- 1National Risk Assessment Laboratory for Antimicrobial Resistance of Animal Original Bacteria, South China Agricultural University, Guangzhou, China
- 2Laboratory of Veterinary Pharmacology, College of Veterinary Medicine, South China Agricultural University, Guangzhou, China
- 3Los Angeles Biomedical Research Institute, Harbor-UCLA Medical Center, Torrance, CA, USA
- 4Division of Infectious Diseases, Department of Medicine, David Geffen School of Medicine, University of California at Los Angeles, Los Angeles, CA, USA
Biofilm formations play an important role in Staphylococcus aureus pathogenesis and contribute to antibiotic treatment failures in biofilm-associated infections. The aim of this study was to evaluate the pharmacokinetic/pharmacodynamic (PK/PD) profiles of cefquinome against an experimental catheter-related biofilm model due to S. aureus, including three clinical isolates and one non-clinical isolate. The minimal inhibitory concentration (MIC), minimal biofilm inhibitory concentration (MBIC), biofilm bactericidal concentration (BBC), minimal biofilm eradication concentration (MBEC) and biofilm prevention concentration (BPC) and in vitro time-kill curves of cefquinome were studied in both planktonic and biofilm cells of study S. aureus strains. The in vivo post-antibiotic effects (PAEs), PK profiles and efficacy of cefquinome were performed in the catheter-related biofilm infection model in murine. A sigmoid Emax model was utilized to determine the PK/PD index that best described the dose-response profiles in the model. The MICs and MBICs of cefquinome for the four S. aureus strains were 0.5 and 16 μg/mL, respectively. The BBCs (32–64 μg/mL) and MBECs (64–256 μg/mL) of these study strains were much higher than their corresponding BPC values (1–2 μg/mL). Cefquinome showed time-dependent killing both on planktonic and biofilm cells, but produced much shorter PAEs in biofilm infections. The best-correlated PK/PD parameters of cefquinome for planktonic and biofilm cells were the duration of time that the free drug level exceeded the MIC (fT > MIC, R2 = 96.2%) and the MBIC (fT > MBIC, R2 = 94.7%), respectively. In addition, the AUC24h/MBIC of cefquinome also significantly correlated with the anti-biofilm outcome in this model (R2 = 93.1%). The values of AUC24h/MBIC for biofilm-static and 1-log10-unit biofilm-cidal activity were 22.8 and 35.6 h; respectively. These results indicate that the PK/PD profiles of cefquinome could be used as valuable guidance for effective dosing regimens treating S. aureus biofilm-related infections.
Introduction
Biofilm-related infections are major medical problems and are usually refractory to antibiotic therapy (Costerton et al., 1999). The treatment failures in clinical cases are consistently reported by both clinician and veterinarians (Fabres-Klein et al., 2015). Staphylococcus aureus is a pathogen commonly associated with biofilm-related infections such as endocarditis, osteomyelitis, prosthetic joint infections, and catheter-related infections (Parra-Ruiz et al., 2012). Antibiotics that are effective against planktonic bacteria often do not prove satisfactory in eradicating biofilms, as biofilm cells are physiologically distinct from non-adherent and planktonic cells (Widmer et al., 1990). S. aureus cells within biofilm are significantly resistant to host defense systems as well as the antimicrobial therapy (Begun et al., 2007). The poor therapeutic outcome may be due to slow bacterial growth rate, limited penetration of the antibiotic and the presence of persister cells (e.g., small-colony variants) within the biofilm matrix (Davies et al., 1998). Therefore, there is a growing need for new approaches to optimize antibiotic regimens in vivo for the treatment of biofilm-related infections.
Cefquinome is a fourth generation cephalosporin which used widely in the veterinary industry with antimicrobial activity against a broad spectrum of Gram-positive and -negative bacterial species, and is regarded as highly stable to β-lactamases (CVMP, 1995). The PK/PD profiles of antibiotics could provide an important approach to establish more-effective treatment strategies and to predict the antimicrobial efficacies (Craig, 1998). However, most previous PK/PD studies were focused on planktonic cells and very limited results regarding biofilm infections were reported (Shan et al., 2014). The extrapolation of these results to biofilm cells was problematic for predicting an efficient doing regimen in biofilm-related infections (Blaser et al., 1995). Therefore, in the present study, we evaluated the in vivo PK/PD profiles of cefquinome against an experimental catheter-related biofilm infection model in murine due to three clinical S. aureus isolates and one non-clinical S. aureus isolate.
Materials and Methods
Antibiotics and Bacterial Strains
Cefquinome was pharmaceutical grade and purchased from Qilu Animal Health Products Co., Ltd. (Jinan, China). Three veterinary clinical isolates from the endocarditis cases [One methicillin-susceptible S. aureus (MSSA; S45) and two methicillin-resistant S. aureus (MRSA; M4 and M21)], and one non-clinical isolate (MSSA; F27) were included in the present study. All S. aureus strains were identified by MALDI-TOF MS system (Axima-Assurance-Shimadzu). For MRSA isolates, the 16S rRNA and mecA genes were detected using a multiplex PCR assay. MRSA strain ATCC 43300 served as a control.
In Vitro Susceptibility Testing and Biofilm Susceptibility Assay
The minimal inhibitory concentrations (MICs) of cefquinome against planktonic S. aureus cells were determined using standard Clinical and Laboratory Standards Institute (CLSI) microdilution method (CLSI, 2008). The minimal biofilm inhibitory concentrations (MBICs), biofilm bactericidal concentrations (BBCs), minimal biofilm eradication concentrations (MBECs), and biofilm prevention concentrations (BPCs) of cefquinome for biofilms were determined using the Calgary Biofilm Device as previously reported (Ceri et al., 1999; Moskowitz et al., 2004; Fernandez-Olmos et al., 2012; Macia et al., 2014; Details in Supplementary Material).
In Vitro Time-Kill Curves
The in vitro time-kill curves were determined as previously described (Zhao et al., 2014). In brief, cefquinome was added into MH broth containing approximately 5 × 105 CFU/mL exponentially growing S. aureus cells to obtain drug concentrations of 0, 0.5, 1, 2, 4, 8, and 16 × MIC, and incubated at 37°C for 24 h. Samples were removed at 0, 3, 6, 9, 12, and 24 h after incubation and then subjected to 10-fold serial dilutions. Twenty-five microliter of each dilution was then plated onto quadrants of MH agar and incubated at 37°C for 24 h for viable counts enumeration. Results were expressed as log10 CFU/mL and the limit of detection was 40 CFU/mL.
Biofilm Formation Assay
Stationary phase of S. aureus cells were resuspended in physiological NaCl solution to an OD650nm of 0.5 (∼108 CFU/mL) and diluted 1:100 into Brain Heart Infusion (BHI) broth supplemented with 0.5% glucose (Seidl et al., 2008). The 96-well plate was inoculated with 200 μL of this suspension and incubated for 18 h at 37°C. Subsequently, the wells were rinsed twice to remove planktonic cells. Biofilm was stained with crystal violet (0.1% in distilled water) for 1 min and washed with PBS three times. After visual observation, the adhering dye was dissolved with 75% alcohol to quantify the biomass measuring optical density at 650 nm.
Experimental Catheter-Associated Biofilm Infection Model in Murine
The animals used for the in vivo experiments were 6-week-old (24–27 g) and pathogen-free female ICR mice (Guangdong Medical Lab Animal Center, Guangzhou, China). Animals were maintained in accordance with the American Association for Accreditation of Laboratory Animal Care criteria. All animal experiments were approved by Animal Research Committees of South China Agricultural University.
