- 1Department of Food Safety, Istituto Zooprofilattico Sperimentale delle Venezie, Legnaro, Italy
- 2Department of Animal Medicine, Production and Health, Università di Padova, Padova, Italy
- 3Department of Information Engineering, Università di Padova, Padova, Italy
Listeria monocytogenes is a serious foodborne pathogen that can contaminate food during processing and can grow during food shelf-life. New types of safe and effective food contact materials embedding antimicrobial agents, like silver, can play an important role in the food industry. The present work aimed at evaluating the in vitro growth kinetics of different strains of L. monocytogenes in the presence of silver, both in its ionic and nano form. The antimicrobial effect was determined by assaying the number of culturable bacterial cells, which formed colonies after incubation in the presence of silver nanoparticles (AgNPs) or silver nitrate (AgNO3). Ionic release experiments were performed in parallel. A different reduction of bacterial viability between silver ionic and nano forms was observed, with a time delayed effect exerted by AgNPs. An association between antimicrobial activity and ions concentration was shown by both silver chemical forms, suggesting the major role of ions in the antimicrobial mode of action.
Introduction
Listeria monocytogenes is a foodborne pathogen responsible for severe disease in immunocompromised people and for non-invasive infections in immunocompetent individuals (Crum, 2002). Its incidence has been estimated as 0.41 and 0.26 cases per 100,000 inhabitants in Europe in 2012 (EFSA and ECDC, 2014) and in the US in 2013 (CDC, 2014), respectively. Despite the low incidence rate, L. monocytogenes is ranked among the top-most serious foodborne pathogens due to its high pathogenicity, as is shown by methods for the quantification of disease burden, such as DALY/100,000 cases (Havelaar et al., 2012). Due to its widespread presence in the environment, L. monocytogenes has a high potential to contaminate food during processing and distribution (Latorre et al., 2007). Ready-to-eat foods (RTE) pose a main risk for human listeriosis infection due to the lack of effective decontamination treatment (e.g., heat), which otherwise would inactivate viable L. monocytogenes cells (Parisi et al., 2010; Lomonaco et al., 2011), or due to the potential for recontamination after applied treatment.
The Scientific Committee on Veterinary Measures relating to Public Health (SCVPH) has recommended, as an objective for public health, that the level of L. monocytogenes in food be below 100 cfu/g (Reg. 2073/2005). This safety criterion has been set in relevant EU regulations for RTE foods during their entire shelf life. US food policy on L. monocytogenes is characterized by more stringent requirements regarding the monitoring process in processing plants, in order to avoid food contamination (FSIS, 2014).
Nevertheless, the ability of Listeria spp. to grow between 0 and 4°C and its tolerance to some commonly applied preservatives, like salt, explain the challenge of applying alternative control strategies along the whole food chain (Latorre et al., 2007).
The shelf life for such products is often calculated based on the kinetics of bacterial growth. Temperature control is critical in the overall control of L. monocytogenes in RTE foods. However, lack of proper control at commercial (production, transport, retail) or consumer levels can result in failure to control growth of the pathogen.
In this context, the development of new types of safe and effective food contact materials able to extend the shelf life of food products or to prevent cross contamination is of great interest. Among different chemicals with antimicrobial activity, silver is considered as a good candidate, as it is known to exert antimicrobial properties toward both Gram positive and Gram negative bacteria (Silver, 2003; Rai et al., 2009; Bae et al., 2010; Fayaz et al., 2010; You et al., 2011; Maillard and Hartemann, 2013).
Previous studies have shown that nanoparticles (NPs) could be used as effective bactericidal materials in antimicrobial formulations due to their enhanced reactivity, resulting from their high surface/volume ratio (Pal et al., 2007; Choi et al., 2008; Park et al., 2009). In particular, silver in NP form (AgNPs) is known to exhibit strong biocidal effects on different bacterial species (Sondi and Salopek-Sondi, 2004; Rai et al., 2009; Losasso et al., 2014; Berton et al., 2015), including multidrug resistant bacteria (Lara et al., 2010). However, it has been demonstrated that not only different bacterial species but also different strains within one species can show different sensitivities to AgNPs (Losasso et al., 2014). The advantage of using AgNPs has been investigated under real life scenarios to increase fruit shelf life (Gudadhe et al., 2014) and to reduce the contamination by coliforms in cheese (Beigmohammadi et al., 2016). However, to our knowledge the antimicrobial effect of AgNPs against L. monocytogenes has never been proved.
Nevertheless, data arising from studies on the effectiveness of NPs as antimicrobials are difficult to interpret due to the specific chemical and physical characteristics of these particles. Thus, it is of primary importance to provide detailed characterization of any tested NPs starting from size and shape evaluation, as this would allow the scientific community to compare the results.
The aims of this work were to evaluate the efficacy of AgNPs as an antimicrobial toward L. monocytogenes and to explore intra-species variability and its relation with antimicrobial resistance. Moreover, the effectiveness of AgNPs as an antimicrobial material is discussed in the light of its mechanism of action.
Materials and Methods
Nanoparticles and Chemicals
Silver nanoparticles colloidal suspension in citrate 2 mM (20 nm Citrate BioPure™ Silver, batch numbers: MGM2167 e KJW1896) and their control dispersant medium were purchased from Nanocomposix (San Diego, CA, USA). Silver nitrate (AgNO3) 1000 mg L–1 stock solution was purchased from Ultra Scientific (North Kingstown, RI, USA).
L. monocytogenes Isolates
Twenty L. monocytogenes wild isolates, originating from different food matrices, were obtained from the collection of pathogenic microorganisms of the Food Microbiology laboratory of the Istituto Zooprofilattico Sperimentale delle Venezie (Legnaro, Italy); details are presented in Table 1. L. monocytogenes ATCC® 13932TM was also examined. The isolates were stored in Microbank™ (Pro-Lab Diagnostics, US) at -80°C until needed. All strains were identified as L. monocytogenes according to ISO standard (ISO, 1998).
