- 1Department of Biochemistry, University of Hyderabad, Hyderabad, India
- 2Department of Animal Biology, University of Hyderabad, Hyderabad, India
Herbal products derived from Hemidesmus indicus (L.) R. Br. ex Schult, Leucas aspera (Wild.), Plumbago zeylanica L., and Tridax procumbens (L.) R. Br. ex Schult. are widely used in traditional medicine. Though the extracts of these plants were found to be antimicrobial in nature and have the potential to be used in clinics, the mechanism of action of is not reported. The ethanolic extracts of Hemidesmus indicus (L.) R. Br. ex Schult, Hemidesmus indicus ethanolic extract (HIEE), Leucas aspera (Wild.), Leucas aspera ethanolic extract (LAEE), Plumbago zeylanica L., Plumbago zeylanica ethanolic extract (PZEE), and Tridax procumbens (L.) R. Br. ex Schult, Tridax procumbens ethanolic extract (TPEE) were tested for their antibacterial activity against E. coli. Antibacterial activity was analyzed by CFU assay and the effect on the bacterial membrane by fluorescence activated cell sorting and scanning electron microscopy. LAEE, PZEE, and HIEE displayed potent bacterial killing activity in a time and concentration dependent manner. TPEE did not display appreciable antibacterial activity. The antibacterial action involved disruption of membrane potential, inner membrane permeabilization, blebbing and leakage of cellular contents. Our results contribute to the understanding of the antibacterial mechanism of alcoholic extracts of the medicinal plants used in this study.
Introduction
Complementary and alternative forms of medicine (CAM) have been practiced through out the world for centuries. Majority of these practices involve the use of crude plant extracts or purified products from different parts of plants and is dependent on the biodiversity available within the regions, thereby forming a local heritage (Purohit and Vyas, 2004a). In India, CAM is practiced for more than 5,000 years in different forms such as Ayurveda, Sidda, Homeopathy, and Unani and these are licensed by the Government. In the recent years, treatment strategies that involve use of natural products derived from plants has gained importance and this is primarily to overcome the side effects of allopathic forms of medicine. Besides this, multidrug resistance by pathogens to currently used antibiotics triggered the search for identifying natural antimicrobial products that are effective in combating infections (Cowan, 1999). The World health organization (WHO) estimates that the traditional systems of medicines are accepted by almost 80% of the population throughout the world for their primary health needs (Purohit and Vyas, 2004b). Further, the National Health Interview Survey (NHIS) conducted by the Centers for Disease Control and Prevention (CDC) in 2007 indicate that around 40% of adults in the United States used some form of complementary and alternative medicine, indicating that such medical practices are prevalent even in the developed nations (Barnes et al., 2008). Hence, CAM is accepted as one of the “mainstream” health care practices. Medicinal plants continue to play an important role in CAM treatment strategies since they produce a wide variety of natural compounds with high therapeutic value (Nostro et al., 2000). Examples include cocaine, codeine, digitoxin, galantamine, quinine, and morphine, which are widely used in many clinical conditions (Newman et al., 2003; Butler, 2004). Similarly, certain plant compounds such as arteether, nitisinone, and tiotropium are currently involved in late-phase clinical trials (Balunas and Kinghorn, 2005).
Hemidesmus indicus (L.) R. Br. ex Schult (HI; Family: Apocynaceae) is a medicinally important species and the extracts obtained from various parts of the plant were found to be diaphoretic, demulcent and antimicrobial (Jain and Basal, 2003). Leucas aspera (Wild.) (LA; Family: Lamiaceae) is an Indian herbal plant, whose extracts have been shown to have antinociceptive, antidiabetic, antimicrobial, antioxidant, and cytotoxic properties (Ali et al., 2001; Ravikumar et al., 2005; Bhagwat et al., 2008; Prajapati et al., 2010). Plumbago zeylanica L. (PZ; Family: Plumbaginaceae) is one of the most widely used medicinal plant because of its antimitotic, antibacterial, antinematicidal and anticancer properties (Pant et al., 2012). Tridax procumbens (L.) R. Br. ex Schult. (TP; Family: Asteraceae) is also a medicinally important plant used for the treatment of liver disorders, diarrhea wound healing, diabetes and infections Though the antimicrobial activity of the above mentioned plants and other medicinally important plants were reported (Cowan, 1999; Araujo et al., 2014; Choi et al., 2014; Marinas et al., 2014; Melo et al., 2014), a systematic study on the kinetics of bacterial killing and the mechanism of action have not yet been investigated. It is well known that the general mechanism of antimicrobial agents involves bacterial membrane permeabilization or disruption of membrane potential or inhibition of macromolecular synthesis (Zasloff, 2002). On the other hand, conventionally used antibiotics exert their action on microbes by influencing cell wall, protein and nucleic acid syntheses and metabolite activity. To overcome the use of increasing doses of antibiotics, treatment strategies that involve the use of additional antimicrobial agent(s) along with antibiotics has gained importance. It would be advantageous if the mechanism of action of the additional antimicrobial agent is different from that of the antibiotic used. Plant extracts or active principles of herbal products being non toxic to the mammalian cells would be the best choice to be combined with antibiotics. Hence, determining the mechanism of antimicrobial action of extracts obtained from medicinally important plants will help to design alternate or combinational treatment strategies to treat infections caused by antibiotic resistant bacteria. Hence, in this study, we determine the bacterial killing kinetics and the mechanism of action of the root extracts of HI and PZ and the whole plant extracts of LA and TP.
Materials and Methods
Plant Material
HI (roots), LA (whole plant), PZ (roots), and TP (whole plant) were collected during July—September, 2012 in and around University of Hyderabad campus (17.4600°N, 78.3350°E). A voucher specimen of HI (UoH/MDP/NA-00004), LA (UoH/MDP/NA-00002), PZ (UoH/MDP/NA-00003), and TP (UoH/MDP/NA-00001) has been preserved in our laboratory and in the herbarium for future reference. Precautions were taken to ensure the specimens are not damaged by insects. The different parts of plants were chosen as per their usage in the traditional system of medicine (THE AYURVEDIC PHARMACOPOEIA OF INDIA, Volumes 1–7 published by Government Of India Ministry Of Health And Family Welfare Department Of Ayush, New Delhi, India).
