavailability in a coastal seawater microbial community. Our results indicated that solar radiation stimulated release of DOM from the microplastics compared to the dark incubation. During the 10 days’ solar incubation, the average DOC release rates were approximately 19.03 and 3.85 µg C L–1 day-1 from each gram of LDPE and PS, respectively. Analyzed by ultra-high-resolution FT-ICR MS, the solar-induced DOM exhibited different molecular composition between the solar-induced LDPE-DOM and PS-DOM. The LDPE-DOM was characterized in higher of average H/C ratio and lower values of average DBE and aromatic index than that in the PS-DOM, suggesting that the LDPE-DOM molecules were relatively saturated and thus bio-active compared to the PS-DOM. In addition, LDPE-DOM contained relatively high abundance of heteroatoms-containing (mainly nitrogen) molecules than PS-DOM. The further 30 days’ microbial incubation experiment showed that nearly 18.7% of the LDPE-DOM and 9.5% of the PS-DOM were utilized or transformed within this period, demonstrating that the LDPE-DOM was relatively labile in coastal microbial community than the PS-DOM. Moreover, a fraction of the solar-induced DOM resisted rapid microbial degradation, can be defined as semi-labile DOM. In molecular level, a proportion of the assigned formulas in LDPE-DOM (22.3%) and PS-DOM (55.8%) could be found in a coastal-DOM sample. Overall, these experimental results suggested that solar radiation stimulates release of DOM from microplastics, some of the DOM molecules potentially contribute to coastal seawater DOM pool, others could have unaccounted consequences that require further experiments.
Data availability statement
The raw data supporting the conclusions of this article will be made available by the authors, without undue reservation.
Author contributions
JW: Conceptualization, Data curation, Funding acquisition, Writing – original draft. RC: Conceptualization, Methodology, Visualization, Writing – original draft, Writing – review & editing.
Funding
The author(s) declare financial support was received for the research, authorship, and/or publication of this article. This work was supported by the High-level talents project 2019 of Xiamen Ocean Vocational College: Development and application of technology for detection of coastal microplastics in Xiamen.
Conflict of interest
The authors declare that the research was conducted in the absence of any commercial or financial relationships that could be construed as a potential conflict of interest.
Publisher’s note
All claims expressed in this article are solely those of the authors and do not necessarily represent those of their affiliated organizations, or those of the publisher, the editors and the reviewers. Any product that may be evaluated in this article, or claim that may be made by its manufacturer, is not guaranteed or endorsed by the publisher.
Supplementary material
The Supplementary Material for this article can be found online at: https://www.frontiersin.org/articles/10.3389/fmars.2023.1284280/full#supplementary-material
References
Andrady A. L. (2011). Microplastics in the marine environment. Mar. Pollut. Bull. 62, 1596–1605. doi: 10.1016/j.marpolbul.2011.05.030
Arp H. P. H., Kuehnel D., Rummel C., Macleod M., Potthoff A., Reichelt S., et al. (2021). Weathering plastics as a planetary boundary threat: exposure, fate, and hazards. Environ. Sci. Technol. 55, 7246–7255. doi: 10.1021/acs.est.1c01512
Birnstiel S., Sebastian M., Romera-Castillo C. (2022). Structure and activity of marine bacterial communities responding to plastic leachates. Sci. Total Environ. 834, 155264. doi: 10.1016/j.scitotenv.2022.155264
Bostick K. W., Zimmerman A. R., Goranov A. I., Mitra S., Hatcher P. G., Wozniak A. S. (2021). Biolability of fresh and photodegraded pyrogenic dissolved organic matter from laboratory-prepared chars. J. Geophysical Research-Biogeosciences 126, e2020JG005981. doi: 10.1029/2020JG005981