The catheter-associated biofilm infection in a murine model was established as previously described (Kadurugamuwa et al., 2003). Briefly, 1-cm segments of 14-gage Teflon intravenous catheter (Abbocath-T; Burns Vet Supply, Vancouver, WA, USA) were infected by study S. aureus strains in 3 mL of BHI broth supplemented with 0.5% glucose. After 6–8 h incubation at 37°C, the infected catheters were washed twice with PBS to remove unbound bacteria, and then implanted subcutaneously on each side of each mouse. Our preliminary data demonstrated that the infected catheter contained ∼5 × 105 CFU/catheter (data not shown). At specific time points, tissue fluid (planktonic cells) was aspirated around each catheter segment and plated on MH agar plates for CFU determination. At sacrifice, catheters were removed from the subcutaneous tunnels and rinsed twice with PBS. Subsequently, the catheters were transferred to a separate tube containing 1 ml of sterile PBS. The tubes were placed in an ultrasonic bath (100 W, 40 kHz) and sonicated for 10 min, followed by vortexing for 1 min to remove biofilm cells from the catheter surface. The disaggregated biofilm was then processed to quantify the number of viable cells in the suspension.
Pharmacokinetics
The animals were administrated intramuscularly using varying doses of cefquinome (2, 8, 16, 32, 64, 128, or 256 mg/kg; six animals/group) as a single administration at 24 h after catheter implantation. Blood samples were collected by retro-orbital puncture following time points: 0, 0.08, 0.17, 0.25, 0.5, 0.75, 1, 2, 3, 4, and 6 h after antibiotic administration. Plasma was immediately isolated by centrifugation at 3000 × g for 10 min at 4°C, and drug concentrations in plasma were determined using a HPLC-ESI-MS/MS method as described previously (Zhou et al., 2015). The limit of quantification (LOQ) and detection were 0.01 and 0.005 μg/mL, respectively. The time-concentration curves of cefquinome were best fitted to a one-compartment model with first-order absorption. PK parameters including half-lives of first-order absorption (T1/2Ka) and elimination (T1/2Kel), volume of distribution during the terminal phase as a function of bioavailability (Vd/F), total area under time-concentration curve (AUC), body clearance as a function of bioavailability (Cl/F), the peak plasma concentration (Cmax) and the time of maximum concentration (Tmax) were conducted using WinNonlin software (version 6.1, Pharsight, St. Louis, MO, USA). The bioavailability (F) was calculated as F% = (AUCi.m./AUCi.v.) × 100% (F = 98.3%, intravenous PK data not shown). The time courses of multiple administrations in PK/PD analysis were extrapolated from the corresponding single dose PK data obtained in the present study. The protein blinding of cefquinome in mouse was previously reported (7.4%; Wang et al., 2014). In addition, cefquinome PKs in healthy animals were also determined as reference.
In Vivo Efficacy of Cefquinome in Planktonic and Biofilm Bacteria and PAEs
To evaluate the in vivo efficacy of cefquinome in the experimental catheter-related biofilm model caused by a representative MRSA strain, M4, animals were treated with a single intramuscular dose of cefquinome (8–256 mg/kg) at 24 h after infection. The control groups received physiological NaCl. At 0, 3, 6, 9, 12, and 24 h post-dosing, animals were sacrificed and tissue fluid around the catheter was collected for planktonic bacterial culture. In addition, the catheter segments were removed aseptically, and sonicated for 10 min as described above, then quantitatively cultured. MRSA densities were expressed as mean log10 CFU/mL and log10 CFU/catheter ±SD for in tissue fluid and biofilm; respectively.
The post-antibiotic effect (PAE) was calculated with the equation: PAE = T – C, where T is the time for the mean growth of 1 log10 CFU in planktonic or biofilm bacteria of treated mice after free drug levels in plasma fell below the MIC or MBIC, and C is the corresponding time for the untreated control mice (Spivey, 1992).
PD Parameter Determination in Catheter-Associated Biofilm Infection Model
To evaluate the regimens of cefquinome, including dose levels as well as dosing intervals, in planktonic and biofilm bacteria, the anesthetized mice infected with catheter segments carrying ∼5 × 105 CFU S. aureus were treated at 24 h after implantation with single or multiple intramuscular administration of cefquinome. Treatment regimens included total doses ranging from 2 to 512 mg/kg/day administered using twice-daily and once-daily. An untreated group received physiological NaCl intramuscularly. All groups of mice were sacrificed after 24 h of therapy. The catheter segments were removed and tissue fluid was collected for CFU determination as described above.
PK/PD Modeling and Data Analysis
For PK/PD integration of cefquinome in planktonic and biofilm bacteria, the surrogate indices the duration of time that free drug level exceed the MIC or MBIC (T > MIC or MBIC), Cmax/MIC or MBIC and AUC from 0 to 24 h (AUC24h)/MIC or MBIC, were calculated for each animal. The numbers of bacteria in planktonic cells and biofilms were correlated to these PK/PD indices for each of the dosing regimens studied. The in vivo PK/PD analysis was performed using the inhibitory sigmoid dose-effect model derived from the following formula: E = E0 + Emax × CeN/(EC50iN + CeN), where E0 is the change in log10 CFU/mL of untreated controls (absence of drug), Emax is the maximal antibacterial effect determined as the difference in log10 CFU/mL, EC50 is the value of the target PK/PD index required to achieve 50% of Emax, Ce is the target PK/PD indices (T > MIC or MBIC, Cmax/MIC or MBIC and AUC24h/MIC or MBIC), and N is the Hill coefficient that described the slope of dose effect curve (Aliabadi et al., 2003). The correlation between the efficacy and each of these PK/PD indices was calculated using the non-linear WinNonlin regression program (version 6.1, Pharsight; Gebru et al., 2009). R2 was used to estimate the variance of regression with each of the PK/PD parameters.
To further compare the antibacterial efficacy of cefquinome between planktonic and biofilm cells, the sigmoid dose-response model derived from the Hill equation also was used to calculate the target values of cefquinome that produced the bacteriostatic action, 0.5-log10-unit and 1-log10-unit of the net bactericidal effect over 24 h (biofilm-static, 0.5-log10-unit and 1-log10-unit biofilm-cidal values, respectively; Zhang et al., 2014).
Results
In Vitro Susceptibility Testing and Biofilm Susceptibility Assays
The biofilm susceptibility of the four S. aureus isolates is shown in Table 1. The MICs and BPCs of cefquinome for the four strains used in this study were nearly identical (0.5 and 1–2 μg/mL, respectively), indicating that cefquinome has high antibacterial activity in vitro against planktonic S. aureus cells and potential ability on preventing of early biofilm formations. The MBICs (16 μg/mL), BBCs (32–64 μg/mL) and MBECs (64–256 μg/mL) of cefquinome against the study S. aureus strain cells within biofilms were significantly higher than their corresponding MIC values. None of these parameters correlated with the methicillin-resistance status of these strains.
