- 1Department of Biological Sciences, Faculty of Sciences, King Abdulaziz University, Jeddah, Saudi Arabia
- 2Marine Natural Products Research Unit, King Fahad Medical Research Centre, King Abdulaziz University, Jeddah, Saudi Arabia
- 3Department of Marine Biology, Faculty of Marine Sciences, King Abdulaziz University, Jeddah, Saudi Arabia
- 4Immunology Unit, King Fahd Medical Research Centre, King Abdulaziz University, Jeddah, Saudi Arabia
Nowadays there is a growing trend towards carotenoids obtained from natural sources such as microalgae. Dunaliella salina is among the most significant natural sources of beta-carotene for commercial scale, which is used in many food industries. Enzymatic extraction of beta-carotene is one of the safe methods that ensure that the food product is not contaminated compared to ex-traction using solvents. In this study, beta-carotene was extracted using two methods: the organic solvent method by tetrahydrofuran and the enzymatic extraction using a bacterial lipase enzyme. The alga was exposed to different stress conditions (salinity/nitrogen) for increasing the beta-carotene production. The highest value of beta-carotene content was recorded in the extraction method using tetrahydrofuran (109.008 µg/mL at 2.5M NaCl/0.5 g/L KNO3), compared to the extraction method using lipase enzyme (19.13 µg/mL at 1 mg/mL at 24 h exposure time). However, the beta-carotene yield slightly increased (21.4 µg/mL) in lipase enzyme extraction method for the algal samples exposed to more than 24 h under different conditions (3M NaCl/1g/L KNO3). The results show that the enzymatic extraction method is less efficient in extracting beta-carotene compared to the tetrahydrofuran solvent extraction method, with the latter showing a higher content of extracted beta-carotene.
1 Introduction
Microalgae constitute a diverse category of tiny organisms that have the ability to carry out photosynthesis, they include unicellular prokaryotic and eukaryotic organisms (Khan et al., 2018; Bonnefond et al., 2024). They are vastly distributed on land and in the sea and various environments, in addition to being an important source rich in nutrients (Barsanti et al., 2008; Weinrich et al., 2019; Yuan et al., 2023). In recent decades there has been an increasing interest in obtaining products from natural sources, including carotenoids. Carotenoids are one of the bioactive products used for the purpose of producing functional foods and these compounds can be acquired from microalgae (Dhandwal et al., 2024; Sousa et al., 2023). Beta-carotene is among the most significant carotenoid compounds, it is also considered an excellent additive for many food and cosmetic manufacturing processes due to its attractive color and its possession of many functional properties (Zarandi-Miandoab et al., 2019). On a commercial scale, the microalgae Dunaliella salina is one of the most important sources of beta-carotene, in addition to some other microalgae species (Monte et al., 2020; Ntzouvaras, 2021; Sousa et al., 2023). In addition, natural sources of beta-carotene extracted from microalgae have many distinctive properties compared to chemically manufactured beta-carotene (Sousa et al., 2024). Natural beta-carotene is characterized by its mixture of all-trans and 9 cis isomers, which makes it more accessible and has antioxidant properties compared to synthetic beta-carotene, which contains only all-trans isomers (Monte et al., 2020). Naturally produced beta-carotene has many properties such as antioxidants, anti-cancer (Balaji and Roy, 2020), and anti-aging properties (Honda, 2023), it also helps control cholesterol levels and thus reduces the risk of cardiovascular disease (Amengual et al., 2020), it is also better absorbed than the synthetic beta-carotene product. Despite the benefits provided by the natural beta-carotene, there is a need for more efforts to make it commercially, and this in turn has attracted the attention of researchers to conduct more approaches including the use of enzymes such as bacterial lipase to extract this compound with great efficiency (Ribeiro et al., 2011; Sangkharak et al., 2011). Many factors affect carotenoid production in microalgae, such as light, salinity, temperature, nutrients, and other environmental factors (Chen et al., 2024).
D. salina is a single-celled green microalgae commonly found in saline environments and used in many biotechnological applications such as health, pharmaceutical, energy, and food industries (El-Baz et al., 2019; Pereira et al., 2024). D. salina is a eukaryotic organism that tolerates elevated salinity conditions and can thrive in salt concentrations varying from 0.05 to 5.5M NaCl (Borowitzka, 2013b). Among the biotechnological applications, the microalga D. salina is used in foods as a nutritional supplement (human and animals) with valuable benefits (Yücel et al., 2021; Alghamdi et al., 2024; Williamson et al., 2023). D. salina has also been used as an ingredient in animal feed, including farmed aquatic species such as fish and shrimp, and has also been used as feed for poultry and livestock such as sheep and goats (Ritu et al., 2023; de Moraes et al., 2022; Valente et al., 2021).
Recently, many D. salina products have been incorporated into human food products and entered bakery foods (biscuits and cakes) and dairy products in order to obtain added nutritional values (da Silva et al., 2021; Bhatnagar et al., 2024). The use of compounds of this alga has also been applied in the manufacture of cosmetics, and skin care products such as anti-aging creams, sunscreen creams, facial lotions, body soaps, and hair care products such as shampoo. It has also been used in makeup products such as eye shadow and lipstick (Stolz and Obermayer, 2005; Havas et al., 2022; Çelebi et al., 2021).
In the years 2010 - 2018, global demand reached a high level, with the market value of beta-carotene reaching about $261-$334 million annually (Zarandi-Miandoab et al., 2019). The production of beta-carotene derived from microalgae has received significant attention due to its potential as a sustainable and economical alternative to many synthetic sources (Khan et al., 2018). D. salina contains about 14% of the dry weight of beta-carotene, and this percentage can increase to greater than that when optimal nutritional conditions and other growth requirements are created (Young and Lowe, 2018; Çalışlar, 2019; Monte et al., 2020). Furthermore, D. salina has emerged as one of the most important species of microalgae for the bioproduction of beta-carotene, as it is expected to provide the production of more than 95% of the overall requirement for this compound (Becker, 2007; Zarandi-Miandoab et al., 2019).The ability to produce beta-carotene from microalgae of the genus Dunaliella has been estimated at approximately 1,200 tons annually (Grima et al., 2003; Borowitzka, 2013a).
Beta-carotene in green algae is usually stored in lipid droplets and chloroplasts. In D. salina, it is stored in lipid droplets surrounded by a phospholipid monolayer (Dixon and Wilken, 2018). D. salina is distinguished by the presence of a wall-free cell structure. Therefore, the absence of a rigid cell wall in D. salina facilitates the extraction process of the beta-carotene compound, as enzymes are used directly to extract carotenoid compounds efficiently (Asevedo et al., 2023). The use of a lipase approach is particularly effective in D. salina, which selectively digests the enveloping membrane of lipid droplets, which in turn extracts beta-carotene molecules. This approach highlights the necessity of adapting extraction techniques to the morphological characteristics and structural composition of the studied species (Dixon and Wilken, 2018). The present study aims to extract beta-carotene using bacterial lipase enzyme from D. salina which was isolated from the local aquatic environment in Jeddah on the Red Sea coast of Saudi Arabia and grown under different salinity and nitrogen levels in the laboratory. Also, the efficiency of the extraction of carotenoid by bacterial lipase enzyme was compared with the traditional method using the organic solvent tetrahydrofuran (THF).
2 Materials and methods
2.1 Samples collection
D. salina samples were collected from two stations on the Al-Shuaibah coast, Red Sea, Saudi Arabia. The coordinates of station 1 and station 2 were 20°42’56”N 39°30’42” E and 20°42’38”N 39°30’34” E respectively.
2.2 Microalgae culture
After the initial isolation of D. salina, it was cultured with F/2 medium according to the recipe of Guillard (1975). The cultured D. salina was transferred to a synthetic Johnson (J/1) medium according to Johnson et al. (1968), in order to improve beta-carotene production. The Johnson medium consists of the following components (g/L): 1.5g MgCl2-6H2o, 0.5g MgSo4.7H2o, 0.2g KCI, 0.2g CaCI2, 1.0g KNO3, 0.043g NaHCO3, 2.45g tris(hydroxymethyl) aminomethane (Tris), 0.035g KH2PO4, 1.89 mg ethylenediaminetetraacetate, 2.44mg FeC13.6H2O, 0.041mg, ZnCl2, 0.61mg H3BO3, 0.041mg VOC12, 0.015mg CoCl2.6H2O, 0.38mg (NH4)6Mo7O24.4H2O, 0.041mg CuC12.2H2O), and 0.41mg MnCI2.4H2O. The final pH was adjusted to 7.5. The medium was autoclaved at 121°C for 20 min. D. salina was cultured in 1 L flask in J/1 medium, under autotrophic conditions, with the flasks supplied with an airflow of 600 ml/min with a constant white light intensity of 2000 lux with a CO2 flow of 4 ml/min and a salinity of 1M NaCl. After 15 days of enrichment, the algae underwent various beta-carotene extraction experiments.
2.3 Experimental design
The experimental framework of this study was set up in two stages as follow; In the first stage, the optimum conditions to produce beta-carotene from D. salina algae at different concentrations of salinity (2.5, 3, 4, and 5M NaCl) and nitrogen (0.25, 0.5, and 1 g/L KNO3) were determined as reported previously (Sathasivam et al., 2018). In the second stage, an organic solvent (tetrahydrofuran) and lipase enzyme were used for extracting beta-carotene from the alga.
2.4 Biomass measurements
2.4.1 Cell volume
The cell volume of the algal cells was measured at the time of harvesting based on measurements of the cell length and width, and the spheroid object equation was used because it is the most appropriate for the shape of the algae cells. The CV was calculated according to the following equation:
Where (π) = 3.14, (W) denotes the cell’s width, and (L) is the length of the cell.
Measurements were performed on approximately 40 active cells randomly withdrawn from growth flasks during the later stage of exponential growth during which the cells were observed and measured with an inverted microscope (Olympus IX-71).
2.4.2 Growth rate
The growth rate of D. salina was measured by determining the abundance of the algae using a Beckman Coulter Counter Multisizer™3. All measurements were made in triplicate and then the GR was calculated according to the following equation:
Where N is the number of cells, Δ(In N) is the change in the natural logarithm of the number of cells and Δt is the change in time (d).
