Erratum: Genotypic and Antimicrobial Susceptibility of Carbapenem-Resistant Acinetobacter baumannii: Analysis of ISAba Elements and blaOXA-23-like Genes Including a New Variant
- 1Department of Microbiology, School of Medicine, Tehran University of Medical Sciences, Tehran, Iran
- 2Legal Medicine Research Center, Legal Medicine Organization, Tehran, Iran
- 3Innovative Medical Research Center, Islamic Azad University, Mashhad, Iran
- 4Pediatrics Infectious Disease Research Center, School of Medicine, Tehran University of Medical Sciences, Tehran, Iran
- 5Department of Microbiology and Virology, Shiraz University of Medical Sciences, Shiraz, Iran
Carbapenem-resistant Acinetobacter baumannii (CR-AB) causes serious nosocomial infections, especially in ICU wards of hospitals, worldwide. Expression of blaOXA genes is the chief mechanism of conferring carbapenem resistance among CR-AB. Although some blaOXA genes have been studied among CR-AB isolates from Iran, their blaOXA-23-like genes have not been investigated. We used a multiplex-PCR to detect Ambler class A, B, and D carbapenemases of 85 isolates, and determined that 34 harbored blaOXA-23-like genes. Amplified fragment length polymorphism (AFLP) genotyping, followed by DNA sequencing of blaOXA-23-like amplicons of CR-AB from each AFLP group was used to characterize their blaOXA-23-like genes. We also assessed the antimicrobial susceptibility pattern of CR-AB isolates, and tested whether they harbored insertion sequences ISAba1 and ISAba4. Sequence comparison with reference strain A. baumannii (NCTC12156) revealed five types of mutations in blaOXA-23-like genes; including one novel variant and four mutants that were already reported from China and the USA. All of the blaOXA-23-like genes mutations were associated with increased minimum inhibitory concentrations (MICs) against imipenem. ISAba1 and ISAba4 sequences were detected upstream of blaOXA-23 genes in 19 and 7% of isolates, respectively. The isolation of CR-AB with new blaOXA-23 mutations including some that have been reported from the USA and China highlights CR-AB pervasive distribution, which underscores the importance of concerted national and global efforts to control the spread of CR-AB isolates worldwide.
Introduction
Carbapenem-resistant Acinetobacter baumannii (CR-AB) can cause severe nosocomial infections particularly among patients in intensive care units (ICUs) around the world (Safari et al., 2013). Inadequate antimicrobial management of CR-AB infections often gives rise to highly resistant strains leading to prolonged hospitalization, treatment failures, and increased mortality (Higgins et al., 2010a). Epidemics of multi-, extensively-, and pandrug-resistant (MDR, XDR, and PDR) CR-AB have been reported from several countries (Kempf and Rolain, 2011; Bahador et al., 2013a; Moradi et al., 2015). In developing countries, such as Iran, challenges in the treatment of CR-AB infections are often exacerbated by widespread nosocomial outbreaks of OXA-type β-lactamase producing MDR-AB (for review see, Moradi et al., 2015). CR-AB are usually resistant to several β-lactams through the expression of chromosomal and plasmid-encoded carbapenemases including Ambler class A (blaGES, and blaKPC), class B (blaIMP, blaNDM−1, blaSPM-1, and blaVIM), and class D (blaOXA-23, 40, and 58-like; Siroy et al., 2005; Lu et al., 2009; Abbott et al., 2013). While the production of OXA-23 by A. baumannii is sufficient to confer resistance to carbapenems, insertion sequence (IS) elements ISAba1 and/or ISAba4 upstream of blaOXA-23-like genes enhance the blaOXA–mediated carbapenem resistance of CR-AB (Turton et al., 2006; Lee et al., 2012; Evans and Amyes, 2014). Although there are a few reports from Iran regarding the distribution and/or frequency of the blaOXA-51-like genes among CR-AB, data about characterization of their blaOXA-23 genes and ISAba elements is not available.
In this study, we have characterized blaOXA genes in CR-AB isolates from Iran, and report new variants that harbor novel mutations in their blaOXA-23-like carbapenemase genes. In addition to analyzing the distribution and frequency of blaOXA-23-like genes, we have determined the antimicrobial susceptibility patterns of isolates and the presence of ISAba1 and ISAba4 enhancer elements upstream of their blaOXA-23-like genes. Characterization of blaOXA genes and assessment of carbapenemase-mediated antibiotic resistance among A. baumannii isolates can help efforts to develop databases, which are essential to a comprehensive national surveillance program in Iran, toward the local and global control of CR-AB outbreaks.
Materials and Methods
Specimens and Bacterial Isolates and Cultures
A total of 85 non-repetitive clinical specimens were collected during 2011 from the intensive care units (ICUs) of Imam Khomeini Medical Center (IKMC) and Children's Medical Center (CMC) in Tehran, Iran. IKMC and CMC are affiliated with Tehran University of Medical Sciences (TUMS), and both are large referral centers that provide tertiary health care to patients from all over Iran. Specimens were collected from ICUs in surgical (S), internal medicine (M), emergency (E), pediatrics (P), and kidney transplantation (T) wards. Clinical isolates were initially identified as A. baumannii using the API20NE system (bioMérieux, Marcy-l'Etoile, France), and were further confirmed by gyrB multiplex PCR, as described previously (Higgins et al., 2010b). Specimen sources for A. baumannii isolates were as follows: respiratory tract (n = 51), urine (n = 16), blood (n = 11), wound (n = 5), and cerebral spinal fluid (CSF; n = 2). Twenty six of the A. baumannii isolates were part of a previous molecular epidemiologic study (Bahador et al., 2014). Brain heart infusion (BHI) agar plates and Mueller-Hinton broth (MHB; both from Merck, Germany) were used to culture the bacterial isolates.
Antimicrobial Susceptibility Testing
To assess susceptibility of A. baumannii clinical isolates, the disk agar diffusion (DAD) method (CLSI, 2015) was carried out according to the Clinical and Laboratory Standards Institute (CLSI) procedures and breakpoint interpretations, using antimicrobial disks containing 19 different antimicrobial agents (Mast Diagnostics, Bootle, UK; Table 2). The CLSI guideline for broth microdilution test for minimum inhibitory concentrations (MICs) was used to assess the susceptibility of MDR-AB isolates to colistin (CST), imipenem (IPM), rifampicin (RIF), and tigecycline (TGC). For tigecycline susceptibility tests, the criteria of the European Committee on Antimicrobial Susceptibility Testing (EUCAST) for Enterobacteriaceae were used, in which an MIC of < 1 μg/mL was defined as susceptible and >2 μg/mL was considered resistant (EUCAST, 2015). Rifampicin susceptibility was interpreted according to CLSI criteria using breakpoint values suggested for Staphylococcus aureus, in which susceptible and resistant were defined as ≤ 1 μg/mL and ≥4 μg/mL, respectively (CLSI, 2015). A. baumannii isolates were defined as MDR, XDR, and PDR according to the definitions provided by Magiorakos (Magiorakos et al., 2012). The MIC geometric mean (MICgm) of imipenem against blaOXA-23-like+ CR-AB isolates were also compared with the MICgm of non-mutant isolates, and fold-increase calculations were measured against MICgm of non-mutant strains.
