- Prince William Sound Science Center, Cordova, AK, United States
The 2014−2016 Pacific marine heatwave (PMH) was an intense and prolonged environmental disturbance that significantly disrupted the marine food web, leading to widespread ecological impacts. The PMH contributed to major shifts in species distributions, mass mortalities, and reproductive failures among upper-trophic level species, including a massive die-off of common murres (Uria aalge) in the Gulf of Alaska (GOA). To assess the impact of the PMH on the winter marine bird community in Prince William Sound (PWS), a large embayment in the northern GOA, we analyzed changes in winter marine bird abundance and species composition in a series of bays before and after the PMH. The overall density of winter marine birds decreased and species composition significantly changed in PWS following the PMH. Specifically, common murres, cormorants, and loons decreased from pre-PMH survey densities, while marbled murrelet (Brachyramphus marmoratus) densities increased. The post-PMH increase in marbled murrelets, likely due to immigration, coincided with the rapid growth and spatial expansion in the PWS Pacific herring (Clupea pallasii) young-of-the-year population and with a smaller, 8-month marine heatwave across the northern GOA. We suggest the mass mortality and lack of recovery by the common murre population provided a competitive release enabling murrelets to exploit a growing forage fish population, and that murrelets may be more effective at shifting to warmer-water zooplankton during marine heatwave events. These results highlight the persistent upper-trophic level changes associated with the PMH and provide important insights into the ecological consequences of environmental disturbances. This is increasingly relevant given the predicted increase in frequency and intensity of marine heatwaves.
1 Introduction
Marine birds serve as valuable indicators of ecosystem conditions since changes in their abundance or community composition can signal broader changes in the marine food web or ocean environment (e.g., Diamond and Devlin, 2003; Piatt et al., 2007). Additionally, because marine birds feed on a variety of fish and invertebrate species, their abundance and trends can indirectly reflect the status of these prey populations (e.g., Dunphy et al., 2020; Ramos and Furness, 2022). For species found in upper latitudes, food availability during the winter is critical during the colder months as conditions become harsher and there is less daylight for foraging. During winter, some marine bird species tend to congregate in waters closer to shore, such as shallow bays that offer protection from wave exposure (Dawson et al., 2015; Stocking et al., 2018; Schaefer and Bishop, 2023), while other marine bird species travel farther offshore (e.g., >50 km) to take advantage of foraging opportunities at continental shelves (Hunt et al., 2014; Cushing et al., 2024).
Marine heatwaves are prolonged periods of anomalously high ocean temperatures (Meehl and Tebaldi, 2004; Hobday et al., 2018) that can impact marine species. Marine heatwaves have been documented in the northeast Pacific Ocean since 1958 (Xu et al., 2022) with the most extensive event occurring between 2014 and 2016. This heatwave stretched from the eastern Bering Sea south to California. During this Pacific marine heatwave (PMH) event, sea surface temperature (SST) anomalies reached record levels that exceeded three standard deviations and were accompanied by high subsurface sea temperatures (Di Lorenzo and Mantua, 2016). Since then, another north Pacific heatwave occurred in 2019, but it was of shorter duration, a smaller geographic extent (but included our study area) and the warmer surface waters did not mix with cooler, deeper waters (Amaya et al., 2020; Chen et al., 2021; Ross et al., 2021; von Biela et al., 2022; R. Campbell pers. comm.). Such warming events are predicted to increase in frequency and intensity (Hobday et al., 2018; Oliver et al., 2018), therefore, understanding the impacts of marine heatwaves on the ecosystem is critical.
The 2014−2016 PMH caused cascading effects across all trophic levels over a vast swath of the north Pacific Ocean (Suryan et al., 2021). Phytoplankton production was reduced, and the largest harmful algal bloom in recorded history extended from the Gulf of Alaska (GOA) to the coast of California in 2015 (Leising et al., 2015; McCabe et al., 2016). In areas along the northern GOA, the overall forage fish population decreased (Arimitsu et al., 2021a; Institute for Seabird Research and Conservation, 2023) and Pacific sand lance (Ammodytes hexapterus), a key forage fish for marine mammals and seabirds, also lost nutritional value (von Biela et al., 2019; Suryan et al., 2021). Concurrently, marine bird and mammal die-offs were documented. A die-off of planktivorous Cassin’s auklets (Ptychoramphus aleuticus) occurred from British Columbia to central California in the winter of 2014−2015 (Jones et al., 2018). From the summer of 2015 into the spring of 2016, approximately 62,000 dead or dying piscivorous common murres (Uria aalge) were documented washed ashore from Alaska to California, with an estimated total loss of 4 million murres, making it the most extensive avian mortality event on record (Piatt et al., 2020; Renner et al., 2024). These seabird deaths were attributed primarily to starvation due to the reduced forage availability and the increased prevalence of low-calorie species coinciding with a decline in high-quality forage. In addition, the lack of forage led to an increase in pinniped mortality in southern California (McClatchie et al., 2016), an unusually large die-off of baleen whales in the GOA (Savage, 2017), and declines in humpback whale (Megaptera novaeangliae) survival and reproductive success in southeast Alaska (Gabriele et al., 2022).
Along portions of the northern GOA, documented impacts on the marine bird community from the PMH included shifts in species distribution and mass mortalities (Piatt et al., 2020; Robinson et al., 2024b; Cushing et al., 2024). In Prince William Sound (PWS), the epicenter of the common murre die-off (Piatt et al., 2020) and where the PMH persisted for a year longer (Campbell, 2023), the impacts on the marine bird community have not yet been assessed. In light of the documented impacts of the PMH on forage availability and quality, we hypothesized that marine bird density would be lower in PWS following the PMH. In this paper, we examined marine bird data collected from at-sea surveys in PWS during the nonbreeding season over nine winters prior to and after the 2014−2016 PMH to estimate marine bird densities, detect shifts in community composition, and determine which species were driving observed changes.
2 Materials and methods
2.1 Study area
Prince William Sound is a large embayment on the northern GOA, primarily between 60 and 61°C N (Figure 1). The Sound is separated from the GOA by large, mountainous islands, but is influenced by hydrography from the GOA (Reister et al., 2024). This region features large ice fields with tidewater glaciers and many freshwater streams contributing to an influx of freshwater. The coastline of PWS is rugged and includes many islands, deep fjords, and shallow bays affecting currents and tides (see site description in Gay and Vaughan, 2001). PWS provides protected wintering habitat for over 20 species of marine birds (Lance et al., 2001; Stocking et al., 2018; Schaefer and Bishop, 2023). In this study, we surveyed a series of bays and fjords (hereafter referred to as bays) ranging in maximum depths from 50 m to 300 m (Gay and Vaughan, 2001).
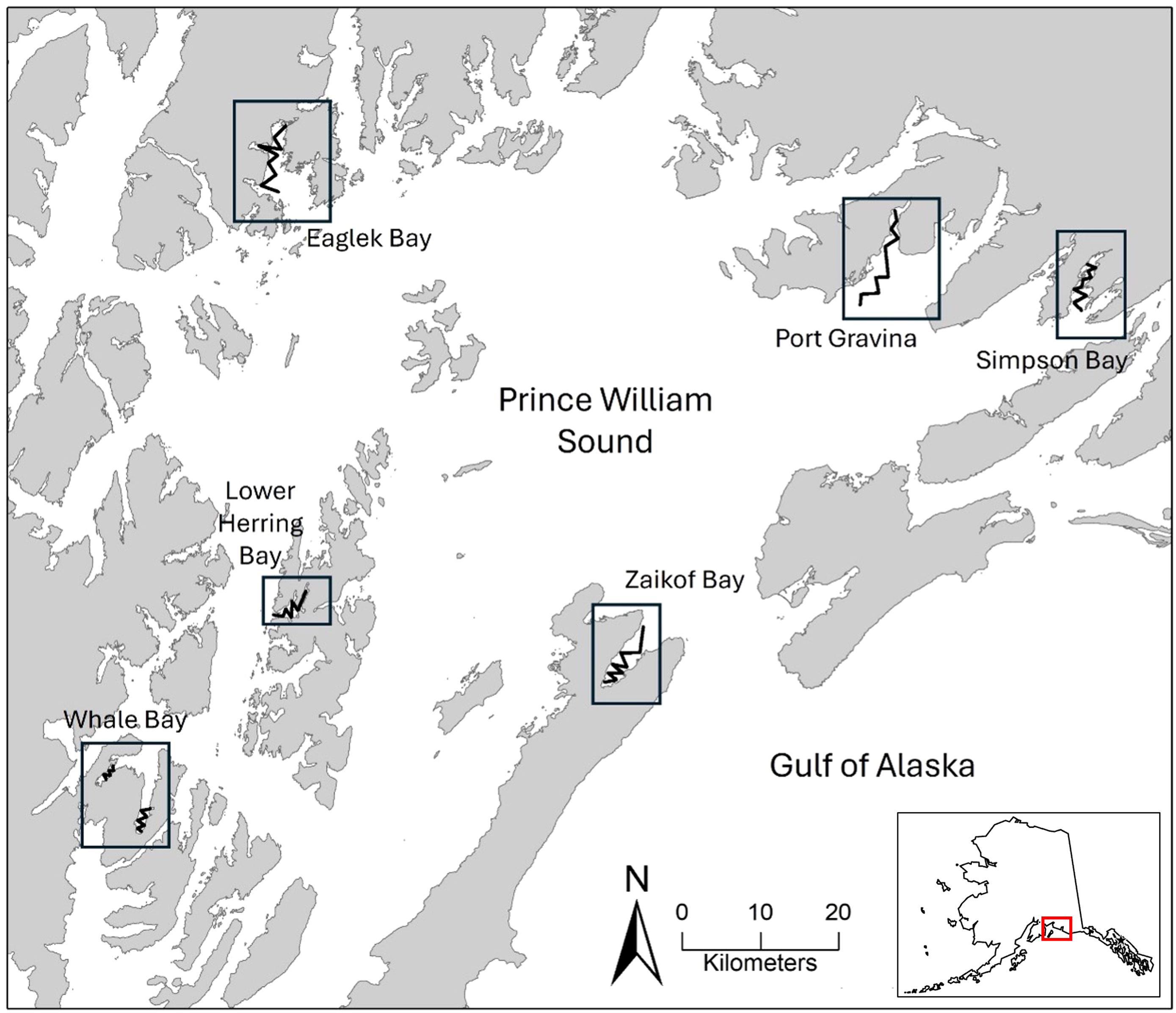
Figure 1. Bays surveyed in 2007−2012 (pre-Pacific marine heatwave) and 2019−2022 (post-Pacific marine heatwave) in Prince William Sound, Alaska. Survey transects are shown by the black zig-zag lines within each bay.
