- 1Department of Clinical Laboratory Medicine, Taizhou Municipal Hospital, Medical College of Taizhou University and the Institute of Molecular Diagnostics of Taizhou University, Zhejiang, China
- 2Department of Infectious Disease, Wenling Hospital, Wenzhou Medical University, Zhejiang, China
- 3Department of Laboratory Medicine, Medical College of Taizhou University and the Institute of Molecular Diagnostics of Taizhou University, Zhejiang, China
- 4Department of Hepatobiliary Surgery, Taizhou Municipal Hospital, Medical College of Taizhou University, Zhejiang, China
- 5Department of Neurology, Taizhou Municipal Hospital, Medical College of Taizhou University, Zhejiang, China
- 6Department of Quality Control, Taizhou Municipal Hospital, Medical College of Taizhou University, Zhejiang, China
QepA is one of the genes that confer quinolone resistance in bacteria. The aim of this study was to analyze the genetic structures of plasmids that carry a qepA3, a recently discovered allele of qepA in Enterobacteriaceae clinical isolates. 656 non-redundant Enterobacteriaceae clinical isolates were screened for the qepA3 gene and five isolates were identified to carry the gene. Plasmids were isolated from these isolates and were found to increase antibiotic resistance once the plasmids were transferred to Escherichia coli. These plasmids were subcloned and sequenced to analyze the genetic structures surrounding the qepA3 gene. The results showed that the five plasmids had different genetic structures; two of the qepA3-containning isolates had either the blaCTX-M-14 or blaTEM-12 gene instead of the blaTEM-1 gene. The structures of both pKP3764 and pECL3786 have not been previously described. In comparison with pHPA, there were a number of changes in DNA sequences up- and down-stream of the qepA3 gene. These findings provide better understanding of the genetic variations in qepA3 and would be useful for diagnosis and control of quinolone resistance in clinical settings.
Introduction
According to past work (Cattoir et al., 2008), three mechanisms have been described for plasmid- mediated quinolone resistance: qnr determinants (Mammeri et al., 2005; Nordmann and Poirel, 2005; Robicsek et al., 2006; Wang et al., 2011), aminoglycoside acetyltransferase aac(6′)- Ib-cr (Robicsek et al., 2006), and the qepA and oqxAB efflux pump genes, which confer decreased susceptibility to quinolones (Périchon et al., 2007; Yamane et al., 2007; Wong et al., 2015). The qepA1 gene was first investigated in 2007 by two groups from Japan and Belgium (Périchon et al., 2007; Yamane et al., 2007). In 2008, the qepA2 was discovered by a French research group (Cattoir et al., 2008). Currently, both the qepA1 and qepA2 genes have been reported worldwide (Liu et al., 2008; Park et al., 2009; Guillard et al., 2011; Ruiz et al., 2012; Chen et al., 2014). Recently, we identified a new qepA allele qepA3 from in a Chinese patient (GenBank with accession number JQ064560). Although the human qepA has a fairly low prevalence in Korea (Kim et al., 2009; Park et al., 2009), it is commonly found in Enterobacteriaceae isolates from food- producing animals in China (Liu et al., 2008; Ma et al., 2009; Chen et al., 2014). Therefore, more surveillance is needed for Enterobacteriaceae harboring the qnr, aac(6′)-Ib-cr and qepA.
In this study, we investigated the qepA gene in 656 Enterobacteriaceae isolates from hospitalized patients and only 13 isolates were found positive. This result confirm that qepA has low prevalence (1.98%) (0.91% for qepA1, 0.31% for qepA2 and 0.76% for qepA3) in patients of our area. However, five isolates were determined to harbor novel qepA3 structures. These structure were characterized to provide better understanding of the gene for potential management of plasmid-mediated antibiotic resistance.
