- 1Department of Basic Veterinary Science, College of Veterinary Medicine, Northeast Agricultural University, Harbin, China
- 2Inspection and Quarantine Technical Center, Sichuan Entry-Exit Inspection and Quarantine Bureau, Chengdu, China
Objectives: The purpose of this study was to investigate the prevalence of extended spectrum β-lactamase (ESBL) genes in Escherichia coli isolated from post-weaning diarrhea (PWD) piglets in Heilongjiang province, China.
Methods: Of 458 E. coli isolated from 589 fecal samples from PWD piglets, a total of 198 isolates were confirmed as ESBL producers by the double-disk synergy test (DDST). Polymerase chain reaction (PCR) and sequencing were performed to identify genes for ESBL, plasmid-mediated quinolone resistance (PMQR), and integrons.
Results: Of the 198 isolates, blaCTX−M and blaTEM were detected in 191 and 149 isolates, respectively. Sequencing revealed that 10 blaCTX−M subtypes were detected, and blaCTX−M−14 was the most prevalent, followed by blaCTX−M−55 and blaCTX−M−65. Of the 149 TEM-positive strains, four were blaTEM−52 and the rest were blaTEM−1. Among the 198 ESBL-positive isolates, 173 isolates were found to harbor at least one PMQR gene, with oqxAB, qnrS, qnrB, qepA, and aac(6′)-Ib-cr being detected alone or in combination in 125, 114, 26, 24, and 45 strains, respectively. One hundred and fifty-five ESBL-positive isolates were also positive for class I integron (int1), and eight different gene cassette arrays were confirmed in 110 isolates by restriction fragment length polymorphism (RFLP) and DNA sequencing analyses, with predominance of dfrA17-aadA5, dfrA12-orfF-aadA2, and dfrA1-aadA1 arrays.
Conclusion: To the best of our knowledge, this is the first report of the blaTEM−52 gene in pig E. coli isolates in China and this is also the first description of the coexistence of the qnrB, qnrS, aac(6′)-Ib-cr, qepA, and oqxAB genes in one E. coli strain.
Introduction
Colibacillosis, caused by pathogenic Escherichia coli, is one of the most significant diseases in the swine industry. Diarrhea is the most common symptom of colibacillosis in pig livestock, and it is classified into two types: neonatal diarrhea (ND), which occurs in sucking piglets, and post-weaning diarrhea (PWD), which usually occurs within 2 weeks of weaning (Luppi et al., 2015). In general, ND and PWD infections can be prevented effectively by passive colostral and lactogenic immunity obtained by vaccination of the sow (Truszczynski and Pejsak, 2014). When immunity treatment fails, the antimicrobials such as cephalosporins, fluroquinolones, and co-trimoxazole are one of effective measures for the treatment of diarrhea. Accompanying the wide use of these antimicrobials in treating piglets diarrhea over the past decades, resistant isolates, especially multidrug-resistant isolates have been observed (Seiffert et al., 2013). The emergence and spread of antimicrobial resistance poses a serious challenge in controlling diarrhea in swine production.
The major mechanisms conferring resistance to cephalosporins rely on the production of extended-spectrum β-lactamases (ESBLs; Perez et al., 2007). ESBLs are a group of enzymes mediating resistance to most β-lactams approved in human and veterinary medicine, including extended-spectrum cephalosporins and monobactams but excluding carbapenems and cephamycins. These enzymes are now widely distributed worldwide in Gram-negative bacteria, which mainly exist in Enterobacteriaceae, especially in E. coli. A large surveillance of antimicrobial resistance has shown that the detection rate of ESBLs has increased significantly not only in human clinical E. coli isolates (Jones et al., 2013), but also in E. coli isolates from food-producing animals and food products, which has been raising concern about their possible transmission through the food chain. ESBLs are categorized into three types, which are TEM, SHV, and CTX-M, while the CTX-M β-lactamases have been divided into five groups known as CTX-M-1, CTX-M-2, CTX-M-8, CTX-M-9, and CTX-M-25 which have become the most prevalent in the world (Bonnet, 2004). ESBL-encoding genes are usually located on plasmids which are highly mobile and can harbor resistance genes to several other unrelated classes of antimicrobials, such as fluroquinolones, and co-trimoxazole (Wang et al., 2014).
The emergence of plasmid-mediated quinolone resistance (PMQR) has been reported since 1998, indicating that quinolone resistance can also be acquired through horizontal gene transfer (Strahilevitz et al., 2009). To date, four known PMQR determinants have been identified include Qnr proteins, aminoglycoside acetyltransferase AAC(6′)-Ib-cr, and the quinolone efflux pumps proteins QepA and OqxAB (Ruiz et al., 2012). Although the PMQR determine relatively small increases in the MICs of quinolones, these changes are sufficient to facilitate the selection of mutants with higher levels of resistance. Integrons, which are capable of capturing, excising and expressing genes cassettes that encode determinants of antimicrobial-resistance, play important roles in the horizontal dissemination of antibiotic resistance genes in bacteria (Cambray et al., 2010). Class 1 integrons were widespread in ESBL-producing isolates and play an important role in multi-drug resistance (Chen et al., 2010). As a matter of growing concern, PMQR genes and integrons have often been found to co-exist on the same plasmid with genes encoding ESBLs and to be co-transferred to recipients. Food animals colonized with ESBL-positive E. coli have been considered as potential sources of resistant E. coli causing infection in the community. E. coli antimicrobial drug resistance is strongly related to phylogenetic grouping (Barguigua et al., 2013). It has been observed that E. coli strains fall into four main phylogenetic groups (A, B1, B2, and D). Virulent extraintestinal strains belong mainly to group B2 and, to a less extent, to group D, whereas most commensal strains belong to group A and B1.