2.2 Data collection and preparation
We conducted marine bird surveys in six PWS bays: Eaglek Bay, Port Gravina, Simpson Bay, Whale Bay, Zaikof Bay, and Lower Herring Bay (added in November 2009);. Bays were surveyed as opposed to open-water areas as marine birds tend to concentrate in these protected, nearshore locations during the winter (Stocking et al., 2018). These bays are important juvenile herring nursery bays and have been included in other oceanographic and forage fish surveys (e.g., Gay and Vaughan, 2001; Lewandoski and Bishop, 2018; McKinstry and Campbell, 2018; Schaefer et al., 2020). Surveys took place during November and March for nine winters both prior to and after the PMH (November 2007−March 2012 and March 2019−March 2022; Figure 1). From 2007−2012 (pre-PMH), our marine bird surveys were conducted concurrently with surveys designed to assess juvenile Pacific herring (Clupea pallasii) biomass (Thorne, 2010) and herring energetics (Gorman et al., 2018). The sampling design developed for hydroacoustic juvenile herring surveys determined the locations and length of transects. More recently (2019−2022; post-PMH), marine bird surveys were repeated using the same survey methods and locations to provide updates on seabird abundance, distribution, habitat use, and community composition following the PMH (Schaefer and Bishop, 2023).
Marine bird observations were conducted using established U.S. Fish and Wildlife Service (USFWS) protocols (USFWS, 2007). Using 10x binoculars, one observer recorded species identification and the number of birds occurring within a strip transect width of 300 m (150 m on both sides and ahead of the boat) from a clear observation platform 2.5 m above the water line. These strip-transect surveys were conducted at standard survey speeds for small vessels of 5–15 km h-1 (slower speeds during hydroacoustic surveys), and we assumed all birds were detected at all speeds based on previous research assessing flushing distances and diving times (Lukacs et al., 2010). Observers used rangefinders to check distances and were trained to estimate distances of birds from the center line at 50 m increments. We assumed that no birds were attracted to or followed the survey vessel and that all birds within the transect strip were counted. The observer recorded observations into a laptop computer integrated with a global positioning system (GPS) using the program DLOG (2007−2012; Ford Consulting, Portland, OR) or SeaLog (2019−2022; ABR, Inc., Fairbanks, AK). These GPS-integrated programs provided location data at 15–20 sec intervals and for every entered observation. The observer also recorded in-situ sea and weather conditions. Data processing was performed using QA/QSea version 2.1 (Drew et al., 2023).
2.3 Statistical analyses
To calculate relative density (birds/km2), observations for each bay survey were sorted into 14 taxonomic groups (Supplementary Table 1), then summed and divided by sample area. Taxonomic groups were grouped by similar species (e.g., loons, mergansers, murres) and foraging strategies (i.e., shallow ducks forage at depths < 30 m and deep ducks > 30 m). We excluded from our analyses surveys where visibility conditions were rated as poor due to weather, glare, or sea state (Beaufort scale > 3), as well as species groups contributing <1% of observed birds over the study period (fewer than 98 birds). We performed a three-way ANOVA to assess overall changes in marine bird density by bay, period (pre-PMH, post-PMH), and month (November, March). T-tests evaluated the significance of species-specific density changes pre- and post-PMH, with a significance level of > 0.05 being considered significant.
We performed an Analysis of Similarity (ANOSIM) to determine statistically significant differences in species group densities between bays, periods, and months. The ANOSIM conducts a ranked dissimilarity matrix, with R values > 0.10 considered dissimilar and p-values < 0.05 indicating significance (Legendre and Legendre, 2012). We then used a Bray-Curtis similarity matrix and a non-parametric multi-dimensional scale (nMDS) ordination plot to examine differences in marine bird assemblages. We conducted pairwise comparisons (similarity percentage, i.e., SIMPER) to identify which species contributed to differences between bays, periods, and months. The nMDS ordination plots were then generated to graphically represent the separation between bays, time periods, and months, and boxplots were used to highlight species-specific differences. We square root transformed the density data included in the ANOSIM, nMDS ordination plots, and SIMPER analyses to decrease the influence of dominant taxa (Clarke and Warwick, 2001). We used R version 4.3.3 (R Core Team, 2023) and the package ‘vegan’ (Oksanen et al., 2013) for all analyses.
3 Results
We completed 17 research cruises, surveying on average 22.4 km2 per cruise (Supplementary Table 2). Four bays (Eaglek, Simpson, Whale, and Zaikof) were surveyed during every cruise, while Port Gravina and Lower Herring Bay were surveyed 13 times (Supplementary Table 2). In total, we recorded 9,812 birds representing 30 species across all cruises (Supplementary Table 1). The most abundant species on pre-PMH cruises included common murre (1,913 birds, 28.2% of observations), long-tailed duck (Clangula hyemalis, 777 birds, 11.5%, 668 of the total ducks observed in one flock), and glaucous-winged gull (Larus glaucescens, 680 birds, 10.0%; Supplementary Table 2). Post-PMH, the most abundant species were marbled murrelet (Brachyramphus marmoratus, 874 birds, 28.7% of observations), black-legged kittiwake (Rissa tridactyla, 311 birds, 10.2%), and Barrow’s goldeneye (Bucephala islandica, 281 birds, 9.2%).
Densities of individual species groups varied significantly between pre- and post-PMH surveys (Figure 2). Significantly different species groups include murres, Brachyramphus murrelets, loons, and cormorants (all p-values ≤ 0.006; Table 1). Compared to pre-PMH, post-PMH mean densities per survey of common murres decreased by a factor of 7.1 (Figure 3), while cormorants and loons decreased by a factor of 2.4 and 3.5, respectively. Among the loon species, common (Gavia immer), red-throated (G. stellata), and yellow-billed loon (G. adamsii) densities all decreased from pre- to post-PMH, especially common and red-throated loons which were not seen on any surveys after the heatwave. Pelagic cormorant (Urile pelagicus) comprised most cormorant sightings, and density decreases were primarily from two bays. Marbled murrelet, the dominant Brachyramphus murrelet species, was the only species that exhibited an increase in density post-PMH, rising by a factor of 1.9 (Figure 4).
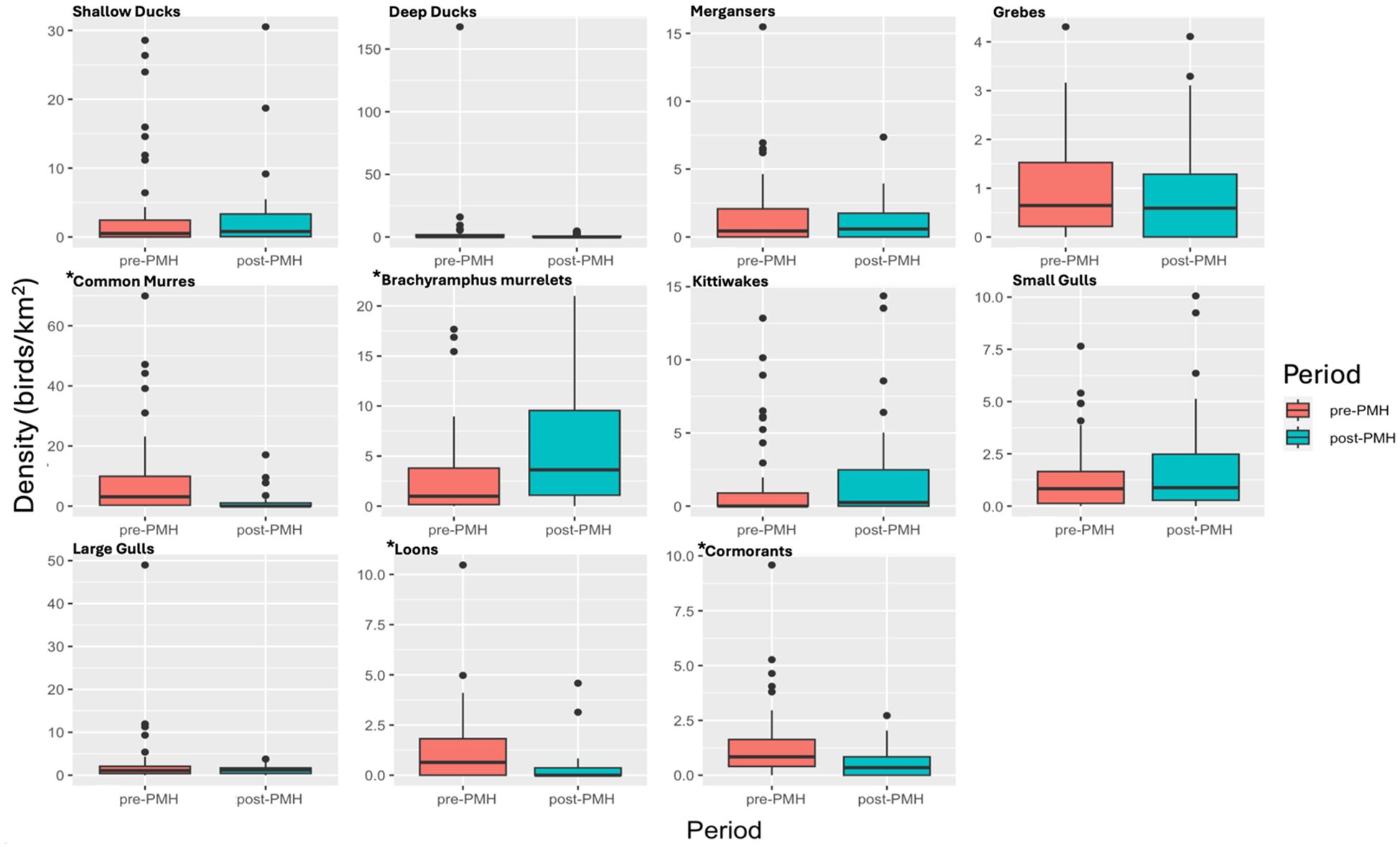
Figure 2. Density of marine bird species groups (birds/km2) observed on surveys in six bays pre-Pacific marine heatwave (PMH; 2007−2012, red) and post-PMH (2019−2022, blue) in Prince William Sound, Alaska. Note the y-axis scale for density is different for each graph. An asterisk denotes a significant change in density.
