- Institute of Microbiology, University of Innsbruck, Innsbruck, Austria
Despite being of high biotechnological relevance, many aspects of organic acid excretion in filamentous fungi like the influence of ambient pH are still insufficiently understood. While the excretion of an individual organic acid may peak at a certain pH value, the few available studies investigating a broader range of organic acids indicate that total organic acid excretion rises with increasing external pH. We hypothesized that this phenomenon might be a general response of filamentous fungi to increased ambient pH. If this is the case, the observation should be widely independent of the organism, growth conditions, or experimental design and might therefore be a crucial key point in understanding the function and mechanisms of organic acid excretion in filamentous fungi. In this study we explored this hypothesis using ammonium-limited chemostat cultivations (pH 2–7), and ammonium or phosphate-limited bioreactor batch cultivations (pH 5 and 7). Two strains of Penicillium ochrochloron were investigated differing in the spectrum of excreted organic acids. Confirming our hypothesis, the main result demonstrated that organic acid excretion in P. ochrochloron was enhanced at high external pH levels compared to low pH levels independent of the tested strain, nutrient limitation, and cultivation method. We discuss these findings against the background of three hypotheses explaining organic acid excretion in filamentous fungi, i.e., overflow metabolism, charge balance, and aggressive acidification hypothesis.
Introduction
Although commercial organic acid production with filamentous fungi is of increasing biotechnological importance (Sauer et al., 2008), many aspects of organic acid excretion are still poorly understood. This is partly due to the multitude of influencing factors such as small changes in medium composition (e.g., sugar concentration, presence or absence of trace elements, phosphate or ammonium limitation) or cultivation conditions (e.g., aeration, agitation, pH). To complicate matters further, the influence of these factors often varies substantially between organisms and strains (Kristiansen et al., 1982; Gradisnik-Grapulin and Legisa, 1996).
Ambient pH strongly influences growth and organic acid excretion in filamentous fungi. When grown in an unbuffered medium, filamentous fungi often rapidly acidify their environment to very low and even sometimes detrimental pH values (Magnuson and Lasure, 2004). The major mechanism behind this acidification is controversially discussed, but is most often attributed to either organic acid excretion (Magnuson and Lasure, 2004; Andersen et al., 2009) or proton release by the plasma membrane H+-ATPase (Sanders, 1988; Jernejc and Legisa, 2001). From a biotechnological point of view, however, ambient pH is an important process parameter, e.g., in Aspergillus niger maximal citric acid production occurs below pH 2 (Papagianni, 2007), gluconic acid production at pH 4.5–6.5 (McCullough et al., 1986) and oxalic acid excretion above pH 5 (Ruijter et al., 1999).
The excretion of a single organic acid may peak at a certain pH value, whereas the total amount of excreted organic acids seems to follow a different progress, although systematic investigations of this phenomenon for a broader range of organic acids are scarce. The only two available studies on this subject, namely on Neurospora crassa (Slayman et al., 1990; C. L. Slayman and P. Kaminski, unpublished results, Figure 1 with kind permission) and A. niger (Andersen et al., 2009), indicate that total organic acid excretion increases with rising pH despite huge differences in experimental conditions (i.e., pH-stat incubation with non-growing hyphae versus bioreactor batch cultures). We therefore hypothesized that this phenomenon might be a general response of filamentous fungi to increased ambient pH. If this hypothesis is correct then this observation should be widely independent of the organism, growth conditions, or experimental design. Furthermore, if this is a general response of filamentous fungi to ambient pH, these finding might be a crucial key point in understanding the function and mechanisms of organic acid excretion in filamentous fungi.
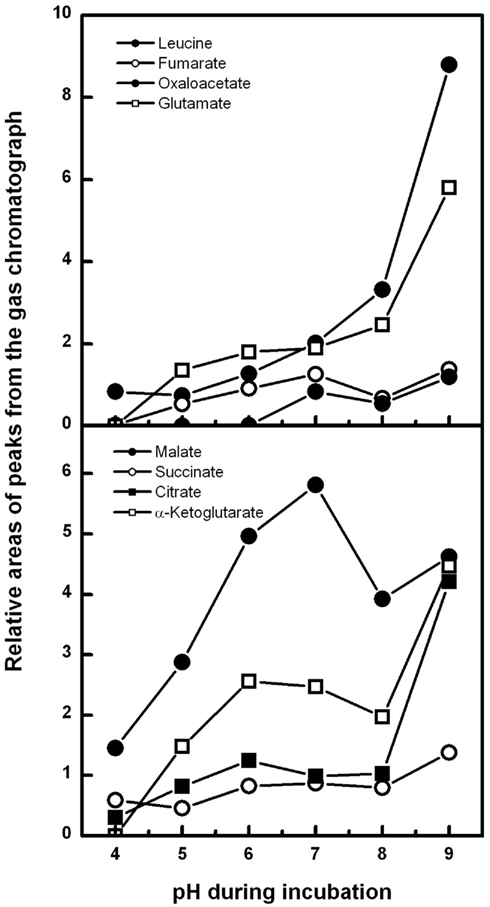
Figure 1. Organic acid excretion of non-growing hyphae of Neurospora crassa in response to different ambient pH. Hyphae (average length ∼300 μm) were grown in shaking flasks cultures, harvested, washed, and transferred to a pH-stat at 25°C (resuspension solution 10 mM KCl, 1% glucose). The culture broth was immediately titrated to a defined pH between 4 and 9. Samples were taken after 40 min and analyzed via gas chromatography. The amount of excreted organic acid anions roughly matched the calculated H+-release. Methods and data derived from Slayman et al. (1990), Rivetta et al. (2011), and unpublished results from C. L Slayman and P. Kaminski (redrawn and modified with kind permission).
This work draws on these two previous studies and explores our hypothesis using two Penicillium ochrochloron strains which differ in the spectrum of excreted organic acids. Experiments comprised ammonium-limited chemostat cultivations ranging from pH 2 to 7 and ammonium or phosphate-limited bioreactor batch experiments with high sampling frequency at pH 5 and 7. P. ochrochloron is a heavy metal-resistant fungus (Tuthill et al., 2001) and was used for bioleaching of industrial waste (Schinner and Burgstaller, 1989; Burgstaller et al., 1992).
Materials and Methods
Organism and Strains
In this work we used two different strains of P. ochrochloron, a fungus, which is known to tolerate high heavy metal concentrations (Tuthill et al., 2001). The first strain, P. ochrochloron CBS 123823, an isolate from the soil of the copper works Montanwerke Brixlegg (Tyrol, Austria) in 1986, was incorrectly identified in 1989 by the Centraalbureau voor Schimmelcultures (CBS, The Netherlands) as Penicillium simplicissimum (Franz et al., 1991). The second strain, P. ochrochloron CBS 123824 emerged from the wild type strain CBS 123823 over 20 years of passaging on rye. Both strains were recently re-identified by three independent institutions as the close related species P. ochrochloron (Vrabl et al., 2008).
