- 1Institute of Disease Control and Prevention, Academy of Military Medical Sciences, Beijing, China
- 2Department of Digestive System, The Second Affiliated Hospital of Dalian Medical University, Dalian, China
Pseudomonas aeruginosa is a major opportunistic pathogen in hospital-acquired infections and exhibits increasing antibiotic resistance. A rapid and sensitive molecular method for its detection in clinical samples is needed to guide therapeutic treatment and to control P. aeruginosa outbreaks. In this study, we established a polymerase spiral reaction (PSR) method for rapid detection of P. aeruginosa by targeting the toxA gene, which regulates exotoxin A synthesis. Real-time turbidity monitoring and a chromogenic visualization using hydroxynaphthol blue were used to assess the reaction. All 17 non- P. aeruginosa strains tested negative, indicating the high specificity of the PSR primers. The detection limit was 2.3 pg/μl within 60 min at isothermal temperature (65°C), 10-fold more sensitive than conventional PCR. Then, the PSR assay was applied to a clinical surveillance of P. aeruginosa in three top hospitals in Beijing, China. Of the 130 sputum samples collected from ICU patients with suspected multi-resistant infections, 37 P. aeruginosa isolates were identified from the positive samples. All clinical strains belonged to 10 different P. aeruginosa multilocus sequence typing groups and exhibited high resistance to carbapenems, cephalosporins, and aminoglycosides. Interestingly, of the 33 imipenem-resistant isolates, 30 (90.9%) had lost the outer membrane porin oprD gene. Moreover, isolate SY-95, containing multiple antibiotic resistance genes, possessed the ability to hydrolyze all antibiotics used in clinic and was susceptible only to polymyxin B. Our study showed the high level of antibiotic resistance and co-occurrence of resistance genes in the clinical strains, indicating a rapid and continuing evolution of P. aeruginosa. In conclusion, we developed a P. aeruginosa PSR assay, which could be a useful tool for clinical screening, especially in case of poor resources, or for point-of-care testing.
Introduction
Pseudomonas aeruginosa is a common opportunistic pathogen capable of infecting both humans and animals. It causes various nosocomial diseases such as pneumonia, respiratory infection (Pereira and Cardoso, 2014), festering wounds (Altoparlak et al., 2005), urinary tract (Mittal et al., 2009), bacteremia (Al-Hasan et al., 2008), and keratitis (Hazlett, 2004). Pulmonary colonization with P. aeruginosa is considered a major cause of morbidity and mortality in patients with cystic fibrosis (Davies, 2002). Moreover, massive use of broad-spectrum antibiotics has increased the resistance of P. aeruginosa to clinical drugs, which has led to serious therapeutic problems (Peng et al., 2014). Thus, timely and accurate diagnosis is necessary for appropriate treatment and to control disease outbreaks.
Various diagnostic methods have been established for P. aeruginosa, including phenotypic methods (Jin et al., 2011), electrochemical techniques (Webster et al., 2014), and molecular methods such as PCR (Aghamollaei et al., 2015), real-time PCR (Qin et al., 2003; Deschaght et al., 2011), and enzyme-linked immunosorbent assay (Mauch et al., 2014). However, these methods are relatively complex, time-consuming, and require specialized, costly instruments, and expertise. Thus, a simple, cost-effective, and rapid detection method is needed.
Polymerase spiral reaction (PSR), a novel nucleic acid amplification method based on the utilization of a DNA polymerase with strand displacement activity under isothermal conditions, meets the requirements of rapidity, high sensitivity and specificity (Liu et al., 2015). Moreover, compared with other established isothermal amplification methods, PSR does not need an initial incubation at 95°C or the inclusion of a DNA helicase in the reaction mixture to achieve denaturation of the DNA double helix (Walker et al., 1992; Notomi et al., 2000; Vincent et al., 2004).
Exotoxin A is an important virulence factor of P. aeruginosa in clinical infections. It is a cytotoxic agent that, similar to diphtheria toxin, inhibits protein biosynthesis at the level of polypeptide chain elongation factor 2, leading to great tissue and organ damage (Jenkins et al., 2004). The toxA gene, an inherent genetic sequence located on the P. aeruginosa chromosome and regulating the synthesis of exotoxin A, has been widely used as a target for P. aeruginosa detection in PCR and RT-PCR methods (Khan and Cerniglia, 1994; Qin et al., 2003; Xu et al., 2004). In this study, we designed PSR primers targeting the toxA gene and optimized the PSR conditions. The specificity and sensitivity of PSR for detection of P. aeruginosa were determined. Finally, on the basis of PSR method, we molecularly characterized clinical P. aeruginosa isolates and investigated their dissemination in intensive care unit (ICU) patients from three top hospitals of Beijing, China.
Materials and Methods
Bacterial Strains, Identification, Multilocus Sequence Typing (MLST), and Antimicrobial Susceptibility Testing
A total of 56 bacterial strains were used in this study (Supplementary Material), including P. aeruginosa ATCC 15442 and P. aeruginosa CMCC 10539 as positive controls. Seventeen non- P. aeruginosa strains including species homologous to P. aeruginosa and other clinical pathogens were included to assess the specificity of the PSR assay. One-hundred and thirty clinical sputum samples were collected from ICU patients with suspected multi-resistant infections in three top hospitals of Beijing. Genomic DNA was extracted from the sputum samples using the Wizard Genomic DNA Purification Kit (Promega, Madison, WI, USA) and then subjected to the PSR assay. The bacterial strains and positive clinical samples were cultured in brain–heart infusion broth according to a standard protocol. Species identification was carried out using the Phoenix Automated Microbiology System (BD Diagnostic Systems, Franklin Lanes, NJ, USA) and sequencing of 16S ribosomal DNA (rDNA).
Multilocus sequence typing was performed to determine the sequence types (STs) of P. aeruginosa strains. Seven housekeeping genes, namely, acsA, aroE, guaA, mutL, nuoD, ppsA, and trpE, were amplified using PCR. The allele sequences for each gene were compared to the P. aeruginosa MLST database1 to yield the allelic profile.