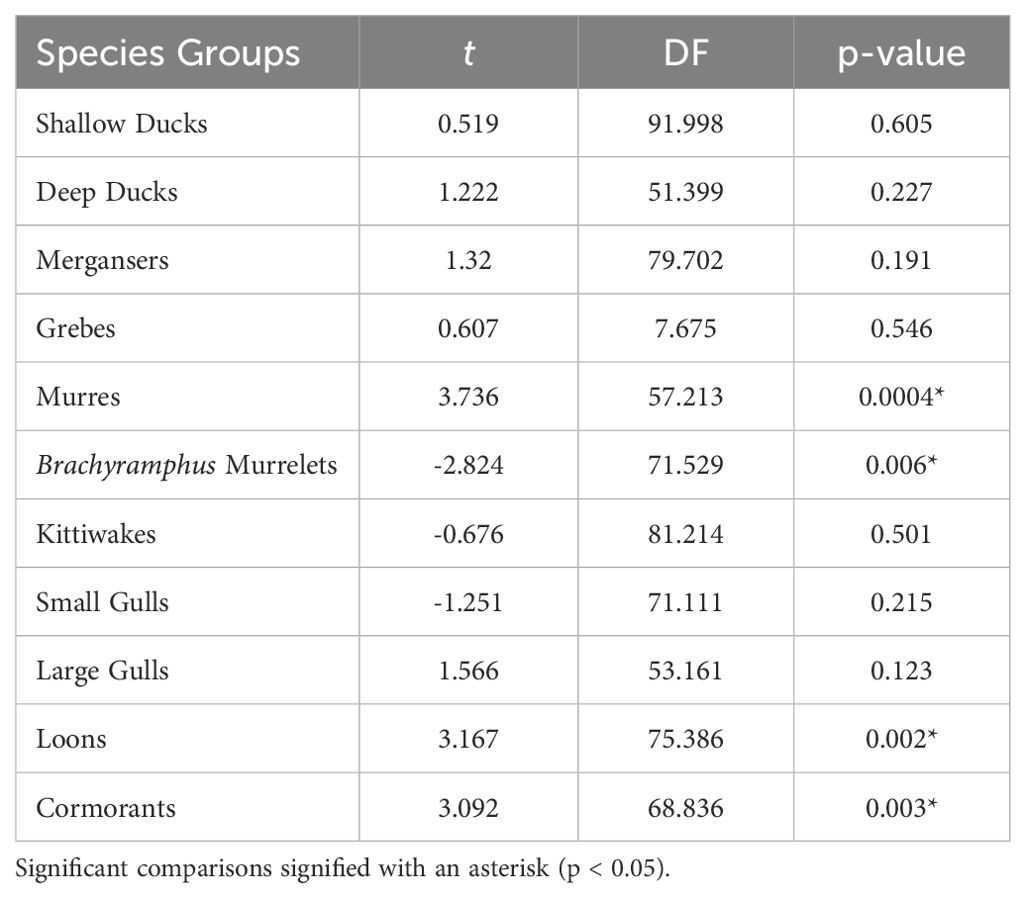
Table 1. T-test results comparing species group densities pre-Pacific marine heatwave (2007−2012) and post-Pacific marine heatwave (2019−2022) during surveys in Prince William Sound, Alaska.
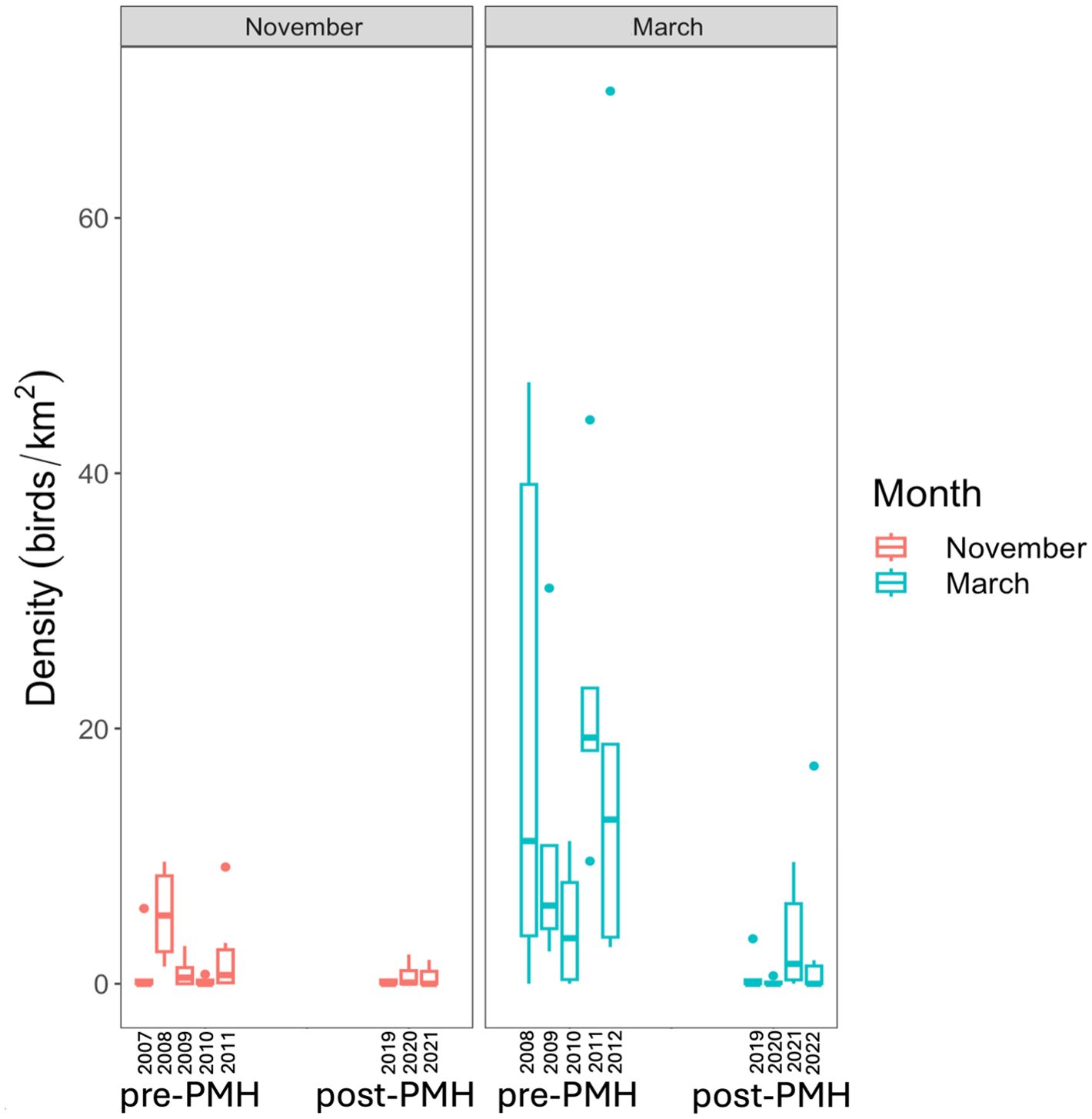
Figure 3. Densities of common murres Uria aalge by month (November=red, March=blue), and grouped by pre-Pacific marine heatwave (pre-PMH; 2007−2012) and post-Pacific marine heatwave (post-PMH; 2019−2022) in Prince William Sound, Alaska.
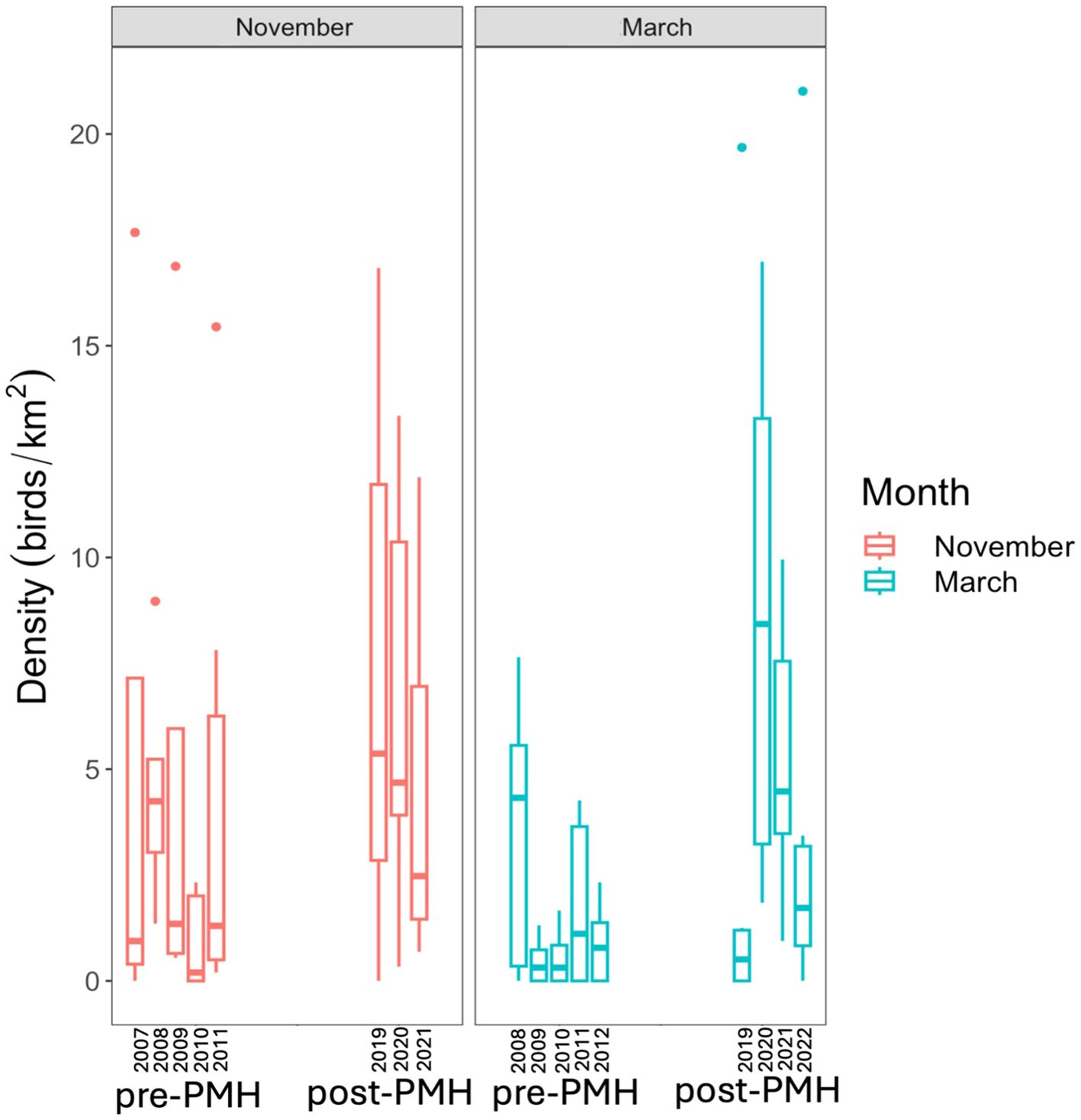
Figure 4. Densities of Brachyramphus murrelets by month (November=red, March=blue), and grouped by pre-Pacific marine heatwave (pre-PMH; 2007−2012) and post-Pacific marine heatwave (post-PMH; 2019−2022) in Prince William Sound, Alaska.
Marine bird densities were significantly higher before the heatwave and varied significantly across bays (both p values ≤ 0.01; Table 2). The highest densities occurred pre-PMH in Port Gravina (59.1 birds/km2, primarily driven by high densities of diving ducks) and post-PMH in Simpson Bay (26.6 birds/km2). Lower Herring Bay hosted the lowest average densities of birds before and after the PMH (Table 3). In four survey bays, the murre species group dominated the marine bird community pre-PMH, whereas post-PMH Brachyramphus murrelets were dominant (Table 3).
