- 1Institute of Soil and Water Resources and Environmental Science, Zhejiang Provincial Key Laboratory of Subtropical Soil and Plant Nutrition, Zhejiang University, Hangzhou, China
- 2Key Laboratory of Soil Contamination Bioremediation of Zhejiang Province, School of Environmental and Resource Sciences, Zhejiang A & F University, Lin’an, China
We investigated the role of arbuscular mycorrhizal fungal (AMF) hyphae in alternation of soil microbial community and Aroclor 1242 dissipation. A two-compartment rhizobox system with double nylon meshes in the central was employed to exclude the influence of Cucurbita pepo L. root exudates on hyphal compartment soil. To assess the quantitative effect of AMF hyphae on soil microbial community, we separated the hyphal compartment soil into four horizontal layers from the central mesh to outer wall (e.g., L1–L4). Soil total PCBs dissipation rates ranged from 35.67% of L4 layer to 57.39% of L1 layer in AMF inoculated treatment, which were significant higher than the 17.31% of the control (P < 0.05). The dissipation rates of tri-, tetrachlorinated biphenyls as well as the total PCBs were significantly correlated with soil hyphal length (P < 0.01). Real-time quantitative PCR results indicated that the Rhodococcus-like bphC gene was 2–3 orders of magnitude more than that of Pseudomonas-like bphC gene, and was found responded positively to AMF. Phylogenetic analyses of the 16S rDNA sequenced by the Illumina Miseq sequencing platform indicated that AMF hyphae altered bacterial community compositions. The phylum Betaproteobacteria and Actinobacteria were dominated in the soil, while Burkholderiales and Actinomycetales were dominated at the order level. Taxa from the Comamonadaceae responded positively to AMF and trichlorinated biphenyl dissipation, while taxa from the Oxalobacteraceae and Streptomycetaceae responded negatively to AMF and PCB congener dissipation. Our results suggested that the AMF hyphal exudates as well as the hyphae per se did have quantitative effects on shaping soil microbial community, and could modify the PCBs dissipation processes consequently.
Introduction
Arbuscular mycorrhizal fungi (AMF) are ubiquitous in the terrestrial ecosystem. It is estimated that 250,000 species of plants worldwide, including many arable crops, are capable of forming the symbiosis with this group of fungi (Smith and Read, 2008). AMF receiving carbon from their host, and in return, delivering nutrients and water back. It has been estimated that in natural ecosystems plants colonized with AMF may invest 10–20% of the photosynthetically fixed carbon in their fungal partners (Johnson et al., 2002), and this significant input of energy and carbon into the soil ecosystem could be crucial to microorganisms associated with the AMF.
As the extraradical hyphae of AMF provides a direct pathway for translocation of photosynthetically derived carbon to the soil, the continuous provision of energy-rich compounds, coupled with the large surface area of the hyphae that intact with the surrounding soil environment (hyphosphere) provide important niches for bacterial colonization and growth (Toljander et al., 2007). The AMF hyphae may have both positive (Johansson et al., 2004; Toljander et al., 2007) and negative (Welc et al., 2010) effects on microbial growth. Using quantitative real-time PCR method, we also detected significant higher 16S rDNA abundance in both the bulk and the rhizosphere soils of Acaulospora laevis and Glomus mosseae inoculated treatments (Qin et al., 2014a). Compared to the quantitative changes in bacterial numbers, more studies have demonstrated shifts in bacterial community occurred in the presence of AMF (Lioussanne et al., 2010; Leigh et al., 2011; Nottingham et al., 2013; Nuccio et al., 2013). However, some studies also found AMF have no discernable effect on the composition of the microbial community present in litter-containing soil (Hodge et al., 2001; Herman et al., 2012). Though some bacterial species can utilize the hyphae themselves as substrate (Toljander et al., 2007), it is trusted that the changes in the bacterial community in the hyphosphere were not due to the amount of mycelium per se, suggesting that the qualitative effects (e.g., composition of exudates) of the AMF on the hyphosphere bacteria are more important than the quantitative development of AMF hyphae (Andrade et al., 1997; Johansson et al., 2004). Carbohydrates (Hooker et al., 2007; Toljander et al., 2007) and citric acid (Tawaraya et al., 2006) were detected in mycorrhizal hyphal exudates. In such a manner, the change of soil microbial biomass and the modification of the soil microbial community could be mainly dependent on quantitative and qualitative changes of hyphal exudates (Filion et al., 1999; Toljander et al., 2007; Lioussanne et al., 2010).
Few studies have explicitly studied how AMF influence the soil bacterial community responsible for PCB degradation. AMF have been proved having great potential on the rhizoremediation of organic pollutants through mycorrhizosphere (the zone influenced by both the root and the mycorrhizal fungus) effect (Joner and Leyval, 2003). Teng et al. (2010) reported that Glomus caledonium and Rhizobium meliloti had a significant synergistic effect on field soil PCBs removal when compared with non-inoculated alfalfa treatment. In our previous study, the dissipation rates of Aroclor 1242, both in bulk and rhizosphere soil, were greatly enhanced by the inoculation of Acaulospora laevis or Glomus mosseae (Qin et al., 2014a). The results also demonstrated a significant contribution of Actinobacteria to the PCB congener profiles in the bulk soil, indicating the important role of mycorrhizal extraradical hyphae on shaping bacterial community and PCB congener profile compositions. However, we did not separate the mycorrhizosphere and hyphosphere effects in the study. To our knowledge, no previous studies have investigated the effect of AMF on the hyphosphere soil microbial community mediating PCBs dissipation. The AMF hyphal exudates not only contain low-molecular-weight sugars and organic acids, but also unidentified high-molecular-weight polymeric compounds (Toljander et al., 2007). These compounds are energy-rich, and can stimulate or otherwise affect the growth of hyphosphere soil bacteria (Toljander et al., 2007). There are also the possibilities that some of the exudates have the similar chemical structure to PCBs, and may act as inducers for PCBs degradation (Singer et al., 2003).
We investigated how the AM fungus, Glomus mosseae, altered the bacterial communities and PCB congeners dissipation in soil spiked with Aroclor 1242. To accomplish this, we used a two-chamber rhizobox to allow AMF hyphae access to the Aroclor 1242 contaminated soil (Supplementary Figure S1). Furthermore, we separated the contaminated soil into four horizontal layers from the central mesh to the outer wall to assess the quantitative effect of mycorrhizal hyphae on soil bacterial community and PCBs dissipation. We hypothesized that different quantity of AMF hyphae results different native bacterial community and PCB congener profile compositions. The results may help us clarify the importance of AM hyphae on soil PCBs dissipation, and promote the field application of AM fungi on soil PCBs bioremediation.
Materials and Methods
Experiment Design
The soil classified as Fe-accumuli-Anthrosol was collected from a paddy field in Zhejiang Province, China. Soil pH (soil to water ratio, 1:2.5), organic carbon, and total N were 7.14, 14.3, 0.72 g kg-1, respectively. There was no PCB congeners detected in the soil. The air-dried soil was mixed thoroughly with 15 mg kg-1 Aroclor 1242 dissolved in hexane and placed in an exhaust hood for 48 h with periodic mixing to allow the hexane to evaporate.