2.4.3 Dry weight
The DW of alga cultures was determined according to Chi et al. (2016). This measurement was performed in the late exponential stage of algal growth in three replicates of the experiment. The culture broth (30 ml) was filtered using a glass microfiber filter (Dorsan FV-130, 47 mm). After filtration, it was washed three times with 0.5M ammonium bicarbonate to remove any remaining media or salts after filtration. The samples were dried at 60°C for 16 h until the weight was constant. The DW of algae was determined based on the difference between the final weight of the filtered sample and the initial weight of the filter paper. The DW of the samples was determined using the following equation:
Where (W) is the weight of the final filter with samples and (W1) is the weight of the initial filter.
2.4.4 Productions
The production efficiency of D. salina culture was determined by calculating the production per dry weight, where the calculation relies on the growth rate and the average DW of the algae cultures. The production was calculated using the following equation:
Where (μ) represents the microalgae growth rate cultures, expressed in divisions per day (d-¹). DW mg/mL average number of DW.
2.5 Beta-carotene extraction
2.5.1 Extraction of beta-carotene by organic solvent THF
Beta-carotene was determined and extracted from D. salina culture using the organic solvent THF according to Hejazi and Wijffels (2003). Algal culture broth (2 ml) was taken and mixed well. The samples were centrifuged at 4000 rpm for 5 min, then the upper phase was carefully poured off, and 2 ml of THF was added to the biomass. The samples were mixed well using a vortex for 2–3 minutes to achieve complete extraction of beta-carotene. The samples were centrifuged again at 4000 rpm for 5 min to separate the colorless D. salina and the solvent phase containing the extracted pigments. The quantity of beta-carotene extracted from the algae was determined by UV/Visible spectrophotometer at 450 nm according to the following equation:
Where A450 is the value of absorbance measured at wavelength 450 nm.
2.5.2 Extraction of beta-carotene by lipase enzyme
Commercially available lipase enzyme was purchased from Sigma-Aldrich®. The lipase enzyme was from the bacteria Pseudomonas cepacia according to manufacturer’s product data. This enzyme was chosen because of its known efficiency in lipid hydrolysis. The enzyme was stored at a temperature ranging from 2-8°C in order to maintain the activity and stability of the enzyme. Lipase enzyme was used at different concentrations (0.5,1, and 1.5 mg/mL) for beta-carotene extraction experiments. The enzyme was dissolved in distilled water to prepare the different concentrations.
Beta-carotene extraction was carried out using lipase enzyme. The culture broth (2 ml) was taken and then centrifuged at 4000 rpm for 5 min. The upper phase was carefully separated and then the organic phase of the algae biomass was suspended in 3.25 ml of the previously prepared enzyme solution at different concentrations (0.5, 1, and 1.5 mg/mL). Then, 7.5 ml of a phosphate buffer solution (0.1M, pH 7.0) was added to the suspension to facilitate the enzymatic lysis of the cells. The mixture was incubated in a shaking water bath at 37°C and 100 rpm for different periods (6, 12, 24 h). After incubation, the biomass was separated by centrifuge at 4000 rpm for 5 min, and then beta-carotene concentrations were determined using the spectrophotometric method as described in the previous section.
2.6 Statistical analysis
Data statistical analysis was conducted using SPSS (v. 27) software. Data were evaluated by one-way analysis of variance (ANOVA) test, followed by Tukey’s HSD test for all biomass characteristics such as growth rate, cell size, dry weight, production per dry weight, and β-carotene content yield across different salinity levels and nitrogen concentrations. Also, Two-way ANOVA (concentrations and exposure times with beta-carotene content) was also performed for the beta-carotene extraction method using lipase enzyme. For statistical tests, P ≤ 0.05 was considered significant. All experiments were performed in three repetitions.
3 Results and discussion
3.1 Cell volume
Two growth phases of the D. salina algae were determined, where the experiments for the first phase focused on growth under optimal conditions such as light intensity 2000 lux at 25°C, salinity 1M, and pH 7.5 in J/1 medium. Under optimal conditions, the local D. salina isolates maintained its characteristics such as the typical oval shape, green color, and other characteristics such as the presence of one chloroplast, two flagella, and red stigma. This in turn was consistent with a previous study by Yaiche-Achour et al. (2018), who observed that the shape of microalgal cells changes according to different culture conditions. Figure 1 shows the CV of D. salina under different conditions. The CV of the of D. salina was about 879.24 µm³ under optimal growth conditions. In the second stage of experiments under stress conditions of salinity and nitrogen, which included salinity levels of (2.5, 3M) and different concentrations of nitrogen (0.25, 0.5, 1 g/l KNO3), the cells transitioned in color from green to brown, and their shape changed to circular, with the disappearance of the flagella, and also a change in the color of the culture broth to orange. Highfield et al. (2021) documented the ability of D. salina to change cell volume in response to changes in growth conditions such as light intensity, salinity, and nutrient levels. Osmotic stress also causes rapid cell shape changes in D. salina due to its flexible cell membrane and lack of a rigid cell wall (Oliveira et al., 1980; Xu et al., 2016). The restricted capacity to alter cell size and shape depends on dissolved organic matter such as proline and glycerol for osmoregulation (Lauritano et al., 2020). Under stress conditions of salinity levels/nitrogen concentration (KNO3), the results of this study recorded the cell volume of D. salina alga to be about 396.59, 458.76, and 462.41 µm³ at different conditions of salinity levels/nitrogen concentrations 2.5M/0.25, 2.5M/0.5 and 2.5M/1 g/L KNO3 respectively. When the salinity levels increased to 3M, the values of cell volume were 289.58, 314.75, and 383.97 µm³ at 3M/0.25, 3M/0.5, and 3M/1 g/L KNO3, respectively. The highest value of cell volume (462.41 µm³) was recorded at the salinity and nitrogen concentration level of 2.5M/1 g/L KNO3. In contrast, a smaller cell volume was observed at the salinity level of 3M. A significant decrease in cell volume was recorded when the salinity level increased to 3M and the nitrogen concentration decreased to 0.25 g/L KNO3, which reached about 289.58 µm³ (Supplementary Table S1). Gallego-Cartagena et al. (2019) reported changes in the cell volume of D. salina alga at different salinity concentrations and exposure to osmotic changes. At salinity concentrations of 4 and 5 M the culture turned colorless within two days of incubation. Sathasivam et al. (2018) reported the growth of D. salina at salinity concentrations (3.5, 4, 5 M) where it turned colorless. In another study by Ravi et al. (2012) the growth of D. salina cells at concentrations higher than 3 M resulted in the occurrence of cell bleaching.
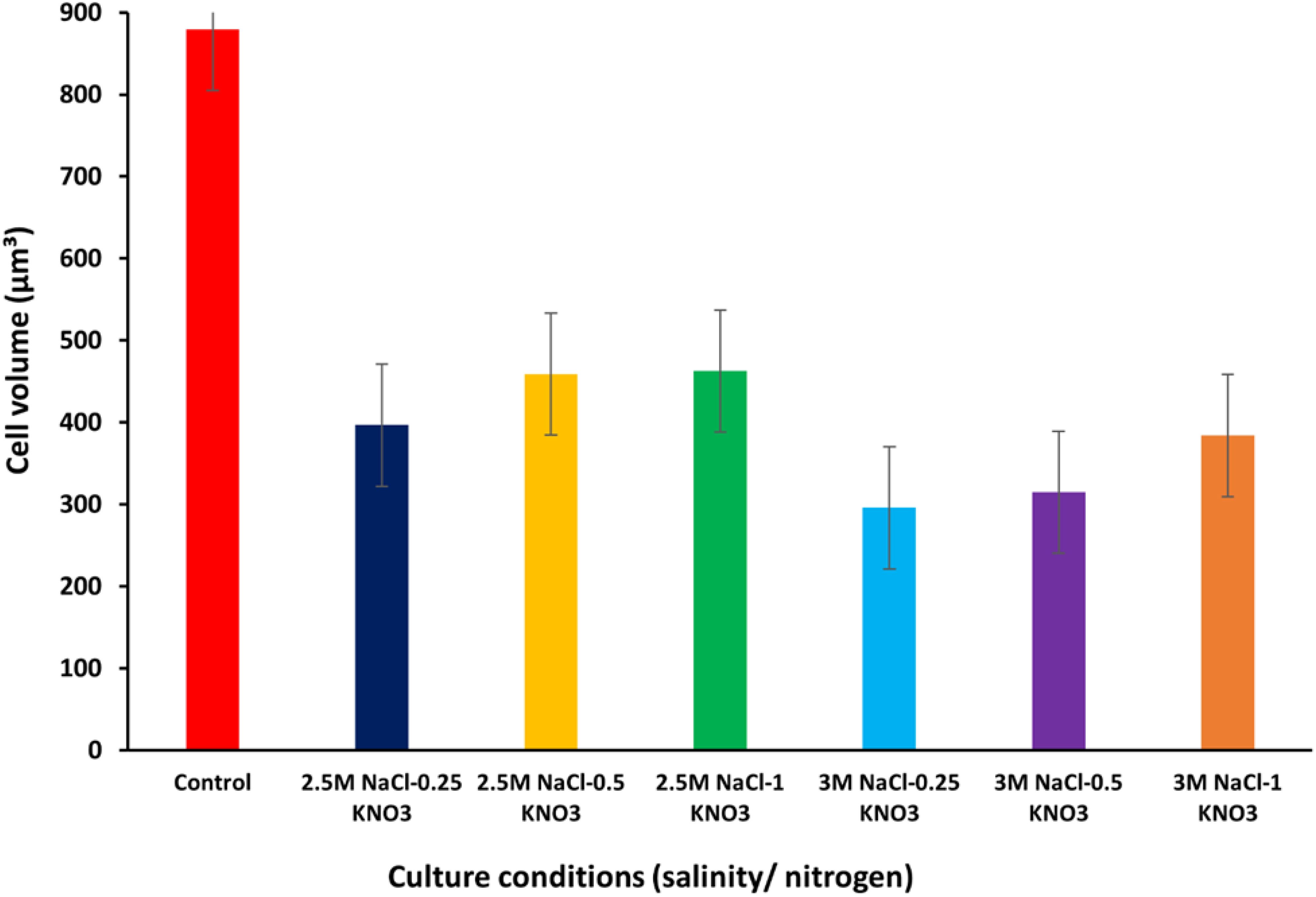
Figure 1. Cell volume values of D. salina under different conditions (salinity/nitrogen). Bars are standard error of mean.