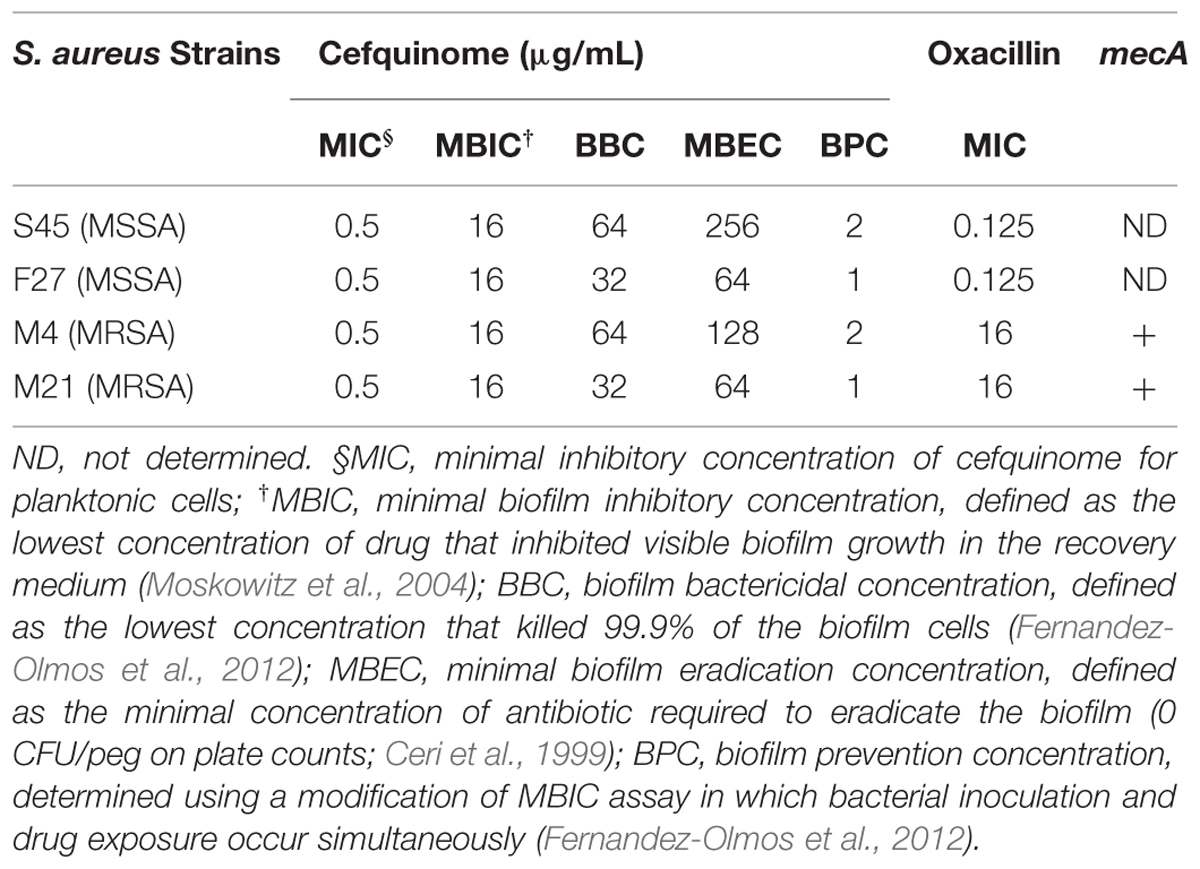
TABLE 1. In vitro susceptibility testing and biofilm susceptibility assays of cefquinome vs. Staphylococcus aureus isolates used in this study.
In Vitro Time-Kill Curves
The in vitro time-kill curves of cefquinome against planktonic MRSA-M4 were illustrated in Figure 1A. In vitro killing profiles demonstrated a time- and concentration-dependent feature. Persisting inhibition of planktonic bacterial growth was observed when S. aureus was exposed to cefquinome at a concentration of 0.5 μg/mL. At 2 × MIC and all higher concentrations of cefquinome, either bactericidal effect or elimination of S. aureus (3- or 4-log10-units reduction) was observed during 12–24 h of incubation, while less than 12 h of incubation was insufficient to eliminate all bacteria.
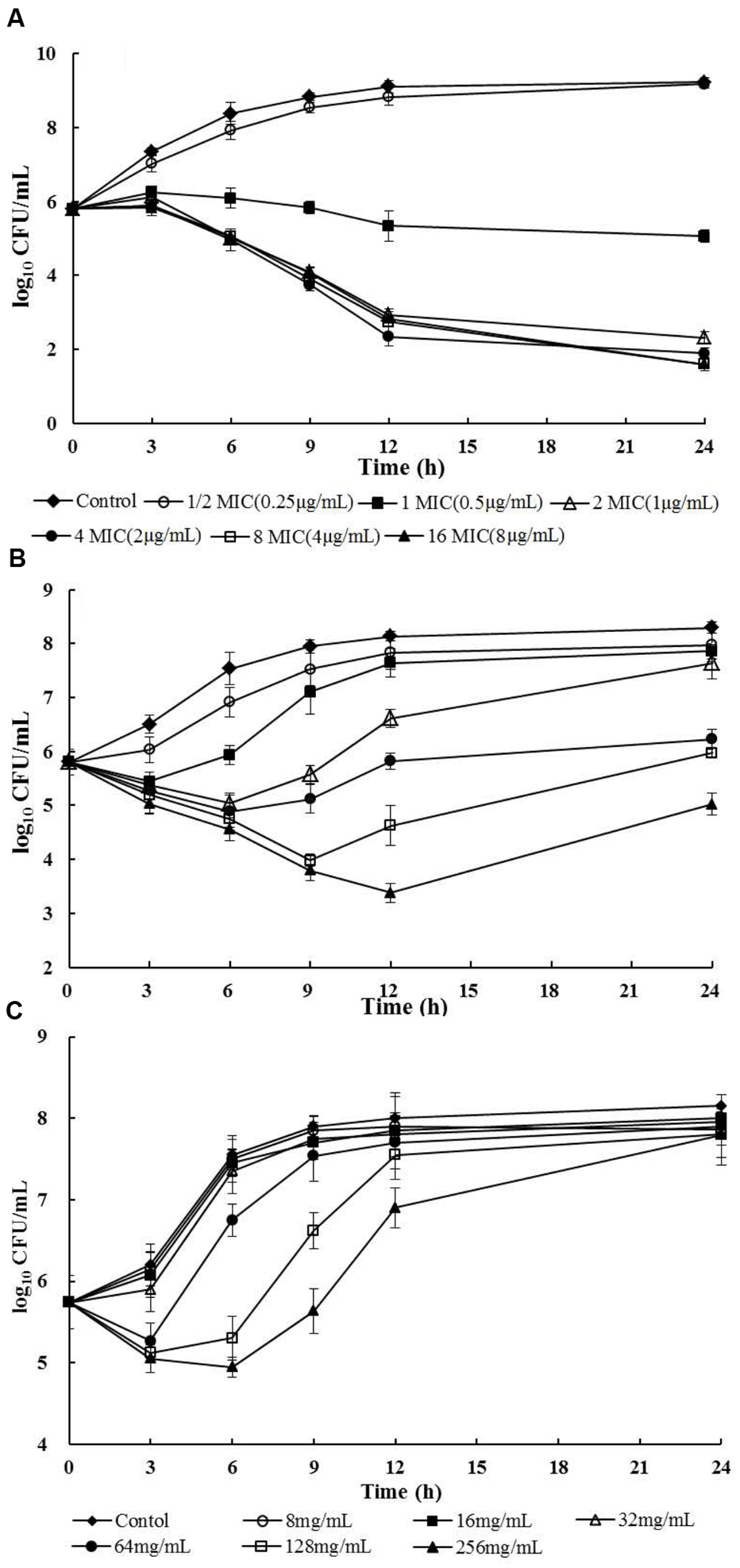
FIGURE 1. In vitro time-kill curves and in vivo treatment efficacies of cefquinome against planktonic and biofilm MRSA-M4 cells in a catheter-associated infection model after a single intramuscular dose of cefquinome (MIC = 0.5 μg/mL). (A) Cefquinome versus planktonic bacteria in vitro; (B) Cefquinome versus planktonic bacteria in vivo; (C) Cefquinome versus biofilm bacteria in vivo.