Size and Morphology of AgNPs
Morphological parameters of AgNPs were verified using a transmission electron microscope (TEM) JEM 2000 EX II (JEOL®) (120 KV) at the Department of Technology and Management of Industrial Systems (University of Padua). AgNPs were diluted with Muller Hinton Broth (MHB) culture media to reach a final concentration of 50 mg/L. TEM observations were performed at 6000X and 100KX and microphotographs were elaborated using ImageJ® software, enabling evaluation of average particle diameter and roundness for a sample of 500 particles.
Antibiotics Susceptibility Testing
Listeria monocytogenes isolates were tested for susceptibility to antimicrobials (detailed in Supplementary Table 1), using a commercial microdilution test (Sensititre® Streptococcus panel ITST4F) according to the manufacturer’s recommendations. The results were visually evaluated after 48 h of incubation at 37°C and the minimum inhibitory concentration (MIC) was defined as the lowest concentration able to completely inhibit bacterial growth.
Silver Susceptibility Assay
In this experiment a two steps susceptibility assay was performed.
First Step
The different susceptibility of the 21 L. monocytogenes isolates to different concentrations of AgNPs and AgNO3 was assessed as follows. L. monocytogenes isolates from Microbank™ were subcultured in Tryptone Soya supplemented with yeast and incubated at 37°C overnight. The day after a loopful was transferred to 15 ml of Mueller Hinton Broth (MHB) and incubated at 37°C overnight to produce the inocula. MHB was used as culture medium with the objective of obtaining a good compromise between L. monocytogenes growth and NPs stability, given that this is a quite simple and non-selective liquid medium, specifically designed for susceptibility studies and having the minimum requirements for bacteria growth (Gilbert et al., 1987). The final liquid culture in MHB consisted of L. monocytogenes in the Lag phase (OD A600 = 0.3 = 108 CFU/ml) (vlab.amrita.edu, 2011; CLSI, 2012) in the presence of different concentration of AgNPs (100, 50 mg/L–1) or AgNO3 (60, 30, 5 mg/L–1). The tubes were incubated in an orbital shaker at 200 rpm at 37°C for 4 h. A positive control (flask containing AgNPs and MHB) and a negative control (flask containing inoculum and MHB) were included. The high rotary shaking speed was selected to prevent the aggregation and settlement of the NPs over the incubation period and Ag concentration levels were selected according to previous observations with Salmonella (Losasso et al., 2014).
After 4 h of incubation, a volume of 50 μl was removed from liquid culture and, after serial 10-fold dilutions in MHB broth up to 10–8 of L. monocytogenes, 100 μl aliquots were spread-plated on ALOA Agar according to Ottaviani et al. (1997). Colonies were counted after incubation of spread-plates at 37°C for 48 h, and counts were expressed as log CFU/ml.
Second Step
The time dependent evaluation of the effectiveness of silver as an antilisterial on selected L. monocytogenes isolates was evaluated. A cell culturability assay was carried out as described above, with different concentrations of AgNPs (300 mg/L–1) and AgNO3 (30, 20, and 10 mg/L–1) at different time points (1, 4, 24, 48, and 72 h). Each experiment was performed in triplicate. Positive and negative controls were included.
Ionic Release
Listeria monocytogenes (strain 5) liquid culture were prepared as in the antimicrobial susceptibility assay and incubated in the presence of AgNPs (300 ppm) and AgNO3 (30 ppm). After 0, 24, 48, and 72 h of incubation in an orbital shaker (200 rpm, 37°C), 250 μl of each suspension was transferred to a centrifuge tube (Microcon-30 kDa Centrifugal Filter Unit with Ultracel-30 membrane – Merckmillipore, Ltd) and centrifuged at 5000 g for 60 min. After filtration, filtered samples were diluted with milli-Q water in the ratio of 1:500 as preparation for atomic absorption analysis.
Total silver was quantified in suitably diluted samples by Electrothermal Atomic Absorption Spectrometry (ETAAS) using an M6 mkII Atomic Absorption Spectrometer (Thermo Electron, Cambridge, UK) with D2 and Zeeman background correction, equipped with a GF95 Graphite Furnace atomiser (GFAAS). Analytical conditions are reported in Supplementary Tables 2 and 3. Calibration solutions for ETAAS were prepared within the range of 0.3–3 μg L–1 (corresponding to 150–1500 μg L–1 in samples) by dilution of the AgNO3 stock solution with Milli-Q water. Data acquisition and processing was conducted using thermo solaar software to evaluate the recovery of ionic silver after filtration, and thus its potential interaction with the centrifuge tube filter, an additional parallel extraction was performed. Briefly, three solutions of 30 ppm of AgNO3 were prepared in MHB without cells. The ion concentrations were measured before and after filtration to obtain a recovery factor applicable to all ionic release data.
For ionic release assessment, two values per time point (24, 48, and 72 h) were acquired and corrected by a recovery factor, obtained by calculating the percentage ratio between the total amount of silver ions released after AgNO3 dilution (before centrifugation) and the amount of total filtered silver (after centrifugation). A mean value was calculated.
Data Analysis
Counts resulting from AgNPs susceptibility assay were log-transformed and then summarized as mean values and standard errors. For statistical purposes a value of 300 colonies was assigned to plates on which more than 300 colonies were observed according to ISO 7218/2007 (ISO, 2007).
A t-test was used to determine whether the differences between strains that arose from graphical analysis were also noteworthy at statistical level. A classical 5% threshold for significance was used to detect inter strains differences at different time points (p-value <0.05). A Wilcoxon test was also performed to verify the accordance to the t-test.
