- 1 Department of Surgery, Harvard Medical School and Massachusetts General Hospital, Boston, MA, USA
- 2 Shriners Burns Institute and Massachusetts General Hospital, Boston, MA, USA
- 3 IYAR, The Israeli Institute for Advanced Research, Rehovot, Israel
The ability of honey to kill bacterial pathogens in vitro and quickly clear even chronic or drug-resistant infections has been demonstrated by several studies. Most current research is focused on identifying the bactericidal compounds in honey, but the action of the compounds discovered is not sufficient to explain honey’s activity. By diluting honey to sub-inhibitory levels, we were able to study its impact on bacterial coordinated behavior, and discovered that honey inhibits bacterial quorum sensing (QS). Experiments to characterize and quantify honey’s effect on the QS networks of Pseudomonas aeruginosa revealed that low concentrations of honey inhibited the expression of MvfR, las, and rhl regulons, including the associated virulence factors. This research also establishes that inhibition of QS is associated with honey’s sugar content. Therefore, honey combats infections by two independent mechanisms acting in tandem: bactericidal components, which actively kill cells, and disruption of QS, which weakens bacterial coordination and virulence.
Introduction
A critical problem facing modern medicine is the emergence of many strains of antibiotic-resistant bacteria (Tomasz, 1994; Arias and Murray, 2009; So et al., 2010). The most dangerous of these are multi-drug-resistant “superbugs,” strains impervious to almost all known antibiotics. One example is the human opportunistic pathogen Pseudomonas aeruginosa, a Gram-negative bacterium notorious for infecting immunocompromised individuals, the cause of many nosocomial acquired infections including those caused in burn and cystic fibrosis patients. In recent years, strains of P. aeruginosa have appeared that resist virtually all known drugs (Aloush et al., 2005), a situation exacerbated by the fact that there are almost no new antibiotics against Gram-negative bacteria in the pipeline (Cornelis, 2008; Freire-Moran et al., 2011).
Honey, known for its medicinal uses since ancient times (Zumla and Luat, 1989), has attracted new attention in the fight against drug-resistant bacteria. It was found to be very effective against various clinical isolates of bacteria, and to boost the effect of current antibiotics when applied to antibiotic disks (Abd-El Aal et al., 2007; Kwakman et al., 2008). Recent research has shown that when tested against clinical isolates of Staphylococcus aureus and P. aeruginosa, honey killed free-living cells in all strains tested (Alandejani et al., 2009). More importantly it was capable of killing bacteria even in their highly resistant biofilm state, proving more effective than any single commonly used antibiotic (rifampin, cefazolin, oxacillin, vancomycin, azithromycin, fusidic acid, gentamicin, and linezolid were tested). In addition to attacking various pathogens in vitro, clinical studies have shown that honey enhances healing and eliminates even chronic or drug-resistant infections in vivo (Efem, 1988). Honey was also reported to be more effective than standard treatments for patients with infected burns (Wijesinghe et al., 2009).
Honey’s antimicrobial properties are still not fully understood. Bees produce honey from flower nectar by evaporating water and adding digestive enzymes (Crane, 1975). The two largest constituents of honey are sugars (81%) and water (17%; White et al., 1962; Jeffrey and Echazarretta, 1996). The remaining 1–2% contains various enzymes and compounds, whose composition plays a significant role in honey’s bactericidal activity and varies widely depending on nectar source (Molan, 1999). Attempts to identify the source of bactericidal activity has led to the discovery of molecules such as methylglyoxal and bee-defensin 1 (Kwakman et al., 2010), but accurately characterizing their effects is difficult due to the large number of trace components and the possibility of combinatorial effects.
Quorum sensing (QS) is a term describing bacterial communication used by many bacterial species and its based on the production and detection of diffusible signal molecules (Atkinson and Williams, 2009). These molecules trigger signaling cascades, resulting in collective changes in behavior. Inhibition of QS would disrupt defensive measures and regulation of virulence, both weakening an infection and making it much more vulnerable to bactericidal elements. Moreover, as QS is not essential to survival, a strategy to inhibit it would reduce virulence while minimizing selection for resistance.
Pseudomonas aeruginosa uses two known QS systems: (1) the acyl-homoserine-lactone (AHL) LasR/RhlR network (Fuqua et al., 2001; Shiner et al., 2005) and (2) the 4-hydroxy-2-alkylquinolines (HAQs) MvfR regulatory network (Gallagher et al., 2002; Déziel et al., 2004; Wade et al., 2005). MvfR is critical for full virulence and leads to the positive regulation of a wide variety of virulence factors, many of which are also influenced by RhlR and LasR. The bacterium uses these networks to modulate its virulence and respond to environmental cues (Bassler, 1999; Déziel et al., 2005; Hazan et al., 2010).
Here we show that non-bactericidal concentrations of honey (6% or less) inhibit both known QS networks used by P. aeruginosa by inhibiting the expression of genes in the MvfR, Las, and Rhl networks and activation of associated virulence factors. Combined with tests of bactericidal effects, this suggests that honey’s ability to combat infections stems from two separate mechanisms: (1) bactericidal effect from unique molecules (which have not been conclusively identified) that are nectar source dependent, and (2) effects on QS systems that are associated with sugar content and independent of nectar source.