The experiment was carried out in an artificially controlled climate chamber, with a day/night (12/12 h) temperature 30/22°C (10,000 Lux), and a relative humidity 50%. The experimental plants of zucchini (Cucurbita pepo L. cv black beauty) were grown in polyvinyl chloride (PVC) rhizoboxes (15 cm × 10.5 cm × 15 cm) divided by two nylon meshes (<35 μm) into one root compartment (15 cm × 5 cm × 15 cm) and one hyphal compartment (15 cm × 5 cm × 15 cm). The two nylon meshes were placed in the central of the rhizobox, with 0.5 cm space between them to prevent the transfer of root exudates to the hyphal compartment soil (Supplementary Figure S1). Seeds of the host plant were surface sterilized in a 1:10 solution of sodium hypochlorite (5% available chlorine) for 15 min and rinsed three times with deionised water. Seedlings were pre-cultured in sterilized sand in a greenhouse. Plantlets were then transplanted into the root compartments of rhizoboxes containing sterilized sand after 1 week. The hyphal compartments of the rhizoboxes were then filled with 1.5 kg of Aroclor 1242 spiked soil. Soil moisture content was adjusted to 50% of water-holding capacity (WHC) before use.
Arbuscular mycorrhizal inoculum used in this study was Glomus mosseae M47V which obtained from the International Culture Collection of (Vesicular) Arbuscular Mycorrhizal Fungi, France. The inoculum was propagated on sudangrass [Sorghum sudanese (Piper) Stapf.] grown in an autoclaved (121°C for 1 h on three successive days) sandy soil for 3 months. At the same time, the control non-mycorrhizal inoculum was also prepared with the same sterilized substratum on which sudangrass was cultivated. The root compartment of each AMF-inoculated treatment or non-mycorrhizal control was mixed with 150 g of mycorrhizal or non-mycorrhizal inoculums before transplanting. There were three replicates with both AMF inoculated treatments and non-mycorrhizal control. The rhizoboxes were arranged in a completely randomized design. During the entire period of the experiment, the soil moisture was maintained at about 50% of the field WHC and the sands in root compartments were fertilized with 30 ml of 10% Hoagland’s solution weekly.
At day 40 after transplanting, the plants were harvested, with shoots and roots sampled separately to analyze root colonization rate and plant biomass. Soils in each hyphal compartment of AMF inoculated treatment were separated into four horizontal layers (1.25 cm thick) by knife, for example, L1–L4 layers were separated according to the distance from the central nylon mesh to the boundary of the rhizobox. The non-mycorrhizal control soil was not separated into different layers. All soil samples were homogenized separately before analyses. Soil samples used for PCB residue and phospholipid fatty acid (PLFA) determination and DNA extraction were freeze-dried, and stored at -40°C. Subsamples for external mycelium determination were stored at 4°C.
AMF Growth Analyses
Root mycorrhizal colonization rate was estimated after clearing and staining, using the acid fuchsin staining-grid intersect method (Giovannetti and Mosse, 1980). The external mycelium of AM fungi was extracted from the soil samples and measured by the filtration-gridline method described by Schreiner et al. (1997). Soil AMF biomass was evaluated using the PLFA biomarker 16:1ω5c (Olsson et al., 1999). PLFA was performed using a modified technique (Wu et al., 2009) similar to that of Frostegård and Bååth (1996). Brifely, fatty acids were extracted from 3 g freeze-dried soil with a chloroform:methanol:citrate buffer. Fatty acids were then separated on a solid phase extraction column (Supelco, Inc., Bellefonte, PA, USA). Following methylation of the phospholipids, the PLFA methyl esters were separated and identified by gas chromatography (N6890, Agilent, USA) combined with a MIDI Sherlocks microbial identification system (Version 4.5, MIDI, USA). The fatty acid 19:0 phosphatidylcholine was added as an internal standard before methylation. Fatty acid peak areas were converted to nmol g-1 soil using internal standards.
PCBs Extraction and Analyses
Soil PCBs were extracted and analyzed using ultrasonic solvent extraction method described by Qin et al. (2014b). All procedures were carried out under room temperature. Briefly, 3 g freeze-dried soil was grounded and spiked with the surrogate standard [Decachlorobiphenyl (DCPB)] for PCB analysis. Then the soil samples were extracted three times by ultrasonics for 30 min (100 KHz, 25°C) in 30 ml 1:1 (v/v) acetone and hexane. The combined extracts were then further purified on a Florisil column with 40 ml hexane, and condensed to 1 ml under a gentle N2 stream. Soil extracts were analyzed for total PCBs using GC/mass spectrometry (MS; N6890/5975B, Agilent, USA), which was equipped with a HP-5MS capillary column (30 m length, 250 μm i.d., 0.25 μm film thickness). All measurements were carried out at full-scan mode with a mass range m/z from 50 to 450. The initial oven temperature was 80°C for 0.8 min, then increased at 25°C min-1 to 140°C for 1 min, followed by 10°C min-1 until the final temperature of 260°C, held for 5 min. Helium was used as the carrier gas at a flow rate of 1.0 ml min-1. A representative GC–MS chromatograph for PCB congeners profile is shown in Supplementary Figure S2.
qPCR Assay and High Throughput Sequencing
Total DNA was extracted from 0.5 g of freeze-dried soil using a MoBio PowersoilTM soil DNA isolation kit (MoBio Laboratories, Carlsbad, CA, USA) according to the manufacturer’s protocol. The abundance of the bacteria and PCB-degrading community was estimated from real-time qPCR assays targeting 16S and bph genes in soil DNA extracts. The qPCR and standard curve determination were act as described by our previous study (Qin et al., 2014a).
Soil microbial community was identified by amplifying approximately 400 bp of the 16S rDNA genes using barcoded primers 519F and 907R (Biddle et al., 2008) and sequencing on the Illumina Miseq platform. The sequences were processed and analyzed using the Quantitative Insights Into Microbial Ecology (QIIME) 1.4.0-dev pipeline1 (Caporaso et al., 2010) as we described previously (Qin et al., 2014a). Taxonomy was assigned to bacterial OTUs against a subset of the Silva 104 database2. OTU representative sequences were classified using RDP’s classifier with a 50% bootstrap confidence (Wang et al., 2007).
Statistical Analyses
All PCB concentrations and gene abundances are reported on a dry weight basis and are the means of 3 replicate analyses. The data were subjected to one-way ANOVA using SPSS 18.0 software. The results were expressed as mean values with standard deviations, and compared by the Duncan’s multiple range test. Statistical significance was determined at the 95% level (P < 0.05). Pearson’s correlation coefficients (r) were calculated to assess the relationships of PCB congeners with soil AMF biomass and microbial taxonomy distributions. Principal component analysis (PCA) of soil PCB congener profiles and bacterial community compositions were performed using Canoco for Windows 4.5 (Ter Braak and Šmilauer, 2002).
Results and Discussion
AMF Biomass in the Rhizoboxes
Zucchini root mycorrhizal colonization rate in the Glomus mosseae M47V inoculated treatment was 52% ± 6% after 40 days of growth, while no mycorrhizal colonized roots were detected in the non-mycorrhizal control, indicating the zucchini could be a good host plant for Glomus mosseae M47V colonization. Soil mycorrhizal hyphal lengths were significant higher (P < 0.05) in the L1-L3 layers of the AM-inoculated treatment when compared to the L4 layer, and no mycorrhizal hypha was detected in the control (Table 1). Moreover, the hyphal length in L3 layer was lower than that in the L1 and L2 layers, though there were no significant differences. The result proved our hypothesis that the mycorrhizal hyphae can penetrate the double meshes and the hyphal length decreasing with the increasing distance from the meshes. Similar decreasing trend was also found when using PLFA biomarker 16:1ω5c as an indicator of AMF biomass (Table 1). However, AMF PLFA biomarker was also detected in the non-mycorrhizal control. One possible reason is the AMF spores which still existed because the soil we used was not sterilized. Furthermore, the biomarker 16:1ω5c can also be detected in some gram-negative bacteria according to Zelles (1997), which could lead to the overestimation of soil AMF biomass.