3.2 Growth rate
Figure 2 shows the GR of local D. salina under different conditions. The growth rate value of D. salina was calculated to be about 0.30 d-¹ under optimum conditions during the first stage of growth. When D. salina was exposed to stress conditions (salinity levels/nitrogen concentration), a decline in growth rates was observed, where the highest value was about 0.23 d-¹ at a salinity level of 2.5M NaCl and an optimum nitrogen concentration of 1 g/L KNO3, while the rest of the growth rate values were low at this level of salinity and different conditions of nitrogen concentration. At 2.5M salinity level, growth rates were recorded as 0.17, 0.22, and 0.23 d-¹ at different nitrogen concentrations of 0.25, 0.5, and 1 g/L KNO3, respectively. At 3M salinity level, a decrease in growth rate values was observed, reaching about 0.13, 0.15, and 0.15 d-¹ at different nitrogen concentrations 0.25, 0.5, and 1 g/L KNO3, respectively, with a close convergence between the growth rate value at nitrogen concentrations 0.5 and 1 g/L KNO3 (Supplementary Table S1). Both Arun and Singh (2013) and Sui et al. (2019) reported growth rates of D. salina of about 0.12 and 0.44 ± 0.02 d-¹, respectively, which confirms that our results are consistent with the value ranges reported in these studies.
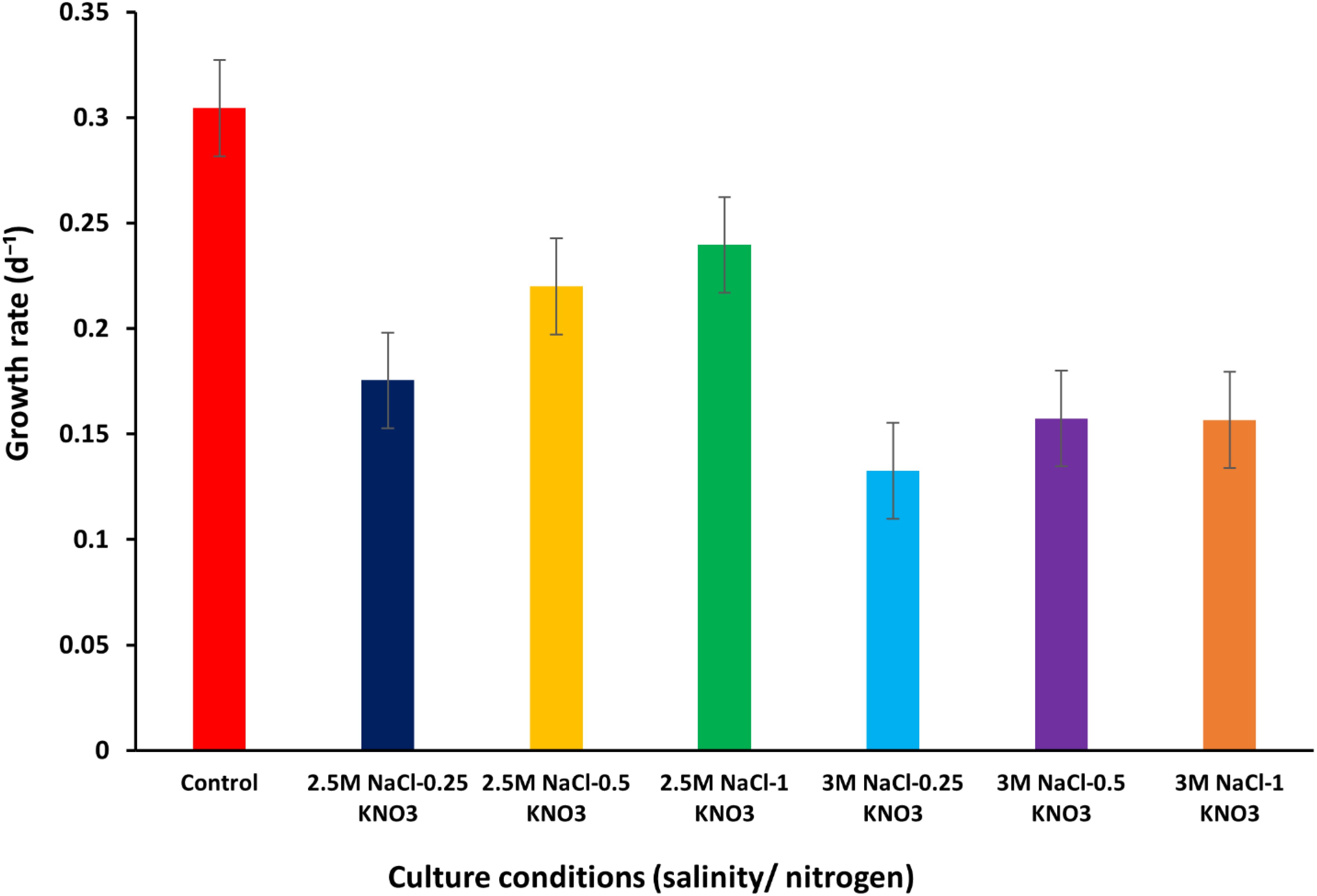
Figure 2. Growth rate of D. salina under different conditions (salinity/nitrogen). Bars are standard error of mean.
The values were significantly affected by the salinity and nitrogen concentration variations, as confirmed by the one-way ANOVA, which showed a P-value of ≤ 0.05. Additionally, the Tukey’s HSD test ranked the cell volume under various salinity conditions, highlighting that salinity levels of 2.5M and 3M produced statistically significant differences in growth rates and cell volumes compared to lower salinity levels (supplementary Table S2).
3.3 Dry weight
In this study, the dry weight of D. salina was measured under optimum growth conditions and was about 0.27 g/L, while when the alga was exposed to different conditions of salinity and nitrogen, the dry weight values at 2.5M NaCl were recorded at about 0.10, 0.12 and 0.14 g/L at 0.25, 0.5 and 1 g/L KNO3, respectively. When the salinity concentration was increased to 3M NaCl with exposure to different concentrations of nitrogen, the dry weight values of the algae were recorded at about 0.07, 0.08, and 0.08 g/L at 0.25, 0.5 and 1 g/L KNO3, respectively. Several previous studies have indicated that the dry weight value of D. salina ranged between 0.14 ± 0.02 to 0.29 ± 0.04 (Wu et al., 2017), and 2.25 ± 0.07 g/l (Wu et al., 2015) which aligns with the findings of this study. The results show a decrease in dry weight values with the rise in salinity concentration from 2.5M to 3M NaCl. On the other hand, the highest DW value was recorded at about 0.14 g/L at a salinity concentration of 2.5M NaCl and an optimum nitrogen concentration of 1 g/L KNO3 (Figure 3) (Supplementary Table S1).
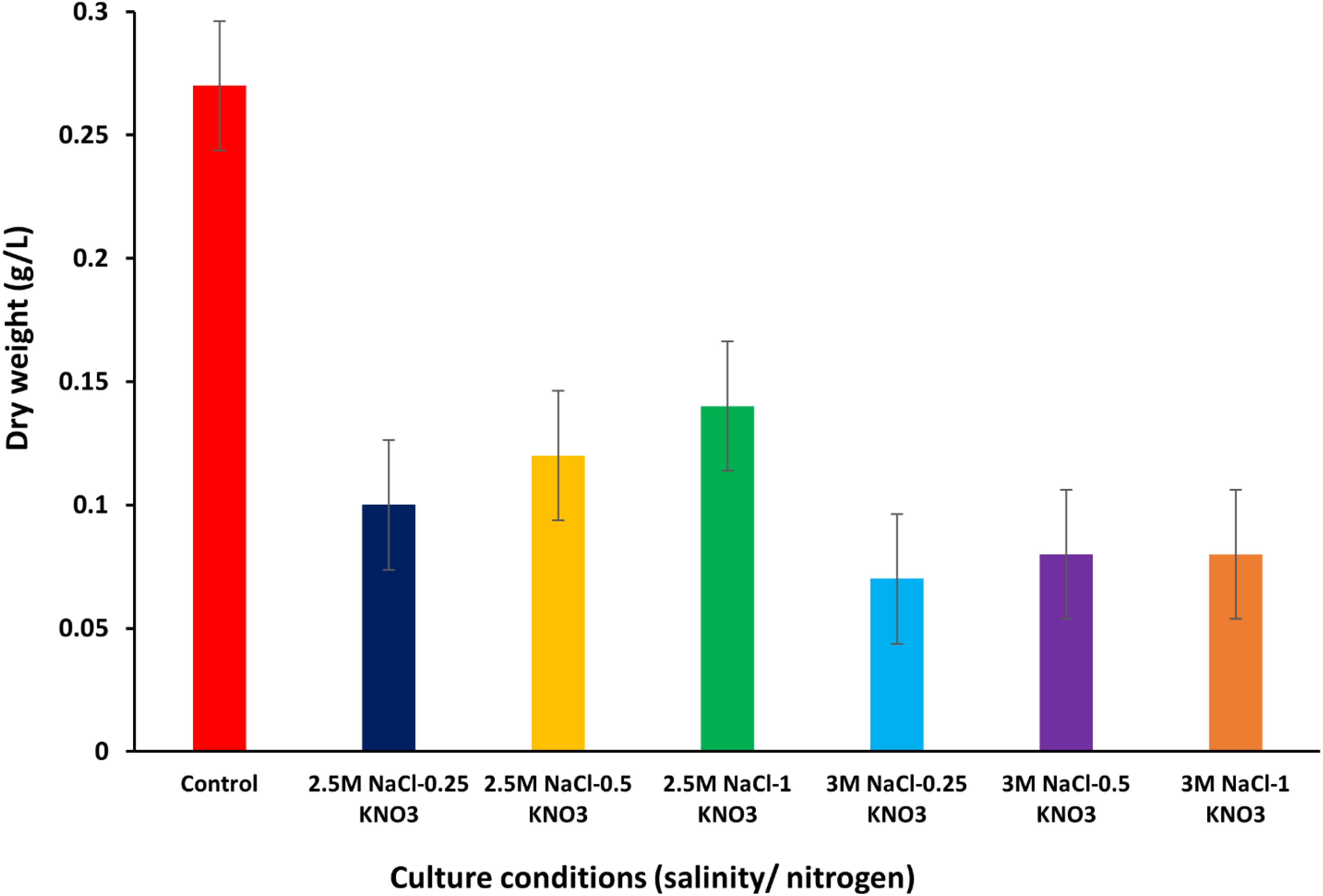
Figure 3. Dry weight values of D. salina under different conditions (salinity/nitrogen). Bars are standard error of mean.