Antimicrobial susceptibility testing was performed using broth microdilution susceptibility testing according to the Clinical and Laboratory Standards Institute guidelines (CLSI, Performance Standards for Antimicrobial Susceptibility Testing; Twenty-third informational supplement CLSI Document M100-S23; Wayne, PA, USA 2013). A screening of the oprD gene which has a function in the P. aeruginosa outer membrane was performed (Wolter et al., 2004). Additionally, the strains were screened for the presence of known metallo-β-lactamase (MBL) and other β-lactamase genes (blaV IM, blaIMP, blaKPC-2, blaTEM, blaSPM-1, blaSIM-1, blaNDM-1, and blaOXA-50) using PCR with primers reported previously (Poirel et al., 2007; Farajzadeh et al., 2014).
PSR Primer Design for the toxA Gene
To design P. aeruginosa specific PSR primers, the nucleotide sequence of toxA was downloaded from the NCBI GenBank database2 The primer sequences and their locations are shown in Figure 1. The uppercase 3′ sequences of the forward primer (F) and reverse primer (B) are complementary to the target toxA gene sequence (nucleotide positions 1365–1381 and 1538–1522, respectively). The lowercase 5′ sequence of the reverse primer (T) is complementary to the target sequence (position 1405–1423), and is reverse to the lowercase 5′ sequence of the forward primer (Tr). Additionally, two accelerated primers IF and IB were included in this study (positions 1403–1387, 1481–1499, respectively).
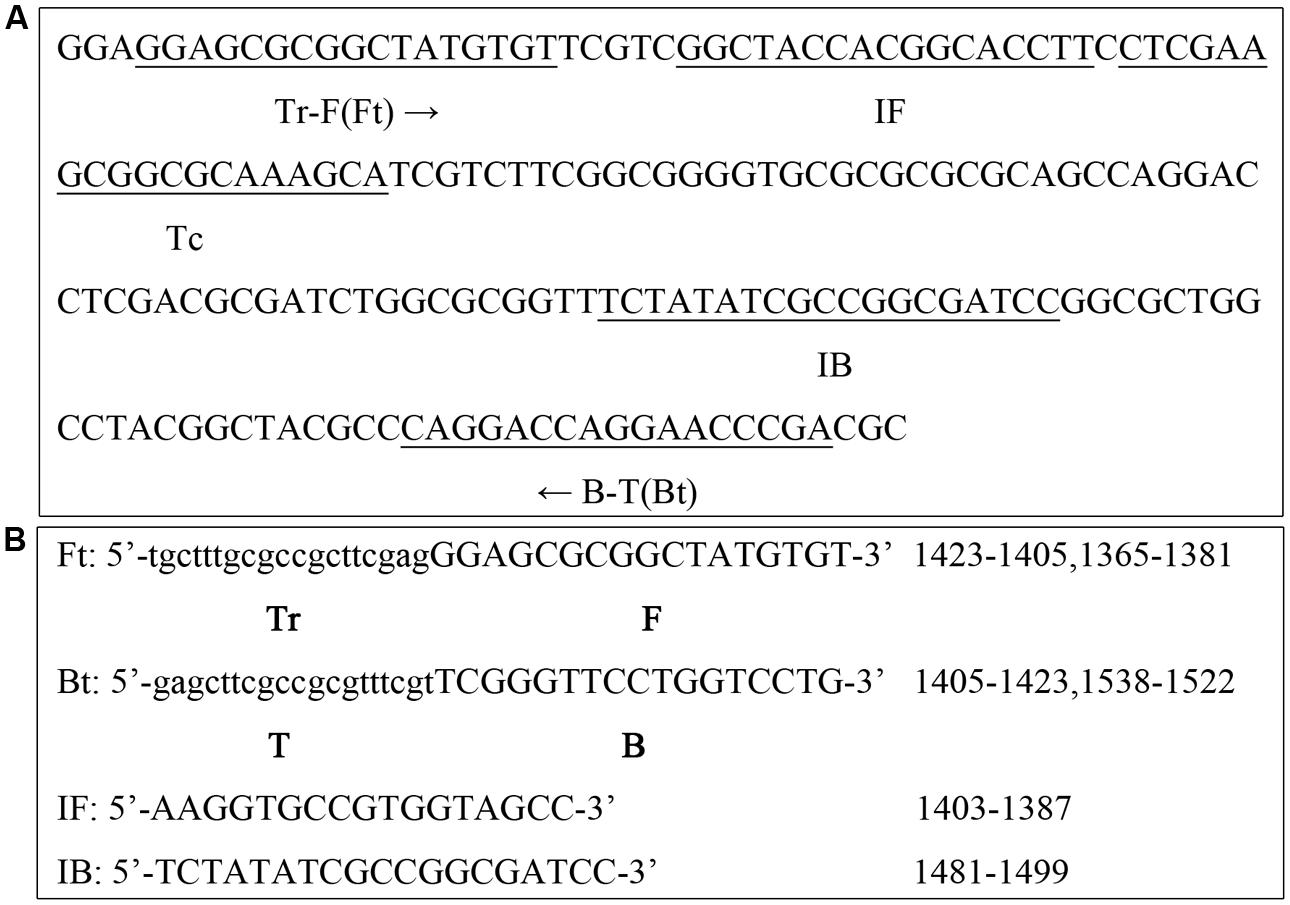
FIGURE 1. Primer design for the polymerase spiral reaction (PSR) assay. (A) Nucleotide sequence of the toxA gene (part) and locations of the primers are underlined. (B) Primer sequences targeting toxA.
PSR Assay
The PSR assay was carried out in 25-μl reaction mixtures containing the following components: 1.0 μl Bst DNA polymerase, large fragment (New England Biolabs, Ipswich, MA, USA), 2.5 μl 10× ThermoPol reaction buffer (New England Biolabs), including 20 mM Tris-HCl, 10 mM KCl, 10 mM (NH4)2SO4, 2 mM MgSO4, and 0.1% Tween 20), 0.8 M betaine (Sigma–Aldrich, Saint Louis, MO, USA), 6 mM MgSO4, 1.4 mM of each dNTP, and an appropriate amount of DNA template. The amount of primers needed for one reaction was 1.6 μM for Ft and Bt and 0.8 μM for IF and IB. At last, the reaction mixture was overlaid with a sealing agent (Patent: ZL201210371448.5 in China) to prevent cross contamination of samples by aerosol and the reactions were performed for 60 min at 65°C.
