- 1Departamento de Bioquímica, Centro de Ciências Biológicas, Universidade Federal de Pernambuco, Recife, Brazil
- 2Mestrado em Biologia Parasitária, Universidade Ceuma, São Luís, Brazil
- 3Departamento de Antibióticos, Centro de Ciências Biológicas, Universidade Federal de Pernambuco, Recife, Brazil
- 4Departamento de Bioquímica, Centro de Ciências Biológicas, Universidade Federal de Pernambuco, Recife, Pernambuco, Brazil
- 5Departamento de Micologia, Centro de Ciências Biológicas, Universidade Federal de Pernambuco, Recife, Pernambuco, Brazil
Due to the use of Cleome spinosa Jacq. (Cleomaceae) in traditional medicine against inflammatory and infectious processes, this study evaluated the in vitro antimicrobial potential and phytochemical composition of extracts from its roots and leaves. From leaves (L) and roots (R) of C. spinosa different extracts were obtained (cyclohexane: ChL and ChR; chloroform: CL and CR; ethyl acetate: EAL and EAR, methanol: ML and MR). The antimicrobial activity was evaluated by the broth microdilution method to obtain the minimum inhibitory (MIC) and microbicidal (MMC) concentrations against 17 species, including bacteria and yeasts. Additionally, antimicrobial and combinatory effects with oxacillin were assessed against eight clinical isolates of Staphylococcus aureus. All C. spinosa extracts showed a broad spectrum of antimicrobial activity, as they have inhibited all tested bacteria and yeasts. This activity seems to be related to the phytochemicals (flavonoid, terpenoids and saponins) detected into the extracts of C. spinosa. ChL and CL extracts were the most actives, with MIC less than 1 mg/mL against S. aureus, Bacillus subtilis, and Micrococcus luteus. It is important to note that these concentrations are much lower than their 50% hemolysis concentration (HC50) values. Strong correlations were found between the average MIC against S. aureus and their phenolic (r = −0.89) and flavonoid content (r = −0.87), reinforcing the possible role of these metabolite classes on the antimicrobial activity of C. spinosa derived extracts. Moreover, CL and CR showed the best inhibitory activity against S. aureus clinical isolates, they also showed synergistic action with oxacillin against all these strains (at least at one combined proportion). These results encourage the identification of active substances which could be used as lead(s) molecules in the development of new antimicrobial drugs.
Introduction
Microbial resistance to antibiotics is one of the most serious public health problems, especially in developing countries where infectious diseases still represent a major cause of human mortality (World Health Organization, 2014). Among the microorganisms that represent a significant health threat, Staphylococcus aureus is highlighted as this species is responsible for a number of human illness conditions, such as skin infections and septicemia (Adhikari et al., 2012). Among fungal pathogens, the genus Candida also has high clinical relevance and it is responsible for a wide variety of infections, from superficial mucocutaneous to more invasive diseases (Kim and Sudbery, 2011). Approximately 75% of women, at least once in their life, develop candidiasis caused by C. albicans, C. glabrata, C. parapsilosis, C. tropicalis, and/or C. krusei (Simonetti et al., 2014). This issue encourages the search for novel antimicrobial agents.
One of the oldest forms of medical practice is the use of plants for therapeutic purposes; teas, syrups, tinctures, among others have been used as medicines and in many cases come to be the sole therapeutic resource of certain communities and ethnic groups (Amorozo, 2002; Oliveira et al., 2012). Thus, knowledge about the therapeutic potential of plants is of great scientific and medical interest, as an effective alternative to the battle against resistant microorganisms (dos Santos et al., 2015).
Some species of the genus Cleome (Cleomaceae) have been investigated for medical properties and some of them had their anti-inflammatory (Albarello et al., 2013), analgesic (Bose et al., 2007), and antimicrobial (Sudhakar et al., 2006; McNeil et al., 2010) activities evaluated. C. spinosa Jacq is a perennial herb that grows in the Central-West, Northeast, North and Southeast of Brazil and is known in Brazil as “Mussambê.” Its leaves and flowers have been used in traditional medicine: leaves infusion is used in the treatment of asthma, cough, and bronchitis, while flowers infusion is used against fever (Agra et al., 2007). So far, some pharmacological actions have been proven such as antimicrobial, antinociceptive, anti-inflammatory, anthelmintic (McNeil et al., 2010; Albarello et al., 2013; Andrade et al., 2014). Regarding the antimicrobial activity, it is only reported for essential oils from leaves, which significantly inhibit Streptococcus pyogenes Group A (McNeil et al., 2010). Based on the uses of C. spinosa in folk medicine, it is attractive to analyze the antimicrobial potential of other tissue, using also different extraction methods. In this sense, the first step of this study was to evaluate the antimicrobial activity of different extracts from leaves and roots of C. spinosa against a set of 17 microbial species. The phytochemical constituents of each extract were determined and correlated with the antimicrobial action. The active extracts were tested against clinical isolates of S. aureus and their combinatory effects with oxacillin were also evaluated.
Materials and Methods
Plant Material
The leaves and roots of C. spinosa were collected at Universidade Federal Rural de Pernambuco – Dois Irmãos (Latitude 8° 01′22.3″; Longitude: 34° 57′15.8″). The botanical material was identified by Dr. Marlene Carvalho de Alencar Barbosa and deposited in the Herbarium UFPE - Geraldo Mariz, at the Department of Botany, Universidade Federal de Pernambuco (UFPE), under the voucher number 76,556.
Extract Preparation
Samples (100 g) from each tissue of C. spinosa were dried and milled and separately subjected to Soxhlet extraction using an eluotropic series of solvents (in the following order: cyclohexane, chloroform, ethyl acetate and methanol) at a temperature below of the boiling point of each solvent. All samples were subjected to saturation at reflux for 24 h. After this time, the extracts were filtered through a Whatman filter paper No 1. All extracts from leaves (L) or roots (R) (cyclohexane: ChL and ChR; chloroform: CL and CR; ethyl acetate: EAL and EAR: methanol: ML and MR) were concentrated until the complete removal of the solvent on a rotating evaporator at 45°C (under reduced pressure) and stored for later analyses. For phytochemical analysis the extracts were dissolved in ethanol (1 mg/mL), while they were solubilized in a sterile dimethylsulfoxide solution (DMSO; 10% in water) at the concentration of 100 mg/mL for all biological assays.