Almutairi (2020) reported a decrease in dry weight and oil content in algae cells because of limiting nitrogen levels. This in turn is consistent with the results of this study due to the decrease in dry weight values with increasing salinity and nitrogen concentration. The dry weight of D. salina under the optimal conditions of 2.5M NaCl and 0.5 g/L KNO3 was measured at 0.12 g/L, with a productivity rate of 8.46 mg/mL/day. The one-way ANOVA test confirmed that dry weight and productivity varied significantly across different salinity and nitrogen levels, with a P-value of ≤ 0.05. The Tukey’s HSD test provided a detailed comparison, indicating that higher salinity levels contributed to a significant increase in dry weight and productivity compared to lower salinity levels (Supplementary Table S3).
3.4 Productions per dry weight
The production per dry weight of D. salina was studied under different conditions of salinity and nitrogen. The results of the study indicate that the production values per dry weight under optimum growth conditions reached 24.69 mg/mL d-¹, while when the D. salina was exposed to different growth conditions of salinity and nitrogen concentrations, varying values were recorded. At a salinity concentration of 2.5M NaCl and nitrogen concentrations of 0.25, 0.5, and 1 g/L KNO3, the production values per dry weight reached about 5.44, 8.46, and 10.25 mg/mL d-¹ respectively, noting that the increase in the production values per dry weight was consistent with the increase in nitrogen values and reached the highest value at the optimum nitrogen concentration of 1 g/L KNO3.
When the salinity concentration was increased to 3M NaCl, the production values per dry weight were recorded to be about 3.30, 3.21, and 4.11 mg/mL d-¹ at nitrogen concentrations (0.25, 0.5, and 1 g/L KNO3) respectively. When the salinity concentration increased to 3M NaCl, the production values per dry weight were recorded to be about 3.30, 3.21, and 4.11 mg/mL d-¹ at nitrogen concentrations (0.25, 0.5, and 1 g/L KNO3), respectively (Figure 4) (Supplementary Table S1). In the literature, a significant difference in the production values per dry weight of D. salina was observed, which ranged from 0.44 ± 0.02 mg/mL d-¹ (Sui et al., 2019) to 25.3 ± 4 mg/mL d-¹ (Wu et al., 2017). It was observed that production tends to decrease when nitrogen concentrations are limited. Previously, Chantzistrountsiou et al. (2023) recorded a decrease in production values of D. salina under nitrogen deficiency conditions in conjunction with growth rates, which confirms the results of this study.
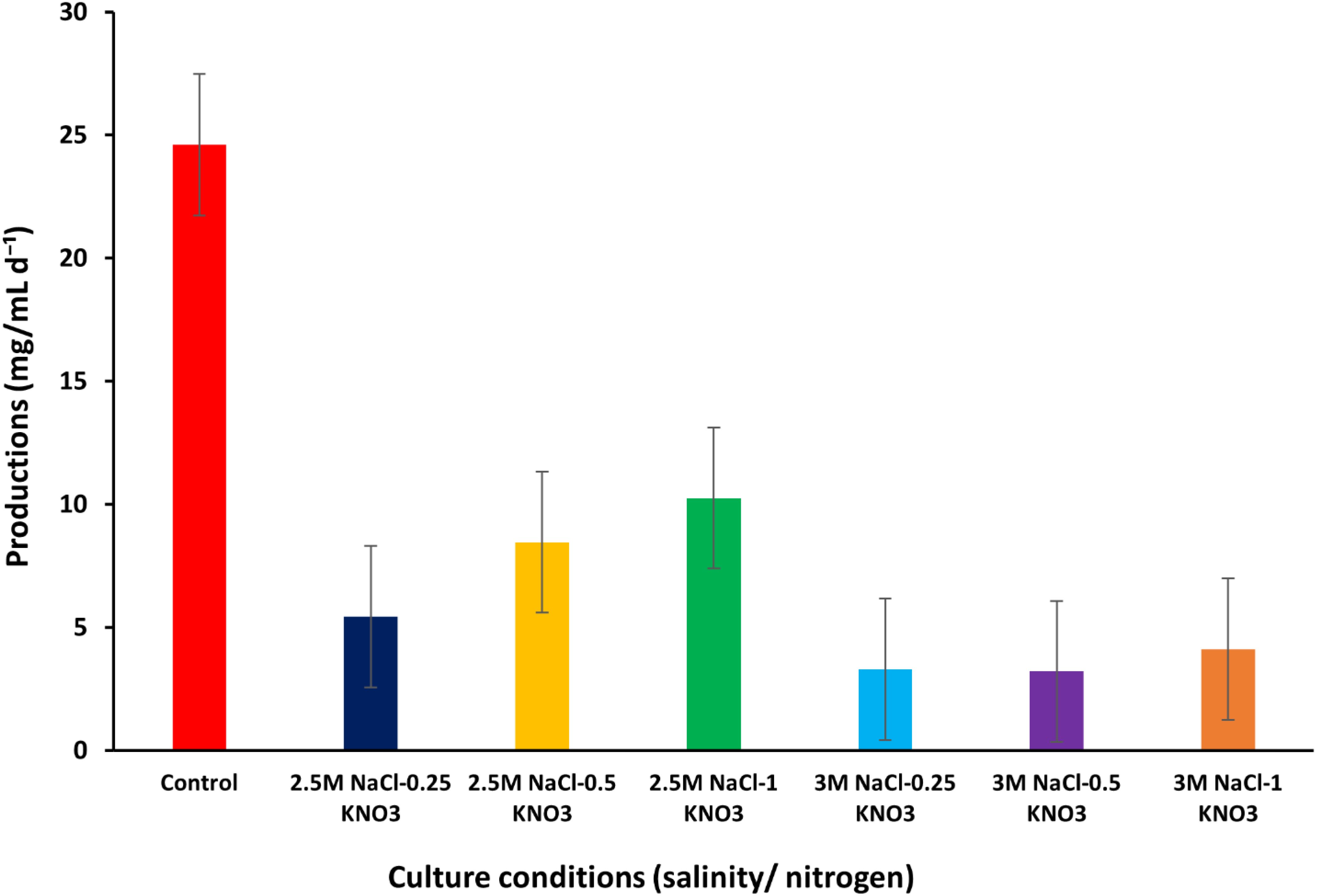
Figure 4. Production per DW values of D. salina under different conditions (salinity/nitrogen). Bars are standard error of mean.
3.5 Extraction and determination of beta-carotene content using tetrahydrofuran
The beta-carotene values were 95.64, 109.00, and 74.78 µg/mL at 2.5M NaCl under nitrogen conditions of 0.25, 0.5 and 1 g/L KNO3, respectively. The highest amount of beta-carotene (109.00 µg/mL) was recorded at a moderate nitrogen concentration of 0.5 g/L KNO3. When the salinity concentration was increased to 3M NaCl, values of 81.49, 86.20, and 103.05 µg/mL were recorded at 0.25, 0.5, and 1 g/L KNO3, respectively (Figure 5) (Supplementary Table S4). In other studies, beta-carotene values were reported to range between 0.18 µg/mL and 115.50 ± 0.40 µg/mL (Celekli and Dönmez, 2006) (Sathasivam et al., 2018). In Figure 5, an oscillating pattern was observed in the values of beta-carotene content extracted with THF at different concentrations of salinity and nitrogen. Xi et al. (2022) reported that the value of beta-carotene content was higher under conditions of low nitrate concentration than under conditions of high nitrate concentration. Many previous studies have shown that several stress factors such as salinity concentrations, light intensity, pH, nitrate concentration, nutrient deficiency, and temperature play an important role in the process of stimulating and accumulating beta-carotene in D. salina, whether these factors are combined or individually (Gallego-Cartagena et al., 2019; Chantzistrountsiou et al., 2023; Papapanagiotou et al., 2024; Al-Mhanna et al., 2023; Yang et al., 2023; Reshma et al., 2021; Wu et al., 2016; Hamed et al., 2018).
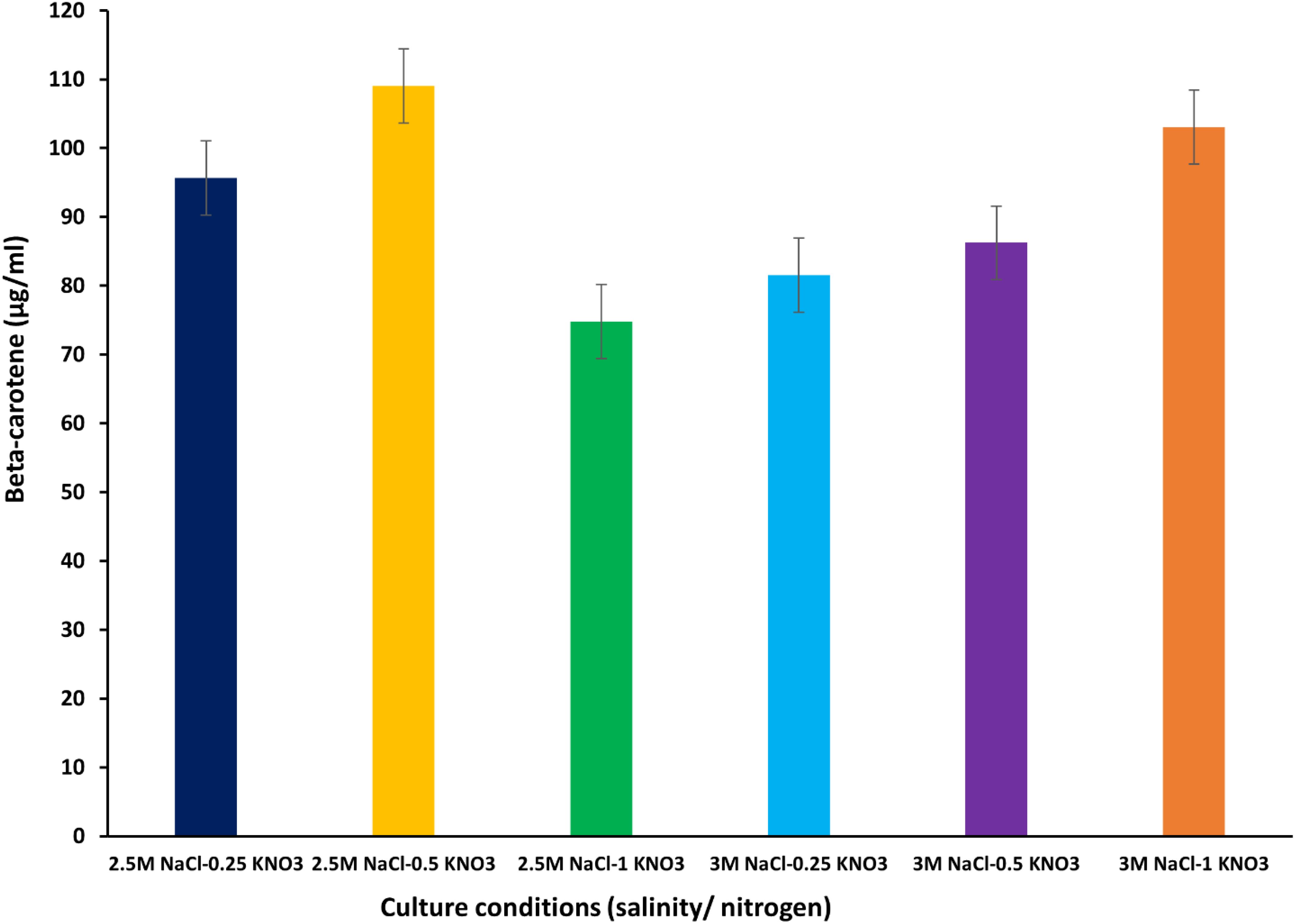
Figure 5. Beta-carotene extracted from D. salina using the organic solvent THF under different cultivation conditions (salinity/nitrogen). Bars are standard error of mean.