In Vitro Biofilm Formation Assays
All study strains possessed the ability to form biofilms. Interestingly, MRSA-M4 and MSSA-S45 strains formed significantly greater biofilms as compared to MRSA-M21 or MSSA-F21 strain (P < 0.01; Figure 2). However, based on the OD650nm values (OD > 2 × OD of media control; 0.2), the MRSA-M21 and MSSA-F21 strains were considered as the strong biofilm producers (Jin et al., 2006). No significant difference was observed between MRSA-M4 and MSSA-S45 biofilms.
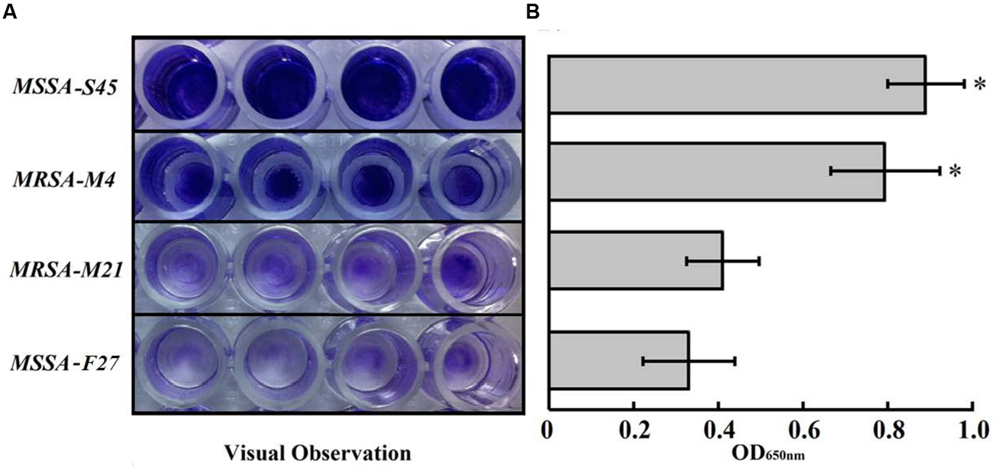
FIGURE 2. (A) Biofilm formation assays of S. aureus strains. Performed biofilm was stained with 0.1% crystal violet. (B) Quantification (OD650nm) of S. aureus biofilm formations. Results represent the mean of three independent experiments. Error bars indicate the standard deviation. ∗P < 0.01 for S45 and M4 strains versus M21 or F21 strains.
Pharmacokinetics of Cefquinome
The PK parameters of cefquinome in the representative S. aureus strain M4 infected mice after a single intramuscular dose of 2–256 mg/kg are shown in Table 2. The drug was absorbed and eliminated according to a one-compartmental model with first-order absorption (Supplementary Figure S1). A dose dependency was observed for Cmax and AUC values of cefquinome with ranges of from 3.02 to 287.6 μg/mL and 1.79 to 331.1 μg × h/mL, respectively. The Tmax varied from 0.10 to 0.19 h with a mean of 0.14 h. The elimination half-life (T1/2Kel) of cefquinome ranged from 0.22 to 0.48 h. We also demonstrated that there were no significant cefquinome PK differences between healthy and infected animals with different S. aureus strains (PK data in healthy animals not shown).
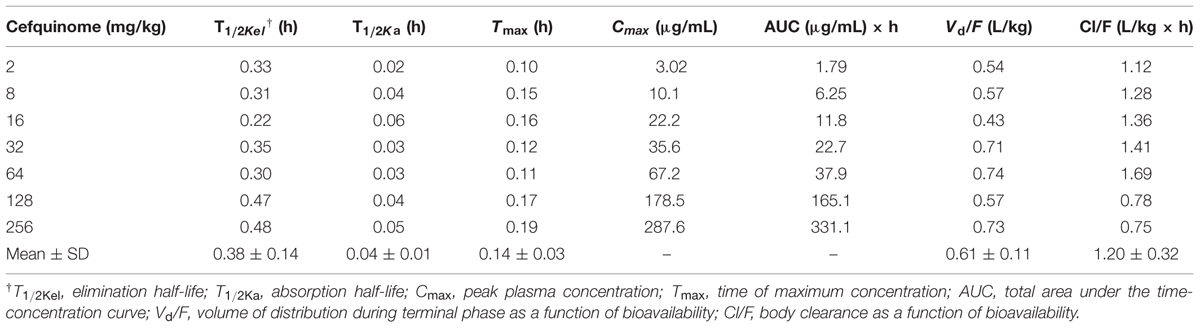
TABLE 2. PK parameters of cefquinome after a single intramuscular administration in catheter-associated biofilm infection model of mice.
Antimicrobial Efficacy of Cefquinome and PAEs In Vivo
As expected, the in vivo activity of cefquinome exhibited time-dependent features both on planktonic cells and on biofilm formations. However, a higher cefquinome administration was acquired to suppress the regrowth of biofilms. The dosage regimen of cefquinome at 256 mg/kg inhibited the planktonic cells for 24 h, but regrowth was observed in the biofilm infections at the same time point (Figures 1B,C). Importantly, an approximately 3-log10 CFU reduction occurred for planktonic cells, while about 1.5 log10 CFU increased in the biofilm infection following 12 h treatment with cefquinome at 256 mg/kg (Figures 1B,C).
A positive relationship between cefquinome dosing and PAEs was seen in planktonic S. aureus cells (R2 = 94.9%). However, less than 1 h PAE with the dose range of cefquinome (8–256 mg/kg) was demonstrated for the biofilm cells (Table 3). Therefore, significant longer PAE of planktonic S. aureus cells was observed as compared to bacteria cells within biofilms (P < 0.01; Table 3).
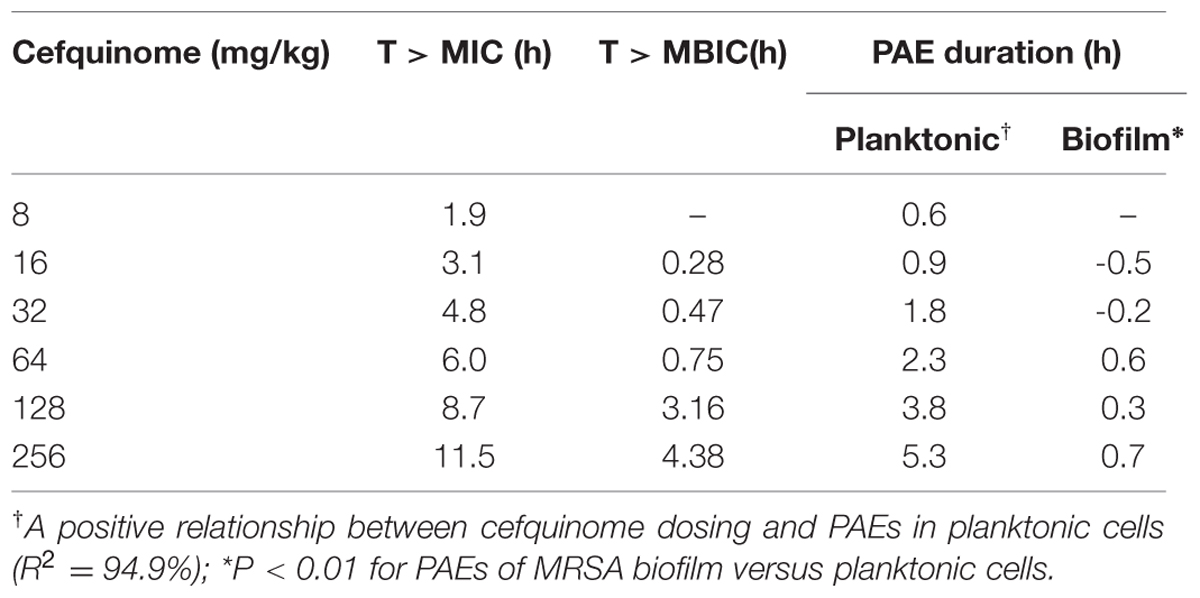
TABLE 3. PAE durations of cefquinome against MRSA-M4 after a single dose of administration in catheter-associated biofilm infection model of mice.