Materials and Methods
Bacterial Strains and Luria-Bertani (LB) Agar Plates
Enterobacteriaceae isolates EC3157, EC3587, CD4359, KP3764, and ECL3786 were identified using the Vitek 2 system (bioMérieux, France) according to the manufacturer’s instructions. Escherichia coli isolates EC3157 and EC3587 were isolated from the blood and sputum samples of ICU inpatients, respectively. CD4359, an isolate of Citrobacter koseri, was obtained from the sputum of an inpatient in Infection Unit, while KP3764, an isolate of Klebsiella pneumoniae, was from a blood sample of an ICU inpatient. ECL3786, an isolate of Enterobacter cloacae, was from the chest wound secretions of a cardiothoracic surgical inpatient. E. coli JM109 was used as the host for cloning and an azide-resistant E. coli (strain J53) was used as the recipient strain for conjugation experiments. The LB1 agar medium for plasmid transformation contained 100 μL of isopropyl β-D-1-thiogalactopyranoside (IPTG, 24 mg/mL), 200 μL of 5-bromo-4-chloro- 3-indolyl β-D-galactopyranoside (X-Gal, 20 mg/mL) and 100 μL of ampicillin (Amp, 100 mg/mL) in 100 mL medium. LB2 agar plates were used for conjugation experiments and were supplemented with sodium azide (150 μg/mL) and ciprofloxacin (0.25 μg/mL).
Susceptibility Testing
The minimum inhibitory concentrations (MICs) of eight antimicrobial agents (nalidixic acid, ofloxacin, ciprofloxacin, cefotaxime, ceftazidime, amikacin, and gentamicin) were determined using the MicroScan microdilution panel (Scott, USA) broth dilution method. E. coli ATCC 25922 and Pseudomonas aeruginosa ATCC 27853 were used as the controls. The results were interpreted according to the CLSI guidelines (Clinical and Laboratory Standards Institute, 2014).
Plasmid Isolation and Sequence Analysis
Bacterial plasmid DNA was extracted using a plasmid extraction kit (TaKaRa, Japan) according to the manufacturer’s instructions. PCR amplifications were preformed using primers based on the pHPA (Table 1, Yamane et al., 2007). PCR was run for 3 min at 94°C followed by 30 cycles of 1 min of denaturing at 94°C and annealing at 56.9°C, with a final elongation of 10 min at 72°C on Life Veriti® PCR machine (Invitrogen, USA). The total reaction volume was 20 μl containing 4 μL 5X PCR buffer, 0.4 μL of 10 mM dNTPs, 1 μL each of 10 μM primers and 0.2 μL Polymerase, with nuclease-free water filled up to 20 μL, and bacterial plasmids harboring the qepA gene as template. The amplicons were digested with DraI and BamHI (TaKaRa, Japan), ligated to linearized pMD19-T (TaKaRa, Japan), and transformed into E. coli JM109 competent cells. Plasmids DNA from Amp resistant colonies recovered on LB1 plate were sequenced.
Conjugation
The five isolates were conjugated with E. coli J53 as described previously (Wang et al., 2003). In the conjugation experiments, the isolates were used as donors and azide-resistant E. coli J53 as the recipient strain by filter mating. Transconjugants were selected on the LB2 agar plates. MICs for the donors, transconjugants, and recipients were also measured as described above.
Results
Plasmid Isolation and Characterization
Plasmids were isolated from the five Enterobacteriaceae isolates and separated on 0.7% agarose gel by gel electrophoresis. Results showed that plasmids pKP3764, pECL3876, pEC3157, pEC3587, and pCD4359 from isolates KP3764, pECL3876, EC3157, EC3587, and CD4359 were estimated to be about 53 kb, 72 kb, 145, 152, and 168 kb, respectively (Figure 1A).