In the past few years, a rapid emergence and dissemination of ESBL-positive E. coli isolates have been increasingly reported in food animals in different countries and have gained considerable attention worldwide (Smet et al., 2010). Although there are many studies describing the molecular characteristics of ESBL genes among healthy food-producing animals, but limited data are available for the distribution and characteristics of ESBL genes in E. coli isolated from diseased animals (Liu et al., 2013a). Therefore, the main purpose of this study was to investigate the prevalence of ESBL genes in E. coli isolates collected from PWD piglets in the Heilongjiang province during 2010–2013 and assess the relatedness of PMQR genes and integrons with ESBL genes within the same strain.
Materials and Methods
Bacterial Isolates
From May 2010 to August 2013, a total of 589 fecal samples from piglets (< 2 months) with PWD were collected from 21 pig farms located in different geographic areas of Heilongjiang province, in the Northeastern China (Table 1). These fecal samples were collected from individual piglets using a sterile swab that was placed into an Eppendorf tube and transported to the laboratory within 12 h. The samples brought to the laboratory were immediately inoculated on MacConkey agar, and five randomly selected colonies with typical E. coli morphology were selected from each sample. The bacterial strains were identified using classing biochemical methods and confirmed as E. coli using the API-20E Bacterial Identification System (bioMérieux, France). All confirmed E. coli isolates were stored in Luria-Bertani (LB) broth, containing 20% glycerol at −80°C for further studies.
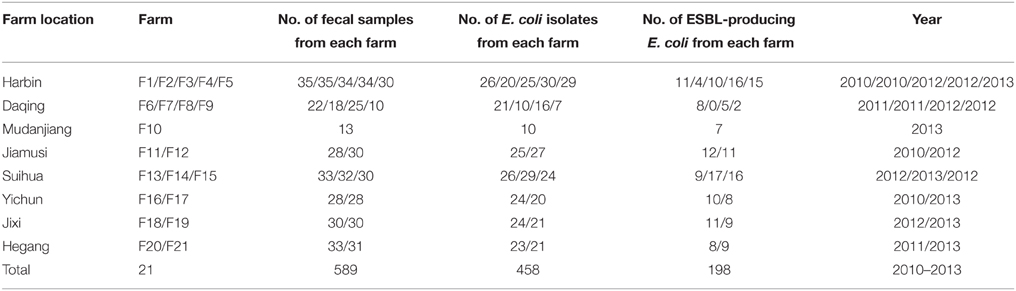
Table 1. Total number of fecal samples collected from piglets with post-weaning diarrhea on different farms in Heilongjiang province.
Antimicrobial Susceptibility Testing and ESBL Determination
The minimum inhibitory concentrations (MICs) of ampicillin, ceftiofur, cefotaxime, amikacin, gentamicin, tetracycline, chloramphenicol, florfenicol, nalidixic acid, ciprofloxacin, enrofloxacin, trimethoprim/sulfamethoxazole, and colistin were determined by the agar dilution method according to the guidelines of the Clinical and Laboratory Standards Institute (CLSI; Clinical Laboratory Standards Institute, 2012). The antimicrobial concentrations were from 0.06 to 256 μg/ml. E. coli ATCC25922 was used as the quality control strain. All E. coli isolates were screened for the production of ESBLs by the double-disk synergy test (DDST) using both cefotaxime and ceftazidime, alone and in combination with clavulanic acid as recommended by the CLSI. The DDST was considered positive when the inhibition zone produced by the combined effects of either ceftazidime or cefotaxime plus clavulanic acid was ≥5 mm larger than that produced by either ceftazidime or cefotaxime alone.
Detection of ESBL and PMQR Genes
Plasmid DNA of E. coli isolates was obtained with the AxyPrep Plasmid Miniprep Kit (Axygen Scientific, California) following the manufacturer's instructions. All ESBL-positive E. coli isolates were screened by polymerase chain reaction (PCR) for the following ESBL genes: blaTEM, blaSHV, blaCTX−M−1 group, blaCTX−M−2 group, blaCTX−M−8 group, blaCTX−M−9 group, and blaCTX−M−25 group (Luo et al., 2011). The ESBL-producing E. coli isolates were also screened for the presence of PMQR genes: qnrA, qnrB, qnrC, qnrD, qnrS, qepA, oqxAB, and aac(6′)-Ib-cr (Robicsek et al., 2005; Gay et al., 2006; Park et al., 2006; Cavaco et al., 2009; Wang et al., 2009). The primer sequences and corresponding annealing temperature used in the PCR reactions are listed in Table 2. PCR was performed in 25 μl mixture containing 2 μl template DNA, 1 μl of each primer (10 nmol/L), 12.5 μl 2 × PCRMix (Haigene, China), 8.5 μl ddH2O. All PCRs were done as follows: 5 min at 94°C; followed by 35 cycles of 30 s at 94°C, 30 s at annealing temperature, 45 s at 72°C; and 7 min at 72°C. All positive PCR products were sequenced using an ABI3730 sequencer (Applied Biosystems, Life Technologies, Foster City, CA, USA) and their sequences were compared with genes in the GenBank using the BLAST program (http://www.ncbi.nlm.nih.gov/BLAST/). All aac(6′)-Ib-positive isolates were further analyzed by direct sequencing of the purified PCR products to identify aac(6′)-Ib-cr.
Integrons Analysis and Characterization of Inserted Gene Cassettes
PCR was performed to check the presence of class 1, 2, and 3 integrons in all 198 ESBL-positive E. coli isolates using primers specific for the integron integrase genes intI1, intI2, and intI3 listed in Table 2 (Dillon et al., 2005). Strains that was positive for intI1 gene were subsequently subjected to PCR for amplification of the variable region of class 1 integrons using primer listed in Table 2 (White et al., 2000). The PCR products of class 1 integrons variable regions with identical amplicon lengths were analyzed by restriction fragment length polymorphism (RFLP) using HinfI (TaKaRa, Japan) according to the method of previous study (Gu et al., 2008). PCR products with identical RFLP profiles were regarded as the same gene cassette array. PCR products with different gene cassette arrays were sequenced and analyzed.