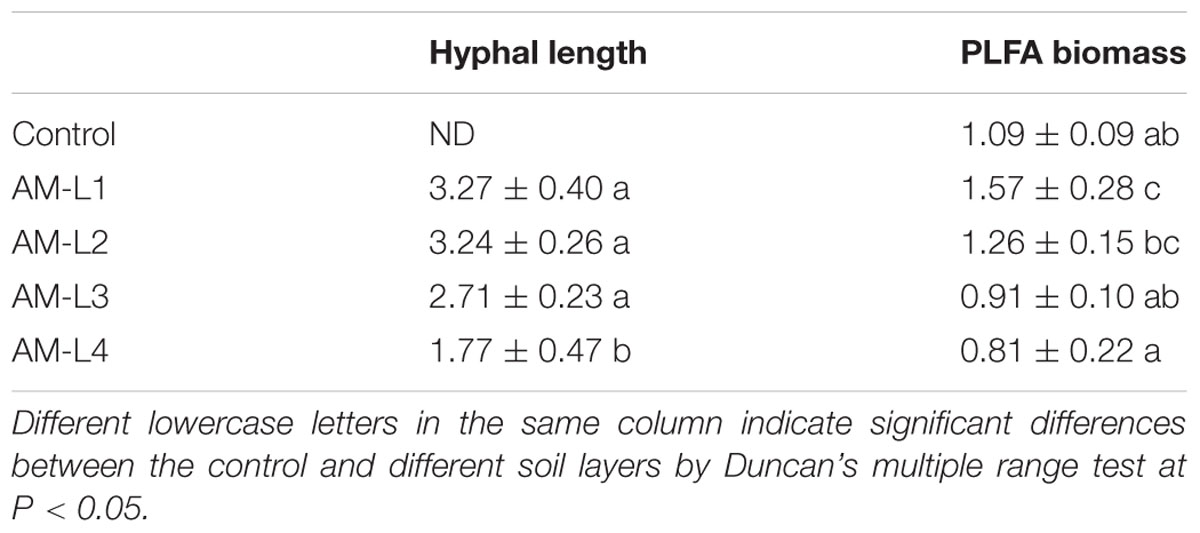
TABLE 1. Soil arbuscular mycorrhizal fungal hyphal length and phospholipid fatty acid (PLFA) biomass in different soil layers under mycorrhiza inoculation treatments and the control (mean ± SD; n = 3).
Soil PCBs Dissipation
After 40 days of incubation, the total PCBs concentration of the rhizobox soil in AMF inoculated treatment was significant lower (P < 0.05) than that in the control, indicating the AMF hyphae had beneficial effect on soil PCBs dissipation. Soil Aroclor 1242 dissipation rates ranged from 35.67% of L4 layer to 57.39% of L1 layer in AMF inoculated treatment, in which the L1 layer was significant higher (P < 0.05) than other three layers (Figure 1). Among the four soil layers, the di-, tri-, and tetrachlorinated biphenyls concentrations were always exhibit the highest value in the L4 layer, and there were significant differences between the L4 and L1 layers (P < 0.05) while no statistical differences were found between the L1 and L2 layers. In general, high overall PCB reduction was observed for tri- and tetrachlorinated biphenyls than for di- and pentachlorinated biphenyls. Pearson’s correlation coefficients indicated that the concentrations of soil tri- (r2 = -0.793), tetrachlorinated biphenyls (r2 = -0.938) and the total PCBs (r2 = -0.923) were significantly and negatively correlated with soil hyphal length (P < 0.01), while only trichlorinated biphenyl concentration was found negatively correlated with AMF PLFA biomass (r2 = -0.605, P < 0.05). AMF remediation of organic pollutants contaminated soils has been well-studied yet, however, with most of them only focused on the whole mycorrhizosphere effect (Joner and Leyval, 2003; Teng et al., 2010; Lu et al., 2014). To our knowledge, this is the first time to demonstrate the PCBs dissipation in the hyphosphere, and the enhanced dissipation of PCBs could be due to the increased bacterial growth and vitality induced by the external mycelial exudates (Toljander et al., 2007).
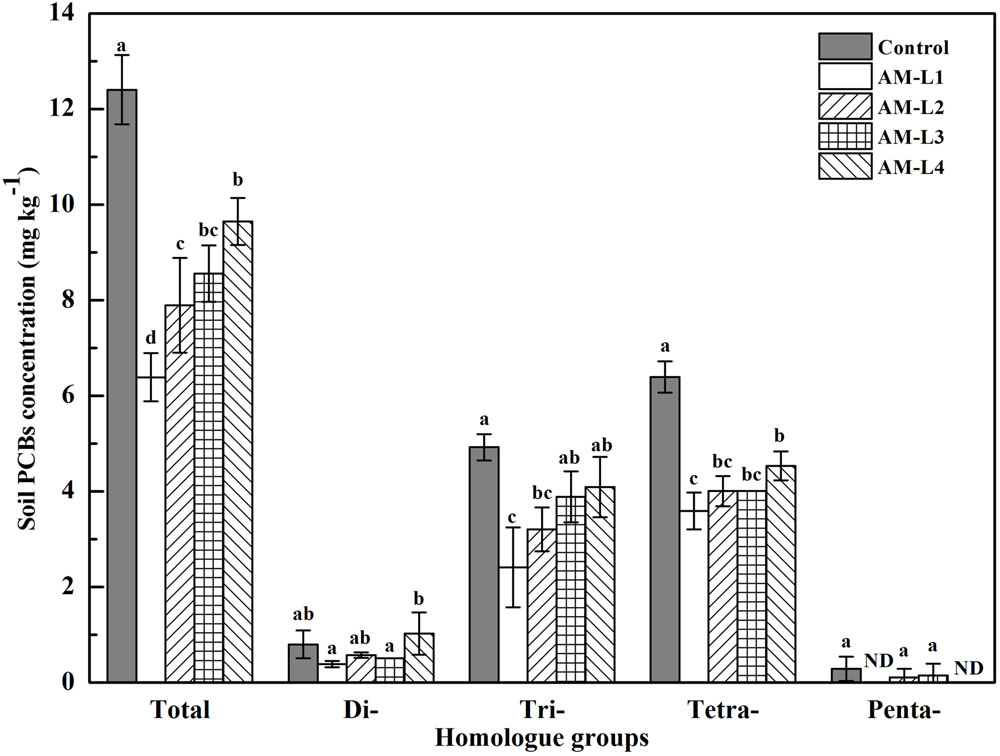
FIGURE 1. Concentration of PCB homolog groups in different soil layers of AMF-inoculated treatments and the control after 40 days of Cucurbita pepo L. growth. AM-L1 to AM-L4 were four horizontal soil layers from the central mesh to outer wall in the hyphal compartment of AMF-inoculated treatment, respectively. Bars represent standard errors and different letters above bars indicate significant differences, determined by Duncan’s multiple range test at P < 0.05.