Results
AgNPs Characterization
As visualized by TEM, AgNPs suspension appeared as a mixture of homogeneous particles by size and shape (Figure 1A). Most particles were spherical, but the sporadic presence of regular polygonal particles was also observed. Particle size distribution showed one major population, with median diameters of 23(±2) nm (Figure 1B).
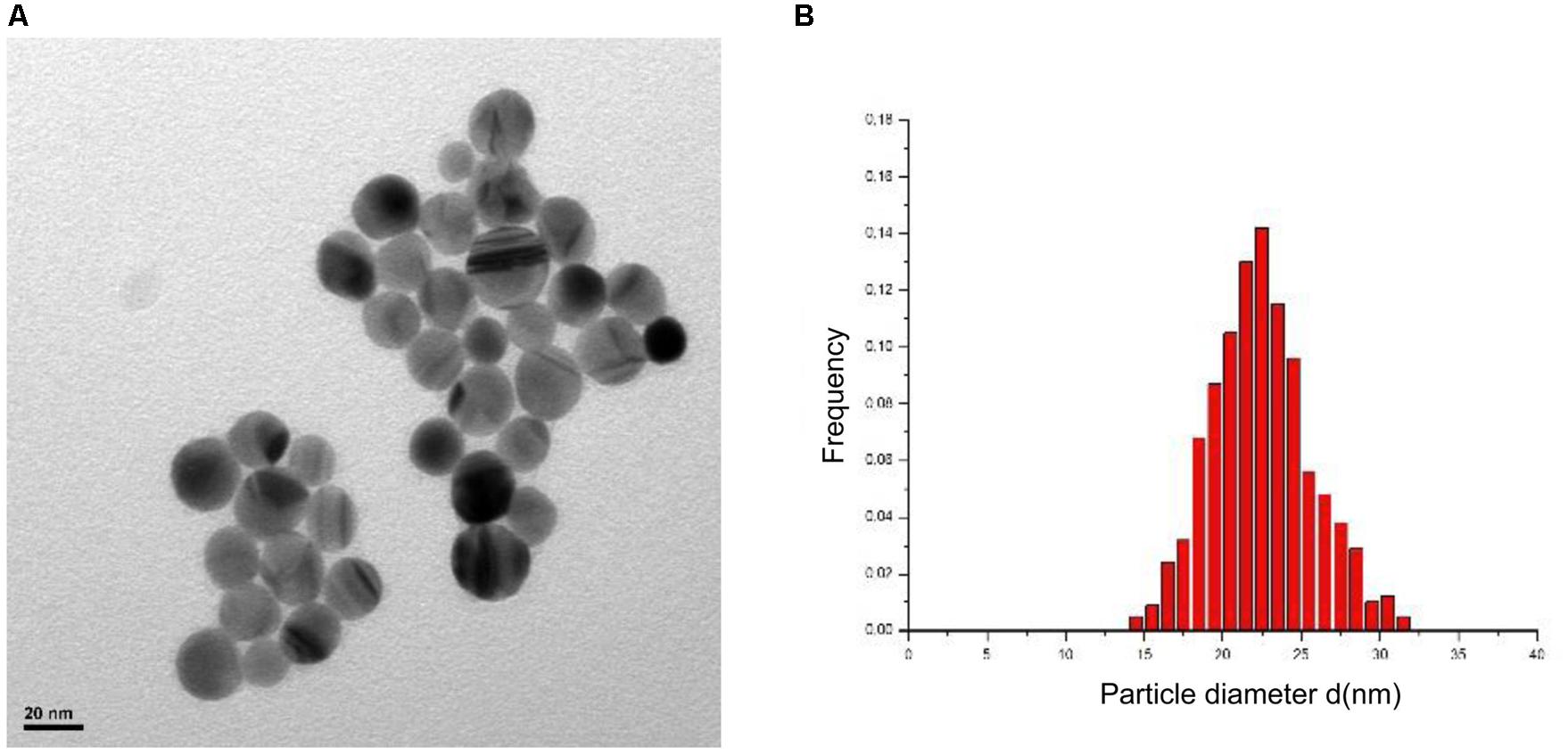
FIGURE 1. Results of silver nanoparticles (AgNPs) characterization with TEM. Morphology (A) and dimensional distribution (B).
Antimicrobial Susceptibility Testing
The results of the phenotypic antimicrobial susceptibility test of the 21 L. monocytogenes isolates are reported in Supplementary Table 1. No relevant differences among strains were observed.
Silver Susceptibility Assay
Differences in the susceptibility of L. monocytogenes strains were evaluated trough a two steps experiments. The first step resulted in the selection of six strains 4,5,7,11,20 according to their susceptibility and ATCC, with strains 4,5,11 being the less susceptible and 7, 20, and ATCC being the more susceptible.
Results of the second step are shown in Figures 2 and 3. L. monocytogenes counts were reduced to differing extents depending on the antimicrobial agent, with AgNO3 being effective at lower doses from the beginning of incubation (Figure 2) and AgNPs showing effectiveness only after 24 h incubation (Figure 3). The effect caused by AgNO3 was proportional to the dose, with 30 mg/L being the most effective, and 10 mg/L the least effective for all six L. monocytogenes isolates (Figure 2). Some statistically significant differences were observed among the selected strains at different time points. The maximum difference among strains was observed after 4 h of incubation both in the presence of AgNO3 and AgNPs. Conversely, no differences were detected after 24 h of incubation in the presence of AgNO3. In detail, in the presence of AgNO3 (30 and 20 ppm) L. monocytogenes ATCC and strain 20 counts were lower than the others (p < 0.05). ATCC strain exerted statistically significant lower counts also when incubated with 10 ppm of AgNO3 difference is confirmed in the case of ATCC. In the case of AgNPs a behavior similar to AgNO3 (30 ppm) could be described with statistically significant differences at 4h (ATCC and strain 20 more susceptible) and a convergence of growth curves at 24 h. In this case, however, differences among strains were also observed after 48 h with strain 20 being more sensitive than strains 4 and 7 (p < 0.05). Interestingly after 72 h ATCC, as also strain 4, showed a statistically significant recovery if compared with other L. monocytogenes strains.