Materials and Methods
Honey
Two honeys of different bactericidal strength were used in these experiments. Local honey (LH) was harvested from a hive of Italian honeybees (Apis mellifera ligustica), as a representative of an average honey. Manuka honey (MH), made from the nectar of Leptospermum scoparium or L. polygalifolium, was purchased from Manuka Health New Zealand (grade MGO 550 or 550 mg/kg methylglyoxal, batch NO. 030308). MH is known for its unusually strong bactericidal properties (Molan, 1999).
The honeys were either used raw or heat-treated for 20 min at 100°C to inactivate any enzymes present (Ahern and Klibanov, 1985), almost entirely eliminating bactericidal activity.
In experiments requiring dilutions the concentrations typically used were 2, 4, or 6% in 5 mL of Luria–Bertani (LB) broth.
Bacteria
Pseudomonas aeruginosa strains used were wild-type PA14 (Rahme et al., 1995) and derivative isogenic strains, including isogenic mutant pqsA (Déziel et al., 2004) from the Rahme lab stock, Mass-General-Hospital, Boston, MA, USA. Two types of reporter genes were used: (1) a pqsA–GFP (ASV) fusion consisting of a pqsA promoter upstream to a short live GFP (ASV) to allow detection of changes in pqs operon expression (Yang et al., 2007) and (2) fusions of the lacZ promoter to lasI and rhlI (Cao et al., 2001; Déziel et al., 2004, 2005). E. coli was the laboratory strain DH5α (NEB). All bacteria were grown in LB broth at 37°C.
Media
LB broth was purchased from BD Diagnostics. M9 minimal media was prepared by adding 200 mL M9 salts (64 g Na2HPO4·7H2O, 15 g KH2PO4, 2.5 g NaCl, 5 g NH4Cl in a total volume of 1000 mL distilled water), 2 mL of 1 M sterile MgSO4, 20 mL of 20% glucose, and 100 μL of 1 M sterile CaCl2 to 700 mL of distilled water and adjusting the volume to 1000 mL.
Bacterial Zone of Inhibition
The zone of inhibition on bacterial lawns was measured to compare the bactericidal properties of different honeys. LB agar plates were inoculated with bacterial lawns by plating out 100 μL of overnight liquid culture. Single drops of honey (10 μL) were pipetted onto these lawns. The plates were incubated 24 h at 37°C and the resulting clear areas around the drops were compared. Gentamicin (60 μg/mL; Sigma) and double distilled water were used as positive and negative controls respectively.
Minimum Inhibitory Concentration and Minimum Bactericidal Concentration Determination and Bacterial Growth
The minimum inhibitory concentration (MIC) of honey was determined by growing bacteria in a 96-well plate. Each row of wells contained a different type of honey or sugar, with initial concentrations of 60% honey or sugar and serial twofold dilutions down the rows. After incubating overnight at 37°C with no shaking, the lowest concentration at which the broth remained clear was defined as the MIC. Media from the wells with no visible bacterial growth were plated onto LB plates to check for surviving bacterial cells, and the lowest concentration that did not result in bacterial colonies was defined as the minimum bactericidal concentration (MBC). These concentrations were then compared to determine relative efficacy of the honey and sugar solutions tested. The growth of bacteria in presence of different types of honey or sugar was assessed after 18 h by measuring the optical density (OD600 nm) using a spectrophotometer (Genesys) at 600 nm. Bacterial growth was calculated and reported as percentage of treated/untreated cultures.
Pyocyanin Assay
Cultures were grown in 5 mL of media overnight in tubes on a roller at 37°C. The following day samples were taken and measured for OD600 nm. For pyocyanin extraction, bacterial cells were removed from 1 mL of culture by centrifuging followed by addition of 1 mL chloroform to the supernatant. Pyocyanin was extracted by the addition of HCl (0.5%) and the upper reddish phase was collected and its OD was measured at 520 nm (Hazan et al., 2010). Both OD’s were plotted as percentages of the control to account for differences in cell density.
pqsA–GFP Assay
A P. aeruginosa strain with a pqsA–GFP (ASV) fusion was grown in 96-well black microtiter plates as previously described (Hazan et al., 2010). Honey and sugar solutions were added to the media in the wells to determine their impact on expression. The growth and fluorescence of the GFP (excitation at 485 nm and emission at 535 nm) were recorded using plate reader Infinite F200 (Tecan).
Liquid Chromatography/Mass Spectrometry of QS Signaling Molecules
The quantification of HAQ concentration in bacterial culture supernatants was performed by liquid chromatography/mass spectrometry (LC–MS) as described previously (Lépine et al., 2003). The HAQs were separated on a C18 reverse-phase column connected to a mass spectrometer using a water/acetonitrile gradient. Positive electrospray in the MRM mode with 2 × 10−3 mTorr argon and 30 V as the collision gas were employed to quantify HAQs using the ion transitions HHQ 244 > 159, HHQ-D4 248 > 163, HQNO 260 > 159, PQS 260 > 175, and PQS-D4 264 > 179. The pseudomolecular ions of each compound were monitored in full scan mode using the unsaturated PA14 HAQs response factors.