The mycorrhizal hyphae did not only increase PCB dissipation rate, but also alter the soil PCB congener profiles. The PCB congener profiles of different soil layers in the AMF-inoculated treatment were grouped together, and distinctly separated from the control in PC1 (Figure 2). Among the different soil layers, the L4 layer was distinguished from the other three layers by positive PC1 values. According to our previous study which indicated that different plant root exudates or secondary metabolites resulted in different PCB congener profiles (Qin et al., 2014b), we can conclude that mycorrhizal hyphal exudates can play an important role in both degrading PCBs and shaping congener profiles.
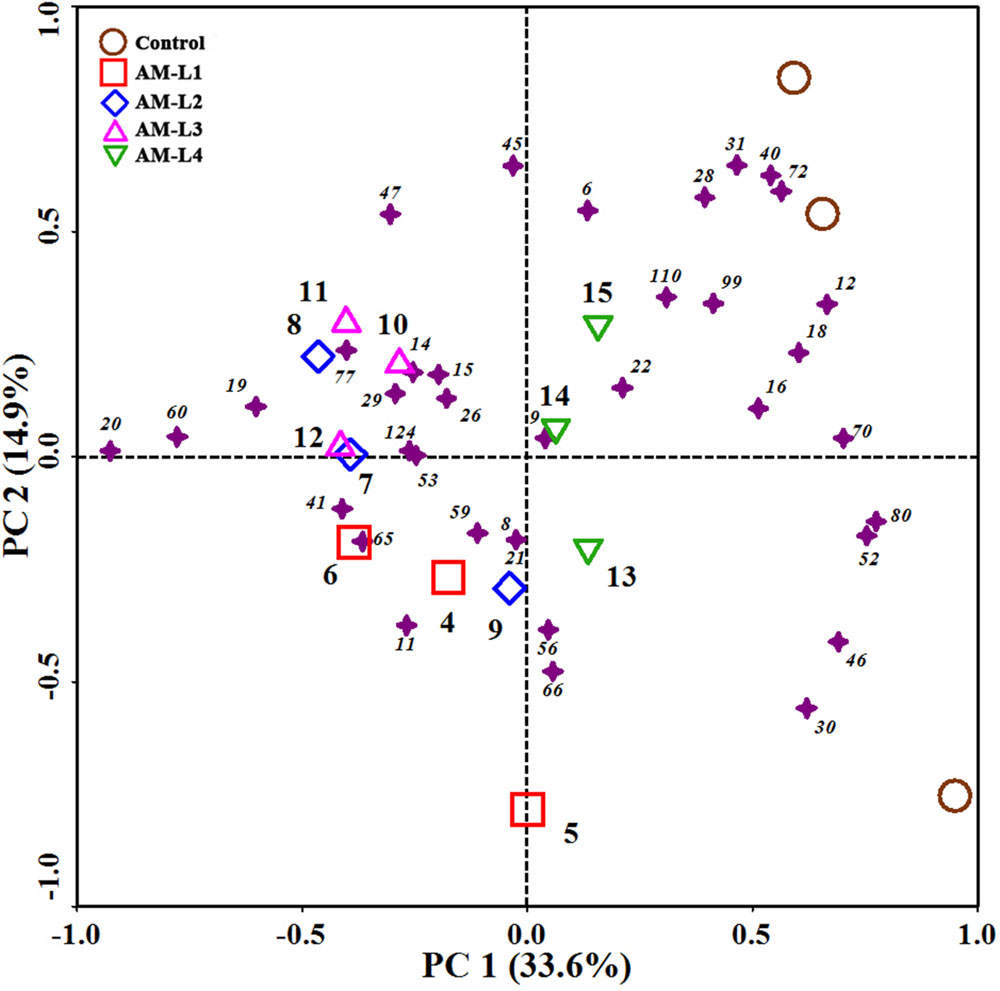
FIGURE 2. Principal component analysis (PCA) of PCB congener profiles in different soil layers of AMF-inoculated treatments and the control after 40 days of Cucurbita pepo L. growth. Samples were represented by open symbols while PCB congeners by solid asterisks. Numbers beside asterisks represent IUPAC congeners.
PCB-Degrading Related Gene Abundance
The copy numbers of soil bacterial 16S rDNA gene varied from 1.57 ± 0.35 × 109 to 2.44 ± 0.32 × 109g-1 dry soil (Table 2). The 16S abundance in the L1 and L2 layers of AMF-inoculated treatment were higher, but only the abundance in the L1 layer was significant higher than the other two layers and the control (P < 0.05). The hyphosphere could provide important niches for bacterial growth through transferring photo-assimilates into the soil or rapid turnover of mycorrhizal mycelium, in which the exudation of carbohydrates by living hyphae may enable a more direct and reciprocal interaction between mycorrhizal fungi and other microorganisms (Staddon et al., 2003; Toljander et al., 2007).

TABLE 2. Abundances of 16S, bphA, bphC(Rh), and bphC(Ps) genes in different soil layers under mycorrhiza inoculation treatments and the control (mean ± SD; n = 3).
Several studies have successfully calculated the PCB-degrading bacterial population in various contaminated soils using qPCR technique to target the bphA or bphC genes (Correa et al., 2010; Petrić et al., 2010; Lu et al., 2014). In the present study, the bphA and bphC genes were detected by qPCR in both AMF-inoculated treatment and the control. The bphA gene population was significant higher in the L1 layer than other soil layers and the control, while no significant difference was observed between the L2-L4 layers and the control (Table 2). The population size of Rhodococcus-like bphC gene [bphC(Rh)] was found approximately 2–3 orders of magnitude more than that of Pseudomonas-like bphC gene [bphC(Ps)], indicating that the bphC(Rh) gene played a key role in Aroclor 1242 dissipation in this spiked soil. The bphC(Rh) gene abundance was significant higher in the L1 layer when compared to the control (Table 2), and was found correlated significantly with the soil AMF hyphal length (r2 = 0.624, P < 0.05) and PLFA biomass (r2 = 0.577, P < 0.05). Evidences have shown that hyphal exudates from Glomus sp. contained low molecular weight (LMW) sugar and organic acids, which could probably be metabolized by bacterial (Toljander et al., 2007). Furthermore, some LMW organic acids, such as salicylate, can act as inducers of PCB degradation by indigenous bacteria (Singer et al., 2003). It can be hypothesized that the mycorrhizal hyphal exudates may contain some LMW organic compounds which could act as inducers of the bphC(Rh) gene and elevate the gene expression level. This could partly explain the correlation between the bphC(Rh) gene abundance and the hyphal length. However, it seems that the hyphal exudates of Glomus mosseae we used in the present study lack of inducers of bphC(Ps) gene. As different AMF or host plant may result in different hyphal exudates, further studies are needed to explore potential inducers for bph gene expression and elucidate their mechanisms.