Detection of Carbapenemase Gene ISAba1 and ISAba4 Insertion Sequences
The overall strategy for the identification of the 34 blaOXA-23-like+ CR-AB isolates is shown in Supplemental Figure 1. Briefly, we tested all 85 A. baumannii isolates for carbapenemase production by the modified Hodge test (Lee et al., 2012), and their chromosomal DNA were tested by two different confirmatory multiplex-PCR assays to identify the most common carbapenemase encoding genes. The criteria to include isolates in this study were the presence of PCR-specific amplicons, confirmed by agarose gel electrophoresis analysis (Supplemental Figure 1). A novel in-house multiplex-PCR, referred to as AB-hexaplex-PCR was optimized for the rapid and simultaneous detection of the most common carbapenemase genes, including Ambler class A and B (blaKPC, blaGES, blaIMP-1, blaVIM-2, blaNDM-1, and blaSPM-1) in A. baumannii using Primer 3 software (version 4.0; http://primer3.wi.mit.edu/; accessed June 05, 2011). Reference gene sequences were accessed from GenBank [http://www.ncbi.nlm.nih.gov/GenBank (blaKPC: GQ140348, blaGES: GU207844, blaIMP-1: EF375699, blaVIM-2: GQ288396, blaNDM-1: JN794561, and blaSPM-1: HM370523; accessed June 04, 2011)], as shown in Table 1. The Ambler class D type carbapenemase genes (blaOXA-23, 24, 51, 58 like) were detected using the Woodford multiplex PCR assay method (Woodford et al., 2006). Additionally, the AB-hexaplex-PCR distinguished amplicons corresponding to the blaIMP-1, blaSPM-1, blaGES and blaKPC, blaNDM-1, and blaVIM-2 genes, and isolates that harbored these genes were excluded from our study (representative gel; Supplemental Figure 2). The frequency of ISAba1 and ISAba4 elements upstream of blaOXA-23-like and blaOXA-51-like genes were assessed using a series of PCR amplifications. A set of primers, referred to as ISAba1F/OXA-23R, ISAba4F/OXA-23R, and ISAba1F/OXA-51R, is shown in Table 1. After our serial screening of isolates, 34 isolates were identified that harbored blaOXA-23-like gene as their sole acquired carbapenemase gene.
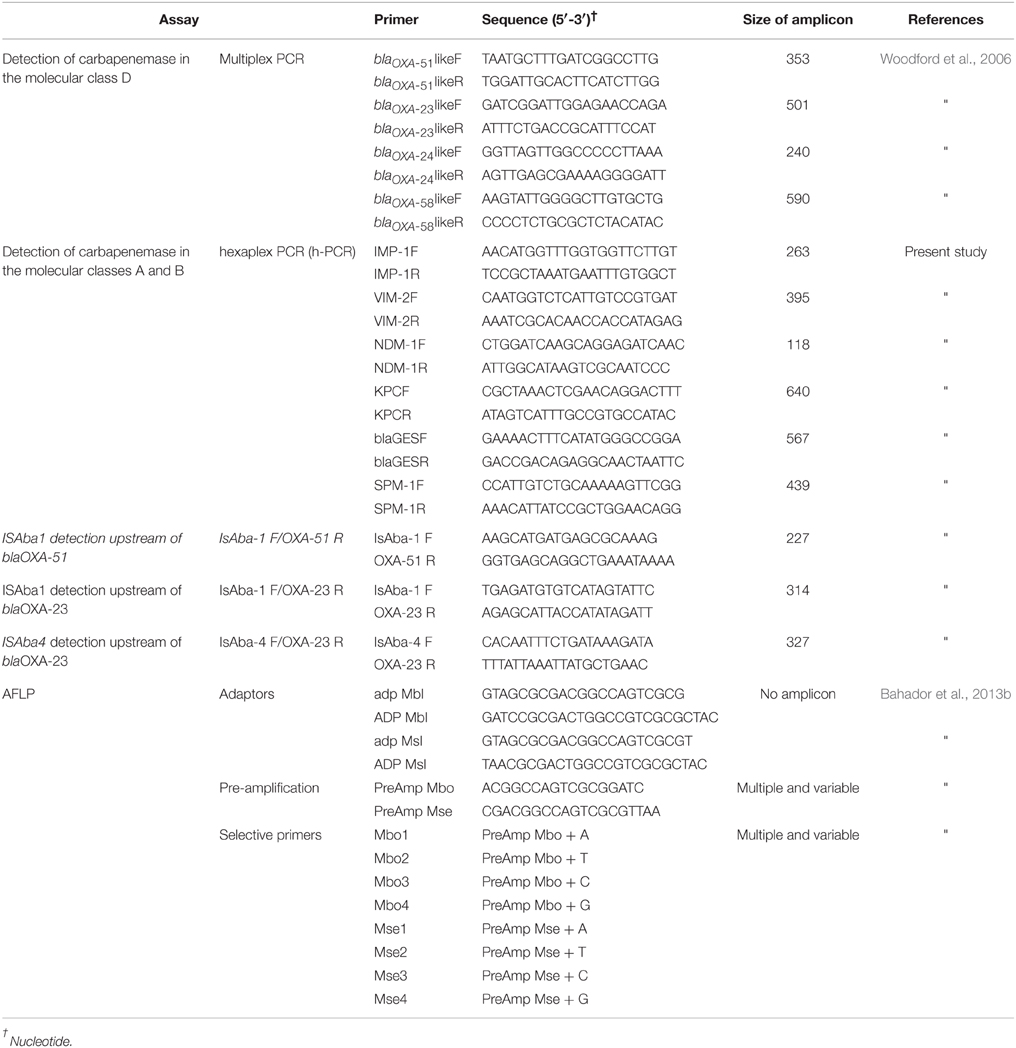
Table 1. Primer sequences and adaptors (and their corresponding reference) utilized in order to identify most common carbapenemase genes in our isolates, and to generate amplicons for AFLP genotyping analysis.
AFLP Genomic Fingerprint Analysis
Amplified fragment length polymorphism (AFLP) genotyping of blaOXA-23-like+ and blaOXA-51-like+ isolates was carried out by a modified Vos method (Vos et al., 1995), as described previously (Bahador et al., 2013b). AFLP typing was carried out prior to sequence analysis to ensure thorough examination of the diversity of CR-AB isolates. Briefly, chromosomal DNA was size-verified and double-digested with MboI and MseI (Fermentas, Lithuania). Then DNA fragments were ligated to corresponding adapters using T4 DNA ligase (350 U/μL, Takara Bio, Japan) followed by the preliminary PCR using PreAmp-Mbo and PreAmp-Mse primers (Table 1). Preliminary PCR amplicons served as templates for selective PCR, which generated AFLP genotype profiles upon agarose gel analysis. Initial testing of 36 combinations of primers, including PreAmp Mbo (PreAmp Mbo+A, +T, +C, +G), and PreAmp Mse (PreAmp Mse +A, +T, +C, +G) and A. baumannii NCTC12156 DNA as a normalization reference showed that the Mbo4-Mse4 combination generated the clearest AFLP profiles when analyzed using BioNumerics version 5.10 (Applied Maths, Sint-Martens-Latem, Belgium). The similarity between band patterns was calculated using the Dice coefficient, with an optimization of 0.5% and a position tolerance of 1%. The AFLP types were grouped at the 90% similarity cutoff on a dendrogram constructed by the unweighted-pair group method using average linkages (UPGMA).
DNA Sequencing of blaOXA-23-like Genes
To evaluate an association between changes in the chromosomal carbapenemase gene sequence of isolates and their antimicrobial resistance pattern, a two-step approach was adopted. An initial AFLP assay was carried out on blaOXA-23-like+ CR-AB, followed by DNA sequence analysis of the blaOXA-23-like gene of a representative isolate from each AFLP genotype group.