Phylogenetic Genotyping
Phylogenetic grouping of the E. coli isolates was determined using a triplex PCR for the genes chuA, yjaA, and TspE4C2 as described previously (Clermont et al., 2000).
Statistical Analysis
Frequency of antimicrobial resistance profiles between ESBL-positive and ESBL-negative E. coli isolates were compared using the Pearson Chi-square test with the software SPSS 17.0, and the level of significance was set at p < 0.05.
Results
Bacterial Strains and Antimicrobial Susceptibility Testing
A total of 458 E. coli isolates were recovered from the 589 fecal samples (Table 1). Of which, 43.2% (n = 198) were suspected to be ESBL producers using DDST. The results of the in vitro antimicrobial susceptibility testing of all E. coli isolates are shown in Table 3. High resistance rates (>90%) were discovered among both ESBL-positive and ESBL-negative E. coli isolates for ampicillin, trimethoprim-sulphamethoxazole, nalidixic acid, and tetracycline. Interestingly, ESBL-positive isolates demonstrated remarkably higher resistance rates to ampicillin, ceftiofur, cefotaxime, amikacin, gentamicin, florfenicol, enrofloxacin, and ciprofloxacin than ESBL-negative isolates (p < 0.05). All of the isolates were resistant to at least one antimicrobial agents tested and 96.1% (n = 440) of the isolates were resistant to three or more classes of antimicrobial thus defined as multi-drug resistant (MDR). With regard to MDR profiles, all isolates grouped in to 65 resistance phenotypes, 92.8% isolates were resistant to more than five, 62.9% were resistant to more than 9, and 34 (7.4%) were resistant to 12 antimicrobial agents. The predominant multi-resistance profile was ampicillin/tetracycline/chloramphenicol/nalidixic acid/enrofloxacin/trimethoprim-sulphamethoxazole, which accounted for 39.1% (179/458) of all the isolates.
Characterization of ESBL and PMQR Genes
PCR was performed on 198 ESBL-positive E. coli to determine the presence of ESBL and PMQR genes. 98.5% (195/198) of the ESBL-positive E. coli isolates harbored one or more of the genes from the three families of TEM, SHV, and CTX-M, whereas the remaining three isolates failed to show the presence of such genes, suggesting that these three isolates perhaps carry other ESBL genes, which will require further studies. The distribution of ESBL genotype among the 198 ESBL-positive E. coli isolates was shown in Table 4. 96.5% (191/198) of the isolates were detected to harbor one or two blaCTX−M genes, and blaCTX−M−1 or blaCTX−M−9 positive groups were detected at 36.7% (70/191) and 60.2% (115/191), respectively; The blaCTX−M−1 and blaCTX−M−9 double-positive group accounted for 3.1% (6/191). None of the isolates contained the blaCTX−M−2, blaCTX−M−8, and blaCTX−M−25 genes. In the blaCTX−M−1 group, blaCTX−M−55, and blaCTX−M−15 were the major genotypes, accounting for 52.6% (40/76) and 28.9% (22/76), respectively; the remaining genotypes included blaCTX−M−3 (4), blaCTX−M−64 (4), and blaCTX−M−123 (6). In the blaCTX−M−9 group, blaCTX−M−14 was the major genotype, accounting for 58.7% (71/121); the remaining included blaCTX−M−27 (15), blaCTX−M−65 (28), blaCTX−M−104 (4), and blaCTX−M−125 (3). More than one type of CTX-M gene was identified in six isolates and their combinations were CTX-M-14 and CTX-M-3 in one isolate, CTX-M-14 and CTX-M-55 in three isolates, and CTX-M-14 and CTX-M-15 in two isolates. 75.3% (149/198) isolates were found to be positive for blaTEM, except four isolates were found to be blaTEM−52 by sequencing, the other 145 isolates were positive for blaTEM−1, and all of the blaTEM-positive isolates simultaneously carried blaCTX−M genes. None of the isolates contained blaSHV gene.
The distribution of PMQR-encoding genes among the 198 ESBL-positive E. coli isolates was shown in Table 5. 87.4% (173/198) of the ESBL-producing E. coli isolates were found to harbor at least one PMQR gene, with oqxAB, qnrS, qnrB, qepA, and aac(6′)-Ib-cr being detected alone or in combination in 125 (63.1%), 114 (57.6%), 26 (13.1%), 24 (12.1%), and 45 (22.7%) strains, respectively. qnrA, qnrC, and qnrD were not found in this study. oqxAB and qnrS were the two most common PMQR genes. Among the 173 PMQR-positive isolates, 105 (60.7%) isolates were positive for more than one PMQR genes, and combinations of oqxAB+qnrS (n = 42) was the most common combination type, followed by oqxAB+qnrS+aac(6′)-Ib-cr (n = 16). Twelve other combination types of PMQR were also found in this study. Sixty-three isolates had two co-existing PMQR genes, 29 isolates harbored three PMQR genes, nine isolates harbored four PMQR genes, and three isolates harbored five PMQR genes. It is interesting to note that oqxAB, qnrB, qnrS, qepA, and aac(6′)-Ib-cr coexisted in three isolates. To our knowledge this is the first time to report one isolate carried six PMQR genes. Among the 125 oqxAB-positive isolates, 52 and 24 isolates harbored blaCTX−M−14 and blaCTX−M−55 genes, respectively. In the 110 qnrS positive isolates, 37 and 30 isolates harbored blaCTX−M−14 and blaCTX−M−55 genes, respectively. qnrS, oqxAB, and qnrS+oqxAB were also detected in three non-ESBL genes isolates respectively.