Changes in Microbial Community
A total of 67513 sequences were obtained after passing several specific filters (Yadav et al., 2014; Yang et al., 2014). The relative abundance of the top 14 taxonomic categories at class level is demonstrated in Supplementary Figure S3. The taxonomic classes other than these results are categorized as “Others.” Sequences that do not show homology in the NCBI database have been labeled as “Unclassified.” The Betaproteobacteria was largely dominant, representing 12.15–14.26% of the population in all soils. The Actinobacteria was the next dominant taxonomy observed in all the soils, followed by the Alpha- and Gammaproteobacteria. Among the 14 selected classes, the Anaerolineae was found negatively correlated with the concentration of dichlorinated biphenyls (r2 = -0.660, P < 0.01) (Table 3). Previous studies have revealed that the phylum Chloroflexi was one of the several most abundant groups associated with Aroclor 1242 degradation (Mercier et al., 2014) or perchloroethene (PCE) dechloronation (Kittelmann and Friedrich, 2008). Mercier et al. (2014) found that Chloroflexi was the second-most abundant phylum shared between three granular activated carbons which were incubated for a month with Aroclor 1242 spiked sediment, and the Anaerolineae class was found dominated in this phylum. The strictly anaerobic genera in Anaerolineae class could ferment carbohydrates and amino acids around the mycorrhizal hyphae, and may also utilize low chlorinated biphenyls such as dichlorinated biphenyls. Aside from the Anaerolineae, the class Alphaproteobacteria was also found negatively correlated with the concentration of tetrachlorinated biphenyls (r2 = -0.525, P < 0.05). Most of the Alphaproteobacteria were assigned to the Rhizobiales and Rhodospirillales orders. One possible reason is that certain members of the Rhizobiales or Rhodospirillales have the ability to biotransform highly chlorinated PCBs (Ahmad et al., 1997; Bertin et al., 2011). Otherwise, there is also the possibility that mycorrhizal hyphal exudates stimulated the growth of Alphaproteobacteria and enhanced the co-metabolization of tetrachlorinated biphenyls by PCB degraders consequently.
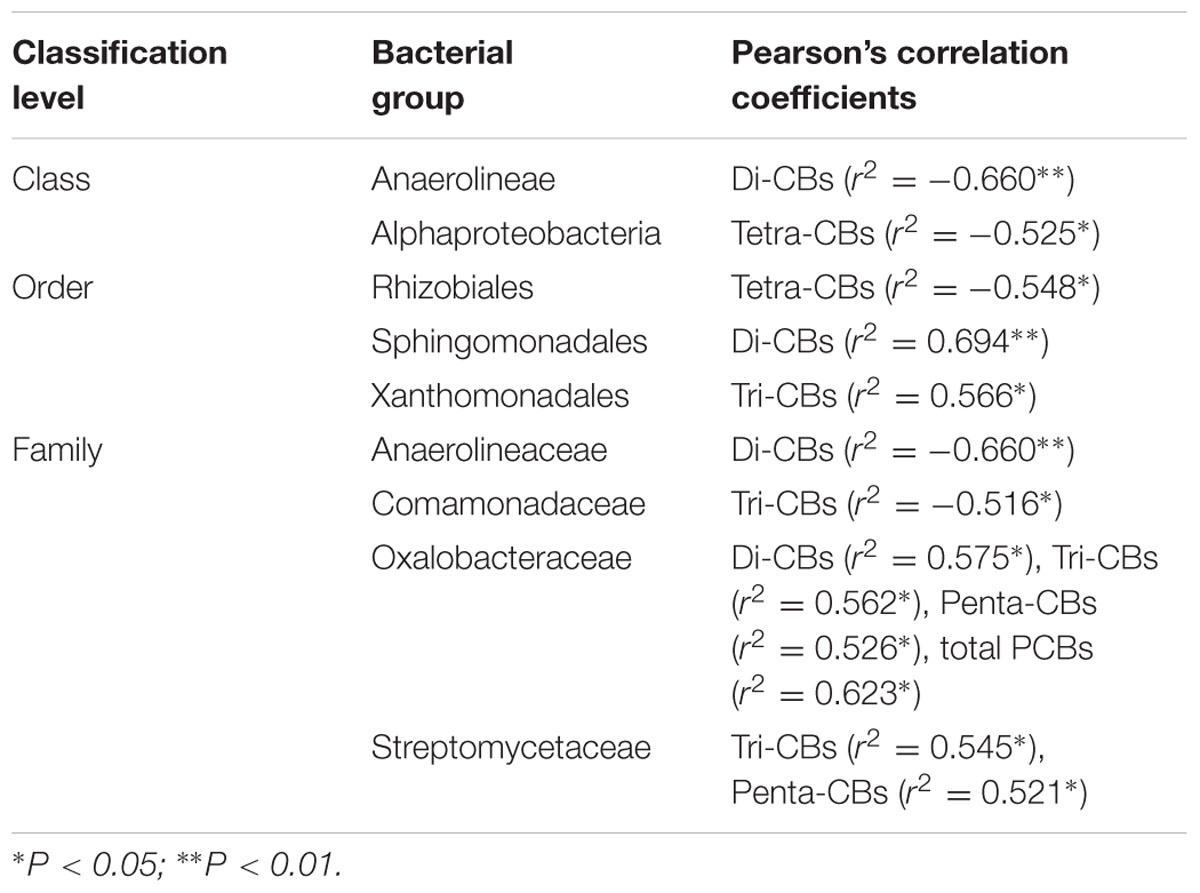
TABLE 3. Pearson’s correlation coefficients relating PCB congener concentrations and the relative abundance of bacterial groups at the class, order, and family level, respectively.
At the order level, Burkholderiales, Actinomycetales, Sphingobacteriales, Bacillales, Xanthomonadales, Rhizobiales are dominated, among which the Burkholderiales is the most abundant. Offre et al. (2008) reported that subgroups such as Comamonadaceae, Oxalobacteraceae and Rubrivivax affiliated to Burkholderiales are preferentially associated with AM. Soil Burkholderiales has been shown to be involved in biological suppression of pathogen, plant-growth promotion, and N-fixation (Xu et al., 2015). Our results were in consistent with the previous studies that species of the genus Burkholderia were abundant both in rRNA and in rDNA clone libraries generated from the polluted soil (Nogales et al., 2001). The Burkholderia xenovorans LB400 has been considered as one model organism to study the aerobic biodegradation of PCBs because of their ability to oxidize wide range of congeners as well as use some congeners as sources of carbon and energy (Tillmann et al., 2005; Parnell et al., 2010). Though no correlation was found between the abundance of Burkholderiales and soil PCB congeners concentrations, the Burkholderiales was more abundant in the L1 layer of the AMF-inoculated treatment than other layers and the control (P < 0.05), indicating mycorrhizal hyphal exudates may benefit the growth of this group of bacteria. The negative correlation between the abundance of Rhizobiales and the concentration of tetrachlorinated biphenyls (r2 = -0.548, P < 0.05) were proved (Table 3), which provided strong evidence for our above hypothesis about the relationship between Alphaproteobacteria and tetrachlorinated biphenyls.