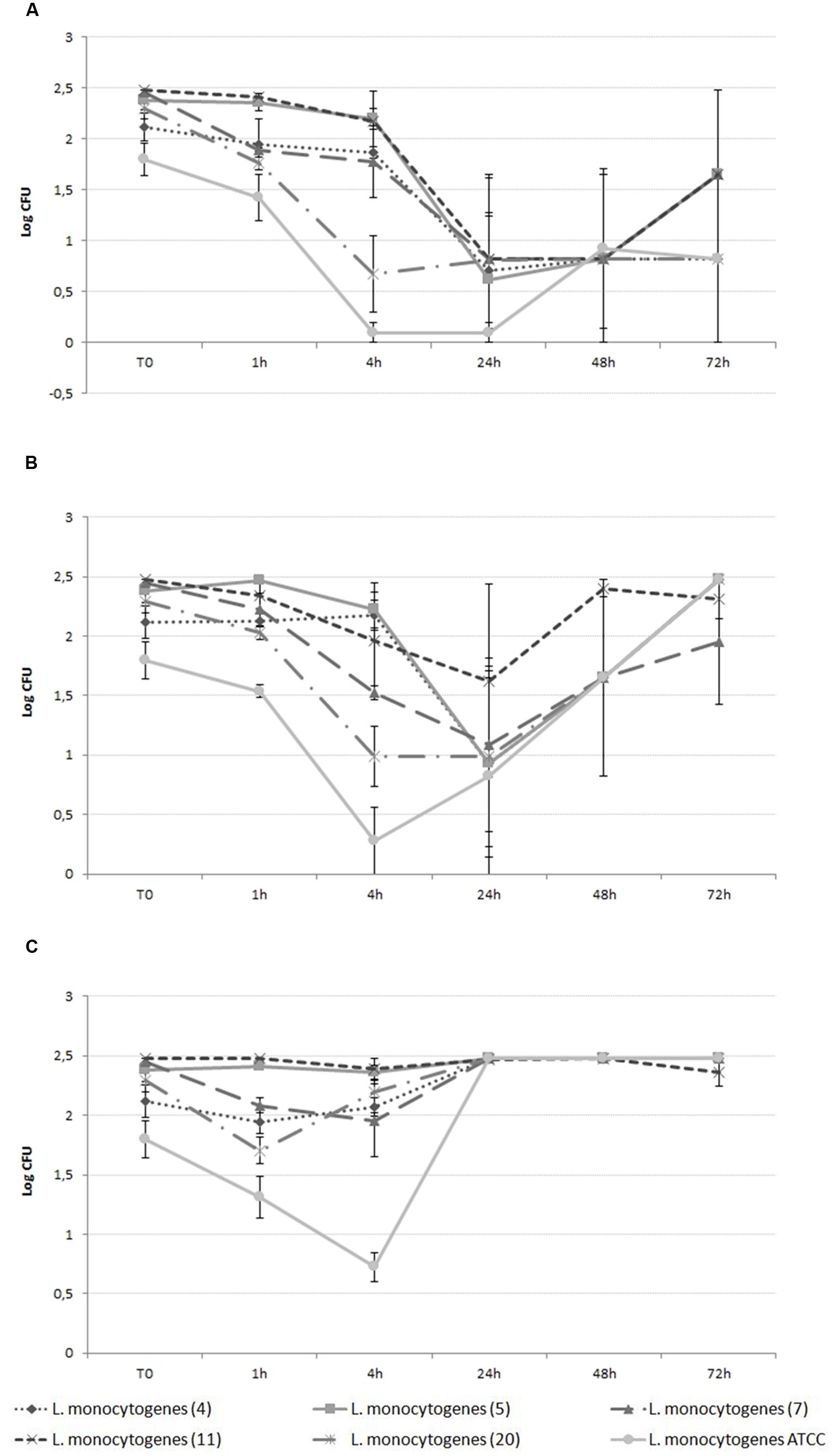
FIGURE 2. Growth curve of L. monocytogenes in the presence of 30 ppm (A), 20 ppm (B), and 10 ppm (C) AgNO3.
Ionic Release
Some correlation between ionic release and strain 5 growth curve was found both in the case of AgNO3 (Figure 4) and AgNPs (Figure 5), even though the relative extents were different. As shown in Figure 4B, in the case of AgNO3 a large amount of released silver ions was detected in the analyzed solution at the beginning of the study, when the measured silver concentration was approximately 22,000 μg/L. At this concentration, AgNO3 was able to immediately exert the expected antilisterial activity (Figure 4A).