Integrons and Gene Cassettes
The class 1 integron integrase gene intI1 was detected in 78.3% (155/198) ESBL-positive E. coli isolates. intI2 and intI3 genes were not detected in any of the isolates. The variable regions of class 1 integron was amplified using primers complementary to the conserved regions flanking the inserted resistance genes. One hundred and ten of the 155 intI1-positive E. coli isolates were positive for the variable region and the inserted gene cassette sizes varied in size from 0.75 to 1.9 kb. BLAST analysis of sequenced products of variable region of class 1 demonstrated the presence of eight different cassette combinations for 10 different genes. These genes encode for the resistance to trimethoprim (dfrA1, dfrA5, dfrA7, dfrA12, dfrA17, and dfrA27) and aminoglycosides (aadA1, aadA2, aadA5, and aad22). Out of 110 intI1-positive isolates 57 carried dfrA17-aadA5 gene cassette; 28 harbored dfrA12-orfF-aadA2 gene cassette; 12 harbored dfrA1-aadA1 gene cassette. The sequences of the dfrA1-aadA1, dfrA17-aadA5, and dfrA12-orfF-aadA2 gene cassettes have been deposited in the GenBank database under the accession numbers KT692980, KT692981, and KT692982, respectively. The distribution of integron among ESBL-positive E. coli was shown in Table 6.
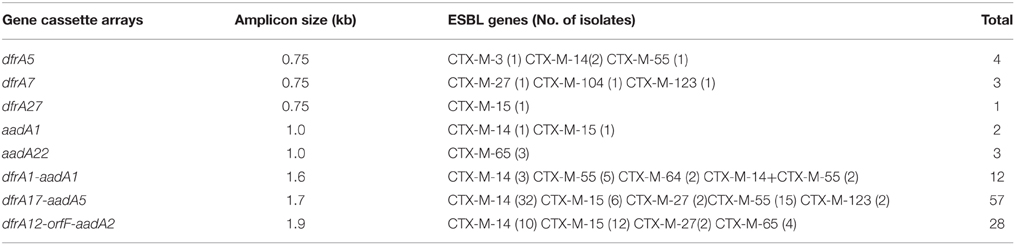
Table 6. Distribution of gene cassette arrays found in class 1 integrons among ESBL-positive E. coli isolates.
Phylogenetic Groups
All ESBL-positive isolates were allocated to one of the four phylogenetic groups A, B1, B2, and D. The results showed that 53.5% (106/198) of these isolates belonged to group A, 24.2% (48/198) to group D, and 21.2% (42/198) to group B1. Only two strains were found in group B2.
Discussion
Over the past few years, with the development of an intensive and large-scale pig industry in China, the occurrence and epidemic of porcine diseases have taken on a lot of new characteristics (Liu et al., 2014). In particular, PWD caused by pathogenic E. coli is one of the most serious threat for the swine industry. During PWD outbreaks in pig farms, it was certainly thought that antimicrobial therapy would be an important control measure (Amezcua et al., 2002). However, commonly used animal antimicrobial agents did not appear to be effective for curing PWD (Moredo et al., 2015). Disturbingly, only third-generation cephalosporins such as ceftiofur and cefquinome are effective for controlling PWD (Hornish and Kotarski, 2002). The frequent use of third-generation cephalosporins as therapy or feed additives in pigs has selected high antimicrobial resistance in bacteria, which is becoming a serious issues in pig industry (Lei et al., 2010). All E. coli isolates in the current study were tested for their susceptibility to 13 antimicrobial agents. Similar to previous studies (Smith et al., 2010), we found a very high frequency of resistance to most of the conventional antimicrobial agents such as gentamicin, tetracycline, chloramphenicol, and trimethoprim-sulphamethoxazole normally used to treat PWD infections (Luppi et al., 2015). Certainly, these antimicrobial agents could not be the good choice to treat piglets with PWD in these farms. The remarkable finding in the present study is that 92.8% isolates were resistant to more than five antimicrobial agents, and 62.9% isolates were resistant to more than nine antimicrobial agents, which is much higher than our previous studies (Zhang et al., 2009). However, all isolates remained susceptible to colistin, which has been shown to be a safe and effective candidate to treat infections caused by E. coli in China pig farms (Wu et al., 2012). Comparing the frequency of resistance to these 13 antimicrobial agents, higher resistance rates were present in ESBL-positive strains than in negative ones, which suggested the former may acquire resistance easier than the latter.
The prevalence of ESBL-positive E. coli isolates in food-producing animals has been increasing worldwide (Liebana et al., 2013). In this study, 43.2% (198/458) of the E. coli isolates screened from PWD piglets were ESBL producers, which is higher than the detection rates (12.6%, 41/326) in E. coli from healthy pigs (P < 0.001; Zheng et al., 2012). These results suggest that ESBLs are more frequently present in isolates from sick animals compared to commensal E. coli from the healthy food-producing animals. The high prevalence rate of ESBL-positive E. coli in diarrhea piglets may be explained by the fact that the third-generation cephalosporins are used more commonly in swine for treatment of ND and PWD diseases (Lei et al., 2010; Barton, 2014). Nevertheless, this constitutes a potential public health concern since the recovered sick animals carrying ESBL resistant gene may enter the food chain. The inappropriate use and/or overuse of third-generation cephalosporins has been associated with the emergence and spread of ESBL-positive E. coli in pigs (Cavaco et al., 2008). For that reason, in many countries, third-generation cephalosporins have been forbidden for use in pig production, as a result of which, the occurrence of ESBL-positive E. coli has been significantly reduced (Agersø and Aarestrup, 2013). In china, however, third-generation cephalosporins are still approved for food-producing animals with no limitation.