β-Galactosidase Activity Assay
PA14 or isogenic mutant cultures carrying pGX5 (pqsA–lacZ) were grown overnight at 37°C, diluted to OD600 = 0.01, and grown at 37°C; and β-galactosidase activity was measured as described (Miller, 1972) at different time points. Briefly, 0.9 mL of Z buffer was added to 0.1 mL of a liquid culture. A drop of SDS (0.1%) and two drops of Chloroform were added followed by 15′′ vortex and mixed with 0.2 mL of ONPG (o-nitrophenyl-β-D-galactoside; 4 mg/mL). Tubes were then vortexed, incubated until sufficient yellow color had developed and measured for optical density at 420 and 550 nm. Results were expressed in Miller units (MU).
Protease Assay
Production of extracellular protease was tested using plates made with brain heart infusion (BHI) and skim milk (Sokol et al., 1979). To test the effects of honey, 4% MH was mixed in before pouring. 2 μL of PA14 culture at OD600 nm = 2.0. Plates were then incubated room temperature (about 24°C) for 48 h and resulting clear halos were measured.
Results
Bactericidal Effect of Honey
Honeys have been shown to have strong bactericidal properties against many species of bacteria that commonly infect wounds (Willix et al., 2008). In order to determine whether inhibition of QS is linked to this, we confirmed previous information on the nature of honey’s activity.
Firstly, it has been shown that the bactericidal effects of honey far exceed those of comparable sugar solutions (Efem and Iwara, 1992). Our tests of MIC/MBC of honey relative to sugars revealed that the minimum concentration of glucose or fructose required to prevent growth of P. aeruginosa cells in liquid culture is roughly fourfold that of honey, as shown in Table 1. This rules out osmotic stress as a deciding factor in antibacterial activity.
Certain honeys are known to be unusually effective against bacteria, a difference which is generally attributed to their nectar source (Molan, 1992). We tested honeys on bacterial lawns and confirmed that MH has a significantly larger zone of inhibition than LH (data not shown). It is also known that heating honey reduces or destroys its bactericidal activity (Molan, 1992), as shown in Figure 1A. The difference between raw and heat-treated MH is clearly visible – raw honey produces a clear zone of 17 mm diameter, while heat limits killing to a small area in direct contact with the drop of honey (9 mm diameter). The decrease in bactericidal activity of heated honey was visible with both PA14 and pqsA isogenic mutant (Figure 1A).
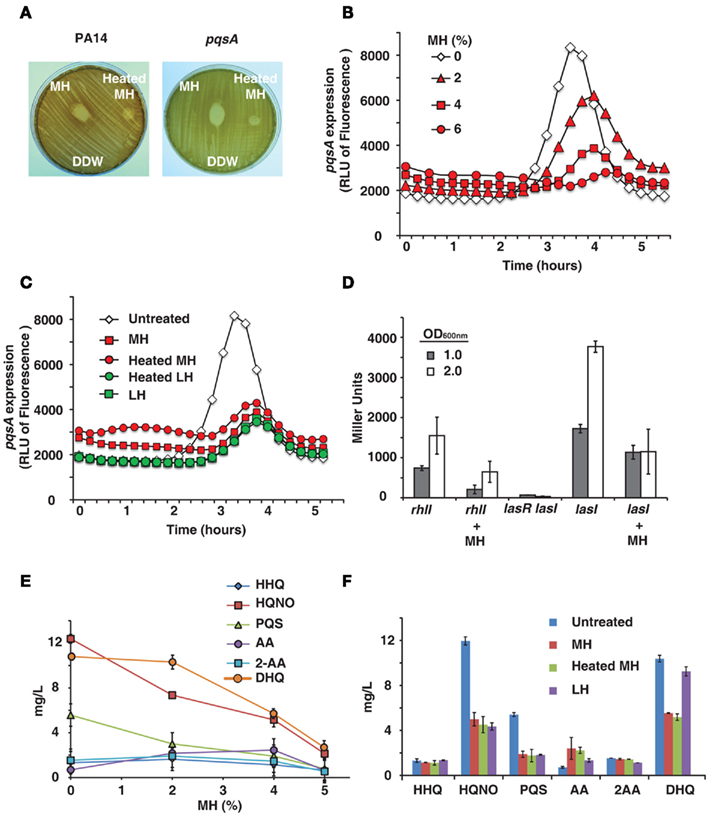
Figure 1. Honey inhibits expression of QS-related genes and molecules. (A) Shows manuka honey (MH) contains heat-sensitive bactericidal compounds. Raw MH, heat-treated MH and distilled water were dropped onto a P. aeruginosa lawn. Strains used were PA14 and a pqsA mutant. (B) Indicates honey contains a compound that reduces expression of pqsA. PA14 was grown in solutions of 2, 4, and 6% MH and pqsA expression was measured using a GFP (ASV) gene as a reporter. Fluorescence indicates pqsA expression, plotted here as a function of time. (C) Indicates inhibition of pqsA gene expression by honey is independent of honey source and unaffected by heat treatment. Experiment was performed as in (A), with all honeys diluted to 4%. Samples used were MH and local honey (LH), with and without heat treatment. (D) The expression of lasI and rhlI genes in the presence of honey was measured using a Miller β-galactosidase assay with PA14 cells expressing lasI or rhlI driven by the lacZ promoter and a lasR<lasI double mutant cells as a negative control. Measurements were taken at OD600 nm = 1.0 and 2.0 to determine gene expression at different cell densities. (E,F) Shows concentrations of MvfR low molecular weight QS-regulated molecules produced by the pqsABCD operon in the presence of honey. Samples used were MH and LH, with and without heat treatment.