3.6 Extraction and determination of beta-carotene content using lipase enzyme
The highest value of beta-carotene (21.4 µg/mL) was extracted from the alga at a concentration of 0.5 mg/mL of lipase enzyme at a salinity level of 3M NaCl and a nitrogen concentration of 1 g/L KNO3 after 24 h of the experiment (Figure 6) (Supplementary Table S5). On the other hand, the lowest beta-carotene content was recorded at this enzyme concentration, reaching about 2.57 µg/mL at 2.5M NaCl/0.25 g/L KNO3 within 6 h exposure. It was observed that the values of beta-carotene content increased with increasing exposure times and higher nitrogen concentration, regardless of the available enzyme concentration. Carvalho et al. (2018) found that the extraction of total carotenoids from palm oil using lipase enzyme was dependent on time, temperature, and water/oil ratio. This is consistent with the results of this study and the importance of increasing exposure time for the total extraction of beta-carotene from D. salina. The results of this study indicate that enzyme concentration, exposure duration, and nitrogen levels play an important role in the effective extraction of beta-carotene using lipase enzyme, as they affect the efficiency of carotenoid extraction from microalgae (Liu et al., 2021; Jo et al., 2020). When the enzyme concentration was increased to 1 mg/mL, the highest extraction value of beta-carotene content (19.5 µg/mL), was recorded, after 24 h of exposure time under 3M NaCl/1 g/L KNO3. A slight difference in beta-carotene content values was observed at this concentration, (Figure 7). At 1.5 mg/mL lipase concentration, a significant variation in the recorded values was observed compared to the values at enzyme concentrations (0.5 and 1 mg/mL), where the highest value of beta-carotene content of about 16.2 µg/mL was recorded after 24 h of exposure time under stress conditions of 3M NaCl/0.5 g/L KNO, (Figure 8). It was noted that the values of the beta-carotene content extracted at this concentration were lower compared to the extraction values at other concentrations. In most beta-carotene extraction experiments, a slight difference in extraction values was observed between 12 and 24 hours of exposure. It may seem that 12 hours is sufficient for extraction, but some values increased significantly. This may explain the importance of time for increasing extraction efficiency. Therefore, all experiments were conducted at three exposure times. Two-way ANOVA was performed to examine the effects of enzyme concentration and exposure time on β-carotene extraction efficiency, which reveals significant interactions between these factors (P ≤ 0.05) (Supplementary Table S6).
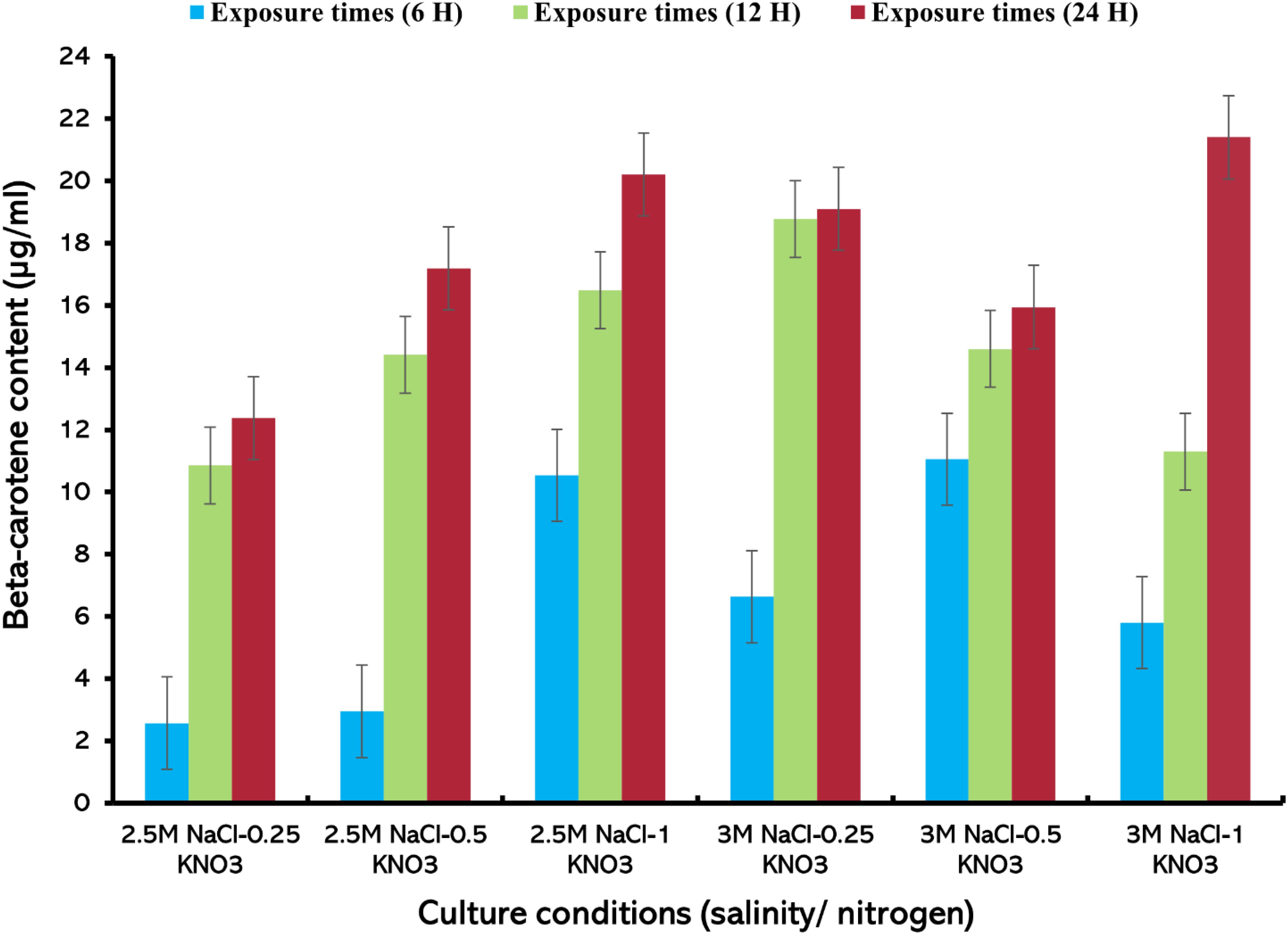
Figure 6. Beta-carotene extracted from D. salina using the lipase enzyme (0.5 mg/mL) under different cultivation conditions [salinity/nitrogen, and exposure times (H)], Bars are standard error of mean.
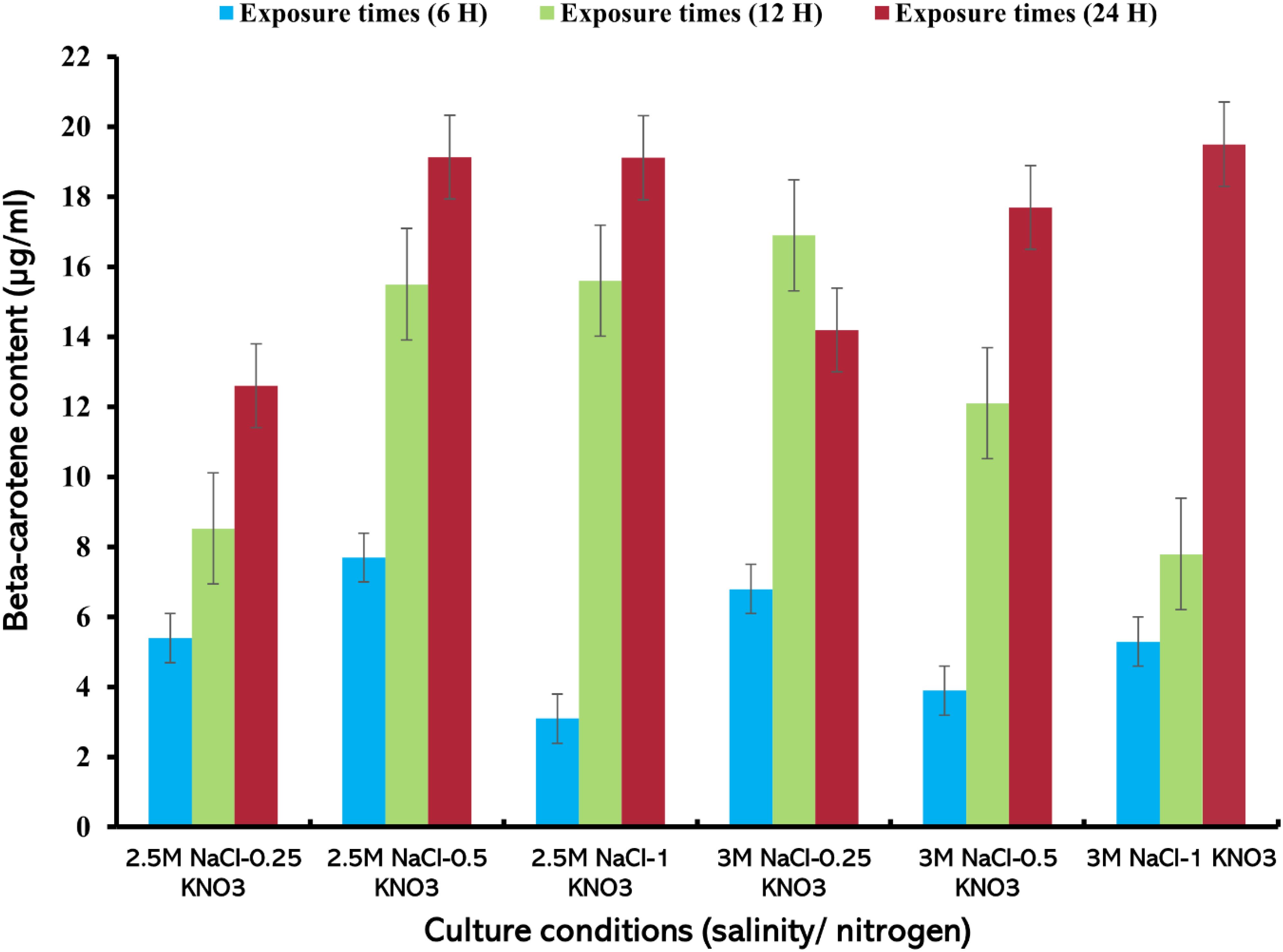
Figure 7. Beta-carotene extracted from D. salina using the lipase enzyme (1 mg/mL) under different cultivation conditions (salinity/nitrogen, and exposure times (H), Bars are standard error of mean.