Cai R., Jiao N. (2023). Recalcitrant dissolved organic matter and its major production and removal processes in the ocean. Deep Sea Res. Part I: Oceanographic Res. Papers 191, 103922. doi: 10.1016/j.dsr.2022.103922
Cai R., Zhou W., He C., Tang K., Guo W., Shi Q., et al. (2019). Microbial processing of sediment-derived dissolved organic matter: implications for Its subsequent biogeochemical cycling in overlying seawater. J. Geophysical Research: Biogeosciences 124, 3479–3490. doi: 10.1029/2019JG005212
Cunningham E. M., Ehlers S. M., Dick J. T. A., Sigwart J. D., Linse K., Dick J. J., et al. (2020). High abundances of microplastic pollution in deep-sea sediments: evidence from Antarctica and the Southern Ocean. Environ. Sci. Technol. 54, 13661–13671. doi: 10.1021/acs.est.0c03441
Delacuvellerie A., Cyriaque V., Gobert S., Benali S., Wattiez R. (2019). The plastisphere in marine ecosystem hosts potential specific microbial degraders including Alcanivorax borkumensis as a key player for the low-density polyethylene degradation. J. Hazardous Materials 380, 120899. doi: 10.1016/j.jhazmat.2019.120899
Dittmar T., Koch B., Hertkorn N., Kattner G. (2008). A simple and efficient method for the solid-phase extraction of dissolved organic matter (SPE-DOM) from seawater. Limnology Oceanography: Methods 6, 230–235. doi: 10.4319/lom.2008.6.230
Fauser P., Vorkamp K., Strand J. (2022). Residual additives in marine microplastics and their risk assessment-A critical review. Mar. Pollut. Bull. 177, 113467. doi: 10.1016/j.marpolbul.2022.113467
Flerus R., Lechtenfeld O. J., Koch B. P., Mccallister S. L., Schmitt-Kopplin P., Benner R., et al. (2012). A molecular perspective on the ageing of marine dissolved organic matter. Biogeosciences 9, 1935–1955. doi: 10.5194/bg-9-1935-2012
Guo X., Wang J. (2019). The chemical behaviors of microplastics in marine environment: A review. Mar. pollut. Bull. 142, 1–14. doi: 10.1016/j.marpolbul.2019.03.019
Hansell D. A. (2013). Recalcitrant dissolved organic carbon fractions. Annu. Rev. Mar. Sci. 5, 421–425. doi: 10.1146/annurev-marine-120710-100757
He C., He D., Chen C., Shi Q. (2022). Application of Fourier transform ion cyclotron resonance mass spectrometry in molecular characterization of dissolved organic matter. Sci. China-Earth Sci. 65, 2219–2236. doi: 10.1007/s11430-021-9954-0
He C., Yi Y., He D., Cai R., Chen C., Shi Q. (2023). Molecular composition of dissolved organic matter across diverse ecosystems: Preliminary implications for biogeochemical cycling. J. Environ. Manage. 344, 118559. doi: 10.1016/j.jenvman.2023.118559
He C., Zhang Y., Li Y., Zhuo X., Li Y., Zhang C., et al. (2020). In-House standard method for molecular characterization of dissolved organic matter by FT-ICR Mass Spectrometry. ACS Omega 5, 11730–11736. doi: 10.1021/acsomega.0c01055
Jambeck J. R., Geyer R., Wilcox C., Siegler T. R., Perryman M., Andrady A., et al. (2015). Plastic waste inputs from land into the ocean. Science 347, 768–771. doi: 10.1126/science.1260352
Kester D. R., Duedall I. W., Connors D. N., Pytkowicz R. M. (1967). Preparation of articifial seawater. Limnology Oceanography 12, 176–179. doi: 10.4319/lo.1967.12.1.0176
Kim S., Kim D., Jung M.-J., Kim S. (2020). Analysis of environmental organic matters by Ultrahigh-Resolution mass spectrometry-A review on the development of analytical methods. Mass Spectrometry Rev. 41, 352–369. doi: 10.1002/mas.21684
Koch B. P., Dittmar T. (2006). From mass to structure: an aromaticity index for high-resolution mass data of natural organic matter. Rapid Commun. Mass Spectrometry 20, 926–932. doi: 10.1002/rcm.2386
Koch B. P., Dittmar T. (2016). From mass to structure: an aromaticity index for high-resolution mass data of natural organic matter (vol 20, pg 926, 2006). Rapid Commun. Mass Spectrometry 30, 250–250. doi: 10.1002/rcm.7433
Kujawinski E. B., Behn M. D. (2006). Automated analysis of electrospray ionization Fourier transform ion cyclotron resonance mass spectra of natural organic matter. Analytical Chem. 78, 4363–4373. doi: 10.1021/ac0600306