PK/PD Parameters Integration and Determination
Integration of PK/PD indices of cefquinome with planktonic and biofilm bacteria are showed in Table 4. The PK/PD parameters of planktonic cells were significantly greater as compared with the PK/PD profiles of biofilm when single doses of cefquinome at 2–256 mg/kg were administrated (P < 0.05; Table 4). For instance, T > MICs were ranged from 1.2 to 11.5 h, but the T > MBICs were from 0.28 to 4.38 h. In addition, Cmax/MICs and Cmax/MBICs were from 6.1 to 575.1 and 0.19 to 17.9; respectively. More importantly, the AUC/MICs were significantly larger than AUC/MBICs (3.6–662 h vs. 0.11–20.7 h).
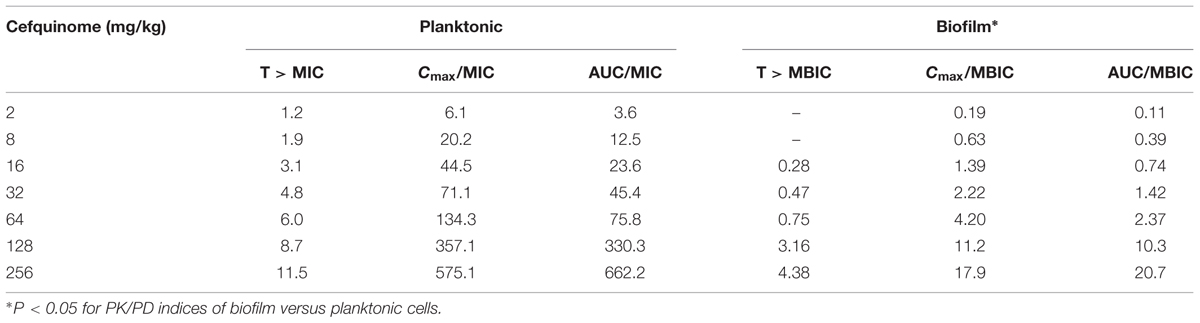
TABLE 4. Integration of PK/PD indices of cefquinome against planktonic and biofilm bacteria in S. aureus catheter-associated biofilm infection model of mice (MRSA-M4).
The relationship of the change of CFU and the PK/PD parameters of cefquinome between planktonic and biofilm cells was shown in Figure 3. T > MIC was the PK/PD index that best correlated with antimicrobial efficacy for planktonic cells (R2 = 96.2%; Figure 3A). However, for biofilm infections, the T > MBIC index (R2 = 94.7%) showed a better correlation than the T > MIC (R2 = 91.9%; Figures 3A,D). Interestingly, the AUC/MIC parameter exhibited a strong correlation with the in vivo efficacy of cefquinome for biofilm cells (R2 = 91.1%), but a poor correlation for planktonic bacteria (R2 = 68.6%; Figure 3C).
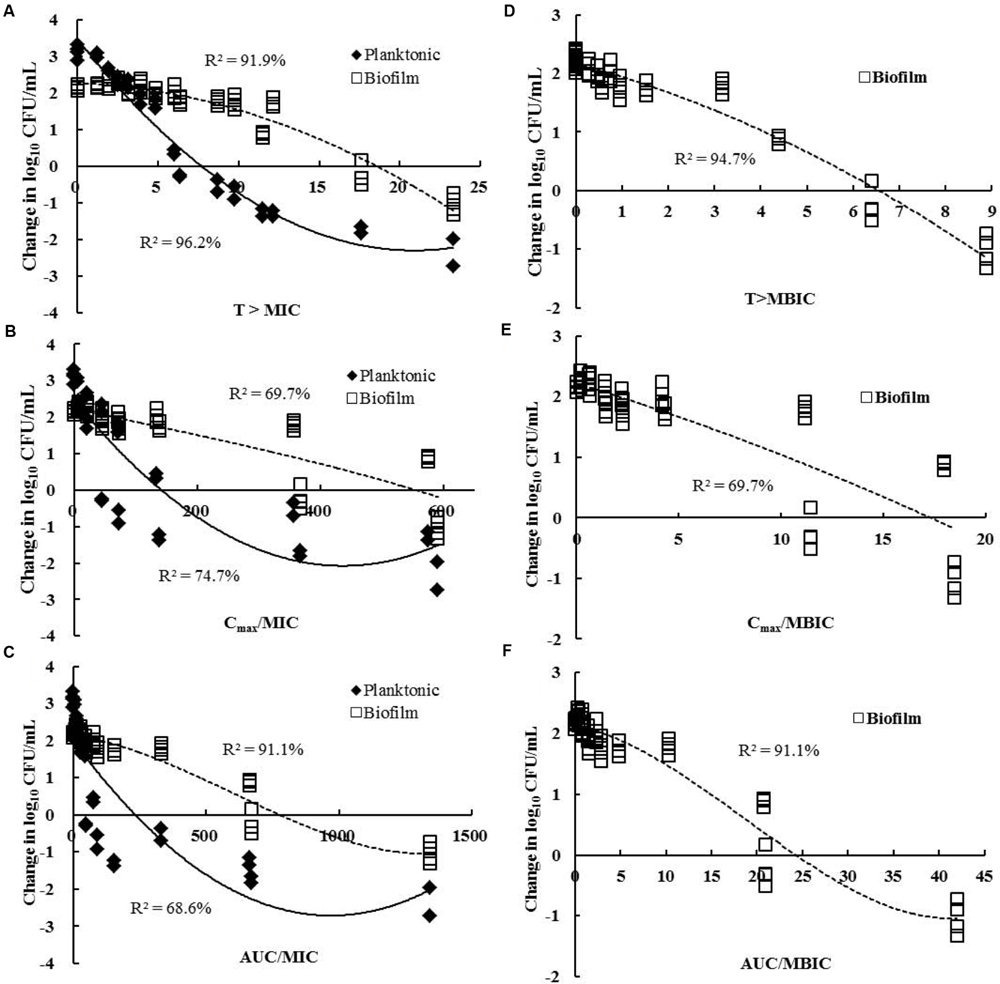
FIGURE 3. Relationships between the change in log10 number of CFU for MRSA-M4 and PK/PD parameters of cefquinome. Each symbol represents the data from each catheter segment or tissue fluid. (A) T > MIC; (B) Cmax/MIC; (C) AUC24h/MIC; (D) T > MBIC; (E) Cmax/MBIC; (F) AUC24h/MBIC. The R2 value is the determination coefficient. Data points below the horizontal line represent killing and points above the horizontal line represent growth. Planktonic cells, filled diamonds; biofilms, and open squares.