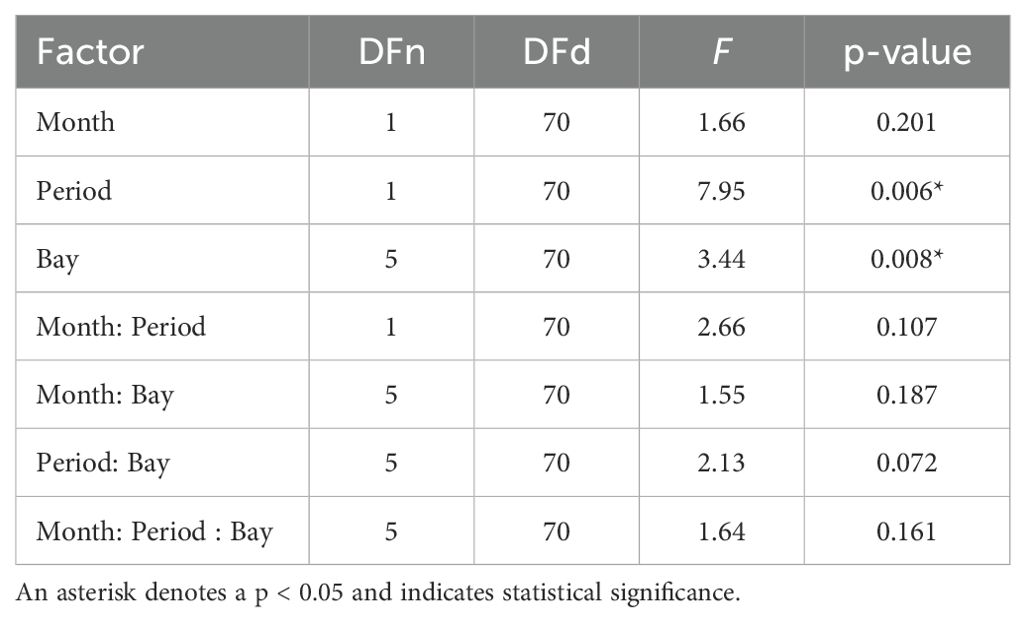
Table 2. Results of the three-way ANOVA to assess changes in marine bird density by bay (Eaglek, Gravina, Lower Herring, Simpson, Whale, Zaikof), period (pre-Pacific marine heatwave, 2007−2012; post-Pacific marine heatwave, 2019−2022), and month (March, November), in Prince William Sound, Alaska.
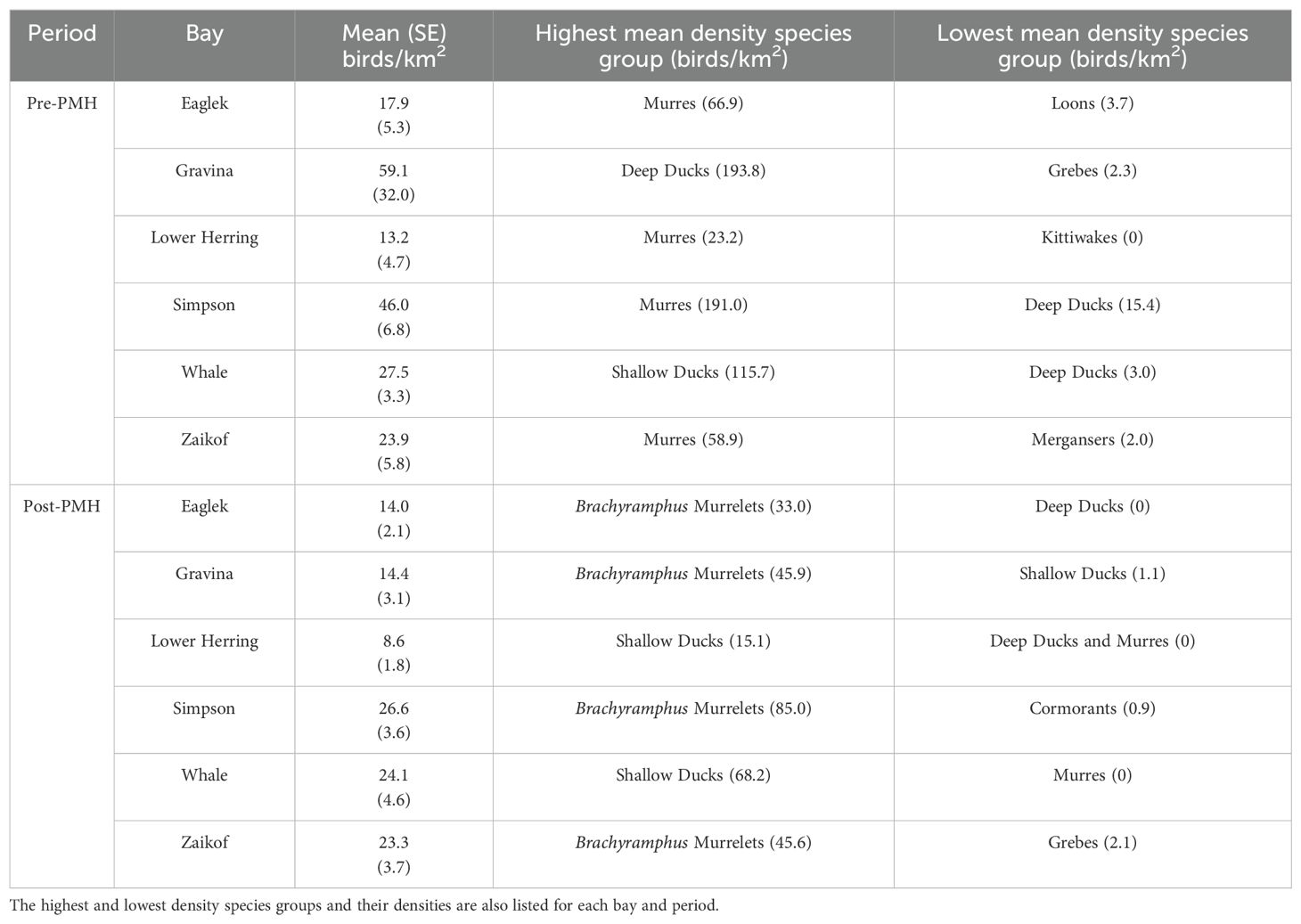
Table 3. Mean density of marine birds (birds/km2) by bay in Prince William Sound, Alaska during 2007−2012 (pre-Pacific marine heatwave; PMH) and 2019−2022 (post-PMH) with standard error (SE).
Multivariate analysis indicated significant variation in bird assemblages between the pre- and post-PMH periods (R = 0.18, p < 0.001; Table 4; Figure 5) and between bays (R = 0.24, p < 0.001; Figure 6). The SIMPER analysis identified three species groups contributing to the dissimilarities between periods: murres (9.2%; p = 0.003), Brachyramphus murrelets (7.5%; p = 0.001), and shallow ducks (6.5%; not significant, p = 0.461; Table 5). Pairwise comparisons indicated significant differences among bays, with Port Gravina being most frequently distinct from others, followed by Lower Herring Bay (Figure 6).
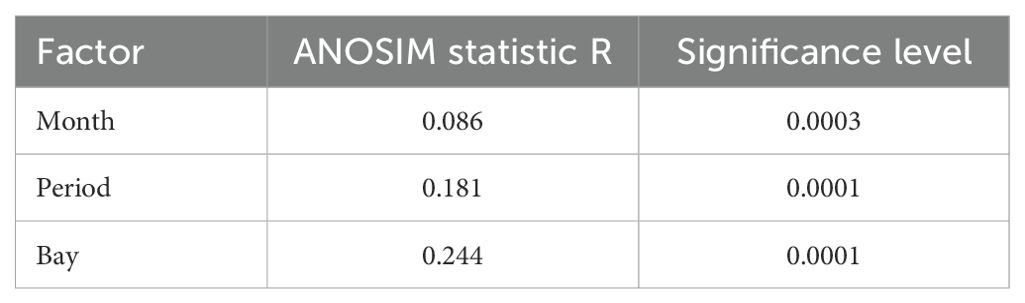
Table 4. Results of the analysis of similarity (ANOSIM) tests for months (March, November), period (pre-Pacific marine heatwave, 2007−2012; post-Pacific marine heatwave, 2019−2022), and bay (Eaglek, Gravina, Lower Herring, Simpson, Whale, Zaikof) using marine bird density for 14 species groups in Prince William Sound, Alaska.
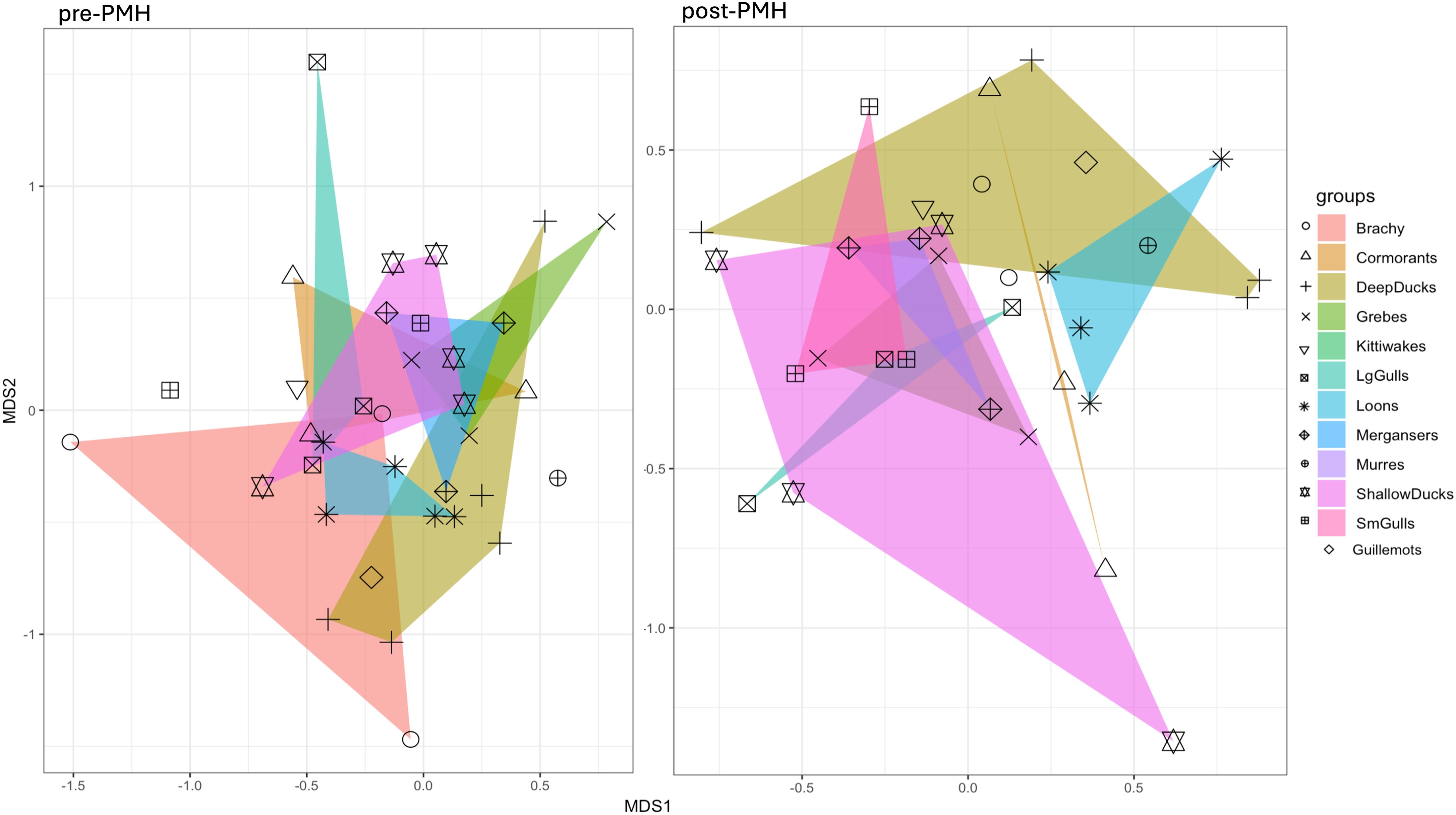
Figure 5. Non-metric multidimensional scaling (nMDS) Non-metric multidimensional scaling (nMDS) ordinations showing changes in winter marine bird assemblages in Prince William Sound bays before (2007-2012, left) and after (2019-2022, right) the Pacific marine heatwave for 2007−2012 (pre-Pacific marine heatwave; left) and 2019−2022 (post-Pacific marine heatwave; right).