Kvale K., Oschlies A. (2023). Recovery from microplastic-induced marine deoxygenation may take centuries. Nat. Geosci. 16, 10–12. doi: 10.1038/s41561-022-01096-w
Lechtenfeld O. J., Hertkorn N., Shen Y., Witt M., Benner R. (2015). Marine sequestration of carbon in bacterial metabolites. Nat. Commun. 6, 6711. doi: 10.1038/ncomms7711
Lee Y. K., Murphy K. R., Hur J. (2020). Fluorescence signatures of dissolved organic matter leached from microplastics: polymers and additives. Environ. Sci. Technol. 54, 11905–11914. doi: 10.1021/acs.est.0c00942
Li Y., Lu Z., Abrahamsson D. P., Song W., Yang C., Huang Q., et al. (2022). Non-targeted analysis for organic components of microplastic leachates. Sci. Total Environ. 816, 151598. doi: 10.1016/j.scitotenv.2021.151598
Li H., Zhang Z., Xiong T., Tang K., He C., Shi Q., et al. (2022). Carbon sequestration in the form of recalcitrant dissolved organic carbon in a seaweed (Kelp) farming environment. Environ. Sci. Technol. 56, 9112–9122. doi: 10.1021/acs.est.2c01535
Lian J., Zheng X., Zhuo X., Chen Y.-L., He C., Zheng Q., et al. (2021). Microbial transformation of distinct exogenous substrates into analogous composition of recalcitrant dissolved organic matter. Environ. Microbiol. 23, 2389–2403. doi: 10.1111/1462-2920.15426
Ling S. D., Sinclair M., Levi C. J., Reeves S. E., Edgar G. J. (2017). Ubiquity of microplastics in coastal seafloor sediments. Mar. Pollut. Bull. 121, 104–110. doi: 10.1016/j.marpolbul.2017.05.038
Luo Y., Sun C., Li C., Liu Y., Zhao S., Li Y., et al. (2022). Spatial patterns of microplastics in surface seawater, sediment, and sand along Qingdao coastal environment. Front. Mar. Sci. 9, 916859. doi: 10.3389/fmars.2022.916859
Martellini T., Guerranti C., Scopetani C., Ugolini A., Chelazzi D., Cincinelli A. (2018). A snapshot of microplastics in the coastal areas of the Mediterranean Sea. Trac-Trends Analytical Chem. 109, 173–179. doi: 10.1016/j.trac.2018.09.028
Medeiros P. M., Seidel M., Powers L. C., Dittmar T., Hansell D. A., Miller W. L. (2015). Dissolved organic matter composition and photochemical transformations in the northern North Pacific Ocean. Geophysical Res. Lett. 42, 863–870. doi: 10.1002/2014GL062663
Mishra S., Swain S., Sahoo M., Mishra S., Das A. P. (2022). Microbial colonization and degradation of microplastics in aquatic ecosystem: A review. Geomicrobiology J. 39, 259–269. doi: 10.1080/01490451.2021.1983670
Mopper K., Zhou X., Kieber R. J., Kieber D. J., Sikorski R. J., Jones R. D. (1991). Photochemical degradation of dissolved organic carbon and its impact on the oceanic carbon cycle. Nature 353, 60–62. doi: 10.1038/353060a0
Onink V., Wichmann D., Delandmeter P., Van Sebille E. (2019). The role of ekman currents, geostrophy, and stokes drift in the accumulation of floating microplastic. J. Geophysical Research-Oceans 124, 1474–1490. doi: 10.1029/2018JC014547
Ou D., Ni Y., Li W., He W., Wang L., Huang H., et al. (2023). Psychrobacter species enrichment as potential microplastic degrader and the putative biodegradation mechanism in Shenzhen Bay sediment, China. J. hazardous materials 464, 132971. doi: 10.1016/j.jhazmat.2023.132971
Romera-Castillo C., Birnstiel S., Alvarez-Salgado X. A., Sebastian M. (2022a). Aged pastic leaching of dissolved organic matter is two orders of magnitude higher than virgin plastic leading to a strong uplift in marine microbial activity. Front. Mar. Sci. 9, 861557. doi: 10.3389/fmars.2022.861557
Romera-Castillo C., Lucas A., Mallenco-Fornies R., Briones-Rizo M., Calvo E., Pelejero C. (2023). Abiotic plastic leaching contributes to ocean acidification. Sci. Total Environ. 854, 158683. doi: 10.1016/j.scitotenv.2022.158683
Romera-Castillo C., Mallenco-Fornies R., Saa-Yanez M., Alvarez-Salgado X. A. (2022b). Leaching and bioavailability of dissolved organic matter from petrol-based and biodegradable plastics. Mar. Environ. Res. 176, 105607. doi: 10.1016/j.marenvres.2022.105607
