- 1Department of Microbiology, Technische Universität München, Freising, Germany
- 2Research Center Juelich, Institute of Bioorganic Chemistry, Heinrich-Heine-Universität Düsseldorf, Juelich, Germany
- 3Instituto de Tecnologia Química e Biológica, Universidade Nova de Lisboa, Oeiras, Portugal
Functional metagenomic screening strategies, which are independent of known sequence information, can lead to the identification of truly novel genes and enzymes. Since E. coli has been used exhaustively for this purpose as a host, it is important to establish alternative expression hosts and to use them for functional metagenomic screening for new enzymes. In this study we show that Thermus thermophilus HB27 is an excellent screening host and can be used as an alternative provider of truly novel biocatalysts. In a previous study we constructed mutant strain BL03 with multiple markerless deletions in genes for major extra- and intracellular lipolytic activities. This esterase-diminished strain was no longer able to grow on defined minimal medium supplemented with tributyrin as the sole carbon source and could be used as a host to screen for metagenomic DNA fragments that could complement growth on tributyrin. Several thousand single fosmid clones from thermophilic metagenomic libraries from heated compost and hot spring water samples were subjected to a comparative screening for esterase activity in both T. thermophilus strain BL03 and E. coli EPI300. We scored a greater number of active esterase clones in the thermophilic bacterium than in the mesophilic E. coli. From several thousand functionally screened clones only two thermostable α/β-fold hydrolase enzymes with high amino acid sequence similarity to already characterized enzymes were identifiable in E. coli. In contrast, five further fosmids were found that conferred lipolytic activities in T. thermophilus only. Four open reading frames (ORFs) were found which did not share significant similarity to known esterase enzymes but contained the conserved GXSXG motif regularly found in lipolytic enzymes. Two of the genes were expressed in both hosts and the novel thermophilic esterases, which based on their primary structures could not be assigned to known esterase or lipase families, were purified and preliminarily characterized. Our work underscores the benefit of using additional screening hosts other than E. coli for the identification of novel biocatalysts with industrial relevance.
Introduction
As only a small fraction of all microorganisms from environmental samples can be isolated and studied by classic microbiological methods, culture-independent strategies, such as metagenomics, have been employed to overcome these restrictions (Torsvik et al., 1990; Amann et al., 1995; Handelsman et al., 1998). The cloning and analysis of environmental DNA has led to the identification of various new genes for valuable enzymes (for a recent review see Leis et al., 2013). Sequence-based metagenomics strategies have been successful in many cases, but sequences without similarity to known genes cannot be identified using this approach (Steele et al., 2009; Liebl, 2011; Liebl et al., 2014). Function-based strategies on the other hand rely on the direct detection of functional gene products via diverse screening setups. Until today, E. coli is the most commonly used bacterial host in functional metagenomics. Since only a fraction of the genes from random (meta)genomic gene libraries are expressed (Gabor et al., 2004; Sorek et al., 2007), the identification of truly novel genes from metagenomes is hampered when a single host is used (Jenney and Adams, 2008). Other hosts than E. coli (e.g., diverse Proteobacteria, Actinobacteria, and eukaryotic fungi) have been shown to be good alternatives and new broad-range expression vectors have been developed for use in functional metagenomics (Courtois et al., 2003; Martinez et al., 2004; Aakvik et al., 2009; Craig et al., 2010; Damon et al., 2011; Kakirde et al., 2011; Kellner et al., 2011; Parachin and Gorwa-Grauslund, 2011; Liebl et al., 2014).
In previous work we have shown that Thermus thermophilus HB27 can be used for screening of genomic libraries using the shuttle fosmid pCT3FK. These studies resulted in the identification of several genes that would not have been found by using the mesophilic E. coli host alone (Angelov et al., 2009; Leis et al., 2013).
In this study we present the generation of large-insert metagenomic libraries and their subsequent screening for lipolytic activities in E. coli and T. thermophilus HB27. For the screenings in T. thermophilus, a multiple clean deletion mutant was used (termed BL03) which lacks several characterized extracellular and putative esterase encoding genes (Leis et al., 2014). The knockout strain had a substantially lower esterase activity than the wild type and the deletions abolished its ability to grow on SH minimal medium supplemented with tributyrin. Therefore, we decided to use this esterase-deficient strain in a high-throughput screening and selection setup where complementation of growth on tributyrin minimal medium by cloned heterologous metagenomic genes served to select for new environmental genes encoding tributyrin-cleaving esterases.
Materials and Methods
Bacterial Strains and Growth Conditions
Transformation and propagation of recombinant plasmids were performed using Escherichia coli XL1-Blue, XL10 GOLD® ultracompetent cells (Stratagene, La Jolla, USA) and DH10B (Invitrogen, Carlsbad, USA). E. coli EPI300-T1® (Epicentre, Madison, USA) was used for the generation and screening of metagenomic libraries cloned in pCT3FK large-insert fosmids (Angelov et al., 2009) based on pCC1FOS) upon addition of fosmid autoinduction solution (Epicentre). E. coli strain BL21(DE3) was used for the expression of target proteins in pET21a(+) (Novagen, Merck KGaA, Darmstadt, Germany). Bacterial cultures were grown in lysogeny broth (LB) or on LB agar plates supplemented with ampicillin (100 μg/ml), kanamycin (20 μg/ml) and chloramphenicol (12.5 μg/ml) as necessary. For T. thermophilus HB27 (DSMZ 7039) and T. thermophilus BL03 (genotype ΔTT_P0042, ΔTT_C0340-1, ΔTT_C0904, ΔTT_C1787), culturing and transformations were done as described in Angelov et al. (2009), using TB complex medium (8 g/l trypticase peptone, 4 g/l yeast extract, and 3 g/l NaCl) (Ramírez-Arcos et al., 1998) and SH minimal medium (Leis et al., 2014) with high-carbonate mineral water Aqua Purania (TSI, Zeven, Germany) at pH 7.5. Cells were cultured at 70°C or at 60°C when using kanamycin (final concentration of 20 μg/ml) for the propagation of transformants. For the growth selection and complementation assay, bacterial suspensions from overnight-grown liquid cultures were washed three times in 50 mM phosphate buffer (pH 7.5) and adjusted to equal optical densities before spotting 5 or 10 μl on complex and minimal medium. For screening and selection purposes on solid medium, tributyrin with a final concentration of 1.0% (v/v) was emulsified with an Ultra-Turrax homogenizer (IKA, Staufen, Germany) immediately before autoclaving of the medium.
Generation of a Large-Insert Fosmid Library and Functional Screenings
Water, sediment and biofilms were sampled from hot springs in the town of Furnas Azores, Portugal (37°46′21.78″N 25°18′14.673″W). Compost heap samples were collected from a composting plant (Bioenergiezentrum GmbH, Göttingen, Germany). After separation of microorganisms from the samples' matrices (filtration and scraping off cells from organic matter), the metagenomic DNA was prepared using the PowerSoil, PowerMax Soil and UltraClean Fecal DNA Isolation Kit (MO BIO, Carlsbad, USA). The generation of a large-insert DNA library was done using the pCC1FOS-derived pCT3FK T. thermophilus/E. coli shuttle fosmid (Angelov et al., 2009) according to the instructions of the pCC1FOS Fosmid Library Production Kit (Epicentre, Madison, USA). Arrayed clones from E. coli were cultured in 96-well microtiter plate format. To overcome labor-intensive transfer of single fosmid clones from E. coli to T. thermophilus, a high-throughput screening strategy was chosen based on growth complementation (Leis et al., 2014). Therefore, each pool of 96 single fosmids from E. coli was transferred in the screening strain T. thermophilus BL03 by a single transformation reaction. The transformants of each reaction were grown on TB plates containing kanamycin as antibiotic. After 2 days of incubation at 60°C, colonies were suspended from the plates and washed three times using 50 mM phosphate buffer (pH 7.5). The washed cell suspensions (each comprising a pool of 96 T. thermophilus transformants obtained by transformation of strain BL03 with pooled fosmids from 96 E. coli clones) were spotted onto SH minimal medium containing tributyrin (1% v/v) and kanamycin. Candidate clone pools were able to grow on tributyrin due to heterologous growth complementation after 2–3 days of incubation at 60°C. In order to determine single clone(s) whose fosmid-derived inserts conferred tributyrase activity within each positive pool, T. thermophilus BL03 was transformed individually with each of the 96 E. coli fosmids in deep well plate format as described before (Angelov et al., 2009). Suspensions of the transformants were washed several times and spotted on minimal medium containing tributyrin. T. thermophilus clones with candidate fosmid inserts grew after several days of incubation at 60°C. The stability of each phenotype was assessed by repeated streaking and growth of the clones on SH with tributyrin.