Preparation of Extracts and Phytochemical Screening
The powdered plant material of HI (roots), LA (whole plant), PZ (roots), and TP (whole plant) were suspended in 95% ethanol, since it is a polar solvent. They were extracted using Soxhlet for 16–24 h or until the solvent was clear. Ethanol solvent used for extraction was recovered under reduced pressure (Buchii rotavapor). The residual extracts were weighed and reconstituted in phosphate buffered saline (PBS) at a concentration of 10 mg/ml. Required amount of this extract was added directly to the assays and the equivalent amount of PBS was added to the controls. The ethanolic extracts obtained from HI (roots), LA (whole plant), PZ (roots), and TP (whole plant) were labeled as HIEE, LAEE, PZEE, and TPEE.
Phytochemical screening was carried out using qualitative tests as described earlier (Sofowora, 1993; Harborne, 1998). All the extracts were diluted with distilled water in the ratio of 1:100 (w/v) except for the cardiac glycoside test. For the alkaloid detection, 5 ml of the extract was added to 2 ml of HCl. To this acidic medium, 1 ml of Dragendroff’s reagent was added. An orange or red precipitate produced immediately indicated the presence of alkaloids. To test the presence of flavonoids, to 1 ml of the extract, a few drops of dilute sodium hydroxide was added. The appearance of an intense yellow color that becomes colorless on addition of a few drops of dilute acid indicates the presence of flavonoids. Steroids were detected by adding 1 ml of the extract to 10 ml of chloroform and an equal volume of concentrated sulphuric acid. Presence of steroids is indicated by the appearance of red and green colors in the upper and sulphuric acids layers. For saponin detection, the extract was diluted with 20 ml of distilled water and was agitated in a graduated cylinder for 15 min. The formation of 1 cm layer of foam showed the presence of saponins. Phenols were detected by warming 2 ml of the extract to 45–50°C followed by addition of 2 ml of 3% FeCl3. Formation of green or blue color indicated the presence of phenols.
Determination of Total Phenolic and Flavonoid Content
The amount of total soluble phenolic content in the plant extracts (HIEE, LAEE, PZEE, and TPEE) was determined as described earlier (Singleton et al., 1999; Yang et al., 2007). Briefly, an aliquot (10 μl) of each extract was mixed with 1 ml of dd H2O and 100 μl of Folin–Ciocalteu’s phenol reagent. 300 μl of 20% Na2CO3 solution was added to the mixture followed by incubating at room temperature in the dark for 2 h and 30 min. The absorbance against a blank was measured at 735 nm using UV–Vis spectrophotometer. Gallic acid was used to prepare a standard curve. The total phenolic content was measured as Gallic acid equivalents [mg GAE/gram of dry weight (DW)] and the values were presented as means of triplicate analysis.
Total flavonoid content of the extracts was estimated using standard protocols (Dewanto et al., 2002; Barreira et al., 2010). An aliquot (20 μl) of each extract was mixed with 500 μl of double distilled water and 30 μl of 5% NaNO2 solution. After proper mixing and 10 min incubation, 60 μl of 10% AlCl3 solution was added. Subsequently, 350 μl of 1 M NaOH and 40 μl of ddH2O were added to make the final volume to 1 ml. Samples were further incubated for 15 min at room temperature and the absorbance of the samples was measured at 510 nm against blank. Quercetin was used as standard reference. The total flavonoids were determined as Quercetin equivalents (mg QE)/g of DW and the values were expressed as means of triplicate analysis.
Antibacterial Activity
The colony forming units (CFU) assay was employed to test the antibacterial activity as described by (Rajesh and Yenugu, 2012). E. coli XL-1 Blue obtained commercially (Stratagene, La Jolla, CA, USA) was used to determine the antibacterial activity of the plant extracts. The bacterial cells were cultured in Luria–Bertani medium at 37°C. Bacterial cells grown to mid-log phase (A600 = 0.4–0.5) were diluted with 10 mM sodium phosphate buffer (pH 7.4). Varying concentrations (10–500 μg/ml) of TPEE, LAEE, HIEE, and PZEE were added to approximately 2 × 106 CFU/ml of bacteria and incubated at 37°C for 30–180 min. After incubation, the assay mixtures were diluted (1:100 and 1:1000) with 10 mM sodium phosphate buffer (pH 7.4) and 50 μl of each was spread on a Luria–Bertani agar plate and incubated at 37°C overnight to allow full colony development. The resulting colonies were hand counted and plotted as log CFU/ml. The antibacterial activity was considered to be potent only when there were more than two logs of reduction in bacterial count.
Determination of Membrane Potential
Membrane potential across the bacterial membrane was measured as described previously (Novo et al., 2000; Yeh et al., 2012). The effect of plant extracts on the membrane potential of bacteria was measured by using two different dyes, namely, 3,3′-diethyloxacarbocyanine iodide, DiOC2(3) and TO-PRO-3. DiOC2(3) can emit green and red fluorescence. The green fluorescence intensity of this dye is largely dependent on the membrane potential, where as the red fluorescence is dependent on the cell size and membrane potential. The ratio of red/green fluorescence gives a measure of the altered membrane potential. In order to eliminate dead cells that may also accumulate DiOC2(3) due to altered membrane permeability, TO-PRO-3, a fluorescent dye that specifically stains dead bacterial cells was included in the assay. Cells that display fluorescence signal of TO-PRO-3 (far red>695 nm) were excluded from the assay so that the membrane potential is measured only in the live cells. E. coli were treated with 100 μg/ml of LAEE or HIEE or PZEE for 2 h followed by 30 μM DiOC2(3) and 100 nM TOPRO-3 for 30 min. Fluorescence intensity was measured using fluorescein isothiocyanate (FITC) and Texas Red channels for green and red fluorescence respectively, whereas TOPRO-3 fluorescence was measured with PerCP-Cy6 channel in fluorescence activated cell sorting (FACS) life science research (LSR) Fortessa flow cytometer (BD Biosciences). Carbonyl cyanide m-chlorophenylhydrazone (CCCP), a proton ionophore was used as a positive control. The ratio of red/green mean fluorescence intensity was calculated to determine the membrane potential.