The PSR products were detected using two methods: turbidity monitoring with a real-time turbidimeter at 650 nm or direct visual detection with the aid of hydroxynaphthol blue (HNB), which is a metal ion indicator (Goto et al., 2009). For visual detection, 1 μl of HNB (Sigma–Aldrich) solution (0.2% mass fraction) was added to the reaction tube. A positive reaction is indicated by a color change from violet to sky blue, while a negative reaction remains violet. Each experiment was performed at least three times to ensure reproducibility.
PCR Assay
To compare the sensitivity of PSR and the traditional PCR assay, PCRs were setup using 0.5 μM forward primer ETA-F (5′-GACAACGCCCTCAGCATCACCAGC-3′) and backward primer ETA-B (5′-CGCTGGCCCATTCGCTCCAGCGCT-3′; Khan and Cerniglia, 1994), and the same amount of DNA template in a 25-μl reaction mixture. PCR was performed using the following cycling conditions: initial PCR activation, 95°C for 10 min; amplification, 30 cycles of 95°C for 30 s, 60°C for 30 s, and 72°C for 30 s; final extension, 72°C for 7 min. The products were separated on a 1% agarose gel, stained with GelRed (Biotium, Hayward, CA, USA), and visualized under an ultraviolet transilluminator (Bio-Rad, Berkeley, CA, USA).
Results
Temperature Optimization of the PSR Assay
Various reaction temperatures ranging from 61°C to 65°C at 1°C intervals were compared for optimal amplification. As shown in Figure 2, optimal results were obtained at 65°C. It was also the optimum temperature for enzymatic activity of the Bst DNA polymerase.
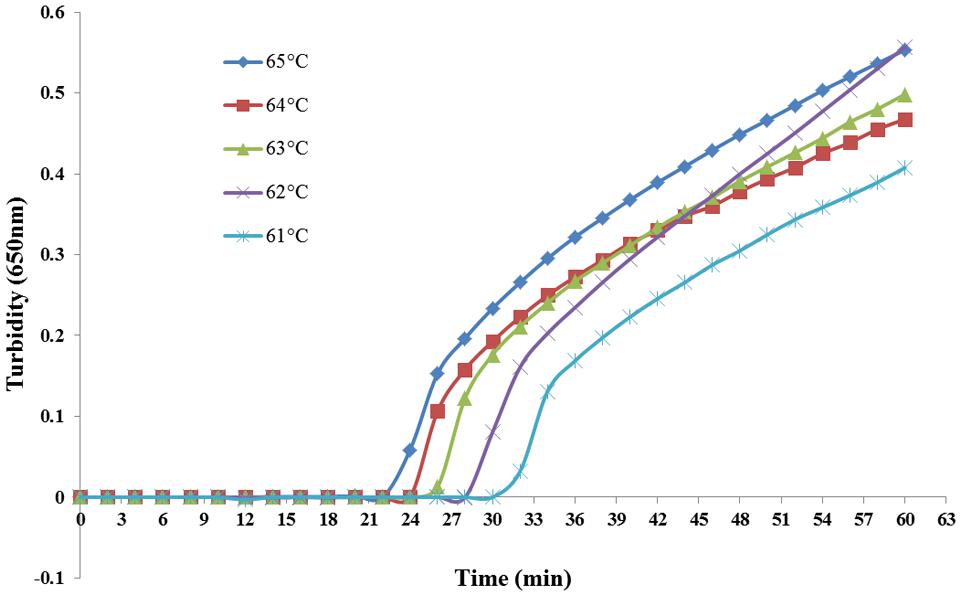
FIGURE 2. PSR assay temperature optimization. Different temperatures (61–65°C at 1°C intervals) were tested and 65°C was chosen as the optimal temperature for PSR amplification.
Specificity of the PSR Method for Detecting P. aeruginosa