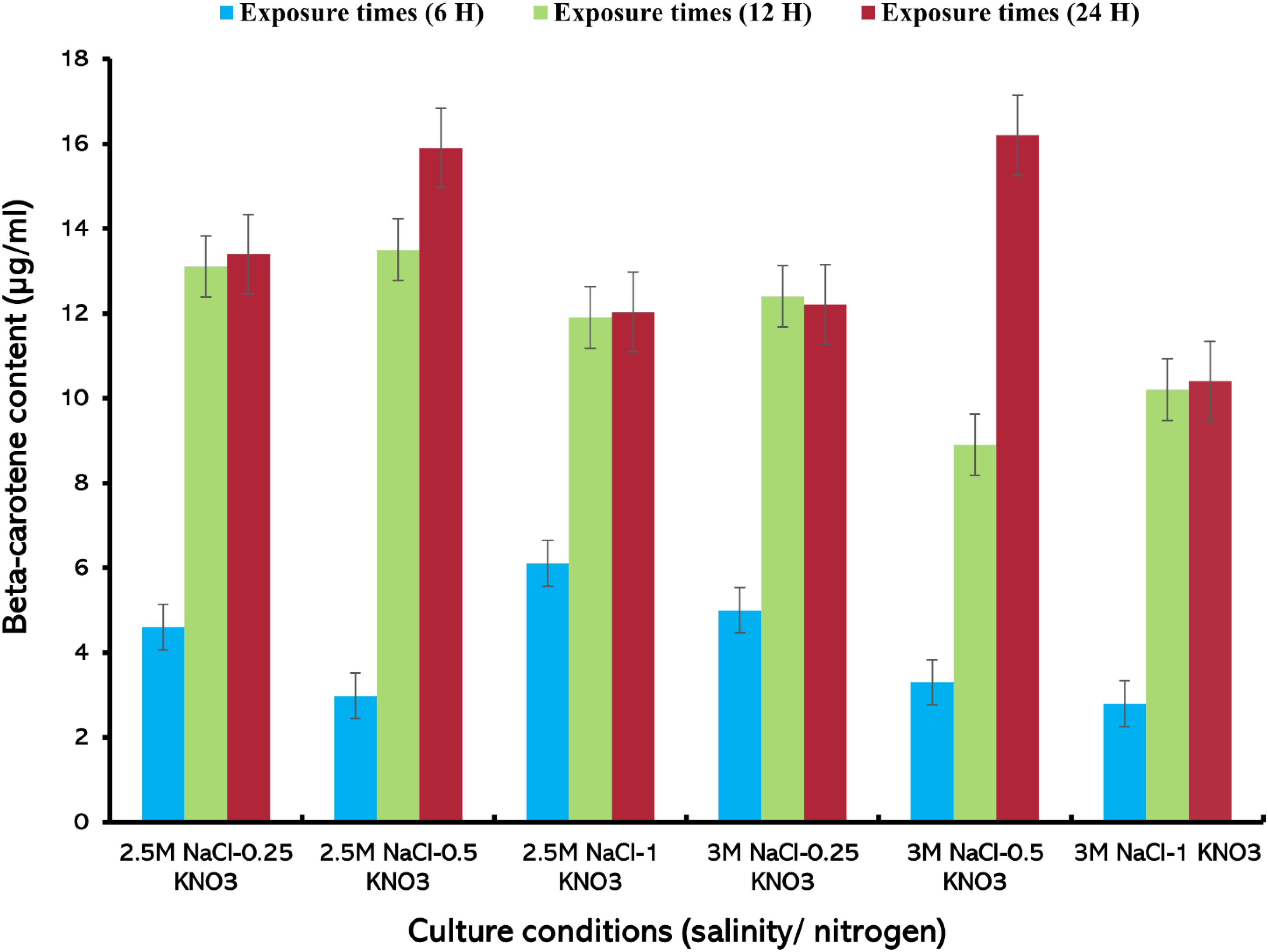
Figure 8. Beta-carotene extracted from D. salina using the lipase enzyme (1.5 mg/mL) under different cultivation conditions (salinity/nitrogen, and exposure times (H), Bars are standard error of mean.
The analysis demonstrated that both enzyme concentration and exposure time had substantial effects on the efficiency of β-carotene extraction. Enzymatic extraction of beta-carotene from D. salina can improve the efficiency and quality of the extracted product as well as maintain the viability of algae cells. The one-way ANOVA revealed significant differences (P ≤ 0.05) in β-carotene yield between the organic solvent method using THF and the enzymatic extraction method using lipase. The Tukey’s HSD test further confirmed these differences by identifying the conditions that produced the highest and lowest yields. Hejazi et al. (2002) observed that the use of biocompatible solvents such as dodecane selectively extracted beta-carotene while maintaining the survival of algal cells, thus enhancing the beta-carotene extraction process further. Enzymatic extraction works selectively, targeting carotenoids primarily, leaving other cellular components behind. This in turn enhances the extraction of pure beta-carotene content free from other impurities compared to other traditional methods (Kleinegris et al., 2010). Enzymatic extraction helps preserve the structural composition and biological activity of carotenoids, enhancing the health benefits of these compounds (Al-naghrani and Sayegh, 2023). The low efficiency of carotenoid extraction using enzymes may be due to the cell wall composition, membrane stability and adaptations of microalgal cells to stress conditions (Taucher et al., 2016). These factors can hinder the accessibility of enzymes to their target sites, ultimately affecting the yield and quality of carotenoids extracted from these organisms (Neagu et al., 2014). Also, it is necessary to maintain optimum nutritional and processing conditions for the efficient extraction of beta-carotene from microalgae (Mussagy et al., 2019; Vernès et al., 2019). Hence, further studies are needed to analyze these parameters to improve the efficiency of beta-carotene extraction. While the quantification of beta-carotene using spectrophotometers may overestimate the concentration in algal cells due to the possible interference of other carotenoids, selective methods such as HPLC are necessary for more accurate values. Despite these methodological limitations, this study showed that lipase enzymes could be used as an alternative to the organic solvents for beta-carotene extraction from microalgae.
4 Conclusions
The results of this study indicate that both the culture system, salinity, and nitrogen concentration significantly affect algae growth, cell size, dry weight, productivity, and beta-carotene content. The beta-carotene extraction process using THF organic solvent recorded a high yield of beta-carotene compared with the extraction process using lipase enzyme. The exposure time factor also plays an important role in beta-carotene extraction. Therefore, this study recommends future studies on the extraction of beta-carotene from D. salina using lipase enzyme at different enzyme concentrations and exposure time which enhances the optimal extraction of beta-carotene.
Data availability statement
The original contributions presented in the study are included in the article/Supplementary Material. Further inquiries can be directed to the corresponding authors.
Author contributions
FS: Formal analysis, Validation, Writing – original draft, Writing – review & editing, Conceptualization, Funding acquisition, Methodology, Supervision. MA-N: Formal analysis, Writing – original draft, Methodology. RA: Formal analysis, Validation, Writing – original draft, Writing – review & editing. MJ: Formal analysis, Validation, Writing – review & editing. NN: Conceptualization, Methodology, Resources, Supervision, Writing – original draft. WF: Resources, Supervision, Writing – review & editing. SJ: Resources, Supervision, Writing – original draft. SS: Formal analysis, Supervision, Validation, Writing – original draft, Writing – review & editing.
Funding
The author(s) declare that financial support was received for the research and/or publication of this article. This research was funded by the Deanship of Scientific Research (DSR) at King Abdulaziz University, Jeddah, under grant no. (GPIP:1825-247-2024).
Acknowledgments
The authors acknowledge with thanks to the Deanship of Scientific Research (DSR) for technical and financial support.
Conflict of interest
The authors declare that the research was conducted in the absence of any commercial or financial relationships that could be construed as a potential conflict of interest.
Generative AI statement
The author(s) declare that no Generative AI was used in the creation of this manuscript.
Publisher’s note
All claims expressed in this article are solely those of the authors and do not necessarily represent those of their affiliated organizations, or those of the publisher, the editors and the reviewers. Any product that may be evaluated in this article, or claim that may be made by its manufacturer, is not guaranteed or endorsed by the publisher.
Supplementary material
The Supplementary Material for this article can be found online at: https://www.frontiersin.org/articles/10.3389/fmars.2025.1543147/full#supplementary-material
References
Alghamdi M. A., Elbaz M. I., Ismail I. E., Reda F. M., Alagawany M., El-Tarabily K. A., et al. (2024). Dietary supplementation with a mixture of Dunaliella salina and Spirulina enhances broiler performance by improving growth, immunity, digestive enzymes and gut microbiota. Poultry Sci. 103, 103337. doi: 10.1016/j.psj.2023.103337
Al-Mhanna N., Pistorius M., and Al Sammarraie L. (2023). Optimization of the cultivation conditions of the green algae dunaliella salina by using simplex method. Processes 11, 292. doi: 10.3390/pr11010292
Almutairi A. W. (2020). Effects of nitrogen and phosphorus limitations on fatty acid methyl esters and fuel properties of Dunaliella salina. Environ. Sci. Pollution Res. 27, 32296–32303. doi: 10.1007/s11356-020-08531-8
Al-Naghrani M. J. and Sayegh F. (2023). Extraction of β-carotene from locally Dunaliella salina using bacterial lipase enzyme. J. Survey Fisheries Sci. 10, 1527–1545.