Membrane Permeabilization
To determine the effects of plant extracts on bacterial membrane integrity, FACS analyses was carried out as described earlier with slight modifications (Mangoni et al., 2004). Bacterial membranes under normal conditions are impermeable to FITC, a fluorescent dye. Membrane destabilization or permeabilization by antibacterial agents would allow the entry of FITC into the cells. Measuring the fluorescence intensity emitted by FITC present inside the bacterial cell gives a direct measure of the extent of membrane permeabilization caused by the test compound. Generally, the fluorescence intensity of FITC inside the bacterial cells is monitored by confocal microscopy and this is only a qualitative measure. To allow quantification, we slightly modified the protocol in which the fluorescence intensity in the bacterial cells was analyzed using a flow cytometer. Briefly, log-phase E. coli were incubated for 2 h with 100 μg/ml of LAEE or HIEE or PZEE in growth medium containing FITC. The cells were then washed thrice to remove the excess FITC present in the medium. Mean fluorescence intensity was measured in the cells using FITC channel in a LSR Fortessa flow cytometer (BD Biosciences). Tetracycline (5 μg/ml), a potent membrane permeabilizing agent was used as the positive control.
Scanning Electron Microscopy
The ability of plant extracts to alter the membrane integrity of E. coli was visualized using scanning electron microscopy as described earlier (Yenugu et al., 2006). Approximately 108 CFU/ml of E. coli resuspended in 10 mM sodium phosphate buffer (pH 7.4) and treated with 200 μg/ml of the plant extract and incubated for 30 min at 37°C. The bacterial cells were washed and resuspended in 10 mM sodium phosphate buffer (pH 7.4) and fixed with an equal volume of 4% glutaraldehyde. Fixed cells were vacuum filtered onto a 0.1 μm polycarbonate membrane filter and dehydrated through a graded series of ethanol (30, 50, 75, and 100%). The filters were then dried and mounted on aluminum specimen supports and coated with 15 nm thickness of gold-palladium metal (60:40 Alloys). Samples were examined on a Cambridge Stereoscan 200 scanning electron microscope using an accelerating voltage of 20 kV.
Statistical Analysis
Statistical analyses were performed using one way ANOVA and Holm-Sidak test available in Sigma Plot software (SPSS Inc., Chicago, IL, USA). Values shown are mean ± SD.
Results
Phytochemical Screening
The phytochemical composition of the plant extracts used in this study (HIME, LAEE, PZEE, and TPEE) was evaluated to determine the variations in the composition of alkaloid, flavonoid, steroid, saponin, and phenolic content. Phytochemical tests revealed that alkaloids were not present in all the extracts analyzed (Table 1). However, the other class of compounds, i.e., steroids, phenolics, flavonoids, and saponins were detected. Further analyses were carried out to determine the flavonoid and phenolic content (Table 1). Flavonoid content (mg GAE/g) was found to be highest (845 ± 0.061) in PZEE followed by LAEE (610 ± 0.011), TPEE (340 ± 0.024), and HIME (75 ± 0.022). On the same lines, the total phenolic content (mg QE/g) was found to be highest in PZEE (552 ± 0.08) followed by LAEE (417 ± 0.09), TPEE (227 ± 0.12), and HIME (18.97 ± 0.16).

Table 1. Phytochemical properties, flavonoid and phenolic composition of plant extracts used in this study.
Antibacterial Activity
To assess the antibacterial property of TP, E. coli were treated with varying concentrations of with TPEE. At concentrations ranging from 10 to 100 μg/ml, bacterial killing was not evident (Figure 1A). When bacteria were incubated with higher concentrations ranging from 200 to 500 μg/ml, bacterial killing was observed with 400 and 500 μg/ml concentrations (Figure 1B). However, the decrease in bacterial count did not go beyond two log units, suggesting that TPEE may not possess appreciable antimicrobial activity. Antibacterial activity was not observed when E. coli were incubated with 10–100 μg/ml of LAEE (Figure 1C), but potent bacterial killing was evident at the higher concentrations in a dose and time dependent manner (Figure 1D). Similar bacterial killing kinetics was observed with HIEE (Figures 2A,B) and PZEE (Figures 2C,D). Complete bacterial killing was observed at the 300 μg/ml concentration for LAEE, HIME, and PZEE. However, the time taken to accomplish complete bacterial killing differed among the three alcoholic extracts, which was 180 min for LAEE and 90 min for both HIEE and PZEE. On the same lines, the time taken to accomplish complete bacterial killing by LAEE was 60 min at 400 and 500 μg/ml concentrations, whereas the same was 30 min for HIEE and PZEE at the same concentrations.