To analyze the specificity of PSR detection of P. aeruginosa, genomic DNA was extracted from P. aeruginosa ATCC 15442, P. aeruginosa CMCC 10539, and 17 non- P. aeruginosa bacterial strains. Both real-time turbidity and visual detection of color change allowed correct identification of the P. aeruginosa isolates. All species homologous to P. aeruginosa and other clinical infectious or opportunistic strains, as well as the blank control, tested negative, indicating that the PSR assay is highly specific for P. aeruginosa (Figure 3).
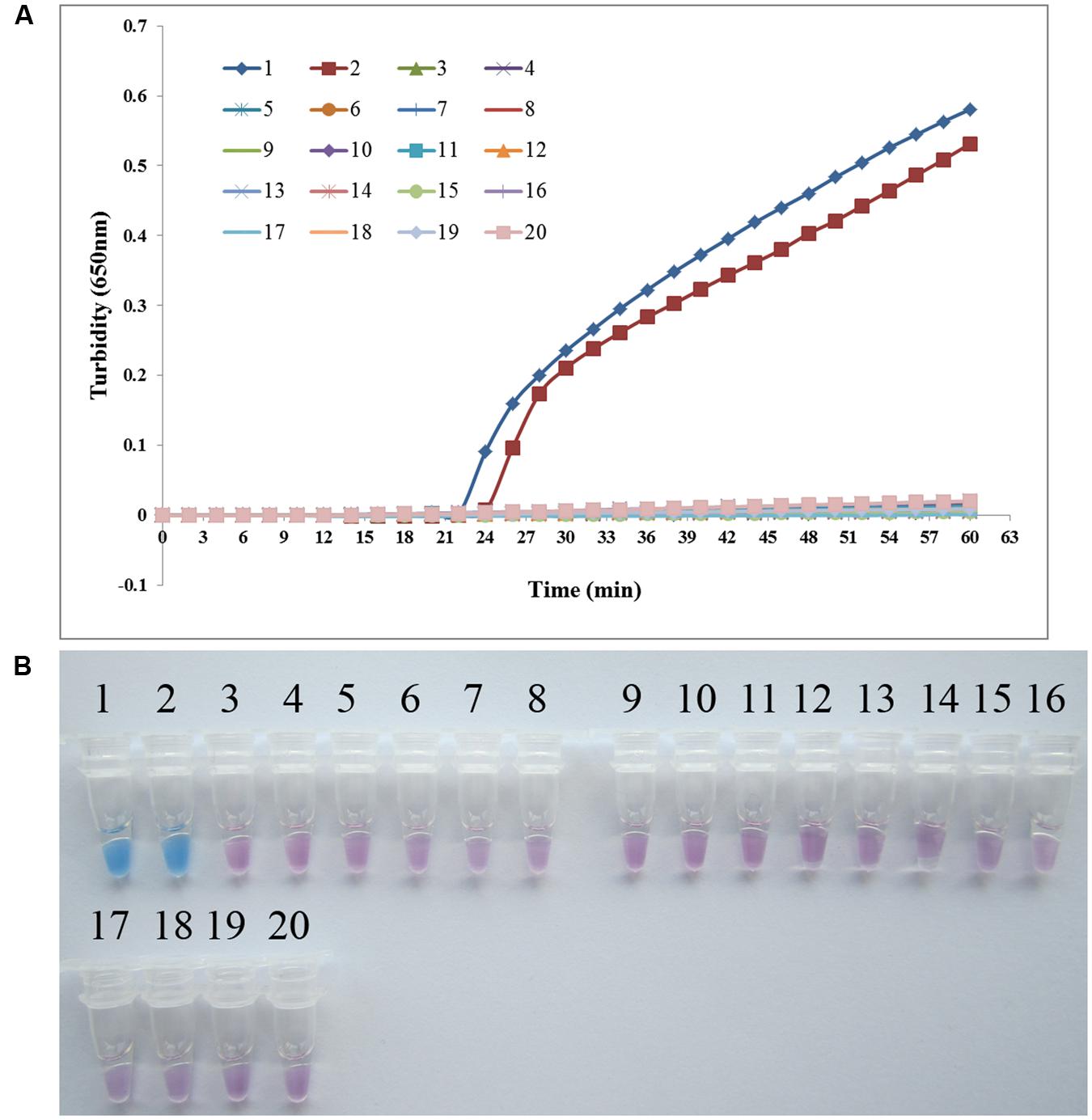
FIGURE 3. PSR assay specificity. Specificity of the PSR method for detecting Pseudomonas aeruginosa by real-time turbidimeter (A) or HNB colorimetric assay (B). Amplification was performed at 65°C for 60 min. 1, P. aeruginosa ATCC 15442; 2, P. aeruginosa CMCC 10539; 3, P. fluorescens CGMCC 1.1802; 4, Burkholderia pseudomallei 029; 5, P. geniculate CGMCC 1.871; 6, P. mendocina CGMCC 1.593; 7, P. putida 2309; 8, Klebsiella pneumoniae ATCC 2146; 9, Streptococcus pneumoniae 112-07; 10, Mycobacterium tuberculosis 005; 11, Staphylococcus aureus 2740; 12, Acinetobacter baumannii 12101; 13, Escherichia coli 44825; 14, Shigella flexneri 4536; 15, Stenotrophomonas maltophilia K279a; 16, Legionella pneumophila 9135; 17, Haemophilus influenza ATCC 49247; 18, Salmonella typhi 9275; 19, Proteus vulgaris CMCC 49027; 20, negative control (double distilled water).
Sensitivity of the PSR Assay versus PCR for P. aeruginosa Detection
To compare the detection limit of PSR using either real-time turbidity measurement or visual detection with that of conventional PCR, genomic DNA extracted from P. aeruginosa ATCC 15442 was subjected to serial 10-fold dilution (23.0 ng/μl to 0.023 pg/μl) using double-distilled water. As shown in Figure 4, the detection limit of both real-time turbidity and the visual method was 2.3 pg/μl, a 10-fold increase in sensitivity compared with conventional PCR.
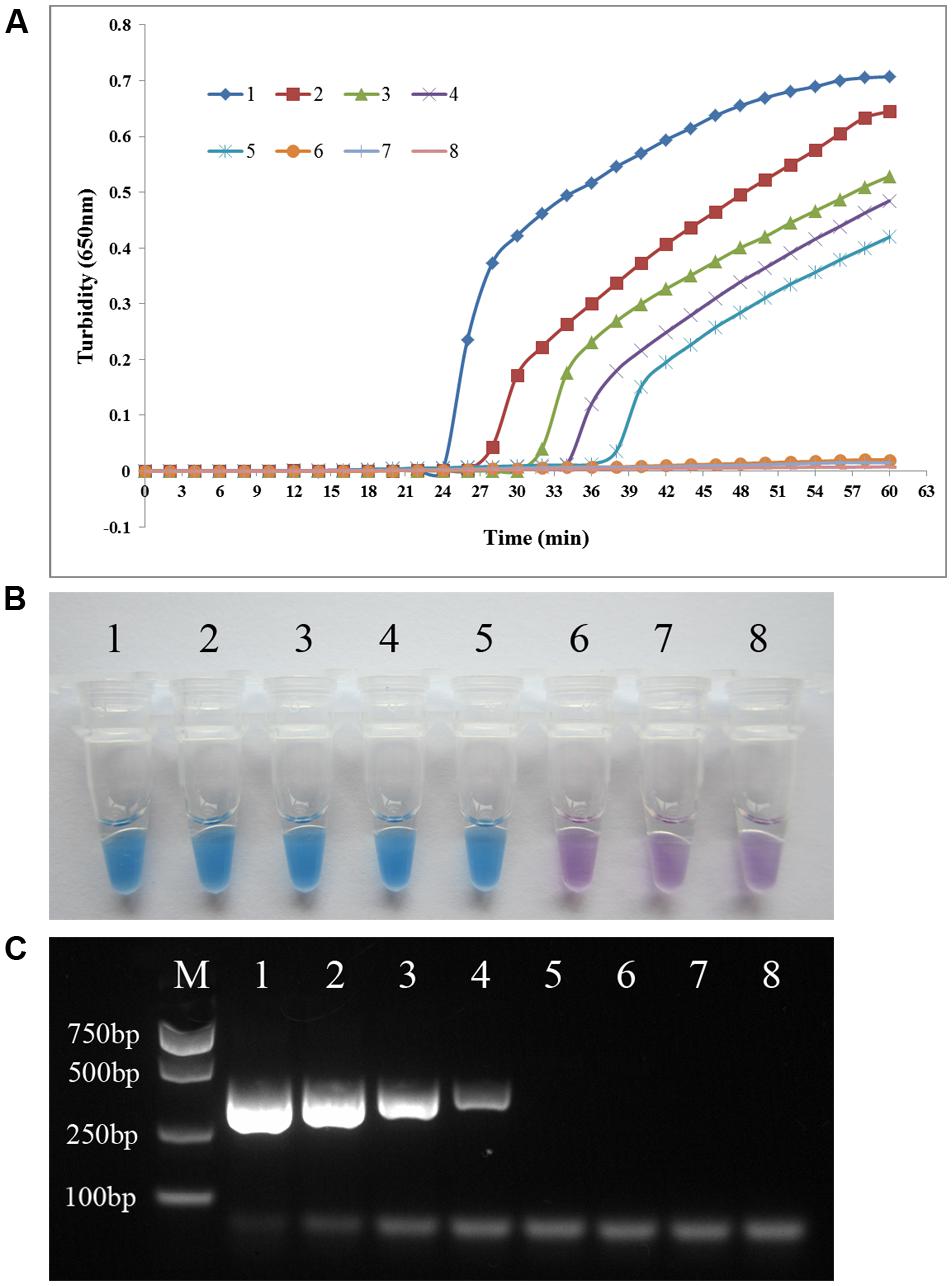
FIGURE 4. PSR assay sensitivity. Comparative sensitivities of the PSR assay (A) and (B) and traditional PCR (C) for detection of the P. aeruginosa toxA gene. 1–7: 10-fold serial dilution of genomic DNA extracted from P. aeruginosa ATCC 15442 (23.0 ng/μl to 0.023 pg/μl); 8: negative control (double-distilled water). The expected PCR product size was 396 bp.