Briefly, we used a high fidelity Pfu DNA polymerase (Fermentas, Lithuania) to generate blaOXA-23-like specific amplicons, which were purified using a AccuPrep® PCR Purification Kit (Bioneer, Daejeon, Korea) and cloned into pTZ57R (InsT/A Clone PCR product cloning kit, Fermentas, Vilnius, Lithuania). DNA was then transferred into competent E. coli TOP10 cells, which were then isolated using Luria-Bertani (LB) agar supplemented with ampicillin (100 μg/mL). Plasmid DNA was prepared with the AccuPrep Plasmid MiniPrep DNA Extraction Kit, (Bioneer, Daejeon, Korea) and sequenced using an ABI3730 automatic sequencer (Applied Biosystems, CA, USA). The sequences were analyzed using a BLAST algorithm against the NCBI GenBank database [http://www.ncbi.nlm.nih.gov/guide/dna-rna/ (accessed 05.06.11)].
Iodometric Assay of β-lactamase Activity
Bacterial β-lactamase enzymatic activity was determined by an iodometric assay, as described previously (Sawai et al., 1978). Briefly, crude lysates of 16 isolates that represented AFLP groups were extracted using the Saino method (Saino et al., 1982). Briefly, overnight bacterial growth were diluted in MHB broth to a concentration of 107 cfu/ml and incubated in a shaker for 2 h at 35°C. As an inducer, imipenem was added at 0.25 of the isolate MIC and incubated for an additional 2 h (Clark, 1996). Bacterial cells were harvested, centrifuged at 4°C, washed twice with 50 mM phosphate buffer saline (PBS; pH 7.0), and re-suspended in 0.1 M PBS (pH 7.0). The suspension was sonicated in an ultrasonic disrupter (Branson Ultrasonics Co., Shanghai, China) at 75 W for 3 min in an ice bath; afterward the disrupted cell suspension was centrifuged at 13,000 × g for 30 min at 4°C. The β-lactamase enzymatic activity of the supernatant fluid (i.e., bacterial lysate) was measured against imipenem using iodometric method described by Doust (Daoust et al., 1973) using reagents prepared, as described previously (Onishi et al., 1974; Sawai et al., 1978; Minami et al., 1980). Briefly, iodine reagent (40 μmol in 0.5 M acetate buffer, pH 4.0) was added to lysate supernatant fluids, after 5 min incubation with imipenem (50 μg/mL) at 30°C. Ten minutes later, samples' absorbance was measured at 620 nm, and imipenem hydrolysis was determined. Activity was reported as the mean of triplicate samples in micromoles of imipenem degraded per minute per milligram of protein in each bacterial extract. Protein concentrations were measured by Bradford assay kit (Pierce™ Coomassie Plus Assay Kit, Thermo Scientific, Ottawa, Canada).
In Silico Analysis and Nucleotide Sequence Accession Numbers
In silico analysis was carried out using GenBank nucleotide database. Predict Protein software (hosted by Rostlab) was also used to predict changes as a result of a frameshift mutation. The nucleotide sequence data were deposited in the GenBank nucleotide database under accession numbers: JQ343842.1, JQ343840.1, JQ343838.1, JQ343836.1, JQ343841.1, JQ343839.1, JQ343837.1, JQ360584.1, JQ360582.1, JQ360580.1, JQ360578.1, JQ360583.1, JQ360581.1, JQ360579.1, JQ360577.1, and JQ061320.1. The novel DNA sequence of blaOXA 23 genes, with “No Full-Match” by GenBank; as well as its corresponding peptide amino acid sequence was submitted to Lahey database (lahey.org/Studies).
Results
Antimicrobial Susceptibility Profiles and AFLP Genomic Fingerprint Analysis
Table 2 shows the susceptibility profiles of all 85 CR-AB isolates against CLSI groups of antimicrobial agents. Overall, CR-AB isolates were most resistant to CLSI group A (51–96%), followed by group B (25–97%) antimicrobials. Overall, up to 96% of isolates were resistant to 12 of the tested antimicrobials; while the rates of resistance to tigecycline, imipenem, and doripenem were 34, 65, and 94%, respectively. The lowest resistance rates among isolates were against colistin (12%), minocycline (25%), and doxycycline (31%). Interestingly, all colistin-resistant isolates were susceptible to tigecycline and/or tobramycin. The frequency of MDR, XDR and PDR isolates were 69, 24, and 0%, respectively; and broadly-resistant CR-AB isolates were most frequently recovered from the surgical and internal medicine ICU wards. However, the frequency of resistant isolates was generally proportional to the number of specimens from each ICU (Table 2).
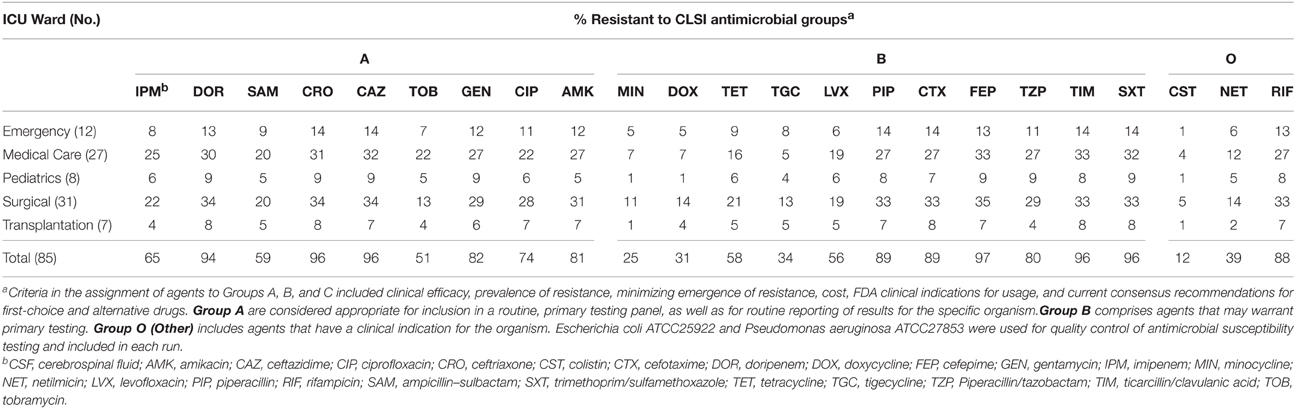
Table 2. In vitro antimicrobial susceptibility results of 85 non-replicate clinical A. baumannii isolates according to CLSI antimicrobial grouping of A, B, and O, as according to the frequency of A. baumannii isolation from the type of ICU ward.
Our analysis revealed that 34 (40%) isolates harbored blaOXA-23-like genes, and 51 isolates were resistant to carbapenems but did not harbor blaOXA-23-like genes (Supplemental Figure 1). AFLP genotype analysis of blaOXA-23-like+ isolates generated 16 distinct AFLP genotypic groups, labeled genotype A through P. Group C (n = 6) was the predominant AFLP type, followed by genotype I (n = 4), and genotypes B, K, L, and N (n = 3 in each group). While each AFLP group consisted of 1 to 6 isolates, 50% (8/16) of the groups consisted of a single isolate, indicative of a high diversity among CR-AB isolates. Despite this diversity, the antimicrobial susceptibility patterns among 13 (82%) genotypes were similar, with the exception of genotypes A, M, and N (Figure 1).