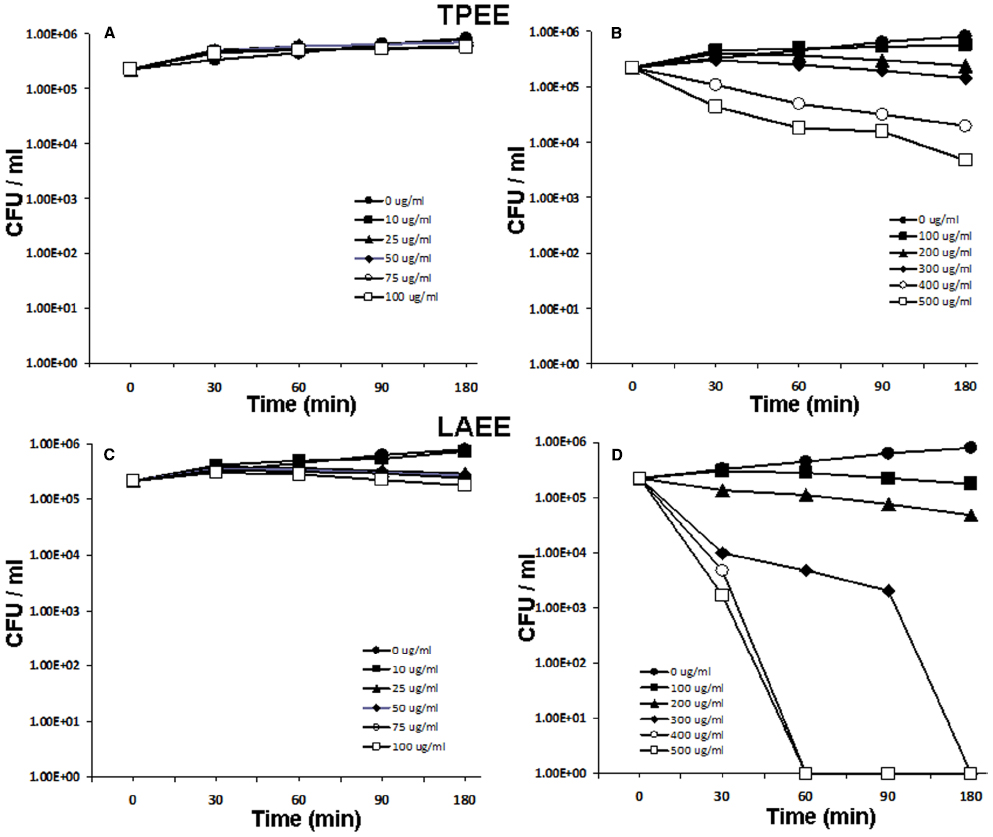
Figure 1. Antibacterial activity of TPEE and LAEE. E. coli were incubated with 0–100 μg/ml (A) or 100–500 μg/ml (B) TPEE or 0–100 μg/ml (C) or 100–500 μg/ml (D) LAEE for 0–180 mins. Bacterial count was analyzed by CFU assay. Values shown are mean ± SD.
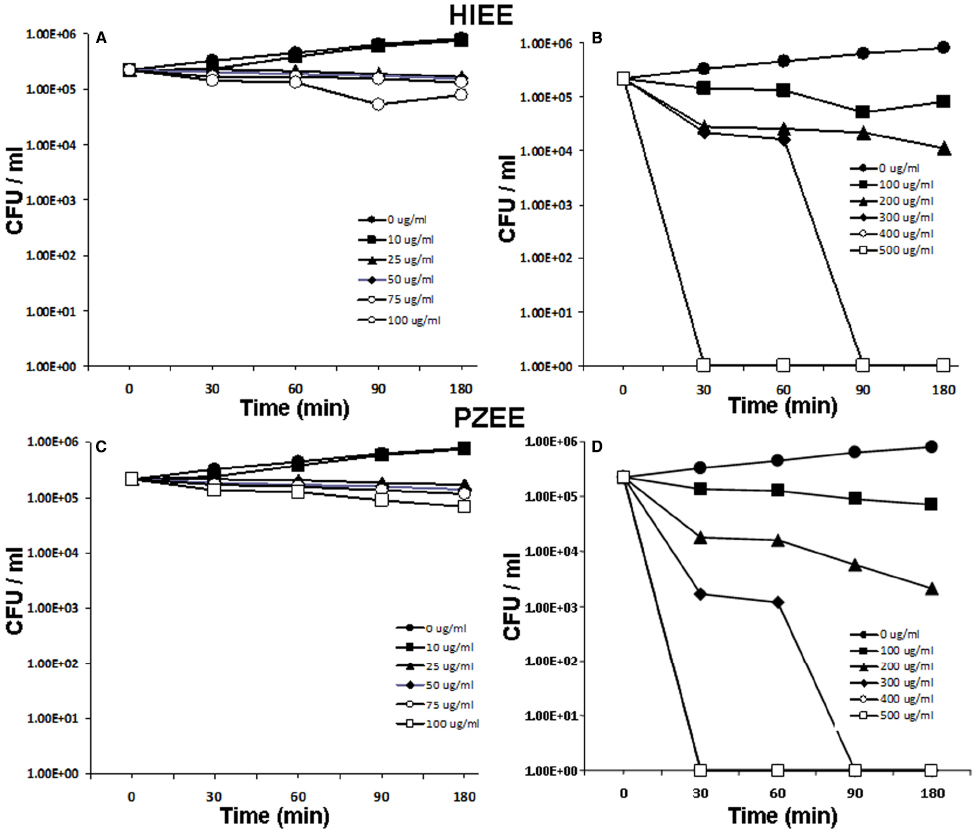
Figure 2. Bacterial killing kinetics of HIEE and PZEE. E. coli were incubated with 0–100 μg/ml (A) or 100–500 μg/ml (B) HIEE or 0–100 μg/ml (C) or 100–500 μg/ml (D) PZEE for 0–180 mins. Bacterial count was analyzed by CFU assay. Values shown are mean ± SD.
Membrane Potential
In bacterial cells with intact cytoplasmic membrane, a difference of electric potential (membrane potential) exists across the membrane with negative charge in the interior and positive in the exterior. To determine whether the antibacterial action of LAEE or HIEE or PZEE involves disruption of bacterial membrane potential, the red and green fluorescence emitted by DiOC2(3) was measured. The ratio of red to green florescence gives and indication of the altered membrane potential. The red and green fluorescence exhibited by the bacterial cells is shown on the X and Y axis respectively (third panel of Figure 3). Treatment of E. coli with the ionophore CCCP resulted in decreased ratio of red to green fluorescence indicating a reduction in membrane potential (Figure 3). Treatment with LAEE or HIEE or PZEE also caused a decrease in the ratio of green to red fluorescence (Figure 3), suggesting that the mechanism of action involves disruption of membrane potential. Among the three ethanolic extracts tested, LAEE seems to be more potent followed by PZEE and HIEE.