Honey Inhibits QS Pathways and Production of QS-Regulated Small Molecules
Some of the most attractive non-lethal targets for an antimicrobial treatment are the QS networks, as inhibiting them would decrease bacterial virulence while possibly avoiding the selection pressure that conventional antibiotics exert (Hentzer and Givskov, 2003). Therefore, we tested whether honey directly affects the expression of QS genes. We initially examined the activity of MvfR regulon via pqsA, since its expression is under direct MvfR control (Déziel et al., 2005). To this end we used GFP (ASV) as a reporter of the pqsA gene expression (Hazan et al., 2010).
PqsA expression was found to be significantly reduced by MH or LH, while cell growth was unaffected by up to 6% honey. Figure 1B shows fluorescence over time – pqsA is expressed in the exponential stage of growth, and the graph shows a peak during this growth phase followed by a decline as the cells exit exponential growth. In addition to drastically reducing the height of this peak in the presence of honey, there was also a slight lag period likely caused by the bacteria adjusting to a new medium. A solution of 4% MH reduced pqsA gene expression by 50%, while not affecting final cell density.
The pqsA gene expression was also measured for different honeys at a fixed concentration of 4%, the highest concentration of honey that did not significantly impact final cell density. Figure 1C shows fluorescence data for MH and LH, with and without heat treatment. No significant difference was observed between the two varieties of honey or between raw and heat-treated honey. A concentration of 4% of any honey sample had a similar impact on pqsA expression.
Honey’s impact on the las and rhl gene expression was quantified by using the β-galactosidase reporter. Figure 1D shows a significant inhibition of both homoserine lactone synthase genes lasI and rhlI at two bacterial cell densities by 4% honey, especially prominent at OD600 nm = 2.0.
As the pqsA gene is required for the synthesis of 4-hydroxy-2-alkylquinolines (HAQs) and activation of the MvfR regulon, the levels of the MvfR-regulated molecules, 4-hydroxy-2-heptylquinoline (HHQ), and its hydroxylated derivative 3,4-dihydroxy-2-heptylquinoline, also known as the Pseudomonas quinolone signal (PQS), as well as other more abundant MvfR-regulated low molecular weight molecules, 4-hydroxy-2-heptylquinoline N-oxide (HQNO), 2,4-dihydroxyquinoline (DHQ), anthranilic acid (AA), and 2-aminoacetophenone (2-AA) were assessed by LC–MS from PA14 cells grown in the presence of honey. Figures 1E,F shows that increasing concentrations of honey led to an incremental reduction of the signal molecule levels, with a maximum reduction of approximately 50%. Most prominent reduction was observed with DHQ, HQNO, and PQS. Reduced levels of signal molecules and an accumulation of AA, a precursor molecule in the synthesis of HHQ, DHQ, HQNO, and 2-AA, are the most direct evidence of an impact on the MvfR regulon, as they are necessary for the feedback loop between MvfR and the genes it regulates. Reduction of DHQ, an abundant intermediate of HAQs further corroborates honey’s impact on MvfR regulon. Similarly, reduction of PQS and HQNO levels also suggests an impact on the LasR, system since HHQ is converted into PQS via the control of LasR, as is the last step of HQNO synthesis.
Observed inhibition of genes and signal molecules combined with impacts on downstream virulence factors in the pqs, las, and rhl operons shows a wide-reaching effect on QS networks.
Honey Impacts QS-Regulated Virulence Factors
Given honey’s impact on QS genes, our next step was to investigate its effect on virulence factors regulated by QS. In order to study this we examined virulence factors downstream to key QS genes in both the AHL and HAQ signal networks.
Pyocyanin is a toxic blue-green compound exclusive to P. aeruginosa, and is frequently measured as an index of the MvfR QS network that regulates it (Rahme et al., 1997; Déziel et al., 2005; Hazan et al., 2010). Figure 2A shows pyocyanin readings for P. aeruginosa grown in LB broth with 4% honey, and control with no honey. Pyocyanin production was reduced to 50% of the control by both MH and LH. Moreover, Figure 2A also shows that heat-treated honey equally inhibits pyocyanin production despite lacking bactericidal properties. Inhibition of pyocyanin was also observed in minimal medium (M9; data not shown).