Precultures
All bioreactors were inoculated with filamentous mycelium pregrown in a HEPES–glucose medium for 60 h as previously described (Gallmetzer et al., 1998).
Bioreactor Chemostat Experiments
Chemostat experiments were performed in a Biostat M bioreactor (Sartorius/Braun, working volume of 1.7 L, bioreactor equipped with an overflow device) under ammonium limitation (medium composition see Gallmetzer and Burgstaller, 2002; cultivation conditions as described in Gallmetzer and Burgstaller, 2001). For pH-shift experiments the culture pH was raised stepwise (Gallmetzer, 2000): starting with an initial pH of 2, the culture pH was adjusted with sodium hydroxide to the desired next pH level (pH 4, 6, and 7). The chemostat cultures typically reached a new steady state (assumed with onset of constant oxygen consumption and biomass concentration) after passing four to six generation times following a shift in pH. Once a steady state was established, samples were withdrawn regularly from the bioreactor over a minimum time period of 30 h (at least four sampling points; for each sampling point three samples were taken and averaged) to monitor residual glucose concentration, organic acid production, and biomass concentration.
Bioreactor Batch Experiments
Prior to inoculation to the bioreactor, the preculture was harvested under sterile conditions by filtration (0.2 μm, cellulose acetate) and washed with approximately 200 mL sterile distilled water. The mycelial cake was transferred aseptically to the bioreactors (either Biostat M, Sartorius/Braun, Melsungen, Germany or KLF 2000 Bioengineering, Wald, Switzerland). Both bioreactors were operated with a working volume of 1.7 L. Equipment and culture conditions were according to Vrabl et al. (2008, 2009). The pH was kept constant with 1 M sodium hydroxide. External pH and dissolved oxygen tension were monitored with an online data acquisition system (Fa. Gatterbauer Messtechnik, Sipbachzell, Austria).
The medium for bioreactor batch cultivation was a modified preculture medium and concentrations are the same for ammonium and phosphate-limited conditions unless stated otherwise (mM): glucose × 1 H2O (400), (NH4)2SO4 (NH4 limitation: 5, PO4 limitation 6.25), NH4Cl (NH4 limitation: 10, PO4 limitation: 12.5), KH2PO4 (NH4 limitation: 5.8, PO4 limitation: 0.5), MgSO4 × 7 H2O (1.6), 1 g L−1 antifoam agent (Clerol FBA 5075, Cognis, Germany) and 10 mL L−1 of trace element solution (composition of the trace element solution as in Vrabl et al., 2008). Glucose and salts were sterilized at 121°C separately, whereas the trace element solution was added after sterilization via filtration. Prior to inoculation, the pH was adjusted with 1 M sodium hydroxide to either pH 5 or 7. Each nutrient limitation was performed with both strains at pH 5 and 7. All conditions were performed at least in triplicates, except for strain CBS 123824 the phosphate limitation at pH 5 was performed in duplicate. The single experiments were limited by the small working volume (1.7 L) of the bioreactors. To disturb the cultivations as little as possible by the high sampling frequency, only one sample per time point was taken. Samples (10 mL) were withdrawn from the bioreactor at regular time intervals and immediately filtrated (cellulose acetate filter, pore size 0.45 μm). The mycelial cake was used for dry weight estimation. The filtrate was rapidly aliquotated into Eppendorf tubes and frozen in a cold bath (−40°C; Frykatherm) for later analysis of extracellular organic acids and residual nutrient concentrations. Cultivations were monitored at least for 40 h after inoculation.
pH Transients
A phosphate-limited culture with the strain CBS 123824 was started at a pH of 7. Without sodium hydroxide titration overnight, the fungus acidified the medium during the lag and exponential phase until the next morning. From then the pH was kept constant at pH 5 throughout the experiment. These experiments were performed twice.
Analytics
Extracellular nutrient and organic acid concentrations were determined in culture filtrates, which were diluted prior to analysis if necessary. Glucose was measured with a Megazyme Test Kit for D-Glucose (GOPOD Format, K-GLUC, Megazyme International Ireland Ltd., Wicklow, Ireland), ammonium according to the macroscale method of Rhine et al. (1998) and phosphate as described by Illmer (1996). Organic acids were determined according to Womersley et al. (1985) on a Bio-Rad Aminex HPX-87H column with 4 mN sulfuric acid as mobile phase, a temperature of 41°C and a flow rate of 0.6 mL min−1. Dry weight was estimated as previously described (Vrabl et al., 2009).
Calculations
Rates for nutrient uptake, organic acid excretion, or sodium hydroxide titration were calculated by differentiation of the respective nutrient, organic acid, or sodium hydroxide versus time curves as previously described (Vrabl et al., 2009).
Although bioreactor batch experiments showed comparable tendencies, there were slight temporal shifts between parallel experiments, e.g., caused by small differences in culture history. These temporal shifts are particularly important when considering dynamic processes like the short spike in pH shown in Figure 4 or 6: Averaging such curves can conceal real dynamics and should be avoided. We therefore depicted one representative (e.g., Figures 4 and 5) dataset or all three datasets for each experimental condition.
Results
This study explores total organic acid excretion in P. ochrochloron in response to ambient pH with a series of ammonium-limited chemostat cultivations ranging from pH 2 to 7 and ammonium or phosphate-limited bioreactor batch cultivations at pH 5 and 7. Chemostat experiments allow the investigation of parameters at a constant physiological state, i.e., unchanging nutritional conditions, growing hyphae, and constant specific growth rate. In contrast, bioreactor batch cultures pass through vastly changing nutritional conditions (from unlimited to limited up to starvation), specific growth rates ranging from zero up to μmax and consequently strongly varying physiological states.
Conditions for the bioreactor batch experiments were chosen for the following reasons: (i) Distinct differences in the level of organic acid excretion were to be expected between pH 5 and 7 (derived from the chemostat experiments, Figure 2). (ii) Ammonium- or phosphate-limited conditions were known to lead to the most pronounced organic acid excretion in P. ochrochloron, and furthermore, differ in the spectrum of excreted organic acids (Gallmetzer and Burgstaller, 2002). (iii) All bioreactor batch experiments were performed with two different P. ochrochloron strains which were known to strongly differ in the spectrum of excreted organic acids (as demonstrated in Figure 3).
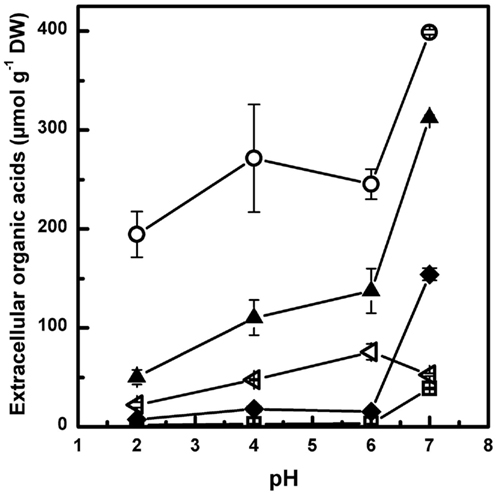
Figure 2. Organic acid excretion of NH4-limited chemostat cultures of Penicillium ochrochloron CBS 123824 in response to different ambient pH (D = 0.090 h−1; 30°C). After a shift in pH, four to six generation times passed before a new steady state was established. Steady state was assumed as soon as the oxygen consumption was constant. Data for each steady state are means of at least four sampling points over a time period of at least 30 h. Citrate (▲), oxalate (♦), fumarate (□), malate (○), and succinate (◁). Redrawn and modified with kind permission from Gallmetzer (2000).