Dissemination of P. aeruginosa in Clinic
A PSR-based surveillance of P. aeruginosa targeting the toxA gene was conducted in 301th hospital, 307th hospital, and Wujing hospital, three top hospitals of Beijing with large patient accommodations. One-hundred and thirty sputum samples were collected from ICU patients with suspected multi-resistant infections. Additionally, 10 sputum samples from healthy people were collected as controls. All clinical samples were simultaneously analyzed by visual PSR assay and traditional PCR. As shown in Figure 5, of the 130 clinical samples, 37 samples tested positive in the PSR assay while 34 were PCR-positive. All of the healthy control samples tested negative in each of the assays. Then, 37 P. aeruginosa strains were successfully cultured from the positive samples. Sequence analysis of the toxA gene from the isolated P. aeruginosa strains showed 100% identity with the nucleotide sequences reported previously (Gray et al., 1984).
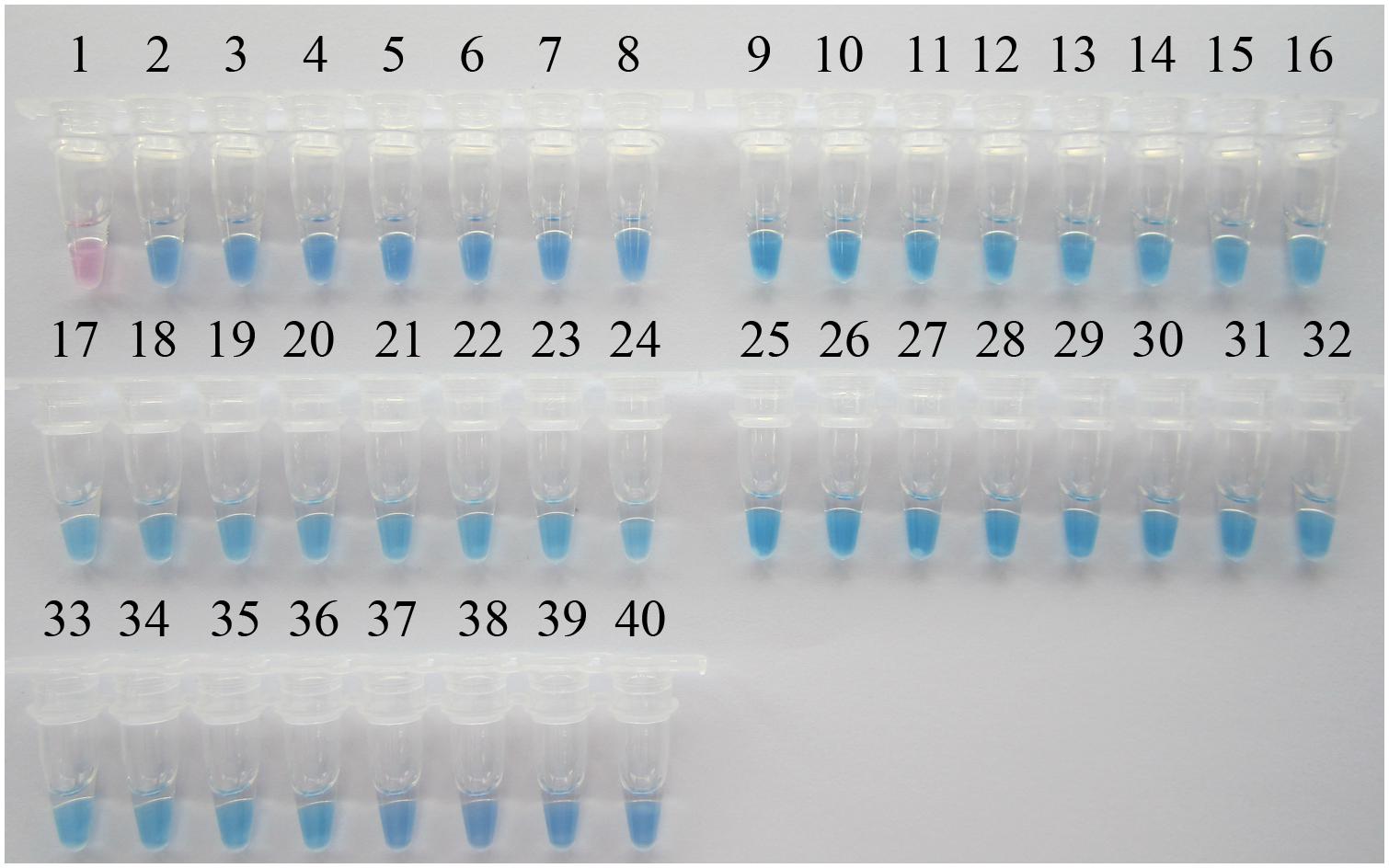
FIGURE 5. Visual PSR detection of P. aeruginosa isolates from clinical samples. The results were visualized by the addition of 1 μl HNB solution to the 25-μl reaction mix before the PSR reaction. 1, Negative control (double-distilled water); 2, positive control (P. aeruginosa ATCC 15442); 3, positive control (P. aeruginosa CMCC 10539); 4, P. aeruginosa SY-79; 5, P. aeruginosa SY-23; 6, P. aeruginosa SY-33; 7, P. aeruginosa SY-11; 8, P. aeruginosa SY-18; 9, P. aeruginosa SY-29; 10, P. aeruginosa SY-69; 11, P. aeruginosa SY-34; 12, P. aeruginosa SY-05; 13, P. aeruginosa SY-24; 14, P. aeruginosa SY-95; 15, P. aeruginosa SY-63; 16, P. aeruginosa SY-59; 17, P. aeruginosa SQ-25; 18, P. aeruginosa SQ-129; 19, P. aeruginosa SQ-09; 20, P. aeruginosa SQ-01; 21, P. aeruginosa SQ-23; 22, P. aeruginosa SQ-29; 23, P. aeruginosa SQ-37; 24, P. aeruginosa SQ-14; 25, P. aeruginosa SQ-73; 26, P. aeruginosa WJ-44; 27, P. aeruginosa WJ-66; 28, P. aeruginosa WJ-98; 29, P. aeruginosa WJ-83; 30, P. aeruginosa WJ-9; 31, P. aeruginosa WJ-26; 32, P. aeruginosa WJ-27; 33, P. aeruginosa WJ-49; 34, P. aeruginosa WJ-57; 35, P. aeruginosa WJ-41; 36, P. aeruginosa WJ-72; 37, P. aeruginosa WJ-95; 38, P. aeruginosa WJ-23; 39, P. aeruginosa WJ-01; 40, P. aeruginosa WJ-06.
Multilocus sequence typing analysis showed that the 37 P. aeruginosa strains belonged to different STs including ST187, ST235, ST244, ST357, ST441, ST986, ST597, ST1175, ST1224, and ST1752. Antimicrobial susceptibility testing revealed a high resistance rate of clinical P. aeruginosa isolates to carbapenems, cephalosporins and aminoglycosides. Only four (10.8%) strains were susceptible to imipenem. However, all clinical strains were susceptible to polymyxin B. Interestingly, mutational inactivation of oprD was found in 30 (90.9%) imipenem-resistant P. aeruginosa strains, which was consistent with a former study which claimed that reduced permeability of the outer membrane due to loss of oprD is the main cause of imipenem resistance (Wolter et al., 2004; Wang et al., 2010). To characterize the clinical P. aeruginosa isolates further, PCR screening for the presence of MBL and other β-lactamase genes (blaV IM, blaIMP, blaKPC-2, blaTEM, blaSPM, blaSIM-1, blaNDM-1, and blaOXA-50) was conducted, the results showed co-occurrence of resistance genes in most P. aeruginosa strains. Moreover, the isolate SY-95 containing multiple antibiotic resistance genes, presented increased resistance to all β-lactams (MIC > 128 μg/ml for meropenem and imipenem), quinolones, and aminoglycosides, and was only susceptible to polymyxin B.