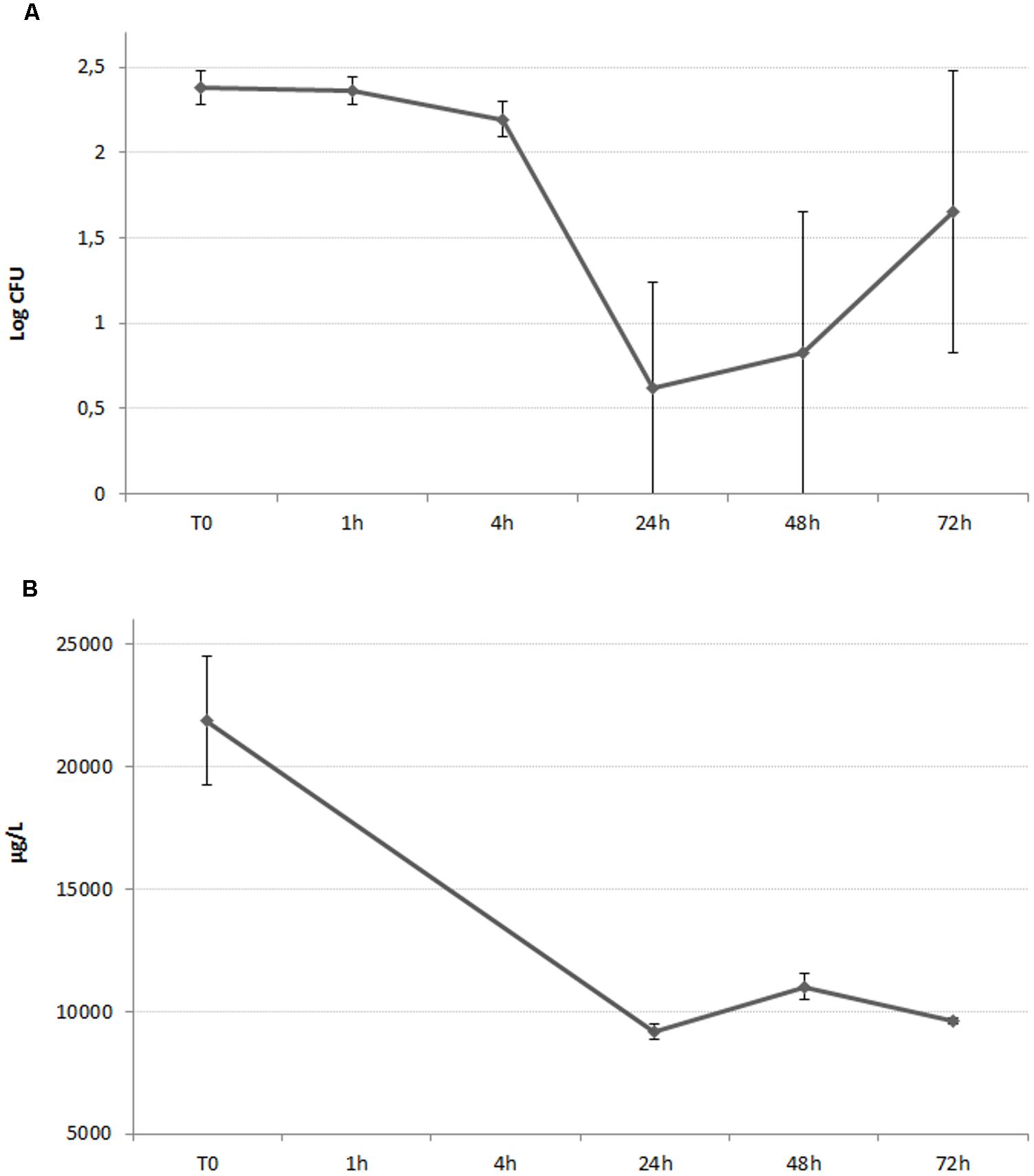
FIGURE 4. (A) Growth curve of L. monocytogenes in the presence of AgNO3, 30 ppm. The graphed values represents the mean of the counts belonging to Listeria monocytogenes strain n°5. (B) Time course assay of the silver ionic release of a 30 ppm solution of AgNO3, incubated in the presence of L. monocytogenes isolate 5.
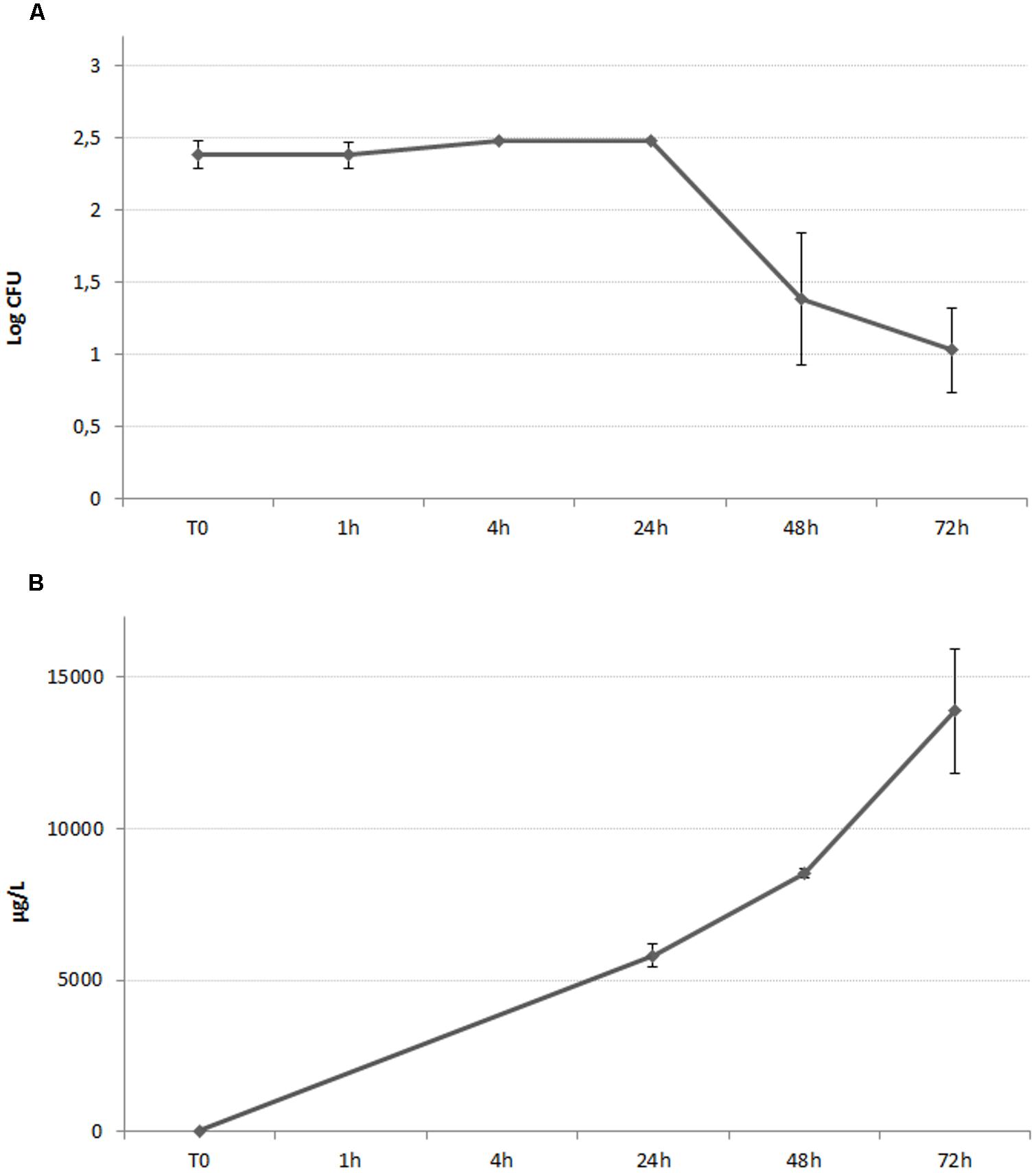
FIGURE 5. (A) Growth curve of L. monocytogenes in the presence of AgNPs 300 ppm. The graphed values represents the mean of the counts belonging to L. monocytogenes strain n°5. (B) Time course assay of the silver ionic release of a 300 ppm suspension of AgNPs, incubated in the presence of L. monocytogenes isolate 5.