Each candidate clone was analyzed for its ability to hydrolyze the substrate in comparison to the control containing only the empty fosmid DNA. The corresponding halo formation was calculated as the area of the clearing zone without the area of the corresponding colony size according to the following equation:
Sequencing and in silico Analysis of Sequence Data
Fosmids conferring significant halo formation were sequenced using Roche's GS-FLX series 454 pyrosequencer at the Göttingen Genomics laboratory (G2L) and LGC Genomics (Berlin, Germany). For phylogenetic analysis, bacterial and archaeal 16S rDNA sequences were amplified by PCR using degenerate primer pairs 616Valt, 100K, AC165, and AC1601, respectively (Supplementary Table S1). Phylogenetic analysis was done using ARB (Ludwig et al., 2004) and the Ribosomal Database Project II (RDP-II) server (Cole et al., 2009). Assembled contig sequences were analyzed in silico using SEED (Overbeek et al., 2005). BlastP (Altschul et al., 1990), conserved domain database (CDD, Marchler-Bauer et al., 2011), and the Pfam database (Punta et al., 2012) were used for similarity searches and annotation. The presence of predicted signal peptides was analyzed with the SignalP server (Petersen et al., 2011). Regular expression algorithm was used to unravel potential esterase candidate genes by GXSXG conserved motif searches. For the generation of multiple sequence alignments and similarity tree reconstruction, representative protein sequences from classified esterases/lipases (Chow et al., 2012) were used. Tree calculations based on multiple sequence alignments were performed with the T-REX webserver (Boc et al., 2012) and the resulting Newick-formatted data was visualized with MEGA Version 5.2 (Tamura et al., 2011).
Cloning, Expression, and Purification of Metagenomic Esterases
Candidate genes were PCR amplified with Phusion DNA polymerase (ThermoFisher Scientific, Waltham, USA) using primers listed in Supplementary Table S1 (oligonucleotides EstA2_for and EstA2_rev for cloning EstA2, EstB1_for and EstB1_rev for EstB1, respectively). The PCR products were cloned using a CloneJET vector (Fermentas) backbone. Insertion of the polyhistidine (6xHis) sequence was performed by the Change-IT Multiple Mutation Site Directed Mutagenesis Kit (Affymetrix, Santa Clara, USA) with 5′-phosphorylated oligonucleotides (EstA2_PHO_F and EstB1_PHO_F) according to the manufacturer's instructions. Metagenomic esterase-encoding genes (EstA2 and EstB1) were cloned in pET21a(+) (Novagen) using NdeI and NcoI, and the same strategy was used for cloning in the T. thermophilus vector pMK18 (de Grado et al., 1999) by isothermal DNA assembly using the Gibson Assembly Master Mix (New England Biolabs, Ipswich, USA) with vector primers pMK18_RBS_for and pMK18_RBS_rev. The insert was amplified with Est_rev and corresponding forward primers of the esterases. For expression, E. coli BL21(DE3) Star cultures (250 ml) were grown in Erlenmeyer flasks to mid-log phase (absorbance at 600 nm ranging from 0.6 to 0.7) and induced with isopropyl-β-D-thiogalactopyranoside (IPTG) at a final concentration of 1.0 mM. After 4 h at 37°C, the cultures were harvested by centrifugation and disrupted by sonication (Hielscher Ultrasonics GmbH, Teltow, Germany). After removal of the cell debris, the lysate was subjected to heat treatment (at least 60°C for 20 min). The supernatant containing the thermostable and soluble protein of interest was purified using Protino Ni-IDA 2000 protein purification system (Macherey-Nagel) according to the manufacturer's instructions. Protein separation and purity was determined with sodium dodecyl sulfate polyacrylamide gel electrophoresis (SDS-PAGE) based on Laemmli (1970).
Enzyme Assays
E. coli and T. thermophilus cells from 5 ml overnight grown cultures were harvested by centrifugation and washed twice in 50 mM Tris-HCl (pH 7.5). All steps were performed on ice. Cells were resuspended in half volume of Tris buffer and disrupted by sonication until the turbid suspensions became clear. Cell debris was removed by centrifugation (21,000 × g, 10 min at 4°C) and supernatants were used for protein quantification and measurement of intracellular lipolytic activities. The para-nitrophenyl (pNP) assays were performed according to Leis et al. (2014) by using 0.1 ml of crude lysates incubated in 50 mM Tris-HCl (pH 7.5) buffer with 1.25 mM pNP-caprylate, -laurate, and palmitate at 60°C. After stopping the reaction with 0.25 ml of 2 M sodium carbonate and incubation on ice for 15 min, the mixture was centrifuged at 21,000 × g for 5 min at 4°C. Esterase activity measurements for purified enzyme preparations were performed using 1.25 mM final concentrations of different para-nitrophenyl-substrates (pNP-propionate (C3), -butyrate (C4), -valerate (C5), -caproate (C6), -caprylate (C8), caprate (C10), -laurate (C12), -myristate (C14), and -palmitate (C16), all obtained from Sigma-Aldrich). The influence of the additives NaCl, KCl, CaCl2, MgCl2 (salts), and inhibitor EDTA (chelating agent) and phenylmethylsulfonylfluorid (PMSF), an agent known to inhibit serine/cysteine proteases as well as acetylcholinesterases, was tested at final concentrations of 1 mM. Other pNP-substrates were derived by esterification with 2-methyldecanoate (Reetz et al., 1997; Franken et al., 2010; Wu et al., 2013), Ibuprofen, Naproxen (Reetz et al., 2010; Sandström et al., 2012), 6-methyl-2-(ortho-tolyl)hept-5-enoate (Gaich and Mulzer, 2009), and indancarboxylic acid (Pietruszka et al., 2009) and the activity assays were performed at 60, 70, and 80°C under the conditions reported by López et al. (2014) and Chow et al. (2012).
One unit of specific enzymatic activity was defined as 1.0 μmol of para-nitrophenol per minute and mg protein released from the substrate. All measurements were performed at least in duplicates (n = 2).
Synthesis of Assay Substrates—Representative Procedure
Synthesis of 4-nitrophenyl 6-methyl-2-(ortho-tolyl)hept-5-enoate: 4-Nitrophenol (538 mg, 3.87 mmol), 4-dimethylaminopyridine (47.3 mg, 387 μmol), and dicyclohexylcarbodiimide (798 mg, 3.87 mmol) were added to a solution of 6-methyl-2-(ortho-tolyl)hept-5-enoic acid (900 mg, 3.87 mmol) in 25 mL of dichloromethane under nitrogen. The mixture was stirred at room temperature. After 24 h the precipitate was filtered off and the solvent was removed under reduced pressure. The residue was purified by flash chromatography (petroleum ether/ethyl acetate, 99/1) to give the product as a yellow oil (1.07 g, 3.03 mmol, 78%). 1H-NMR (600 MHz, CDCl3,): δH [ppm] = 1.55 (s, 3 H, 1-H), 1.71 (s, 3 H, 2-H), 1.88 [dddd, 2J(6a,6b) = 13.7 Hz, 3J(6a,5b) =7.2 Hz, 3J(6a,5a) = 7.2 Hz, 3J(6a,7) = 6.9 Hz, 1 H, 6-Ha], 2.07 [dd,3J(5,6) = 7.2 Hz, 3J(5,4) = 7.2 Hz, 2 H, 5-H], 2.26 [dddd, 3J(6b,7) = 6.7 Hz, 3J(6b,5a) = 7.2 Hz, 3J(6b,5b) = 7.2 Hz, 2J(6b,6a) = 13.6 Hz, 1 H, 6-Hb], 2.44 (s, 3 H, arom.-CH3), 4.12 [dd, 3J(7,6a) = 6.8 Hz, 3J(7,6b) = 6.7 Hz, 1 H, 7-H], 5.14 [t, 3J(4,5) = 7.2 Hz, 1 H, 4-H], 7.15- (mc, 2 H, arom.-CH), 7.18–7.25 (m, 3 H, arom.-CH), 7.35 (mc, 1 H, arom.-CH), 8.22 (mc, 2 H, arom.-CH). 13C-NMR (151 MHz, CDCl3): δC [ppm] = 17.7 (C-1), 19.8 (arom.-CCH3), 25.7 (C-5), 25.8 (C-2), 32.8 (C-6), 46.3 (C-7), 122.3 (arom.-CH), 122.3 (arom.-CH), 123.1 (C-4), 125.1 (arom.-CH), 125.1 (arom.-CH), 126.6 (arom.-CH), 127.5 (arom.-CH), 130.8 (arom.-CH), 130.8 (arom.-CH), 133.2 (C-3), 136.6 (arom.-Cipso), 136.6 (arom.-Cipso), 145.3 (COOpNP), 155.6 (arom.-Cipso), 171.9 (arom.-CNO2). HR-MS (ESI, cation): calculated [C21H23NO4+Na+]: m/z = 376.15193; found [C21H23NO4+Na+]: m/z = 376.15211.