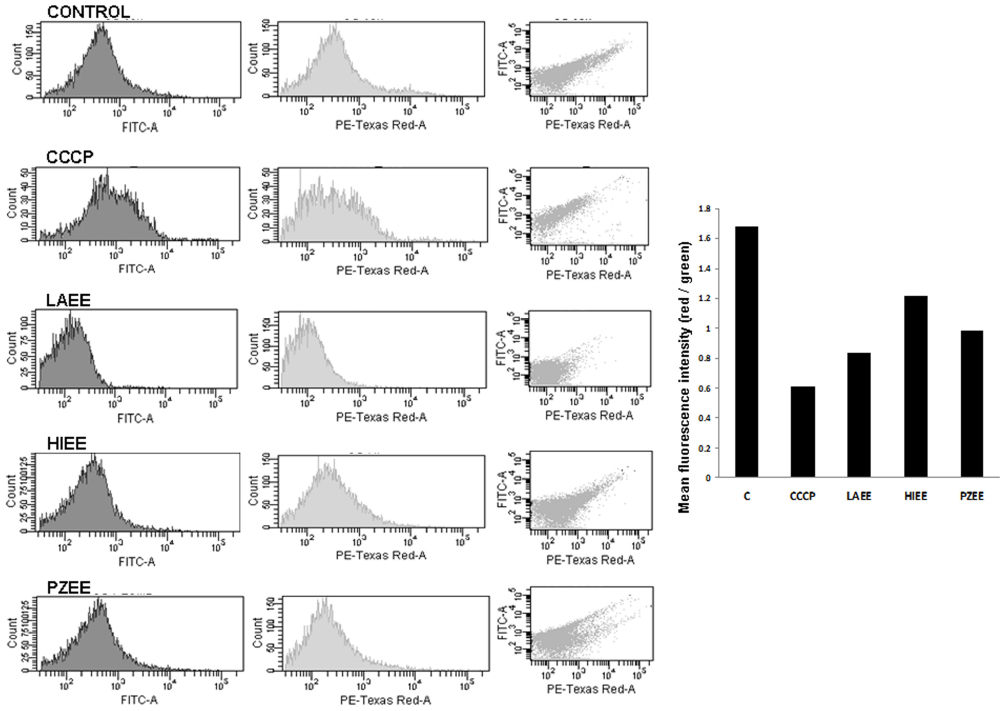
Figure 3. Disruption of membrane potential by plant extracts. E. coli treated with 100 μg/ml of LAEE or HIEE or PZEE or CCCP (15 μM) and incubated with 30 μM DiSO2(3). Red and green fluorescence intensity was measured using FITC and Texas red channels in a FACS fortessa flow cytometer. Intensity of red (left panel) and green fluorescence (middle panel) and cells exhibiting both the fluorescence intensities (left panel) are shown. Bar graph shows the membrane potential of E. coli without (control; C) or with CCCP or different plant extracts treatment.
Membrane Permeabilization
Antibacterial agents, in general, act on the membranes of microbes to cause destabilization and permeabilization. Bacterial cells in general are impermeable to FITC, a fluorescent dye; but upon membrane destabilization or permeabilization, FITC can enter the cells. Intense fluorescence was observed in bacterial cells treated with PZEE (Figure 4A), suggesting inner membrane permeabilization. The ability and the extent of plant extracts to permeabilize E. coli membrane was assessed by FACS analyses (Figure 4B). The first and second panels show the side scatter and FITC fluorescence exhibited by the bacterial cells respectively, whereas the third panel displays the mean fluorescence intensity of FITC. To validate the assay, tetracycline, a membrane permeabilizing agent was used as a positive control. The mean fluorescence intensity was increased in cells treated with tetracycline (Figure 4C). Treatment with HIEE or PZEE also caused an increase in the fluorescence intensity indicating the entry of FITC into the cells, thus membrane permeabilization (Figure 4C). Among the two, HIME seems to be more a potent membrane permeabilization agent than PZEE is comparable with that of tetracycline. However, LAEE treatment did not cause an increase in mean fluorescence intensity.
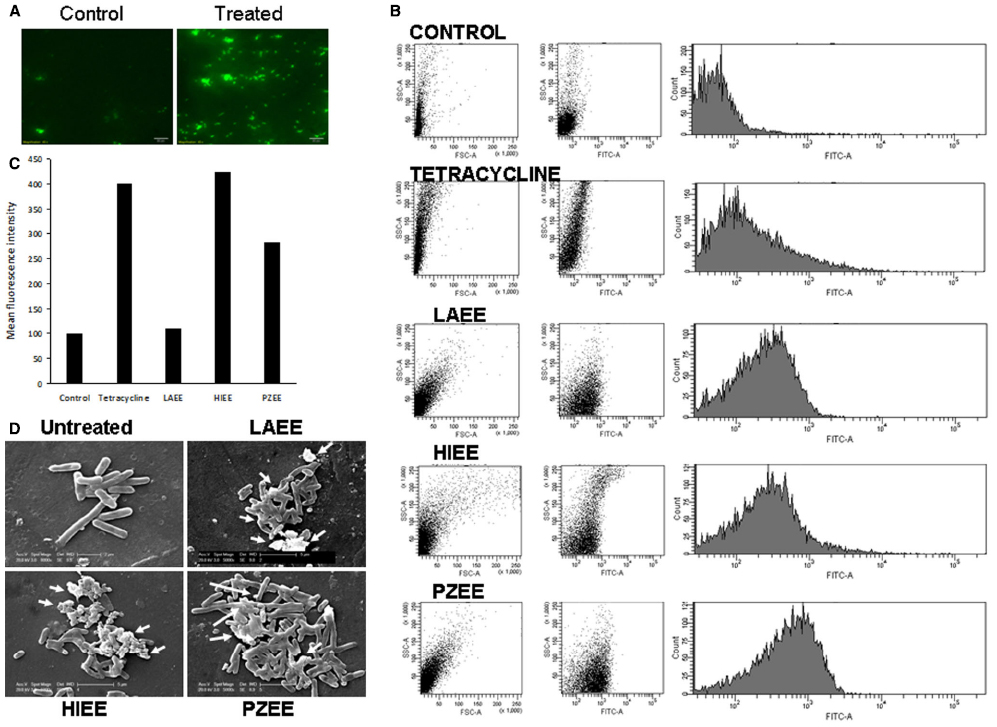
Figure 4. Inner membrane permeabilization induced by plant extracts. E. coli were incubated with 100 μg/ml of LAEE or HIEE or PZEE or tetracycline (5 μg/ml) for 2 h followed by incubation with 5 μg/ml of FITC. (A) Representative fluorescence microscopy images of E. coli showing influx of FITC with or without PZEE treatment. (B) FACS cytograms of control, LAEE or HIEE or PZEE treated E. coli. Left panel indicates forward and side scatter measurement; middle panel indicates population of FITC positive cells; left panel indicates fluorescence intensity range of FITC positive cells. (C) Mean fluorescence intensity in E. coli treated with different plant extracts. (D) Scanning electron microscopy. Bacterial cells treated with 200 μg/ml of LAEE or HIEE or PZEE for 2 h were visualized under scanning electron microscope. Arrows indicate the damage observed.