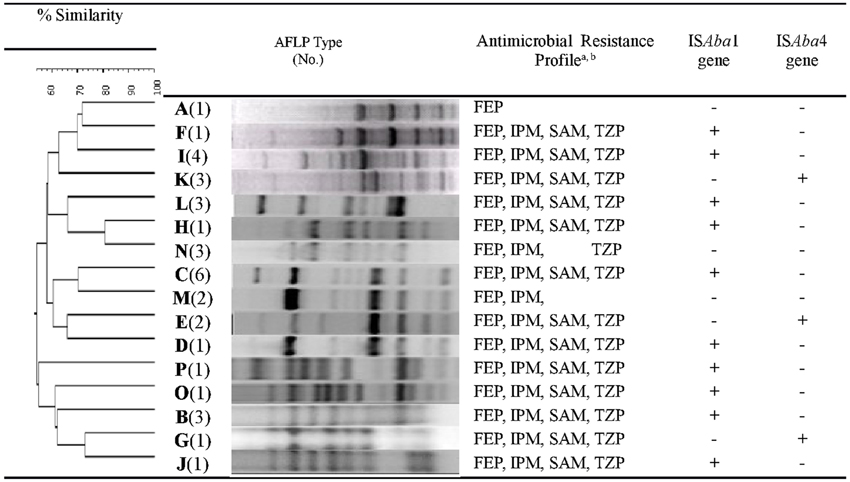
Figure 1. Dendrogram of amplified fragment length polymorphism (AFLP) analysis of genomic DNA extracted from bla(+)OXA-23-like CR-AB isolates. Susceptibility to select antimicrobials and ISAba presence is also indicated. Dice coefficient with 0.5% optimization and 1% position tolerance was used. Dendrogram was constructed by unweighted-pair group method using average linkages (UPGMA); and AFLP type identification was defined by groups formed at 90% similarity cutoff. aFEP, cefepime; IPM, imipenem; SAM, ampicillin-sulbactam; TZP, Piperacillin/tazobactam. bAll 16 AFLP types were resistant to the following beta-lactam antimicrobial agents: CAZ, ceftazidime; CRO, ceftriaxone; CTX, cefotaxime; PIP, piperacillin; TIM, ticarcillin/clavulanic acid.
Detection of ISAba1 and ISAba4
Overall, ISAba1 and ISAba4 sequences were present in 61% and 7% of all tested CR-AB isolates, respectively. Among 34 blaOXA-23-like+ isolates, 67% were ISAba1+, and 18% were ISAba4+ (Figure 1); whereas 10 (63%) and 3 (19%) of AFLP genotypes harbored ISAba1 and ISAba4 elements, respectively. ISAba4 was only present among AFLP types G, E, and K isolates, while genotypes A, M, and N has neither ISAba1, nor ISAba4 element (Table 3; Figure 1). Interestingly, even though the ISAba1+ isolates of genotype B (N = 3) exhibited an XDR profile, all genotype B isolates remained susceptible to tobramycin and ampicillin–sulbactam.
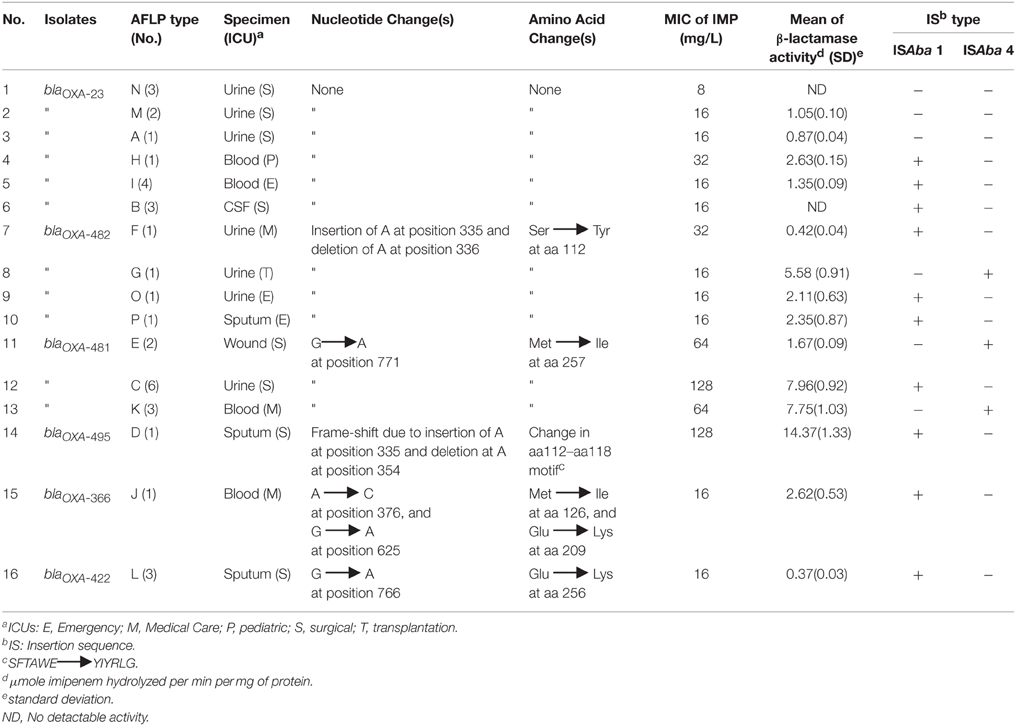
Table 3. Comparison of blaOXA-23-like gene sequences among CR-AB isolates belonging to various AFLP genotype groups, as compared to blaOXA-23 gene sequence of A. baumannii reference strain, according to the specimen source, MIC against imipenem, β-lactamase activity, and ISAba element status.
Among the blaOXA-23-like+ isolates that harbored either ISAba1 or ISAba4 elements, a majority (82%) displayed a distinctive profile of resistance to 9 antimicrobial agents, namely, CAZ, CRO, CTX, DOR, FEP, IPM, PIP, SAM, and TZP (Figure 1). The presence of either ISAba1, or ISAba4 imparted resistance to imipenem and doripenem among blaOXA-23-like+ genotypes. However, the genotype A isolate (which was ISAba1− and ISAba4−) also showed resistance to imipenem (MIC = 16μg/mL).
Sequence Analysis of blaOXA-23-like Genes
Table 3 demonstrates sequence differences between the blaOXA-23-like specific amplicon among members of AFLP groups vs. the blaOXA-23 sequence of the A. baumannii reference strain (referred to as “wild type”). The blaOXA-23 gene sequences of six (37%) AFLP genotypes did not differ from the wild-type strain; however, isolates from 10 (63%) AFLP types had mutations in blaOXA-23 genes. Five different blaOXA-23 gene mutations were detected, and a Genbank search revealed that one of the mutant sequences had been recently reported from the USA, namely blaOXA-366, and three were reported from China (i.e., blaOXA-422, 481, and 482-like genes). One novel blaOXA-23-like gene sequence was thus submitted to the Lahey database and assigned as the blaOXA-495-like gene.