Amengual J., Coronel J., Marques C., Aradillas-García C., Morales J. M. V., Andrade F. C., et al. (2020). β-Carotene oxygenase 1 activity modulates circulating cholesterol concentrations in mice and humans. J. Nutr. 150, 2023–2030. doi: 10.1093/jn/nxaa143
Arun N. and Singh D. (2013). Differential response of Dunaliella salina and Dunaliella tertiolecta isolated from brines of Sambhar Salt Lake of Rajasthan (India) to salinities: a study on growth, pigment and glycerol synthesis. J. Marine Biol. Assoc. India 55, 65–70. doi: 10.6024/jmbai.2013.55.1.01758-11
Asevedo E. A., Das Chagas B. M. E., De Oliveira Júnior S. D., and Dos Santos E. S. (2023). Recovery of lipids and carotenoids from Dunaliella salina microalgae using deep eutectic solvents. Algal Res. 69, 102940. doi: 10.1016/j.algal.2022.102940
Balaji S. and Roy A. (2020). Beta-carotene–A versatile antioxidant in oral cancer: A review. Drug Invention Today 13.
Barsanti L., Coltelli P., Evangelista V., Frassanito A. M., Passarelli V., Vesentini N., et al. (2008). “Oddities and curiosities in the algal world,” in Algal toxins: nature, occurrence, effect and detection (Netherlands: Springer), 353–391.
Becker E. W. (2007). Micro-algae as a source of protein. Biotechnol. Adv. 25, 207–210. doi: 10.1016/j.biotechadv.2006.11.002
Bhatnagar P., Gururani P., Singh N., Gautam P., Vlaskin M. S., and Kumar V. (2024). Review on microalgae protein and its current and future utilisation in the food industry. Int. J. Food Sci. Technol. 59, 473–480. doi: 10.1111/ijfs.16586
Bonnefond H., Combe C., Cadoret J.-P., Sciandra A., and Bernard O. (2024). “Potential of microalgae,” in Green Chemistry and Agro-food Industry: Towards a Sustainable Bioeconomy (Switzerland: Springer Nature).
Borowitzka M. A. (2013a). “Dunaliella: biology, production, and markets,” in Handbook of microalgal culture: applied phycology and biotechnology. Chichester, UK: Wiley-Blackwell 359–368.
Borowitzka M. A. (2013b). High-value products from microalgae—their development and commercialisation. J. Appl. phycol. 25, 743–756. doi: 10.1007/s10811-013-9983-9
Çalışlar S. (2019). The important of beta carotene on poultry nutrition. Selcuk J. Agric. Food Sci. 33, 252–259. doi: 10.15316/SJAFS.2019.185
Carvalho T., Pereira A., Finotelli P. V., and Amaral P. F. (2018). Palm oil fatty acids and carotenoids extraction with lipase immobilized in magnetic nanoparticles. Adv. Mater. Lett. 9, 643–646. doi: 10.5185/amlett.2018.2059
Çelebi H., Bahadır T., Şimşek İ., and Tulun Ş. (2021). “Use of dunaliella salina in environmental applications,” in Proceedings of 1st international electronic conference on biological diversity, ecology and evolution. 9411.
Celekli A. and Dönmez G. (2006). Effect of pH, light intensity, salt and nitrogen concentrations on growth and β-carotene accumulation by a new isolate of Dunaliella sp. World J. Microbiol. Biotechnol. 22, 183–189. doi: 10.1007/s11274-005-9017-0
Chantzistrountsiou X., Ntzouvaras A., Papadaki S., Tsirigoti A., Tzovenis I., and Economou-Amilli A. (2023). Carotenogenic Activity of Two Hypersaline Greek Dunaliella salina Strains under Nitrogen Deprivation and Salinity Stress. Water 15, 241. doi: 10.3390/w15020241
Chen D., Li Z., Shi J., Suen H., Zheng X., Zhang C., et al. (2024). Genomics and transcriptomics reveal β-carotene synthesis mechanism in Dunaliella salina. Front. Microbiol. 15, 1389224. doi: 10.3389/fmicb.2024.1389224
Chi L., Yao C., Cao X., and Xue S. (2016). Coordinated regulation of nitrogen supply mode and initial cell density for energy storage compounds production with economized nitrogen utilization in a marine microalga Isochrysis zhangjiangensis. Bioresource Technol. 200, 598–605. doi: 10.1016/j.biortech.2015.10.059
Da Silva M. R. O. B., Moura Y. A. S., Converti A., Porto A. L. F., Marques D. D. A. V., and Bezerra R. P. (2021). Assessment of the potential of Dunaliella microalgae for different biotechnological applications: A systematic review. Algal Res. 58, 102396. doi: 10.1016/j.algal.2021.102396
De Moraes L. B. S., Santos R. F. B., Goncalves Junior G. F., Mota G. C. P., Dantas D. M. D. M., De Souza Bezerra R., et al. (2022). Microalgae for feeding of penaeid shrimp larvae: an overview. Aquaculture Int. 30, 1295–1313. doi: 10.1007/s10499-022-00857-z
Dhandwal A., Bashir O., Malik T., Salve R. V., Dash K. K., Amin T., et al. (2024). Sustainable microalgal biomass as a potential functional food and its applications in food industry: a comprehensive review. Environ. Sci. Pollution Res. 32, 1–19. doi: 10.1007/s11356-024-33431-6
Dixon C. and Wilken L. R. (2018). Green microalgae biomolecule separations and recovery. Bioresources Bioprocessing 5, 1–24. doi: 10.1186/s40643-018-0199-3
El-Baz F. K., Hussein R. A., Saleh D. O., and Abdel Jaleel G. A. R. (2019). Zeaxanthin isolated from Dunaliella salina microalgae ameliorates age associated cardiac dysfunction in rats through stimulation of retinoid receptors. Mar. Drugs 17, 290. doi: 10.3390/md17050290
Gallego-Cartagena E., Castillo-Ramírez M., and Martínez-Burgos W. (2019). Effect of stressful conditions on the carotenogenic activity of a Colombian strain of Dunaliella salina. Saudi J. Biol. Sci. 26, 1325–1330. doi: 10.1016/j.sjbs.2019.07.010
Grima E. M., Belarbi E.-H., Fernández F. A., Medina A. R., and Chisti Y. (2003). Recovery of microalgal biomass and metabolites: process options and economics. Biotechnol. Adv. 20, 491–515. doi: 10.1016/S0734-9750(02)00050-2
Guillard R. R. (1975). “Culture of phytoplankton for feeding marine invertebrates,” in Culture of marine invertebrate animals: proceedings—1st conference on culture of marine invertebrate animals greenport. (New York: Springer) 29–60.
Hamed I., Ak B., Işık O., Uslu L., and Vursavuş K. K. (2018). The Effect of Temperature and Salinity on the Growth and Carotenogenesis of Three Dunaliella Species (Dunaliella sp. Lake Isolate, D. salina CCAP 19/18 and D. bardawil LB 2538) Cultivated under Laboratory Conditions. Int. J. Biotechnol. Bioeng. 11, 806–811.
Havas F., Krispin S., Cohen M., Loing E., Farge M., Suere T., et al. (2022). A Dunaliella salina extract counteracts skin aging under intense solar irradiation thanks to its antiglycation and anti-inflammatory properties. Mar. Drugs 20, 104. doi: 10.3390/md20020104
Hejazi M. A., De Lamarliere C., Rocha J., Vermue M., Tramper J., and Wijffels R. (2002). Selective extraction of carotenoids from the microalga Dunaliella salina with retention of viability. Biotechnol. bioeng. 79, 29–36. doi: 10.1002/bit.10270
Hejazi M. and Wijffels R. (2003). Effect of light intensity on β-carotene production and extraction by Dunaliella salina in two-phase bioreactors. Biomolecular Eng. 20, 171–175. doi: 10.1016/S1389-0344(03)00046-7
Highfield A., Ward A., Pipe R., and Schroeder D. C. (2021). Molecular and phylogenetic analysis reveals new diversity of Dunaliella salina from hypersaline environments. J. Marine Biol. Assoc. United Kingdom 101, 27–37. doi: 10.1017/S0025315420001319
Honda M. (2023). Z-Isomers of lycopene and β-carotene exhibit greater skin-quality improving action than their all-E-isomers. Food Chem. 421, 135954. doi: 10.1016/j.foodchem.2023.135954
Jo S.-W., Hong J. W., Do J.-M., Na H., Kim J.-J., Park S.-I., et al. (2020). Nitrogen deficiency-dependent abiotic stress enhances carotenoid production in indigenous green microalga Scenedesmus rubescens KNUA042, for use as a potential resource of high value products. Sustainability 12, 5445. doi: 10.3390/su12135445
Johnson M. K., Johnson E. J., Macelroy R. D., Speer H. L., and Bruff B. S. (1968). Effects of salts on the halophilic alga Dunaliella viridis. J. Bacteriol. 95, 1461–1468. doi: 10.1128/jb.95.4.1461-1468.1968
Khan M. I., Shin J. H., and Kim J. D. (2018). The promising future of microalgae: current status, challenges, and optimization of a sustainable and renewable industry for biofuels, feed, and other products. Microbial. Cell factories 17, 1–21. doi: 10.1186/s12934-018-0879-x
Kleinegris D. M., Janssen M., Brandenburg W. A., and Wijffels R. H. (2010). The selectivity of milking of Dunaliella salina. Marine Biotechnol. 12, 14–23. doi: 10.1007/s10126-009-9195-0
Lauritano C., Rizzo C., Lo Giudice A., and Saggiomo M. (2020). Physiological and molecular responses to main environmental stressors of microalgae and bacteria in polar marine environments. Microorganisms 8, 1957. doi: 10.3390/microorganisms8121957
Liu C., Hu B., Cheng Y., Guo Y., Yao W., and Qian H. (2021). Carotenoids from fungi and microalgae: A review on their recent production, extraction, and developments. Bioresource Technol. 337, 125398. doi: 10.1016/j.biortech.2021.125398
Monte J., Bernardo J., Sá M., Parreira C., Galinha C. F., Costa L., et al. (2020). Development of an integrated process of membrane filtration for harvesting carotenoid-rich Dunaliella salina at laboratory and pilot scales. Sep. Purif. Technol. 233, 116021. doi: 10.1016/j.seppur.2019.116021
Mussagy C. U., Winterburn J., Santos-Ebinuma V. C., and Pereira J. F. B. (2019). Production and extraction of carotenoids produced by microorganisms. Appl. Microbiol. Biotechnol. 103, 1095–1114. doi: 10.1007/s00253-018-9557-5
Neagu D., Leopold L. F., Thonart P., Destain J., and Socaciu C. (2014). Enzyme-assisted extraction of carotenoids and phenolic derivatives from tomatoes. Bulletin of University of Agricultural Sciences and Veterinary Medicine Cluj-Napoca. Animal Science and Biotechnologies. 71.