PK/PD Model Parameter Estimates for the Target Efficacy Against Study Isolates
The PK/PD indices and the corresponding target values of cefquinome required to achieve various efficacies against S. aureus M4 in planktonic cells and biofilm infections are listed in Table 5. The EC50 value of cefquinome was 6.61 h for planktonic cells versus 17.4 h for a biofilm infection (P < 0.01). The 1-log10-unit reduction effect of cefquinome required value of T > MIC at least 10.4 h for planktonic cells, but 22.7 h for biofilm formations (T > MBIC; 8.76 h; Table 5). The in vivo dose-effect relationship of cefquinome for three additional strains of S. aureus in both planktonic and biofilm cells were also calculated using the inhibitory sigmoid Emax model and a similar result was observed (Supplementary Table S1).

TABLE 5. PK/PD model parameter estimates and target values of cefquinome for T > MIC or MBIC required to achieve the various antibacterial efficacies against a representative MRSA isolates M4 in planktonic or in catheter-associated biofilm infection model.
In order to predict an effective dose, we determined the dose-response relationships between PK/PD index (AUC24h/MBIC) and the in vivo efficacy of cefquinome. The dose response curve strongly correlated with the AUC24h/MBIC index for all study S. aureus biofilm infections (R2 = 93.1%; Supplementary Figure S2). The target values of cefquinome necessary to produce a biofilm-static action and a 1-log10-unit biofilm-cidal action as well as the corresponding AUC24h/MBIC index values were listed in Table 6. The mean values of AUC24h/MBIC associated with stasis and 1-log10-unit reduction were 22.8 and 35.6 h; respectively.
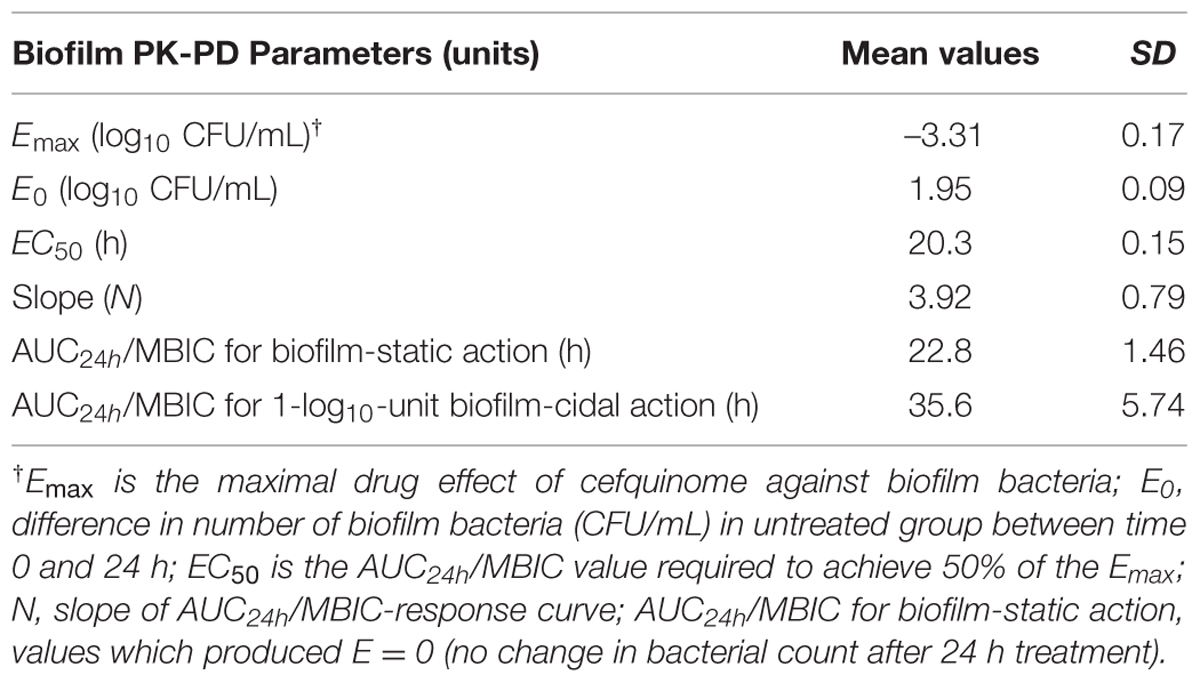
TABLE 6. In vivo pharmacokinetics and pharmacodynamics model of cefquinome against a S. aureus catheter-associated biofilm infection using AUC24h/MBIC as the predictive PK-PD index (all study S. aureus strains included).
Discussion
In this study, an experimental catcher-associated biofilm murine infection model was developed for evaluation of the PK/PD profiles of cefquinome against S. aureus, including MSSA and MRSA strains, growing as planktonic cells and within biofilms. To the best of our knowledge, the present study represents the first time PK/PD evaluation of cefquinome in vivo in S. aureus biofilm-related infections.
Several key insights emerged from this study. First, we investigated in vivo PAEs of cefquinome in a catheter-related biofilm infection model due to S. aureus, including MSSA and MRSA strains, and observed that the PAEs in biofilms showed significantly shorter than those in planktonic cells (Table 3). It is well accepted that the importance of long PAEs for optimizing treatment regimens in clinical practice (Spivey, 1992). Thus, longer PAE is positively correlated with greater S. aureus counts reduction in the catheter-related biofilm model which is consistent with previous reports (den Hollander et al., 1998; Ahmad et al., 2015). For instance, a recent study developed in a neutropenic mouse thigh model demonstrated that exposure of planktonic S. aureus ATCC 29213 cells to cefquinome led to a visibly longer PAE of 2.9 h as compared to below 1 h PAE observed in vitro (Wang et al., 2014). Similarly, another study also proved that the longer in vitro PAE could achieve a better in vivo treatment efficacy in a rabbit meningitis model (Tauber et al., 1984).
In addition, we demonstrated that cefquinome was fairly effective, causing ∼2- to 3-log10 CFU reductions for planktonic cells but only ∼1.0- to 1.5-log10 CFU reductions for biofilm cells within the catheter. This observation proved that higher concentrations and longer treatment times of cefquinome are requested to kill S. aureus cells within biofilm as compared with planktonic cells in the catheter-associated biofilm infections in murine duo to S. aureus strains. Unfortunately, the treatment strategies aimed at eradicating biofilm cells was not achievable in the present model, even at the highest dosing regimen (256 mg/kg; q12h). The lack of the antimicrobial efficacy for biofilm-related infections may be related to intrinsic tolerance of biofilm-grown bacterial populations to antibiotics, as well as the poor penetration of cefquinome through extracellular polymeric matrix of biofilm formations and inadequate antibiotic-exposure for bacterial cells embedded in biofilms (Macia et al., 2014). Other mechanisms such as the contributions of matrix components and chromosomal β-lactamase increases may also lead to this resistance (Hengzhuang et al., 2013).