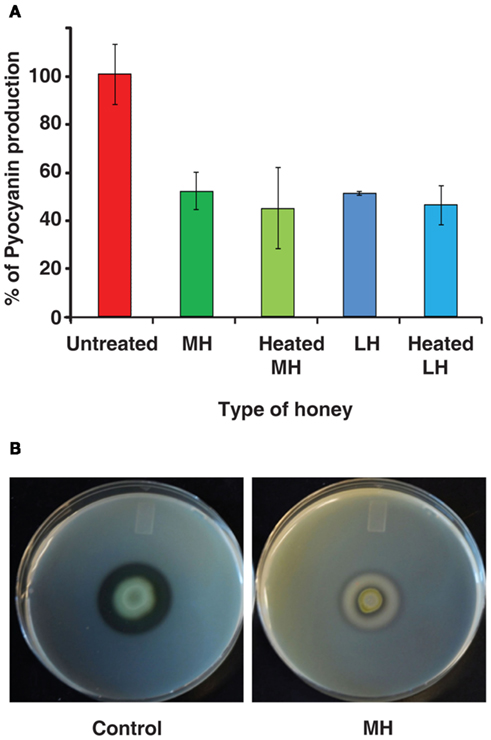
Figure 2. Honey impacts production of virulence factors. (A) Indicates honey decreases the production of pyocyanin, a virulence factor regulated by the MvfR QS network of P. aeruginosa. Pyocyanin is displayed as a percentage normalized to the control (no honey added). (B) demonstrates the effect on extracellular protease production by honey. Milk plates were inoculated with 2 μL of bacterial culture and incubated for 48 h at 24°C.
In addition, we found that honey also inhibits production of extracellular proteases, which are known to be under the control of MvfR and the las operon – part of the AHL network (Déziel et al., 2004). When P. aeruginosa was grown on skim milk plates, the addition of honey to the plates almost completely eliminated the clear halo around colonies caused by breakdown of casein. Control plates exhibited a 7-mm halo, while colonies on honey plates only created a 2-mm halo (Figure 2B).
Impact on two separate QS-regulated virulence factors suggests that honey affects the expression of the genes that regulate them. This would indicate an underlying interaction between honey and the QS networks of P. aeruginosa.
Quorum Sensing Inhibition by Honey is Associated with Sugar Content
Our results have shown that the components of honey responsible for QS inhibition are likely: (a) present in all types of honey regardless of nectar source, and (b) unaffected by heat treatment. The most obvious candidate is sugar, which is the largest component in all types of honey. To examine the role of honey’s sugar component, we substituted sugar solutions for honey in tests of QS-related behaviors.
A pqsA/GFP assay carried out with sugar solutions in LB broth recreated honey’s inhibitory effect almost exactly. As honey is roughly 80% sugar, the solutions used were compared to equivalent honey dilutions. Figure 3A shows that sugar solutions decreased pqsA gene expression and that glucose was the most potent of the ones tested. A concentration of only 1% glucose decreased pqsA gene expression by about 50% similarly to MH or LH (Figure 3A).
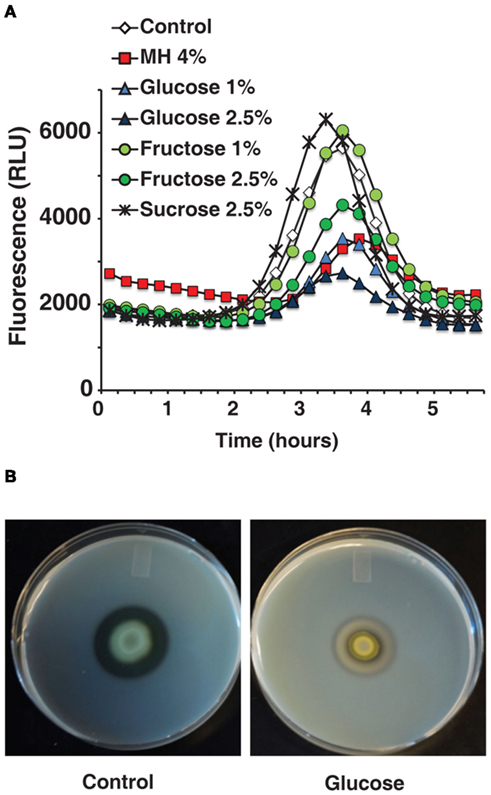
Figure 3. The effects of honey on QS-related genes and virulence factors can be reproduced by comparable sugar solutions. (A) Indicates that the inhibitory effect of diluted honey on pqsA can be reproduced using sugar solutions. (B) Shows the effect on extracellular proteases caused by 2.5% glucose in milk plates.
Figure 3B shows that 2.5% glucose added to a milk plate inhibited production of extracellular proteases in a similar manner to 4% MH, again almost eliminating the clear halo around PA14 cells. Additionally, in a pyocyanin assay in M9 minimal medium the addition of 1% glucose inhibited pyocyanin almost completely with no effect on growth (data not shown). The low concentration of glucose and the simple nature of the medium rule out osmotic stress or related effects as a possible cause.