At the points of carbapenemase gene mutations, comparison of a 21-nucleotide sequence of PCR amplicons obtained from isolates in mutant AFLP groups that had >2 members, i.e., groups L, E, C, and K, showed strong homologies within these AFLP types (Supplemental Figure 3). Additionally, Table 3 displays the AFLP type, imipenem MIC, and ISAba status among various blaOXA-23+ isolates according to their specimen source. The absence of mutation(s) in blaOXA-23 genes was associated with lower imipenem MICs as compared to mutant isolates. Imipenem MICgm for all non-mutant blaOXA-23 gene isolates was 16 μg/mL (range = 8−32μg/mL), whereas the mean MIC for mutants was almost 50 μg/mL (range = 16−128μg/mL). Conversely, alterations of the blaOXA-23 gene were associated with an increased MIC to imipenem among A. baumannii isolates. While 16 (47%) of blaOXA-23-like+ isolates harbored ISAba1 sequences upstream of their carbapenemase gene, only six (18%) isolates were ISAba4+. ISAba1 elements were detected upstream of either blaOXA-23 or blaOXA-51 genes, orboth these genes. Since, none of the blaOXA-23-like+ isolates were both ISAba1+ and ISAba4+, the presence of these elements appeared mutually exclusive among isolates (Table 3). The ISAba4 sequence was absent among blaOXA-23-like genes of non-mutant CR-AB isolates, whereas six of mutant isolates, namely genotypes G, E, and K, were ISAba4+ with moderate MICs, ranging from 16 to 64 μg/mL (Table 3). Although mutant isolates of genotypes C and D were ISAba1+ had the highest imipenem MICs (128 μg/mL), the imipenem MIC of ISAba1+ mutants among genotypes O, P, J, and L was 16 μg/mL. Furthermore, all ISAba1− and ISAba4− CR-AB isolates had non-mutant blaOXA-23+ genes, namely genotypes A, M, and N isolates, which showed the lowest imipenem MICs (8–16 μg/mL), as well. Surprisingly, three blaOXA-23-like+ isolates (genotypes A and M) had imipenem MICs of 16 μg/mL, but harbored neither ISAba1, nor ISAba4 elements upstream of the blaOXA-23-like gene, suggestive of other resistance mechanisms in these isolates (Table 3).
As shown in Table 3, among mutations of the blaOXA-23-like genes, insertions/deletions at nucleotide positions 335 and 336 were most frequent (40%), followed by a single substitution at position 771 (30%). Isolates with F, G, O, and P genotypes showed the insertion/deletion mutations at position 335, with imipenem MICs of 16–32 μg/mL, whereas isolates with genotypes E, C, and K had the substitution at position 771 and showed the highest imipenem MICs of 64–128 μg/mL (Table 3). Further analysis revealed that three other single substitutions also occurred at positions 376, 625, and 766 among genotypes J and L, but their imipenem MICs were not higher than non-mutants (16 μg/mL). In addition, three isolates with the substitution at position 771 had a 3–4-fold increase in imipenem MIC over that of the non-mutants. A frame-shift mutation at position 355, which corresponds to a change at aa112–118, in genotype D isolate (strain TUMS/BTRF 661) was associated with a four-fold increase in imipenem MICgm (128 μg/mL) over that of non-mutant isolates. Software prediction showed that the frame-shift mutation may change the subcellular localization of carbapenemase and enhance its secretion rate, which can explain the high MIC of the isolate against carbapenems. In silico comparison of carbapenemase binding domains of wild-type vs. the frame-shift mutant also showed that the binding domain changed from “aa15–24 and aa28–29” to “aa15–22 and aa24–25” motif, which may lead to higher affinity of mutant OXA-23 enzyme for the binding cleft of carbapenems.
Five blaOXA-23 mutants and one non-mutant isolate showed imipenem MICs of >16 μg/mL. Further in silico analysis revealed that the blaOXA-23-like gene mutations would lead to up to six amino acid changes in the carbapenemase protein. However, the highest imipenem MICs were associated with a single substitution at position 771 of blaOXA-23-like gene, corresponding to aa 257 substitution in carbapenemase among genotypes E, C, and K, which represented 55% of the mutant isolates. All AFLP types with non-mutant blaOXA-23 genes showed MICs of ≤ 16 μg/mL for imipenem, except the genotype H isolate (MIC = 32μg/mL); however, all blaOXA-23-like mutants showed high imipenem resistance (MIC = 16–128 μg/mL).
As shown in Table 3, we compared the β-lactamase activity of the 16 representative isolates from each AFLP group. Overall, the range of β-lactamase activity of non-mutant isolates was lower (not detectable–2.63 μmoles/min/ mg protein) than the blaOXA-23-like mutants (0.37–14.37 μmoles/min/mg protein). Mutant isolates, such as the frame-shift mutant genotype D, showed the highest imipenem MICs (i.e., 64 and 128 μg/mL), as well as the highest β-lactamase activities, i.e., 7.96 and 14.37 μmoles/min/mg protein for genotype C and K, respectively. In contrast, lysates from isolates with no blaOXA-23-like gene mutation that had the lowest MICs (e.g., genotypes N and B), and showed no detectable β-lactamase activity. The β-lactamase activity of a imipenem-susceptible (MIC < 4 μg/ml), clinical A. baumannii isolate, was also below assay's detection level (data not shown). Among the 4 isolates that had the same mutation at position 335, the β-lactamase activity was between 0.42 and 5.58 μmoles/min/ mg protein, while imipenem their MIC was 16–32 μg/mL. However, with the exception of genotype E, the β-lactamase activity of mutants with position 771 mutation, was increased concomitant with high MICs in these isolates. The majority (70%) of mutant isolates were recovered from two ICU wards; namely, the surgical (n = 4), and the internal medicine (n = 3) ICU; however, no mutant isolates were recovered from the pediatric ICU ward. Among the blaOXA-23-like mutant isolates, eight (80%) were recovered from either urine or sputum specimens (Table 3).
Table 4 shows the distribution of ISAba1 or ISAba4 sequences upstream of various blaOXA-23- and blaOXA-51-like genes among the isolates that harbored ≥1 carbapenemase genes; and also their resistance rate against carbapenems. The presence of ISAba upstream of the blaOXA-51-like andblaOXA-23-like genes was associated with high rate of carbapenem resistance. Among all blaOXA-51-like+ or blaOXA-23-like+ isolates, almost 32% (n = 27) lacked either ISAba1, or ISAba4 sequences. CR-AB isolates were consistently more resistant to doripenem than to imipenem, regardless of their blaOXA- genes (Table 4). There was no marked difference in carbapenem resistance rates whether the isolates harbored the “blaOXA-51-like gene alone,” or “blaOXA-51-like plus blaOXA-24-like” genes. Overall, the ISAba1 element was more often associated with blaOXA-51-like gene (20–100%) than with blaOXA-23-like genes; and ISAba+ isolates showed high rates of carbapenem resistance, especially against doripenem. Despite this high resistance rate, 13% of blaOXA-23-like+/blaOXA-51+ isolates that harbored both ISAba1 and ISAba4 were imipenem susceptible (Table 4). Among ISAba+ isolates, these elements were upstream of the blaOXA-51-like gene in 60% (31/52) of the isolates, whereas only 31% harbored ISAba1 upstream of the blaOXA-23-like gene. All 13 (15%) CR-AB isolates with blaOXA-51-like gene as their sole carbapenamase gene had ISAba1 elements. Interestingly, even though test isolates showed an overall high resistance rate against carbapenems, 32% of isolates did not harbor either ISAba1 or ISAba4 elements. By and large, there was no marked change in resistance rate among isolates that harbored the blaOXA-24-like gene in combination with other carbapenemase genes (Table 4).