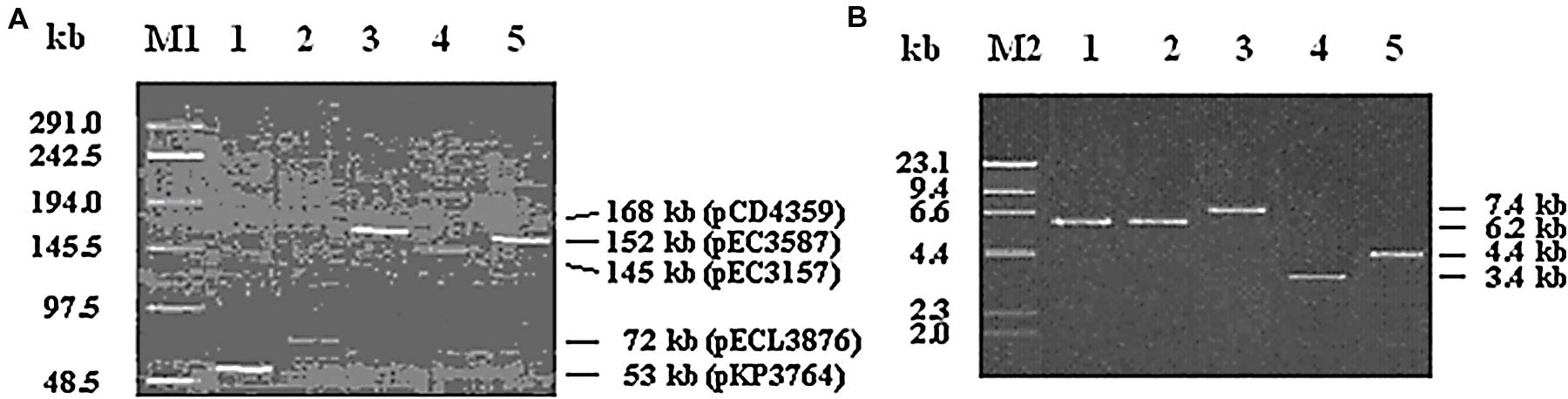
FIGURE 1. Electrophoresis of plasmids isolated from clinical isolates and the recombinant plasmids used for sequence analysis. The left columns indicate the DNA markers while the right column denote the plasmid sizes. (A) Lane M1, a λ DNA marker (Amersham Biosciences, USA); Lanes 1–5, plasmids pKP3764, pECL3876, pEC3157, pEC3587, and pCD4359. (B) Lane M2, λ HindIII DNA marker (Amersham Biosciences, USA); Lanes 1–5, recombinant plasmids pECD2, pECD4, pCDD2, pKPD1, and pECLD1.
The qep A-containing sequences in the five strains were amplified, digested with DraI and/or BamHI and subcloned in pMD19-T to generate recombinant plasmids pECD1, pECD2, pECD3, pECD4, pCDD2, pKPD1, and pECLD1 (Figure 1B). The inserts in these plasmids were sequenced and analyzed for qep A and its flanking structures (GenBank accession numbers KR259130, KR259131, KR259132, KR259133, and KR259134, Supplementary Material) (Figure 2). The results showed that in addition to qepA3, which was present in all plasmids, the inserts from pEC3157 and pCD4359 contained the blaCTX-M-14 and rmtB genes; the inserts from pEC3587 had the blaTEM-12 gene; inserts from all plasmids except pCD4359 had the truncated dfr2 gene; the inserts from pCD4539 contained the blaTEM-1 and rmtB genes; the insert from pKPD1 and pECLD1 contained truncated dfr2 gene. No other resistant genes were found in these sequences.