The amount of silver ions released from AgNPs increased over time, with a maximum observed concentration of approximately 14,000 μg/L after 72 h of incubation (Figure 5B). As suggested by Figure 5A, the antilisterial effect exerted by AgNPs started after 24 h of incubation, and after the released silver ions reached the concentration of approximately 6,000 μg/L.
Discussion
This study aimed to investigate the effectiveness of silver in ionic and nano form as antimicrobial toward a panel of L. monocytogenes isolates.
The results obtained showed that both chemical forms of silver exerted antimicrobial activity and should, therefore, be considered as suitable to be used as biocide against L. monocytogenes. However, the timing of the inhibitory effect was different as AgNPs exhibited time-delayed inhibition of L. monocytogenes, compared with AgNO3.
The antimicrobial activity of AgNPs has been commonly described in the literature, and a wide array of microbes, including drug-resistant bacteria, fungi, and viruses, have been examined (Sondi and Salopek-Sondi, 2004; Pal et al., 2007; Choi et al., 2008; Fayaz et al., 2010; Lara et al., 2010; Samberg et al., 2011; You et al., 2011). To our knowledge, however, delayed effects of AgNPs on bacterial inhibition have never been observed. The delayed effect of AgNPs on the pathogen may have been caused by the gradual release of Ag ions from the AgNPs.
Our previous study demonstrated that the effectiveness of AgNPs as an antimicrobial toward Salmonella enterica was strongly strain-dependent due to the expression of silver specific resistance determinant by the resistant strains (Losasso et al., 2014). In the present study, despite the selection of L. monocytogenes isolates originating from different food matrices, the inclusion of an ATCC strain and the initial screening to select strains showing different behaviors toward silver, no relevant differences in Ag susceptibility assays were detected, and no differences among the examined isolates were noted.
In our study toxicity and dissolution tests were performed in parallel, as silver ions released upon dissolution of AgNPs have been proposed to be the primary contributor to the antibacterial activity of these compounds (Silver, 2003). The relation between the sensitivity assay and the amount of ions at the tested time points, in both cases (AgNPs and AgNO3) suggests that ions are the more effective elements exerting antibacterial activity. This findings agrees with scientific literature where several studies demonstrates that cations could interfere with the normal function of the bacterial electron transport chain, and thus, be responsible for ROS (Reactive Oxygen Species) formation at the cell membrane (Park et al., 2009; Xiu et al., 2012; Agnihotri et al., 2013). However, another study, using gene deletion strains, revealed that different pathways could be involved in the bacterial response to silver stress, as NPs can also exert a direct toxic effect (Ivask et al., 2014). Both ion-mediated toxicity as well as the physical interaction between NPs and the bacterial wall appear to contribute to the inhibitory effect, thus physicochemical properties of the NPs examined and the media used for NP suspension might play an important role (Prabhu and Poulose, 2012; Agnihotri et al., 2013).
Conclusion
Our study suggests that L. monocytogenes is sensitive to silver and that the efficacy is linked to ionic release. We speculate that silver-based food contact materials could play a useful role in the food industry, perhaps to reduce surface contamination, or eventually, to prolong shelf life. However, to confirm this, studies under industrial conditions are needed, to evaluate silver efficacy as well as the potential, hazardous migration of silver from food contact materials into food (Pezzuto et al., 2015). As persistent nanomaterials, like silver, raise some concerns in terms of potential migration and consequent ingestion by consumers, its inclusion in food contact materials should take into account the predicted use to avoid such drawbacks. In this context the development of nanocomposite materials embedding silver is of particular interest (Abreu et al., 2015). It is important to emphasize that the present study took into consideration only one type of AgNP; the current results cannot be generalized due to the broad variability of AgNPs on biological activity, which depends, not only on their chemical forms, but also on their specific shapes and dimensions (Gogoi et al., 2006; Xiu et al., 2012).
Author Contributions
SB, CL, AR conceived and designed the research, acquired and interpreted the data, drafted the work; IP analyzed the data; LR, DC, FG acquired the data; VC, PC, SS conceived the research and interpreted the data.
Conflict of Interest Statement
The authors declare that the research was conducted in the absence of any commercial or financial relationships that could be construed as a potential conflict of interest.