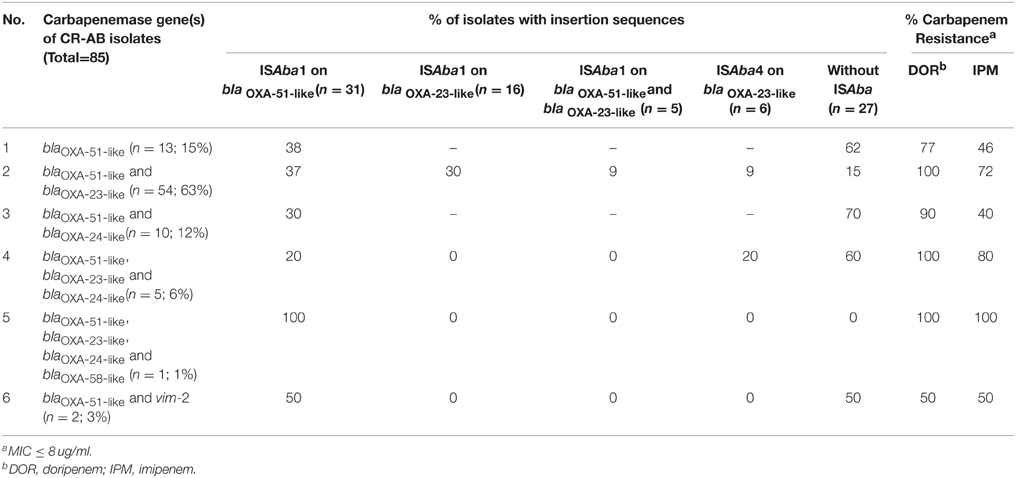
Table 4. Frequency of ISAba1 or ISAba4 sequences upstream of various blaOXA- genes among test CR-AB isolates that harbored ≥ 1 carbapenemase genes, and the comparison of percent resistance against carbapenems among CR-AB isolates according to the isolate's blaOXA- gene combination.
Discussion
Infections caused by carbapenem-resistant A. baumannii are among the most difficult to treat, especially among ICU patients (Alfandari et al., 2014). In several countries, including Iran, clinicians face serious challenges in choosing an effective combination of antimicrobial agents while treating patients with severe nosocomial CR-AB infections. Efforts to control MDR-AB outbreaks have prompted widespread use of antimicrobials, such as tigecycline and colistin, as therapeutic measures to combat severe infections (Garnacho-Montero et al., 2015). However, appropriate treatment and effective infection control measures require local susceptibility patterns, as well as molecular epidemiologic data, such as the blaOXA gene status of CR-AB isolates. Several surveillance studies have reported widespread nosocomial outbreaks of OXA-type producing A. baumannii, and a high prevalence of blaOXA gene-carrying CR-AB in Iran, but data regarding their blaOXA-23-like gene is not available (Moradi et al., 2015).
In the present study, we have genetically evaluated blaOXA-23-like+ CR-AB isolates and found high genotypic diversity among the isolates, including variants with new blaOXA-23-like gene mutations. These mutations were associated with up to four-fold increases in MIC levels against imipenem, as compared to non-mutant isolates. Mutations in certain codons associated with a high degree of resistance to imipenem. For instance, substitutions at position 355 of the blaOXA-23-like gene (i.e., the frame-shift mutation) were associated with high-level resistance, whereas position 256 mutations were associated with low-level resistance. Surprisingly, newly-found mutations correspond to regions of the carbapenemase molecule that are outside the standard “S-T-F-K, S-X-I, Y-G-N” and “K-S-G” oxacillinase motifs (Couture et al., 1992), suggesting that configurational changes due to novel mutations may also affect oxacillinase activity against carbapenems.
Although a high frequency of MDR (69%) and XDR (24%) A. baumannii isolates from this region is consistent with previous reports (D'Arezzo et al., 2011; Potron et al., 2011; Sung et al., 2011), our finding of 26 and 6% increases in resistance to tigecycline and colistin, respectively, (Bahador et al., 2014) is quite worrisome. Fortunately, while all isolates harboring mutations in blaOXA-23-like genes showed resistance to carbapenem-class antibiotics; they were susceptible to tigecycline and/or tobramycin, which concurs with a recent report that shows potential activity of a number of combinations against MDR A. baumannii (Garnacho-Montero et al., 2015). Our data regarding to a high prevalence of blaOXA-23-like genes among CR-AB from Tehran confirms previous reports (Shahcheraghi et al., 2011), and implies that extra efforts should be focused on controlling the spread of blaOXA-23-like+ A. baumannii in this area. Clonal outbreaks of OXA-23-producing CR-AB have been reported from several countries (Mugnier et al., 2010). Interestingly, isolates in this study did not harbor any NDM-1 metallo-β-lactamase genes, nor the “blaSPM−1 and blaGES−1” genes, which have recently been reported from India and Pakistan (Jones et al., 2014; Sartor et al., 2014), and Tehran (Shahcheraghi et al., 2011), respectively. However, we detected three mutant blaOXA-23-like genes with identical sequences reported from China.
The data on the presence of ISAba1 sequences upstream from blaOXA genes and enhancement of OXA–enzyme expression confirms previous reports (Sung et al., 2011); however, these findings are in contrast with a report from northwestern Iran, which detected no ISAba1 sequences upstream of the blaOXA gene (Peymani et al., 2012). Together, these results suggest that the CR-AB populations in various parts of Iran are diverse and distinct, which may hint on probable differences in antimicrobial management of A. baumannii infections in various regions. Moreover, most of the blaOXA-23 mutant A. baumannii isolates were obtained from urine and sputum samples, suggesting that specific infection control protocols regarding urinary catheters and ventilators are possible primary sources of CR-AB transmission.
Although increased imipenem resistance due to a mutation in the blaOXA-23 gene has been reported previously (Lin et al., 2011), to the best of our knowledge, this is the first report of CR-AB blaOXA-23 gene mutants from Iran. It is noteworthy that the TUMS/BTRF661 strain showed the highest MIC (128 μg/mL), implying a greater influence of the frame-shift mutation on carbapenem resistance than any of the substitution mutations. Our future studies will focus on exploring the difference in the MICs of the various mutants (16 vs. 128 μg/mL) and the potential complex interactions between antimicrobial agents and carbapenemase at the molecular level, where the position of the affected motif plays a critical role. While production of carbapenemase remains to be the chief mechanism of carbapenem-resistance in A. baumannii, whether additional factors, such as alterations in outer membrane permeability, efflux pumps as with AdeABC (Potron et al., 2015), or OprD porin (Potron et al., 2015), contribute to carbapenem-resistance amongmutants. The variability in the β-lactamase activity and MIC values of variants that share a mutation, suggests that other factors play a role in high MIC levels among mutant CR-AB isolates, and they remain to be explored.
Assuming future confirmation of the correlation of specific mutations with high MICs, carbapenem resistance levels may be predictable by DNA sequence-based detection methods. Also, determination of predominant blaOXA-23 genotype(s) of isolates in various areas and their blaOXA-23-like gene mutations may serve as a tool for molecular epidemiologic investigations to control the spread of CR-AB infections. While the present study focused on the chromosomal OXA-encoding genes in CR-AB, we also plan to explore the role of mutations in plasmid-encoded blaOXA-23-like genes among carbapenem-resistant A. baumannii, since many of these genes are plasmid-borne.
In conclusion, we report the identification of CR-AB variants that harbor blaOXA-23-like gene mutations, which are associated with an increased MIC against imipenem. Several blaOXA-23-like mutant isolates are widespread and have been reported from the USA, and China. The detection of new blaOXA-23 mutant isolates from Iran highlights the importance of concerted efforts, at the national and global levels, toward the control of carbapenem-resistance among A. baumannii isolates worldwide.
Funding
This research has been supported by Tehran University of Medical Sciences and Health Services grant no. 89. 01-30-10430.