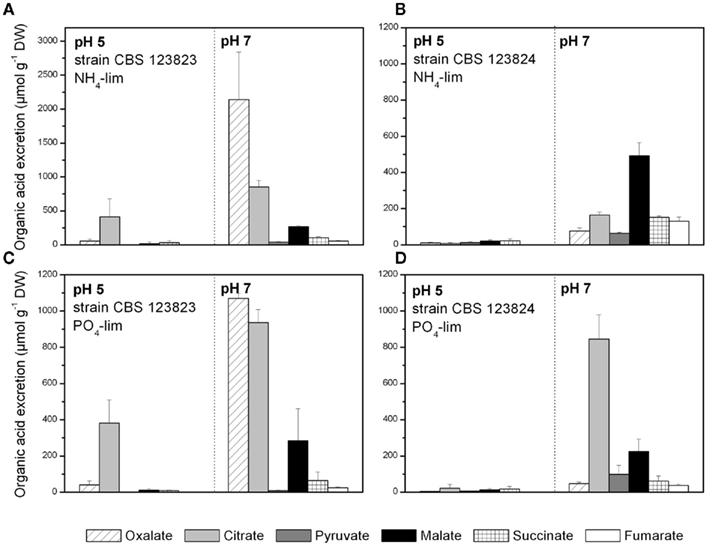
Figure 3. Organic acid excretion of ammonium (A,B) or phosphate (C,D) limited Penicillium ochrochloron in bioreactor batch cultures at pH 5 and 7. (A,C) Strain CBS 123823; (B,D) strain CBS 123824. Sampling points for the depicted data were for (A) pH 5 at 41.8 ± 1.38 h and pH 7 at 39.3 ± 0.32 h of cultivation, (B) pH 5 at 42.2 ± 0.48 h and pH 7 at 41.4 ± 0.57 h of cultivation, (C) pH 5 at 35.0 ± 0.65 h and pH 7 at 34.33 ± 0.88 h of cultivation, and (D) pH 5 at 36.7 ± 0.33 h and pH 7 at 34.5 ± 0.49 h of cultivation. Data are means of three independent bioreactor batch cultivations (single curves see Figures 4–6), except phosphate-limited cultures of strain CBS123824 at pH 5 (D), which were performed twice.
Overall Findings
Under all tested conditions, total organic acid excretion in P. ochrochloron increased with rising external pH. This observation was independent whether the fungus was grown under ammonium-limited conditions in a chemostat between pH 2 and 7 (Figure 2) or in highly dynamic bioreactor batch cultivations under ammonium or phosphate-limited conditions (Figures 3–5). In contrast, the spectrum of excreted organic acids varied strongly with pH, strain and nutrient limitation: While oxalate and citrate were the predominant organic acids of the strain CBS 123823 of both nutrient limitations at pH 7 (Figures 3A,C), solely citrate was excreted in considerable amounts at pH 5. In strain CBS 123824 malate was the main excreted organic acid during ammonium-limited batch cultures at pH 7, whereas at pH 5 succinate and malate were predominant (Figure 3B). The same strain excreted mainly citrate during phosphate limitation (Figure 3D).
In addition to cultivations at pH 5 and 7, phosphate-limited cultures of the strain CBS 123824 were exposed to a transient in pH from pH 7 to 5 during the lag and exponential phase, i.e., starting with an initial pH of 7, the fungus was allowed to acidify the medium until reaching pH 5 which was kept constant until the end of cultivation. In these experiments the amount of excreted organic acids was in the magnitude of cultivations at pH 5 (data not shown).
Detailed Dynamics of the Bioreactor Batch Experiments
In contrast to chemostat studies, bioreactor batch experiments depict complex nutritional and physiological changes. Because both strains showed comparable dynamics (Figures 4 and 5), we demonstrate the main findings with the P. ochrochloron strain CBS 123823, i.e., the strain which excretes more organic acids (as demonstrated in Figure 3). At any pH P. ochrochloron excreted organic acids during the whole cultivation, i.e., low amounts of organic acids were excreted during exponential growth but the excretion was strongly enhanced on depletion of either ammonium or phosphate (exception see Unusual Findings).
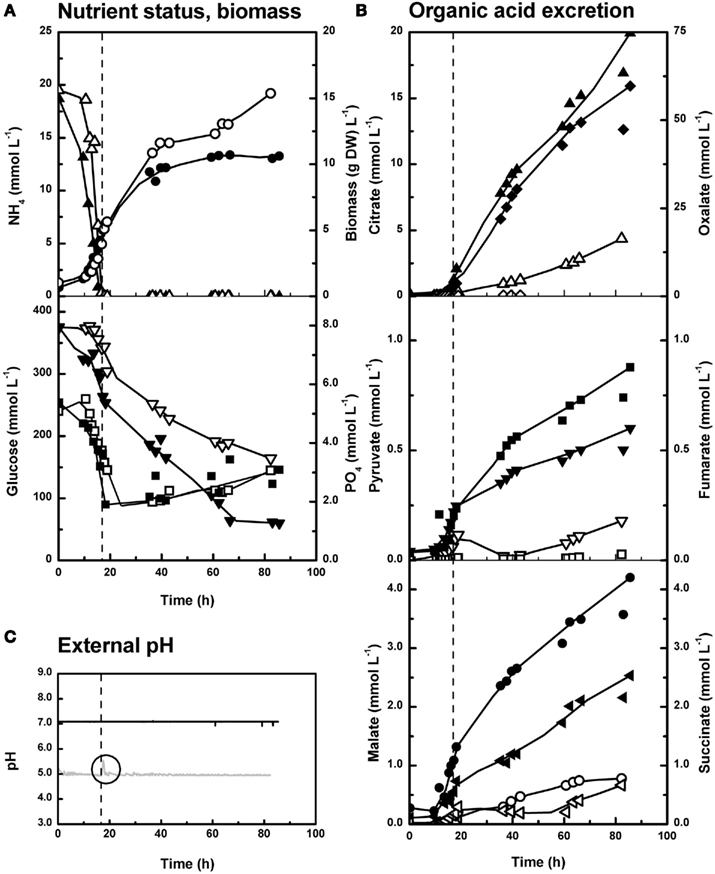
Figure 4. Organic acid excretion of Penicillium ochrochloron CBS 123823 in NH4-limited bioreactor batch cultures at pH 5 and 7. Closed symbols, pH 7; open symbols pH 5. The vertical line marks the exhaustion of ammonium and thus the end of exponential growth. (A) Dry weight (●○) and residual concentrations of ammonium (▲△), phosphate (■□), and glucose (▼▽). (B) Extracellular concentrations of citrate (▲△), oxalate (♦◊), pyruvate (▼▽), fumarate (■□), malate (●○), and succinate (◀◁). (C) External pH. Note the short rise in pH at pH 5 after exhaustion of ammonium. Representative experiments are shown. Average of three independent experiments from one specific time point of cultivation is depicted in Figure 3 (for pH 5 at 41.8 ± 1.38 h and pH 7 at 39.3 ± 0.32 h of cultivation).