Among the families classified by the RDP classifier, Chitinophagaceae, Xanthomonadaceae, Cyanobacteria Family XIII, Comamonadaceae and Burkholderiaceae are the most abundant five families. The relative abundance of family Comamonadaceae was negatively correlated with trichlorinated biphenyls (r2 = -0.516, P < 0.05) (Table 3). The Comamonadaceae are a physiologically heterogeneous group of bacteria; they are known to consume a broad spectrum of organic carbon compounds that range from simple sugars to complex aromatic compounds (Kersters et al., 2006). Genera in this family such as Acidovorax sp. (formerly Pseudomonas sp.) strain KKS102 and Hydrogenophaga taeniospiralis IA3-A have the well-known ability of PCB- and biphenyl-degrading or cometabolizing (Lambo and Patel, 2006; Ohtsubo et al., 2006). It is suggested that members of the Comamonadaceae may be stimulated by the presence of AMF in PCBs contaminated soil. The results was in accordance with Offre et al. (2007), who found that in the Medicago truncatula rhizosphere, the presence of AMF increased the relative abundance of Comamonadaceae taxa. However, results from Pivato et al. (2009) and Nuccio et al. (2013) indicated that taxa from Comamonadaceae responded negatively to AMF. The mechanisms for these interactions are still unknown, and may result from the direct or indirect manipulation of the community through hyphal exudates (Toljander et al., 2007; Nuccio et al., 2013). Similar to the class Anaerolineae, the family Anaerolineaceae was also negatively correlated with the concentration of dichlorinated biphenyls (r2 = -0.660, P < 0.01). We also found positive correlations between the relative abundance of family Oxalobacteraceae and di- (r2= 0.575, P < 0.05), tri- (r2= 0.562, P < 0.05), pentachlorinated biphenyls (r2= 0.526, P < 0.05) and the total PCB concentrations (r2= 0.632, P < 0.05), while the relative abundance of family Streptomycetaceae was correlated with tri- (r2= 0.545, P < 0.05) and pentachlorinated biphenyls (r2= 0.521, P < 0.05) (Table 3). Given that these two families were relatively lower in the L1 and L2 layers when compared with the other two layers and the control, we can speculate that the mycorrhizal hyphae may secrete certain inhibitors or compete nutrition with them and resulted in decreased biomass consequently.
Principal component analysis showed distinct separation of the microbial community from the control in the PC2 to the microbial community that developed under different soil layers of the AMF-inoculated treatment (Figure 3). Among the four soil layers in the AMF-inoculated treatment, the L1 layer was distinguished from the other three layers by positive PC1 value, while no obvious difference in microbial community was found between each of the three soil layers. The PCA results strongly supported our hypothesis that the existence as well as the abundance of arbuscular mycorrhizal hyphae could play an important role in shaping the mycorrhizosphere bacterial community which responsible for PCB dissipation.
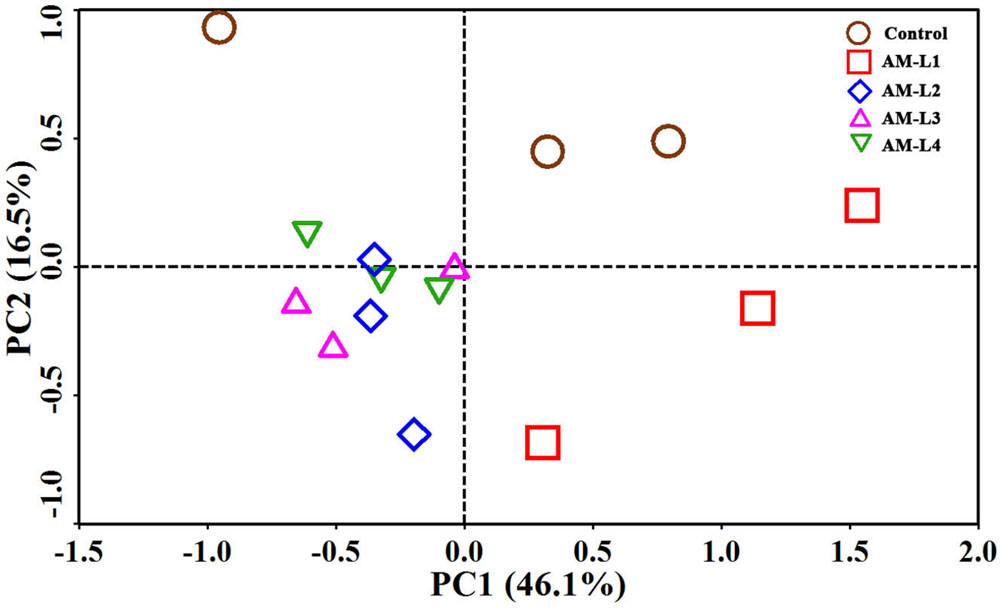
FIGURE 3. Principal component analysis of bacterial communities in different soil layers of AMF-inoculated treatments and the control after 40 days of Cucurbita pepo L. growth. AM-L1 to AM-L4 were four horizontal soil layers from the central mesh to outer wall in the hyphal compartment of AMF-inoculated treatment, respectively.
Conclusion
This study demonstrates the important role of arbuscular mycorrhizal hyphae in degradative efficiency of different PCB congeners. Analysis of bacterial growth, bph gene abundance, and bacterial community composition were also shown to vary with different soil mycorrhizal hyphal biomass. The results strongly supported our previous hypothesis that the mycorrhizal hyphae could accelerate the dissipation of low-chlorinated biphenyls as well as shaping the soil PCB congener profiles via altering bacterial growth and community compositions in the mycorrhizosphere. As the AMF-plant symbiosis is ubiquitous in terrestrial ecosystems, the influence of AMF on PCB congener dissipation is broadly relevant across terrestrial ecosystems for the remediation of PCB contaminated soils. These data improved our understanding of mycorrhizal hyphae-bacteria interactions in PCB dissipation. Further investigation is needed to identify the PCBs degraders in AM fungal hyphosphere soil, and understand the metabolic pathways involved in PCBs degradation.
Author Contributions
HQ: conceived and designed the work that led to the submission, acquired data, and played an important role in interpreting the results; PB: revised the manuscript; JX: revised the manuscript and approved the final version.
Funding
This work was financially supported by the National Natural Science Foundation of China (41101243, 41090284, and 41271256), Program of Shanghai Cooperative Centre for WEEE Recycling (ZF1224-09).
Conflict of Interest Statement
The authors declare that the research was conducted in the absence of any commercial or financial relationships that could be construed as a potential conflict of interest.
Acknowledgment
We are grateful to Youzhi Feng, Jiangbing Xu, and Yongjie Yu at Institute of Soil Science – Chinese Academy of Sciences for help with DNA sequencing and data analyses.