Scanning Electron Microscopy
Disruption of membrane integrity, results in leakage of cellular contents and death. In order to determine whether the plant extracts used in this study exert their antibacterial action by destabilizing the membrane, scanning microscopy was performed. Untreated E. coli displayed smooth and even membrane morphology. E. coli treated with HIEE or LAEE or PZEE showed distorted membrane morphology, blebbing of membrane, aggregation and leakage of cellular contents (Figure 4D). These results demonstrate that the plant extracts act on the membrane potential and elicit bacterial killing effects.
Discussion
Multi drug resistance by pathogens to conventionally used antibiotics led to the need for identifying novel anti infectives with mechanisms of actions that cannot be circumvented by the microbes. Proteins, peptides and many other small molecules derived from animal and plant sources were found to be antimicrobial. However, the mechanism of action of the plant products has received little attention. Hence, in this study, we attempted to understand the structural changes that occur in E. coli due to the antimicrobial action of ethanolic extracts of HI, LA, TP and PA. HIEE, LAEE, and PZEE exhibited potent antimicrobial activity. The antimicrobial activity of extracts prepared from different parts of HI, LA, PA, and TP is reported (Malathy and Sini, 2009; Naqash and Nazeer, 2011; Chew et al., 2012; Jindal and Kumar, 2012). Our results are in agreement with the previously reported studies. Though the antimicrobial, plasmodicidal, and antileishmanial activities of TP were reported (Martin-Quintal et al., 2010; Appiah-Opong et al., 2011; Naqash and Nazeer, 2011), we did not observe appreciable antibacterial activity with the alcoholic extract of TP. In our study, we used the extract of the whole plant, whereas in previous studies leaf extract or flavonoids obtained from this plant were studied (Martin-Quintal et al., 2010; Appiah-Opong et al., 2011; Naqash and Nazeer, 2011). It is possible that the minimal antibacterial activity of TPEE observed in our study could be due to use of whole plant extract, which may have lesser concentration of the active compounds when compared to extracts obtained from a single organ of the plant.
The crude and partially purified extracts of plants tend to contain a mixture of molecules that vary in chemical structure and composition, which in turn may influence the biological actions. The flavonoid and total phenolic content differed among the plant extracts used in this study; with the highest content observed in PZEE. However, a correlation between the flavonoid or phenolic content and the antibacterial activity. However, we observed that PZEE that has the highest content was the most potent among the four extracts used. The antibacterial activity observed could also be due to the phytochemicals other than flavonoids.
Evaluating the time course killing of bacterial cells by antimicrobials is essential to speculate whether the antimicrobial action of a compound is instantaneous (affecting the membrane integrity) or time dependent (affecting the cellular processes). In order to determine the mode of action of the alcoholic extracts used in this study, the kinetics of bacterial killing at different concentrations was evaluated. The time dependent killing of E. coli by alcoholic extracts of the plants suggests that the antimicrobial action observed could be due to the effect on a variety of physiological factors inside the cell. Further studies are required to test the ability of the plant extracts to affect the cellular process such as inhibition of macromolecular synthesis.
Antimicrobial agents disrupt bacterial membrane integrity thereby affecting the membrane potential. In this study, we observed disruption of membrane potential by LAEE, HIEE, and PZEE. Further, influx of FITC into E. coli, was evident, indicating that HIEE and PZEE have the ability to permeabilize inner membrane of E. coli. Such a mechanism of action was not reported till date using whole plant extracts or compounds isolated from different parts of the plant. It is very interesting to note that LAEE, which exhibited potent antibacterial activity, induced membrane potential disruption, but failed to induce inner membrane permeabilization. It is possible that the antibacterial action of LAEE could be restricted to membrane potential disruption, whereas the action of HIEE and PZEE could involve both membrane potential disruption and membrane permeabilization. The membrane potential disrupting ability of the extracts of medicinally important plants is reported. For example, the methanolic extract of Gracilaria tenuistipitata significantly decreased the mitochondrial membrane potential [measured by the fluorescence intensity emitted by DiOC2(3)] in MEGT-treated Ca9-22 cancer cells (Yeh et al., 2012). The extracts of Annona muricata had the ability to disrupt mitochondrial potential to induce apoptosis in cancer cells (Pieme et al., 2014). Further, electron microscopy revealed membrane blebbing and leakage of bacterial contents when bacterial cells were treated with HIEE or LAEE or PZEE. It is widely believed that the antimicrobial action of a variety of proteins and small molecules result in formation of pores in the bacterial membranes and cause leakage of cellular contents (Yenugu et al., 2006). The ability of HIEE or LAEE or PZEE to cause leakage of cellular contents suggests that they act to cause pores in the bacterial membranes. The membrane damaging action of these extracts could be due to the detergent properties of phenols and flavonoids. However, other mechanisms such as inhibition of macromolecular synthesis that may operate during bacterial killing by these plant extracts needs further investigation.
In conclusion, we report that the ethanolic extracts of HI, LP, and PZ are antibacterial and their action is time and concentration dependent. The mechanism of action of involves disrupting the bacterial membrane potential, permeabilization and leakage of cellular contents. The antibacterial potential of these plant extracts can be exploited to treat infections in place of conventional antibiotics.