Acknowledgments
This work was financially supported by Fondazione CARIVERONA (project Tre Poli Tre - call 2011) and by the Ministry of Health, IT (project code RC IZSVe 08/13).
Supplementary Material
The Supplementary Material for this article can be found online at: http://journal.frontiersin.org/article/10.3389/fmicb.2016.00307
References
Abreu, A. S., Oliveira, M., de Sá, A., Rodrigues, R. M., Cerqueira, M. A., Vicente, A. A., et al. (2015). Antimicrobial nanostructured starch based films for packaging. Carbohydr. Polym. 129, 127–134. doi: 10.1016/j.carbpol.2015.04.021
Agnihotri, S., Mukherji, S., and Mukherji, S. (2013). Immobilized silver nanoparticles enhance contact killing and show highest efficacy: elucidation of the mechanism of bactericidal action of silver. Nanoscale 5, 7328–7340. doi: 10.1039/c3nr00024a
Bae, E., Park, H. J., Lee, J., Kim, Y., Yoon, J., Park, K., et al. (2010). Bacterial cytotoxicity of the silver nanoparticle related to physicochemical metrics and agglomeration properties. Environ. Toxicol. Chem. 29, 2154–2160. doi: 10.1002/etc.278
Beigmohammadi, F., Peighambardoust, S. H., Hesari, J., Azadmard-Damirchi, S., Peighambardoust, S. J., and Khosrowshahi, N. K. (2016). Antibacterial properties of LDPE nanocomposite films in packaging of UF cheese. LWT Food Sci. Technol. 65, 106–111. doi: 10.1016/j.lwt.2015.07.059
Berton, V., Montesi, F., Losasso, C., Facco, D. R., Toffan, A., and Terregino, C. (2015). Study of the interaction between silver nanoparticles and Salmonella as revealed by transmission electron microscopy. J. Prob. Health 03, 123. doi: 10.4172/2329-8901.1000123
CDC (2014). Incidence and trends of infection with pathogens transmitted commonly through food — Foodborne diseases active surveillance network, 10 U.S. sites, 2006–2013. Morb. Mortal. Wkly. Rep. 63, 328–332.
Choi, O., Deng, K. K., Kim, N. J., Ross, L. Jr., Surampalli, R. Y., and Hu, Z. (2008). The inhibitory effects of silver nanoparticles, silver ions, and silver chloride colloids on microbial growth. Water Res. 42, 3066–3074. doi: 10.1016/j.watres.2008.02.021
CLSI (2012). Methods for Dilution Antimicrobial Test for Bacteria that Grow Aerobically. M7-A. Wayne, PA: NCCLS.
Crum, N. F. (2002). Update on Listeria monocytogenes infection. Curr. Gastroenterol. Rep. 4, 287–296. doi: 10.1007/s11894-002-0078-z
EFSA and ECDC (2014). The European Union summary report on trends and sources of zoonoses, zoonotic agents and Food-borne outbreaks in 2012. EFSA J. 12, 3547.
Fayaz, A. M., Balaji, K., Girilal, M., Yadav, R., Kalaichelvan, P. T., and Venketesan, R. (2010). Biogenic synthesis of silver nanoparticles and their synergistic effect with antibiotics: a study against gram-positive and gram-negative bacteria. Nanomedicine 6, 103–109. doi: 10.1016/j.nano.2009.04.006
FSIS (2014). FSIS Compliance Guideline: Controlling Listeria monocytogenes in Post-Lethality Exposed Ready-to-Eat Meat and Poultry Products. Available at: http://www.fsis.usda.gov/wps/wcm/connect/d3373299-50e6-47d6-a577-e74a1e549fde/Controlling-Lm-RTE-Guideline.pdf?MOD=AJPERES
Gilbert, P., Brown, M. R. W., and Costerton, J. W. (1987). Inocula for antimicrobial sensitivity testing: a critical review. J. Antimicrob. Chemother. 20, 147–154. doi: 10.1093/jac/20.2.147
Gogoi, S. K., Gopinath, P., Paul, A., Ramesh, A., Ghosh, S. S., and Chattopadhyay, A. (2006). Green fluorescent protein-expressing Escherichia coli as a model system for investigating the antimicrobial activities of silver nanoparticles. Langmuir 22, 9322–9328. doi: 10.1021/la060661v
Gudadhe, J. A., Yadav, A., Gade, A., Marcato, P. D., Durán, N., and Rai, M. (2014). Preparation of an agar-silver nanoparticles (A-AgNp) film for increasing the shelf-life of fruits. IET Nanobiotechnol. 8, 190–195. doi: 10.1049/iet-nbt.2013.0010
Havelaar, A. H., Haagsma, J. A., Mangen, M.-J. J., Kemmeren, J. M., Verhoef, L. P. B., Vijgen, S. M. C., et al. (2012). Disease burden of foodborne pathogens in the Netherlands, 2009. Int. J. Food Microbiol. 156, 231–238. doi: 10.1016/j.ijfoodmicro.2012.03.029
ISO (1998). ISO 11290-2:1998 – Microbiology of Food and Animal Feeding Stuffs – Horizontal Method for the Detection and Enumeration of Listeria monocytogenes. London: BSI Corporate.
ISO (2007). ISO 7218/2007 Microbiology of Food and Animal Feedingstuffs – General Requirements and Guidance for Microbiological Examinations. London: BSI Corporate.
Ivask, A., Elbadawy, A., Kaweeteerawat, C., Boren, D., Fischer, H., Ji, Z., et al. (2014). Toxicity mechanisms in Escherichia coli vary for silver nanoparticles and differ from ionic silver. ACS Nano 8, 374–386. doi: 10.1021/nn4044047
Lara, H. H., Ayala-Núnez, N. V., Turrent, L., del, C. I., and Padilla, C. R. (2010). Bactericidal effect of silver nanoparticles against multidrug-resistant bacteria. World J. Microbiol. Biotechnol. 26, 615–621. doi: 10.1007/s11274-009-0211-3
Latorre, L., Parisi, A., Fraccalvieri, R., Normanno, G., La Porta, M. C. N., Goffredo, E., et al. (2007). Low prevalence of Listeria monocytogenes in foods from Italy. J. Food Prot. 70, 1507–1512.