Discussion
As a non-fermentative Gram-negative bacterium, P. aeruginosa is currently the second most prevalent nosocomial bacterium, only after Acinetobacter species. P. aeruginosa widely exists in hospital environments such as air, water distribution systems (Loveday et al., 2014), and medical equipment (Cobben et al., 1996). According to the 2012 CHINET surveillance of antimicrobial resistance in P. aeruginosa in China, 71.1% of clinical P. aeruginosa isolates came from respiratory specimens. Infections caused by P. aeruginosa are notably challenging because this organism has innate resistance to a large number of antimicrobial agents. Moreover, with the acquisition of antibiotic resistance genes (such as blaNDM-1 and blaIMP), it is becoming increasingly difficult to cure infections caused by MBL-producing P. aeruginosa (Potron et al., 2015). Thus, early diagnosis and control of this pathogen have become increasingly important.
To meet this challenge, we established a PSR method targeting the toxA gene to detect P. aeruginosa. During the PSR reaction, a large amount of insoluble magnesium pyrophosphate as well as DNA is synthesized, resulting in increased turbidity (Liu et al., 2015). PSR amplification was monitored continuously in a real-time turbidimeter instrument or visually detected with the aid of the metal ion indicator HNB, and could be completed within 60 min with a high sensitivity of 2.3 pg/μl. The toxA gene regulating the synthesis of exotoxin A was targeted in the PSR assay, which did not yield false-positive amplification of species homologous to P. aeruginosa or of other clinical pathogens. The visual detection was as sensitive and specific as real-time turbidity measurement and further simplifies the assay, as it is visible to the naked eye. In addition, since the reaction is carried out at a constant temperature, an energy-intensive thermal cycler is not needed. A thermostatic water bath is sufficient to initiate the PSR reaction. Furthermore, the Bst DNA polymerase used for PSR is not influenced by the different components often present in clinical samples. Thus, DNA purification from the sample is not necessary. It is worth mentioning that the use of a wax seal to cover the reaction mixture was essential to minimize cross contamination by aerosol since the amplification efficiency of the PSR assay is extremely high, which frequently resulted in false positives.
The P. aeruginosa PSR assay was applied to sputum samples taken from ICU patients. The test results indicated that P. aeruginosa was prevalent with nearly 30% of samples tested positive. Diverse MLSTs of P. aeruginosa and co-occurrence of resistance genes indicated a rapid and continuing evolution of P. aeruginosa resulting from their wide occurrence in clinical infections, and it would be difficult to control.