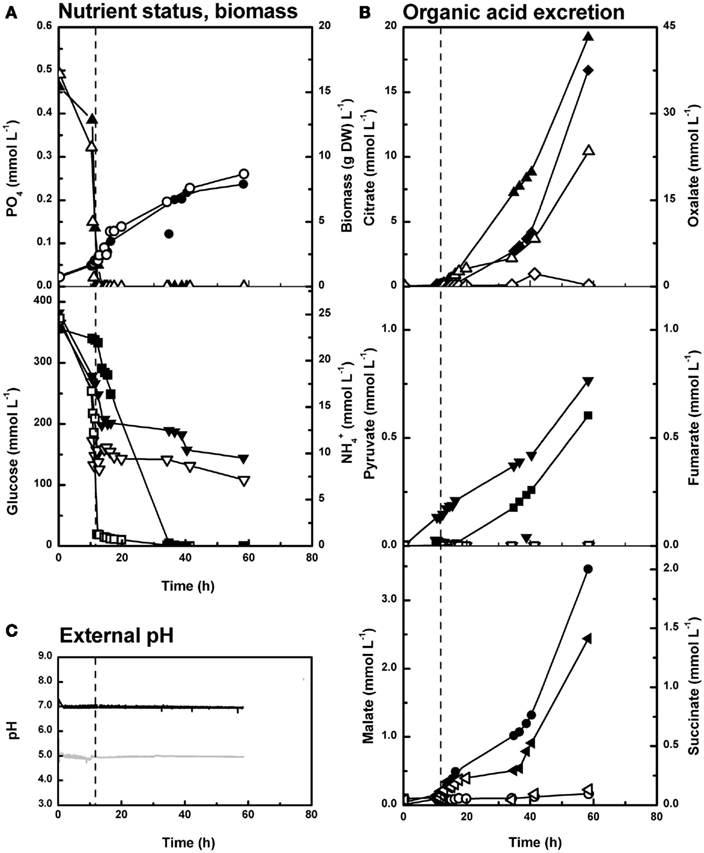
Figure 5. Organic acid excretion of Penicillium ochrochloron CBS 123823 in PO4-limited bioreactor batch cultures at pH 5 and 7. Closed symbols, pH 7; open symbols pH 5. The vertical line marks the exhaustion of phosphate and thus the end of exponential growth. (A) Dry weight (●○) and residual concentrations of phosphate (▲△), ammonium (■□), and glucose (▼▽). (B) Extracellular concentrations of citrate (▲△), oxalate (♦◊), pyruvate (▼▽), fumarate (■□), malate (●○), and succinate (◀◁). (C) External pH. Representative experiments are shown. Average of three independent experiments from one specific time point of cultivation is depicted in Figure 3 (pH 5 at 42.2 ± 0.48 h and pH 7 at 41.4 ± 0.57 h of cultivation).
The course of ammonium or phosphate uptake in ammonium-limited cultures (Figure 4A) did not significantly differ between pH 5 and 7 (note that phosphate uptake more or less halted after ammonium had been exhausted). In contrast to ammonium and phosphate, glucose was consumed faster at pH 7 than at pH 5 despite a lower biomass concentration at pH 7 (Figure 4A). This is also reflected in a decreasing biomass yield on glucose with increasing pH (Table 1).
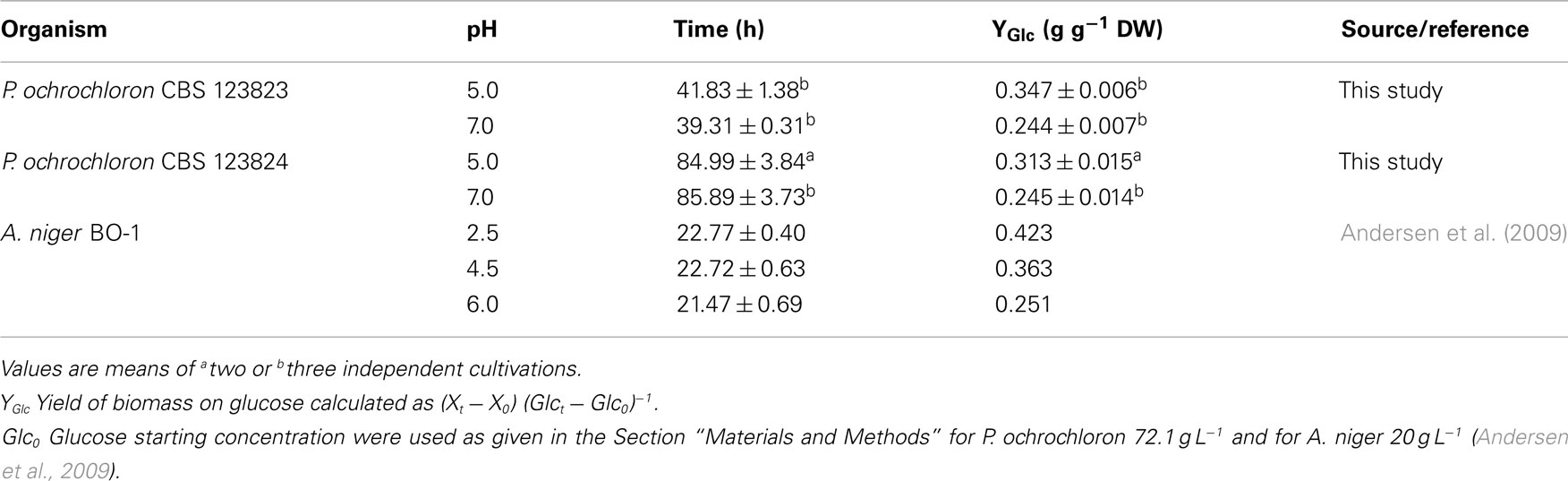
Table 1. Yield on glucose at different ambient pH for Aspergillus niger and ammonium-limited batch cultures of Penicillium ochrochloron.