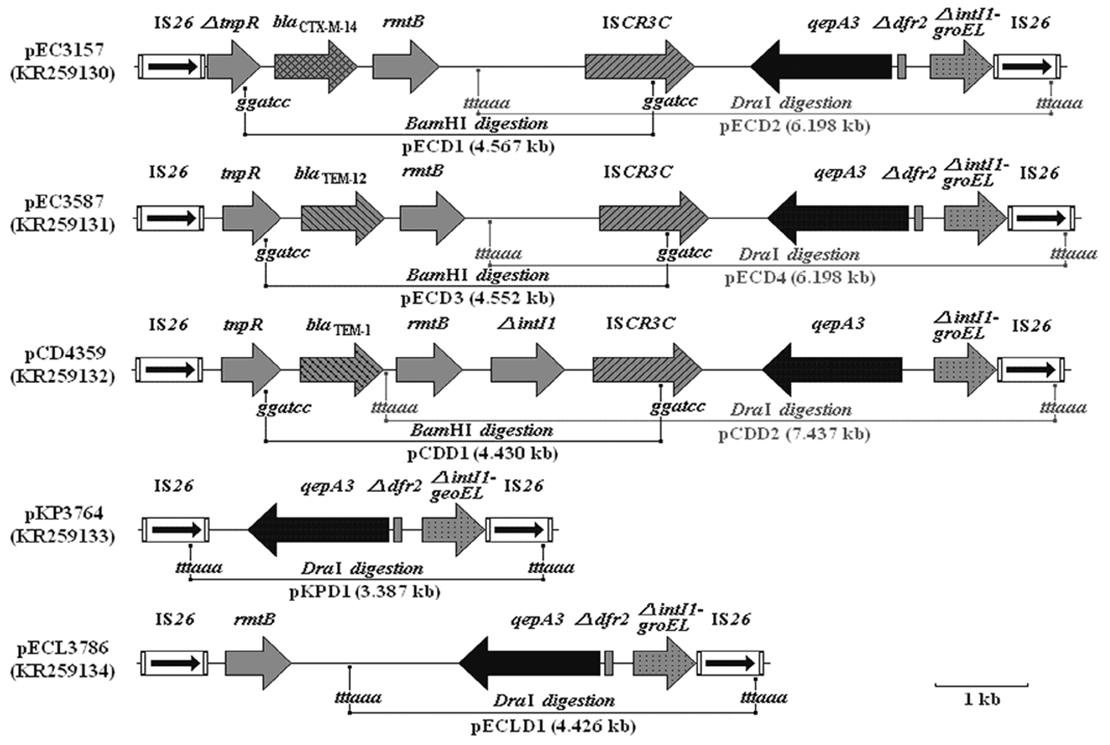
FIGURE 2. Genetic structures surrounding the qepA3 gene in pEC3157, pEC3587, pCD4359, pKP3764, and pECL3786. The numbers in parentheses are GenBank accession numbers; “ggatcc” and “tttaaa” indicate restriction sites; Δ (delta) denotes truncated gene; ΔintI1-groEL denotes the truncated intI1 and groEL fusion gene. The figures in the parentheses following the names of the plasmids denote the plasmid size.
Antibiotic Susceptibility
Through conjugation, the plasmids from the five isolates (donors) were transferred to E. coli J53 (recipients). The MIC values for cefotaxime and ceftazidime in the transconjugants with the isolates EC3157, EC3587, and CD4359 were much higher than those of the recipient E. coli J53 (Table 2). Similarly, the MIC values for nalidixic acid, norfloxacin, ofloxacin, and ciprofloxacin in the transconjugants with the five isolates were generally higher than those of E. coli J53 (Table 2). Contrastingly, the MIC values nalidixic acid, ofloxacin, ciprofloxacin, amikacin, and gentamicin in the transconjugant involving the EC3157, EC3587, CD4357, and ECL3786 were generally higher than those of E. coli J53 (recipient) (Table 2). These results demonstrated that the antibiotic resistant genes are located in the plasmids.