Results
Generation of Fosmid Libraries and Phylogenetic Analysis of Hot Spring and Compost Samples
Environmental samples were obtained from strong acidic and neutral hot spring water sediments and biofilms (pH ranging between 2.0 and 7.0, temperature from 60 to 62°C) from the São Miguel island, Azores, Portugal. In addition, a naturally heated lumber waste compost heap (63.3°C after 4 days) sample was obtained from a compost facility in Göttingen (Germany) (Table 1). High molecular weight DNA from both samples could be obtained and enabled the generation of a large-insert metagenomic library in the pCT3FK shuttle fosmid. The library comprised around 6048 single fosmid clones for the Azores samples and 1920 clones for the compost sample with each clone carrying approximately 35–40 kbp metagenomic inserts (Table 1). Phylogenetic analysis of the metagenomic DNA inserts was done by sequencing of PCR products obtained with degenerate primers which amplify bacterial and archaeal 16S rDNA sequences (Supplementary Table S1). This analysis showed a very low archaeal diversity in the hot spring water, with only representatives of the genera Stygiolobus azoricus DSM 6296 (99% sequence identity) and Sulfolobus species (e.g., S. acidocaldarius DSM 639 with 95% sequence identity) found. In the compost sample a more diverse representation of bacteria was observed with Firmicutes being the most highly represented phylum in which the majority of sequences were assigned to the orders Bacillales and Clostridiales (Table 2).
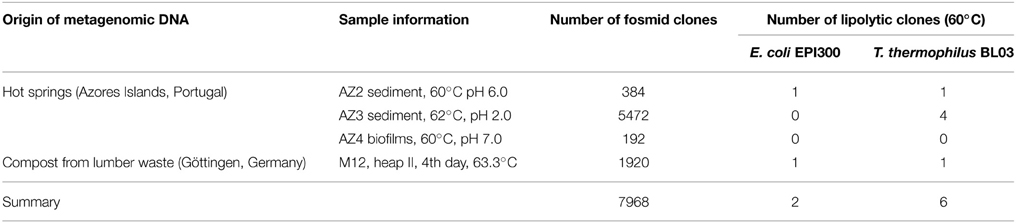
Table 1. Overview of the constructed metagenomic libraries and the esterase screening results in E. coli EPI300 and T. thermophilus BL03.
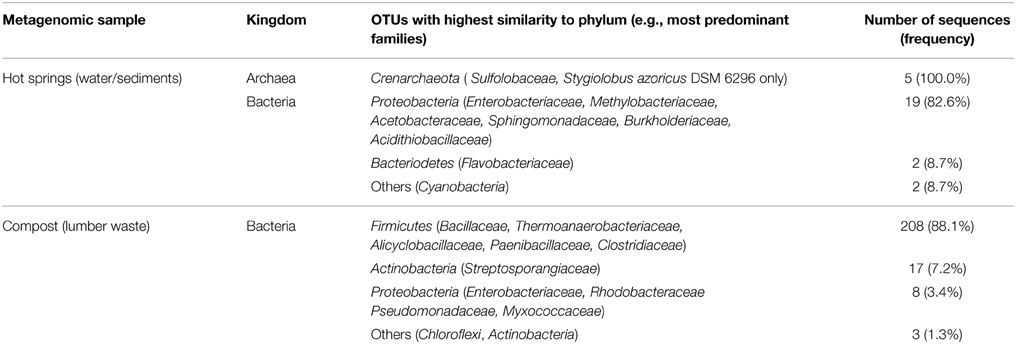
Table 2. Frequency of operational taxonomic units (OTUs) identified by 16S rDNA-analysis of the metagenomic samples.
Functional Screening of Fosmid Libraries in E. coli and T. thermophilus
After screening of the generated libraries in E. coli using a plate assay at 60°C, only two halo-forming colonies were identified on tributyrin agar plates, one from the Azores sample (termed AZ2-4-B6) and one from the compost sample (M12-4-D9). In order to perform the screening in T. thermophilus, the BL03 strain was transformed with 83 fosmid pools, each prepared from 96 fosmid clones. The transformant pools obtained in this way were examined for their ability to grow on minimal medium plates supplemented with tributyrin. A total of 13 pools showed substantial growth on these plates (namely fosmid pools AZ2-4; AZ3-14; AZ3-25; AZ3-30; AZ3-32; AZ3-33; AZ3-37; AZ3-38; AZ3-47; AZ4-2; M12-3; M12-4; and M12-6). From these candidate pools, single fosmid transformations were performed in 96 well plate format. Again, growth on minimal medium was monitored, and positive clones were selected. After re-streaking them two more times to confirm the stability of their phenotype, six fosmid-containing T. thermophilus clones could be isolated from five different fosmid pools. They stably grew on minimal medium agar supplemented with tributyrin, and hydrolysis halos on tributyrin substrate plates were monitored in comparison with the BL03 strain carrying an empty fosmid (Figure 1A). In addition, the esterase activity of crude cell extracts obtained from the respective strains was measured with various pNP-substrates (Figure 1B). Almost all fosmid clones showed preferences for medium to short acyl chain fatty acid pNP-esters. Crude extract from the strain AZ3-14-D2 exhibited the highest activities of all crude extracts tested. This extract was most active over a broad range of pNP-substrates tested and its long-chain palmitate fatty acid hydrolysis was approximately three-fold higher than in the BL03 empty fosmid control. The two fosmids that conferred tributyrase activity in E. coli, AZ2-4-B6 and M12-4-D9, were also found to confer activity in T. thermophilus when tested in pNP-assays. Fosmid M12-4-D9 could not be identified by heterologous growth complementation screenings in T. thermophilus BL03, but showed higher activity on pNP-C8 compared to E. coli. In general, the pNP-substrate preference of the fosmid-encoded esterases for short-chain acyl esters was in agreement with the observed capabilities of halo formation on tributyrin agar plates.
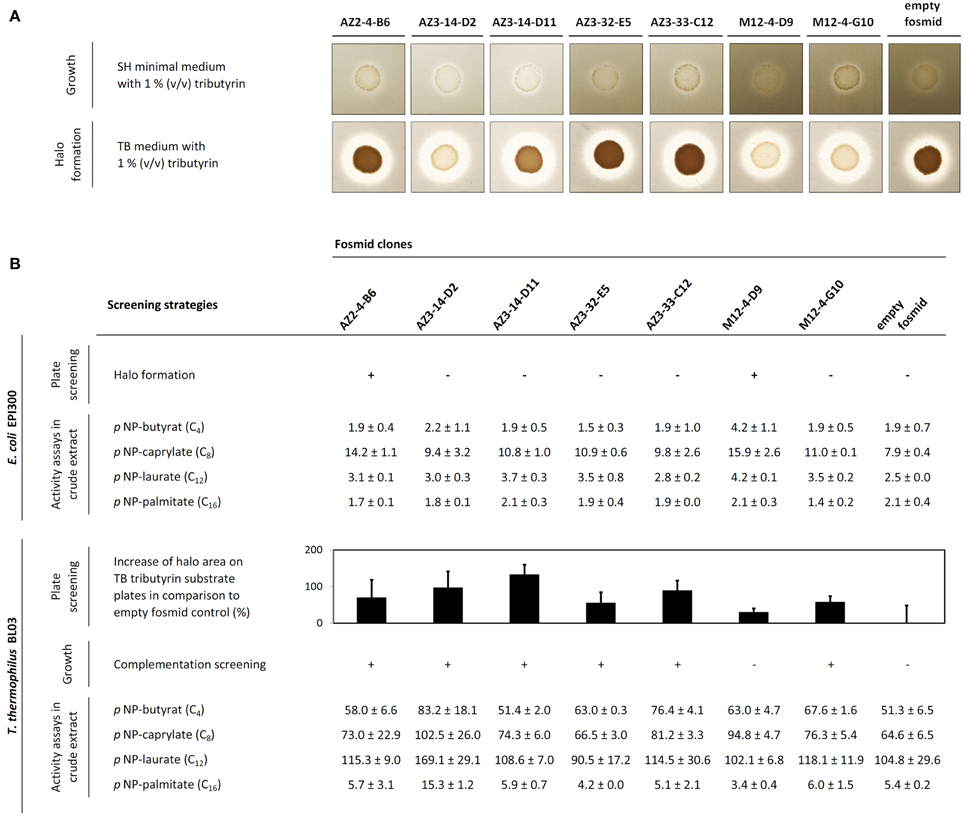
Figure 1. (A) Growth complementation screening results of T. thermophilus BL03 transformed with metagenomic fosmid DNA (upper scan picture) and corresponding tributyrase halo formation capabilities on substrate plates (lower scan picture). (B) Tributyrase activity of 8 single fosmids in E. coli EPI300 (upper part of the table) and T. thermophilus BL03 (below). In this representation, the increase of halo formation (black bars, halo area was calculated as described in the Materials and Methods section) on 1% (v/v) tributyrin substrate plates after 3 days of incubation at 60°C is shown (from triplicate measurements, n = 3; error bars indicate the standard deviation). In T. thermophilus, heterologous growth complementation results after 3 days of growth at 60°C on minimal medium are depicted. Comparative pNP-activity data from crude extracts (specific activity mU × mg−1) is shown for each single fosmid clone as average values from duplicate measurements (± standard deviation).