Phytochemical Analysis
An aliquot (10 μL) of each extract (1 mg/mL in ethanol) obtained from leaves and roots of C. spinosa was subjected to qualitative phytochemical analysis to ascertain the presence of secondary metabolites such as: alkaloids, coumarins, anthracene and cinnamic acid derivatives, flavonoids, monoterpenoides, sesquiterpenoids, diterpenoids (Wagner and Bladt, 1996); tannins, triterpenoids and steroids (Harborne, 1998); proanthocyanidins and leucoanthocyanidins (Robertson et al., 1957); reducing sugars (Russell and Morris, 1982) and saponins (Costa, 2002) respectively. The compounds classes were visualized as aid thin layer chromatography (TLC) on silica gel 60 F254 (Merck, Germany), different systems of development and adequate visualization techniques were used: Dragendorff, NEU-PEG, KOH-Ethanol, Liebermann-Burchard, vanillin-sulfuric acid and others reagents, according to the respective method.
Determination of Total Phenolic Content
The total phenol content in each extract was determined as reported by Li et al. (2008). Samples (200 μL) of each extract at 1 mg/mL were mixed with 1 mL of diluted Folin-Ciocalteu reagent (1:10 in water). After 4 min, this mixture received 800 μL of saturated solution sodium carbonate (75 mg/mL), followed by 2 h of incubation at room temperature (protected from light). The absorbance was measured at 765 nm. Gallic acid (0–500 mg/L) was used as standard in a calibration curve. The results were expressed as mg of gallic acid equivalents (GAE)/g dry weight of plant extract.
Determination of Flavonoids
The quantification of total flavonoid content in each extract followed the methodology proposed by Woisky and Salatino (1998). In briefly, to 0.5 mL of diluted samples (1 mg) was added 0.5 mL of 2% AlCl3 (w/v) solution prepared in methanol. After 30 min of incubation at room temperature, protected from light, the absorbance was measured at 420 nm. All measurements were done in triplicate. The results were expressed as mg of quercetin equivalent (QE)/g dry weight of plant extract.
Antimicrobial Assays
Microorganisms
Twenty bacterial strains from 12 species (Bacillus subtilis, Enterococcus faecalis, Micrococcus luteus, Mycobacterium smegmatis, Staphylococcus epidermidis, Streptococcus mutans, Escherichia coli, Klebsiella pneumoniae, Proteus mirabilis, Pseudomonas aeruginosa, Salmonella enteritidis) provided by the Culture Collection UFPEDA (Department of Antibiotics, UFPE) and five Candida species (Candida albicans, Candida krusei, Candida glabrata, Candida parapsilosis, Candida tropicalis) obtained from the Culture Collection URM (Department of Mycology, UFPE) were used for the antimicrobial tests, according to Table 1.
Determination of Minimum Inhibitory Concentration (MIC) and Minimal Microbicidal Concentration (MMC)
The minimum inhibitory (MIC) and minimal microbicidal (MMC) concentrations were determined by the broth microdilution method (Oliveira et al., 2012). Sabouraud broth was used for yeasts and Mueller Hinton broth for bacterial strains, except for Streptococcus mutans, which was cultivated in Brain-Heart infusion broth (BHI). Tested microorganisms were standardized by the Mcfarland turbidity scale equivalent to the tube 0.5, corresponding to a concentration of approximately 107 CFU/mL for yeasts and 108 CFU/mL for bacteria. Serial dilutions of all extracts were made in 96-well plates to obtain concentrations ranging from 50 to 0.09 mg/mL. Following that, each well received 10 μL of microorganism suspensions and the plates were incubated at 25°C for 48 h for yeasts and at 37°C for 24 h for bacteria. After each period, 15 μL of 0.01% resazurin was added, as a colorimetric indicator of cell viability. Then, the microplates were re-incubated for 4 h and the lowest concentration of extract that inhibited microbial growth was recorded as the MIC. Then 50 μL of the solution from each inhibited well was collected and transferred to agar plates and re-incubated as described above for the yeasts and bacteria. The complete absence of growth on the agar surface with the lowest concentration of the sample was defined as the MMC. Commercially available antibiotics were used as positive control for bacteria (ampicillin and oxacillin) and yeast (amphotericin B, fluconazole and itraconazole). Sterile DMSO aqueous solution (10%) was used as negative control.
Evaluation of Combinatory Effects of Chloroform Extracts and Oxacillin
Combinatory effects between chloroform extracts of C. spinosa and oxacillin were assessed using different S. aureus strains (UFPEDA 670, 672, 683, 700, 705, 709, 726, and 731). Briefly, samples were combined at different proportions of plant extract and drug (1:1, 1:2 and 1:3; final volume: 200 μL) using stock solutions of each extract (6.25 mg/mL) and oxacillin (0.5 mg/mL). Serial dilutions of each combination were made in 96-well plates to obtain concentrations ranging from 3.15 mg/mL to 0.003 mg/mL and 0.25 mg/mL to 0.0002 mg/mL; 2.08 mg/mL to 0.002 mg/mL and 0.33 mg/mL to 0.0003 mg/mL; and 1.56 mg/mL to 0.001 mg/mL and 0.375 mg/mL to 0.0004 mg/mL for plant extract and drug combined at 1:1, 1:2 and 1:3. The antibacterial activity was performed as described for MIC determination. The Fractional Inhibitory Concentration (ΣFIC) was calculated according to the equation:
ΣFIC = (MICE + D/MICE) + (MICD + E/MICD) MICE + D: minimal inhibitory concentration of extract in combination with oxacillin; MICD+E: minimal inhibitory concentration of oxacillin in combination with extract. Results were considered: synergistic (ΣFIC < 0.5); additive (0.5 < ΣFIC < 1); non-interactive (1 < ΣFIC < 4); or antagonist (ΣFIC > 4) (Odds, 2003; Vuuren and Viljoen, 2011).