The ability to replicate the impact caused by honey in multiple tests, both on gene expression and on QS-related virulence factors, supports the hypothesis that sugar is in active component in QS inhibition.
Discussion
This study characterizes honey’s interactions with the QS systems of P. aeruginosa, using sub-inhibitory concentrations (6% honey and below) to systematically examine the impact of honey on living bacterial cells. Our novel approach differs significantly from the use of high concentrations of honey to study bactericidal effects, and reveals another layer of information regarding honey’s unusual ability to fight infections.
Firstly, honey causes a significant reduction in expression of both P. aeruginosa QS pathways. Inhibition of pqsA reveals an impact on the synthesis of the HAQ signaling molecules, PQS and HHQ, which are required for the activity of MvfR and full expression of the MvfR-regulated virulence factors such as pyocyanin – a finding further reinforced by the accumulation of AA observed, as it is used as a precursor in the synthesis of these molecules (Figure 1E). lasI and rhlI, however, are part of the lasR and rhlR systems that make up the homoserine lactone network (Hazan et al., 2010). The rhlR system is linked to MvfR, in addition to regulating another array of virulence factors such as protease production, motility, and biofilm formation (Caiazza et al., 2007; Patriquin et al., 2008). However, the connection between the LasR and MvfR systems, in reference to honey, means that further research is needed to determine whether honey’s inhibition of the MvfR regulon is a direct impact or a secondary effect through the LasR system.
More directly, honey’s negative impact on virulence factors regulated by these networks serves to confirm our hypothesis. Reduced production of pyocyanin is consistent with the observed inhibition of the hydroxyquinoline-based MvfR system, which controls its production. Honey also inhibited expression of extracellular proteases, which are regulated by the las operon (Déziel et al., 2004). This operon is known to be regulated both by MvfR and by lasR QS systems. Honey’s impact on bacterial behaviors associated with QS combined with its inhibition of key genes in both known signaling networks strongly suggests that it has a wide-reaching effect on QS and associated virulence factors.
Comparisons between the nature of honey’s bactericidal components and those inhibiting QS show that there are two separate mechanisms at work. Previous studies show that bactericidal activity is likely caused by enzymes or proteins. Our results show that honey’s previously unknown ability to inhibit QS is at least partially caused by honey’s sugar content. Impacts on pqsA, pyocyanin, and extracellular proteases were all reproducible by comparable glucose or fructose solutions. Sugar content, mainly glucose and fructose, is constant regardless of nectar source because honeybees judge the ripeness of honey by sugar concentration. It is not destroyed by heat, which matches the characteristics of the component inhibiting QS.
The above results provide valuable insights on the medicinal use of honey. Consistent with previous studies, the nectar source is important to honey’s bactericidal properties, with MH generally acknowledged as one of the most active. In addition to being a potent treatment in its own right, honey’s ability to disrupt QS explains the synergistic effect seen when honey is combined with antibiotics, as interfering with cell-to-cell communication prevents bacteria from acting as a multicellular entity and makes them more susceptible to attack. QS-related behaviors are one of the most dangerous aspects of a bacterial infection – QS is key in activating multiple virulence factors, forming highly resilient biofilms and even encouraging the formation of antibiotic tolerant cells. Therefore, inhibiting QS provides a new and little-explored tactic against bacteria: the ability to weaken an infection and disrupt virulence, potentially without exerting selection pressure for resistance.
The lingering question is the exact mechanism of QS inhibition by honey’s sugar content. The concentrations of sugar used did not inhibit bacterial growth at all, indicating that the effect is not related to osmotic stress. As QS controls responses to environmental stimuli such as nutrition, one possible explanation is that the increased sugar causes a change in the metabolic pathways used by the bacteria in such a way that QS genes are not activated or are inhibited. It is possible that the presence of an easy food source in the form of sugar leads to less stress on the cells and a corresponding lack of aggression. This might also explain the difference in effect between the two sugars added – perhaps different genes are being activated to metabolize each. Additional experiments are needed to determine whether the effect is limited to simple sugars like glucose and fructose or whether other sugars or compounds could produce similar results. Future studies will be needed to address: (1) how sugar impacts QS-related behavior; and (2) whether there are other elements in honey that catalyze or enhance sugar’s effect.
In summary, honey’s ability to counter bacterial infections arises from two main factors: bactericidal compounds and QS inhibition. As honey is a highly complex substance there may be further contributing factors or interdependent components, necessitating further research, including possible metabolic changes that may occur as a result of sugars utilization. In the meantime it stands out as a promising rediscovered treatment whose strength lies in its ability to counter bacterial virulence on multiple levels. Honey itself may prove to be one new weapon in the battle against antibiotic resistance, but understanding and replicating its attack on bacteria by multiple mechanisms could be the key to an entirely new strategy to counter infections.
Conflict of Interest Statement
The authors declare that the research was conducted in the absence of any commercial or financial relationships that could be construed as a potential conflict of interest.