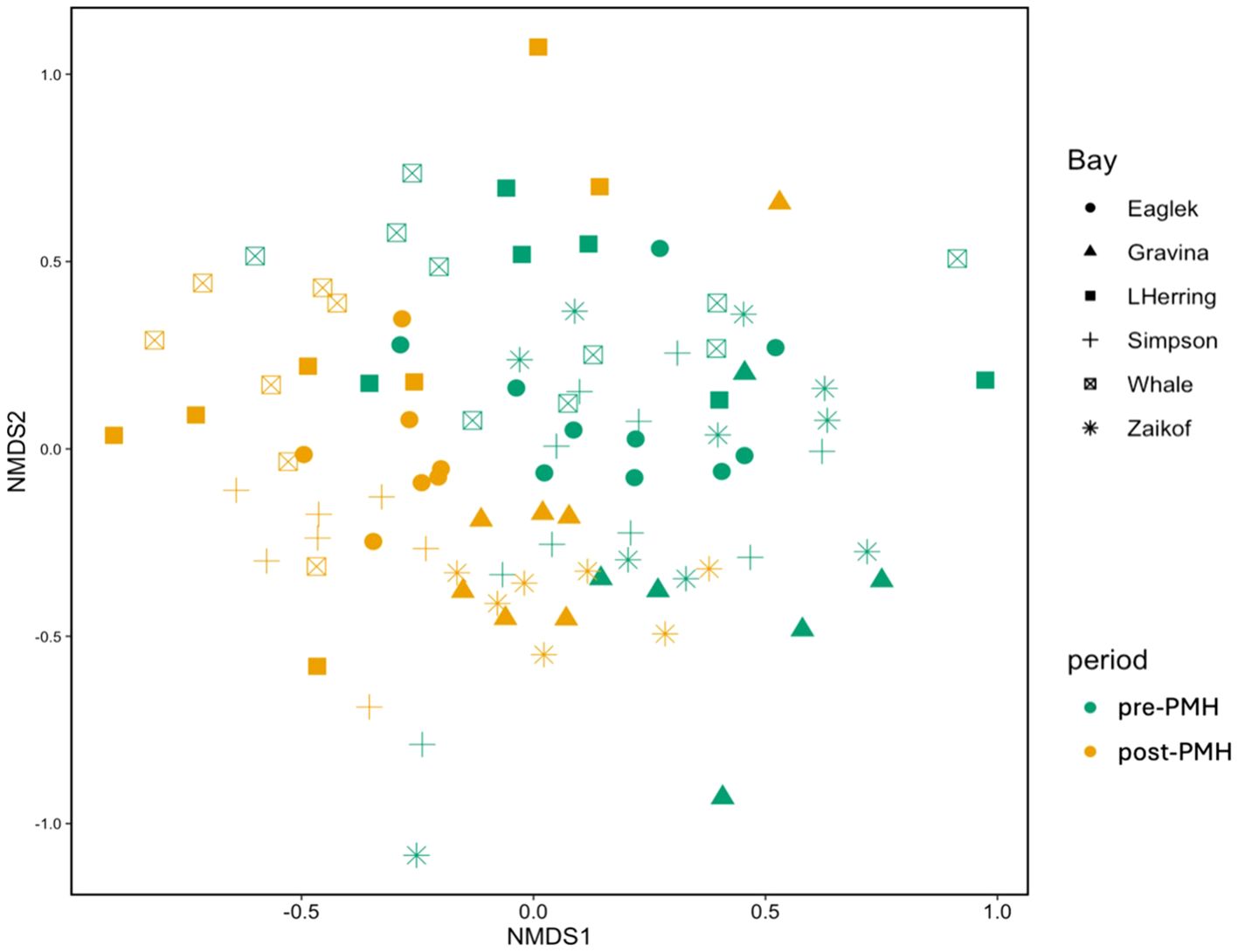
Figure 6. Non-metric multidimensional scaling (nMDS) ordinations of survey bays (symbols) based on marine bird assemblages and abundance data pre-Pacific marine heatwave (PMH; 2007−2012, green) to post-PMH (2019−2022, yellow) in Prince William Sound, Alaska.
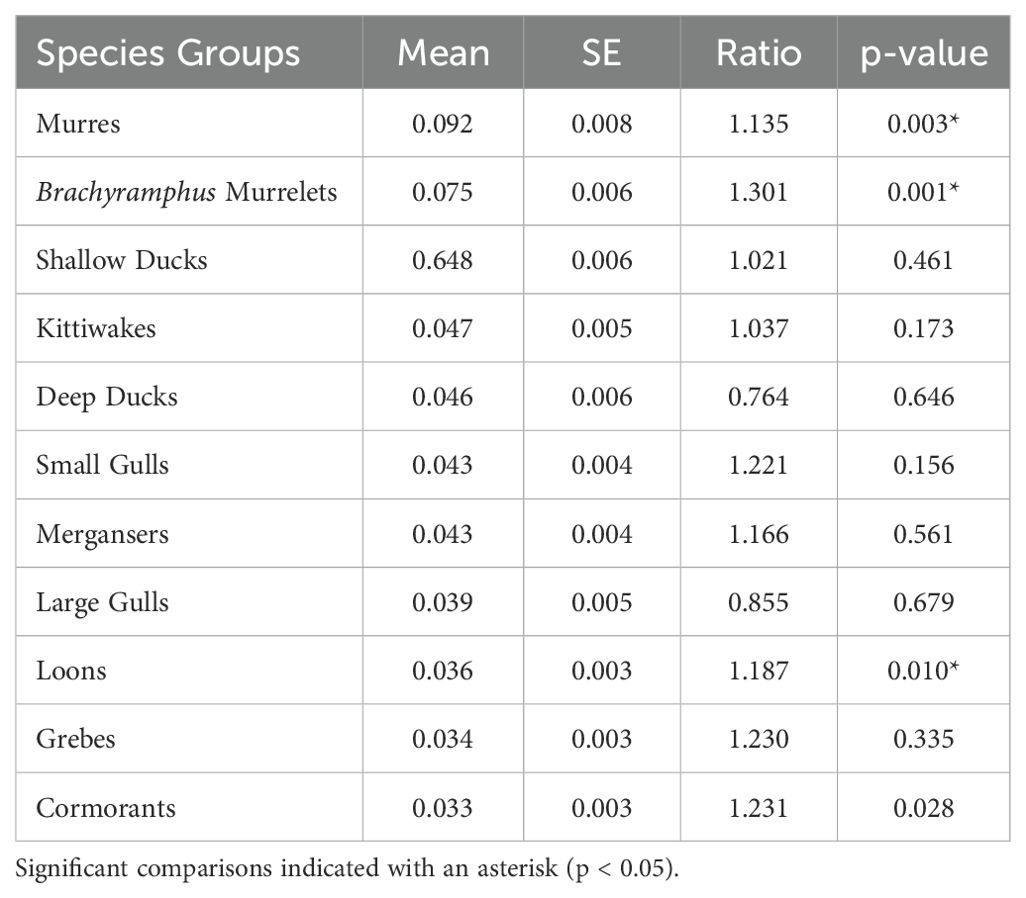
Table 5. SIMPER pairwise comparison showing species contributions to differences in pre- (2007-2012) and post-Pacific marine heatwave (2019-2022) winter marine bird assemblages in Prince William Sound, Alaska.
4 Discussion
We documented decreased marine bird density and significant changes in the community composition in PWS bays during the nonbreeding season in the years after the PMH as compared to the years prior to the PMH. Notably, densities declined post-PMH for murres, loons, and cormorants, all piscivorous divers that forage at depths of ~40–200 m (Roberts, 1932; Kotzerka et al., 2011; Kokubun et al., 2016). In contrast, densities increased post-PMH for Brachyramphus murrelets, which typically forage at depths of 40–50 m (Nelson, 2020). We suggest these documented changes in species groups were due to the decreased availability of high-lipid forage fish and zooplankton species post-PMH, the differences in diet between the three deeper-diving piscivorous species groups and marbled murrelets, and the differences in oceanographic conditions during each period and between bays.
The link between warmer ocean temperatures and the collapse of forage fish populations, as well as seabird die-offs and population declines, has been documented previously in the Pacific Ocean, both in the GOA (e.g., Agler et al., 1999) and as far south as the California Current (Hyrenbach and Veit, 2003; Hipfner, 2008; LeValley, 2009; Oliver et al., 2018). During the 2014−2016 PMH, multiple population collapses occurred across trophic levels. Euphausiid biomass decreased overall, with cooler-temperature species decreasing and some warmer-temperature species increasing (Arimitsu et al., 2021a; Pinchuk et al., 2021; Batten et al., 2022). These dramatic declines reduced prey availability and altered the prey composition available for predatory fish and seabirds.
Pacific herring, capelin (Mallotus catervarius), and sand lance are critical high-lipid forage species for marine birds in PWS (Anthony et al., 2000; Kuletz, 2005; Bishop et al., 2015; Hatch et al., 2020; Ainley et al., 2021; Paruk et al., 2021). During the PMH all three species were documented as being smaller in size and containing lower energy content in PWS and the GOA (Rand and Thorne, 2018; von Biela et al., 2019; Arimitsu et al., 2021a; Robinson et al., 2024a). In PWS, adult herring abundance decreased such that by spring 2018, a record-setting-low of 4.5 mile-days of spawn (sum of daily linear miles of herring milt observed during aerial surveys) was recorded (10-year average = 20.9 mile-days; Botz et al., 2021; 2022), reflecting a reduced spawning population available to predators (McGowan et al., 2021). Capelin, a northern species associated with cooler ocean temperatures, crashed during the PMH from record-high levels and remained low into 2019 (Arimitsu et al., 2021a). Diet studies at Middleton Island, a GOA island just south of PWS, documented the decrease of capelin from diets of diving and surface feeding birds (Suryan et al., 2021; Institute for Seabird Research and Conservation, 2023). Of the three forage fish species, sand lance did not experience as significant a decline in abundance and energy content. However, 90% of sand lance sampled from diving seabirds’ diets in the GOA in 2016 were from the smallest size class, and population numbers had not recovered to historical values by 2019 (Arimitsu et al., 2021a; Suryan et al., 2021).