It is notable that we were able to take advantage of the PK/PD parameters to optimize the in vivo efficacy against planktonic cells, as well as biofilm infections in the catheter-related biofilm model. The dose-response profiles of cefquinome for planktonic and biofilm cells showed the best correlation with T > MIC and T > MBIC; respectively (R2, 96.2% for planktonic cells and 94.7% for biofilms; Figures 3A,D). More recently, Wang et al. (2014) investigated the pharmacodynamics of cefquinome for planktonic MRSA infections and also noted that the same index (T > MIC) correlated the best with treatment efficacy in a murine thigh infection model. In our study, although the T > MBIC index exhibited the best correlation, the AUC/MIC of cefquinome was significantly correlated with biofilm killing (R2 = 91.1%) as compared to planktonic cell infections (R2 = 68.6%; Figure 3C). This was almost equivalent with the correlation of the T > MIC for biofilms (R2 = 91.9%). Interestingly, the AUC/MBIC index which is based on the antibiotic susceptibility assay of biofilms showed a significant correlation with the in vivo efficacy for all study S. aureus biofilm cells infections (R2 = 93.1%). Additionally, considering the higher dosage and longer the treatment periods required to treat biofilm infections in vivo, our results suggest that the AUC/MBIC would be the recommended predictive PK/PD index in computation of dosing regimens for S. aureus biofilm-related infections.
The development of device-related biofilm infections is correlated with the density of adherent cells on the implant surfaces (Joo and Otto, 2012). Once the bacterial density increases and the colony changes to biofilm formation, the eradication is virtually impossible in vivo (Fernandez-Olmos et al., 2012). Therefore, the important treatment strategy for biofilm-producing bacteria is to prevent the progression of early biofilm formation instead of finding a final dosing regimen for eradication of the mature biofilms. In this study, the BPCs of cefquinome for all study S. aureus isolates were only slightly higher than their MICs, which is an interesting parameter that could be used with the aim of reducing the cell density to prevent biofilm formation (Macia et al., 2014). More importantly, the present PK/PD model on S. aureus biofilms provided an AUC24h/MBIC ratio of 35.6 h that could theoretically predict 1-log10 CFU biofilm-cidal effect. These results in conjunction with BPC90 data of S. aureus biofilms may provide an additional approach to the design of dosing regimens that prevent the early stages of biofilms caused by planktonic S. aureus cells during antibiotic treatment. Additionally, a recent study also showed that daptomycin and rifampin alone and in combination were successful in preventing S. aureus biofilm infections at the early stages in a subcutaneous rat pouch model (Cirioni et al., 2010).
For the treatment of biofilm infections, more frequent administrations are needed to obtain a longer treatment period in form of T > MBIC. However, it is usually impractical to administer drug more frequently in the veterinary clinical trials. Routinely, once-daily or twice-daily schedule is considered a good compliance target. Furthermore, cefquinome (and the majority of β-lactams in general) have short elimination half-lives and the limitations are obvious with this routine dosing strategy (Turnidge, 1998). Thus, new formulations of cefquinome with prolonged half-life profiles should be developed to achieve a better therapeutic outcome in biofilm-related infections.
Our investigations have several limitations. For example, only four representative S. aureus strains were evaluated in this study. Thus, the results need to be verified in a larger population of strains. In addition, the combination regimens were not tested against S. aureus biofilm infections model. Studies to define the efficacy of antibiotic combinations in the same catheter-related biofilm model are ongoing in our laboratory. Nonetheless, this is the first study to our best knowledge to demonstrate PK/PD relationships of cefquinome against MSSA and MRSA growing as planktonic and biofilm cells in an in vivo experimental catcher-associated biofilm infection model. In the present study, cefquinome showed time-dependent activities against S. aureus biofilms in vitro as well as in vivo. In addition, significantly shorter PAEs were observed on biofilms vs. planktonic cells. The PK/PD index of cefquinome that best correlated with anti-biofilm efficacy was the T > MBIC in this study. More importantly, the AUC/MBIC of cefquinome also significantly correlated with therapeutic outcomes for biofilm-related infections. These results could potentially provide a new perspective for establishing appropriate strategies of antibiotic treatment in S. aureus biofilm related formation.
Author Contributions
Y-HL conceived of this study and participated in its design and coordination. Y-FZ designed the experiment and drafted the manuscript. Y-FZ, WS, and YY carried out the in vivo animal experiments and in vitro time-kill curve studies. M-TT carried out the in vivo experiment about the additional non-clinical S. aureus strain in the revision of manuscript. YX and JS participated in the data analysis and revision of manuscript. All authors read and approved the final manuscript.
Conflict of Interest Statement
The authors declare that the research was conducted in the absence of any commercial or financial relationships that could be construed as a potential conflict of interest.
Acknowledgments
This work was supported by the National Science Fund for Distinguished Young Scholars (grant no. 31125026); Program for Changjiang Scholars and Innovative Research Team in University of Ministry of Education of China (grant no. IRT13063); the Natural Science Foundation of Guangdong Province (grant no. S2012030006590); Science and Technology Planning Project of Guangdong Province China (grant no. 2012A020800004).
Supplementary Material
The Supplementary Material for this article can be found online at: http://journal.frontiersin.org/article/10.3389/fmicb.2015.01513
References
Ahmad, I., Hao, H., Huang, L., Sanders, P., Wang, X., Chen, D., et al. (2015). Integration of PK/PD for dose optimization of Cefquinome against Staphylococcus aureus causing septicemia in cattle. Front. Microbiol. 6:588. doi: 10.3389/fmicb.2015.00588
Aliabadi, F. S., Ali, B. H., Landoni, M. F., and Lees, P. (2003). Pharmacokinetics and PK-PD modelling of danofloxacin in camel serum and tissue cage fluids. Vet. J. 165, 104–118. doi: 10.1016/S1090-0233(02)00258-7
Begun, J., Gaiani, J. M., Rohde, H., Mack, D., Calderwood, S. B., Ausubel, F. M., et al. (2007). Staphylococcal biofilm exopolysaccharide protects against Caenorhabditis elegans immune defenses. PLoS Pathog. 3:e57. doi: 10.1371/journal.ppat.0030057
Blaser, J., Vergeres, P., Widmer, A. F., and Zimmerli, W. (1995). In vivo verification of in vitro model of antibiotic treatment of device-related infection. Antimicrob. Agents Chemother. 39, 1134–1139. doi: 10.1128/AAC.39.5.1134
Ceri, H., Olson, M. E., Stremick, C., Read, R. R., Morck, D., and Buret, A. (1999). The calgary biofilm device: new technology for rapid determination of antibiotic susceptibilities of bacterial biofilms. J. Clin. Microbiol. 37, 1771–1776.
Cirioni, O., Mocchegiani, F., Ghiselli, R., Silvestri, C., Gabrielli, E., Marchionni, E., et al. (2010). Daptomycin and rifampin alone and in combination prevent vascular graft biofilm formation and emergence of antibiotic resistance in a subcutaneous rat pouch model of staphylococcal infection. Eur. J. Vasc. Endovasc. Surg. 40, 817–822. doi: 10.1016/j.ejvs.2010.08.009
CLSI (2008). Performance Standards for Antimicrobial Disk and Dilution Susceptibility Tests for Bacteria Isolated From Animals: Approved Standard, 3rd Edition. Wayne, PA: CLSI.
Costerton, J. W., Stewart, P. S., and Greenberg, E. P. (1999). Bacterial biofilms: a common cause of persistent infections. Science 284, 1318–1322. doi: 10.1126/science.284.5418.1318
Craig, W. A. (1998). Pharmacokinetic/pharmacodynamic parameters: rationale for antibacterial dosing of mice and men. Clin. Infect. Dis. 26, 1–10. doi: 10.1086/516284
CVMP (1995). Cefquinome Summary Report EMEA/MRL/005/95. London: The European Agency for the Evaluation of Medicinal Products.