Sequencing and in silico Analysis of the Esterase Activity-Conferring Fosmid Inserts
The candidate fosmids were subjected to 454-sequencing and plasmids containing shotgun libraries of positive fosmids were sequenced with Sanger technology. The bioinformatic analysis of the fosmids is summarized in Table 3. Annotated α/β hydrolase 6 family proteins sharing high similarity to already characterized proteins were predicted from the sequence of two fosmids, namely M12-4-D9 and AZ2-4-B6. Not all positive fosmids from high-throughput T. thermophilus screenings could be completely assembled and sequenced (in average, 32.86 kb sequence information was obtained from each fosmid). The assembly data is summarized in Supplementary Table S2. Only in the case of two fosmids, AZ3-33-C12 (GenBank accession number KP892656) and M12-4-G10 (GenBank accession number KP892657) complete sequence assemblies where available for bioinformatic analysis. By motif search, the conserved GXSXG pentapeptide signature was identified in two of the predicted protein-encoding ORFs (EstA2 from fosmid AZ3-33-C12 and EstB1 from M12-4-G10). Both candidates showed no or only weak sequence similarity to lipolytic enzymes. Pfam prediction did not reveal any esterase/lipase-encoding function. For fosmid clones AZ3-32-E5, AZ3-14-D2, and AZ3-14-D11, sequence information was incomplete, therefore hampering further in silico analysis.
Heterologous Expression and Preliminary Characterization of Two Novel Metagenomic Esterases
From all candidate esterase-encoding ORFs, we selected EstA2 and EstB1 for expression, purification and characterization using T. thermophilus as an expression host. Expression of these ORFs in pMK18 (de Grado et al., 1999) led to significant pNP-butyrate-cleaving activity (measured at 60°C). Further investigation revealed that short-chain acyl esters were the preferred substrates, such as pNP-butyrate, that was hydrolyzed most optimally around 80–85°C and pH 8.0. EstA2 (encoded on fosmid AZ3-33-C12) resulted in a significant activity increase in the extracellular fraction, resulting in a 1.54-fold induction of activity, while EstB1 showed a 1.53-fold increased activity level compared to 338.4 ± 4.8 mU/mg protein in BL03 with the empty pMK18 vector. However, no recombinant protein band could be detected by SDS-PAGE analysis. We then attempted to produce C-terminally tagged versions of both proteins in E. coli by using the pET21a(+) expression vector. For both proteins, purification using heat treatment followed by IMAC gravity flow columns resulted in functional protein preparations (Figure 2).
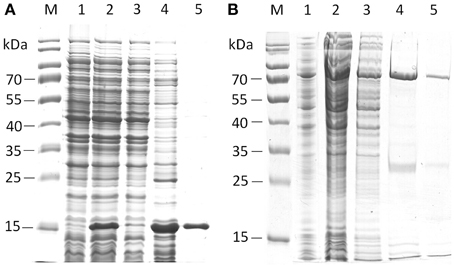
Figure 2. SDS-PAGE analysis of the purification steps of his-tagged EstA2 (A) and EstB1 (B). Lanes M, protein ladder; Lanes 1, empty vector control; lanes 2, crude lysate; lanes 3, flow-through; Lanes 4–5, eluate fractions of target proteins. Predicted sizes of the proteins with C-terminal his-tag fusions are 18.7 kDa for EstA2 and 64.6 kDa for EstB1, respectively.
The characterization of the recombinant enzymes revealed similar substrate preferences for short acyl chain substrates (pNP-C3 > C4 > C5 > C6 > C8) as the ones observed in T. thermophilus, while activities on long-chain substrates (over C10) were comparably low (Figure 3). Under the assay conditions used here, optimum activity of EstA2 was observed around 80°C and pH 8.0, while EstB1 was most active at 75°C and pH 8.0. The specific activities of both enzymes on para-nitrophenyl butyrate were 44.05 ± 2.06 U/mg protein for EstA2 and 4.13 ± 0.71 U/mg for EstB1. The influence of several additives, NaCl, KCl, CaCl2, MgCl2, EDTA, and PMSF at 1–10 mM final concentration was negligible. Our data suggests both proteins to be esterases rather than lipases due to their characteristic substrate specificities.
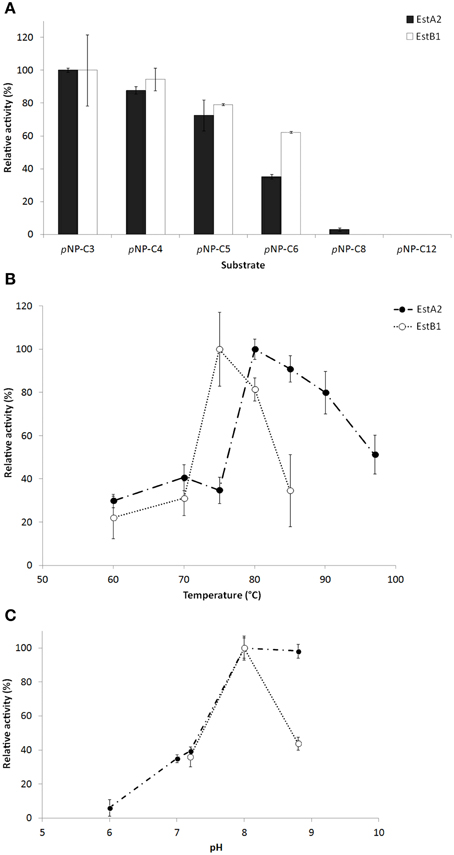
Figure 3. Characterization of the purified esterase-active proteins EstA2 and EstB1. (A) Substrate specificity of various acyl chain length pNP-esters. (B) Temperature and (C) pH optimum. The assays were performed in 50 mM phosphate buffer (pH 6–7) or 50 mM Tris-HCl buffer (pH 7.2–8.8) under optimal activity parameters (80°C for EstA2 and 75°C for EstB1, respectively) against 1.25 mM pNP-butyrate. 0.065–1.3 μg of purified enzyme was used for the assays. Data represents average values and standard deviations (error bars).
EstA2 and EstB1 were tested toward various racemic mixtures of a selection of pNP-esters (Table 4). Activity was measured over a wide range of temperatures (60–80°C) to overcome constraints by autohydrolysis of the substrates at elevated temperatures (Chow et al., 2012; López et al., 2014). In general, the activities measured were comparably low (mU range). The highest activities under the conditions tested were found with the 4-nitrophenolates of-Naproxen and Ibuprofen. No activity could be detected with the pNP-esters of indancarboxylic acid while 2-methyldecanoate and 6-methyl-2-(ortho-tolyl)hept-5-enoate were only hydrolyzed weakly in the presence of EstB1 at the highest temperature tested so far.
Classification of Lipolytic Enzymes
In order to classify the gene products identified in our metagenomic screenings, we performed multiple sequence alignments with known esterases and lipases from families I to VIII (Arpigny and Jaeger, 1999). Recently, the number of known lipolytic enzyme families has been extended by two new families, termed LipS and LipT (Chow et al., 2012). From the positive fosmids identified in E. coli (AZ2-4-B6 and M12-4-D9), both encoding α/β-hydrolase ORFs were identified as esterase class V and LipS family protein, respectively. The lipolytic enzymes discovered by using T. thermophilus for functional library screening, EstA2 and EstB1, could not be assigned to any known esterase/lipase family (Figure 4).