Hemolytic Assay
Blood (5–10 mL) was obtained from healthy nonsmoking volunteers by venipuncture, after a written informed consent was obtained. Human erythrocytes from citrated blood were immediately isolated by centrifugation at 1500 rpm for 10 min at 4°C. After removal of plasma and buffy coat, the erythrocytes were washed three times with phosphate-buffered saline (PBS; pH 7.4) and then resuspended using the same buffer and a 1% erythrocyte suspension was prepared. The hemolytic activity of the crude extract was tested under in vitro conditions. Each tube received 1.1 mL of erythrocyte suspension and 0.4 mL of extract of various concentrations (31.25–1000 μg/mL) were added. The negative control was only solvent and the positive control received 0.4 mL of Triton X-100. After 60-min incubation at room temperature, cells were centrifuged and the supernatant was used to measure the absorbance of the liberated hemoglobin at 540 nm (Oliveira et al., 2012). The average value was calculated from triplicate assays. The relative hemolytic activity was expressed in relation to Triton X-100 and calculated by the following formula:
Where Ab was the absorbance of the control (blank, without extract), As was the absorbance in the presence of the extract, and Ac was the absorbance in the presence of Triton X-100. Hemolysis concentration was calculated.
Statistical Analyses
Each experiment was performed in triplicate in at least two independent experiments. Statistical analyses were performed by One-way analysis of variance (ANOVA). All analyses were carried out using GraphPrism, version 4. Differences were considered significant at p < 0.05. The concentration needed for 50% of hemolysis (HC50 values) was calculated graphically by linear regression analysis. The correlation indices were calculated using the Pearson coefficient (r) and were classified as strong (r: −1.0 to −0.7), moderate (r: −0.69 to −0.5), week (r: −0.49 to −0.3) or negligible (r < −0.3).
Results
Phytochemical Analyses of the Samples
Analysis of the yield of all the extractions showed that the C. spinosa leaves had a better result, in comparison with the roots. With regards to the solvents, methanol extracts showed the better yield, when compared to the hexane, chloroform and ethyl acetate extracts, as showed in Table 2.
The qualitative phytochemical analysis detected the presence of reducing sugars, antracenic derivatives, flavonoids and terpenes into all extracts. Additionally, ChL, CL, CR and EAR also presented monoterpenes, sesquiterpenes, diterpenes, triterpenes, and steroids; the EAL showed saponins, triterpenes, and steroids; ML exhibited saponins, monoterpenes, and sesquiterpenes; ChR revealed coumarins, triterpenes, and steroids; and MR presented coumarins, cinnamic acid derivatives, saponins, monoterpenes, sesquiterpenes, and diterpenes.
The estimation of total phenolic content revealed that EAR (256.94 ± 0.48 mg GAE/g), ML (255.69 ± 0.28 mg GAE/g) and CR (215.23 ± 0.64 mg GAE/g) exhibited the highest phenolic content (p < 0.05). The other extracts showed phenolic content values ranging from 72.73 mg GAE/g to 201.71 mg GAE/g (Table 2). On the hand, CL and ML showed the highest flavonoids content with values of 153.70 ± 0.58 mg QE/g and 71.83 ± 0.76 mg QE/g, respectively. The total phenolic and flavonoids contents showed a weak correlation (r = 0.48).
Antimicrobial Screening
The antimicrobial activity of the organic extracts from leaves and roots of C. spinosa are presented in Tables 3–5. Overall, all extracts from both leaves and roots of C. spinosa exhibited antimicrobial activity with broad spectrum, as they inhibited all tested bacteria and yeasts.
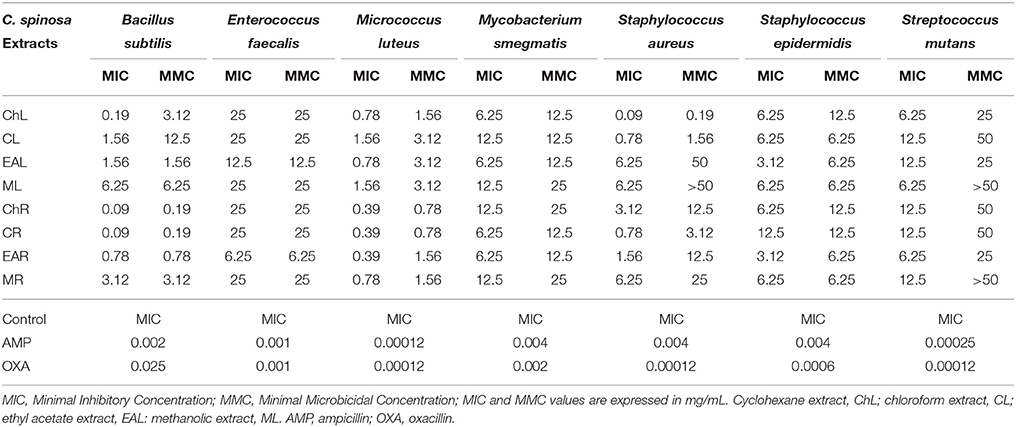
Table 3. Antimicrobial Activity of organic extracts from leaves and roots of C. spinosa against selected Gram-positive bacteria.
Furthermore, the best antibacterial results observed were provided by the extracts obtained using ciclohexane and chloroform, whose MIC ranged from 0.09 mg/mL to 12.5 mg/mL (Tables 3, 4). We considered MIC less than or equal to 1.56 mg/mL as considerable inhibition. Among extracts from leaves, ChL presented the best antibacterial activity against S. aureus, B. subtilis and M. luteus with MIC values of 0.09 mg/mL, 0.19 mg/mL and 0.78 mg/mL, respectively. CL was also more active against these same microorganisms (MIC ≤ 1.56 mg/mL). EAL best inhibited M. luteus (MIC = 0.78 mg/mL) and B. subtilis (MIC = 1.56 mg/mL) and ML best inhibited M. luteus (MIC = 1.56 mg/mL). In the case of the root extracts, ChR and CR were particularly effective against B. subtilis (MIC of 0.09 mg/mL for both extracts) and M. luteus (MIC of 0.39 mg/mL for both extracts). Additionally, CR demonstrated anti-S. aureus potential (MIC = 0.78 mg/mL). EAR showed markedly activity against M. luteus (MIC = 0.39 mg/mL), B. subtilis (MIC = 0.78 mg/mL), S. aureus (MIC = 1.56 mg/mL). The MR extract exhibited its best activity against M. luteus (MIC = 0.78 mg/mL).