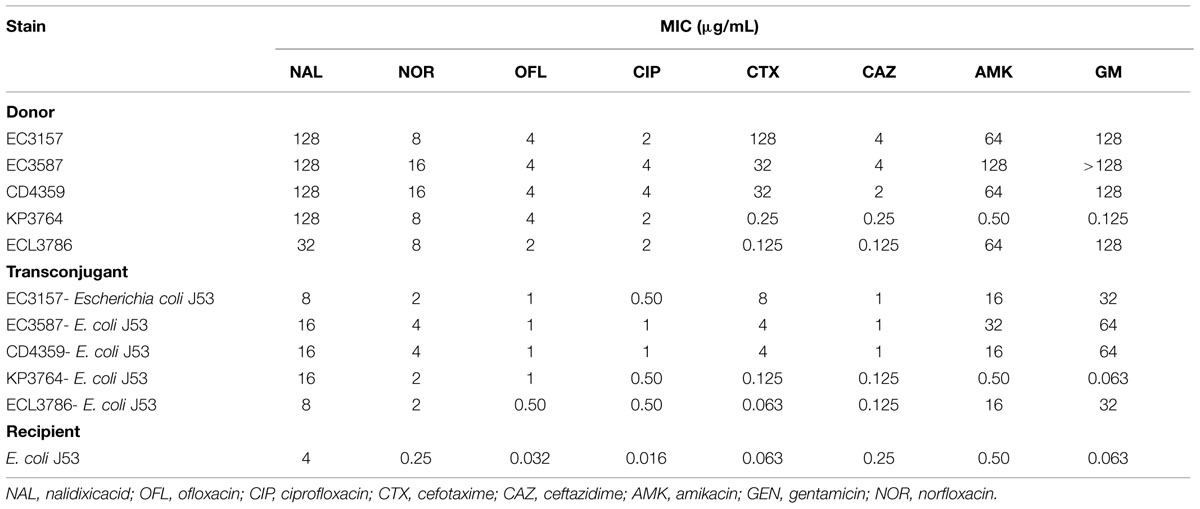
TABLE 2. Minimum inhibitory concentrations of antimicrobial agents for donors, transconjugants, and their recipients.
Discussion
Previous studies have shown that quinolone and fluoroquinolone resistance genes in E. coli and Klebsiella isolates are located on plasmids, which often carry other antimicrobial resistant genes, and can be transferred to other strains by conjugation or transformation (Ruiz et al., 2012). Since the discovery of qepA1 and qepA2 in 2007 and 2008, the genetic environment and location of the qepA genes in the plasmid pHPA have both been well-established (Périchon et al., 2007; Yamane et al., 2007; Cattoir et al., 2008). In this study, five Enterobacteriaceae isolates carrying the qepA3 and surrounding genes were investigated, and are found to have different genetic structures (Figure 2) surrounding qepA3.
The plasmid pCD4359 from isolate CD4359 showed a similar structure to pHPA, except for the qepA allele and a truncated dfr2, a gene closely related to vertebrate FGF-receptor. The plasmids pEC3587 and pEC3157 were different from pHPA, where blaTEM-1 in pHPA was replaced by blaTEM-12 or blaCTX-M-14, respectively (Figure 2). blaCTX-M-14,blaTEM-1, and blaTEM-12 code β-lactamase and are important determinants of drug resistance. In addition, in pCD4359, there is an additional ΔintI1 downstream of the rmtB which confers high-level resistance to all aminoglycoside and missing Δdfr2. The sequence between IS26 and tnpR was also truncated. Furthermore, rmtB or ISCR3C are not linked with qepA3 directly in pKP3764 as reported previously (Périchon et al., 2007; Yamane et al., 2007; Cattoir et al., 2008; Kim et al., 2009; Rocha-Gracia et al., 2010; Cao et al., 2014).