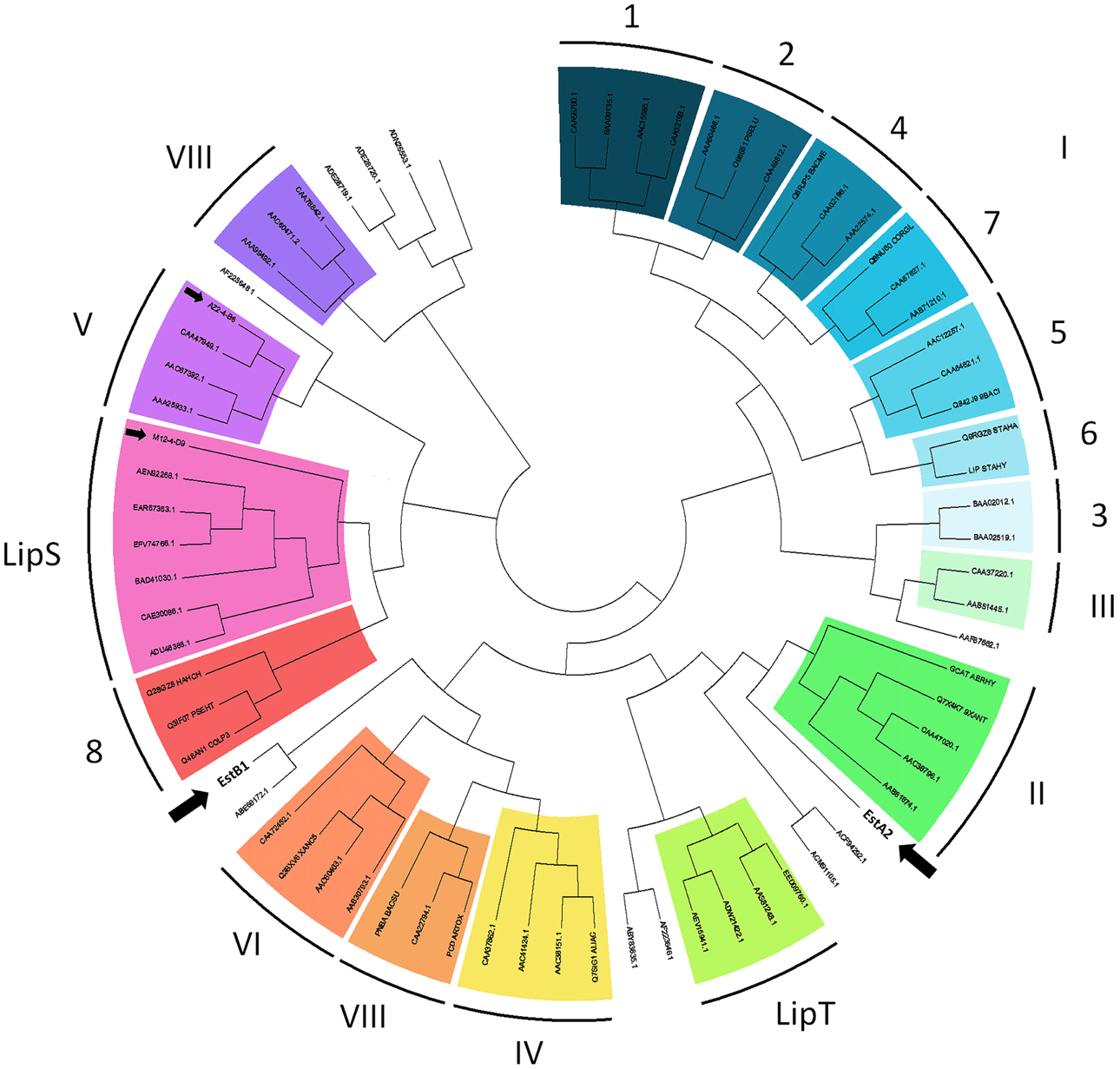
Figure 4. Topologic view of a similarity tree of all lipolytic esterase and lipase protein families I to VIII (according to Arpigny and Jaeger, 1999), including the recently defined families LipS and LipT as well as classified, but still unknown protein families (Chow et al., 2012) and the new esterases from our functional metagenomic screenings (AZ2-4-B6, M12-4-D9, EstA2, EstB1). The data was generated from multiple sequence alignments of selected representative protein sequences (according to Chow et al., 2012) using the ClustalW algorithm (Boc et al., 2012). Tree reconstruction and visualization was performed with MEGA Version 5.2 (Tamura et al., 2011). The metagenomic proteins identified in this study are marked with black arrows. The length of the tree branches does not represent the phylogenetic distance between the protein sequences.
Discussion
In the past, E. coli has been shown to be a good provider of interesting biocatalysts and enzymes from metagenomic screenings. Although it is clear that E. coli has restricted expression capabilities, it is the best developed and most commonly used host until today. Here, we show that using an additional host in functional screenings of metagenomic libraries can lead to the discovery of enzymes that would have remained hidden if only E. coli had been used.
How heterologous sequences are recognized and expressed into functional proteins by the transcriptional and translational machinery of an expression host is poorly understood. Factors influencing these processes, amongst other unknown factors, are: (i) toxicity of the foreign DNA and encoded proteins (Sorek et al., 2007), (ii) recognition of transcriptional signals (promoters) (Warren et al., 2008), (iii) ribosomal binding and translation initiation (Sørensen and Mortensen, 2005; Villegas and Kropinski, 2008), (iv) mRNA stability (Kudla et al., 2009), (v) codon usage (Sørensen and Mortensen, 2005), (vi) (post-translational) protein modification and secretion (Mergulhão et al., 2005). To overcome these restrictions, other screening hosts have been implemented in function-based metagenomic screening approaches in order to increase the detection frequency of active clones. First attempts using additional screening hosts involved Streptomyces and Pseudomonas species (Courtois et al., 2003; Martinez et al., 2004; Lussier et al., 2011). By the use of broad-host range vectors, the expression could recently be extended to other members of the Proteobacteria, i.e., the same taxon as E. coli (Aakvik et al., 2009; Craig et al., 2010; Kakirde et al., 2011), and to eukaryotic expression hosts (Damon et al., 2011; Kellner et al., 2011; Parachin and Gorwa-Grauslund, 2011). Our approach is distinguished from these “broad-host vectors” strategies by performing the library construction steps in the well-established E. coli system, followed by transfer of the metagenomic fosmids in the alternative host (Angelov et al., 2009). In order to facilitate the detection of esterase-encoding fosmid inserts, we used a selection-based strategy which relies on the complementation of growth of a custom, esterase-deficient strain of T. thermophilus (strain BL03, Leis et al., 2014). The BL03 strain is a multiple esterase deletion mutant which exhibits significantly reduced extracellular lipolytic activities compared with the parent strain HB27 and was shown to be severely impaired in growth on minimal medium supplemented with tributyrin (Leis et al., 2014). The growth could be regained via heterologous complementation when metagenomic DNA was expressed by the host. Low detection limits of classical phenotypic plate screenings (clearing zones around the colonies) could thus be overcome, as even weak gene expression levels yield enough metabolites to reconstitute growth. By implementing a high-throughput pooling strategy, approximately 8000 single fosmid clones yielded six single candidate fosmid clones, which represents a detection frequency that is comparable with screening results obtained from other metagenomics studies aimed at lipolytic activities from hot environments (Rhee et al., 2005; Chow et al., 2012). To our knowledge, this is the first report of identifying novel metagenome-borne esterases by the use of a screening organism other than traditional E. coli.
Metagenomic esterases having similarity to β-lactamase family proteins have been reported from various environmental or anthropogenic sources, e.g., leachate samples (Rashamuse et al., 2009), arctic soil (Yu et al., 2011), alkaline compost (Kim et al., 2010), drinking water and soil metagenomes (Elend et al., 2006). They share the conserved sequence motif common for class C β-lactamases (Ser-Xaa-Xaa-Lys, whereas Xaa is any amino acid) that is important for catalytic activity of esterases with similarity to penicillin-binding proteins (Petersen et al., 2001), and have been assigned to the less characterized family VIII carboxylesterases (Arpigny and Jaeger, 1999). EstB1 lacks this conserved sequence motif and does not belong to the class C β-lactamase protein family. Instead, it could be assigned to class B metallo-β-lactamase proteins (PF00753). This group comprises hydrolases acting on thioester (thioesterase) and sulfate ester (sulfatase) bonds. Furthermore, thioesterases have also been shown to convert para-nitrophenol esters (Shahi et al., 2006; Kotowska et al., 2009). EstB1 shared 30% sequence identity with SdsA1 from Pseudomonas aeruginosa, which is a characterized alkyl-sulfatase (Hagelueken et al., 2006). The other esterase-acting enzyme identified in our metagenomic studies was EstA2. It is most similar to hypothetical proteins from diverse archaeal origins. One characterized enzyme, to which EstA2 shows very weak similarity, is a phospholipase from the hyperthermophilic archaeon Aeropyrum pernix K1 encoded by ORF APE2325 (Wang et al., 2004). Also being a small protein with 18 kDa in size, its reported substrate spectrum and activity pattern is comparable to EstA2, as it also preferred short acyl chain pNP-substrates and was most active at elevated temperatures (above 80°C).