Davies, D. G., Parsek, M. R., Pearson, J. P., Iglewski, B. H., Costerton, J. W., and Greenberg, E. P. (1998). The involvement of cell-to-cell signals in the development of a bacterial biofilm. Science 280, 295–298. doi: 10.1126/science.280.5361.295
den Hollander, J. G., Fuursted, K., Verbrugh, H. A., and Mouton, J. W. (1998). Duration and clinical relevance of postantibiotic effect in relation to the dosing interval. Antimicrob. Agents Chemother. 42, 749–754.
Fabres-Klein, M. H., Caizer Santos, M. J., Contelli Klein, R., Nunes De Souza, G., De Oliveira Barros, and Ribon, A. (2015). An association between milk and slime increases biofilm production by bovine Staphylococcus aureus. BMC Vet. Res. 11:3. doi: 10.1186/s12917-015-0319-7
Fernandez-Olmos, A., Garcia-Castillo, M., Maiz, L., Lamas, A., Baquero, F., and Canton, R. (2012). In vitro prevention of Pseudomonas aeruginosa early biofilm formation with antibiotics used in cystic fibrosis patients. Int. J. Antimicrob. Agents 40, 173–176. doi: 10.1016/j.ijantimicag.2012.04.006
Gebru, E., Lee, J. S., Chang, Z. Q., Hwang, M. H., Cheng, H., and Park, S. C. (2009). Integration of pharmacokinetic and pharmacodynamic indices of orbifloxacin in beagle dogs after a single intravenous and intramuscular administration. Antimicrob. Agents Chemother. 53, 3024–3029. doi: 10.1128/AAC.01346-08
Hengzhuang, W., Ciofu, O., Yang, L., Wu, H., Song, Z., Oliver, A., et al. (2013). High beta-lactamase levels change the pharmacodynamics of beta-lactam antibiotics in Pseudomonas aeruginosa biofilms. Antimicrob. Agents Chemother. 57, 196–204. doi: 10.1128/AAC.01393-12
Jin, H., Zhou, R., Kang, M., Luo, R., Cai, X., and Chen, H. (2006). Biofilm formation by field isolates and reference strains of Haemophilus parasuis. Vet. Microbiol. 118, 117–123. doi: 10.1016/j.vetmic.2006.07.009
Joo, H. S., and Otto, M. (2012). Molecular basis of in vivo biofilm formation by bacterial pathogens. Chem. Biol. 19, 1503–1513. doi: 10.1016/j.chembiol.2012.10.022
Kadurugamuwa, J. L., Sin, L., Albert, E., Yu, J., Francis, K., Deboer, M., et al. (2003). Direct continuous method for monitoring biofilm infection in a mouse model. Infect. Immun. 71, 882–890. doi: 10.1128/IAI.71.2.882-890.2003
Macia, M. D., Rojo-Molinero, E., and Oliver, A. (2014). Antimicrobial susceptibility testing in biofilm-growing bacteria. Clin. Microbiol. Infect. 20, 981–990. doi: 10.1111/1469-0691.12651
Moskowitz, S. M., Foster, J. M., Emerson, J., and Burns, J. L. (2004). Clinically feasible biofilm susceptibility assay for isolates of Pseudomonas aeruginosa from patients with cystic fibrosis. J. Clin. Microbiol. 42, 1915–1922. doi: 10.1128/JCM.42.5.1915-1922.2004
Parra-Ruiz, J., Bravo-Molina, A., Pena-Monje, A., and Hernandez-Quero, J. (2012). Activity of linezolid and high-dose daptomycin, alone or in combination, in an in vitro model of Staphylococcus aureus biofilm. J. Antimicrob Chemother. 67, 2682–2685. doi: 10.1093/jac/dks272
Seidl, K., Goerke, C., Wolz, C., Mack, D., Berger-Bachi, B., and Bischoff, M. (2008). Staphylococcus aureus CcpA affects biofilm formation. Infect. Immun. 76, 2044–2050. doi: 10.1128/IAI.00035-08
Shan, Q., Liang, C., Wang, J., Li, J., and Zeng, Z. (2014). In vivo activity of cefquinome against Escherichia coli in the thighs of neutropenic mice. Antimicrob. Agents Chemother. 58, 5943–5946. doi: 10.1128/AAC.03446-14
Tauber, M. G., Zak, O., Scheld, W. M., Hengstler, B., and Sande, M. A. (1984). The postantibiotic effect in the treatment of experimental meningitis caused by Streptococcus pneumoniae in rabbits. J. Infect. Dis. 149, 575–583. doi: 10.1093/infdis/149.4.575
Turnidge, J. D. (1998). The pharmacodynamics of beta-lactams. Clin. Infect. Dis. 27, 10–22. doi: 10.1086/514622
Wang, J., Shan, Q., Ding, H., Liang, C., and Zeng, Z. (2014). Pharmacodynamics of cefquinome in a neutropenic mouse thigh model of Staphylococcus aureus infection. Antimicrob. Agents Chemother. 58, 3008–3012. doi: 10.1128/AAC.01666-13
Widmer, A. F., Frei, R., Rajacic, Z., and Zimmerli, W. (1990). Correlation between in vivo and in vitro efficacy of antimicrobial agents against foreign body infections. J. Infect. Dis. 162, 96–102. doi: 10.1093/infdis/162.1.96
Zhang, B. X., Lu, X. X., Gu, X. Y., Li, X. H., Gu, M. X., Zhang, N., et al. (2014). Pharmacokinetics and ex vivo pharmacodynamics of cefquinome in porcine serum and tissue cage fluids. Vet. J. 199, 399–405. doi: 10.1016/j.tvjl.2013.12.015
Zhao, D. H., Zhou, Y. F., Yu, Y., Shi, W., Yang, X., Xiao, X., et al. (2014). Integration of pharmacokinetic and pharmacodynamic indices of valnemulin in broiler chickens after a single intravenous and intramuscular administration. Vet. J. 201, 109–115. doi: 10.1016/j.tvjl.2014.05.010
Keywords: biofilms, Staphylococcus aureus, PK/PD, cefquinome, catheter-associated infection
Citation: Zhou Y-F, Shi W, Yu Y, Tao M-T, Xiong YQ, Sun J and Liu Y-H (2016) Pharmacokinetic/Pharmacodynamic Correlation of Cefquinome Against Experimental Catheter-Associated Biofilm Infection Due to Staphylococcus aureus. Front. Microbiol. 6:1513. doi: 10.3389/fmicb.2015.01513
Received: 13 September 2015; Accepted: 15 December 2015;
Published: 07 January 2016.
Edited by:
Andres M. Perez, University of Minnesota, USAReviewed by:
A. Gnanamani, Central Leather Research Institute, IndiaMargaret Ip, Chinese University of Hong Kong, Hong Kong
Copyright © 2016 Zhou, Shi, Yu, Tao, Xiong, Sun and Liu. This is an open-access article distributed under the terms of the Creative Commons Attribution License (CC BY). The use, distribution or reproduction in other forums is permitted, provided the original author(s) or licensor are credited and that the original publication in this journal is cited, in accordance with accepted academic practice. No use, distribution or reproduction is permitted which does not comply with these terms.
*Correspondence: Ya-Hong Liu, bHloQHNjYXUuZWR1LmNu