In pEC3587, the blaTEM-12 gene took the place of blaTEM-1 and had a truncated dfr2 gene as compared with pHPA. These results are also different from previous reports (Périchon et al., 2007; Yamane et al., 2007; Cattoir et al., 2008; Kim et al., 2009; Rocha-Gracia et al., 2010; Cao et al., 2014). Surprisingly, in pKP3764 there are three genes qepA3, truncated dfr2, and truncated intI1-groEL fusion gene between the two IS26 insertions, while in pKP3764 and pECL3786, therefore are four genes: rmtB, qepA3, truncated dfr2, and truncated intI1-groEL fusion genes. To date, these genetic structures have not been identified in previous studies (Kim et al., 2009; Rocha-Gracia et al., 2010; Cao et al., 2014). Moreover, pKP3764 is the only plasmid that does not contain the rmtB gene among the five plasmids studied in this study. According to a previous report, 58.3% (28/48) of rmtB-positive E. coli isolates harbored the qepA gene (Grape et al., 2005). Whether or not these results suggest a strong link between qepA and rmtB remains to be investigated. In these five genetic structures (Figure 2), the qepA3 gene and its downstream genes were nearly all identical except for the truncated dfr2 deletion in pCD4359. After pEC3157 and pEC3587 were digested by the DraI, the size of both products were identical at approximate 6.2 kb. It should be noted that it is also possible that the ISCR3C is not a stable link between ISCR3C and the qepA gene.
QepA gene is a quinolone pump gene that confers resistance to nalidixic acid and norfloxacin. Stains carrying the gene may be resistant or sensitive to ofloxacin and ciprofloxacin with increased MIC. In the conjugation experiments, we found that E. coli cells with pEC3587 and pCD4359 were resistant to penicillins and aminoglycoside antibiotics drugs such as amikacin, gentamicin, gentamicin, and tobramycin. They were tolerant or sensitive to ofloxacin and ciprofloxacin, but the MICs were increased. These results indicate that there might be synergistic effect against antibiotics when the gepA, blaCTX-M 14, and rmtB are present in the same plasmids, leading to multidrug resistance.
Taken together, our works suggest that there are sequence variations surrounding the qepA3 even if a limited number of isolates are analyzed, and it is likely that more variations would exist that may impact the resistance profiles, and subsequently the clinical implications of the bacteria. More studies are needed to link these structure variation to resistance profiles and potential clinical outcomes.
Conclusion
The novel genetic structures surrounding the qepA3 gene have been discovered in the isolates obtained from five patients in China although the prevalence for the qepA allele in hospital patients are low. One of the isolates is linked to the non-rmtB- or non- ISCR3C- producing genetic structure in the qepA3 genetic environment. Moreover, the blaCTX-M-14 or blaTEM-12 genes are found to be associated with the qepA3 gene in these structures instead of the blaTEM-1 gene. These results provide new insight into the variation in genetic environments of the qepA3 gene and would be useful for further investigation of the clinical implications in antibiotic resistance management.
Conflict of Interest Statement
The authors declare that the research was conducted in the absence of any commercial or financial relationships that could be construed as a potential conflict of interest.
Acknowledgments
We thank Professor George A. Jacoby from the Lahey Clinic (Massachusetts, USA) for designating the qepA3 gene. This study was supported by grants from the Department of Science and Technology of Zhejiang Province (2014C33153), the Zhejiang Health Department (2014KYB313), and the Taizhou Science and Technology Bureau (1301KY36, 1201KY22, 102KY15, and 081KY30).
Supplementary Material
The Supplementary Material for this article can be found online at: http://journal.frontiersin.org/article/10.3389/fmicb.2015.01147
References
Cao, X., Xu, X., Zhang, Z., Shen, H., Chen, J., and Zhang, K. (2014). Molecular characterization of clinical multidrug-resistant Klebsiella pneumoniae isolates. Ann. Clin. Microbiol. Antimicrob. 13:16. doi: 10.1186/1476-0711-13-16
Cattoir, V., Poirel, L., and Nordmann, P. (2008). Plasmid-mediated quinolone resistance pump qepA2 in an Escherichia coli isolate from France. J. Antimicrob. Chemother. 52, 3801–3804. doi: 10.1016/j.diagmicrobio.2009.07.006
Chen, X., He, L., Li, Y., Zeng, Z., Deng, Y., Liu, Y., et al. (2014). Complete sequence of a F2: A-: B- plasmid pHN3A11 carrying rmtB and qepA, and its dissemination in China. Vet. Microbiol. 174, 267–271. doi: 10.1016/j.vetmic.2014.08.023
Clinical and Laboratory Standards Institute (2014). Performance Standards for Antimicrobial Susceptibility Testing; Twenty-Four Informational Supplement. M100–S24. Wayne, PA: Clinical and Laboratory Standards Institute.