In phosphate-limited cultures at pH 5 (Figure 5A), P. ochrochloron utilized ammonium considerably faster than at pH 7, leading surprisingly to an early double limitation. Thus, biomass at pH 5 and 7 remained approximately at the same level. Similar to ammonium-limited experiments, the course of phosphate uptake did not differ between pH 5 and 7. When measuring the glucose concentrations of phosphate-limited cultures, we often observed an extreme scattering of the values, especially at pH 7. This scattering was independent of the analytical method, i.e., photometrical assay or HPLC, or the bioreactor used. Interestingly we did not observe this problem with any ammonium-limited experiment, although sampling and sample preparation were the same. From experiments with less scattering such as depicted in Figure 5, the glucose consumed per g dry weight seemed to be almost in the same order of magnitude.
Unusual Findings
The pH was kept constant with sodium hydroxide throughout all cultivations, because we expected only an acidification of the medium in these early stages. Surprisingly the external pH shortly increased in ammonium-limited cultures of the strain CBS 123823 at pH 5 after exhaustion of ammonium (Figure 4C). This effect was even more distinct with the strain CBS 123824 (Figure 6A). In this strain the short spike in pH after exhaustion of ammonium was followed by a wavelike sequence of increases and decreases.
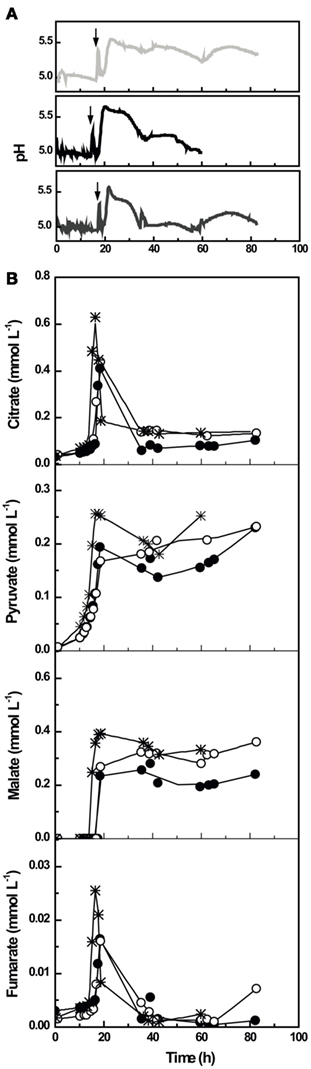
Figure 6. Organic acid excretion and reuptake of organic acids in NH4-limited bioreactor batch cultures of Penicillium ochrochloron CBS 123824 at pH 5 illustrated with three independent cultivations. (A) Progress of extracellular pH of three independent cultivations in the following order: Batch #1 (gray line), batch #2 (black line), batch #3 (dark gray line). The exhaustion of ammonium is marked with an arrow. Note that culture pH was controlled only by sodium hydroxide. (B) Progress of organic acid excretion of three independent cultivations exemplified with citrate, pyruvate, malate, and fumarate. Batch #1 (○), batch #2 (✻), batch #3 (●). Average of three independent experiments from one specific time point of cultivation is depicted in Figure 3 (at 35.0 ± 0.65 h of cultivation).
Although glucose was present in excess at this time, some organic acids seemed to be reutilized (Figure 6B): While citrate and fumarate seemed to be almost completely taken up, oxalate excretion increased steadily (data for oxalate not shown). On the other hand, pyruvate, malate, and succinate (data for succinate not shown) remained on the same level or followed a wavelike progress. Likewise, the strain CBS 123823 occasionally seemed to reutilize organic acids (e.g., pyruvate in Figure 4B) despite extracellular abundance of glucose.
Discussion
Increased Extracellular pH Leads to Enhanced Total Organic Acid Excretion
The main finding of this study was that the total amount of excreted organic acids in P. ochrochloron increased with rising pH – irrespective of the mode of cultivation (chemostat, batch cultivation), nutrient limitation (ammonium, phosphate limitation), or strain (Figures 2–5). As far as we are aware, only two other studies address this topic for a broader range of organic acids, i.e., a pH-stat study of N. crassa (Slayman et al., 1990; C. L. Slayman and P. Kaminski, unpublished results, Figure 1 with kind permission) and a bioreactor batch study of A. niger (Andersen et al., 2009). Both studies observe increasing organic acid excretion with rising external pH.
Apparently this observation holds true despite huge differences in experimental setting (e.g., time frame, cultivation mode, or nutrient limitation) or physiological status of the mycelia (e.g., chemostat mycelium growing at a defined specific growth rate versus batch cultures at varying growth rates or non-growing hyphae in a pH-stat). This confirms our hypothesis that this observation might be very well a general response of filamentous fungi to increased ambient pH. It could thus provide a key point in understanding the function and mechanisms of organic acid excretion in these organisms.
The physiological reason behind this phenomenon still remains unclear and becomes particularly intriguing when one considers the comparably high metabolic costs involved in organic acid production. Why would total organic acid excretion be increased at higher pH levels at all?
One possible explanation is that at higher pH levels the glycolytic flux is elevated and leads as a consequence of energy spilling mechanisms to enhanced level of organic acid excretion (theoretical details on the “overflow metabolism hypothesis” see Box 1). In P. ochrochloron, the data suggest an elevated glycolytic flux at higher pH levels (present study; Gallmetzer, 2000). Similar results can be found for other filamentous fungi, for instance, glucose uptake rates increase with external pH in A. niger (Mischak et al., 1984; Torres et al., 1996) and in Trichoderma harzianum (Delgado-Jarana et al., 2003). In the latter study the authors, however, observed a slight decrease in glucose uptake at pH 6 compared to pH 5. Also decreased yields on glucose at higher pH levels (Table 1) hint in this direction and are frequently reported (e.g., current study, Pitt and Bull, 1982; Andersen et al., 2009).
Box 1. Overflow metabolism hypothesis. The term was coined by Foster (1949) for filamentous fungi. Neijssel and Tempest elaborated the hypothesis and expanded it to bacteria (Tempest and Neijssel, 1992). Organic acid excretion is regarded as one of several mechanisms to spill energy when growth is limited by a non-carbon nutrient and the carbon source is in excess. Various sub-hypotheses differ in the location of the bottleneck (glycolysis, TCA cycle, respiratory chain). Overflow metabolism was often found to be linked with an increased glycolytic flux (Mattey, 1992; Papagianni et al., 1999; Karaffa and Kubicek, 2003; Papagianni, 2007).
A second possible explanation for increased organic acid production at high external pH is that organic acid anion excretion serves as charge balance mechanism (theoretical details on the “charge balance hypothesis” see Box 2). In literature there is some experimental evidence for an electrical compensation of proton efflux via organic anion excretion, e.g., in non-growing and multiple starved hyphae of N. crassa (personal communication C. L. Slayman), the proton efflux was almost matched by organic anion excretion (Slayman et al., 1990; Rivetta et al., 2011). Roos and Luckner (1984) reported for P. cyclopium that as long as was present in the medium,
uptake provided the necessary charge balance for proton efflux via the plasma membrane H+-ATPase with a 1:1 stoichiometry. After
was exhausted, however, charge balance for proton efflux was ensured by the stoichiometric excretion of organic acid anions. A stoichiometric relationship between ammonium uptake and proton release (or sodium hydroxide titration) has also been reported for several other fungi (e.g., Mattey, 1992; Burgstaller et al., 1994).