Although the antibiotic resistance rate to β-lactams, aminoglycosides, and quinolones was high, all clinical P. aeruginosa strains were sensitive to polymyxin B. Polymyxin B is a polypeptide antibiotic of which the injectable formulation has not been introduced to China because of its potential renal toxicity. Only polymyxin B unguent for scratches and mild scalds is available. However, generalized infections need to be treated with intravenous injection or infusion. Last year, a child and his grandfather were severely burned in a fire accident in Hangzhou, China. Multiple antibiotics were invalid for the treatment, and multi-drug resistant P. aeruginosa strains were isolated from their wounds. It was not until the local newspaper launched a nationwide search that the required dose of polymyxin B was collected and the two patients were cured. Therefore, we think there’s a need for an increase in the reserves of this medicine in Chinese hospitals, especially those specialized in burns, as the last resort for severe injuries.
Conclusion
We established a PSR detection method for P. aeruginosa, which meets the ASSURED criteria (affordable, sensitive, specific, user friendly, robust and rapid, equipment free and deliverable) proposed by the World Health Organization for developing diagnostic techniques (Mabey et al., 2004). We anticipate its routine use in clinical screening and on-site diagnosis, particularly in situations where resources are limited. Furthermore, our study provides new insights into the mechanisms of P. aeruginosa antibiotic resistance and warns that future therapeutic options may be seriously limited.
Conflict of Interest Statement
The authors declare that the research was conducted in the absence of any commercial or financial relationships that could be construed as a potential conflict of interest.
Acknowledgments
We are grateful to the 301th hospital, 307th hospital, and Wujing hospital located in Beijing for kindly providing P. aeruginosa strains and helpful information. This work received financial support from Mega-projects of Science and Technology Research of China (grant 2013ZX10004-203).
Supplementary Material
The Supplementary Material for this article can be found online at: http://journal.frontiersin.org/article/10.3389/fmicb.2015.01100
Footnotes
References
Aghamollaei, H., Moghaddam, M. M., Kooshki, H., Heiat, M., Mirnejad, R., and Barzi, N. S. (2015). Detection of Pseudomonas aeruginosa by a triplex polymerase chain reaction assay based on lasI/R and gyrB genes. J. Infect. Public Health 8, 314–322. doi: 10.1016/j.jiph.2015.03.003
Al-Hasan, M. N., Wilson, J. W., Lahr, B. D., Eckel-Passow, J. E., and Baddour, L. M. (2008). Incidence of Pseudomonas aeruginosa bacteremia: a population-based study. Am. J. Med. 121, 702–708. doi: 10.1016/j.amjmed.2008.03.029
Altoparlak, U., Aktas, F., Celebi, D., Ozkurt, Z., and Akcay, M. N. (2005). Prevalence of metallo-beta-lactamase among Pseudomonas aeruginosa and Acinetobacter baumannii isolated from burn wounds and in vitro activities of antibiotic combinations against these isolates. Burns 37, 707–710. doi: 10.1016/j.burns.2005.02.017
Cobben, N. A., Drent, M., Jonkers, M., Wouters, E. F., Vaneechoutte, M., and Stobberingh, E. E. (1996). Outbreak of severe Pseudomonas aeruginosa respiratory infections due to contaminated nebulizers. J. Hosp. Infect. 33, 63–70. doi: 10.1016/S0195-6701(96)90030-4
Davies, J. C. (2002). Pseudomonas aeruginosa in cystic fibrosis: pathogenesis and persistence. Paediatr. Respir. Rev. 3, 128–134. doi: 10.1016/S1526-0550(02)00003-3
Deschaght, P., Van Daele, S., De Baets, F., and Vaneechoutte, M. (2011). PCR and the detection of Pseudomonas aeruginosa in respiratory samples of CF patients. A literature review. J. Cyst. Fibros. 10, 293–297. doi: 10.1016/j.jcf.2011.05.004
Farajzadeh, S. A., Rostami, S., Jolodar, A., Tabatabaiefar, M. A., Khorvash, F., Saki, A., et al. (2014). Detection of metallo-beta lactamases among carbapenem-resistant Pseudomonas aeruginosa. Jundishapur J. Microbiol. 7:e12289. doi: 10.5812/jjm.12289
Goto, M., Honda, E., Ogura, A., Nomoto, A., and Hanaki, K. (2009). Colorimetric detection of loop-mediated isothermal amplification reaction by using hydroxy naphthol blue. Biotechniques 46, 167–172. doi: 10.2144/000113072
Gray, G. L., Smith, D. H., Baldridge, J. S., Harkins, R. N., Vasil, M. L., Chen, E. Y., et al. (1984). Cloning, nucleotide sequence, and expression in Escherichia coli of the exotoxin A structural gene of Pseudomonas aeruginosa. Proc. Natl. Acad. Sci. U.S.A. 81, 2645–2649. doi: 10.1073/pnas.81.9.2645
Hazlett, L. D. (2004). Corneal response to Pseudomonas aeruginosa infection. Prog. Retin. Eye Res. 23, 1–30. doi: 10.1016/j.preteyeres.2003.10.002
Jenkins, C. E., Swiatoniowski, A., Issekutz, A. C., and Lin, T. J. (2004). Pseudomonas aeruginosa exotoxin A induces human mast cell apoptosis by a caspase-8 and -3-dependent mechanism. J. Biol. Chem. 27, 37201–37207. doi: 10.1074/jbc.M405594200
Jin, W. Y., Jang, S. J., Lee, M. J., Park, G., Kim, M. J., Kook, J. K., et al. (2011). Evaluation of VITEK 2, MicroScan, and Phoenix for identification of clinical isolates and reference strains. Diagn. Microbiol. Infect. Dis. 70, 442–447. doi: 10.1016/j.diagmicrobio.2011.04.013
Khan, A. A., and Cerniglia, C. E. (1994). Detection of Pseudomonas aeruginosa from clinical and environmental samples by amplification of the exotoxin A gene using PCR. Appl. Environ. Microbiol. 60, 3739–3745.