In this study we have demonstrated that T. thermophilus is an excellent host bacterium for the detection of novel metagenome-borne enzymes that could not readily have been detected by the use of E. coli or by in silico analysis. To our knowledge, this is the first report that metagenome-derived esterases could be identified in an expression host other than E. coli. The functional screening implementing T. thermophilus BL03 could uncover several lipolytic enzymes from underrepresented species and archaeal origin, and most strikingly, some of them could not be assigned to classical esterase and lipase enzyme families and therefore represent truly novel esterase-active proteins. The confirmation of predicted ORFs responsible for the lipolytic activity of the remaining fosmids will be addressed in the future. The use of complementation screens in T. thermophilus with shotgun subclones will help to identify more active genes that do not share any known sequence signatures at all.
Conflict of Interest Statement
The authors declare that the research was conducted in the absence of any commercial or financial relationships that could be construed as a potential conflict of interest.
Acknowledgments
This work was supported by the German Research Foundation (DFG) and the Technische Universität München within the funding programme Open Access Publishing. The authors gratefully acknowledge the support by the Faculty Graduate Center Weihenstephan of TUM Graduate School at Technische Universität München, Germany. This work was funded by the German Federal Ministry of Education and Research (BMBF) in the collaborative project ExpresSys within the funding measure GenoMik-Transfer. JP thanks T. Pham, C. Lorenz, M. Schölzel, R. C. Simon, D. Schumacher, and K. Morka for supporting experiments.
Supplementary Material
The Supplementary Material for this article can be found online at: http://www.frontiersin.org/journal/10.3389/fmicb.2015.00275/abstract
References
Aakvik, T., Degnes, K. F., Dahlsrud, R., Schmidt, F., Dam, R., Yu, L., et al. (2009). A plasmid RK2-based broad-host-range cloning vector useful for transfer of metagenomic libraries to a variety of bacterial species. FEMS Microbiol. Lett. 296, 149–158. doi: 10.1111/j.1574-6968.2009.01639.x
PubMed Abstract | Full Text | CrossRef Full Text | Google Scholar
Altschul, S. F., Gish, W., Miller, W., Myers, E. W., and Lipman, D. J. (1990). Basic local alignment search tool. J. Mol. Biol. 215, 403–410. doi: 10.1016/S0022-2836(05)80360-2
PubMed Abstract | Full Text | CrossRef Full Text | Google Scholar
Amann, R. I., Ludwig, W., and Schleifer, K. H. (1995). Phylogenetic identification and in situ detection of individual microbial cells without cultivation. Microbiol. Rev. 59, 143–169.
Angelov, A., Mientus, M., Liebl, S., and Liebl, W. (2009). A two-host fosmid system for functional screening of (meta)genomic libraries from extreme thermophiles. Syst. Appl. Microbiol. 32, 177–185. doi: 10.1016/j.syapm.2008.01.003
PubMed Abstract | Full Text | CrossRef Full Text | Google Scholar
Arpigny, J. L., and Jaeger, K. E. (1999). Bacterial lipolytic enzymes: classification and properties. Biochem. J. 343, 177–183. doi: 10.1042/0264-6021:3430177
PubMed Abstract | Full Text | CrossRef Full Text | Google Scholar
Boc, A., Diallo, A. B., and Makarenkov, V. (2012). T-REX: a web server for inferring, validating and visualizing phylogenetic trees and networks. Nucleic Acid Res. 40, W573–W579. doi: 10.1093/nar/gks485
PubMed Abstract | Full Text | CrossRef Full Text | Google Scholar
Chow, J., Kovacic, F., Dall Antonia, Y., Krauss, U., Fersini, F., Schmeisser, C., et al. (2012). The metagenome-derived enzymes LipS and LipT increase the diversity of known lipases. PLoS ONE 7:e47665. doi: 10.1371/journal.pone.0047665
PubMed Abstract | Full Text | CrossRef Full Text | Google Scholar
Cole, J. R., Wang, Q., Cardenas, E., Fish, J., Chai, B., Farris, R. J., et al. (2009). The Ribosomal Database Project: improved alignments and new tools for rRNA analysis. Nucleic Acid. Res. 37, D141–D145. doi: 10.1093/nar/gkn879
PubMed Abstract | Full Text | CrossRef Full Text | Google Scholar
Courtois, S., Cappellano, C. M., Ball, M., Francou, F.-X., Normand, P., Helynck, G., et al. (2003). Recombinant environmental libraries provide access to microbial diversity for drug discovery from natural products. Appl. Environ. Microbiol. 69, 49–55. doi: 10.1128/AEM.69.1.49-55.2003
PubMed Abstract | Full Text | CrossRef Full Text | Google Scholar
Craig, J. W., Chang, F.-Y., Kim, J. H., Obiajulu, S. C., and Brady, S. F. (2010). Expanding small-molecule functional metagenomics through parallel screening of broad-host-range cosmid environmental DNA libraries in diverse proteobacteria. Appl. Environ. Microbiol. 76, 1633–1641. doi: 10.1128/AEM.02169-09
PubMed Abstract | Full Text | CrossRef Full Text | Google Scholar
Damon, C., Vallon, L., Zimmermann, S., Haider, M. Z., Galeote, V., Dequin, S., et al. (2011). A novel fungal family of oligopeptide transporters identified by functional metatranscriptomics of soil eukaryotes. ISME J. 5, 1871–1880. doi: 10.1038/ismej.2011.67
PubMed Abstract | Full Text | CrossRef Full Text | Google Scholar
de Grado, M., Castán, P., and Berenguer, J. (1999). A high-transformation-efficiency cloning vector for Thermus thermophilus. Plasmid 42, 241–245. doi: 10.1006/plas.1999.1427
PubMed Abstract | Full Text | CrossRef Full Text | Google Scholar
Elend, C., Schmeisser, C., Leggewie, C., Babiak, P., Carballeira, J. D., Steele, H. L., et al. (2006). Isolation and biochemical characterization of two novel metagenome-derived esterases. Appl. Environ. Microbiol. 72, 3637–3645. doi: 10.1128/AEM.72.5.3637-3645.2006
PubMed Abstract | Full Text | CrossRef Full Text | Google Scholar
Franken, B., Jaeger, K.-E., and Pietruszka, J. (2010). “Protocols to screen for enantioselective lipases,” in Handbook of Hydrocarbon and Lipid Microbiology, ed K. N. Timmes (Berlin-Heidelberg: Springer), 4581–4586.