References
Abd-El Aal, A. M., El-Hadidy, M. R., El-Mashad, N. B., and El-Sebaie, A. H. (2007). Antimicrobial effect of bee honey in comparison to antibiotics on organisms isolated from infected burns. Ann. Burns Fire Disasters 20, 83–88.
Ahern, T. J., and Klibanov, A. M. (1985). The mechanisms of irreversible enzyme inactivation at 100C. Science 228, 1280–1284.
Alandejani, T., Marsan, J., Ferris, W., Slinger, R., and Chan, F. (2009). Effectiveness of honey on Staphylococcus aureus and Pseudomonas aeruginosa biofilms. Otolaryngol. Head Neck Surg. 141, 114–118.
Aloush, V., Navon-Venezia, S., Seigman-Igra, Y., Cabili, S., and Carmeli, Y. (2005). Multidrug-resistant Pseudomonas aeruginosa: risk factors and clinical impact. Antimicrob. Agents Chemother. 50, 43–48.
Arias, A. A., and Murray, B. E. (2009). Antibiotic-resistant bugs in the 21st century – a clinical super-challenge. N. Engl. J. Med. 360, 439–443.
Atkinson, S., and Williams, P. (2009). Quorum sensing and social networking in the microbial world. J. R. Soc. Interface 6, 959–978.
Bassler, B. L. (1999). How bacteria talk to each other: regulation of gene expression by quorum sensing. Curr. Opin. Microbiol. 2, 582–587.
Caiazza, H. C., Merritt, J. H., Brothers, K. M., and O’Toole, G. A. (2007). Inverse regulation of biofilm formation and swarming motility by Pseudomonas aeruginosa PA14. J. Bacteriol. 189, 3603–3612.
Cao, H., Krishnan, G., Goumnerov, B., Tsongalis, J., Tompkins, R., and Rahme, L. G. (2001). A quorum sensing-associated virulence gene of Pseudomonas aeruginosa encodes a LysR-like transcription regulator with a unique self-regulatory mechanism. Proc. Natl. Acad. Sci. U.S.A. 98, 14613.
Cornelis, P. (ed.). (2008). Pseudomonas: Genomics and Molecular Biology. Norfolk: Caister Academic Press.
Déziel, E., Gopalan, S., Tampakaki, A. P., Lépine, F., Padfield, K. E., Saucier, M., Xiao, G., and Rahme, L. G. (2005). The contribution of MvfR to Pseudomonas aeruginosa pathogenesis and quorum sensing circuitry regulation: multiple quorum sensing-regulated genes are modulated without affecting lasRI, rhlRI or the production of N-acyl-L-homoserine lactones. Mol. Microbiol. 55, 998–1014.
Déziel, E., Lépine, F., Milot, S., He, J., Mindrinos, M. N., Tompkins, R. G., and Rahme, L. G. (2004). Analysis of Pseudomonas aeruginosa 4-hydroxy-2-alkyquinolines (HAQs) reveals a role for 4-hydroxy-2-heptylquinoline in the cell- to-cell communication. Proc. Natl. Acad. Sci. U.S.A. 101, 1339–1344.
Efem, S. E. E. (1988). Clinical observations on the wound-healing properties of honey. Br. J. Surg. 75, 679–681.
Efem, S. E. E., and Iwara, C. I. (1992). The antimicrobial spectrum of honey and its clinical significance. Infection 20, 227–229.
Freire-Moran, L., Aronsson, B., Manz, C., Gyssens, I. C., So, A. D., Monnet, D. L., and Cars, O. (2011). Critical shortage of new antibiotics in development against multidrug resistant bacteria – time to react is now. Drug Resist. Updat. 14, 118–124.
Fuqua, C., Parsek, M. R., and Greenberg, E. P. (2001). Regulation of gene expression by cell-to-cell communication: acyl-homoserine lactone quorum sensing. Annu. Rev. Genet. 35, 439–468.
Gallagher, L. A., McKnight, S. L., Kuznetsova, M. S., Pesci, E. C., and Manoil, C. (2002). Functions required for extracellular quinolone signaling by Pseudomonas aeruginosa. J. Bacteriol. 184, 6472–6480.
Hazan, R., He, J., Xiao, G., Dekimpe, V., Apidianakis, Y., Lesic, B., Astrakas, C., Déziel, E., Lépine, F., and Rahme, L. G. (2010). Homeostatic interplay between bacterial cell–cell signaling and iron in virulence. PLoS Pathog. 6, e1000810. doi:10.1371/journal.ppat.1000810
Hentzer, M., and Givskov, M. (2003). Pharmacological inhibition of quorum sensing for the treatment of chronic bacterial infections. J. Clin. Invest. 112, 1300–1307.
Kwakman, P. H. S., te Velde, A. A., de Boer, L., Speijer, D., Vandenbroucke-Grauls, C., and Zaat, S. (2010). How honey kills bacteria. FASEB J. 24, 2576–2582.