Romera-Castillo C., Pinto M., Langer T. M., Alvarez-Salgado X. A., Herndl G. J. (2018). Dissolved organic carbon leaching from plastics stimulates microbial activity in the ocean. Nat. Commun. 9, 1430. doi: 10.1038/s41467-018-03798-5
Shi W., Zhuang W.-E., Hur J., Yang L. (2021). Monitoring dissolved organic matter in wastewater and drinking water treatments using spectroscopic analysis and ultra-high resolution mass spectrometry. Water Res. 188, 116406. doi: 10.1016/j.watres.2020.116406
Soursou V., Campo J., Pico Y. (2023). A critical review of the novel analytical methods for the determination of microplastics in sand and sediment samples. Trends Analytical Chem. 166, 117190. doi: 10.1016/j.trac.2023.117190
Stedmon C. A., Markager S., Tranvik L., Kronberg L., Slatis T., Martinsen W. (2007). Photochemical production of ammonium and transformation of dissolved organic matter in the Baltic Sea. Mar. Chem. 104, 227–240. doi: 10.1016/j.marchem.2006.11.005
Stubbins A., Zhu L., Zhao S., Spencer R. G. M., Podgorski D. C. (2023). Molecular signatures of dissolved organic matter generated from the photodissolution of microplastics in sunlit seawater. Environ. Sci. Technol. 57, 20097–20106. doi: 10.1021/acs.est.1021c03592
Thompson R. C., Olsen Y., Mitchell R. P., Davis A., Rowland S. J., John A. W. G., et al. (2004). Lost at sea: Where is all the plastic? Science 304, 838–838. doi: 10.1126/science.1094559
Villarrubia-Gomez P., Cornell S. E., Fabres J. (2018). Marine plastic pollution as a planetary boundary threat - The drifting piece in the sustainability puzzle. Mar. Policy 96, 213–220. doi: 10.1016/j.marpol.2017.11.035
Wu X., Liu P., Shi H., Wang H., Huang H., Shi Y., et al. (2021). Photo aging and fragmentation of polypropylene food packaging materials in artificial seawater. Water Res. 188, 116456. doi: 10.1016/j.watres.2020.116456
Xu Y., Huang D., Liu P., Ouyang Z., Jia H., Guo X. (2022). The characteristics of dissolved organic matter release from UV-aged microplastics and its cytotoxicity on human colonic adenocarcinoma cells. Sci. Total Environ. 826, 154177. doi: 10.1016/j.scitotenv.2022.154177
Yaseen A. A., Al-Tikrity E. T. B., Yousif E., Ahmed D. S., Kariuki B. M., El-Hiti G. A. (2021). Effect of ultraviolet irradiation on polystyrene containing cephalexin schiff bases. Polymers 13, 2982. doi: 10.3390/polym13172982
Yi Y., He C., Klaproth K., Merder J., Li P., Qi Y., et al. (2023). Will various interpretation strategies of the same ultrahigh-resolution mass spectrometry data tell different biogeochemical stories? A first assessment based on natural aquatic dissolved organic matter. Limnology Oceanography: Methods 21, 320–333. doi: 10.1002/lom3.10548
Keywords: microplastic, DOC, DOM, photochemical degradation, solar radiation, coastal seawater
Citation: Wang J and Cai R (2023) Solar radiation stimulates release of semi-labile dissolved organic matter from microplastics. Front. Mar. Sci. 10:1284280. doi: 10.3389/fmars.2023.1284280
Received: 28 August 2023; Accepted: 06 December 2023;
Published: 19 December 2023.
Edited by:
Hernando Bacosa, Mindanao State University, PhilippinesReviewed by:
Kaijun Lu, The University of Texas at Austin, United StatesShuting Liu, Kean University, United States
Copyright © 2023 Wang and Cai. This is an open-access article distributed under the terms of the Creative Commons Attribution License (CC BY). The use, distribution or reproduction in other forums is permitted, provided the original author(s) and the copyright owner(s) are credited and that the original publication in this journal is cited, in accordance with accepted academic practice. No use, distribution or reproduction is permitted which does not comply with these terms.
*Correspondence: Jun Wang, d2FuZ2p1bkB4bW9jLmVkdS5jbg==; Ruanhong Cai, Y3JoMTk4N0AxNjMuY29t
†These authors have contributed equally to this work