Supplementary Material
The Supplementary Material for this article can be found online at: http://journal.frontiersin.org/article/10.3389/fmicb.2016.00939
Footnotes
References
Ahmad, D., Mehmannavaz, R., and Damaj, M. (1997). Isolation and characterization of symbiotic N2-fixing Rhizobium meliloti from soils contaminated with aromatic and chloroaromatic hydrocarbons: PAHs and PCBs. Int. Biodeterior. Biodegr. 39, 33–43. doi: 10.1016/S0964-8305(96)00065-0
Andrade, G., Mihara, K. L., Linderman, R. G., and Bethlenfalvay, G. J. (1997). Bacteria from rhizosphere and hyphosphere soils of different arbuscular-mycorrhizal fungi. Plant Soil 192, 71–79. doi: 10.1023/a:1004249629643
Bertin, L., Capodicasa, S., Fedi, S., Zannoni, D., Marchetti, L., and Fava, F. (2011). Biotransformation of a highly chlorinated PCB mixture in an activated sludge collected from a Membrane Biological Reactor (MBR) subjected to anaerobic digestion. J. Hazard. Mater. 186, 2060–2067. doi: 10.1016/j.jhazmat.2010.12.131
Biddle, J. F., Fitz-Gibbon, S., Schuster, S. C., Brenchley, J. E., and House, C. H. (2008). Metagenomic signatures of the Peru Margin subseafloor biosphere show a genetically distinct environment. Proc. Natl. Acad. Sci. U.S.A. 105, 10583–10588. doi: 10.1073/pnas.0709942105
Caporaso, J. G., Kuczynski, J., Stombaugh, J., Bittinger, K., Bushman, F. D., Costello, E. K., et al. (2010). QIIME allows analysis of high-throughput community sequencing data. Nat. Methods 7, 335–336. doi: 10.1038/nmeth.f.303
Correa, P. A., Lin, L., Just, C. L., Hu, D., Hornbuckle, K. C., Schnoor, J. L., et al. (2010). The effects of individual PCB congeners on the soil bacterial community structure and the abundance of biphenyl dioxygenase genes. Environ. Int. 36, 901–906. doi: 10.1016/j.envint.2009.07.015
Filion, M., St-Arnaud, M., and Fortin, J. A. (1999). Direct interaction between the arbuscular mycorrhizal fungus Glomus intraradices and different rhizosphere microorganisms. New Phytol. 141, 525–533. doi: 10.1046/j.1469-8137.1999.00366.x
Frostegård, A., and Bååth, E. (1996). The use of phospholipid fatty acid analysis to estimate bacterial and fungal biomass in soil. Biol. Fertil. Soils 22, 59–65. doi: 10.1007/bf00384433
Giovannetti, M., and Mosse, B. (1980). An evaluation of techniques for measuring vesicular arbuscular mycorrhizal infection in roots. New Phytol. 84, 489–500. doi: 10.1111/j.1469-8137.1980.tb04556.x
Herman, D. J., Firestone, M. K., Nuccio, E., and Hodge, A. (2012). Interactions between an arbuscular mycorrhizal fungus and a soil microbial community mediating litter decomposition. FEMS Microbiol. Ecol. 80, 236–247. doi: 10.1111/j.1574-6941.2011.01292.x
Hodge, A., Campbell, C. D., and Fitter, A. H. (2001). An arbuscular mycorrhizal fungus accelerates decomposition and acquires nitrogen directly from organic material. Nature 413, 297–299. doi: 10.1038/35095041
Hooker, J. E., Piatti, P., Cheshire, M. V., and Watson, C. A. (2007). Polysaccharides and monosaccharides in the hyphosphere of the arbuscular mycorrhizal fungi Glomus E3 and Glomus tenue. Soil Biol. Biochem. 39, 680–683. doi: 10.1016/j.soilbio.2006.08.006
Johansson, J. F., Paul, L. R., and Finlay, R. D. (2004). Microbial interactions in the mycorrhizosphere and their significance for sustainable agriculture. FEMS Microbiol. Ecol. 48, 1–13. doi: 10.1016/j.femsec.2003.11.012
Johnson, D., Leake, J. R., Ostle, N., Ineson, P., and Read, D. J. (2002). In situ13CO2 pulse-labelling of upland grassland demonstrates a rapid pathway of carbon flux from arbuscular mycorrhizal mycelia to the soil. New Phytol. 153, 327–334. doi: 10.1046/j.0028-646X.2001.00316.x
Joner, E. J., and Leyval, C. (2003). Phytoremediation of organic pollutants using mycorrhizal plants: a new aspect of rhizosphere interactions. Agronomie 23, 495–502. doi: 10.1051/agro:2003021
Kersters, K., De Vos, P., Gillis, M., Swings, J., Vandamme, P., and Stackebrandt, E. (2006). “Introduction to the Proteobacteria,” in The Prokaryotes: Proteobacteria: Alpha and Beta Subclasses, eds M. Dwarkin, S. Falkow, E. Rosenberg, K.-H. Schleifer, and E. Stackebrandt (New York, NY: Springer), 3–37.
Kittelmann, S., and Friedrich, M. W. (2008). Novel uncultured Chloroflexi dechlorinate perchloroethene to trans-dichloroethene in tidal flat sediments. Environ. Microbiol. 10, 1557–1570. doi: 10.1111/j.1462-2920.2008.01571.x
Lambo, A. J., and Patel, T. R. (2006). Isolation and characterization of a biphenyl-utilizing psychrotrophic bacterium, Hydrogenophaga taeniospiralis IA3-A, that cometabolize dichlorobiphenyls and polychlorinated biphenyl congeners in Aroclor 1221. J. Basic Microbiol. 46, 94–107. doi: 10.1002/jobm.200510006
Leigh, J., Fitter, A. H., and Hodge, A. (2011). Growth and symbiotic effectiveness of an arbuscular mycorrhizal fungus in organic matter in competition with soil bacteria. FEMS Microbiol. Ecol. 76, 428–438. doi: 10.1111/j.1574-6941.2011.01066.x
Lioussanne, L., Perreault, F., Jolicoeur, M., and St-Arnaud, M. (2010). The bacterial community of tomato rhizosphere is modified by inoculation with arbuscular mycorrhizal fungi but unaffected by soil enrichment with mycorrhizal root exudates or inoculation with Phytophthora nicotianae. Soil Biol. Biochem. 42, 473–483. doi: 10.1016/j.soilbio.2009.11.034
Lu, Y. F., Lu, M., Peng, F., Wan, Y., and Liao, M. H. (2014). Remediation of polychlorinated biphenyl-contaminated soil by using a combination of ryegrass, arbuscular mycorrhizal fungi and earthworms. Chemosphere 106, 44–50. doi: 10.1016/j.chemosphere.2013.12.089
Mercier, A., Joulian, C., Michel, C., Auger, P., Coulon, S., Amalric, L., et al. (2014). Evaluation of three activated carbons for combined adsorption and biodegradation of PCBs in aquatic sediment. Water Res. 59, 304–315. doi: 10.1016/j.watres.2014.04.021
Nogales, B., Moore, E. R. B., Llobet-Brossa, E., Rossello-Mora, R., Amann, R., and Timmis, K. N. (2001). Combined use of 16S ribosomal DNA and 16S rRNA to study the bacterial community of polychlorinated biphenyl-polluted soil. Appl. Environ. Microbiol. 67, 1874–1884. doi: 10.1128/aem.67.4.1874-1884.2001
Nottingham, A. T., Turner, B. L., Winter, K., Chamberlain, P. M., Stott, A., and Tanner, E. V. J. (2013). Root and arbuscular mycorrhizal mycelial interactions with soil microorganisms in lowland tropical forest. FEMS Microbiol. Ecol. 85, 37–50. doi: 10.1111/1574-6941.12096
Nuccio, E. E., Hodge, A., Pett-Ridge, J., Herman, D. J., Weber, P. K., and Firestone, M. K. (2013). An arbuscular mycorrhizal fungus significantly modifies the soil bacterial community and nitrogen cycling during litter decomposition. Environ. Microbiol. 15, 1870–1881. doi: 10.1111/1462-2920.12081