Kwakman, P. H. S., Van den Akker, J. P. C., Gûçlû, A., Aslami, H., Binnekade, J. M., de Boer, L., Boszhard, L., Paulus, F., Middelhoek, P., te Velde, A. A., Vandebroucke-Grauls, C. M. J. E., Schultz, M. J., and Zaat, S. A. J. (2008). Medical-grade honey kills antibiotic-resistant bacteria in vitro and eradicates skin colonization. Clin. Infect. Dis. 46, 1677–1682.
Lépine, F., Déziel, E., Milot, S., and Rahme, L. G. (2003). A stable isotope dilution assay for the quantification of the Pseudomonas quinolone signal in Pseudomonas aeruginosa cultures. Biochim. Biophys. Acta 1622, 36–41.
Miller, J. H. (1972). “Assay of β-galactosidase” in Experiments in Molecular Genetics, ed. J. H. Miller (Cold Spring Harbor, NY: Cold Spring Harbor Laboratory), 352–355.
Molan, P. C. (1992). The antibacterial activity of honey: 2. Variation in the potency of antibacterial activity. Bee World 73, 59–76.
Patriquin, G. M., Banin, E., Gilmour, C., Tuchman, R., Greenberg, E. P., and Poole, K. (2008). Influence of quorum sensing and iron on twitching motility and biofilm formation in Pseudomonas aeruginosa. J. Bacteriol. 190, 662–671.
Rahme, L. G., Stevens, E. J., Wolfort, S. F., Shao, J., Tompkins, R. G., and Ausubel, F. M. (1995). Common virulence factors for bacterial pathogenicity in plants and animals. Science 268, 1899–1902.
Rahme, L. G., Tan, M.-W., Le, L., Wong, S. M., Tompkins, R. G., Calderwood, S. B., and Ausubel, F. M. (1997). Use of model plants hosts to identify Pseudomonas aeruginosa virulence factors. Proc. Natl. Acad. Sci. U.S.A. 94, 13245–13250.
Shiner, E. K., Rumbaugh, K. P., and Williams, S. C. (2005). Inter-kingdom signaling: deciphering the language of acyl homoserine lactones. FEMS Microbiol. Rev. 29; 935–947.
Sokol, P. A., Ohman, D. E., and Iglewski, H. B. (1979). A more sensitive plate assay for detection of protease production by Pseudomonas aeruginosa. J. Clin. Microbiol. 9, 538–540.
Tomasz, A. (1994). Multiple-antibiotic-resistant pathogenic bacteria – a report on the Rockefeller University Workshop. N. Engl. J. Med. 330, 1247–1251.
Wade, D. S., Calfee, M. W., Rocha, E. R., Ling, E. A., Engstrom, E., Coleman, J. P., and Pesci, E. C. (2005). Regulation of Pseudomonas quinolone signal synthesis in Pseudomonas aeruginosa. J. Bacteriol. 187, 4372–4380.
White, J. W., Reithof, M. L., Subers, M. H., and Kushnir, I. (1962). Composition of American Honey. US Dept. Agric. Tech. Bull. 1261, 1–124.
Wijesinghe, M., Weatherall, M., Perrin, K., and Beasley, R. (2009). Honey in the treatment of burns: a systematic review and meta-analysis of its efficacy. N. Z. Med. J. 122, 47–60.
Willix, D. J., Molan, P. C., and Haroot, C. G. (2008). A comparison of the sensitivity of wound-infecting species of bacteria to the antibacterial activity of manuka honey and other honey. J. Appl. Microbiol. 73, 388–394.
Yang, L., Barken, K. B., Skindersoe, M. E., Christensen, A. B., Givskov, M., and Tolker-Nielsen, T. (2007). Effects of iron on DNA release and biofilm development by Pseudomonas aeruginosa. Microbiology 153, 1318–1328.
Keywords: honey, Pseudomonas, quorum-sensing, infection, anti-infective, QS inhibition, mvfR(pqsR), sugars
Citation: Wang R, Starkey M, Hazan R and Rahme LG (2012) Honey’s ability to counter bacterial infections arises from both bactericidal compounds and QS inhibition. Front. Microbio. 3:144. doi: 10.3389/fmicb.2012.00144
Received: 19 January 2012; Paper pending published: 09 February 2012;
Accepted: 26 March 2012; Published online: 11 April 2012.
Edited by:
Sylvain S. Kamdem, Université de Yaoundé 1, CameroonReviewed by:
Sylvain S. Kamdem, Université de Yaoundé 1, CameroonAnushree Malik, Indian Institute of Technology Delhi, India
Copyright: © 2012 Wang, Starkey, Hazan and Rahme. This is an open-access article distributed under the terms of the Creative Commons Attribution Non Commercial License, which permits non-commercial use, distribution, and reproduction in other forums, provided the original authors and source are credited.
*Correspondence: Laurence G. Rahme, Molecular Surgical Laboratory, Department of Surgery, Harvard Medical School and Massachusetts General Hospital, 340 Thier Research Building, 50 Blossom Street, Boston, MA 02114, USA. e-mail:cmFobWVAbW9sYmlvLm1naC5oYXJ2YXJkLmVkdQ==
†Present address: Melissa Starkey, American College of Physicians, Philadelphia, PA, USA