CTX-M-type ESBLs have been isolated increasingly from human, companion, and food-producing animals E. coli isolates in different countries in the last several years (Zhao and Hu, 2013). A 2003–2012 survey in China showed that CTX-M-type ESBLs accounted for 87.1% of ESBL-positive E. coli strains isolated from food animals (Rao et al., 2014). CTX-M-14 remains the most abundant genotype, although the detection rate of CTX-M-55 and CTX-M-65 have shown a continuously increasing trend in recent years (Sun et al., 2010; Zheng et al., 2012). Similar findings were obtained in the present study, the proportion of CTX-M-producing E. coli had increased to 96.5%, and CTX-M-14 remained the most common genotype of ESBLs, followed by CTX-M-55 and CTX-M-65. In addition, we also found the blaCTX−M−3, blaCTX−M−15, blaCTX−M−27, blaCTX−M−64, blaCTX−M−65, blaCTX−M−104, blaCTX−M−123, and blaCTX−M−125 genes in these isolates, indicating a high diversity of blaCTX−M genes in E. coli isolates from PWD piglets in Heilongjiang province. In the past, blaTEM−52 has only been detected in E. coli isolates from chicken in China (Li et al., 2010), but now it has been found in four E. coli isolates from piglets of different farms. To our knowledge, this is the first report of blaTEM−52 in E. coli from pigs in China.
Emergence of PMQR gene has been reported worldwide and is being documented in ESBL producers in recent days (Poirel et al., 2012). In the present study, we found that the detection rate of PMQR genes was 87.4% among a collection of 198 ESBL-positive E. coli isolates. Somewhat similar finding has also been reported in previous study in China, where 83.8% (160/191) of ESBL-positive E. coli from swine contained the PMQR genes (Liu et al., 2013b). However, the detection rate of PMQR among ESBL-positive E. coli in other countries was low, with 50% of the E. coli isolates from human in Tunisian and 13.6% E. coli isolates from human in Mexico (Silva-Sánchez et al., 2013; Ferjani et al., 2015). Over the past 10 years, PMQR determinants have emerged as an important issue. Their detection among ESBL-positive E. coli from human and food-producing animals has been widely investigated and different rates have been reported depending on the country, origin of isolates, and the number of E. coli included (Seiffert et al., 2013). The widespread dissemination of PMQR determinants may lead to the rapid development of fluoroquinolone resistance because they can confer low-level resistance to fluoroquinolone and promote the selection of high-level resistant strains with mutations on the chromosome. In the present study, 52 of the 125 oqxAB positive strains possessed blaCTX−M−14, and a significant association between the blaCTX−M−14 and oqxAB genes have been found, which validated the finding further that isolates with the oqxAB gene often possess a blaCTX−M−14-producing plasmid (Liu et al., 2013b). This suggests that spread of the oqxAB gene might occur concurrently with the blaCTX−M−14 gene. Notably, the blaCTX−M−55, qnrB, qnrS, aac(6′)-Ib-cr, qepA, and oqxAB genes were found to be carried together in three isolates. Only a few previous studies have found bacteria harboring more than three PMQR genes (Yang et al., 2014). Whether multiple PMQR determinants have an additive effect on the MIC of fluoroquinolones is unknown and warrants further investigation.
Integrons are important genetic elements responsible for carriage and spread of antibiotic resistant determinants including ESBL enzymes. ESBL genes located on integrons-like structures are being increasingly reported worldwide (Bonnet, 2004). Hence, we examined the presence of class 1, class 2, and class 3 integrons. In this study, 78.3% (155/198) of ESBL-positive E. coli isolates harbored class 1 integron while none of the isolates contained class 2 and class 3 integron as found in previous studies (Sun et al., 2013). Sequence analysis of the amplified variable region of class 1 integron revealed that most of the integrons possessed dfrA and aadA gene cassettes conferring resistance to trimethoprim and streptomycin as reported by several researchers previously (Kar et al., 2015). This kind of gene cassette arrangement may be responsible for emergence of multiple drug resistance among ESBL producers isolated in this study. However, further study is needed to investigate the association between class 1 integron production and dissemination of ESBL type genes in E. coli from China.
Several authors have analyzed the distribution of the main phylogenetic groups among E. coli strains isolated from human and animals. We showed that E. coli clinical isolates from PWD piglets carrying ESBLs, collected over 3 years, belonged mainly to phylogroup A and, to a lesser extent, to phylogroups D and B1, whereas B2 strains were very little. This is in agreement with other studies on pathogenic E. coli of pig origin (Wang et al., 2010; Abraham et al., 2014).
In our study we have detected a high prevalence of ESBL-positive E. coli isolated from PWD piglets. Future studies will be performed with the ESBL-positive isolates to find possible association among serotypes, virulence factors, resistance mechanisms, and transmission mechanisms that will be useful for epidemiological research.
In conclusion, in this study a high prevalence of ESBL-positive E. coli has been observed in fecal samples of PWD piglets collected from Heilongjiang province, and the high prevalence of PMQR genes and integrons were also widely detected among the ESBL-positive E. coli strains. The blaCTX−M−14 was found to be the dominant ESBL-encoding gene in this study, while oqxAB and qnrS were the dominant PMQR genes in the ESBL-positive E. coli. This is the first report of TEM-52 ESBL in bacterial isolates from pig in China. To our knowledge, this is also the first description of the coexistence of the qnrB, qnrS, aac(6′)-Ib-cr, qepA, and oqxAB genes in one E. coli strain. More studies should be carried out in the future in order to ensure that the six PMQR genes were located on the same plasmid or not.
Conflict of Interest Statement
The authors declare that the research was conducted in the absence of any commercial or financial relationships that could be construed as a potential conflict of interest.
Acknowledgments
This study was supported by the Harbin Science and Technology Innovation Fund (2013RFXXJ035).