In PWS, significant post-PMH declines and range contractions were observed in murres, loons, and cormorants. For example, common murres were recorded in most pre-PMH surveys in Lower Herring Bay where they represented the highest-density species group. However, no murres were recorded during any post-PMH surveys in Lower Herring Bay and one other bay. The decline in wintering murre density was not unexpected given the unprecedented murre mortality event that occurred across the north Pacific during the PMH (Piatt et al., 2020; Renner et al., 2024). Notably, murre populations have been slow to recover on their breeding grounds, with post-PMH productivity remaining below average or close to total reproductive failure (Schoen et al., 2024; Marsteller et al., 2024; Renner et al., 2024), despite some forage fish populations showing signs of recovery (Arimitsu et al., 2021b; McGowan et al., 2021). Post-PMH no common loons were observed on surveys in any bays despite being present (minimally) on pre-PMH surveys. Pelagic cormorants experienced significant density declines in two bays, whereas densities in other bays remained low and did not show a marked difference between periods. During the same period as this study, winter surveys in the Kenai and Katmai Fjords (approx.150–250 km from PWS) saw minimal changes in loons, cormorants, or murres (Coletti et al., 2023). Among other species groups, we determined there was minimal change in the pre- and post-PMH densities of shallow- and deep-diving ducks, a result similar to other studies within the GOA that found limited impacts to the nearshore food web (Robinson et al., 2024b; Weitzman et al., 2021).
Brachyramphus murrelets, consisting almost entirely of marbled murrelets, were the only species group to increase significantly in PWS bays post-PMH. Marbled murrelets may be migrating from elsewhere to winter in PWS in response to specific local forage availability and/or winter conditions in the GOA. Marbled murrelets have been documented moving between British Columbia and Alaska during the breeding and post-breeding seasons, possibly inflating population counts in Alaska (Bertram et al., 2023), especially during certain ocean conditions (e.g., storms, increased SST, currents; Agler et al., 1998; Becker and Beissinger, 2003). Additionally, alcids have been observed shifting inshore in Washington and the GOA, potentially increasing their presence and detectability on our surveys post-PMH (Pearson et al., 2022; Cushing et al., 2024). However, in Kenai and Katmai Fjords, post-PMH winter surveys recorded no observable differences in Brachyramphus murrelet densities (Coletti et al., 2023). It is surprising that two relatively close regions would experience different trends during the same period, suggesting local rather than regional conditions may be driving observed differences. Further investigation into changes in marbled murrelet movements across their range (annually and seasonally) and in response to ocean conditions is necessary to understand the drivers of the localized density increase in PWS. Additionally, it would be valuable to examine how other species similarly shifted their distributions in search of food or refuge in response to the PMH. For example, unusually high numbers of common murres were present in PWS in search of food immediately preceding the die-off event (Suryan et al., 2021). Such localized distribution changes may have masked broader population trends during and after the heatwave.
The striking increases in murrelet densities recorded during November 2019 and March 2020 surveys (Figure 4) coincided with the marine heatwave that occurred across much of the GOA from June 2019 to January 2020 (Amaya et al., 2020). During this period, the abundance of cool-water associated zooplankton declined, while warm-water associated zooplankton became more prevalent (Pinchuk et al., 2021; Batten et al., 2022; Campbell, 2023). Murrelets are euryphagous, eating a variety of foods, including young and small forage fish, squid, mysid shrimp (Mysis spp.), amphipods, euphausiids, and zooplankton (Hobson, 1990; Becker et al., 2007; Nelson, 2020). Compared to other species, PWS murrelets are more likely to be present in warmer surface waters (Dawson et al., 2015), suggesting they may be effective at shifting from cool-water to warmer-water zooplankton during marine heatwave events. Beginning in spring 2019, PWS murrelets also may have benefited from increases in young-of-the-year herring with the maturation of the 2016 hatch-year cohort of Pacific herring. This numerically dominant fish cohort began to spawn in areas outside of the historical southeast PWS spawning grounds as the mile-days of spawn increased from the low of 4.5 mile-days in 2018 incrementally to 26.8 mile-days in 2021 (McGowan et al., 2021; Morella, 2023). Historically, young-of-the-year (age 0) and age 1+ herring have been major diet components for marbled murrelets in PWS (Kuletz, 2005). Further, the population crash of common murres throughout the GOA may have created a competitive release enabling murrelets, the smaller of the two alcid species, to exploit prey resources such as the increasing Pacific herring population more fully than if common murres were present (Ronconi and Burger, 2011). Further investigation into this potential competitive release in relation to environmental change is needed to assess its validity, as well as to determine whether the increase in marbled murrelets could cause a cascading effect leading to a decline in the less dominant Kittlitz’s murrelet (Brachyramphus brevirostris).
We also documented marine bird abundance and species composition differences between PWS bays. The main drivers of the observed differences between time periods were Brachyramphus murrelets in Simpson Bay, shallow ducks in Whale Bay, and deep ducks in Port Gravina. Further study to understand and evaluate the ocean conditions (i.e., bathymetry, SST, current patterns, storms) and physical differences (i.e., depth, substrate, freshwater sources) between bays may help explain patterns of abundance based on species’ foraging strategies and the prey available. For example, Port Gravina hosted higher densities of loons, deep ducks, and large gulls than other bays pre-PMH. These higher densities in Port Gravina may reflect optimal foraging conditions for Pacific herring, with overwintering adult herring and extensive spawn regularly occurring in the area, as well as retention of both young-of-the-year and juvenile herring schools due to local ocean currents (Norcross et al., 2001).
4.1 Conclusion
Post-PMH, wintering marine bird densities in PWS declined, accompanied by a significant shift in species composition. We do not have survey data from the years of the PMH and can only make inferences on the impacts as a result of the PMH, however the decline in forage availability and its effects may not have been as evident during the event. These results present the longer-term effects to marine bird populations in PWS. The decrease in piscivorous species and species groups, in particular murres, loons, and cormorants, is concerning in light of predictions that marine heatwaves will occur more often and with greater intensity (Meehl and Tebaldi, 2004; Hobday et al., 2018; Oliver et al., 2018). Conversely, the increase in marbled murrelet density warrants further assessment of their annual cycle and geographic distribution.
Our results align with the findings of other GOA studies, including beach surveys for marine bird mortalities (Parrish et al., 2017) colony productivity assessments (Renner et al., 2024), and winter surveys in nearby areas (Cushing et al., 2024; Robinson et al., 2024b). Long-term monitoring efforts such as these are essential for documenting the impacts of events like the PMH, enabling researchers to evaluate interannual variation and identify long-term trends, which are crucial for understanding how different factors, such as storms, ocean temperatures, prey availability, and foraging conditions, affect marine bird populations over time (e.g., Ainley and Hyrenbach, 2010; Chambers et al., 2015; Dawson et al., 2015). Understanding changes in upper trophic level communities resulting from the PMH may provide valuable insights for updating and informing management strategies for these species as ecosystems continue to face increasing pressures from climate-driven disturbances.
Data availability statement
The datasets presented in this study can be found in online repositories. The names of the repository/repositories and accession number(s) can be found below: Fall and Winter Seabird Abundance Data, Prince William Sound, 2007-2023, Gulf Watch Alaska Pelagic Component:https://search.dataone.org/view/10.24431/rw1k32x.
Ethics statement
Ethical approval was not required for the study involving animals in accordance with the local legislation and institutional requirements because solely observational data were collected.
Author contributions
SH: Data curation, Formal analysis, Writing – original draft, Writing – review & editing. AS: Conceptualization, Data curation, Funding acquisition, Investigation, Methodology, Project administration, Writing – original draft, Writing – review & editing. MB: Conceptualization, Data curation, Funding acquisition, Investigation, Methodology, Project administration, Writing – original draft, Writing – review & editing.
Funding
The author(s) declare that financial support was received for the research and/or publication of this article. Funding for this research was provided by the Exxon Valdez Oil Spill Trustee Council (Projects 078814, 10100132-H, 17120114-E); however, the findings and conclusions of the authors are their own and do not necessarily reflect the views or position of the Trustee Council. Reference to trade, firm, or product names is for descriptive purposes only, and does not imply endorsement by the PWS Science Center, United States Government or State of Alaska.
Acknowledgments
This work would not have been possible without the observers that helped collect this data: A. Lang, B. Hsu, E. Owen, K. Brenneman, N. Dawson, R. Kaler, and T. Morgan. We also thank the boat captains D. Janka (and crew of R/V Auklet) and R. Campbell (M/V New Wave). We thank R. Thorne (deceased) and M. Buckhorn (deceased) for allowing us to share their vessel time. We are grateful to R. Suryan for his constructive input on an earlier draft, and to the reviewers (Z. Cannizzo, S. Schoen, A. Gall). Finally, we thank K. Kuletz for her collaborative efforts during the pre-PMH portion of this study.
Conflict of interest
The authors declare that the research was conducted in the absence of any commercial or financial relationships that could be construed as a potential conflict of interest.
Generative AI statement
The author(s) declare that no Generative AI was used in the creation of this manuscript.
Publisher’s note
All claims expressed in this article are solely those of the authors and do not necessarily represent those of their affiliated organizations, or those of the publisher, the editors and the reviewers. Any product that may be evaluated in this article, or claim that may be made by its manufacturer, is not guaranteed or endorsed by the publisher.
Supplementary material
The Supplementary Material for this article can be found online at: https://www.frontiersin.org/articles/10.3389/fmars.2025.1575748/full#supplementary-material
References
Agler B. A., Kendall S. J., Irons D. B. (1998). Abundance and distribution of Marbled and Kittlitz’s murrelets in southcentral and southeast Alaska. Condor 100, 254–265. doi: 10.2307/1370266
Agler B. A., Kendall S. J., Irons D. B., Klosiewski S. P. (1999). Declines in marine bird populations in Prince William Sound, Alaska coincident with a climatic regime shift. Waterbirds 22, 98–103. doi: 10.2307/1521998
Ainley D. G., Hyrenbach K. D. (2010). Top-down and bottom-up factors affecting seabird population trends in the California current system, (1985–2006). Prog. Oceanography 84, 242–254. doi: 10.1016/j.pocean.2009.10.001
Ainley D. G., Nettleship D. N., Storey A. E. (2021). Common Murre (Uria aalge), version 2.0. In Birds of the World. Eds. Billerman S. M., Rodewald P. G., Keeney B. K. (Ithaca, NY, USA: Cornell Lab of Ornithology). doi: 10.2173/bow/commur/02
Amaya D. J., Miller A. J., Xie S. P., Kosaka Y. (2020). Physical drivers of the summer 2019 North Pacific marine heatwave. Nat. Commun. 11, 903. doi: 10.1038/s41467-020-15820-w
Anthony J. A., Roby D. D., Turco K. R. (2000). Lipid content and energy density of forage fishes from the northern Gulf of Alaska. J. Exp. Marine Biol. Ecol. 248, 53–78. doi: 10.1016/S0022-0981(00)00159-3
Arimitsu M. L., Piatt J. F., Hatch S., Suryan R. M., Batten S., Bishop M. A., et al. (2021a). Heatwave-induced synchrony within forage fish portfolio disrupts energy flow to top pelagic predators. Global Change Biol. 27, 1859–1878. doi: 10.1111/gcb.15556
Arimitsu M. L., Schoen S., Piatt J., Marsteller C., Drew G. (2021b). Monitoring the recovery of seabirds and forage fish following a major ecosystem disruption in Lower Cook Inlet Vol. 31 (Anchorage, AK: US Department of the Interior, Bureau of Ocean Energy Management. OCS Study BOEM), 50.