Grape, M., Farra, A., Kronvall, G., and Sundstrëm, L. (2005). Integrons and gene cassettes in clinical isolates of co-trimoxazole-resistant gram-negative bacteria. Clin. Microbiol. Infect. 11, 185–192. doi: 10.1111/j.1469-0691.2004.01059.x
Guillard, T., Moret, H., Brasme, L., Carlier, A., Vernet-Garnier, V., Cambau, E., et al. (2011). Rapid detection of qnr and qepA plasmid-mediated quinolone resistance genes using real-time PCR. Diagn. Microbiol. Infect. Dis. 70, 253–259. doi: 10.1016/j.diagmicrobio.2011.01.004
Han, H. S., Koh, Y. J., Hur, J.-S., and Jung, J. S. (2004). Occurrence of the strA-strB Streptomycin resistance genes in Pseudomonas species isolated from Kiwifruit plants. J. Microbiol. 42, 365–368.
Kim, H. B., Park, C. H., Kim, C. J., Kim, E. C., Jacoby, G. A., and Hooper, D. C. (2009). Prevalence of plasmid-mediated quinolone resistance determinants over a 9-year period. Antimicrob. Agents Chemother. 53, 639–645. doi: 10.1128/AAC.01051-08
Lee, H., Yong, D., Yum, J. H., Roh, K. H., Lee, K., Yamane, K., et al. (2006). Dissemination of 16 S rRNA methylase-mediated highly amikacin-resistant isolates of Klebsiella pneumoniae and Acinetobacter baumannii in Korea. Diagn. Microbiol. Infect. Dis. 56, 305–312. doi: 10.1016/j.diagmicrobio.2006.05.002
Lee, K., Yong, D., Yum, J. H., Kim, H. H., and Chong, Y. (2003). Diversity of TEM-52 extended-spectrum β-lactamase-producing non-typhoidal Salmonella isolates in Korea. J. Antimicrob. Chemother. 52, 493–496. doi: 10.1093/jac/dkg385
Liu, J. H., Deng, Y. T., Zeng, Z. H., Gao, J. H., Chen, L., Arakawa, Y., et al. (2008). Coprevalence of plasmid-mediated quinolone resistance determinants QepA, Qnr, and AAC(6’)- Ib-cr among 16 S rRNA methylase RmtB-producing Escherichia coli isolates from pigs. Antimicrob. Agents Chemother. 52, 2992–2993. doi: 10.1128/AAC.01686-07
Ma, J., Zeng, Z., Chen, Z., Xu, X., Wang, X., Deng, Y., et al. (2009). High prevalence of plasmid-mediated quinolone resistance determinants qnr, aac(6’)-Ib-cr, and qepA among ceftiofur-resistant Enterobacteriaceae isolates from companion and food-producing animals. Antimicrob. Agents Chemother. 53, 519–524. doi: 10.1128/AAC.00886-08
Mammeri, H., Van De Loo, M., Poirel, L., Martinez-Martinez, L., and Nordmann, P. (2005). Emergence of plasmid-mediated quinolone resistance in Escherichia coli in Europe. Antimicrob. Agents Chemother. 49, 71–76. doi: 10.1128/AAC.49.1.71-76.2005
Nordmann, P., and Poirel, L. (2005). Emergence of plasmid-mediated resistance to quinolones in Enterobacteriaceae. J. Antimicrob. Chemother. 56, 463–469. doi: 10.1093/jac/dki245
Park, Y.-J., Jin, K. Y., Kim, S.-I., Lee, K., and Arakawa, Y. (2009). Accumulation of plasmid-mediated fluoroquinolone resistance genes, qepA and qnrS1, in Enterobacter aerogenes co-producing RmtB and class A β-lactamase LAP-1. Ann. Clin. Lab. Sci. 39, 55–59.