Gabor, E. M., Alkema, W. B. L., and Janssen, D. B. (2004). Quantifying the accessibility of the metagenome by random expression cloning techniques. Environ. Microbiol. 6, 879–886. doi: 10.1111/j.1462-2920.2004.00640.x
PubMed Abstract | Full Text | CrossRef Full Text | Google Scholar
Gaich, T., and Mulzer, J. (2009). Total synthesis of (-)-Penifulvin A, an insecticide with a dioxafenestrane skeleton. J. Am. Chem. Soc. 131, 452–453. doi: 10.1021/ja8083048
PubMed Abstract | Full Text | CrossRef Full Text | Google Scholar
Hagelueken, G., Adams, T. M., Wiehlmann, L., Widow, U., Kolmar, H., Tümmler, B., et al. (2006). The crystal structure of SdsA1, an alkylsulfatase from Pseudomonas aeruginosa, defines a third class of sulfatases. Proc. Natl. Acad. Sci. U.S.A. 103, 7631–7636. doi: 10.1073/pnas.0510501103
PubMed Abstract | Full Text | CrossRef Full Text | Google Scholar
Handelsman, J., Rondon, M. R., Brady, S. F., Clardy, J., and Goodman, R. M. (1998). Molecular biological access to the chemistry of unknown soil microbes: a new frontier for natural products. Chem. Biol. 5, R245–R249. doi: 10.1016/S1074-5521(98)90108-9
PubMed Abstract | Full Text | CrossRef Full Text | Google Scholar
Jenney, F. E., and Adams, M. W. W. (2008). The impact of extremophiles on structural genomics (and vice versa). Extremophiles 12, 39–50. doi: 10.1007/s00792-007-0087-9
PubMed Abstract | Full Text | CrossRef Full Text | Google Scholar
Kakirde, K. S., Wild, J., Godiska, R., Mead, D. A., Wiggins, A. G., Goodman, R. M., et al. (2011). Gram negative shuttle BAC vector for heterologous expression of metagenomic libraries. Gene 475, 57–62. doi: 10.1016/j.gene.2010.11.004
PubMed Abstract | Full Text | CrossRef Full Text | Google Scholar
Kellner, H., Luis, P., Portetelle, D., and Vandenbol, M. (2011). Screening of a soil metatranscriptomic library by functional complementation of Saccharomyces cerevisiae mutants. Microbiol. Res. 166, 360–368. doi: 10.1016/j.micres.2010.07.006
PubMed Abstract | Full Text | CrossRef Full Text | Google Scholar
Kim, Y. H., Kwon, E. J., Kim, S. K., Jeong, Y. S., Kim, J., Yun, H. D., et al. (2010). Molecular cloning and characterization of a novel family VIII alkaline esterase from a compost metagenomic library. Biochem. Biophys. Res. Comm. 393, 45–49. doi: 10.1016/j.bbrc.2010.01.070
PubMed Abstract | Full Text | CrossRef Full Text | Google Scholar
Kotowska, M., Pawlik, K., Smulczyk-Krawczyszyn, A., Bartosz-Bechowski, H., and Kuczek, K. (2009). Type II Thioesterase ScoT, Associated with Streptomyces coelicolor A3(2) modular polyketide synthase Cpk, hydrolyzes acyl residues and has a preference for propionate. Appl. Environ. Microbiol. 75, 887–896. doi: 10.1128/AEM.01371-08
PubMed Abstract | Full Text | CrossRef Full Text | Google Scholar
Kudla, G., Murray, A. W., Tollervey, D., and Plotkin, J. B. (2009). Coding-sequence determinants of gene expression in Escherichia coli. Science 324, 255–258. doi: 10.1126/science.1170160
PubMed Abstract | Full Text | CrossRef Full Text | Google Scholar
Laemmli, U. K. (1970). Cleavage of structural proteins during the assembly of the head of bacteriophage T4. Nature 227, 680–685. doi: 10.1038/227680a0
PubMed Abstract | Full Text | CrossRef Full Text | Google Scholar
Lane, D. J. (1991). “16S/23S rRNA sequencing,” in Nucleic Acid Techniques in Bacterial Systematics, eds E. Stackebrandt and M. Goodfellow (Chichester, NY: Wiley), 115–175.
Leis, B., Angelov, A., and Liebl, W. (2013). Screening and expression of genes from metagenomes. Adv. Appl. Microbiol. 83, 1–68. doi: 10.1016/B978-0-12-407678-5.00001-5
PubMed Abstract | Full Text | CrossRef Full Text | Google Scholar
Leis, B., Angelov, A., Li, H., and Liebl, W. (2014). Genetic analysis of lipolytic activities in Thermus thermophilus HB27. J. Biotechnol. 191, 150–157. doi: 10.1016/j.jbiotec.2014.07.448
PubMed Abstract | Full Text | CrossRef Full Text | Google Scholar
Li, A., Qu, Y., Zhou, J., and Ma, F. (2009). Enzyme-substrate interaction and characterization of a 2,3-dihydroxybiphenyl 1,2-dioxygenase from Dyella ginsengisoli LA-4. FEMS Microbiol. Lett. 292, 231–239. doi: 10.1111/j.1574-6968.2009.01487.x
PubMed Abstract | Full Text | CrossRef Full Text | Google Scholar
Liebl, W. (2011). “Metagenomics,” in Encyclopedia of Geobiology, Encyclopedia of Earth Sciences Series, eds J. Reitner and V. Thiel (Dordrecht, NL: Springer), 553–558.
Liebl, W., Angelov, A., Juergensen, J., Chow, J., Loeschcke, A., Drepper, T., et al. (2014). Alternative hosts for functional (meta)genome analysis. Appl. Microbiol. Biotechnol. 98, 8099–8109. doi: 10.1007/s00253-014-5961-7
PubMed Abstract | Full Text | CrossRef Full Text | Google Scholar
Liu, P., Wang, Y.-F., Ewis, H. E., Abdelal, A. T., Lu, C.-D., Harrison, R. W., et al. (2004). Covalent reaction intermediate revealed in crystal structure of the Geobacillus stearothermophilus carboxylesterase Est30. J. Mol. Biol. 342, 551–561. doi: 10.1016/j.jmb.2004.06.069
PubMed Abstract | Full Text | CrossRef Full Text | Google Scholar
López, G., Chow, J., Bongen, P., Lauinger, B., Pietruszka, J., Streit, W. R., et al. (2014). A novel thermostable esterase from Acidicaldus sp. strain USBA-GBX-499 with enantioselectivity isolated from an acidic hot spring of Colombian Andes. Appl. Microbiol. Biotechnol. 98, 8603–8616. doi: 10.1007/s00253-014-5775-7
PubMed Abstract | Full Text | CrossRef Full Text | Google Scholar
Ludwig, W., Strunk, O., Westram, R., Richter, L., Meier, H., Yadhukumar, et al. (2004). ARB: a software environment for sequence data. Nucleic Acids Res. 32, 1363–1371. doi: 10.1093/nar/gkh293
Lussier, F.-X., Chambenoit, O., Côté, A., Hupé, J.-F., Denis, F., Juteau, P., et al. (2011). Construction and functional screening of a metagenomic library using a T7 RNA polymerase-based expression cosmid vector. J. Ind. Microbiol. Biotechnol. 38, 1321–1328. doi: 10.1007/s10295-010-0915-2
PubMed Abstract | Full Text | CrossRef Full Text | Google Scholar
Marchler-Bauer, A., Lu, S., Anderson, J. B., Chitsaz, F., Derbyshire, M. K., DeWeese-Scott, C., et al. (2011). CDD: a Conserved Domain Database for the functional annotation of proteins. Nucleic Acids Res. 39, D225–D229. doi: 10.1093/nar/gkq1189
PubMed Abstract | Full Text | CrossRef Full Text | Google Scholar
Martinez, A., Kolvek, S. J., Yip, C. L. T., Hopke, J., Brown, K. A., MacNeil, I. A., et al. (2004). Genetically modified bacterial strains and novel bacterial artificial chromosome shuttle vectors for constructing environmental libraries and detecting heterologous natural products in multiple expression hosts. Appl. Environ. Microbiol. 70, 2452–2463. doi: 10.1128/AEM.70.4.2452-2463.2004
PubMed Abstract | Full Text | CrossRef Full Text | Google Scholar
Mergulhão, F. J. M., Summers, D. K., and Monteiro, G. A. (2005). Recombinant protein secretion in Escherichia coli. Biotechnol. Adv. 23, 177–202. doi: 10.1016/j.biotechadv.2004.11.003
PubMed Abstract | Full Text | CrossRef Full Text | Google Scholar
Overbeek, R., Begley, T., Butler, R. M., Choudhuri, J. V., Chuang, H.-Y., and Cohoon, M. (2005). The subsystems approach to genome annotation and its use in the project to annotate 1000 genomes. Nucleic Acids Res. 33, 5691–5702. doi: 10.1093/nar/gki866
PubMed Abstract | Full Text | CrossRef Full Text | Google Scholar
Parachin, N. S., and Gorwa-Grauslund, M. F. (2011). Isolation of xylose isomerases by sequence- and function-based screening from a soil metagenomic library. Biotechnol. Biofuels 4:9. doi: 10.1186/1754-6834-4-9
PubMed Abstract | Full Text | CrossRef Full Text | Google Scholar
Petersen, E. I., Valinger, G., Sölkner, B., Stubenrauch, G., and Schwab, H. (2001). A novel esterase from Burkholderia gladioli which shows high deacetylation activity on cephalosporins is related to beta-lactamases and DD-peptidases. J. Biotechnol. 89, 11–25. doi: 10.1016/S0168-1656(01)00284-X
PubMed Abstract | Full Text | CrossRef Full Text | Google Scholar
Petersen, T. N., Brunak, S., Heijne, G., and von Nielsen, H. (2011). SignalP 4.0: discriminating signal peptides from transmembrane regions. Nat. Methods 8, 785–786. doi: 10.1038/nmeth.1701
PubMed Abstract | Full Text | CrossRef Full Text | Google Scholar
Pietruszka, J., Simon, R. C., Kruska, F., and Braun, M. (2009). Dynamic enzymatic kinetic resolution of methyl 2,3-dihydro-1H-indene-1-carboxylate. Eur. J. Org. Chem. 2009, 6217–6224. doi: 10.1002/ejoc.200901025
Punta, M., Coggill, P. C., Eberhardt, R. Y., Mistry, J., Tate, J., Boursnell, C., et al. (2012). The Pfam protein families database. Nucleic Acids Res. 40, D290–D301. doi: 10.1093/nar/gkr1065
Ramírez-Arcos, S., Fernández-Herrero, L. A., Marín, I., and Berenguer, J. (1998). Anaerobic growth, a property horizontally transferred by an Hfr-like mechanism among extreme thermophiles. J. Bacteriol. 180, 3137–3143.