Batten S. D., Ostle C., Hélaouët P., Walne A. W. (2022). Responses of Gulf of Alaska plankton communities to a marine heat wave. Deep Sea Res. Part II 195, 105002. doi: 10.1016/j.dsr2.2021.105002
Becker B. H., Beissinger S. R. (2003). Scale-dependent habitat selection by a nearshore seabird, the marbled murrelet, in a highly dynamic upwelling system. Marine Ecol. Prog. Ser. 256, 243–255. doi: 10.3354/meps256243
Becker B. H., Peery M. Z., Beissinger S. R. (2007). Ocean climate and prey availability affect the trophic level and reproductive success of the marbled murrelet, an endangered seabird. Marine Ecol. Prog. Ser. 329, 267–279. doi: 10.3354/meps329267
Bertram D. F., MacDonald C. A., Hara P. O., Cragg J. L., Corcoran R., Greene R., et al. (2023). Summer movements of marbled murrelets from Canada to Alaska. Endangered Species Res. 51, 215–225. doi: 10.3354/esr01252
Bishop M. A., Watson J. T., Kuletz K., Morgan T. (2015). Pacific herring (Clupea pallasii) consumption by marine birds during winter in Prince William Sound, Alaska. Fisheries Oceanography 24, 1–13. doi: 10.1111/fog.2015.24.issue-1
Botz J., Russell C. W., Morella J., Haught S. (2021). 2020 Prince William Sound area finfish management report. Alaska Department of Fish and Game, Fishery Management Report No. 21-18, Anchorage.
Botz J., Scannell H., Morella J. (2022). Prince William Sound Herring Announcement 2 (Alaska Department of Fish and Game). Available at: https://www.adfg.alaska.gov/static/applications/dcfnewsrelease/1441717667.pdf (Accessed March 28, 2024).
Campbell R. W. (2023). “Monitoring the oceanographic conditions of prince william sound,” in Exxon Valdez Oil Spill Long-term Monitoring Program (Gulf Watch Alaska) Final Report (Exxon Valdez Oil Spill Trustee Council Project 21120114-G) (Exxon Valdez Oil Spill Trustee Council, Anchorage, Alaska).
Chambers L. E., Patterson T., Hobday A. J., Arnould J. P., Tuck G. N., Wilcox C., et al. (2015). Determining trends and environmental drivers from long-term marine mammal and seabird data: examples from Southern Australia. Regional Environ. Change 15, 197–209. doi: 10.1007/s10113-014-0634-8
Chen Z., Shi J., Liu Q., Chen H., Li C. (2021). A persistent and intense marine heatwave in the Northeast Pacific during 2019–2020. Geophysical Res. Lett. 48, e2021GL093239. doi: 10.1029/2021GL093239
Clarke K. R., Warwick R. M. (2001). Change in marine communities. approach to Stat. Anal. interpretation 2, 1–168.
Coletti H., Esler D., Ballachey B., Bodkin J., Esslinger G., Kloecker K., et al. (2023). “Gulf watch alaska: nearshore ecosystems in the gulf of alaska,” in Exxon Valdez Oil Spill Restoration Project 2017–2021 Final Report (Restoration Project 21120114-H) (Exxon Valdez Oil Spill Trustee Council, Anchorage, Alaska).
Cushing D. A., Kuletz K. J., Sousa L., Day R. H., Danielson S. L., Labunski E. A., et al. (2024). Differential response of seabird species to warm-and cold-water events in a heterogeneous cross-shelf environment in the Gulf of Alaska. Marine Ecol. Prog. Series. 737, 31–58. doi: 10.3354/meps14239
Dawson N. M., Bishop M. A., Kuletz K. J., Zuur A. F. (2015). Using ships of opportunity to assess winter habitat associations of seabirds in subarctic coastal Alaska. Northwest Sci. 89, 111–128. doi: 10.3955/046.089.0203
Diamond A. W., Devlin C. M. (2003). Seabirds as indicators of changes in marine ecosystems: ecological monitoring on Machias Seal Island. Environ. monitoring assessment. 88, 153–181. doi: 10.1023/A:1025560805788
Di Lorenzo E., Mantua N. (2016). Multi-year persistence of the 2014/15 North Pacific marine heatwave. Nat. Climate Change. 6, 1042–1047. doi: 10.1038/nclimate3082
Drew G., Swingley C., Schoen S. (2023). “U.S. Geological survey, alaska science center,” in SeaLog User Manual, v2.2 (AK 99508, & ABR, Inc., Environmental Research & Services, 2842 Goldstream Rd., Fairbanks, AK 99709, Anchorage). Available at: https://sealog.abrinc.com/installers/SeaLog_User_Manual_v2.2.pdf (Accessed June 10, 2024).
Dunphy B. J., Vickers S. I., Zhang J., Sagar R. L., Landers T. J., Bury S. J., et al. (2020). Seabirds as environmental indicators: foraging behaviour and ecophysiology of common diving petrels (Pelecanoides urinatrix) reflect local-scale differences in prey availability. Marine Biol. 167, 1–12. doi: 10.1007/s00227-020-3672-4
Gabriele C. M., Amundson C. L., Neilson J. L., Straley J. M., Baker C. S., Danielson S. L. (2022). Sharp decline in humpback whale (Megaptera novaeangliae) survival and reproductive success in southeastern Alaska during and after the 2014–2016 Northeast Pacific marine heatwave. Mamm. Biol. 102, 1113–1131. doi: 10.1007/s42991-021-00187-2
Gay S. M. III, Vaughan S. L. (2001). Seasonal hydrography and tidal currents of bays and fjords in Prince William Sound, Alaska. Fisheries Oceanography. 10, 159–193. doi: 10.1046/j.1054-6006.2001.00041.x
Gorman K. B., Kline T. C. Jr., Roberts M. E., Sewall F. F., Heintz R. A., Pegau W. S. (2018). Spatial and temporal variation in winter condition of juvenile Pacific herring (Clupea pallasii) in Prince William Sound, Alaska: Oceanographic exchange with the Gulf of Alaska. Deep Sea Res. Part II 147, 116–126. doi: 10.1016/j.dsr2.2017.10.010
Hatch S. A., Robertson G. J., Baird P. H. (2020). “Black-legged Kittiwake (Rissa tridactyla), version 1.0,” in Birds of the world. Ed. Billerman S. M. (Cornell Lab of Ornithology, Ithaca, NY, USA). doi: 10.2173/bow.bklkit.01
Hipfner J. M. (2008). Matches and mismatches: ocean climate, prey phenology and breeding success in a zooplanktivorous seabird. Marine Ecol. Prog. Series. 368, 295–304. doi: 10.3354/meps07603
Hobday A. J., Oliver E. C., Gupta A. S., Benthuysen J. A., Burrows M. T., Donat M. G., et al. (2018). Categorizing and naming marine heatwaves. Oceanography. 31, 162–173. doi: 10.5670/oceanog.2018.205
Hobson K. A. (1990). Stable isotope analysis of marbled murrelets: evidence for freshwater feeding and determination of trophic level. Condor. 92, 897–903. doi: 10.2307/1368725
Hunt G. L. Jr, Renner M., Kuletz K. (2014). Seasonal variation in the cross-shelf distribution of seabirds in the southeastern Bering Sea. Deep Sea Res. Part II: Topical Stud. Oceanography. 109, 266–281. doi: 10.1016/j.dsr2.2013.08.011
Hyrenbach K. D., Veit R. R. (2003). Ocean warming and seabird communities of the southern California Current System, (1987–98): response at multiple temporal scales. Deep Sea Res. Part II 50, 2537–2565. doi: 10.1016/S0967-0645(03)00123-1
Institute for Seabird Research and Conservation (2023). Middleton Island Seabird Research and Monitoring 2023 Field Report. Anchorage, Alaska.
Jones T., Parrish J. K., Peterson W. T., Bjorkstedt E. P., Bond N. A., Balance L. T., et al. (2018). Massive mortality of a planktivorous seabird in response to a marine heatwave. Geophysical Res. Letters. 45, 3193–3202. doi: 10.1002/2017GL076164
Kokubun N., Yamamoto T., Sato N., Watanuki Y., Will A., Kitaysky A. S., et al. (2016). Foraging segregation of two congeneric diving seabird species breeding on St. George Island, Bering Sea. Biogeosciences. 13, 2579–2591. doi: 10.5194/bg-13-2579-2016
Kotzerka J., Hatch S. A., Garthe S. (2011). Evidence for foraging-site fidelity and individual foraging behavior of pelagic cormorants rearing chicks in the Gulf of Alaska. Condor. 113, 80–88. doi: 10.1525/cond.2011.090158
Kuletz K. J. (2005). Foraging behavior and productivity of a non-colonial seabird, the marbled murrelet (Brachyramphus marmoratus), relative to prey and habitat. University of Victoria, Victoria, BC, 173–195.
Lance B. K., Iron D. B., Kendall S. J., McDonald L. L. (2001). An evaluation of marine bird population trends following the Exxon Valdez oil spill, Prince William Sound, Alaska. Marine Pollution Bulletin. 42, 298–309. doi: 10.1016/S0025-326X(00)00155-7
Leising A. W., Schroeder I. D., Bograd S. J., Abell J., Durazo R., Gaxiola-Castro G., et al. (2015). “State of the california current 2014-15: impacts of the warm-water” Blob”,” in California Cooperative Oceanic Fisheries Investigations Reports (San Diego, California: Scripps Institution of Oceanography), 56.
LeValley R. (2009). Seabird and marine mammal monitoring at Gualala Point Island, California, Sonoma County, May to August 2008 (The Sea Ranch California Coastal National Monument Stewardship Task Force and the Bureau of Land Management, Department of Interior), 51 pp.
Lewandoski S., Bishop M. A. (2018). Distribution of juvenile Pacific herring relative to environmental and geospatial factors in Prince William Sound, Alaska. Deep Sea Res. Part II 147, 98–107. doi: 10.1016/j.dsr2.2017.08.002
Lukacs P. M., Kissling M. L., Reid M., Gende S. M., Lewis S. B. (2010). Testing assumptions of distance sampling on a pelagic seabird. The Condor 112(3), 455-459.
Marsteller C. E., Arimitsu M. L., Schoen S. K., Stark S. B., Piatt J. F. (2024). Predator disturbance contributed to Common Murre Uria aalge breeding failures in Cook Inlet, Alaska following the 2014–2016 Pacific marine heatwave. Marine Ornithology. 52, 129–139.