References
Abraham, S., Trott, D. J., Jordan, D., Gordon, D. M., Groves, M. D., Fairbrother, J. M., et al. (2014). Phylogenetic and molecular insights into the evolution of multidrug-resistant porcine enterotoxigenic Escherichia coli in Australia. Int. J. Antimicrob. Agents 44, 105–111. doi: 10.1016/j.ijantimicag.2014.04.011
Agersø, Y., and Aarestrup, F. M. (2013). Voluntary ban on cephalosporin use in Danish pig production has effectively reduced extended-spectrum cephalosporinase-producing Escherichia coli in slaughter pigs. J. Antimicrob. Chemother. 68, 569–572. doi: 10.1093/jac/dks427
Amezcua, R., Friendship, R. M., Dewey, C. E., Gyles, C., and Fairbrother, J. M. (2002). Presentation of postweaning Escherichia coli diarrhea in southern Ontario, prevalence of hemolytic E. coli serogroups involved, and their antimicrobial resistance patterns. Can. J. Vet. Res. 66, 73–78.
Barguigua, A., Otmani, F. E., Talmi, M., Zerouali, K., and Timinouni, M. (2013). Prevalence and types of extended spectrum β-lactamases among urinary Escherichia coli isolates in Moroccan community. Microb. Pathog. 61–62, 16–22. doi: 10.1016/j.micpath.2013.04.010
Barton, M. D. (2014). Impact of antibiotic use in the swine industry. Curr. Opin. Microbiol. 19, 9–15. doi: 10.1016/j.mib.2014.05.017
Bonnet, R. (2004). Growing group of extended-spectrum beta-lactamases: the CTX-M enzymes. Antimicrob. Agents Chemother. 48, 1–14. doi: 10.1128/AAC.48.1.1-14.2004
Cambray, G., Guerout, A. M., and Mazel, D. (2010). Integrons. Annu. Rev. Genet. 44, 141–166. doi: 10.1146/annurev-genet-102209-163504
Cavaco, L. M., Abatih, E., Aarestrup, F. M., and Guardabassi, L. (2008). Selection and persistence of CTX-M-producing Escherichia coli in the intestinal flora of pigs treated with amoxicillin, ceftiofur, or cefquinome. Antimicrob. Agents Chemother. 52, 3612–3616. doi: 10.1128/AAC.00354-08
Cavaco, L. M., Hasman, H., Xia, S., and Aarestrup, F. M. (2009). qnrD, a novel gene conferring transferable quinolone resistance in Salmonella enterica serovar Kentucky and Bovismorbificans strains of human origin. Antimicrob. Agents Chemother. 53, 603–608. doi: 10.1128/AAC.00997-08
Chen, H., Shu, W., Chang, X., Chen, J. A., Guo, Y., and Tan, Y. (2010). The profile of antibiotics resistance and integrons of extended-spectrum beta-lactamase producing thermotolerant coliforms isolated from the Yangtze River basin in Chongqing. Environ. Pollut. 158, 2459–2464. doi: 10.1016/j.envpol.2010.03.023
Clermont, O., Bonacorsi, S., and Bingen, E. (2000). Rapid and simple determination of the Escherichia coli phylogenetic group. Appl. Environ. Microbiol. 66, 4555–4558. doi: 10.1128/AEM.66.10.4555-4558.2000
Clinical Laboratory Standards Institute. (2012). Performance Standards for Antimicrobial Susceptibility Testing: Twenty-Second Informational Supplement M100-S22, Vol. 32. Wayne, PA: National Committee for Clinical Laboratory Standards.
Dillon, B., Thomas, L., Mohmand, G., Zelynski, A., and Iredell, J. (2005). Multiplex PCR for screening of integrons in bacterial lysates. J. Microbiol. Methods 62, 221–232. doi: 10.1016/j.mimet.2005.02.007
Ferjani, S., Saidani, M., Amine, F. S., and Boutiba-Ben Boubaker, I. (2015). Prevalence and characterization of plasmid-mediated quinolone resistance genes in extended-spectrum β-lactamase-producing Enterobacteriaceae in a Tunisian hospital. Microb. Drug Resist. 21, 158–166. doi: 10.1089/mdr.2014.0053
Gay, K., Robicsek, A., Strahilevitz, J., Park, C. H., Jacoby, G., Barrett, T. J., et al. (2006). Plasmid-mediated quinolone resistance in non-Typhi serotypes of Salmonella enterica. Clin. Infect. Dis. 43, 297–304. doi: 10.1086/505397
Gu, B., Pan, S., Wang, T., Zhao, W., Mei, Y., Huang, P., et al. (2008). Novel cassette arrays of integrons in clinical strains of Enterobacteriaceae in China. Int. J. Antimicrob. Agents 32, 529–533. doi: 10.1016/j.ijantimicag.2008.06.019
Hornish, R. E., and Kotarski, S. F. (2002). Cephalosporins in veterinary medicine - ceftiofur use in food animals. Curr. Top. Med. Chem. 2, 717–731. doi: 10.2174/1568026023393679
Jones, R. N., Castanheira, M., Hu, B. J., Ni, Y. X., Lin, S. S. F., Mendes, R. E., et al. (2013). Update of contemporary antimicrobial resistance rates across China: reference testing results for 12 medical centers (2011). Diagn. Microbiol. Infect. Dis. 77, 258–266. doi: 10.1016/j.diagmicrobio.2013.07.003
Kar, D., Bandyopadhyay, S., Bhattacharyya, D., Samanta, I., Mahanti, A., Nanda, P. K., et al. (2015). Molecular and phylogenetic characterization of multidrug resistant extended spectrum beta-lactamase producing Escherichia coli isolated from poultry and cattle in Odisha, India. Infect. Genet. Evol. 29, 82–90. doi: 10.1016/j.meegid.2014.11.003