Périchon, B., Courvalin, P., and Galimand, M. (2007). Transferable resistance to aminoglycosides by methylation of G1405 in 16S rRNA and to hydrophilic fluoroquinolones by qepA-mediated efflux in Escherichia coli. Antimicrob. Agents Chemother. 51, 2464–2469. doi: 10.1128/AAC.00143-07
Robicsek, A., Jacoby, G. A., and Hooper, D. C. (2006). The worldwide emergence of plasmid-mediated quinolone resistance. Lancet Infect. Dis. 6, 629–640. doi: 10.1016/S1473-3099(06)70599-0
Rocha-Gracia, R., Ruiz, E., Romero-Romero, S., Lozano-Zarain, P., Somalo, S., Palacios-Hernández, J., et al. (2010). Detection of the plasmid- borne quinolone resistance determinant qepA1 in a CTX-M-15-producing Escherichia coli strain from Mexico. J. Antimicrob. Chemother. 65, 169–171. doi: 10.1093/jac/dkp418
Ruiz, E., Sáenz, Y., Zarazaga, M., Rocha-Gracia, R., Martínez-Martínez, L., Arlet, G., et al. (2012). Qnr, aac(6’)-Ib-cr and qepA genes in Escherichia coli and Klebsiella spp: genetic environments and plasmid and chromosomal location. J. Antimicrob. Chemother. 67, 886–897. doi: 10.1093/jac/dkr548
Wang, D., Wang, H., Qi, Y., Liang, Y., Zhang, J., and Yu, L. (2011). Novel variants of the qnrB gene, qnrB31 and qnrB32, in Klebsiella pneumoniae. J. Med. Microbiol. 60, 1849–1852. doi: 10.1099/jmm.0.034272-0
Wang, M., Tran, J. H., Jacoby, G. A., Zhang, Y., Wang, F., and Hooper, D. C. (2003). Plasmid-mediated quinolone resistance in clinical isolates of Escherichia coli from Shanghai, China. Antimicrob. Agents Chemother. 47, 2242–2248. doi: 10.1128/AAC.47.7.2242-2248.2003
Wong, M. H., Chan, E. W., and Chen, S. (2015). Evolution and dissemination of oqxAB- like efflux pumps, an emerging quinolone resistance determinant among members of Enterobacteriaceae. Antimicrob. Agents Chemother. 59, 3290–3297. doi: 10.1128/AAC.00310-15
Keywords: plasmid-mediated quinolone resistance, QepA3 gene, recombinant plasmid, genetic structure, gene variation
Citation: Wang D, Huang X, Chen J, Mou Y, Li H and Yang L (2015) Characterization of genetic structures of the QepA3 gene in clinical isolates of Enterobacteriaceae. Front. Microbiol. 6:1147. doi: 10.3389/fmicb.2015.01147
Received: 05 August 2015; Accepted: 05 October 2015;
Published: 15 October 2015.
Edited by:
Firas H. Kobeissy, University of Florida, USAReviewed by:
Ziad Daoud, University of Balamand and CHN Hospital, LebanonElias Adel Rahal, American University of Beirut, Lebanon
Copyright © 2015 Wang, Huang, Chen, Mou, Li and Yang. This is an open-access article distributed under the terms of the Creative Commons Attribution License (CC BY). The use, distribution or reproduction in other forums is permitted, provided the original author(s) or licensor are credited and that the original publication in this journal is cited, in accordance with accepted academic practice. No use, distribution or reproduction is permitted which does not comply with these terms.
*Correspondence: Dongguo Wang, d2RndHpzQDE2My5jb20=; Jiayu Chen, Y2hlbnl1amlhMTBAMTYzLmNvbQ==
†These authors have contributed equally to this work.