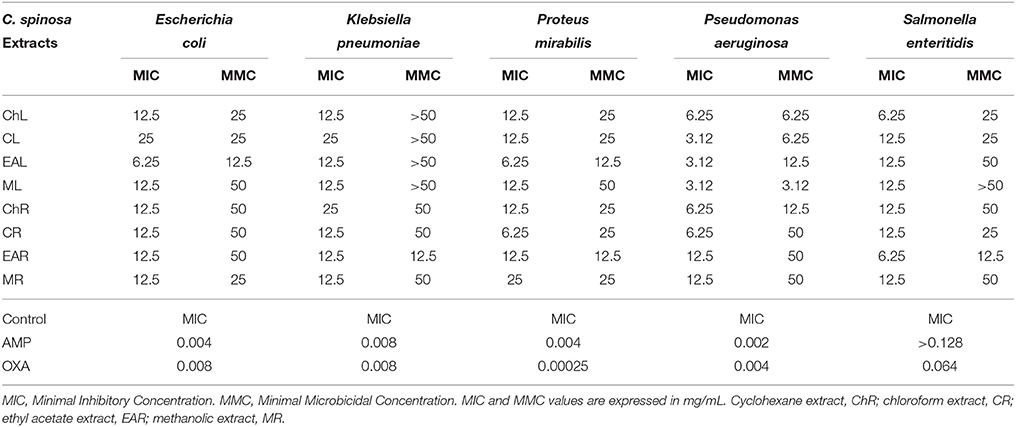
Table 4. Antimicrobial Activity of organic extracts from leaves and roots of C. spinosa against selected Gram-negative bacteria.
The antifungal activity of the C. spinosa extracts is presented in Table 5. The leaf extracts showed MIC values ranging from 3.12 to 12.5 mg/mL and MMC between 6.25 and 50 mg/mL. The extracts from leaves were less active, as they inhibited yeasts in concentrations between 6.25 and 12.5 mg/mL and killed them at dosage from 12.5 mg/mL. Among leaves extracts, the CL was the most active against all Candida species tested, except C. krusei, followed by ChL. The extracts from the root part showed a circumspect activity, with ChR effective against C. glabrata, C. krusei and C. parasiplosis; CR against C. krusei and C. parasiplosis; EAR against C. parasiplosis; and MR against C. tropicalis and C. parasiplosis (Table 5).
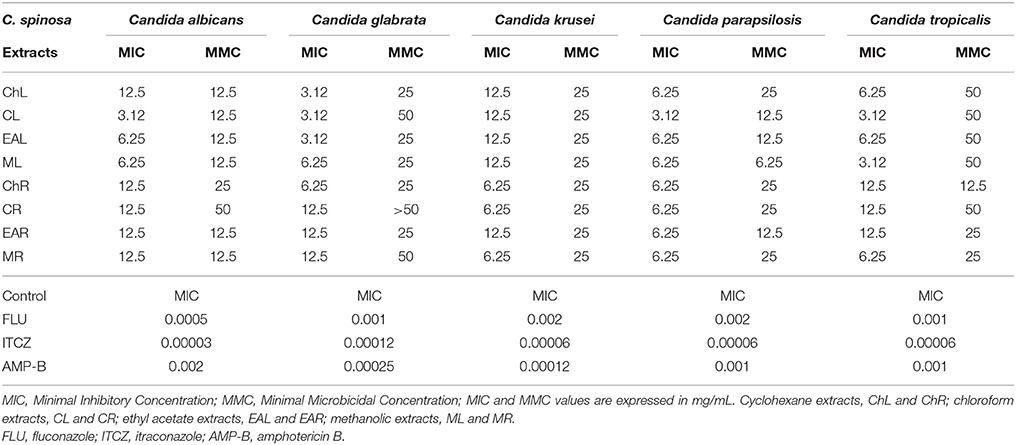
Table 5. Antimicrobial Activity of organic extracts from leaves and roots of C. spinosa against Candida spp.
Hemolytic Assay
The hemolytic activity of each extract was performed using fresh human erythrocytes and they showed low cytotoxicity (Figure 1). Even at the highest tested concentration (1000 μg/mL), the extracts CR, CL and ChL induced low levels of hemolysis (1.18, 4.54, and 11.13%, respectively). Regarding the HC50, ChR was the most toxic extract (470.88 μg/mL), followed by EAL (648.97 μg/mL), ML (725.58 μg/mL), EAR (870.72 μg/mL), MR (808.51 μg/mL). The less toxic extracts had theoretical HC50 values of 4773.602 μg/mL, 13409.35 μg/mL and 151.389.2 μg/mL for ChL, CL and MR, respectively. In addition, these HC50 values were not correlated with either flavonoid (r = 0.23) or phenolic content (r = −0.10).
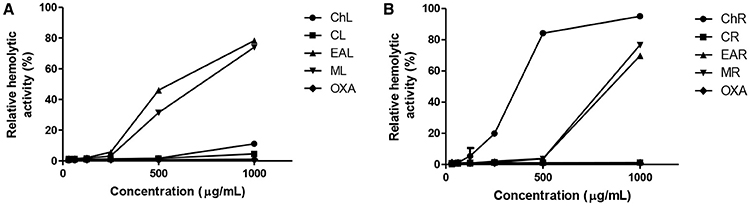
Figure 1. Hemolytic Activity of organic extracts from leaves (A) and roots (B) of C. spinosa. Cyclohexane extracts, ChL and ChR; chloroform extracts, CL and CR; ethyl acetate extracts, EAL and EAR; methanolic extracts, ML and MR; Oxacillin, OXA. The hemotytic activity in relation to Triton X-100 (0.4–1.1 mL of erythrocyte suspension).