Offre, P., Pivato, B., Mazurier, S., Siblot, S., Berta, G., Lemanceau, P., et al. (2008). Microdiversity of Burkholderiales associated with mycorrhizal and nonmycorrhizal roots of Medicago truncatula. FEMS Microbiol. Ecol. 65, 180–192. doi: 10.1111/j.1574-6941.2008.00504.x
Offre, P., Pivato, B., Siblot, S., Gamalero, E., Corberand, T., Lemanceau, P., et al. (2007). Identification of bacterial groups preferentially associated with mycorrhizal roots of Medicago truncatula. Appl. Environ. Microbiol. 73, 913–921. doi: 10.1128/AEM.02042-06
Ohtsubo, Y., Goto, H., Nagata, Y., Kudo, T., and Tsuda, M. (2006). Identification of a response regulator gene for catabolite control from a PCB-degrading β-proteobacteria. Acidovorax sp. KKS102. Mol. Microbiol. 60, 1563–1575. doi: 10.1111/j.1365-2958.2006.05197.x
Olsson, P. A., Thingstrup, I., Jakobsen, I., and Bååth, E. (1999). Estimation of the biomass of arbuscular mycorrhizal fungi in a linseed field. Soil Biol. Biochem. 31, 1879–1887. doi: 10.1016/S0038-0717(99)00119-4
Parnell, J. J., Denef, V., Park, J., Tsoi, T., and Tiedje, J. (2010). Environmentally relevant parameters affecting PCB degradation: carbon source- and growth phase-mitigated effects of the expression of the biphenyl pathway and associated genes in Burkholderia xenovorans LB400. Biodegradation 21, 147–156. doi: 10.1007/s10532-009-9289-4
Petrić, I., Hršak, D., Fingler, S., Udiković-Kolić, N., Bru, D., and Martin-Laurent, F. (2010). Insight in the PCB-degrading functional community in long-term contaminated soil under bioremediation. J. Soil Sediments 11, 290–300. doi: 10.1007/s11368-010-0299-y
Pivato, B., Offre, P., Marchelli, S., Barbonaglia, B., Mougel, C., Lemanceau, P., et al. (2009). Bacterial effects on arbuscular mycorrhizal fungi and mycorrhiza development as influenced by the bacteria, fungi, and host plant. Mycorrhiza 19, 81–90. doi: 10.1007/s00572-008-0205-2
Qin, H., Brookes, P., Xu, J. M., and Feng, Y. Z. (2014a). Bacterial degradation of Aroclor 1242 in the mycorrhizosphere soils of zucchini (Cucurbita pepo L.) inoculated with arbuscular mycorrhizal fungi. Environ. Sci. Pollut. Res. 21, 1–10. doi: 10.1007/s11356-014-3231-y
Qin, H., Brookes, P. C., and Xu, J. M. (2014b). Cucurbita spp. and Cucumis sativus enhance the dissipation of polychlorinated biphenyl congeners by stimulating soil microbial community development. Environ. Pollut. 184, 306–312. doi: 10.1016/j.envpol.2013.09.006
Schreiner, R. P., Mihara, K. L., McDaniel, H., and Bethlenfalvay, G. J. (1997). Mycorrhizal fungi influence plant and soil functions and interactions. Plant Soil 188, 199–209. doi: 10.1023/a:1004271525014
Singer, A. C., Crowley, D. E., and Thompson, I. P. (2003). Secondary plant metabolites in phytoremediation and biotransformation. Trends Biotechnol. 21, 123–130. doi: 10.1016/S0167-7799(02)00041-0
Staddon, P. L., Ramsey, C. B., Ostle, N., Ineson, P., and Fitter, A. H. (2003). Rapid turnover of hyphae of mycorrhizal fungi determined by AMS microanalysis of 14C. Science 300, 1138–1140. doi: 10.1126/science.1084269
Tawaraya, K., Naito, M., and Wagatsuma, T. (2006). Solubilization of insoluble inorganic phosphate by hyphal exudates of arbuscular mycorrhizal fungi. J. Plant Nutr. 29, 657–665. doi: 10.1080/01904160600564428
Teng, Y., Luo, Y., Sun, X., Tu, C., Xu, L., Liu, W., et al. (2010). Influence of arbuscular mycorrhiza and rhizobium on phytoremediation by alfalfa of an agricultural soil contaminated with weathered PCBs: a field study. Inter. J. Phytorem. 12, 516–533. doi: 10.1080/15226510903353120
Ter Braak, C. J. F., and Šmilauer, P. (2002). CANOCO Reference Manual and CanoDraw for Windows, Users Guide: Software for Canonical Community Ordination (Version 4.5). Ithaca, NY: Microcomputer Power.
Tillmann, S., Strömpl, C., Timmis, K. N., and Abraham, W. R. (2005). Stable isotope probing reveals the dominant role of Burkholderia species in aerobic degradation of PCBs. FEMS Microbiol. Ecol. 52, 207–217. doi: 10.1016/j.femsec.2004.11.014
Toljander, J. F., Lindahl, B. D., Paul, L. R., Elfstrand, M., and Finlay, R. D. (2007). Influence of arbuscular mycorrhizal mycelial exudates on soil bacterial growth and community structure. FEMS Microbiol. Ecol. 61, 295–304. doi: 10.1111/j.1574-6941.2007.00337.x
Wang, Q., Garrity, G. M., Tiedje, J. M., and Cole, J. R. (2007). Naïve bayesian classifier for rapid assignment of rRNA sequences into the new bacterial taxonomy. Appl. Environ. Microbiol. 73, 5261–5267. doi: 10.1128/AEM.00062-07
Welc, M., Ravnskov, S., Kieliszewska-Rokicka, B., and Larsen, J. (2010). Suppression of other soil microorganisms by mycelium of arbuscular mycorrhizal fungi in root-free soil. Soil Biol. Biochem. 42, 1534–1540. doi: 10.1016/j.soilbio.2010.05.024
Wu, Y., Ma, B., Zhou, L., Wang, H., Xu, J., Kemmitt, S., et al. (2009). Changes in the soil microbial community structure with latitude in eastern China, based on phospholipid fatty acid analysis. Appl. Soil Ecol. 43, 234–240. doi: 10.1016/j.apsoil.2009.08.002
Xu, J., Feng, Y., Wang, Y., Luo, X., Tang, J., and Lin, X. (2015). The foliar spray of Rhodopseudomonas palustris grown under Stevia residue extract promotes plant growth via changing soil microbial community. J. Soil Sediments 65, 180–192. doi: 10.1007/s11368-015-1269-1
Yadav, T. C., Khardenavis, A. A., and Kapley, A. (2014). Shifts in microbial community in response to dissolved oxygen levels in activated sludge. Bioresour. Technol. 165, 257–264. doi: 10.1016/j.biortech.2014.03.007
Yang, Y., Quensen, J., Mathieu, J., Wang, Q., Wang, J., Li, M., et al. (2014). Pyrosequencing reveals higher impact of silver nanoparticles than Ag+ on the microbial community structure of activated sludge. Water Res. 48, 317–325. doi: 10.1016/j.watres.2013.09.046
Keywords: Glomus mosseae, microbial community, mycorrhizal hyphae, polychlorinated biphenyls, bph gene
Citation: Qin H, Brookes PC and Xu J (2016) Arbuscular Mycorrhizal Fungal Hyphae Alter Soil Bacterial Community and Enhance Polychlorinated Biphenyls Dissipation. Front. Microbiol. 7:939. doi: 10.3389/fmicb.2016.00939
Received: 02 November 2015; Accepted: 01 June 2016;
Published: 15 June 2016.
Edited by:
Varenyam Achal, East China Normal University, ChinaReviewed by:
Jiandong Jiang, Nanjing Agricultural University, ChinaDayananda Chandrappa, University of Exeter, UK
Copyright © 2016 Qin, Brookes and Xu. This is an open-access article distributed under the terms of the Creative Commons Attribution License (CC BY). The use, distribution or reproduction in other forums is permitted, provided the original author(s) or licensor are credited and that the original publication in this journal is cited, in accordance with accepted academic practice. No use, distribution or reproduction is permitted which does not comply with these terms.
*Correspondence: Jianming Xu, am14dUB6anUuZWR1LmNu; Hua Qin, cWluaHVhQHphdHUuZWR1LmNu