Conflict of Interest Statement
The authors declare that the research was conducted in the absence of any commercial or financial relationships that could be construed as a potential conflict of interest.
Supplementary Material
The Supplementary Material for this article can be found online at: http://journal.frontiersin.org/article/10.3389/fmicb.2015.01249
Supplemental Figure 1. Presentation of study strategy to select blaOXA-23+ A. baumannii clinical isolates for AFLP genotype determination and DNA sequence analysis. Step-wise selection of blaOXA+ isolates was carried out using 2 sets of multiplex PCR assay, followed by AFLP genotypic analysis and DNA sequencing of blaOXA-23-like gene amplicons.
Supplemental Figure 2. Agarose gel electrophoresis analysis of PCR amplicons specific for Ambler Class A and B carbapenemases. Genomic DNA from clinical A. baumannii isolates were analyzed by uniplex and multiplex PCR assay as described (M&M). Lanes 1 through 6; specific bands for (1) NDM1, (2) IMP1, (3) VIM2, (4) SPM1, (5) blaGES, and (6) KPC encoding genes. Lane 7; AB-hexaplex (AB-h) PCR products of the above genes. Lane 8; 100 bp DNA markers.
Supplemental Figure 3. Comparison between DNA sequences of AB0057Ref.seq and PCR amplicon sequences obtained from mutant CR-AB isolates in genotype groups with >2 members showing a 10-nucleotide span in each direction of the point of mutation (total 21 nucleotides). (A) Alignment of amplicons from three isolates of AFLP group L (i.e., TUMS/BTRF443, L2, and L3) as compared to the AB0057Ref.seq sequence, with the mutation at position 766. (B) Alignment of AFLP groups E, C, and K amplicons with the AB0057Ref.seq reference sequence, with the mutation at position 771. In both panels (*) indicates sequence identity (or homology), and (−) shows the position of carbapenemase gene mutation.
References
Abbott, I., Cerqueira, G. M., Bhuiyan, S., and Peleg, A. Y. (2013). Carbapenem resistance in Acinetobacter baumannii: laboratory challenges, mechanistic insights and therapeutic strategies. Expert Rev. Anti Infect. Ther. 11, 395–409. doi: 10.1586/eri.13.21
Alfandari, S., Gois, J., Delannoy, P. Y., Georges, H., Boussekey, N., Chiche, A., et al. (2014). Management and control of a carbapenem-resistant Acinetobacter baumannii outbreak in an intensive care unit. Med. Mal. Infect. 44, 229–231. doi: 10.1016/j.medmal.2014.03.005
Bahador, A., Bazargani, A., Taheri, M., Hashemizadeh, Z., Khaledi, A., Rostami, H., et al. (2013a). Clonal lineages and virulence factors among Acinetobacter baumannii isolated from Southwest of Iran. J. Pure Appl Microbiol. 7, 1559–1566.
Bahador, A., Taheri, M., Pourakbari, B., Hashemizadeh, Z., Rostami, H., and Mansoori, N. (2013b). Emergence of rifampicin, tigecycline, and colistin-resistant Acinetobacter baumannii in Iran; spreading of MDR strains of novel International Clone variants. Microb. Drug. Resist. 19, 397–406. doi: 10.1089/mdr.2012.0233
Bahador, A., Raoofian, R., Taheri, M., Pourakbari, B., Hashemizadeh, Z., and Hashemi, F. B. (2014). Multidrug resistance among Acinetobacter baumannii isolates from Iran: changes in antimicrobial susceptibility patterns and genotypic profile. Microb. Drug Resist. 20, 632–640. doi: 10.1089/mdr.2013.0146
Clark, R. B. (1996). Imipenem resistance among Acinetobacter baumannii: association with reduced expression of a 33-36 kDa outer membrane protein. J. Antimicrob. Chemother. 38, 245–251.
Clinical Laboratory Standards Institute (CLSI) (2015). Performance Standards for Antimicrobial Susceptibility Testing: Twenty fourth Informational Supplement M100-S25. Wayne, PA: CLSI.
Couture, F., Lachapelle, J., and Levesque, R. C. (1992). Phylogeny of LCR-1 and OXA-5 with class A and class D β-lactamases. Mol. Microbiol. 6, 1693–1705. doi: 10.1111/j.1365-2958.1992.tb00894.x
Daoust, D. R., Onishi, H. R., Wallick, H., Hendlin, D., and Stapley, E. O. (1973). Cephamycins, a new family of beta-lactam antibiotics: antibacterial activity and resistance to β -lactamase degradation. Antimicrob. Agents Chemother. 3, 254–261. doi: 10.1128/AAC.3.2.254
D'Arezzo, S., Principe, L., Capone, A., Petrosillo, N., Petrucca, A., and Visca, P. (2011). Changing carbapenemase gene pattern in an epidemic multidrug-resistant Acinetobacter baumannii lineage causing multiple outbreaks in central Italy. J. Antimicrob. Chemother. 66, 54–61. doi: 10.1093/jac/dkq407
Evans, B. A., and Amyes, S. G. (2014). OXA β-lactamases. Clin. Microbiol. Rev. 27, 241–263. doi: 10.1128/CMR.00117-13
Garnacho-Montero, J., Amaya-Villar, R., Ferrándiz-Millón, C., Díaz-Martín, A., López-Sánchez, J. M., Gutiérrez-Pizarraya, A., et al. (2015). Optimum treatment strategies for carbapenem-resistant Acinetobacter baumannii bacteremia. Expert. Rev. Anti. Infect. Ther. 13, 769–777. doi: 10.1586/14787210.2015.1032254
Higgins, P. G., Dammhayn, C., Hackel, M., and Seifert, H. (2010a). Global spread of carbapenem-resistant Acinetobacter baumannii. J. Antimicrob. Chemother. 65, 233–238. doi: 10.1093/jac/dkp428
Higgins, P. G., Lehmann, M., Wisplinghoff, H., and Seifert, H. (2010b). gyrB multiplex PCR to differentiate between Acinetobacter calcoaceticus and Acinetobacter genomic species 3. J. Clin. Microbiol. 48, 4592–4594. doi: 10.1128/JCM.01765-10
Jones, L. S., Toleman, M. A., Weeks, J. L., Howe, R. A., Walsh, T. R., and Kumarasamy, K. K. (2014). Plasmid carriage of bla NDM-1 in clinical Acinetobacter baumannii isolates from India. Antimicrob Agents Chemother. 58, 4211–4213. doi: 10.1128/AAC.02500-14
Kempf, M., and Rolain, J. M. (2011). Emergence of resistance to carbapenems in Acinetobacter baumannii in Europe: clinical impact and therapeutic options. Int. J. Antimicrob. Agents 39, 105–114. doi: 10.1016/j.ijantimicag.2011.10.004
Lee, H. Y., Chang, R. C., Su, L. H., Liu, S. Y., Wu, S. R., Chuang, C. H., et al. (2012). Wide spread of Tn2006 in an AbaR4-type resistance island among carbapenem-resistant Acinetobacter baumannii clinical isolates in Taiwan. Int. J. Antimicrob. Agents 40, 163–167. doi: 10.1016/j.ijantimicag.2012.04.018
Lin, M. F., Kuo, H. Y., Yeh, H. W., Yang, C. M., Sung, C. H., Tu, C. C., et al. (2011). Emergence and dissemination of blaOXA-23-carrying imipenem-resistant Acinetobacter sp in a regional hospital in Taiwan. J. Microbiol. Immunol. Infect. 44, 39–44. doi: 10.1016/j.jmii.2011.01.008