Rashamuse, K., Magomani, V., Ronneburg, T., and Brady, D. (2009). A novel family VIII carboxylesterase derived from a leachate metagenome library exhibits promiscuous beta-lactamase activity on nitrocefin. Appl. Microbiol. Biotechnol. 83, 491–500. doi: 10.1007/s00253-009-1895-x
PubMed Abstract | Full Text | CrossRef Full Text | Google Scholar
Reetz, M. T., Prasad, S., Carballeira, J. D., Gumulya, Y., and Bocola, M. (2010). Iterative saturation mutagenesis accelerates laboratory evolution of enzyme stereoselectivity: rigorous comparasion with traditional methods. J. Am. Chem. Soc. 132, 9144–9152. doi: 10.1021/ja1030479
PubMed Abstract | Full Text | CrossRef Full Text | Google Scholar
Reetz, M. T., Zonta, A., Schimossek, K., Jaeger, K.-E., and Liebeton, K. (1997). Creation of enantioselective biocatalysts for organic chemistry by in vitro evolution. Angew. Chem. Int. Ed. Engl. 36, 2830–2832. doi: 10.1002/anie.199728301
Rhee, J. K., Ahn, D. G., Kim, Y. G., and Oh, J. W. (2005). New thermophilic and thermostable esterase with sequence similarity to the hormone-sensitive lipase family, cloned from a metagenomic library. Appl. Environ. Microbiol. 71, 817–825. doi: 10.1128/AEM.71.2.817-825.2005
PubMed Abstract | Full Text | CrossRef Full Text | Google Scholar
Sandström, A. G., Wikmark, Y., Engström, K., Nyhlén, J., and Bäckvall, J.-E. (2012). Combinatorial reshaping of the Candida Antarctica lipase A substrate pocket for enantioselectivity using an extremely condensed library. Proc. Natl. Acad. Sci. U.S.A. 109, 78–83. doi: 10.1073/pnas.1111537108
PubMed Abstract | Full Text | CrossRef Full Text | Google Scholar
Satokari, R. M., Vaughan, E. E., Akkermans, A. D., Saarela, M., and de Vos, W. M. (2001). Bifidobacterial diversity in human feces detected by genus-specific PCR and denaturing gradient gel electrophoresis. Appl. Environ. Microbiol. 67, 504–513. doi: 10.1128/AEM.67.2.504-513.2001
PubMed Abstract | Full Text | CrossRef Full Text | Google Scholar
Shahi, P., Kumar, I., Sharma, R., Sanger, S., and Jolly, R. S. (2006). Characterization of a novel long-chain acyl-CoA thioesterase from Alcaligenes faecalis. FEBS J. 273, 2374–2387. doi: 10.1111/j.1742-4658.2006.05244.x
PubMed Abstract | Full Text | CrossRef Full Text | Google Scholar
Sorek, R., Zhu, Y., Creevey, C. J., Francino, M. P., Bork, P., and Rubin, E. M. (2007). Genome-wide experimental determination of barriers to horizontal gene transfer. Science 318, 1449–1452. doi: 10.1126/science.1147112
PubMed Abstract | Full Text | CrossRef Full Text | Google Scholar
Sørensen, H. P., and Mortensen, K. K. (2005). Advanced genetic strategies for recombinant protein expression in Escherichia coli. J. Biotechnol. 115, 113–128. doi: 10.1016/j.jbiotec.2004.08.004
PubMed Abstract | Full Text | CrossRef Full Text | Google Scholar
Steele, H. L., Jaeger, K.-E., Daniel, R., and Streit, W. R. (2009). Advances in recovery of novel biocatalysts from metagenomes. J. Mol. Microbiol. Biotechnol. 16, 25–37. doi: 10.1159/000142892
PubMed Abstract | Full Text | CrossRef Full Text | Google Scholar
Tamura, K., Peterson, D., Peterson, N., Stecher, G., Nei, M., and Kumar, S. (2011). MEGA5: molecular evolutionary genetics analysis using maximum likelihood, evolutionary distance, and maximum parsimony methods. Mol. Biol. Evol. 28, 2731–2739. doi: 10.1093/molbev/msr121
PubMed Abstract | Full Text | CrossRef Full Text | Google Scholar
Torsvik, V., Goksøyr, J., and Daae, F. L. (1990). High diversity in DNA of soil bacteria. Appl. Environ. Microbiol. 56, 782–787.
Villegas, A., and Kropinski, A. M. (2008). An analysis of initiation codon utilization in the Domain Bacteria—concerns about the quality of bacterial genome annotation. Microbiology 154, 2559–2661. doi: 10.1099/mic.0.2008/021360-0
PubMed Abstract | Full Text | CrossRef Full Text | Google Scholar
Wang, B., Lu, D., Gao, R., Yang, Z., Cao, S., and Feng, Y. (2004). A novel phospholipase A2/esterase from hyperthermophilic archaeon Aeropyrum pernix K1. Protein Expr. Purif. 35, 199–205. doi: 10.1016/j.pep.2004.01.010
PubMed Abstract | Full Text | CrossRef Full Text | Google Scholar
Warren, R. L., Freeman, J. D., Levesque, R. C., Smailus, D. E., Flibotte, S., and Holt, R. A. (2008). Transcription of foreign DNA in Escherichia coli. Genome Res. 18, 1798–1805. doi: 10.1101/gr.080358.108
PubMed Abstract | Full Text | CrossRef Full Text | Google Scholar
Wu, Q., Soni, P., and Reetz, M. T. (2013). Laboratory evolution of enantiocomplementary Candida antarctica lipase B mutants with broad substrate scope. J. Am. Chem. Soc. 135, 1872–1881. doi: 10.1021/ja310455t
PubMed Abstract | Full Text | CrossRef Full Text | Google Scholar
Yu, E. Y., Kwon, M.-A., Lee, M., Oh, J. Y., Choi, J.-E., Lee, J. Y., et al. (2011). Isolation and characterization of cold-active family VIII esterases from an arctic soil metagenome. Appl. Microbiol. Biotechnol. 90, 573–581. doi: 10.1007/s00253-011-3132-7
PubMed Abstract | Full Text | CrossRef Full Text | Google Scholar
Keywords: Thermus thermophiles, novel screening host, functional metagenomics, comparative screening, novel metagenomic esterases
Citation: Leis B, Angelov A, Mientus M, Li H, Pham VTT, Lauinger B, Bongen P, Pietruszka J, Gonçalves LG, Santos H and Liebl W (2015) Identification of novel esterase-active enzymes from hot environments by use of the host bacterium Thermus thermophilus. Front. Microbiol. 6:275. doi: 10.3389/fmicb.2015.00275
Received: 18 December 2014; Accepted: 19 March 2015;
Published: 08 April 2015.
Edited by:
Katharina Riedel, University of Greifswald, GermanyReviewed by:
Rene Sutherland, University of Pretoria, South AfricaJustin Joseph Donato, University of St. Thomas, USA
Copyright © 2015 Leis, Angelov, Mientus, Li, Pham, Lauinger, Bongen, Pietruszka, Gonçalves, Santos and Liebl. This is an open-access article distributed under the terms of the Creative Commons Attribution License (CC BY). The use, distribution or reproduction in other forums is permitted, provided the original author(s) or licensor are credited and that the original publication in this journal is cited, in accordance with accepted academic practice. No use, distribution or reproduction is permitted which does not comply with these terms.
*Correspondence: Wolfgang Liebl, Department of Microbiology, School of Life Sciences Weihenstephan, Technische Universität München, Emil-Ramann-Str. 4, 85354 Freising, Germanyd2xpZWJsQHd6dy50dW0uZGU=