Box 2. Charge balance hypothesis. The excretion of organic acids as anions is regarded to be the main charge compensating ion flux for proton excretion via the H+-ATPase when proton return via proton/substrate symports is hindered. This avoids a detrimental hyperpolarization of the plasma membrane (Gradmann et al., 1978). At low external pH the majority of excreted protons return into the cell via proton symport of nutrients. At high external pH – especially when excreted protons are neutralized with sodium hydroxide – proton return is impaired and another charge compensating ion flux must occur. Excretion of organic acid anions can balance proton efflux more ore less stoichiometrically (Conway and Brady, 1950; Sanders, 1988; Slayman et al., 1990; Sigler and Höfer, 1991; Burgstaller et al., 1994).
A third possible explanation for increased organic acid excretion at higher pH levels originates from a hypothesis of Andersen et al. (2009) for A. niger. The authors suggest that fungi excrete at high external pH more protons (in form of organic acids) to acidify the environment and to outcompete rivals (theoretical details on the “aggressive acidification hypothesis” see Box 3). Simulations predicted oxalic acid as the major source of protons at pH levels above pH 3 for strains which are able to excrete all organic acids.
Box 3. Aggressive acidification hypothesis. This hypothesis states that “A. niger strives to produce – at a given pH – the organic acid that most efficiently acidifies the medium” to outcompete rivals (Andersen et al., 2009). Strains, which are able to produce all organic acids, are said to preferably excrete oxalic acid. Citric acid was predicted to be the next best acid for acidification when oxalic acid could not be produced. This hypothesis assumes organic acids to be transported completely protonated (i.e., uncharged) across the plasma membrane so that in this scenario organic acids would be the major source for extracellular acidification. The hypothesis was derived from a stoichiometric model of A. niger metabolism and acid production, optimized for maximal proton generation.
In this context three issues are of special interest. Firstly, some authors attribute the major mechanism behind medium acidification to proton release by the plasma membrane H+-ATPase (e.g., Sanders, 1988; Jernejc and Legisa, 2001) and not to organic acid excretion. The latter would require the transport of fully protonated organic acids across the plasma membrane which is questionable (in depth discussion in “Are Organic Acids Excreted as Anions or Fully Protonated?”). Secondly, in P. ochrochloron but also in other fungi, medium acidification seems to be at least partly a matter of the N-source. When ammonium is present as sole N-source, P. ochrochloron rapidly acidifies the medium to very low pH values (Burgstaller et al., 1994), but not when nitrate is the sole N-source (although organic acids were excreted at the same time; Vrabl, Schinagl, and Burgstaller unpublished results). Similar observations have been reported for other filamentous fungi including A. niger: External pH typically decreases in cultures with ammonium as N-source, but on other N-sources like nitrite or glutamate a slower acidification or even an alkalinization of the medium can be observed (Usami, 1978; Amrane et al., 1999; Jernejc and Legisa, 2001). Thirdly, whilst the model explained the experimental data for A. niger very well (Ruijter et al., 1999; Andersen et al., 2009), it does not seem to apply to the data available from P. ochrochloron (e.g., Figures 2 and 3). For example, oxalic acid was the predominant acid for the strain CBS 123823 only at pH 7 and never for the strain CBS 123824, although both strains are capable of excreting all organic acids. Citric acid was excreted at both pH levels. It was even the predominant excreted organic acid for strain CBS 123823 at pH 5 (Figures 3A,C) and for phosphate-limited cultures of strain CBS 123824 at pH 7 (Figure 3D). These results indicate that oxalic acid is not the major source of protons in P. ochrochloron. Thus in P. ochrochloron the shift in the spectrum of excreted organic acids has to have other reasons than to most efficiently acidify the medium and might be explained as a consequence of changes in cytoplasmatic pH. Although filamentous fungi keep their cytoplasmatic pH surprisingly stable over a broad range of external pH, the cytoplasmatic pH is not entirely unaffected and changes slightly (Hesse et al., 2002). Because the activity of several enzymes is very sensitive to changes between pH 6 and 7 (Mattey, 1992), a shift in external pH can cause a shift in enzyme activity and thus also a shift in the spectrum of excreted organic acids.
Evidently, in each of the three above mentioned explanations organic acid excretion fulfills a completely different metabolic function. Moreover, the assumption on how organic acids are transported across the plasma membrane is mutually exclusive concerning the charge balance hypothesis (transport of organic acid anions) and the aggressive acidification hypothesis (transported fully protonated). We will therefore consider this topic in more detail in the following section.
Are Organic Acids Excreted as Anions or Fully Protonated?
The charge balance hypothesis and the aggressive acidification hypothesis differ in their assumption on which organic acid species are transported across the plasma membrane. This is of particular interest when one considers the question, if organic acids are the major source of protons for ambient acidification or not. The charge balance hypothesis requires excretion of organic acid anions. Thus, depending on the degree of deprotonation and the ambient pH, organic acid anions would contribute only little. In contrast, the aggressive acidification hypothesis postulates organic acids to be excreted fully protonated (Andersen et al., 2009), and consequently, to contribute strongly to the medium acidification.
So far, experimental data on the transported species of organic acids are scarce (Rivetta et al., 2011), but there is a significant argument in favor of anions as the transported species (Mattey, 1977; Roos and Luckner, 1984; Burgstaller, 2006; Rivetta et al., 2011). The cytoplasmic pH is around neutral (Hesse et al., 2002) or, maybe, slightly more acidic near the interior surface of the plasma membrane (Roos and Slavik, 1987). Considering the pKa values of the organic acids and the cytoplasmic pH it follows that organic acids are almost completely in their anionic forms in the cytoplasm. Inversely, the concentrations of fully protonated organic acids are extremely low (Burgstaller, 2006; Garcia and Torres, 2011). This implies that the excretion rates, if calculated from the concentrations of fully protonated species, are far too low to explain the measured excretion rates of organic acids (Burgstaller, 2006). Taken together, it is unlikely that organic acids are transported fully protonated across the fungal plasma membrane and therefore they cannot be considered as major source of protons for extracellular acidification.
What does this mean for medium acidification in general? The plasma membrane H+-ATPase is most probably the main source of protons for medium acidification in fungi (Sanders, 1988; Sigler and Höfer, 1991; Jernejc and Legisa, 2001). Net extracellular acidification, however, results from an interplay between the excreted species of organic acids, proton excretion by the plasma membrane H+-ATPase, the extracellular pH, and the extracellular buffering capacity. Net extracellular acidification may thus be similar whether or not organic acids are excreted fully protonated or as anions accompanied by protons expelled via the H+-ATPase. Because the latter is the more probable scenario, organic acid excretion must serve another purpose (e.g., charge balance, energy spilling, or chelation of trace elements) than merely acidification of the external medium.