Lei, T., Tian, W., He, L., Huang, X. H., Sun, Y. X., Deng, Y. T., et al. (2010). Antimicrobial resistance in Escherichia coli isolates from food animals, animal food products and companion animals in China. Vet. Microbiol. 146, 85–89. doi: 10.1016/j.vetmic.2010.04.025
Li, L., Jiang, Z. G., Xia, L. N., Shen, J. Z., Dai, L., Wang, Y., et al. (2010). Characterization of antimicrobial resistance and molecular determinants of beta-lactamase in Escherichia coli isolated from chickens in China during 1970-2007. Vet. Microbiol. 144, 505–510. doi: 10.1016/j.vetmic.2010.02.005
Liebana, E., Carattoli, A., Coque, T. M., Hasman, H., Maqiorakos, A. P., Mevius, D., et al. (2013). Public health risks of enterobacterial isolates producing extended-spectrum β-lactamases or AmpC β-lactamases in food and food-producing animals: an EU perspective of epidemiology, analytical methods, risk factors, and control options. Clin. Infect. Dis. 56, 1030–1037. doi: 10.1093/cid/cis1043
Liu, B. T., Liao, X. P., Yue, L., Chen, X. Y., Li, L., Yang, S. S., et al. (2013a). Prevalence of β-lactamase and 16S rRNA methylase genes among clinical Escherichia coli isolates carrying plasmid-mediated quinolone resistance genes from animals. Microb. Drug Resist. 19, 237–245. doi: 10.1089/mdr.2012.0179
Liu, B. T., Yang, Q. E., Li, L., Sun, J., Liao, X. P., Fang, L. X., et al. (2013b). Dissemination and characterization of plasmids carrying oqxAB-blaCTX−M genes in Escherichia coli isolates from food-producing animals. PLoS ONE 8:e73947. doi: 10.1371/journal.pone.0073947
Liu, W. X., Yuan, C. W., Meng, X. Q., Du, Y. C., Gao, R. Z., Tang, J., et al. (2014). Frequency of virulence factors in Escherichia coli isolated from suckling pigs with diarrhoea in China. Vet. J. 199, 286–289. doi: 10.1016/j.tvjl.2013.11.019
Luo, Y., Yang, J., Zhang, Y., Ye, L., Wang, L., and Guo, L. (2011). Prevalence of β-lactamases and 16S rRNA methylase genes amongst clinical Klebsiella pneumoniae isolates carrying plasmid-mediated quinolone resistance determinants. Int. J. Antimicrob. Agents 37, 352–355. doi: 10.1016/j.ijantimicag.2010.12.018
Luppi, A., Bonilauri, P., Dottori, M., Gherpelli, Y., Biasi, G., Merialdi, G., et al. (2015). Antimicrobial resistance of F4+ Escherichia coli isolated from swine in Italy. Transbound. Emerg. Dis. 62, 67–71. doi: 10.1111/tbed.12081
Moredo, F. A., Piñeyro, P. E., Márquez, G. C., Sanz, M., Colello, R., Etcheverría, A., et al. (2015). Enterotoxigenic Escherichia coli subclinical infection in pigs: bacteriological and genotypic characterization and antimicrobial resistance profiles. Foodborne Pathog. Dis. 12, 704–711. doi: 10.1089/fpd.2015.1959
Park, C. H., Robicsek, A., Jacoby, G. A., Sahm, D., and Hooper, D. C. (2006). Prevalence in the United States of aac(6′)-Ib-cr encoding a ciprofloxacin-modifying enzyme. Antimicrob. Agents Chemother. 50, 3953–3955. doi: 10.1128/AAC.00915-06
Perez, F., Endimiani, A., Hujer, K. M., and Bonomo, R. A. (2007). The continuing challenge of ESBLs. Curr. Opin. Pharmacol. 7, 459–469. doi: 10.1016/j.coph.2007.08.003
Poirel, L., Cattoir, V., and Nordmann, P. (2012). Plasmid-mediated quinolone resistance; interactions between human, animal, and environmental ecologies. Front. Microbiol. 3:24. doi: 10.3389/fmicb.2012.00024
Rao, L., Lv, L., Zeng, Z., Chen, S., He, D., Chen, X., et al. (2014). Increasing prevalence of extended-spectrum cephalosporin-resistant Escherichia coli in food animals and the diversity of CTX-M genotypes during 2003-2012. Vet. Microbiol. 172, 534–541. doi: 10.1016/j.vetmic.2014.06.013
Robicsek, A., Sahm, D. F., Strahilevitz, J., Jacoby, G. A., and Hooper, D. C. (2005). Broader distribution of plasmid-mediated quinolone resistance in the United States. Antimicrob. Agents Chemother. 49, 3001–3003. doi: 10.1128/AAC.49.7.3001-3003.2005
Ruiz, J., Pons, M. J., and Gomes, C. (2012). Transferable mechanisms of quinolone resistance. Int. J. Antimicrob. Agents 40, 196–203. doi: 10.1016/j.ijantimicag.2012.02.011
Seiffert, S. N., Hilty, M., Perreten, V., and Endimiani, A. (2013). Extended-spectrum cephalosporin-resistant Gram-negative organisms in livestock: an emerging problem for human health? Drug Resist. Updat. 16, 22–45. doi: 10.1016/j.drup.2012.12.001
Silva-Sánchez, J., Cruz-Trujillo, E., Barrios, H., Reyna-Flores, F., Sánchez-Pérez, A., Bacterial Resistance, C., et al. (2013). Characterization of plasmid-mediated quinolone resistance (PMQR) genes in extended-spectrum β-lactamase-producing Enterobacteriaceae pediatric clinical isolates in Mexico. PLoS ONE 8:e77968. doi: 10.1371/journal.pone.0077968