Anti-S. aureus Activity and Combinatory Effects with Oxacillin
Given the high medical importance of the antibiotic resistant in S. aureus strains, the action of the extracts was evaluated in association with oxacillin against clinical isolates (Table 6). The extracts were effective against all strains. However, those obtained using chloroform showed the best efficiencies (p < 0.05), with MIC50 values (concentration able to inhibit 50% of the strains) of 0.78 mg/mL for leaves and 3.12 mg/mL for roots; while the cyclohexane extracts exhibited MIC50 values of 6.25 mg/mL. Then, the combinatorial effect of the chloroform extracts with oxacillin was evaluated. Almost all combinations had synergistic activities, the exceptions were the non-interactive (for the combination CLF:OXA at 1:1) and additive effects (CLR:OXA at 1:1; and CLF:OXA at 1:2) observed against the isolate 683 (Figure 2). CLR only exhibited synergetic and additive effects.
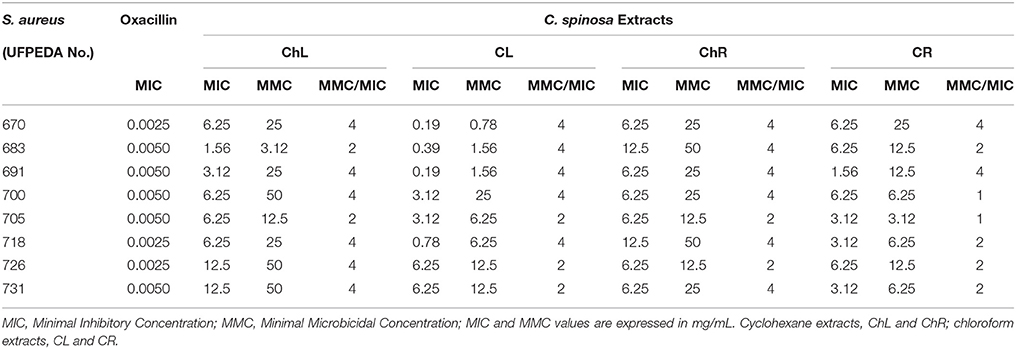
Table 6. Antimicrobial Activity of organic extracts from leaves and roots of C. spinosa against Staphylococcus aureus.
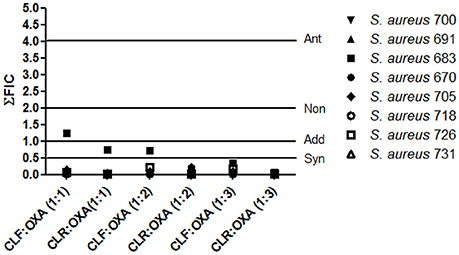
Figure 2. Combinatory effect of Oxacillin and chloroform extracts from leaves (CLF) and roots (CLR) of C. spinosa against clinical isolates of Staphylococcus aureus. Each extract solution (6.25 mg/mL) was mixed with oxacillin solution (OXA; 0.5 mg/mL) at different proportion extract/drug (1:1, 1:2; 1:3). The Fractional Inhibitory Concentration (ΣFIC) was calculated as demonstrated at methods section. Interpretation: Syn, synergistic effect (ΣFIC < 0.5); Add, additive effect (0.5 < ΣFIC < 1); Non, non-interactive effect (1 < ΣFIC < 4); Ant, antagonist effect (ΣFIC > 4)
Discussion
Considering that the World is facing a growing number of multidrug-resistant microorganisms, numerous studies have been conducted in order to select new compounds, such as those from natural resources which are of extremely importance (World Health Organization, 2014; dos Santos et al., 2015). The plants are, admittedly, a valuable reservoir of bioactive compounds of substantial medical importance (Harvey et al., 2015). So, this study has evaluated the antimicrobial activity of C. spinosa, a species known for their use in traditional medicine to treat infections. Some studies have shown antimicrobial, insecticidal, and antioxidant activities in essential oils obtained from their aerial parts of C. spinosa (McNeil et al., 2010), others had evaluated the anti-inflammatory and antinociceptive effects of methanol extracts from different ways of cultivation (Albarello et al., 2013).
Qualitative phytochemical analysis clearly demonstrated the presence of number important active constituents and revealed that both C. spinosa tissues have similar phytochemical constitution. Only the extracts from leaves (cyclohexane, chloroform, ethyl acetate) showed proanthocyanidins and leucoanthocyanidins. Indeed, all kind of metabolite classes detected into the samples (saponins, flavonoids, tannins, coumarins and terpenoids) are well known to have significant inhibitory action against bacteria and fungi (Hayek et al., 2013). This fact can justify why all extracts showed antimicrobial activity. On the other hand, quantitative assays revealed that the extracts have different phenolic and flavonoid contents which can be correlated with each biological activity. For example, for roots derived extracts a strong direct correlation was found between their average MIC values (for all tested bacteria) and phenolic (r = −0.87) and flavonoid contents (r = −0.96). For leaves extracts inverse strong and moderate correlations were found (r-value of 0.56 and 0.88 for correlation with phenolic and flavonoid contents, respectively), suggesting that the antimicrobial activity might be an effect of other types of compounds. It is important to note that when the correlations between polyphenols/flavonoid content were determined using and average MIC or HC50, a negative r-value (r = −1) is considered as perfect direct correlation.
On the basis of the results shown in Tables 4, 5, C. spinosa extracts demonstrated to be more active on Gram-positive than on Gram-negative bacteria. This was not a surprise since previous studies have reported that generally plant extracts are usually more active against Gram-positive bacteria than Gram-negative bacteria, and the susceptibility may be due to structural differences in the cell wall of these classes of bacteria. Cells of Gram-negative bacteria are surrounded by an additional outer membrane, which provide them with a hydrophilic surface that functions as a permeability barrier for many substances including natural compounds (Hemaiswarya et al., 2008; Briers and Lavigne, 2015). Additional contribution to intrinsic resistance in Gram-negative bacteria is provided by efflux pumps (Eps) which actively pump out a broad spectrum of compounds (such as antibiotics, toxins, β-lactamase inhibitors, dyes, detergents, lipids, and molecules involved in quorum sensing) from the periplasm to the outside of the cell. The overexpression of EPs (such as Resistance-Nodulation-Division-type efflux pumps) is recognized as a major component in the development of the multidrug resistance phenotype in Gram-negative bacteria (Opperman and Nguyen, 2015; Venter et al., 2015). The ineffectiveness of plant compounds toward Gram-negative pathogens has been proposed to be strongly related to EPs as the combination of plant antimicrobials with EPs inhibitors leads to a striking increase in antimicrobial activity (Tegos et al., 2002).