Reuptake of Organic Acids in the Presence of Glucose Indicate an Additional Metabolic Function of Organic Acids
Although filamentous fungi typically tend to acidify their medium especially when ammonium serves as N-source, we observed a short uprise in pH after exhaustion of ammonium at pH 5 for both P. ochrochloron strains (Figure 4 and 6) which might be explained the reuptake of organic acids (Figure 6). Several studies report protons disappearing from the medium during dicarboxylic acid uptake, for example in Schizosaccharomyces pombe (Sousa et al., 1992) or Hansenula anomala (Corte-Real and Leao, 1990), which could be the consequence of a dicarboxylate proton symport (Corte-Real and Leao, 1990; Sousa et al., 1992). In some fungi like Candida utilis the transport is repressed when glucose is present (Saayman et al., 2000), whereas in other fungi like S. pombe the transporter seems to be constitutively active, even at high external glucose concentrations (Sousa et al., 1992; Saayman et al., 2000). This might also be the case in P. ochrochloron. Strikingly, in the bacterium Salmonella typhimurium the formate transporter FocA changes its function depending on the external pH, i.e., performing as an exporter at high ambient pH and as an importer at acidic pH values (Lü et al., 2011).
In this respect the work of Roos and Luckner (1984) is of particular interest. After adding [1.5-14C] citric acid to cultures of P. cyclopium under organic acid excreting conditions (i.e., glucose excess), they found a continuous uptake and excretion of citric acid. Uptake dominated at later phases of cultivation which resulted in a net uptake of citric acid. This experiment points out two very important issues: First, external organic acid concentrations require cautious interpretation because they might be the consequence of a continuous influx and efflux and thus represent only a net flux. Second, organic acids may serve an additional, yet unknown metabolic function. Clearly this issue needs more experimental evidences.
Conflict of Interest Statement
The authors declare that the research was conducted in the absence of any commercial or financial relationships that could be construed as a potential conflict of interest.
Acknowledgments
This work was supported by the Austrian Science Fund (FWF) project P22220-B11. The authors thank M. Gallmetzer for kind permission to present Figure 2. We are furthermore indebted to C. L. Slayman and P. Kaminski for their kind permission to present the unpublished data of Figure 1. We thank Olivia Vrabl for critically reading the manuscript.
References
Amrane, A., Plihon, F., and Prigent, Y. (1999). Proton transfer in relation to growth of Geotrichum candidum and Penicillium camemberti in synthetic liquid media. Enzyme Microb. Technol. 24, 561–568.
Andersen, M. R., Lehmann, L., and Nielsen, J. (2009). Systemic analysis of the response of Aspergillus niger to ambient pH. Genome Biol. 10, R47.
Burgstaller, W. (2006). Thermodynamic boundary conditions suggest that a passive transport step suffices for citrate excretion in Aspergillus and Penicillium. Microbiology 152, 887–893.
Burgstaller, W., Strasser, H., Wöbking, H., and Schinner, F. (1992). Solubilization of zinc oxide from filter dust with Penicillium simplicissimum: bioreactor leaching and stoichiometry. Environ. Sci. Technol. 26, 340–346.
Burgstaller, W., Zanella, A., and Schinner, F. (1994). Buffer-stimulated citrate efflux in Penicillium simplicissimum: an alternative charge balancing ion flow in case of reduced proton backflow? Arch. Microbiol. 161, 75–81.
Conway, E. J., and Brady, T. G. (1950). Biological production of acid and alkali. 1. Quantitative relations of succinic and carbonic acids to the potassium and hydrogen ion exchange in fermenting yeast. Biochem. J. 47, 360–369.
Corte-Real, M., and Leao, C. (1990). Transport of malic acid and other carboxylic acids in the yeast Hansenula anomala. Appl. Environ. Microbiol. 56, 1109–1113.
Delgado-Jarana, J., Moreno-Mateos, M. A., and Benítez, T. (2003). Glucose uptake in Trichoderma harzianum: role of gtt1. Eukaryot. Cell 2, 708–717.
Franz, A., Burgstaller, W., and Schinner, F. (1991). Leaching with Penicillium simplicissimum: influence of metals and buffers on proton extrusion and citric acid production. Appl. Environ. Microbiol. 57, 769–774.
Gallmetzer, M. (2000). Wachstum und Säure-Ausscheidung von Penicillium simplicissimum in kontinuierlicher Kultur. Ph.D. thesis, University of Innsbruck, Innsbruck.
Gallmetzer, M., and Burgstaller, W. (2001). Citrate efflux in glucose-limited and glucose-sufficient chemostat culture of Penicillium simplicissimum. Antonie Van Leeuwenhoek 79, 81–87.
Gallmetzer, M., and Burgstaller, W. (2002). Efflux of organic acids in Penicillium simplicissimum is an energy-spilling process, adjusting the catabolic carbon flow to the nutrient supply and the activity of catabolic pathways. Microbiology 148, 1143–1149.
Gallmetzer, M., Müller, B., and Burgstaller, W. (1998). Net efflux of citrate in Penicillium simplicissimum is mediated by a transport protein. Arch. Microbiol. 169, 353–359.
Garcia, J., and Torres, N. (2011). Mathematical modelling and assessment of the pH homeostasis mechanisms in Aspergillus niger while in citric acid producing conditions. J. Theor. Biol. 282, 23–35.
Gradisnik-Grapulin, M., and Legisa, M. (1996). Comparison of specific metabolic characteristics playing a role in citric acid excretion between some strains of the genus Aspergillus. J. Biotechnol. 45, 265–270.
Gradmann, D., Hansen, U.-P., Long, W. S., Slayman, C. L., and Warncke, J. (1978). Current-voltage relationships for the plasma membrane and its principal electrogenic pump in Neurospora crassa: I. Steady-state conditions. J. Membr. Biol. 39, 333–367.
Hesse, S. J. A., Ruijter, H. J. G., Dijkema, C., and Visser, J. (2002). Intracellular pH homeostasis in the filamentous fungus Aspergillus niger. Eur. J. Biochem. 269, 3485–3494.
Illmer, P. (1996). “Total, organic, inorganic and plant-available phosphorus,” in Methods in Soil Biology, eds F. Schinner, R. Öhlinger, E. Kandeler, and R. Margesin (Berlin: Springer Verlag), 412–416.
Jernejc, K., and Legisa, M. (2001). Activation of plasma membrane H+-ATPase by ammonium ions in Aspergillus niger. Appl. Microbiol. Biotechnol. 57, 368–373.
Karaffa, L., and Kubicek, C. P. (2003). Aspergillus niger citric acid accumulation: do we understand this well working black box? Appl. Microbiol. Biotechnol. 61, 189–196.
Kristiansen, B., Charley, R. C., Seviour, B., Harvey, L., Habeeb, S., and Smith, J. E. (1982). “Over-production of metabolites by filamentous fungi,” in Overproduction of Microbial Products, eds V. Krumphanzl, B. Sikyta, and Z. Vanek (London: Academic Press), 195–210.