Conflict of Interest Statement
The authors declare that the research was conducted in the absence of any commercial or financial relationships that could be construed as a potential conflict of interest.
Acknowledgments
The financial support received from University with Potential for Excellence (UPE) grant of University of Hyderabad is acknowledged. SK and RA received fellowship from Council of Scientific and Industrial Research, Government of India. KM received financial assistance under the Women Scientist Scheme, Department of Science and Technology, Government of India. We thank the support extended by UGC-SAP, UGC-CAS, DBT-CREBB, FIST programs, FACS facility and Scanning Electron Microscopy facility at School of Life Sciences, University of Hyderabad.
Abbreviations
CAM, Complementary and alternative forms of medicine; CCCP, Carbonyl cyanide m-chlorophenylhydrazone; DiOC2(3), 3,3′-diethyloxacarbocyanine iodide; FACS, fluorescence activated cell sorting; FITC, Fluorescein isothiocyanate; HI, Hemidesmus indicus; HIEE, Hemidesmus indicus ethanolic extract; LA, Leucas aspera; LAEE, Leucas aspera ethanolic extract; PZ, Plumbago zeylanica; PZEE, Plumbago zeylanica ethanolic extract; TP, Tridax procumbens; TPEE, Tridax procumbens ethanolic extract.
References
Ali, M., Ravinder, E., and Ramachandram, R. (2001). A new flavonoid from the aerial parts of Tridax procumbens. Fitoterapia 72, 313–315. doi: 10.1016/S0367-326X(00)00296-3
Appiah-Opong, R., Nyarko, A. K., Dodoo, D., Gyang, F. N., Koram, K. A., and Ayisi, N. K. (2011). Antiplasmodial activity of extracts of Tridax procumbens and Phyllanthus amarus in in vitro Plasmodium falciparum culture systems. Ghana Med. J. 45, 143–150.
Araujo, R. C., Neves, F. A., Formagio, A. S., Kassuya, C. A., Stefanello, M. E., Souza, V. V., et al. (2014). Evaluation of the anti-mycobacterium tuberculosis activity and in vivo acute toxicity of Annona sylvatic. BMC Complement. Altern. Med. 14:209. doi: 10.1186/1472-6882-14-209
Balunas, M. J., and Kinghorn, A. D. (2005). Drug discovery from medicinal plants. Life Sci. 78, 431–441. doi: 10.1016/j.lfs.2005.09.012
Barnes, P. M., Bloom, B., and Nahin, R. L., (2008). Complementary and alternative medicine use among adults and children: United States, 2007. Natl. Health Stat. Report. 10, 1–23.
Barreira, J. C., Ferreira, I. C., Oliveira, M. B., and Pereira, J. A. (2010). Antioxidant potential of chestnut (Castanea sativa L.) and almond (Prunus dulcis L.) by-products. Food Sci. Technol. Int. 16, 209–216. doi: 10.1177/1082013209353983
Bhagwat, D. A., Killedar, S. G., and Adnaik, R. S. (2008). Anti-diabetic activity of leaf extract of Tridax procumbens. Int. J. Green Pharm. 2, 126–128. doi: 10.4103/0973-8258.41188
Butler, M. S. (2004). The role of natural product chemistry in drug discovery. J. Nat. Prod. 67, 2141–2153. doi: 10.1021/np040106y
Chew, A. L., Jessica, J. J., and Sasidharan, S. (2012). Antioxidant and antibacterial activity of different parts of Leucas aspera. Asian Pac. J. Trop. Biomed. 2, 176–180. doi: 10.1016/S2221-1691(12)60037-9
Choi, J. S., Lee, Y. R., Ha, Y. M., Seo, H. J., Kim, Y. H., Park, S. M., et al. (2014). Antibacterial effect of grapefruit seed extract (GSE) on Makgeolli-brewing microorganisms and its application in the preservation of fresh Makgeolli. J. Food Sci. 79, M1159–M1167. doi: 10.1111/1750-3841.12469
Dewanto, V., Wu, X., Adom, K. K., and Liu, R. H. (2002). Thermal processing enhances the nutritional value of tomatoes by increasing total antioxidant activity. J. Agric. Food Chem. 50, 3010–3014. doi: 10.1021/jf0115589
Harborne, J. B. (1998). Phytochemical Methods: A Guide to Modern Techniques of Plant Analysis. London: Chapman & Hall.
Jain, A., and Basal, E. (2003). Inhibition of Propionibacterium acnes-induced mediators of inflammation by Indian herbs. Phytomedicine 10, 34–38. doi: 10.1078/094471103321648638
Jindal, A., and Kumar, P. (2012). Antimicrobial flavonoids from Tridax procumbens. Nat. Prod. Res. 26, 2072–2077. doi: 10.1080/14786419.2011.617746
Malathy, N. S., and Sini, S. (2009). Antimicrobial activities of Ichnocarpus frutescens (L.) R.Br. and Hemidesmus indicus R.Br. Roots. Anc. Sci. Life 28, 13–15.
Mangoni, M. L., Papo, N., Barra, D., Simmaco, M., Bozzi, A., Di Giulio, A., et al. (2004). Effects of the antimicrobial peptide temporin L on cell morphology, membrane permeability and viability of Escherichia coli. Biochem. J. 380, 859–865. doi: 10.1042/BJ20031975
Marinas, I. C., Chifiriuc, C., Oprea, E., and Lazăr, V. (2014). Antimicrobial and antioxidant activities of alcoholic extracts obtained from vegetative organs of A. retroflexus. Roum. Arch. Microbiol. Immunol. 73, 35–42.
Martin-Quintal, Z., del Rosario García-Miss, M., Mut-Martín, M., Matus-Moo, A., Torres-Tapia, L. W., and Peraza-Sánchez, S. R. (2010). The leishmanicidal effect of (3S)-16,17-didehydrofalcarinol, an oxylipin isolated from Tridax procumbens, is independent of NO production. Phytother. Res. 24, 1004–1008.