McCabe R. M., Hickey B. M., Kudela R. M., Lefebvre K. A., Adams N. G., Bill B. D., et al. (2016). An unprecedented coastwide toxic algal bloom linked to anomalous ocean conditions. Geophysical Res. letters. 43, 10–366. doi: 10.1002/2016GL070023
McClatchie S., Field J., Thompson A. R., Gerrodette T., Lowry M., Fiedler P. C., et al. (2016). Food limitation of sea lion pups and the decline of forage off central and southern California. R. Soc. Open Science. 3, 150628. doi: 10.1098/rsos.150628
McGowan D. W., Branch T. A., Haught S., Scheuerell M. D. (2021). Multi-decadal shifts in the distribution and timing of Pacific herring (Clupea pallasii) spawning in Prince William Sound, Alaska. Can. J. Fisheries Aquat. Sci. 78, 1611–1627. doi: 10.1139/cjfas-2021-0047
McKinstry C. A., Campbell R. W. (2018). Seasonal variation of zooplankton abundance and community structure in Prince William Sound, Alaska 2009–2016. Deep Sea Res. Part II 147, 69–78. doi: 10.1016/j.dsr2.2017.08.016
Meehl G. A., Tebaldi C. (2004). More intense, more frequent, and longer lasting heat waves in the 21st century. Science. 305, 994–997. doi: 10.1126/science.1098704
Morella J. (2023). Aerial survey observations of Pacific herring biomass, marine birds, and marine mammals in Prince William Sound, Alaska, 2008-2021. Research Workspace. doi: 10.24431/rw1k43z
Nelson S. K. (2020). “Marbled Murrelet (Brachyramphus marmoratus), version 1.0,” in Birds of the World. Eds. Poole A. F., Gill F. B. (Cornell Lab of Ornithology, Ithaca, NY, USA). doi: 10.2173/bow.marmur.01
Norcross B. L., Brown E. D., Foy R. J., Frandsen M., Gay S. M., Kline T. C. Jr., et al. (2001). A synthesis of the life history and ecology of juvenile Pacific herring in Prince William Sound, Alaska. Fisheries Oceanography. 10, 42–57. doi: 10.1046/j.1054-6006.2001.00040.x
Oksanen J., Blanchet F. G., Kindt R., Legendre P., Minchin P., O’Hara R., et al. (2013). Package ‘vegan’ (Community ecology package), 1–295. Available at: https://CRAN.R-project.org/package=vegan (Accessed July 10, 2024).
Oliver E. C., Donat M. G., Burrows M. T., Moore P. J., Smal D. A., Alexander L. V., et al. (2018). Longer and more frequent marine heatwaves over the past century. Nat. Communications. 9, 1–12. doi: 10.1038/s41467-018-03732-9
Parrish J. K., Litle K., Dolliver J., Hass T., Burgess H. K., Frost E., et al. (2017). “Defining the baseline and tracking change in seabird populations: the Coastal Observation and Seabird Survey Team (COASST),” in Citizen science for coastal and marine conservation (Routledge) (Milton Park, Abingdon-on-Thames, UK: Taylor & Francis), 19–38.
Paruk J. D., Ever J. W., Barr J. F., Mager J., Piper W. H. (2021). “Common Loon (Gavia immer), version 2.0,” in Birds of the World. Eds. Rodewald P. G., Keeney B. K. (Ithaca, NY, USA: Cornell Lab of Ornithology). doi: 10.2173/bow.comloo.02
Pearson S. F., Keren I., Lance M. M., Raphael M. G. (2022). Non-breeding changes in at-sea distribution and abundance of the threatened marbled murrelet (Brachyramphus marmoratus) in a portion of its range exhibiting long-term breeding season declines. PLoS One 17, e0267165. doi: 10.1371/journal.pone.0267165
Piatt J. F., Sydeman W. J., Wiese F. (2007). Introduction: a modern role for seabirds as indicators. Marine Ecol. Prog. Series. 352, 199–204. doi: 10.3354/meps07070
Piatt J. F., Parrish J. K., Renner H. M., Schoen S. K., Jones T. T., Arimitsu M. L., et al. (2020). Extreme mortality and reproductive failure of common murres resulting from the northeast Pacific marine heatwave of 2014-2016. PLoS One 15, e0226087. doi: 10.1371/journal.pone.0226087
Pinchuk A. I., Batten S. D., Strasburger W. W. (2021). Doliolid (Tunicata, thaliacea) blooms in the southeastern Gulf of Alaska as a result of the recent marine heat wave of 2014–2016. Front. Marine Science. 8, 625486. doi: 10.3389/fmars.2021.625486
Ramos J. A., Furness R. W. (2022). “Seabirds as indicators of forage fish stocks,” in Volume 1: Seabird Biodiversity and Human Activities (CRC Press) (Milton Park, Abingdon-on-Thames, UK: Taylor & Francis), 137–148.
Rand P. S., Thorne R. E. (2018). “Long-term herring research and monitoring program final report,” in Expanded Adult Herring surveys. Final Report (Exxon Valdez Oil Spill Trustee Council Project 16120111-E) (Exxon Valdez Oil Spill Trustee Council Project, Anchorage, Alaska).
R Core Team (2023). R: A Language and Environment for Statistical Computing (Vienna, Austria: The R Foundation for Statistical Computing).
Reister I., Danielson S., Aguilar-Islas A. (2024). Perspectives on Northern Gulf of Alaska salinity field structure, freshwater pathways, and controlling mechanisms. Prog. Oceanography. 229, 103373. doi: 10.1016/j.pocean.2024.103373
Renner H. M., Piatt J. F., Renner M., Drummond B. A., Laufenberg J. S., Parrish J. K. (2024). Catastrophic and persistent loss of common murres after a marine heatwave. Science. 386, 1272–1276. doi: 10.1126/science.adq4330
Roberts T. S. (1932). Manual for the identification of the birds of Minnesota and neighboring states (Minneapolis, Minnesota: U of Minnesota Press).
Robinson C. L., Bertram D. F., Shannon H., von Biela V. R., Greentree W., Duguid W., et al. (2024a). Reduction in overwinter body condition and size of Pacific sand lance has implications for piscivorous predators during marine heatwaves. Marine Ecol. Prog. Series. 737, 89–99. doi: 10.3354/meps14257
Robinson B., Coletti H. A., Ballachey B., Bodkin J. L., Kloecker K., Traiger S. B., et al. (2024b). Lack of strong responses to the Pacific marine heatwave by benthivorous marine birds indicates importance of trophic drivers. Marine Ecol. Prog. Series. 737, 215–226. doi: 10.3354/meps14384
Ronconi R. A., Burger A. E. (2011). Foraging space as a limited resource: inter-and intra-specific competition among sympatric pursuit-diving seabirds. Can. J. Zoology. 89, 356–368. doi: 10.1139/z11-006
Ross T., Jackson J., Hannah C. (2021). The Northeast Pacific: Update on marine heatwave status and trends. PICES Press 29, 46–48.
Savage K. (2017). Alaska and British Columbia large whale unusual mortality event summary report. (Juneau, Alaska: NOAA).
Schaefer A. L., Bishop M. A. (2023). “Long-term monitoring of marine bird abundance and habitat associations during fall and winter in Prince William Sound,” in Exxon Valdez Oil Spill Long-term Monitoring Program (Gulf Watch Alaska) Final Report (Exxon Valdez Oil Spill Trustee Council Project 21120114-E) (Exxon Valdez Oil Spill Trustee Council Project, Anchorage, Alaska).
Schaefer A. L., Bishop M. A., Thorne R. (2020). Marine bird response to forage fish during winter in subarctic bays. Fisheries Oceanography. 29, 297–308. doi: 10.1111/fog.12472
Schoen S. K., Arimitsu M. L., Marsteller C. E., Piatt J. F. (2024). Lingering impacts of the 2014–2016 northeast Pacific marine heatwave on seabird demography in Cook Inlet, Alaska (USA). Marine Ecol. Prog. Series. 737, 121–136. doi: 10.3354/meps14177
Stocking J., Bishop M. A., Arab A. (2018). Spatio-temporal distributions of piscivorous birds in a subarctic sound during the nonbreeding season. Deep Sea Res. Part II 147, 138–147. doi: 10.1016/j.dsr2.2017.07.017
Suryan R. M., Arimitsu M. L., Coletti H. A., Hopcroft R. R., Lindeberg M. R., Barbeaux S. J., et al. (2021). Ecosystem response persists after a prolonged marine heatwave. Sci. Reports. 11, 6235. doi: 10.1038/s41598-021-83818-5
Thorne R. E. (2010). Trends in adult and juvenile herring distribution and abundance in Prince William Sound Exxon Valdez Oil Spill Restoration Project Final Report, (Restoration Project 070830) (Cordova, Alaska: Prince William Sound Science Center).
USFWS (2007). North Pacific pelagic seabird observer program observer’s manual, inshore/small vessel version, November 2007. U.S. Fish and Wildlife Service, Migratory Bird Management Nongame Program, Anchorage, Alaska. Unpublished protocol manual, 25 pp.
von Biela V. R., Arimitsu M. L., Piatt J. F., Heflin B., Schoen S. K., Trowbridge J. L., et al. (2019). Extreme reduction in nutritional value of a key forage fish during the Pacific marine heatwave of 2014-2016. Marine Ecol. Prog. Series. 613, 171–182. doi: 10.3354/meps12891
von Biela V. R., Sergeant C. J., Carey M. P., Liller Z., Russell C., Quinn-Davidson S., et al. (2022). Premature mortality observations among Alaska’s Pacific Salmon during record heat and drought in 2019. Fisheries. 47, 157–168. doi: 10.1002/fsh.10705
Weitzman B., Konar B., Iken K., Coletti H., Monson D., Suryan R., et al. (2021). Changes in rocky intertidal community structure during a marine heatwave in the northern Gulf of Alaska. Front. Marine Science. 8, 556820. doi: 10.3389/fmars.2021.556820
Keywords: Brachyramphus marmoratus, Gulf of Alaska, marine bird, marine heatwave, nonbreeding season, Prince William Sound, Uria aalge
Citation: Hoepfner SA, Schaefer AL and Bishop MA (2025) Shifts in marine bird abundance and species composition following the 2014−2016 Pacific marine heatwave. Front. Mar. Sci. 12:1575748. doi: 10.3389/fmars.2025.1575748
Received: 12 February 2025; Accepted: 14 April 2025;
Published: 12 May 2025.
Edited by:
Stelios Katsanevakis, University of the Aegean, GreeceReviewed by:
Zachary Cannizzo, National Oceanic and Atmospheric Administration (NOAA), United StatesSarah K. Schoen, U.S. Geological Survey, Alaska, United States
Adrian Gall, ABR, Inc., United States
Copyright © 2025 Hoepfner, Schaefer and Bishop. This is an open-access article distributed under the terms of the Creative Commons Attribution License (CC BY). The use, distribution or reproduction in other forums is permitted, provided the original author(s) and the copyright owner(s) are credited and that the original publication in this journal is cited, in accordance with accepted academic practice. No use, distribution or reproduction is permitted which does not comply with these terms.
*Correspondence: Anne L. Schaefer, YXNjaGFlZmVyQHB3c3NjLm9yZw==