Liu, W., Dong, D., Yang, Z., Zou, D., Chen, Z., Yuan, J., et al. (2015). Polymerase Spiral Reaction (PSR): a novel isothermal nucleic acid amplification method. Sci. Rep. 5:12723. doi: 10.1038/srep12723
Loveday, H. P., Wilson, J. A., Kerr, K., Pitchers, R., Walker, J. T., and Browne, J. (2014). Association between healthcare water systems and Pseudomonas aeruginosa infections: a rapid systematic review. J. Hosp. Infect. 86, 7–15. doi: 10.1016/j.jhin.2013.09.010
Mabey, D., Peeling, R. W., Ustianowski, A., and Perkins, M. D. (2004). Diagnostics for the developing world. Nat. Rev. Microbiol. 2, 231–240. doi: 10.1038/nrmicro841
Mauch, R. M., Rossi, C. L., Ribeiro, J. D., Ribeiro, A. F., Nolasco da Silva, M. T., and Levy, C. E. (2014). Assessment of IgG antibodies to Pseudomonas aeruginosa in patients with cystic fibrosis by an enzyme-linked immunosorbent assay (ELISA). Diagn. Pathol. 9 :158. doi: 10.1186/s13000-014-0158-z
Mittal, R., Aggarwal, S., Sharma, S., Chhibber, S., and Harjai, K. (2009). Urinary tract infections caused by Pseudomonas aeruginosa: a minireview. J. Infect. Public Health 2, 101–111. doi: 10.1016/j.jiph.2009.08.003
Notomi, T., Okayama, H., Masubuchi, H., Yonekawa, T., Watanabe, K., Amino, N., et al. (2000). Loop-mediated isothermal amplification of DNA. Nucleic Acids Res. 28:E63. doi: 10.1093/nar/28.12.e63
Peng, Y., Bi, J., Shi, J., Li, Y., Ye, X., Chen, X., et al. (2014). Multidrug-resistant Pseudomonas aeruginosa infections pose growing threat to health care-associated infection control in the hospitals of Southern China: a case-control surveillance study. Am. J. Infect. Control 42, 1308–1311. doi: 10.1016/j.ajic.2014.08.006
Pereira, S. G., and Cardoso, O. (2014). Mobile genetic elements of Pseudomonas aeruginosa isolates from hydrotherapy facility and respiratory infections. Clin. Microbiol. Infect. 20, 203–206. doi: 10.1111/1469-0691.12359
Poirel, L., Pitout, J. D., and Nordmann, P. (2007). Carbapenemases: molecular diversity and clinical consequences. Future Microbiol. 2, 501–512. doi: 10.2217/17460913.2.5.501
Potron, A., Poirel, L., and Nordmann, P. (2015). Emerging broad-spectrum resistance in Pseudomonas aeruginosa and Acinetobacter baumannii: mechanisms and epidemiology. Int. J. Antimicrob. Agents. 45, 568–585. doi: 10.1016/j.ijantimicag.2015.03.001
Qin, X., Emerson, J., Stapp, J., Stapp, L., Abe, P., and Burns, J. L. (2003). Use of real-time PCR with multiple targets to identify Pseudomonas aeruginosa and other nonfermenting gram-negative bacilli from patients with cystic fibrosis. J. Clin. Microbiol. 41, 4312–4317. doi: 10.1128/JCM.41.9
Vincent, M., Xu, Y., and Kong, H. (2004). Helicase-dependent isothermal DNA amplification. EMBO Rep. 5, 795–800. doi: 10.1038/sj.embor.7400200
Walker, G. T., Fraiser, M. S., Schram, J. L., Little, M. C., Nadeau, J. G., and Malinowski, D. P. (1992). Strand displacement amplification–an isothermal, in vitro DNA amplification technique. Nucleic Acids Res. 11, 1691–1696. doi: 10.1093/nar/20.7.1691
Wang, J., Zhou, J. Y., Qu, T. T., Shen, P., Wei, Z. Q., Yu, Y. S., et al. (2010). Molecular epidemiology and mechanisms of carbapenem resistance in Pseudomonas aeruginosa isolates from Chinese hospitals. Int. J. Antimicrob. Agents 35, 486–491. doi: 10.1016/j.ijantimicag.2009.12.014
Webster, T. A., Sismaet, H. J., Conte, J. L., Chan, I. P., and Goluch, E. D. (2014). Electrochemical detection of Pseudomonas aeruginosa in human fluid samples via pyocyanin. Biosens. Bioelectron. 60, 265–270. doi: 10.1016/j.bios.2014.04.028
Wolter, D. J., Hanson, N. D., and Lister, P. D. (2004). Insertional inactivation of oprD in clinical isolates of Pseudomonas aeruginosa leading to carbapenem resistance. FEMS Microbiol. Lett. 236, 137–143. doi: 10.1016/j.femsle.2004.05.039
Xu, J., Moore, J. E., Murphy, P. G., Millar, B. C., and Elborn, J. S. (2004). Early detection of Pseudomonas aeruginosa–comparison of conventional versus molecular (PCR) detection directly from adult patients with cystic fibrosis (CF). Ann. Clin. Microbiol. Antimicrob. 3:21. doi: 10.1186/1476-0711-3-21
Keywords: P. aeruginosa, PSR, toxA, rapid diagnosis, isothermal
Citation: Dong D, Zou D, Liu H, Yang Z, Huang S, Liu N, He X, Liu W and Huang L (2015) Rapid detection of Pseudomonas aeruginosa targeting the toxA gene in intensive care unit patients from Beijing, China. Front. Microbiol. 6:1100. doi: 10.3389/fmicb.2015.01100
Received: 29 July 2015; Accepted: 23 September 2015;
Published: 06 October 2015.
Edited by:
Tzi Bun Ng, The Chinese University of Hong Kong, Hong KongReviewed by:
M. Habibur Rahman, Rajshahi University, BangladeshElizabeth Fozo, University of Tennessee, USA
Copyright © 2015 Dong, Zou, Liu, Yang, Huang, Liu, He, Liu and Huang. This is an open-access article distributed under the terms of the Creative Commons Attribution License (CC BY). The use, distribution or reproduction in other forums is permitted, provided the original author(s) or licensor are credited and that the original publication in this journal is cited, in accordance with accepted academic practice. No use, distribution or reproduction is permitted which does not comply with these terms.
*Correspondence: Liuyu Huang and Wei Liu, Institute of Disease Control and Prevention, Academy of Military Medical Sciences, No. 20 Dongda Street, Fengtai District, Beijing 100071, China, aHVhbmdsaXV5dWx5QDE2My5jb20=; bGl1d2VpNTI2OUBxcS5jb20=
†These authors have contributed equally to this work.