Ntzouvaras A. (2021). “Commercial Cultivation of Dunaliella salina for the Production of Beta-Carotene,” in Algae for Food (CRC Press).
Oliveira L., Bisalputra T., and Antia N. J. (1980). Ultrastructural observation of the surface coat of Dunaliella tertiolecta from staining with cationic dyes and enzyme treatments. New Phytol. 85, 385–392. doi: 10.1111/j.1469-8137.1980.tb03177.x
Papapanagiotou G., Panou M., Giannakopoulos C., Florokapi G., Iakovou G., Zalidis G., et al. (2024). Light, salinity, and nitrogen modulation of growth and carotenoid synthesis in six Dunaliella strains. J. Biol. Research-Thessaloniki 31. doi: 10.26262/jbrt.v31i0.9952
Pereira J. R. D. A. G., Fehrenbach G. W., Murray P., Pedrosa R., and Chen Y. (2024). Evaluation of dunaliella salina growth in different salinities for potential application on saline wastewater treatment and biomass production. doi: 10.20944/preprints202405.0013.v1
Ravi S., Ambati R. R., Kamath S. B., Chandrappa D., Narayanan A., Chauhan V. S., et al. (2012). Influence of different culture conditions on yield of biomass and value added products in microalgae. Dyn. Biochem. Process Biotechnol. Mol. Biol 6 (2), 77–85.
Reshma R., Devi K. C., Kumar S. D., Santhanam P., Perumal P., Krishnaveni N., et al. (2021). Enhancement of pigments production in the green microalga Dunaliella salina (PSBDU05) under optimized culture condition. Bioresource Technol. Rep. 14, 100672. doi: 10.1016/j.biteb.2021.100672
Ribeiro B. D., Barreto D. W., and Coelho M. A. Z. (2011). Technological aspects of β-carotene production. Food Bioprocess Technol. 4, 693–701. doi: 10.1007/s11947-011-0545-3
Ritu J. R., Ambati R. R., Ravishankar G. A., Shahjahan M., and Khan S. (2023). Utilization of astaxanthin from microalgae and carotenoid rich algal biomass as a feed supplement in aquaculture and poultry industry: An overview. J. Appl. Phycol. 35, 145–171. doi: 10.1007/s10811-022-02817-9
Sangkharak K., Pasitkul P., Paiyit N., and Aonchart P. (2011). Application of lipase treatment on carotenoids extraction from Chili (Capsicum annuum L.). Afr. J. Microbiol. Res. 5, 5057–5061. doi: 10.5897/AJMR11.795
Sathasivam R., Pongpadung P., Praiboon J., Chirapart A., Trakulnaleamsai S., Roytrakul S., et al. (2018). Optimizing NaCl and KNO3 Concentrations for High b-carotene Production in Photobioreactor by Dunaliella salina KU11 Isolated from Saline Soil Sample. Chiang Mai J Sci.. 45, 106–115.
Sousa V., Hijo A. A. T., Ludtke F. L., Vicente A. A., Dias O., and Geada P. (2024). Recovery and encapsulation of Dunaliella salina β-carotene through a novel sustainable approach: Sequential application of an ionic liquid as naturally-derived solvent and emulsifier. Food Chem., 140232. doi: 10.1016/j.foodchem.2024.140232
Sousa V., Pereira R. N., Vicente A. A., Dias O., and Geada P. (2023). Microalgae biomass as an alternative source of biocompounds: New insights and future perspectives of extraction methodologies. Food Res. Int. 173, 113282. doi: 10.1016/j.foodres.2023.113282
Stolz P. and Obermayer B. (2005). Manufacturing microalgae for skin care. Cosmetics toiletries 120, 99–106.
Sui Y., Muys M., Van De Waal D. B., D’adamo S., Vermeir P., Fernandes T. V., et al. (2019). Enhancement of co-production of nutritional protein and carotenoids in Dunaliella salina using a two-phase cultivation assisted by nitrogen level and light intensity. Bioresource Technol. 287, 121398. doi: 10.1016/j.biortech.2019.121398
Taucher J., Baer S., Schwerna P., Hofmann D., Hummer M., Buchholz R., et al. (2016). Cell disruption and pressurized liquid extraction of carotenoids from microalgae. J. Thermodyn. Catal 7, 1–7. doi: 10.4172/2157-7544.1000158
Valente L. M., Cabrita A. R., Maia M. R., Valente I. M., Engrola S., Fonseca A. J., et al. (2021). “Microalgae as feed ingredients for livestock production and aquaculture,” in Microalgae (Elsevier).
Vernes L., Li Y., Chemat F., and Abert-Vian M. (2019). Biorefinery concept as a key for sustainable future to green chemistry—the case of microalgae. Plant Based “Green Chemistry 2.0. Moving Evolutionary to Revolutionary, 15–50. doi: 10.1007/978-981-13-3810-6_2
Weinrich T., Xu Y., Wosu C., Harvey P. J., and Jeffery G. (2019). Mitochondrial function, mobility and lifespan are improved in Drosophila melanogaster by extracts of 9-cis-β-Carotene from Dunaliella salina. Mar. Drugs 17, 279. doi: 10.3390/md17050279
Williamson E., Ross I. L., Wall B. T., and Hankamer B. (2023). Microalgae: Potential novel protein for sustainable human nutrition. Trends Plant Sci. 29 (3), 370–382.
Wu Z., Akter R., Arirob W., Juntawong N., Ma C., and Duangmanee P. (2015). Effects of light intensity and the remaining nitrate concentration on the beta-carotene accumulation of a wild Dunaliella salina strain isolated from the saline soil. Microbiol. Res. (Pavia) 6, 6233. doi: 10.4081/mr.2015.6233
Wu Z., Dejtisakdi W., Kermanee P., Ma C., Arirob W., Sathasivam R., et al. (2017). Outdoor cultivation of Dunaliella salina KU 11 using brine and saline lake water with raceway ponds in northeastern Thailand. Biotechnol. Appl. Biochem. 64, 938–943. doi: 10.1002/bab.2017.64.issue-6
Wu Z., Duangmanee P., Zhao P., Juntawong N., and Ma C. (2016). The effects of light, temperature, and nutrition on growth and pigment accumulation of three Dunaliella salina strains isolated from saline soil. Jundishapur J. Microbiol. 9. doi: 10.5812/jjm.26732
Xi Y., Zhang J., Kong F., Che J., and Chi Z. (2022). Kinetic modeling and process analysis for photo-production of β-carotene in Dunaliella salina. Bioresources Bioprocessing 9, 4. doi: 10.1186/s40643-022-00495-6
Xu Y., Ibrahim I. M., and Harvey P. J. (2016). The influence of photoperiod and light intensity on the growth and photosynthesis of Dunaliella salina (chlorophyta) CCAP 19/30. Plant Physiol. Biochem. 106, 305–315. doi: 10.1016/j.plaphy.2016.05.021
Yaiche-Achour H., Doumandji A., Bouras N., Sabaou N., and Assunção P. (2018). Isolation, molecular identification and the carotenogenesis process of the microalgae Dunaliella salina strain DunaDZ1 isolated from an Algerian salt lake. Turkish J. Fisheries Aquat. Sci. 19, 399–407. doi: 10.4194/1303-2712-v19_5_05
Yang Y., Zhou X., Xu Z., and Hu H. (2023). “Effects of nitrogen and phosphorus concentration changes on the growth of dunaliella salina in reverse osmosis concentrated brine,” in International Conference on Environmental Pollution and Governance. (Switzerland: Springer Nature), 125–139.
Yuan S., Lei W., Liu Q., Liu R., Liu J., Fu J., et al. (2023). Distribution and environmental impact of microalgae production potential under the carbon-neutral target. Energy 263, 125584. doi: 10.1016/j.energy.2022.125584
Yucel H. G., Karatay S. E., Aksu Z., and Dönmez G. (2021). Lithium (I) biofortified Dunaliella salina as a potential functional nutrition supplement. Algal Res. 56, 102257. doi: 10.1016/j.algal.2021.102257
Keywords: beta-carotene, Dunaliella salina, lipase enzyme, extraction, microalgae, tetrahydrofuran
Citation: Sayegh F, Al-naghrani MJ, Amran RH, Jamal MT, Nass NM, Felemban WF, Jastaniah SD and Satheesh S (2025) Extraction of beta-carotene from the microalga Dunaliella salina using bacterial lipase enzyme and organic solvent under varying stress conditions. Front. Mar. Sci. 12:1543147. doi: 10.3389/fmars.2025.1543147
Received: 10 December 2024; Accepted: 28 April 2025;
Published: 22 May 2025.
Edited by:
Roland Wohlgemuth, Lodz University of Technology, PolandReviewed by:
Angel Llamas, University of Cordoba, SpainTonmoy Ghosh, Durban University of Technology, South Africa
Copyright © 2025 Sayegh, Al-naghrani, Amran, Jamal, Nass, Felemban, Jastaniah and Satheesh. This is an open-access article distributed under the terms of the Creative Commons Attribution License (CC BY). The use, distribution or reproduction in other forums is permitted, provided the original author(s) and the copyright owner(s) are credited and that the original publication in this journal is cited, in accordance with accepted academic practice. No use, distribution or reproduction is permitted which does not comply with these terms.
*Correspondence: Fotoon Sayegh, ZnNheWdoQGthdS5lZHUuc2E=; Ramzi H. Amran, cmFtemlhbXJhbjA2QGdtYWlsLmNvbQ==