Melo, M. C., Gadelha, D. N., Oliveira, T. K., and Brandt, C. T. (2014). Alcohol extract of Schinu sterebinthifolius raddi (anacardiaceae) as a local antimicrobial agent in severe autogenously fecal peritonitis in rats. Acta Cir. Bras. 29(Suppl. 1), 52–56. doi: 10.1590/S0102-86502014001300010
Naqash, S. Y., and Nazeer, R. A. (2011). Anticoagulant, antiherpetic and antibacterial activities of sulphated polysaccharide from Indian medicinal plant Tridax procumbens L. (Asteraceae). Appl. Biochem. Biotechnol. 165, 902–912. doi: 10.1007/s12010-011-9307-y
Newman, D. J., Cragg, G. M., and Snader, K. M. (2003). Natural products as sources of new drugs over the period 1981–2002. J. Nat. Prod. 66, 1022–1037. doi: 10.1021/np030096l
Nostro, A., Germanò, M. P., D’angelo, V., Marino, A., and Cannatelli, M. A. (2000). Extraction methods and bioautography for evaluation of medicinal plant antimicrobial activity. Lett. Appl. Microbiol. 30, 379–384. doi: 10.1046/j.1472-765x.2000.00731.x
Novo, D. J., Perlmutter, N. G., Hunt, R. H., and Shapiro, H. M. (2000). Multiparameter flow cytometric analysis of antibiotic effects on membrane potential, membrane permeability, and bacterial counts of Staphylococcus aureus and Micrococcus luteus. Antimicrob. Agents Chemother. 44, 827–834. doi: 10.1128/AAC.44.4.827-834.2000
Pant, M., Lal, A., Rana, S., and Rani, A. (2012). Plumbago zeylanica L.: a mini review. Int. J. Pharm. Appl.3, 399–405.
Pieme, C. A., Kumar, S. G., Dongmo, M. S., Moukette, B. M., Boyoum, F. F., Ngogang, J. Y., et al. (2014). Antiproliferative activity and induction of apoptosis by Annona muricata (Annonaceae) extract on human cancer cells. BMC Complement. Altern. Med. 14:516.
Prajapati, M. S., Patel, J. B., Modi, K., and Shah, M. B. (2010). Leucas aspera: a review. Pharmacogn. Rev. 4, 85–87. doi: 10.4103/0973-7847.65330
Purohit, S. S., and Vyas, P. S. (2004a). A Scientific Approach Including Processing and Financial Guidelines. Jodhpur: Agrobios India.
Purohit, S. S., and Vyas, S. P. (2004b). Medicinal Plants: Cultivation A Scientific Approach. Jodhpur: Agrobios (India).
Rajesh, A., and Yenugu, S. (2012). Genomic organization, tissue distribution and functional characterization of the rat Pate gene cluster. PLoS ONE 7:e32633. doi: 10.1371/journal.pone.0032633
Ravikumar, V., Shivashangari, K. S., and Devaki, T. (2005). Effect of Tridax procumbens on liver antioxidant defense system during lipopolysaccharide-induced hepatitis in D-galactosamine sensitised rats. Mol. Cell. Biochem. 269, 131–136. doi: 10.1007/s11010-005-3443-z
Singleton, V. L., Orthofer, R., and Lamuela-Raventós, R. M. (1999). Analysis of total phenols and other oxidation substrates and antioxidants by means of folin–ciocalteu reagent. Methods Enzymol. 299, 152–178. doi: 10.1016/s0076-6879(99)99017-1
Yang, J., Paulino, R., Janke-Stedronsky, S., and Abawi, F. (2007). Free-radical-scavenging activity and total phenols of noni (Morinda citrifolia L.) juice and powder in processing and storage. Food Chem. 102, 302–308. doi: 10.1016/j.foodchem.2006.05.020
Yeh, C. C., Yang, J. I., Lee, J. C., Tseng, C. N., Chan, Y. C., Hseu, Y. C., et al. (2012). Anti-proliferative effect of methanolic extract of Gracilaria tenuistipitata on oral cancer cells involves apoptosis, DNA damage, and oxidative stress. BMC Complement. Altern. Med. 12:142. doi: 10.1186/1472-6882-12-142
Yenugu, S., Hamil, K. G., French, F. S., and Hall, S. H. (2006). Antimicrobial actions of human and macaque sperm associated antigen (SPAG)11 isoforms: influence of the N-terminal peptide. Mol. Cell. Biochem. 284, 25–35. doi: 10.1007/s11010-005-9009-2
Keywords: herbal products, antibacterial activity, membrane permeabilization, flow cytometry
Citation: Saritha K, Rajesh A, Manjulatha K, Setty OH and Yenugu S (2015) Mechanism of antibacterial action of the alcoholic extracts of Hemidesmus indicus (L.) R. Br. ex Schult, Leucas aspera (Wild.), Plumbago zeylanica L., and Tridax procumbens (L.) R. Br. ex Schult. Front. Microbiol. 6:577. doi: 10.3389/fmicb.2015.00577
Received: 02 March 2015; Accepted: 26 May 2015;
Published: 09 June 2015.
Edited by:
Márcia V. Da Silva, Universidade Federal de Pernambuco, BrazilReviewed by:
Vishvanath Tiwari, Central University of Rajasthan, IndiaVijay Kumar, Doon PG College of Agriculture Science and Technology, India
Ladislav Kokoska, Czech University of Life Sciences Prague, Czech Republic
Copyright © 2015 Saritha, Rajesh, Manjulatha, Setty and Yenugu. This is an open-access article distributed under the terms of the Creative Commons Attribution License (CC BY). The use, distribution or reproduction in other forums is permitted, provided the original author(s) or licensor are credited and that the original publication in this journal is cited, in accordance with accepted academic practice. No use, distribution or reproduction is permitted which does not comply with these terms.
*Correspondence: Suresh Yenugu, Department of Animal Biology, University of Hyderabad, P.O. Central University, Hyderabad 500046, Andhra Pradesh, India, ysnaidu@yahoo.com; sureshsl@uohyd.ernet.in
†These authors have contributed equally to this work.