Although the of action mechanisms natural products are distinct, the cytoplasmic membrane ranks as the most common site of action for secondary metabolites. They usually act through cell lysis, triggering the leakage of cellular contents and consequently cell death (Da Silva et al., 2013). The interaction with genetic material and protein synthesis is also a possible factor regarded to the promotion of the therapeutic action. In this case, when there is a contact with the genetic material, the compound is able to promote changes in the genetic machinery, whose result is ineffective transcription and disturbance of vital functions for the cell (Hayek et al., 2013; Gyawali and Ibrahim, 2014). The phenolic compounds (polyphenols, tannins, and flavonoid) can act at two different levels: the cell membrane and cell wall of the microorganisms (Taguri et al., 2006). They can also penetrate into bacterial cells and coagulate cell content (Tian et al., 2009). The antimicrobial property of saponins is due to a lipophilic portion into its structure (aglycon or sapogenin) and a hydrophilic core comprising one or more sugars (Costa et al., 2010).
Among all extracts, ChL and CR were the most active and inhibited the growth of B. subtilis, M. luteus, S. aureus. They demonstrated an average MIC of 0.35 mg/mL and 0.42 mg/mL (these values were not significantly different at the p > 0.05 level), and a predominantly bactericidal behavior: MMC/MIC ratio < 4 (Pankey and Sabath, 2004). To those same pathogens, the average MIC for ChL was up to 13-fold lower than the other extracts, while for CR was up to 8-fold lower. These extracts, qualitatively, showed the same phytochemical composition than the others, except for the presence of proanthocyanidins and leucoanthocyanidins in ChL. CR showed a higher phenolic and flavonoid content and a lower hemolytic activity than ChL (p < 0.05). Interestingly, a negative correlation was found between their MIC values (r = −0.21), whereas the inhibition order was S. aureus > B. subtilis > M. luteus to ChL and it was B. subtilis > M. luteus > S. aureus to CR. In fact, all extracts from roots showed markedly inhibitory activity against B. subtilis. This species is a model organism present in the soil and widely known for its ability to promote plant growth, and thus has great importance in agriculture. However, little information is available about the ecological role and the mechanisms involved in the interaction with plants (Kobayashi, 2015).
On the other hand, C. spinosa extracts had not shown an efficacy against Candida spp. as good as that observed for the Gram-positive bacteria. Among all yeasts tested, C. glabrata and C. tropicalis were the most sensitive to the fungistatic action (ratio of MMC/MIC > 4). Infections caused by Candida spp. are especially found in immunosuppressed patients, as those with cancer, transplantation, postoperative, HIV, especially in developing countries (Pitman et al., 2011). Allied to this, the lack of new antifungals and the growing microbial resistance (Odom, 2014) also make these results relevant and important.
Therefore, due to the great potential shown by C. spinosa extracts against S. aureus, we decided to investigate the effect of cyclohexanic and chloroformic extracts against clinical isolates. The search for new anti-S. aureus agents is extremely important, since infections caused by multidrug resistant strains have been growing worldwide and are one of the most serious problems within health care facilities (Stryjewski and Corey, 2014). Such issues have been accounted to the excessive use of drugs (Kim et al., 2015), which results in development of new resistance mechanisms (Dordel et al., 2014). Our data shows that the chloroform extracts were able to inhibit and kill with good efficiency against different clinical strains, the most active was CL, with MIC50 values ranging from 4- to 8-fold lower than other extracts. It is important to note that these concentrations are much lower than their HC50 values. Both chloroform extracts showed bactericidal action, whereas their MIC values and MMC showed weak positive (r = 0.09) and moderate negative (r = −0.49) correlations, respectively. Then, it is possible that different mechanisms are responsible for the results observed. Furthermore, strong correlations were found between the average MIC against S. aureus and their phenolic (r = −0.89) and flavonoid content (r = −0.87), reinforcing the possible role of these metabolite classes on the antimicrobial activity of C. spinosa derived extracts.
In addition, given the importance of the S. aureus pathogenicity and its ability to acquire resistance, new ways to combat this pathogen must be developed, among them is combination therapy using compounds that act or not on the same target. Therefore, to test the combining action became a key step in phytochemical studies (Wagner and Ulrich-Merzenich, 2009; Bessa et al., 2015; dos Santos et al., 2015). CL and CR extracts were able to increase the effectiveness of this antibiotic, mainly through synergistic interaction. Some plant-derived products have demonstrated the ability to reverse resistance to oxacillin (Jenkins and Cooper, 2012; Bessa et al., 2015), and the perspective is that the use of therapies based on the combination of phytochemicals and antibiotics grow in conventional medicine, as it may reduce the likelihood of dose-dependent toxicity and mutagenicity of antimicrobials (Boucher and Tam, 2006; Wagner and Ulrich-Merzenich, 2009).
Conclusion
This research demonstrates the antimicrobial potential of leaves and roots from C. spinosa. These chloroform and cyclohexane extracts were especially active against Gram-positive organisms, and they inhibited S. aureus strains with different phenotypes of resistance. Similarly, the most active extracts increased the action of oxacillin against different S. aureus strains. The next steps are focused on the isolation and identification of the active (s) compound (s) in each extract which could be used as lead(s) molecule (s) in the development of new antimicrobial drugs.
Author Contributions
AS, MC, VD designed the study protocol, and participated in its design and coordination. AS, JA, MS carried out the antimicrobial assays. AS, LS, CF, MS, VD contributed to drafting the manuscript and/ or critically revising the paper and intellectual content. All authors read and approved the final manuscript.
Conflict of Interest Statement
The authors declare that the research was conducted in the absence of any commercial or financial relationships that could be construed as a potential conflict of interest.
Acknowledgments
The authors are grateful to Conselho Nacional de Desenvolvimento Científico e Tecnológico (CNPq), Coordenação de Aperfeiçoamento de Pessoal de Nível Superior (CAPES) and Fundação de Amparo à Ciência do Estado de Pernambuco (FACEPE) for the financial support to this study. We express our gratitude to Dr. Haroudo Satiro Xavier (UFPE) for performing the phytochemical analysis.