Lu, P. L., Doumith, M., Livermore, D. M., Chen, T. P., and Woodford, N. (2009). Diversity of carbapenem resistance mechanisms in Acinetobacter baumannii from a Taiwan hospital: spread of plasmid-borne OXA-72 carbapenemase. J. Antimicrob. Chemother. 63, 641–647. doi: 10.1093/jac/dkn553
Magiorakos, A. P., Srinivasan, A., Carey, R. B., Carmeli, Y., Falagas, M. E., Giske, C. G., et al. (2012). Multidrug-resistant, extensively drug-resistant and pandrug-resistant bacteria: an international expert proposal for interim standard definitions for acquired resistance. Clin. Microbiol. Infect. 18, 268–281. doi: 10.1111/j.1469-0691.2011.03570.x
Minami, S., Inoue, M., and Mitsuhashi, S. (1980). Purification and properties of cephalosporinase in Escherichia coli. Antimicrob. Agents Chemother. 18, 77–80. doi: 10.1128/AAC.18.1.77
Moradi, J., Hashemi, F. B., and Bahador, A. (2015). Antibiotic Resistance of Acinetobacter baumannii in Iran: a systemic review of the published literature. Osong. Public. Health. Res. Perspect. 6, 79–86. doi: 10.1016/j.phrp.2014.12.006
Mugnier, P. D., Poirel, L., Naas, T., and Nordmann, P. (2010). Worldwide dissemination of the blaOXA-23 carbapenemase gene of Acinetobacter baumannii. Emerg. Infect. Dis.16, 35–40. doi: 10.3201/eid1601.090852
Onishi, H. R., Daoust, D. R., Zimmerman, S. B., Hendlin, D., and Stapley, E. O. (1974). Cefoxitin, a semisynthetic cephamycin antibiotic: resistance to β-lactamase inactivation. Antimicrob. Agents. Chemother. 5, 38–48. doi: 10.1128/AAC.5.1.38
Peymani, A., Higgins, P. G., Nahaei, M. R., Farajnia, S., and Seifert, H. (2012). Characterisation and clonal dissemination of OXA-23-producing Acinetobacter baumannii in Tabriz, northwest Iran. Int. J. Antimicrob. Agents 39, 526–528. doi: 10.1016/j.ijantimicag.2012.02.014
Potron, A., Munoz-Price, L. S., Nordmann, P., Cleary, T., and Poirel, L. (2011). Genetic features of CTX-M-15-producing Acinetobacter baumannii from Haiti. Antimicrob. Agents Chemother. 55, 5946–5948. doi: 10.1128/AAC.05124-11
Potron, A., Poirel, L., and Nordmann, P. (2015). Emerging broad-spectrum resistance in Pseudomonas aeruginosa and Acinetobacter baumannii: mechanisms and epidemiology. Int. J. Antimicrob. Agents 45, 568–585. doi: 10.1016/j.ijantimicag.2015.03.001
Safari, M., Saidijam, M., Bahador, A., Jafari, R., and Alikhani, M. Y. (2013). High prevalence of multidrug resistance and metallo- β-lactamase (MβL) producing Acinetobacter baumannii isolated from patients in ICU wards, Hamadan, Iran. J. Res. Health Sci. 17, 162–167.
Saino, Y., Kobayashi, F., Inoue, M., and Mitsuhashi, S. (1982). Purification and properties of inducible penicillin β -lactamase isolated from Pseudomonas maltophilia. Antimicrob. Agents Chemother. 22, 564–570. doi: 10.1128/AAC.22.4.564
Sartor, A. L., Raza, M. W., Abbasi, S. A., Day, K. M., Perry, J. D., Paterson, D. L., et al. (2014). Molecular epidemiology of NDM-1-producing Enterobacteriaceae and Acinetobacter baumannii isolates from Pakistan. Antimicrob. Agents Chemother. 58, 5589–5593. doi: 10.1128/AAC.02425-14
Sawai, T., Takahashi, I., and Yamagishi, S. (1978). Iodometric assay method for β-lactamase with various β-lactam antibiotics as substrates. Antimicrob. Agents Chemother. 13, 910–913. doi: 10.1128/AAC.13.6.910
Shahcheraghi, F., Abbasalipour, M., Feizabadi, M., Ebrahimipour, G., and Akbari, N. (2011). Isolation and genetic characterization of metallo-β-lactamase and carbapenamase producing strains of Acinetobacter baumannii from patients at Tehran hospitals. Iran. J. Microbiol. 3, 68–74.
Siroy, A., Molle, V., Lemaître-Guillier, C., Vallenet, D., Pestel-Caron, M., Cozzone, A. J., et al. (2005). Channel formation by CarO, the carbapenem resistance-associated outer membrane protein of Acinetobacter baumannii. Antimicrob Agents Chemother. 49, 4876–4883. doi: 10.1128/AAC.49.12.4876-4883.2005
Sung, J. Y., Kwon, K. C., Cho, H. H., and Koo, S. H. (2011). Antimicrobial resistance determinants in imipenem-nonsusceptible Acinetobacter calcoaceticus-baumannii complex isolated in Daejeon, Korea. Korean. J. Lab. Med. 31, 265–270. doi: 10.3343/kjlm.2011.31.4.265
The European Committee on Antimicrobial Susceptibility Testing (EUCAST) (2015). Breakpoint Tables for Interpretation of MICs and Zone Diameters Version 5.0. Available online at: http://www.eucast.org/clinical_breakpoints [Accessed January 01, 2015].
Turton, J. F., Ward, M. E., Woodford, N., Kaufmann, M. E., Pike, R., Livermore, D. M., et al. (2006). The role of ISAba1 in expression of OXA carbapenemase genes in Acinetobacter baumannii. FEMS. Microbiol. Lett. 258, 72–77. doi: 10.1111/j.1574-6968.2006.00195.x
Vos, P., Hogers, R., Bleeker, M., Reijans, M., van de Lee, T., Hornes, M., et al. (1995). AFLP: a new technique for DNA fingerprinting. Nucleic.Acids. Res. 23, 4407–4414. doi: 10.1093/nar/23.21.4407
Keywords: Acinetobacter baumannii, blaOXA-23-like gene, carbapenemase, novel mutations
Citation: Bahador A, Raoofian R, Pourakbari B, Taheri M, Hashemizadeh Z and Hashemi FB (2015) Genotypic and Antimicrobial Susceptibility of Carbapenem-resistant Acinetobacter baumannii: Analysis of ISAba Elements and blaOXA-23-like Genes Including a New Variant. Front. Microbiol. 6:1249. doi: 10.3389/fmicb.2015.01249
Received: 28 June 2015; Accepted: 27 October 2015;
Published: 13 November 2015.
Edited by:
Margaret Ip, Chinese University of Hong Kong, Hong KongReviewed by:
Marcelo Tolmasky, California State University Fullerton, USAXian-Zhi Li, Health Canada, Canada
Copyright © 2015 Bahador, Raoofian, Pourakbari, Taheri, Hashemizadeh and Hashemi. This is an open-access article distributed under the terms of the Creative Commons Attribution License (CC BY). The use, distribution or reproduction in other forums is permitted, provided the original author(s) or licensor are credited and that the original publication in this journal is cited, in accordance with accepted academic practice. No use, distribution or reproduction is permitted which does not comply with these terms.
*Correspondence: Farhad B. Hashemi, hashemi@fbh.hiv