Smet, A., Martel, A., Persoons, D., Dewulf, J., Heyndrickx, M., Herman, L., et al. (2010). Broad-spectrum beta-lactamases among Enterobacteriaceae of animal origin: molecular aspects, mobility and impact on public health. FEMS Microbiol. Rev. 34, 295–316. doi: 10.1111/j.1574-6976.2009.00198.x
Smith, M. G., Jordan, D., Chapman, T. A., Chin, J. J. C., Barton, M. D., Do, T. N., et al. (2010). Antimicrobial resistance and virulence gene profiles in multi-drug resistant enterotoxigenic Escherichia coli isolated from pigs with post-weaning diarrhoea. Vet. Microbiol. 145, 299–307. doi: 10.1016/j.vetmic.2010.04.004
Strahilevitz, J., Jacoby, G. A., Hooper, D. C., and Robicsek, A. (2009). Plasmid-mediated quinolone resistance: a multifaceted threat. Clin. Microbiol. Rev. 22, 664–689. doi: 10.1128/CMR.00016-09
Sun, J., Zheng, F., Wang, F., Wu, K., Wang, Q., Chen, Q., et al. (2013). Class 1 integrons in urinary isolates of extended-spectrum β-lactamase-producing Escherichia coli and Klebsiella pneumoniae in Southern China during the past five years. Microb. Drug Resist. 19, 289–294. doi: 10.1089/mdr.2012.0130
Sun, Y., Zeng, Z., Chen, S., Ma, J., He, L., Liu, Y., et al. (2010). High prevalence of bla(CTX-M) extended-spectrum β-lactamase genes in Escherichia coli isolates from pets and emergence of CTX-M-64 in China. Clin. Microbiol. Infect. 16, 1475–1481. doi: 10.1111/j.1469-0691.2010.03127.x
Truszczynski, M., and Pejsak, Z. (2014). Vaccines against porcine colibacillosis, particularly after weaning. Med. Weter. 70, 3–6.
Wang, J., Stephan, R., Power, K., Yan, Q., Hächler, H., and Fanning, S. (2014). Nucleotide sequences of 16 transmissible plasmids identified in nine multidrug-resistant Escherichia coli isolates expressing an ESBL phenotype isolated from food-producing animals and healthy humans. J. Antimicrob. Chemother. 69, 2658–2668. doi: 10.1093/jac/dku206
Wang, M., Guo, Q., Xu, X., Wang, X., Ye, X., Wu, S., et al. (2009). New plasmid-mediated quinolone resistance gene, qnrC, found in a clinical isolate of Proteus mirabilis. Antimicrob. Agents Chemother. 53, 1892–1897. doi: 10.1128/AAC.01400-08
Wang, X. M., Jiang, H. X., Liao, X. P., Liu, J. H., Zhang, W. J., Zhang, H., et al. (2010). Antimicrobial resistance, virulence genes, and phylogenetic background in Escherichia coli isolates from diseased pigs. FEMS Microbiol. Lett. 306, 15–21. doi: 10.1111/j.1574-6968.2010.01917.x
White, P. A., McIver, C. J., Deng, Y., and Rawlinson, W. D. (2000). Characterisation of two new gene cassettes, aadA5 and dfrA17. FEMS Microbiol. Lett. 182, 265–269. doi: 10.1111/j.1574-6968.2000.tb08906.x
Wu, S., Zhang, F., Huang, Z., Liu, H., Xie, C., Zhang, J., et al. (2012). Effects of the antimicrobial peptide cecropin AD on performance and intestinal health in weaned piglets challenged with Escherichia coli. Peptides 35, 225–230. doi: 10.1016/j.peptides.2012.03.030
Yang, T., Zeng, Z., Rao, L., Chen, X., He, D., Lv, L., et al. (2014). The association between occurrence of plasmid-mediated quinolone resistance and ciprofloxacin resistance in Escherichia coli isolates of different origins. Vet. Microbiol. 170, 89–96. doi: 10.1016/j.vetmic.2014.01.019
Zhang, X. Y., Ding, L. J., and Yue, J. (2009). Occurrence and characteristics of class 1 and class 2 integrons in resistant Escherichia coli isolates from animals and farm workers in northeastern China. Microb. Drug Resist. 15, 323–328. doi: 10.1089/mdr.2009.0020
Zhao, W. H., and Hu, Z. Q. (2013). Epidemiology and genetics of CTX-M extended-spectrum β-lactamases in Gram-negative bacteria. Crit. Rev. Microbiol. 39, 79–101. doi: 10.3109/1040841X.2012.691460
Keywords: post-weaning diarrhea, ESBL, PMQR, integron, TEM-52
Citation: Xu G, An W, Wang H and Zhang X (2015) Prevalence and characteristics of extended-spectrum β-lactamase genes in Escherichia coli isolated from piglets with post-weaning diarrhea in Heilongjiang province, China. Front. Microbiol. 6:1103. doi: 10.3389/fmicb.2015.01103
Received: 27 June 2015; Accepted: 24 September 2015;
Published: 08 October 2015.
Edited by:
Teresa M. Coque, Hospital Universitario Ramón y Cajal, SpainReviewed by:
Daniela Ceccarelli, University of Maryland, USAAshima Kushwaha Bhardwaj, Indian Institute of Advanced Research, India
Copyright © 2015 Xu, An, Wang and Zhang. This is an open-access article distributed under the terms of the Creative Commons Attribution License (CC BY). The use, distribution or reproduction in other forums is permitted, provided the original author(s) or licensor are credited and that the original publication in this journal is cited, in accordance with accepted academic practice. No use, distribution or reproduction is permitted which does not comply with these terms.
*Correspondence: Xiuying Zhang, emhhbmd4aXV5aW5nMDQ1MUAxMjYuY29t