References
Adhikari, R. P., Ajao, A. O., Aman, M. J., Karauzum, H., Sarwar, J., Lydecker, A. D., et al. (2012). Lower antibody levels to Staphylococcus aureus exotoxins are associated with sepsis in hospitalized adults with invasive Staphylococcus aureus infections. J. Infect. Dis. 206, 915–923. doi: 10.1093/infdis/jis462
Agra, M. F., França, P. F., and Barbosa-Filho, J. M. (2007). Synopsis of the plants known as medicinal and poisonous in Northeast of Brazil. Rev. Bras. Farmacogn. 17, 114–140. doi: 10.1590/S0102-695X2007000100021
Albarello, N., Simões-Gurgel, C., Castro, T. C., Gayer, C. R. M., Coelho, M. G. P., Moura, R. S., et al. (2013). Anti-inflammatory and antinociceptive activity of field-growth plants and tissue culture of Cleome spinosa (Jacq) in mice. J. Med. Plants. Res. 7, 1043–1049. doi: 10.5897/JMPR12.153
Amorozo, M. C. D. M. (2002). Uso e diversidade de plantas medicinais em Santo Antônio do Leverger, MT, Brasil. Acta Bot. Bras. 16, 189–203. doi: 10.1590/S0102-33062002000200006
Andrade, F. D., Ribeiro, A. R. C., Medeiros, M. C., Fonseca, S. S., Athayde, A. C. R., Ferreira, A. F., et al. (2014). Anthelmintic action of the hydroalcoholic extract of the root of Tarenaya spinosa (Jacq) Raf. for Haemonchus contortus control in sheep. Pes. Vet. Bras. 34, 942–946. doi: 10.1590/S0100-736X2014001000003
Bessa, L. J., Palmeira, A., Gomes, A. S., Vasconcelos, V., Sousa, E., Pinto, M., et al. (2015). Synergistic Effects between thioxanthones and oxacillin against methicillin-resistant Staphylococcus aureus. Microb. Drug Resist. 21, 404–415. doi: 10.1089/mdr.2014.0162
Bose, A., Gupta, J. K., Dash, G. K., Ghosh, T., SI, S., and Panda, D. S. (2007). Diuretic and antibacterial activity of aqueous extract of Cleome rutidosperma. Indian J. Pharm. Sci. 69, 292–294. doi: 10.4103/0250-474X.33162
Boucher, A. N., and Tam, V. H. (2006). Mathematical formulation of additivity for antimicrobial agents. Diagn. Microbiol. Infect. Dis. 55, 319–325. doi: 10.1016/j.diagmicrobio.2006.01.024
Briers, Y., and Lavigne, R. (2015). Breaking barriers: expansion of the use of endolysins as novel antibacterials against Gram-negative bacteria. Future Microbiol. 10, 377–390. doi: 10.2217/fmb.15.8
Costa, D. A., Chaves, M. H., Silva, W. C. S., and Costa, C. L. S. (2010). Constituintes químicos, fenóis totais e atividade antioxidante de Sterculiastriata St. Hil. et Naudin. Acta Am. 40, 207–212. doi: 10.1590/S0044-59672010000100026
Da Silva, L. C. N., Sandes, J. M., de Paiva, M. M., de Araújo, J. M., Figueiredo, R. C. B. Q. D., da Silva, M. V., et al. (2013). Anti-Staphylococcus aureus action of three Caatinga fruits evaluated by electron microscopy. Nat. Prod. Res. 27, 1492–1496. doi: 10.1080/14786419.2012.722090
Dordel, J., Kim, C., Chung, M., de la Gándara, M. P., Holden, M. T., Parkhill, J., et al. (2014). Novel determinants of antibiotic resistance: identification of mutated loci in highly methicillin-resistant subpopulations of methicillin-resistant Staphylococcus aureus. mBio 5:e01000–e01013. doi: 10.1128/mbio.01000-e01013
dos Santos, A. T. B., da Silva Araújo, T. F., Da Silva, L. C. N., da Silva, C. B., de Oliveira, A. F. M., Araújo, J. M., et al. (2015). Organic extracts from Indigofera suffruticosa leaves have antimicrobial and synergic actions with Erythromycin against Staphylococcus aureus. Front. Microbiol. 6:13. doi: 10.3389/fmicb.2015.00013
Gyawali, R., and Ibrahim, S. A. (2014). Natural products as antimicrobial agents. Food Control 46, 412–429. doi: 10.1016/j.foodcont.2014.05.047
Harvey, A. L., Edrada-Ebel, R., and Quinn, R. J. (2015). The re-emergence of natural products for drug discovery in the genomics era. Nat. Rev. Drug Discov. 14, 111–129. doi: 10.1038/nrd4510
Hayek, S. A., Gyawali, R., and Ibrahim, S. A. (2013). “Antimicrobial natural products” in Microbial Pathogens and Strategies for Combating them: Science, Technology and Education, ed A. Méndez-Vilas (Badajoz: FORMATEX), 910–921.
Hemaiswarya, S., Kruthiventi, A. K., and Doble, M. (2008). Synergism between natural products and antibiotics against infectious diseases. Phytomedicine 15, 639–652. doi: 10.1016/j.phymed.2008.06.008
Jenkins, R. E., and Cooper, R. (2012). Synergy between oxacillin and manuka honey sensitizes methicillin-resistant Staphylococcus aureus to oxacillin. J. Antimicrob. Chemother. 67, 1405–1407. doi: 10.1093/jac/dks071
Kim, J., and Sudbery, P. (2011). Candida albicans, a major human fungal pathogen. J. Microbiol. 49, 171–177. doi: 10.1007/s12275-011-1064-7
Kim, N. H., Koo, H. L., Choe, P. G., Cheon, S., Kim, M. S., Lee, M. J., et al. (2015). Inappropriate continued empirical vancomycin use in a hospital with a high prevalence of methicillin-resistant Staphylococcus aureus. Antimicrob. Agents Chemother. 59, 811–817. doi: 10.1128/AAC.04523-14
Kobayashi, K. (2015). Plant methyl salicylate induces defense responses in the rhizobacterium Bacillus subtilis. Environ. Microbiol. 17, 1365–1376. doi: 10.1111/1462-2920.12613
Li, A. B., Wonga, C. C., Ka-Wing, C., and Chen, F. (2008). Antioxidant properties in vitro and total phenolic contents in methanol extracts from medicinal plants. LWT-Food Sci. Technol. 41, 385–390. doi: 10.1016/j.lwt.2007.03.011
McNeil, M. J., Porter, R. B., Williams, L. A., and Rainford, L. (2010). Chemical composition and antimicrobial activity of the essential oils from Cleome spinosa. Nat. Prod. Commun. 5, 1301–1306.