Lomonaco, S., Nucera, D., Parisi, A., Normanno, G., and Bottero, M. T. (2011). Comparison of two AFLP methods and PFGE using strains of Listeria monocytogenes isolated from environmental and food samples obtained from Piedmont, Italy. Int. J. Food Microbiol. 149, 177–182. doi: 10.1016/j.ijfoodmicro.2011.06.012
Losasso, C., Belluco, S., Cibin, V., Zavagnin, P., Mièetiæ, I., Gallocchio, F., et al. (2014). Antibacterial activity of silver nanoparticles: sensitivity of different Salmonella serovars. Front. Microbiol. 5:227. doi: 10.3389/fmicb.2014.00227
Maillard, J.-Y., and Hartemann, P. (2013). Silver as an antimicrobial: facts and gaps in knowledge. Crit. Rev. Microbiol. 39, 373–383. doi: 10.3109/1040841X.2012.713323
Ottaviani, F., Ottaviani, M., and Agosti, M. (1997). Differential Agar Medium for Listeria monocytogenes [Foods]. Ind. Aliment. Available at: http://agris.fao.org/agris-search/search.do?recordID=IT1998061248 [accessed February 19, 2016].
Pal, S., Tak, Y. K., and Song, J. M. (2007). Does the antibacterial activity of silver nanoparticles depend on the shape of the nanoparticle? A study of the Gram-negative bacterium Escherichia coli. Appl. Environ. Microbiol. 73, 1712–1720.
Parisi, A., Latorre, L., Normanno, G., Miccolupo, A., Fraccalvieri, R., Lorusso, V., et al. (2010). Amplified fragment length polymorphism and multi-locus sequence typing for high-resolution genotyping of Listeria monocytogenes from foods and the environment. Food Microbiol. 27, 101–108. doi: 10.1016/j.fm.2009.09.001
Park, H. J., Kim, J. Y., Kim, J., Lee, J. H., Hahn, J. S., Gu, M. B., et al. (2009). Silver-ion-mediated reactive oxygen species generation affecting bactericidal activity. Water Res. 43, 1027–1032. doi: 10.1016/j.watres.2008.12.002
Pezzuto, A., Losasso, C., Mancin, M., Gallocchio, F., Piovesana, A., Binato, G., et al. (2015). Food safety concerns deriving from the use of silver based food packaging materials. Front. Microbiol. 6:1109. doi: 10.3389/fmicb.2015.01109
Prabhu, S., and Poulose, E. K. (2012). Silver nanoparticles: mechanism of antimicrobial action, synthesis, medical applications, and toxicity effects. Int. Nano Lett. 2, 1–10. doi: 10.1186/2228-5326-2-32
Rai, M., Yadav, A., and Gade, A. (2009). Silver nanoparticles as a new generation of antimicrobials. Biotechnol. Adv. 27, 76–83. doi: 10.1016/j.biotechadv.2008.09.002
Samberg, M. E., Orndorff, P. E., and Monteiro-Riviere, N. A. (2011). Antibacterial efficacy of silver nanoparticles of different sizes, surface conditions and synthesis methods. Nanotoxicology 5, 244–253. doi: 10.3109/17435390.2010.525669
Silver, S. (2003). Bacterial silver resistance: molecular biology and uses and misuses of silver compounds. FEMS Microbiol. Rev. 27, 341–353. doi: 10.1016/S0168-6445(03)00047-0
Sondi, I., and Salopek-Sondi, B. (2004). Silver nanoparticles as antimicrobial agent: a case study on E. coli as a model for Gram-negative bacteria. J. Colloid Interface Sci. 275, 177–182. doi: 10.1016/j.jcis.2004.02.012
vlab.amrita.edu (2011). Bacterial Growth Curve. Available at: vlab.amrita.edu/?sub=3&brch=73&sim=1105&cnt=1
Xiu, Z., Zhang, Q., Puppala, H. L., Colvin, V. L., and Alvarez, P. J. J. (2012). Negligible particle-specific antibacterial activity of silver nanoparticles. Nano Lett. 12, 4271–4275. doi: 10.1021/nl301934w
Keywords: food safety, nanoparticles, food preservation, cross-contamination, foodborne pathogens
Citation: Belluco S, Losasso C, Patuzzi I, Rigo L, Conficoni D, Gallocchio F, Cibin V, Catellani P, Segato S and Ricci A (2016) Silver As Antibacterial toward Listeria monocytogenes. Front. Microbiol. 7:307. doi: 10.3389/fmicb.2016.00307
Received: 12 November 2015; Accepted: 24 February 2016;
Published: 07 March 2016.
Edited by:
Amit Kumar Tyagi, The University of Texas MD Anderson Cancer Center, USAReviewed by:
Antonio Valero, University of Cordoba, SpainNitin Chauhan, Indian Institute of Technology Delhi, India
Copyright © 2016 Belluco, Losasso, Patuzzi, Rigo, Conficoni, Gallocchio, Cibin, Catellani, Segato and Ricci. This is an open-access article distributed under the terms of the Creative Commons Attribution License (CC BY). The use, distribution or reproduction in other forums is permitted, provided the original author(s) or licensor are credited and that the original publication in this journal is cited, in accordance with accepted academic practice. No use, distribution or reproduction is permitted which does not comply with these terms.
*Correspondence: Carmen Losasso, Y2xvc2Fzc29AaXpzdmVuZXppZS5pdA==