Lü, L., Juan, D., Wackerl, T., Gergib-Smentek, E., Andradel, S. L. A., and Einsle, O. (2011). pH-Dependent gating in a FocA formate channel. Science 332, 352–354.
Magnuson, J. K., and Lasure, L. L. (2004). “Organic acid production by filamentous fungi,” in Advances in Fungal Biotechnology for Industry, Agriculture, and Medicine, eds J. S. Tkacz and L. Lange (New York: Kluwer Academic/Plenum Publishers), 307–340.
Mattey, M. (1977). Citrate regulation of citric acid production in Aspergillus niger. FEMS Microbiol. Lett. 2, 71–74.
McCullough, W., Roberts, C. F., Osmani, S. A., and Scrutton, M. C. (1986). “Regulation of carbon metabolism in filamentous fungi,” in Carbohydrate Metabolism in Cultured Cells, ed. M. J. Morgan (New York: Plenum), 287–356.
Mischak, H., Kubicek, C. P., and Röhr, M. (1984). Citrate inhibition of glucose uptake in Aspergillus niger. Biotechnol. Lett. 6, 425–430.
Papagianni, M. (2007). Advances in citric acid fermentation by Aspergillus niger: biochemical aspects, membrane transport and modeling. Biotechnol. Adv. 25, 244–263.
Papagianni, M., Mattey, M., and Kristiansen, B. (1999). The influence of glucose concentration on citric acid production and morphology of Aspergillus niger in batch and culture. Enzyme Microb. Technol. 25, 710–717.
Pitt, D. E., and Bull, A. T. (1982). The adenine nucleotide composition of growing and stressed cultures of Trichoderma aureoviride. Exp. Mycol. 6, 41–51.
Rhine, E. D., Sims, G. K., Mulvaney, R. L., and Pratt, E. J. (1998). Improving the Berthelot reaction for determining ammonium in soil extracts and water. Soil Sci. Soc. Am. J. 62, 473–480.
Rivetta, A., Kuroda, T., and Slayman, C. (2011). Anion currents in yeast K+ transporters (TRK) characterize a structural homologue of ligand-gated ion channels. Pflügers Arch. Eur. J. Physiol. 462, 315–330.
Roos, W., and Luckner, M. (1984). Relationships between proton extrusion and fluxes of ammonium ions and organic acids in Penicillium cyclopium. J. Gen. Microbiol. 130, 1007–1014.
Roos, W., and Slavik, J. (1987). Intracellular pH topography of Penicillium cyclopium protoplasts. Maintenance of pH by both passive and active mechanisms. Biochim. Biophys. Acta 899, 67–75.
Ruijter, G. J. G., van der Vondervoort, P. J. I., and Visser, J. (1999). Oxalic acid production by Aspergillus niger: an oxalate-non-producing mutant produces citric acid at pH 5 and in the presence of manganese. Microbiology 145, 2569–2576.
Saayman, M., van Vuuren, H. J. J., van Zyl, W. H., and Viljoen-Bloom, M. (2000). Differential uptake of fumarate by Candida utilis and Schizosaccharomyces pombe. Appl. Microbiol. Biotechnol. 54, 792–798.
Sanders, D. (1988). “Fungi,” in Solute Transport in Plant Cells and Tissues, eds D. A. Baker, and J. L. Hall (Harlow: Longman Scientific and Technical, Longman House), 106–165.
Sauer, M., Porro, D., Mattanovich, D., and Branduardi, P. (2008). Microbial production of organic acids: expanding the markets. Trends Biotechnol. 26, 100–108.
Schinner, F., and Burgstaller, W. (1989). Extraction of zinc from industrial waste by a Penicillium sp. Appl. Environ. Microbiol. 55, 1153–1156.
Sigler, K., and Höfer, M. (1991). Mechanisms of acid extrusion in yeasts. Biochim. Biophys. Acta 1071, 375–391.
Slayman, C. L., Kaminski, P., and Stetson, D. (1990). “Structure and function of fungal plasma-membrane ATPases” in Biochemistry of Cell Walls and Membranes in Fungi, eds P. J. Kuhn, A. P. J. Trinci, M. J. Jung, M. W. Goosey, and L. G. Copping (Berlin: Springer Verlag), 299–316.
Sousa, M. J., Mota, M., and Leão, C. (1992). Transport of malic acid in the yeast Schizosaccharomyces pombe: evidence for a protondicarboxylate symport. Yeast 8, 1025–1031.
Tempest, D. W., and Neijssel, O. M. (1992). Physiological and energetic aspects of bacterial metabolite overproduction. FEMS Microbiol. Lett. 100, 169–176.
Torres, N. V., Riol-Cimas, J. M., Wolschek, M., and Kubicek, C. P. (1996). Glucose transport by Aspergillus niger: the low-affinity carrier is only formed during growth on high glucose concentrations. Appl. Microbiol. Biotechnol. 44, 790–794.
Tuthill, D. E., Frisvad, J. C., and Christensen, M. (2001). Systematics of Penicillium simplicissimum based on sDNA sequences, morphology and secondary metabolites. Mycologia 93, 298–308.
Usami, S. (1978). Production of citric acid by submerged culture. Mem. School Sci. Eng. Waseda Univ. 42, 17–26.
Vrabl, P., Mutschlechner, W., and Burgstaller, W. (2008). Characteristics of glucose uptake by glucose- and NH4-limited grown Penicillium ochrochloron at low, medium and high glucose concentration. Fungal Genet. Biol. 45, 1380–1392.
Vrabl, P., Mutschlechner, W., and Burgstaller, W. (2009). Dynamics of energy charge and adenine nucleotides during uncoupling of catabolism and anabolism in Penicillium ochrochloron. Mycol. Res. 113, 1422–1432.
Keywords: ambient pH, organic acids, chemostat, bioreactor batch culture, Neurospora crassa, overflow metabolism, charge balance, aggressive acidification
Citation: Vrabl P, Fuchs V, Pichler B, Schinagl CW and Burgstaller W (2012) Organic acid excretion in Penicillium ochrochloron increases with ambient pH. Front. Microbio. 3:121. doi: 10.3389/fmicb.2012.00121
Received: 07 October 2011; Accepted: 13 March 2012;
Published online: 04 April 2012.
Edited by:
Helio K. Takahashi, Universidade Federal de Sao Paulo, BrazilReviewed by:
Jose M. Diaz-Minguez, University of Salamanca, SpainMarcelo Tolmasky, California State University Fullerton, USA
Copyright: © 2012 Vrabl, Fuchs, Pichler, Schinagl and Burgstaller. This is an open-access article distributed under the terms of the Creative Commons Attribution Non Commercial License, which permits non-commercial use, distribution, and reproduction in other forums, provided the original authors and source are credited.
*Correspondence: Pamela Vrabl, Institute of Microbiology, University of Innsbruck, Technikerstrasse 25, 6020 Innsbruck, Austria. e-mail:cGFtZWxhLnZyYWJsQHVpYmsuYWMuYXQ=