Odds, F. C. (2003). Synergy, antagonism, and what the chequerboard puts between them. J. Antimicrob. Chem. 52, 1–1. doi: 10.1093/jac/dkg301
Odom, A. R. (2014). The triphenylethylenes, a novel class of antifungals. mBio 5:e01126–14. doi: 10.1128/mBio.01126-14
Oliveira, Y. L. C., Silva, L. C. N., Silva, A. G., Macedo, A. J., Araújo, J. M., Correia, M. T. S., et al. (2012). Antimicrobial activity and phytochemical screening of Buchenavia tetraphylla (Aubl) R. A. Howard (Combretaceae: Combretoideae). Sci. World J. 6:849302. doi: 10.1100/2012/849302
Opperman, T. J., and Nguyen, S. T. (2015). Recent advances toward a molecular mechanism of efflux pump inhibition. Front. Microbiol. 6:421. doi: 10.3389/fmicb.2015.00421
Pankey, G. A., and Sabath, L. D. (2004). Clinical relevance of bacteriostatic versus bactericidal mechanisms of action in the treatment of Gram-positive bacterial infections. Clin. Infect. Dis. 38, 864–870. doi: 10.1086/381972
Pitman, S. K., Drew, R. H., and Perfect, J. R. (2011). Addressing current medical needs in invasive fungal infection prevention and treatment with new antifungal agents, strategies and formulations. Expert. Opin. Emerg. Drugs. 16, 559–586. doi: 10.1517/14728214.2011.607811
Robertson, E. H., Cartwright, R. A., and Wood, D. J. M. (1957). The flavones of tea. J. Sci. Food. Agr. 7, 637–646. doi: 10.1002/jsfa.2740071003
Russell, C. R., and Morris, D. A. (1982). Invertase activity, soluble carbohydrates and inflorescence development in the tomato (Lycopersicon esculentum Mill). Ann. Bot. 49, 89–98.
Simonetti, G., Santamaria, A. R., D'Auria, F. D., Mulinacci, N., Innocenti, M., Cecchini, F., et al. (2014). Evaluation of Anti-Candida activity of Vitis vinifera L. seed extracts obtained from wine and table cultivars. BioMed. Res. Int. 2014, 2314–6141. doi: 10.1155/2014/127021
Stryjewski, M. E., and Corey, G. R. (2014). Methicillin-resistant Staphylococcus aureus: an evolving pathogen. Clin. Infect. Dis. 58, S10–S19. doi: 10.1093/cid/cit613
Sudhakar, M., Rao, C. V., Rao, P. M., and Raju, D. B. (2006). Evaluation of antimicrobial activity of Cleome viscosa and Gmelina asiatica. Fitoterap. 77, 47–49. doi: 10.1016/j.fitote.2005.08.003
Taguri, T., Tanaka, T., and Kouno, I. (2006). Antibacterial spectrum of plant polyphenols and extracts depending upon hydroxyphenyl structure. Biol. Pharm. Bull. 29, 2226–2235. doi: 10.1248/bpb.29.2226
Tegos, G., Stermitz, F. R., Lomovskaya, O., and Lewis, K. (2002). Multidrug pump inhibitors uncover remarkable activity of plant antimicrobials. Antimicrob Agents Chemother. 46, 3133–3141. doi: 10.1128/AAC.46.10.3133-3141.2002
Tian, F., Li, B., Ji, B., Zhang, G., and Luo, Y. (2009). Identification and structure–activity Relationship of gallotannins separated from Galla chinensis. LWT-Food Sci. Technol. 42, 1289–1295. doi: 10.1016/j.lwt.2009.03.004
Venter, H., Mowla, R., Ohene-Agyei, T., and Ma, S. (2015). RND-type drug eux pumps from Gram-negative bacteria: molecular mechanism and inhibition. Front. Microbiol. 28:377. doi: 10.3389/fmicb.2015.00377
Vuuren, S., and Viljoen, A. (2011). Plant-based antimicrobial studies – methods and approaches to study the interaction between natural products. Plant Med. 77, 1168–1182. doi: 10.1055/s-0030-1250736
Wagner, H., and Bladt, S. (1996). Plant Drug Analysis: A Thin Layer Chromatography Atlas. Berlin: Springer Science Business Media.
Wagner, H., and Ulrich-Merzenich, G. (2009). Synergy research: approaching a new generation of phytopharmaceuticals. Phytomedicine 16, 97–110. doi: 10.1016/j.phymed.2008.12.018
Keywords: plant-derived products, drug discovery, antibacterial agents, synergistic action, S. aureus
Citation: Silva APS, Nascimento da Silva LC, Martins da Fonseca CS, Araújo JM, Correia MTS, Cavalcanti MS and Lima VLM (2016) Antimicrobial Activity and Phytochemical Analysis of Organic Extracts from Cleome spinosa Jaqc. Front. Microbiol. 7:963. doi: 10.3389/fmicb.2016.00963
Received: 22 November 2015; Accepted: 03 June 2016;
Published: 28 June 2016.
Edited by:
Yuji Morita, Aichi Gakuin University, JapanReviewed by:
Francesco Imperi, Sapienza University of Rome, ItalyHenrietta Venter, University of South Australia, Australia
Nathalie Tufenkji, McGill University, Canada
Copyright © 2016 Silva, Nascimento da Silva, Martins da Fonseca, Araújo, Correia, Cavalcanti, and Lima. This is an open-access article distributed under the terms of the Creative Commons Attribution License (CC BY). The use, distribution or reproduction in other forums is permitted, provided the original author(s) or licensor are credited and that the original publication in this journal is cited, in accordance with accepted academic practice. No use, distribution or reproduction is permitted which does not comply with these terms.
*Correspondence: Vera L. de Menezes Lima, bGltYS52ZXJhLnVmcGVAZ21haWwuY29t